- 1Key Laboratory of Plant Protection Resources and Pest Management of Ministry of Education, Entomological Museum, College of Plant Protection, Northwest A&F University, Xianyang, Shaanxi, China
- 2Illinois Natural History Survey, Prairie Research Institute, University of Illinois, Champaign, IL, United States
Tribes of the leafhopper subfamily Typhlocybinae have traditionally been defined based on differences in hind wing venation, but the forewing venation also differs among some tribes. Here we used geometric morphometric analysis to determine whether previously recognized tribes can be distinguished based on the configuration of forewing veins. Focusing on the apical area of the male right forewing, 76 semi-landmarks in six curves corresponding to individual wing veins were measured for representatives of four previously recognized tribes and the data were analyzed using principal component analysis (PCA), canonical variable analysis (CVA), and UPGMA clustering analysis. The study showed that differences in the apical area of the forewing mainly occur in RP, MP′, and MP″ + CuA′. PCA, CVA, and cluster analysis showed three distinct clusters representing tribes Empoascini, Erythroneurini, and Typhlocybini (sensu lato) but failed to distinguish Typhlocybini (sensu stricto) from Zyginellini, which has been considered as either separate tribe or a synonym of Typhlocybini by recent authors. The results show that the forewing venation differs among tribes of Typhlocybinae, but also agree with recent molecular phylogenetic analyses, indicating that Zyginellini is derived from within Typhlocybini.
Introduction
The leafhopper family Cicadellidae is one of the 10 largest insect families, and is widely distributed in across all zoogeographical regions (Balme, 2007). Typhlocybinae is the second largest subfamily in this family (after Deltocephalinae), comprising about 6,000 described species (Lu et al., 2021). Typhlocybinae includes many important agricultural pests, which injure plants by sucking the sap and spreading plant diseases (Zhou et al., 2020).
The taxonomic classification of Typhlocybinae was long controversial with various scholars proposing alternative arrangements of genera into tribes based on study of different regional faunas and morphological characters (Distant, 1908; Matsumura, 1931; McAtee, 1934; Oman, 1949; Young, 1952; Mahmood, 1967; Mahmood and Ahmed, 1968; Ahmed, 1983; Ruppel, 1987).
Most recent authors follow the classification of Dworakowska (1979), who proposed the recognition of six tribes, i.e., Alebrini, Dikraneurini, Empoascini, Erythroneurini, Typhlocybini, and Zyginellini, mainly according to the venation of forewing and hind wing. In this classification, Zyginellini are distinguished from Typhlocybini by the hind wing with one cross vein. Some authors have suggested that Zyginellini is an artificial group and should be considered a synonym of Typhlocybini (Ahmed, 1983; Balme, 2007; Dietrich, 2013). Recent molecular phylogenetic analyses of Typhlocybinae have either recovered Zyginellini as a monophyletic sister group of Typhlocybini (Lu et al., 2021) or as a polyphyletic group comprising different lineages derived from within Typhlocybini (Yan et al., 2022). Therefore, additional analyses are needed to clarify the status of Zyginellini.
To explore the relationship of Typhlocybini and Zyginellini based on their wing venation, here we analyzed the configuration of apical forewing veins using geometric morphometrics.
The forewing of Typhlocybinae may be divided into three regions: clavus, basal part of corium and apical part of corium. The arrangement of veins in the basal region of the forewing is relatively simple, with only the three longitudinal veins, i.e., R, MP, and CuA, and varies little among tribes (Figure 1A). The apical region is delimited by transverse veins and has three longitudinal veins, RP, MP′, and MP″ + CuA′, divided into four apical cells of variable shape. From the hind margin to costal margin, these cells are numbered from 1 to 4 (Figure 1A). The branching of the longitudinal veins, their relative length, and the relative size of each apical cell are generally stable within a genus but vary among genera and, therefore, have been useful in taxonomy (Zhan, 2014).
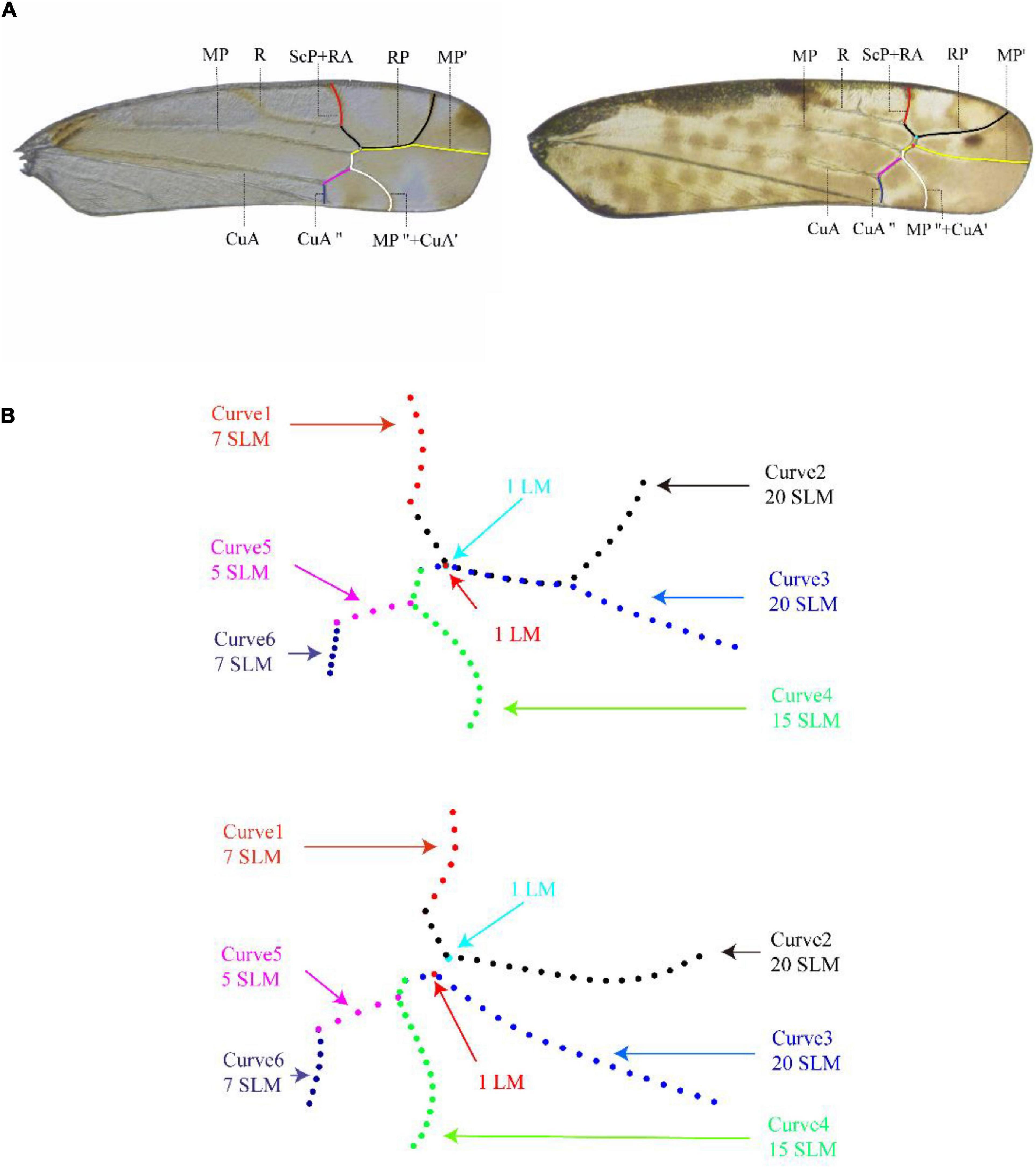
Figure 1. Description of the curves on the apical area of forewing of Typhlocybinae used in the geometric morphometric analysis [right forewing of Aguriahana triangularis Matsumura, 1932 (left picture) and Parathailocyba orla Dworakowska, 1977 (right picture)]. (A) The apical area of forewing venation described by the six curves in red, black, yellow, white, pink, and mazarine blue are ScP + RA, RP, MP′, MP″ + CuA′, CuA′, and CuA″, respectively (main venation of wings). (B) Curves 1 and 6 are 7 equally spaced semi-landmarks, curves 2, and 3 are 20 equally spaced semi-landmarks, curve 4 are 15 equally spaced semi-landmarks, and curve 5 are 5 equally spaced semi-landmarks.
Geometric morphometric methods allow quantitative comparison of morphological structures (Bai et al., 2014) by using statistical analyses of measurement data to reveal patterns of similarity and difference in shape. Most available methods use variation in the relative positions of morphological landmarks thought to be homologous in order to describe shape differences quantitatively and describe transformations in shape that may reflect evolutionary change (Zelditch et al., 2004).
Here we used landmark-based measurements of the forewing apex of Typhlocybinae to characterize shape differences in venation species and among genera and assess the degree of congruence between wing characteristics and taxonomic classification with special reference to the tribes Typhlocybini and Zyginellini.
Materials and methods
Taxa examined
The study analyzed 241 species representing 42 genera (344 samples) of Typhlocybini and 85 species of 28 genera (100 samples) of Zyginellini (Table 1). Since the number of transverse veins on hindwing is an important taxonomic feature, we divided Typhlocybini into two groups which one with two transverse veins and other one with three transverse veins on hindwing. There were 157 species of 33 genera (234 samples) of the Typhlocybini with two transverse veins on the hindwing, and 84 species of 9 genera (110 samples) of Typhlocybini with three transverse veins on the hindwing. Erythroneurini and Empoascini were selected as outgroups based on the results of recent phylogenetic analyses (Lu et al., 2021; Yan et al., 2022) with 2 genera, 3 species, and 17 samples from Erythroneurini and 2 genera, 2 species, and 10 samples from Empoascini included.
Image acquisition and pre-processing
In order to reduce the error, we uniformly selected the male right forewing. All study images were collected from the entomological museum of Northwest A&F University (NWAFU, China) and literatures. The right forewing of the specimen of each species was gently pried off with a dissecting needle. Then, the wing was put into a glass dish filled with 75% alcohol and cleaned with a brush. The cleaned wing was put on the cover slip by a pipette, before the alcohol drying, covered with another cover slip, then it was put in a carton and sealed with white glue. The sealed cover slips were left for 3 h to ensure that there was no alcohol between cover slips before the photo was taken. Finally, the advanced stereoscopic microscope system (Zeiss, Oberkochen, Germany) was used to take the pictures of forewings to obtain pictures. Slide-mounted forewings were then photographed with images (TIFF files) saved at 96 dpi. To facilitate accurate representation, images from literature were only used if the forewing were not covered or blurry and the images possessed adequate resolution (the smallest one was 96 pixels). For the convenience of analysis, the pictures were numbered that A represents Typhlocybini with two transverse veins on the hindwing, B represents Typhlocybini with three transverse veins on the hindwing, C represents Zyginellini, D represents Erythroneurini, and E represents Empoascini.
All images acquired were input into tps-UTIL (Rohlf, 2006b), and the shapes of branches of apical forewing veins were digitized with tps-DIG (Rohlf, 2006a). Six curves were extracted from apical area of forewing as shown in Figure 1A. Curve 1 is vein ScP + RA, curve 2 is vein RP, curve 3 is vein MP′, curve 4 is vein MP″ and MP″ + CuA′, curve 5 is vein CuA″, and curve 6 is vein CuA″. Curves 1 and 6 are divided into 7 equally spaced semi-landmarks, curves 2 and 3 are divided into 20 equally spaced semi-landmarks, curve 4 is divided into 15 equally spaced semi-landmarks, and curve 5 is divided into 5 equally spaced semi-landmarks (Figure 1B). We used the method of Bardua et al. (2019) to solve the problem of absence of certain transverse veins in some genera: in cases where the transverse vein is present, we marked both ends of the veins; where the transverse vein is absent, we marked two points at the point of fusion of the veins (respectively: light blue dots and red dots) that only for preserves the location of the crossvein (Figure 1).
Because some analytical software cannot use semi-landmark data, so data captured for semi-landmark points were converted to landmarks using a text editor (MacLeod, 2017). The specific operations performed are as follows: deleting the curve and the number of points, replacing points with landmark, modifying the ID, and saving the information in a folder for subsequent analysis (Tong et al., 2021).
The wing venation terminology follows Dworakowska (1993).
Morphometric analyses
The landmark data were imported into tps-SMALL (Rohlf, 2003), and the distribution of points in tangent space was compared with that of shape space (Rohlf, 1999; Slice, 2001). Landmark coordinates were aligned using the Procrustes superposition method in MorphoJ 1.04 software (Klingenberg, 2011). Procrustes superposition is used to rotate, translate, and scale configurations, remove information other than shape, and superimpose objects in a common coordinate system (Rohlf, 1999; Slice, 2001; Adams et al., 2004).
Principal component analysis (PCA), canonical variable analysis (CVA), and cluster analysis were used. Among them, PCA and CVA were analyzed in MorphoJ (Klingenberg, 2011), and cluster analysis was performed in PAST 4.04 (Hammer et al., 2001). A spatial geometric model was created using PCA and the PC axes were used to explain the shape change of the veins of the forewing apex of individuals (Shi and Macleod, 2016; Tong et al., 2021). The purpose of CVA is to simplify the description of differences among groups. Cluster analysis was performed by first calculating the average landmark configuration using tps-SUPER (Rohlf, 2004) and then importing these data into tps-SMALL to calculate the Procrustes distances. Procrustes distance were imported into PAST software, and cluster analysis was performed using distance matrices using the unweighted pair-group method with arithmetic means (UPGMA) to determine phenotypic similarity among species (Rohlf, 2002; Cardini and O’higgins, 2004; Bai et al., 2011). Procrustes distance has been considered the best way to measure shape differences between taxa (Pretorius and Scholtz, 2001; Bai et al., 2011).
Results
Procrustes distance analysis in tps-SAMLL showed that there is a strong correlation between tangent space and shape space, with a correlation between tangent space (Y) regression and Procrustes distance of 0.999952.
Based on the landmark information, a PCA was performed on measurements taken from the apical area of the forewing. A total of 148 principal components were obtained, of which the first two (PC1 and 2) accounted for 36.171 and 15.319% of the total variation, respectively. As shown in Figure 2, a large number of individuals Typhlocybini and Zyginellini strongly overlap on both PC1 and 2 while individuals of Erythroneurini and Empoascini are well separated from each other and distributed on the right side of 0.2 the positive direction of PC1. This largely reflects the greater elongation of the inner apical cell (particularly vein MP″ + CuA′) in the latter two tribes. The distortion graph (Figure 2) showed that variation on PC1 is explained by differences in the relative length of Curves 2 and 4 while variation along PC2 mostly reflects the degree of separation of Curves 2 and 3. As a result of these changes, the 1st and 3rd apical cells are either decreasing or increasing in size, while the 2nd and 4th apical cell are either expanding or contracting. This result showed no obvious differentiation between Typhlocybini and Zyginellini in venational configuration.
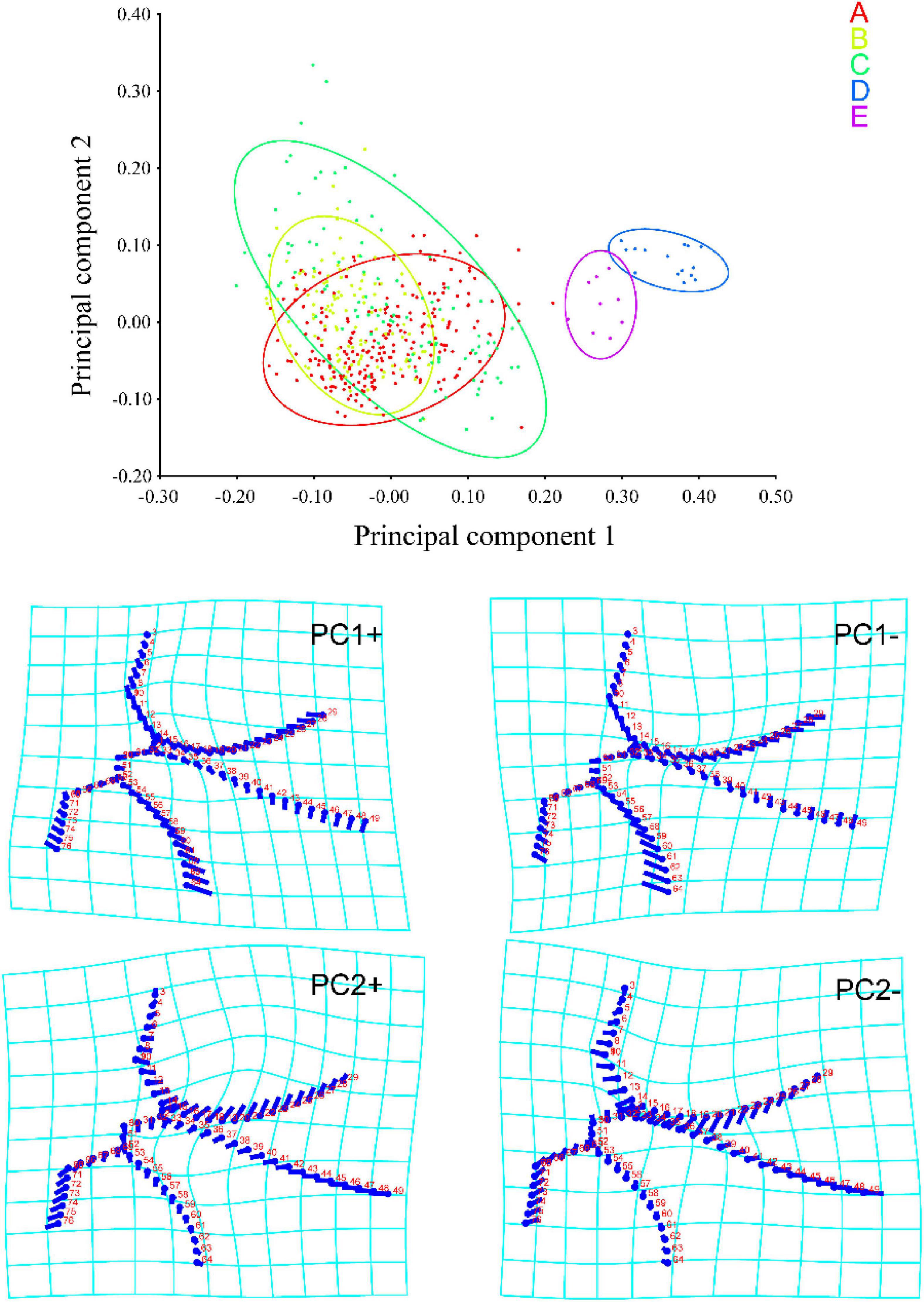
Figure 2. Principal component analysis (PCA) scores and shape changes of the four tribes of Typhlocybinae: A, Typhlocybini (2 hind wing crossveins); B, Typhlocybini (3 hind wing crossveins); C, Zyginellini; D, Erythroneurini, E, Empoascini.
A total of 15 principal components were obtained in CVA, of which the first two (CV1 and 2) accounted for 59.890 and 19.173% of the total variation, respectively. As shown in Figure 3, Typhlocybini and Zyginellini strongly overlap on both CV1 and 2 while Erythroneurini and Empoascini are well separated from each other and distributed the negative direction of CV1. Combined with the distortion graph (Figure 3), it can be seen that the results of CVA performance are consistent with PCA. This result also shows no obvious differentiation between Typhlocybini and Zyginellini in venational configuration.
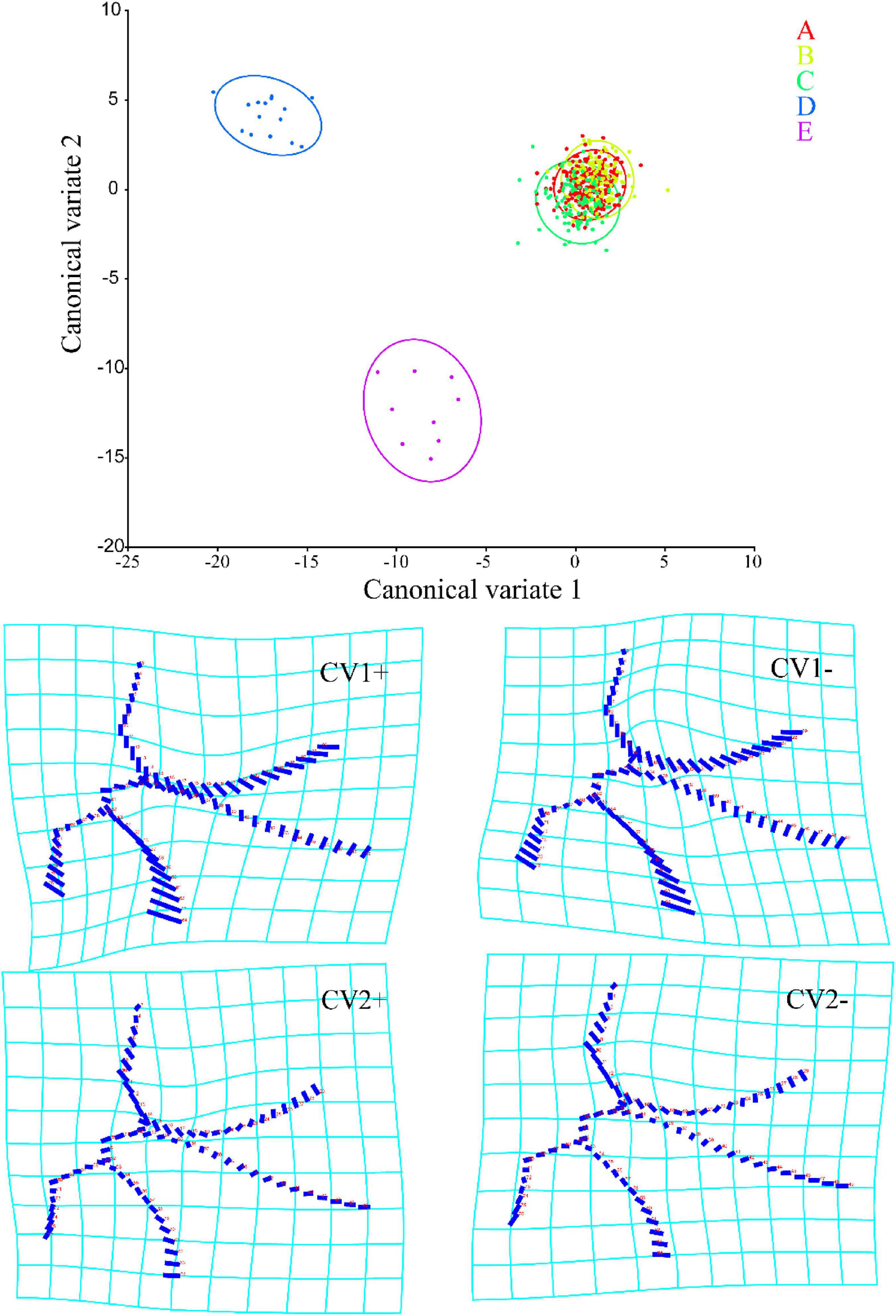
Figure 3. Canonical variable analysis (CVA) scores and shape changes of the four tribes of Typhlocybinae: A, Typhlocybini (2 hind wing crossveins); B, Typhlocybini (3 hind wing crossveins); C, Zyginellini; D, Erythroneurini, E, Empoascini.
Unweighted pair-group method with arithmetic means cluster analysis based on the average landmark configurations yielded the phenogram in Figure 4. The results showed that when the distance is around 2.0, the four tribes are divided into two main clusters, one comprising Erythroneurini and Empoascini, and the other comprising Typhlocybini and Zyginellini. When the distance is about 0.8, Erythroneurini and Empoascini are separated. The genera of Erythroneurini and the genera of Empoascini separated, the distance is about 0.5 and 0.4, respectively. But Typhlocybini and Zyginellini are mixed, with members of the different tribes scattered among multiple clusters. The genera of Typhlocybini and the genera of Zyginellini first branched, the Procrustes distance is about 1.0. Based on the phenogram and the results from PCA and CVA, Zyginellini cannot be separated from Typhlocybini based on the forewing venation.
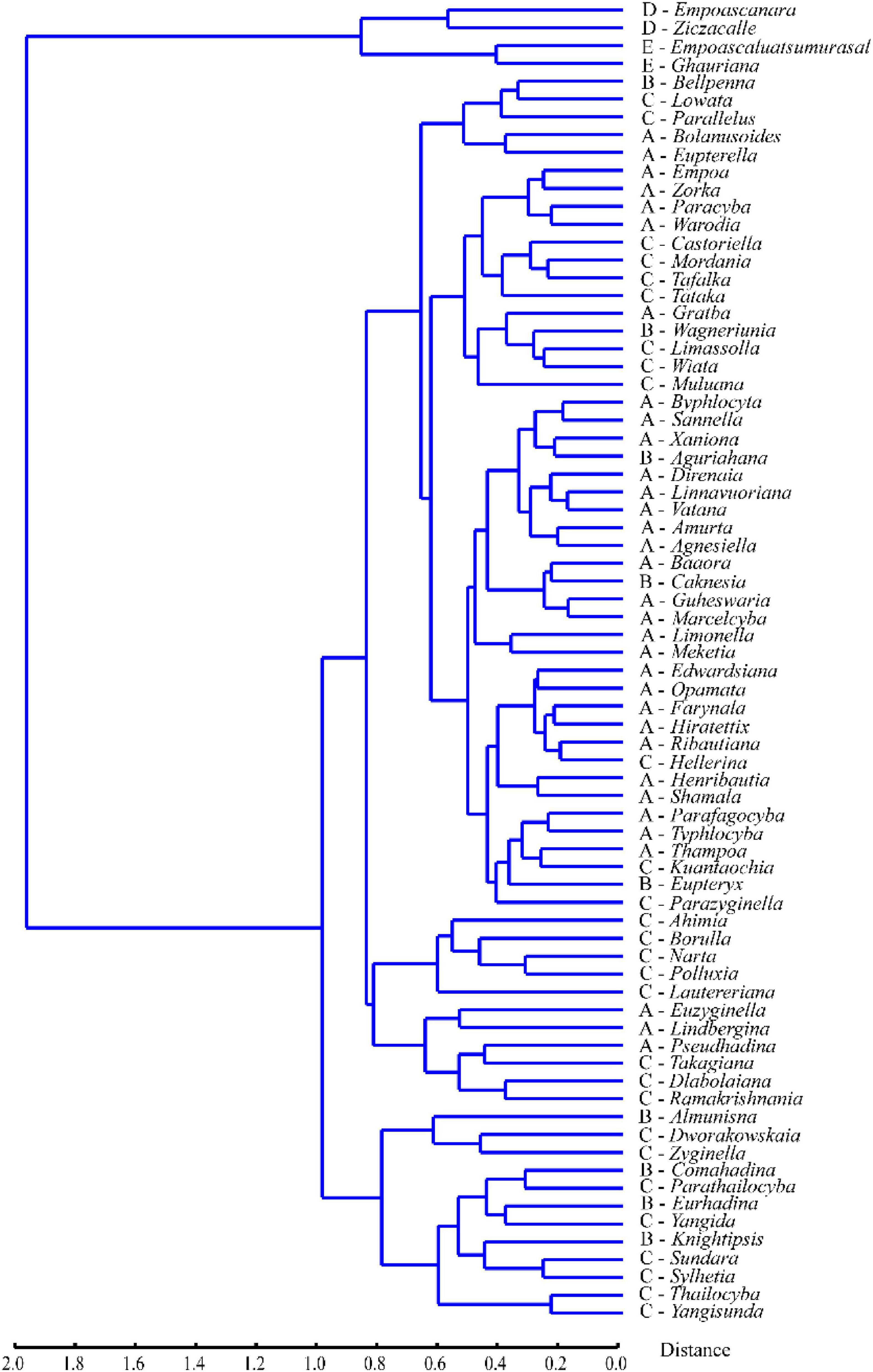
Figure 4. Phenogram of the four tribes of Typhlocybinae based on unweighted pair-group method with arithmetic means (UPGMA) clustering of Procrustes distances.
Discussion and conclusion
The classification status of Zyginellini
On the phenogram (Figure 4), Typhlocybini and Zyginellini group together on a single large branch and genera of Zyginellini are scattered among members of Typhlocybini, in agreement with a recent molecular phylogenetic analysis (Yan et al., 2022) showing that different genera of Zyginellini are derived from independent branches within Typhlocybini. Although these tribes may be separated by the hind wing venation (Dworakowska, 1979) and another recent molecular phylogenetic study (Lu et al., 2021) provided some support for the monophyly of both tribes, some Neotropical species of Typhlocybini may have Typhlocybini-like venation on one hind wing and Zyginellini-like venation on the other hind wing of the same individual (Dietrich, 2013), suggesting that this character is unstable. Balme (2007) and Dietrich (2013) previously proposed Zyginellini as a junior synonym of Typhlocybini based on morphological and molecular data. Our morphometric analyses of the apical area of the forewing of 326 species representing 70 genera previously placed in Typhlocybini and Zyginellini also support treating these two tribes as synonyms.
Taxonomic significance of forewing venation in Typhlocybinae
The forewing venation of Typhlocybinae is simplified compared to that of other leafhopper groups. It has only three primary veins on the basal area and four cells on the apical area, with the appendix present only in the tribe Alebrini. The patterns of venation in this group have rarely been traditionally used to characterize tribes.
Recent molecular phylogenetic analyses (Lu et al., 2021; Yan et al., 2022) suggested that some venational characters used for taxonomy are homoplastic. Nevertheless, Bourgoin et al. (2015) argued that such characters can be useful if they are carefully analyzed in terms of homology. Variation in the forewing apical area of Typhlocybinae including the shapes of apical cells and branching sequence of veins includes a large amount of geometric information that represent useful taxonomic characters.
In present study, we used morphometric analysis to determine whether tribes of Typhlocybini can be differentiated based on the forewing apical area. Dworakowska (personal communication) previously divided genera of the Typhlocybini into several generic complexes according to the characters of wings and genitalia; Huang and Zhang (2006) reconstructed the genetic level phylogenetic relationship by the morphological characteristics and the results confirmed the validity of most of these informal complexes, including the Linnavuoriana complex which with body outline fusiformis, male subgenital plate without macroseta that are autamorphic characters of this complex. In this study, members of the Linnavuoriana complex, i.e., Vatana, Linnavuoriana, Amurta, and Agnesiella, clustered together (Figure 4), supporting previous studies.
On the other hand, in the traditional classification of Typhlocybinae, Eupterygini, distinguished by some previous authors from Typhlocybini (sensu stricto) according to the hind wing with three transverse veins (McAtee, 1934) accepted in a short duration, and Zyginellini, was proposed according to the hind wing with a transverse vein (Dworakowska, 1979), but both of these two tribes were treated as junior synonyms of Typhlocybini by later authors (Balme, 2007; Dietrich, 2013) and did not form distinct clusters (Figure 4, denoted by “B” and “C,” respectively) in present study, in agreement with recent molecular phylogenetic results (Lu et al., 2021; Yan et al., 2022). It also indicates that compared with the number of transverse veins on the hind wing, the venation on the forewing is more stable.
Moreover, the results of differentiating tribes based on the character of forewing apical veins in present study also support the result by the male genitalia. As Figures 2–4 showed, outgroups of tribes Erythroneurini and Empoascini are distinctly separated from the ingroups, excepting the distinct differences on the venation, they have numerous macrosetae on the pygofor or subgenital plate, developed anal process and simple aedeagus, while to Typhlocybini and Zyginellini, they share these characters that have less macrosetae just on subgenital plate or even absent, seldom with anal process, aedeagus usually with developed and complex process. Excepting the number of transverse veins on the hind wing, we hardly differentiate Typhlocybini and Zyginellini.
Therefore, we believe that the characteristics of forewing apical veins are reliable and they should be considered in the further studies on the phylogenetic analysis and the evolution of Typhlocibinae.
The application of morphometrics to classification
Geometric morphometrics has been widely used in insect classification, and wing shape and venation are the most frequently selected features (Yan, 2011; Janczyk et al., 2020). Such analyses have also been used for phylogenetic reconstruction (e.g., Zhan, 2014) but they have not previously been used for distinguishing groups of typhlocybine leafhoppers. Although the tribal classification of Typhlocybinae has traditionally been based mostly on characters of the hind wing venation, the forewing also offers useful characters for recognizing tribes, as shown by our results. In our analysis a total of 76 semi-landmarks points were selected to represent geometric shape information of the forewing tip region. The results of our study confirmed that tribes Erythroneurini and Empoascini are significantly different from each other and from Typhlocybini in the configuration of forewing veins but that two groups of Typhlocybini traditionally considered to be separate tribes (Eupterygini and Zyginellini) cannot be consistently differentiated based on forewing venation.
Data availability statement
The raw data supporting the conclusions of this article will be made available by the authors, without undue reservation.
Author contributions
HW: conceptualization, data curation, writing—original draft, methodology, and software. XZ: methodology and resources. CD: validation and supervision. MH: conceptualization, methodology, and supervision. All authors contributed to the article and approved the submitted version.
Funding
This research was funded by the National Natural Science Foundation of China (32070478 and 31372233) and The Ministry of Science and Technology of the People’s Republic of China (2015FY210300).
Acknowledgments
We are very grateful to Dr. I. Dworakowska for her contribution to the knowledge of Chinese Typhlocybinae. Deep gratitude to the reviewers for their helpful suggestions. Sincere thanks to students in the Entomological Museum of Northwest A&F University for their great help in obtaining specimens: Juan Zhou and Junjie Wang. We are also grateful to Juncai Li for his kind advice in analyses.
Conflict of interest
The authors declare that the research was conducted in the absence of any commercial or financial relationships that could be construed as a potential conflict of interest.
Publisher’s note
All claims expressed in this article are solely those of the authors and do not necessarily represent those of their affiliated organizations, or those of the publisher, the editors and the reviewers. Any product that may be evaluated in this article, or claim that may be made by its manufacturer, is not guaranteed or endorsed by the publisher.
References
Adams, D. C., Rohlf, F. J., and Slice, D. E. (2004). Geometric morphometries: Ten years of progress following the ‘revolution’. Ital. J. Zool. 71, 5–16. doi: 10.1080/11250000409356545
Ahmed, M. (1983). “Biotaxonomy of typhlocybine leafhoppers of Pakistan,” in Proceedings of the 1st international workshop on biotaxonomy, classification and biology of Leafhoppers and Planthoppers (Auchenorrhyncha) of economic importance, eds W. J. Knight, N. C. Pant, T. S. Robertson, and M. R. Wilson (London: Commonwealth Institute of Entomology), 179–183.
Bai, M., McCullough, E., Song, K. Q., Liu, W. G., and Yang, X. K. (2011). Evolutionary constraints in hind wing shape in Chinese dung beetles (Coleoptera: Scarabaeinae). PLoS One 6:e21600. doi: 10.1371/journal.pone.0021600
Bai, M., Yang, X. K., Li, J., and Wang, W. C. (2014). Geometric morphology: A scientific computing tool for quantitative comparison of morphology. Sci. Bull. (Beijing) 59, 887–894. doi: 10.1360/972012-1561
Balme, G. R. (2007). Phylogeny and systematics of the leafhopper subfamily Typhlocybinae (Insecta: Hemiptera: Typhlocybinae). Ph.D. thesis. Raleigh, NC: North Carolina State University.
Bardua, C., Felice, R. N., Watanabe, A., Fabre, A. C., and Goswami, A. (2019). A practical guide to sliding and surface semilandmarks in morphometric analyses. Integr. Org. Biol. 1:obz016. doi: 10.1093/iob/obz016
Bourgoin, T., Wang, R. R., Asche, M., Hoch, H., Soulier-Perkins, A., Stroiński, A., et al. (2015). From micropterism to hyperpterism: Recognition strategy and standardized homology-driven terminology of the forewing venation patterns in Planthoppers (Hemiptera: Fulgoromorpha). Zoomorphology 34, 63–77. doi: 10.1007/s00435-014-0243-6
Cardini, A., and O’higgins, P. (2004). Patterns of morphological evolution in Marmota (Rodentia, Sciuridae): Geometric morphometrics of the cranium in the context of marmot phylogeny, ecology and conservation. Biol. J. Linn. Soc. Lond. 82, 385–407. doi: 10.1111/j.1095-8312.2004.00367.x
Dietrich, C. H. (2013). South American leafhoppers of the tribe Typhlocybini (Hemiptera: Cicadellidae: Typhlocybinae). Zoologia 30, 519–568. doi: 10.1590/S1984-46702013000500008
Distant, W. L. (1908). Rhynchota. IV. Homoptera and appendix (Pt.). The fauna of British India, including Ceylon and Burma. London: Taylor & Francis.
Dworakowska, I. (1977). On some typhlocybinae from vietnam (Homoptera: Cicadellidae). Folia Entomol. Hung. 30, 9–47.
Dworakowska, I. (1979). On the leafhopper tribe Zyginellini (Homoptera: Auchenorrhyncha, Cicadellidae, Typhlocybinae). Rev. Zool. Afr. 93, 288–331.
Dworakowska, I. (1993). Remarks on Alebra Fieb and Eastern Hemisphere Alebrini (Auchenorrhyncha: Cicadellidae: Typhlocybinae). Entomotaxonomia 15, 91–121.
Hammer, Y., Harper, D. A., and Ryan, P. D. (2001). PAST: Paleontological statistics software package for education and data analysis. Palaeontol. Electron. 4:9.
Huang, M., and Zhang, Y. L. (2006). Systematic study of the oriental genus Vatana dworakowska (Hemiptera: Cicadellidae: Typhlocybinae: Typhlocybini). Proc. Entomol. Soc. Wash. 108, 945–952.
Janczyk, A., Meixner, M. D., and Tofilski, A. (2020). Morphometric identification of the endemic Maltese honey bee (Apis mellifera ruttneri). J. Apic. Res. 60, 157–164. doi: 10.1080/00218839.2020.1827705
Klingenberg, C. P. (2011). MorphoJ: An integrated software package for geometric morphometrics. Mol. Ecol. Resour. 11, 353–357. doi: 10.1111/j.1755-0998.2010.02924.x
Lu, L., Dietrich, C. H., Cao, Y. H., and Zhang, Y. L. (2021). A multi-gene phylogenetic analysis of the leafhopper subfamily Typhlocybinae (Hemiptera: Cicadellidae) challenges the traditional view of the evolution of wing venation. Mol. Phylogenet. Evol. 165:107299. doi: 10.1016/j.ympev.2021.107299
MacLeod, N. (2017). Morphometrics: History, development methods and prospects. Zool. Syst. 42, 4–33. doi: 10.11865/zs.201702
Mahmood, S. H. (1967). A study of the typhlocybine genera of the Oriental region (Thailand, the Philippines and adjoining areas). Pac. Insects Monogr. 12, 1–55.
Mahmood, S. H., and Ahmed, M. (1968). Problems of higher classification of Typhlocybinae (Cicadellidae: Homoptera). Univ. Stud. Univ. Karachi 5, 72–79. doi: 10.3897/zookeys.1037.63671
Matsumura, S. (1931). A revision of the palaearctic and Oriental Typhlocybid genera, with descriptions of new species and new genera. Insecta Matsumurana 6, 55–91.
McAtee, W. L. (1934). Genera and subgenera of Eupteryginae (Homoptera; Jassidae). Proc. Zool. Soc. Lond. 104, 93–117. doi: 10.1111/j.1469-7998.1934.tb06225.x
Oman, P. M. (1949). The nearctic leafhoppers (Homoptera: Cicadellidae), a generic classification and check list. Mem. Entomol. Soc. Wash. 3, 1–253.
Pretorius, E., and Scholtz, C. H. (2001). Geometric morphometries and the analysis of higher taxa: A case study based on the metendosternite of the Scarabaeoidea (Coleoptera). Biol. J. Linn. Soc. Lond. 74, 35–50. doi: 10.1006/bijl.2001.0568
Rohlf, F. J. (1999). Shape statistics: Procrustes superimpositions and tangent spaces. J. Classificat. 16, 197–223. doi: 10.1007/s003579900054
Rohlf, F. J. (2002). “Geometric morphometrics and phylogeny,” in Morphology, shape and phylogenetics, eds N. MacLeod and P. L. Forey (London: Taylor & Francis), 175–193. doi: 10.1201/9780203165171.ch9
Rohlf, F. J. (2003). tps-SMALL. Version 1.20 [software and manual]. Stony Brook, NY: Department of Ecology and Evolution, State Universityof New York at Stony Brook, New York.
Rohlf, F. J. (2004). tps-SUPER. Version 1.14 [software and manual]. New York, NY: Department of Ecology and Evolution, State University of New York at Stony Brook.
Rohlf, F. J. (2006a). tps-DIG, digitize landmarks and outlines, version 2.05 [software and manual]. Stony Brook, NY: Department of Ecology and Evolution, State University of New York at Stony Brook.
Rohlf, F. J. (2006b). tps-UTIL, file utility program, version1.38 [software and manual]. Stony Brook, NY: Department of Ecology and Evolution, State University of New York at Stony Brook.
Ruppel, R. F. (1987). A summary of the tribes proposed in Typhlocybinae (Hemiptera, Cicadellidae). Mich. Acad. 19, 29–35.
Shi, Y., and Macleod, N. (2016). Identification of life-history stages in fusulinid foraminifera. Mar. Micropaleontol. 122, 87–98. doi: 10.1016/j.marmicro.2015.12.002
Slice, D. E. (2001). Landmark coordinates aligned by Procrustes analysis do not lie in Kendall’s shape space. Syst. Biol. 50, 141–149. doi: 10.1080/10635150119110
Tong, Y. J., Yang, H. D., Jenkins Shaw, J., Yang, X. K., and Bai, M. (2021). The Relationship between Genus/Species Richness and Morphological Diversity among Subfamilies of Jewel Beetles. Insects 12:24. doi: 10.3390/insects12010024
Yan, B., Dietrich, C. H., Yu, X., Jiao, M., Dai, R., and Yang, M. (2022). Mitogenomic phylogeny of Typhlocybinae (Hemiptera: Cicadellidae) reveals homoplasy in tribal diagnostic morphological traits. Ecol. Evol. 12:e8982. doi: 10.1002/ece3.8982
Yan, B. R. (2011). A study on the phylogeny of panorpidae based on geometric morphometrics. Master’s thesis. Xianyang: Northwest A&F University.
Young, D. A. (1952). A reclassification of western hemisphere typhlocybinae (Homoptera, Cicadellidae). Univ. Kans. Sci. Bull. 35, 3–217. doi: 10.5962/bhl.part.4327
Zelditch, M. L., Swiderski, D. L., Sheets, D. H., and Fink, W. L. (2004). Geometric morphometrics for biologists: A primer. London: Elsevier Academic Press.
Zhan, Q. B. (2014). Study on the geometric morphology of the wings of Laceyidae in China and the revision of the classification of the four genera. Ph.D. thesis. Beijing: China Agricultural University.
Keywords: Hemiptera, Cicadellidae, Typhlocybini, Zyginellini, geometric morphology, apical area of forewing
Citation: Wen H, Zhou X, Dietrich CH and Huang M (2022) Morphometric analysis of forewing venation does not consistently differentiate the leafhopper tribes Typhlocybini and Zyginellini. Front. Ecol. Evol. 10:1003817. doi: 10.3389/fevo.2022.1003817
Received: 26 July 2022; Accepted: 05 September 2022;
Published: 30 September 2022.
Edited by:
Yuyu Wang, Agricultural University of Hebei, ChinaReviewed by:
Yongjie Wang, Guangdong Academy of Sciences, ChinaYuehua Song, Guizhou Normal University, China
Copyright © 2022 Wen, Zhou, Dietrich and Huang. This is an open-access article distributed under the terms of the Creative Commons Attribution License (CC BY). The use, distribution or reproduction in other forums is permitted, provided the original author(s) and the copyright owner(s) are credited and that the original publication in this journal is cited, in accordance with accepted academic practice. No use, distribution or reproduction is permitted which does not comply with these terms.
*Correspondence: Min Huang, aHVhbmdtaW5AbndzdWFmLmVkdS5jbg==
†These authors share first authorship