- 1Agricultural Science and Technology Research Institute, Andong National University, Andong, GB, South Korea
- 2Department of Plant Medicals, Andong National University, Andong, GB, South Korea
Dietary nutrients provide fuel for the growth and development of insects as well as chemicals for their tissue construction. Apis mellifera L., an important pollinator, collects nectar and pollens from different plants to get their nutritional needs. Honey bees use protein for growth and development and carbohydrates as energy sources. Pollens predominantly contain proline and glutamic acid (non-essential amino acids). This is the first study to evaluate the role of proline, glutamic acid and sorbitol on bee broods. The composition of the diet can optimize the in vitro rearing process. Therefore, we elaborated on the possible impact of these amino acids and sugar alcohol on bee broods. This study aimed to achieve this objective by rearing honey bee larvae under different concentrations of proline, glutamic acid, and sorbitol (1, 4 and 8%), which were supplemented into the standard larval diet. The supplementation of proline helped the quick development of larvae and pupae of honey bees, whereas developmental time only decreased in pupae in the case of glutamic acid. The duration of the total bee brood development was the shortest (20.1 and 20.6 days) on Pro8 and Glu4, respectively. Proline only increased larvae survival (93.8%), whereas glutamic acid did not increase the survival of any brood stage. Pupal and adult weights were also increased with proline and glutamic acid-supplemented diets. Sorbitol did not change the developmental period of the honey bee brood but increased larval survival (93.7%) only at the lowest concentration (Sor1). The small concentration of sorbitol can be used to increase the survival of the honey bee brood. However, a higher concentration (Sor8) of sorbitol reduced the body weight of both pupae and adults. This study predicted that rearing bee brood could be one of the factors for the selectivity of pollen with higher proline and glutamic acid during the foraging of bees.
Introduction
Insects as pollinators provide an imperative ecosystem service for 76% of global crops and wild plants (Klein et al., 2007; Stanley et al., 2020; Layeka et al., 2022). Plants including Fagopyrum esculentum Moench and Zinnia elegans Cav. can conserve pollinators (Lee and Jung, 2019). Factors including pests, microorganisms, pesticides, and poor nutrition are threatening pollinator richness and abundance (Goulson et al., 2015). Nutritional demands for these pollinators can be moderated by humans using landscape management (Vaudo et al., 2015). However, studies on the nutritional composition of pollens are required for such landscape management for both solitary and social pollinators. The value of contribution of pollinators to global food production is approximately €153 billion (Gallai et al., 2009).
Honey bee foraging depends on pollen and nectar quality (Begna et al., 2020) to meet the requirements of their dietary nutrients, which are essential to maintaining healthy colonies. Nutrients help in tissue building for growth and development (Simpson and Raubenheimer, 2011). Pollens provide protein for the development and growth of honey bees, whereas nectars are a source of carbohydrates providing the energy needed for flight (Brodschneider and Crailsheim, 2010). The protein content of pollens varies from plant to plant and also geographically (Roulston et al., 2000; Taha et al., 2019; Al-Kahtani and Taha, 2020).
Insects mostly choose their foraging diet that meets their nutritive criteria to maintain a balance of their physiological needs (Waldbauer and Friedman, 1991; Simpson et al., 2015). Honeybees are also selective to feed on specific pollens in diverse flowering landscapes (Smart et al., 2017). Honey bees choose pollen sources via complex criteria, including quality (nutrients) and availability (Layek et al., 2020). The longevity, physiological metabolism, and ovarian activation of bees are also dependent on pollen quality (Schmidt et al., 1987; Human et al., 2007; Alaux et al., 2011).
Bee pollens contain a high level of protein including essential and non-essential amino acids based on their origin and season (Tasei and Aupinel, 2008; Margoan et al., 2012; Taha, 2015; Al-Kahtani et al., 2020). Proline and glutamic acids are predominant non-essential amino acids found in most pollens (Szczesna, 2006; Mondal et al., 2009; Nicolson and Human, 2013; Bayram et al., 2021). Glutamic acid is a vital neurotransmitter that regulates the processes of learning and memory (Locatelli et al., 2005). It is also believed that glutamic acid increases the cell growth of an insect (Chou, 2014). Proline is needed to act as fuel for the flight of honey bees (Carter et al., 2006; Campbell et al., 2016). These findings led us to consider the possible role of these non-essential amino acids in honey bees.
The sugars are a critical source of energy metabolism in insects (Vaulont et al., 2000) and also act as a feeding stimulant (Ramirez, 1990). Sugar alcohols belong to another class of carbohydrates that can affect the development and survivorship of insects (Lee et al., 2021). Sorbitol, a polyol, plays an important role in the development and thermotolerance of bees (Storey and Storey, 1992; Lee et al., 2021). Therefore, the objective of the present study was to evaluate the possible role of proline, glutamic acid, and sorbitol on bee brood and improve the artificial diet of bee brood.
Materials and methods
Honey bee larvae collection and diet preparation
The eggs of A. mellifera were collected from a single hive maintained in an apiary of Andong National University. The queen and a few workers were excluded on a new frame using a cage for a period of 24 h. Queen was then released, and newly laid eggs containing the frame were again maintained in the hive by putting a cage on it for an additional 70 h. The frame was then transferred to the laboratory for grafting. Artificial diets (see detail below) were placed into cups of each well of a 48-well-plate and placed at 35oC for 30 min before larval grafting. The larvae were individually transplanted into wells of a 48-well-plate. Larval grafting was carried out following the protocol of Schmehl et al. (2016). Grafted larvae were then reared in the desiccators at 35oC with a 94% relative humidity obtained using K2SO4 saturated solution. We tested the role of nutrients in bee brood rearing by adding nutrients at various concentrations (1, 4, and 8%) to the standard diet (Table 1) developed by Schmehl et al. (2016).
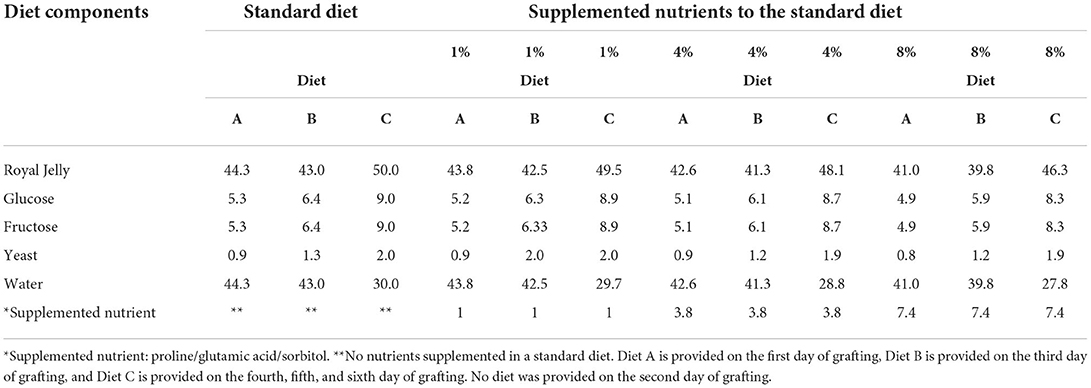
Table 1. The percentage of diet components of the standard diet and different percentages of supplemented nutrients added to the standard diet.
Measuring development and survival
To test the role of a prepared diet on larval development and survival of honey bees, predetermined volumes of the diet were subjected to individual larvae in rearing plates following the protocol of Schmehl et al. (2016). In each treatment, 48 individual larvae were subjected and were repeated two times.
Survival and development were recorded daily in larval and pupal stages. Larvae were considered dead if they were found immobile on a diet and appeared black. Change of color and appearance of fungus on the pupae body were indications of their mortality. The body weight of pupae and adults was measured with an electronic balance (1 mg) (AINSWORTH, US/Model:10).
Statistical analysis
One-way analysis of variance (ANOVA) was applied to determine the effect of different concentrations of nutrients on the development, survival, and weight of the bee brood. The percentage data were arcsine transformed for the standardization purpose for analysis. Means for all ANOVAs were compared by the least significant difference (LSD) test at p ≤ 0.05.
Result
Table 2 shows that proline significantly varied the duration of development of larvae (F3, 311 = 25.3; P < 0.001), pupae (F3, 304 = 52.5; P < 0.001), and total brood (F3, 300 = 55.6; P < 0.001). Pro1 did not change the development of larvae from the standard diet. However, higher concentrations of proline (Pro4 and Pro8) quickly completed the development of larvae, while, all concentrations of proline (Pro1, Pro4, and Pro8) quickly completed the development of pupae and total brood duration. The shortest development of larvae (10.7 d), pupae (9.5 d), and total brood duration (20.1 d) were recorded on Pro8.
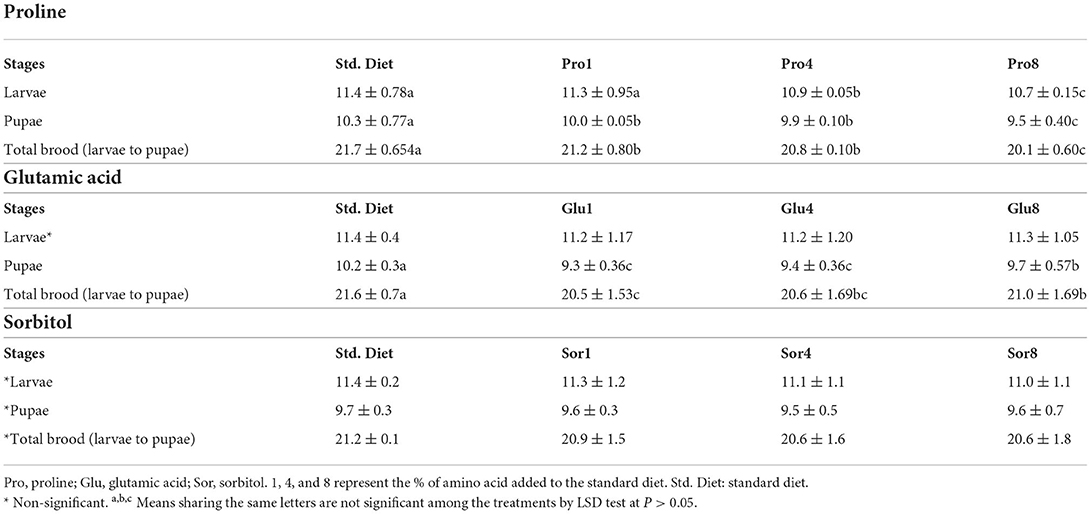
Table 2. The effects of different concentrations of proline, glutamic acid, and sorbitol (1, 4 and, 8%) supplementation on the development of bee brood (days ± S.E) and standard diets fed to honey bee larvae.
The development of bee larvae was unaffected by a supplemented diet of glutamic acid (F3, 303 = 1.2; P > 0.05). However, glutamic acid concentrations of Glu4 quickly completed the development of bee pupae in 9.4 d (F3, 280 = 33.3; P < 0.001) and total brood in 20.6 d (F3, 280 = 8.27; P < 0.001) when compared to the other glutamic acid concentrations. Sorbitol have no impact on larval development (F3, 291 = 0.64; P > 0.05), pupae (F3, 283 = 1.68; P > 0.05), and total brood duration (F3, 255 = 1.84; P > 0.05) of honey bees.
The proline concentrations significantly varied the survival of larvae (F3, 4 = 9.75; P < 0.05) and total brood (F3, 4 = 66.7; P < 0.001) but pupal survival was unchanged (F3, 4 = 0.87; P > 0.05). The highest survival of larvae was 93.7% when fed on the Pro8 diet (Table 3).
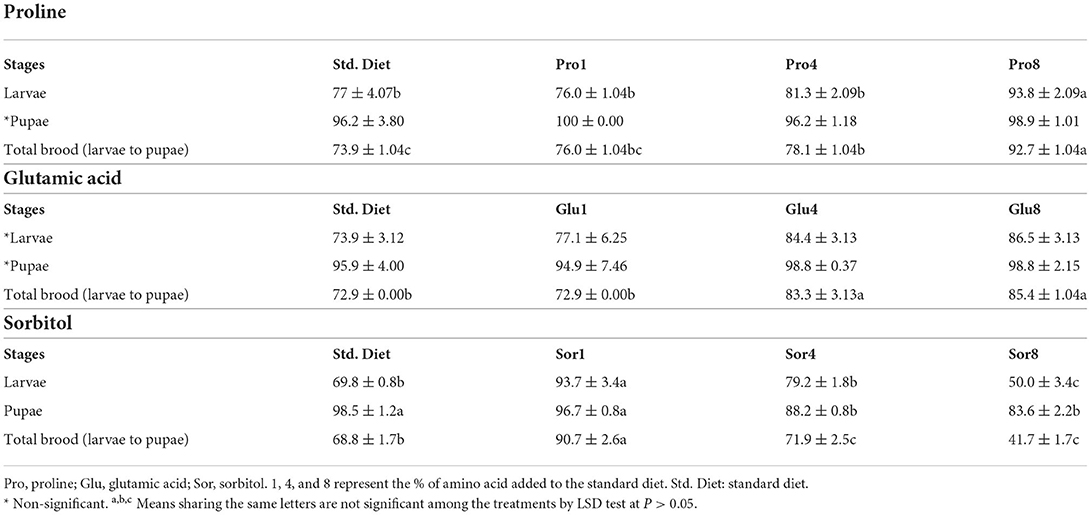
Table 3. The effects of different concentrations of proline, glutamic acid, and sorbitol (1, 4, and 8%) supplementation on the survival of bee brood (%±S.E) and standard diets fed to honey bee larvae.
The glutamic acid neither varied the survival of larvae (F3, 4 = 3.0; P > 0.05) nor the survival of pupae (F3, 4 = 0.36; P > 0.05). However, the survival of the total brood varied across the different concentrations of glutamic acid (F3, 4 = 13.7; P = 0.014). The maximum survival of the total brood was 85.4% and 83.3% when fed with the Glu8 and Glu4 diets.
Sorbitol significantly varied the survival of larvae (F3, 4 = 32.8; P < 0.01), pupae (F3, 4 = 16.9; P < 0.01) and total brood (F3, 4 = 57.8; P < 0.001). Sor1 increased the survival of larvae (90.7%) as compared to all tested concentrations of sorbitol including the standard diet. Pupal survival was the lowest (83.6%) at Sor8. Total brood survival was reduced to 41.7% when honey bee larvae were fed with higher sorbitol concertation, Sor8.
The proline significantly increased the weight of both pupae (F3, 311 = 18.4; P < 0.001) and adults (F3, 304 = 28.3; P < 0.001) when larvae were fed with proline diets (Table 4). Both pupae and adults weighed 0.100 mg on the Pro8 diet, which was higher than the other lower concentrations of proline.
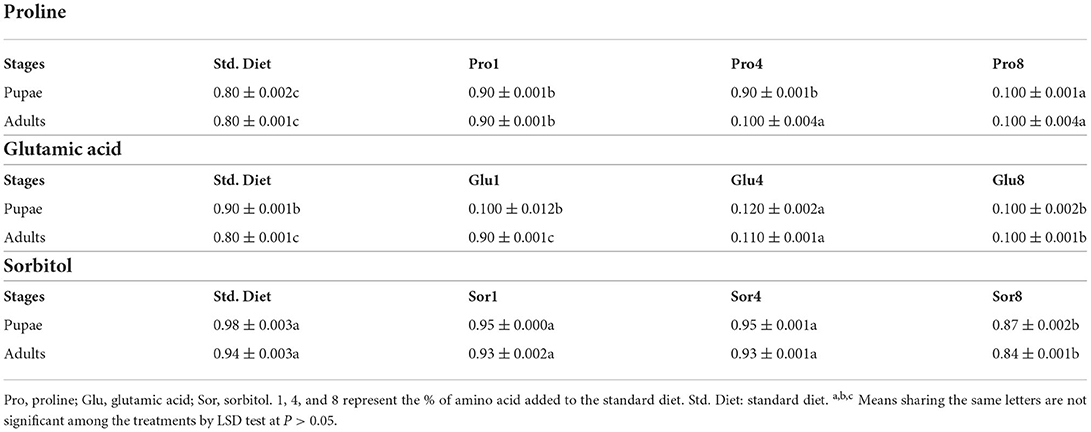
Table 4. The effect of different concentrations of proline, glutamic acid, and sorbitol (1, 4, and 8%) supplementation on body weight of bee brood (mg±S.E) and standard diets fed to honey bee larvae.
Similarly, the weights of pupae (F3, 303 = 4.14; P < 0.01) and adults (F3, 280 = 14.0; P < 0.001) were also increased when larvae were fed with the glutamic acid diet. The Glu4 increased the weights to 0.120 and 0.110 mg of pupae and adults, respectively. The sorbitol only significantly decreased the weight of adults (F3, 255 = 5.07; P < 0.01) but had no impact on pupae (F3, 278 = 1.11; P > 0.05). The Sor8 decreased the weight of adults to 0.84 mg when compared to the standard diet.
Discussion
The proline and glutamic acid are predominant non-essential amino acids found in bee pollens (Nicolson and Human, 2013). However, their roles in the development and survival of bee brood were not clearly defined. This study presented that the addition of proline and glutamic acid in 1–8% to the diet resulted in fast development and reduced mortality in honey bee brood.
An increase in proline concentration in the standard diet, especially higher concentrations of proline, reduced the development time of all stages of bee brood. The proline is well-known for its role in energy metabolism (Bursell, 1981). Ito and Arai (1966) showed that proline is an important nutrient for the larval development of silkworms. Another study showed that proline predominantly plays a major role in energy metabolism as compared to other amino acids (Lamour et al., 2005). The proline has been proven as a phagostimulant in adult honey bees (Lanza, 1988; Darvishzadeh et al., 2015). Similarly, the higher consumption of Africanized bee bread than European bee bread is also believed for its higher concentration of proline (Degrandi-Hoffman et al., 2013). However, our results for proline were indirectly contradictory with the previous study where higher protein did not affect the larval development of honey bees (Helm et al., 2017). This discrepancy may be due to diet composition. They increased protein content by increasing the dose of royal jelly whereas we only increased the proline content.
In contrast, the supplementation of glutamic acid did not have any impact on larval development. Similar findings have been reported in a previous study (Friend et al., 1957; Chang, 2004). However, it reduced the developmental time of pupae compared to a standard diet. This difference could be due to the differential nutrient requirements of larvae and pupae of honey bees. The amount of glutamic acid present in larvae of the honey bee sub-species (A. mellifera ligustica) is 5.0 g/100 g DM while 8.4 g/100 g DM in pupae (Ghosh et al., 2016). The reduction of muscle contraction was shown by the deletion of glutamic acid-rich C-terminal extension of the Drosophila TnT gene (Troponin T) (Cao et al., 2020). Glutamic acid functions as a neurotransmitter for learning and memory in honey bees (Locatelli et al., 2005). Proline and glutamic acid may also enhance the thermotolerance of insects (Fields et al., 1998; Koštál et al., 2016). Sorbitol did not affect the development of brood. This finding is consistent with a previous study on the German roach, Blattella germanica L., which was fed sorbitol supplementation along with casein (Gordon, 1959).
The survival of larvae and total bee brood was also increased with the increasing proline concentration in the standard diet. However, only the highest concentration of proline (Pro8) supplemented diet increased the survival during the larval period, whereas Pro4 and Pro8 increased the survival of total bee brood. This showed that proline might better oxidize only at higher concentrations in the presence of carbohydrates in the bee brood. Proline act as a co-substrate extensively with carbohydrate in insects (Zebe and Gade, 1993). Glutamic acid only increased the survival of the total bee brood when added to the standard diet. The larval diet composition of honey bees affects the developmental period and survival of immature bees to adults (Nicholls et al., 2021). Our results did not agree with earlier studies that showed higher mortalities of larvae (Helm et al., 2017) and adult (Pirk et al., 2010) bees when fed on a diet with higher protein concentrations. These contradictory results are possibly due to diet composition. In the present study, only proline and glutamic acid concentrations were increased. This differential response not only leads to inferring that individual components may have different roles in the total proteinous diet but also in the developmental stage. The lowest tested concentration (1%) of sorbitol increased the survival of larvae to 20% and the total survival of larvae emergence into an adult. The 5% of sorbitol also increased the survival of Aedes aegypti against water only (Galun and Fraenkel, 1957). Sorbitol is a feeding stimulant for some insects (Lee et al., 2021) and its role is well-known as a thermoprotectant (Salvucci et al., 2000). Higher concentrations (4 and 8%) of sorbitol decreased the survival of the bee brood in the present study. The survival of whitefly adults was reduced when fed on a diet supplemented with 10% sorbitol. Hu et al. (2010) confirmed the insecticidal effect of sorbitol on bee brood at higher concentrations (4 and 8%). This suggested that the toxicity of sorbitol is concentration dependent on honey bee brood.
Supplementation of both proline and glutamic acid increased the body weight of pupae and adults of honey bees. Body size is typically associated with nutrient storage (Sun et al., 2015). However, storage of nutrients could be more in the case of glutamic acid because bees did not use glutamic acid as a source of energy in development as compared to proline. The availability of nutrients during developmental stages determines the body size of an adult which is a prominent parameter to describe the fitness of individuals (Nijhout, 2003). There is no study available on any insects regarding sorbitol's impact on honey bee's weight that directly relates to our results. Our result showed that sorbitol reduced the body weight of bee brood but at a higher concentration. Therefore, this weight reduction cannot be because of starvation and also because higher concentrations of sorbitol did not reduce the brood. This weight reduction may be due to the ingestion of food supplemented with higher concentrations. Some polyols, such as erythritol, can reduce the body weight of insects (Díaz-Fleischer et al., 2019).
Overall, this is the first study that highlights the importance of proline and glutamic acid to the development and survival of bee brood. Both non-essential amino acids have divergent roles in the bee brood lives. The proline is more readily available as energy metabolism for all developmental stages, whereas glutamic acid only affects the development of pupae. The proline influenced higher survival of bee larvae but larval survival was unchanged with supplementation of glutamic acid. Both non-essential amino acids are important for the body size of pupae and adults. These beneficial effects of both proline and glutamic acid on bee brood may be due to honey bees' preferences to forage on pollen containing higher proline and glutamic acid. However, this needs to be tested experimentally. These nutrients may also be supplemented to the diets used for bee hives to increase brood production and can help to cope with poor nutrition due to the low availability and quality of floral resources. The role of sorbitol on bee brood is concentration dependent. A lower concentration of sorbitol improved the survival of bee brood and increased the thermotolerance of insects (Salvucci et al., 2000). The effect of sorbitol on the thermoregulation of honey bee brood should be tested in the future. The mortality rate of honey bee larvae is one of the biggest issues for rearing honey bee brood under laboratory conditions. These can be attributed to handling and growing environment. This study also showed that sorbitol could potentially be one of the chemical options to reduce issues such as decreasing mortality.
Data availability statement
The original contributions presented in the study are included in the article/supplementary material, further inquiries can be directed to the corresponding author.
Author contributions
CJ: project administration, data analysis guide, supervision, resources, review and editing manuscript, and secured funding. MN-u-A: conceptualization, execution, data analysis, writing-original draft, and review and editing manuscript. All authors have read and agreed to the published version of the manuscript.
Funding
This research was funded by the BSRP through the National Research Foundation of Korea (NRF), Ministry of Education, Grant Number NRF-2018R1A6A1A03024862.
Acknowledgments
We appreciate Prof. V. B. Meyer-Rochow for editing the English in the article.
Conflict of interest
The authors declare that the research was conducted in the absence of any commercial or financial relationships that could be construed as a potential conflict of interest.
Publisher's note
All claims expressed in this article are solely those of the authors and do not necessarily represent those of their affiliated organizations, or those of the publisher, the editors and the reviewers. Any product that may be evaluated in this article, or claim that may be made by its manufacturer, is not guaranteed or endorsed by the publisher.
References
Alaux, C., Dantec, C., Parrinello, H., and Le Conte, Y. (2011). Nutrigenomics in honey bees: digital gene expression analysis of pollen's nutritive effects on healthy and varroa-parasitized bees. BMC Genom. 12, 496. doi: 10.1186/1471-2164-12-496
Al-Kahtani, S. N., and Taha, E. K. A. (2020). Seasonal variations in nutritional composition of honeybee pollen loads. J. Kans. Entomol. Soc. 93, 105–112. doi: 10.2317/0022-8567-93.2.105
Al-Kahtani, S. N., Taha, E. K. A., Khan, K.H. A., Ansari, M. J., Farag, S. A., Shawer, D. M. B., et al. (2020). Effect of harvest season on the nutritional value of bee pollen protein. PLoS ONE 15, e0241393. doi: 10.1371/journal.pone.0241393
Bayram, N. E., Gercek, Y. C., Celik, S., Mayda, N., Kostic, A. Z., Dramicanin, A. M., et al. (2021). Phenolic and free aino acid profiles of bee bread and bee pollen with the same botanical origin–similarities and differences. Arab. J. Chem. 14, 103004. doi: 10.1016/j.arabjc.2021.103004
Begna, T., Ulziibayar, D., Noor-ul-Ane, M., Shin, J. H., and Jung, C. (2020). Offering pollen as reward enhances foraging activity of honey bee, Apis mellifera on strawberry greenhouse during winter season. J. Apic. 35, 111–118. doi: 10.17519/apiculture.2020.06.35.2.111
Brodschneider, R., and Crailsheim, K. (2010). Nutrition and health in honey bees. Apidologie . 41, 278–294. doi: 10.1051/apido/2010012
Bursell, E. (1981). “The role of proline in energy metabolism,” in Energy Metabolism in Insects, ed. R. G. H. Downer (New York, NY, Plenum Press), 135–154.
Campbell, J. B., Nath, R., Gadau, J., Fox, T., DeGrandi-Hoffman, G., and Harrisson, J. F. (2016). The fungicide Pristine® inhibits mitochondrial function in vitro but not flight metabolic rates in honey bees. J. Insect Physiol. 86, 11–16. doi: 10.1016/j.jinsphys.2015.12.003
Cao, T., Sujkowski, A, Cobb, T, Wessells, R. J, and Jin, J. P. (2020). The glutamic acid-rich-long C-terminal extension of troponin T has a critical role in insect muscle functions. J. Biol. Chem. 295, 3794–3807. doi: 10.1074/jbc.RA119.012014
Carter, C., Shafir, S., Yehonatan, L., Palmer, R. G., and Thornburg, R. (2006). A novel role for proline in plant floral nectars. Sci. Nat. 93, 72–79. doi: 10.1007/s00114-005-0062-1
Chang, C. L. (2004). Effect of amino acids on larvae and adults of Ceratitis capitata (Diptera: Tephritidae). Ann. Entomol. Soc. Am. 97, 529–535. doi: 10.1603/0013-8746(2004)097[0529:EOAAOL]2.0.CO;2
Chou, C. C. (2014). Identification of the pivotal role of glutamate in enhancing insect cell growth using factor analysis. Cytotechnology 66, 853–860. doi: 10.1007/s10616-013-9637-4
Darvishzadeh, A., Hosseininaveh, V., Nehzati, G., and Nozari, J. (2015). Effect of proline as a nutrient on hypopharyngeal glands during development of Apis mellifera (Hymenoptera: Apidae). Arthropods 4, 137–143.
Degrandi-Hoffman, G., Eckholm, B. J., and Huang, M. H. (2013). A comparison of bee bread made by Africanized and European honey bees (Apis mellifera) and its effects on hemolymph protein titers. Apidologie 44, 52–63. doi: 10.1007/s13592-012-0154-9
Díaz-Fleischer, F., Arredondo, J., Lasa, R., Bonilla, C., Debernardi, D., and Pérez-Staples, D. (2019). Sickly sweet: insecticidal polyols induce lethal regurgitation in dipteran pests. Insects 10, 53. doi: 10.3390/insects10020053
Fields, P. G., Fleurat-Lessard, F., Lavenseau, L., Febvay, G., Peypelut, L., and Bonnot, G. (1998). The effect of cold acclimation and deacclimation on cold tolerance, trehalose and free amino acid levels in Sitophilus granarius and Cryptolestes ferrugineus (Coleoptera). J. Insect Physiol. 44, 955–965. doi: 10.1016/S0022-1910(98)00055-9
Friend, W. G., Backs, R. H., and Cass, L. M. (1957). Studies on amino acid requirements of larvae of the onion maggot, Hylemya antique (MG.), under aseptic conditions. Can. J. Zool. 35, 535–543. doi: 10.1139/z57-045
Gallai, N., Salles, J. M., Settele, J., and Vaissière, B. E. (2009). Economic valuation of the vulnerability of world agriculture confronted with pollinator decline. Ecol. Econom. 68, 810–821. doi: 10.1016/j.ecolecon.2008.06.014
Galun, R., and Fraenkel, G. (1957). Physiological effects of carbohydrates in the nutrition of a mosquito, Aedes aegypti and two flies, Sarcophaga bullata and Musca domestica. J. Cell. Comp. Physiol. 50, 1–23. doi: 10.1002/jcp.1030500102
Ghosh, S., Jung, S., and Meyer-Rochow, V. B. (2016). Nutritional value and chemical composition of larvae, pupae and adults of worker honey bee Apis mellifera ligustica as a sustainable food source. J Asia Pacific Entomol. 19, 487–495. doi: 10.1016/j.aspen.2016.03.008
Gordon, H. T. (1959). Minimal nutritional requirements of the German roach, Blattella germanica L. Ann. N.Y. Acad. Sci. 77, 290–351. doi: 10.1111/j.1749-6632.1959.tb36910.x
Goulson, D, Nicholls, E., Botías, C., and Rotheray, E. L. (2015). Bee declines driven by combined stress from parasites, pesticides, and lack of flowers. Sci. 27, 347. doi: 10.1126/science.1255957
Helm, B. R., Slater, G. P., Rajamohan, A., Yocum, G. D., Greenlee, K. J., and Bowsher, J. H. (2017). The geometric framework for nutrition reveals interactions between protein and carbohydrate during larval growth in honey bees. Biol. Open. 6, 872–880. doi: 10.1242/bio.022582
Hu, J. S., Gelman, D. B., Salvucci, M. E., Chen, Y. P., and Blackburn, M. B. (2010). Insecticidal activity of some reducing sugars against the sweet potato whitefly, Bemisia tabaci, biotype B. J. Insect Sci. 10, 203. doi: 10.1673/031.010.20301
Human, H., Nicolson, S. W., Strauss, K., Pirk, C. W. W., and Dietemann, V. (2007). Influence of pollen quality on ovarian development in honeybees Apis mellifera scutellata. J. Insect Physiol. 53, 649–655. doi: 10.1016/j.jinsphys.2007.04.002
Ito, T., and Arai, N. (1966). Nutrition of silkworm, Bombyx mori, VIII. Amino acid requirements and nutritive effect of various proteins. Bull. Seric. Expt. Stn., Tokyo 19, 345–373.
Klein, A. M., Vaissiere, B. E., Cane, J. H., Steffan-Dewenter, I., Cunningham, S. A., Kremen, C., et al. (2007). Importance of pollinators in changing landscapes for world crops. Proc. R. Soc. B: Biol. Sci. 274, 303–313. doi: 10.1098/rspb.2006.3721
Koštál, V., Korbelová, J., Poupardin, R., Moos, M., and Šimek, P. (2016). Arginine and proline applied as food additives stimulate high freeze tolerance in larvae of Drosophila melanogaster. J. Exp. Biol. 219, 2358–2367. doi: 10.1242/jeb.142158
Lamour, N., Riviere, L., Coustou, V., Coombs, G. H., Barrett, M. P., and Bringaud, F. (2005). Proline metabolism in procyclic Trypanosoma brucei is down-regulated in the presence of glucose. J. Biol. Chem. 280, 11902–11910. doi: 10.1074/jbc.M414274200
Lanza, J. (1988). Ant preferences for passiflora nectar mimics that contain amino-acids. Biotropica. 20, 341-344. doi: 10.2307/2388328
Layek, U., Manna, S. S., and Karmakar, P. (2020). Pollen foraging behaviour of honey bee (Apis mellifera L.) in southern West Bengal, India. Palynol. 44, 114–126. doi: 10.1080/01916122.2018.1533898
Layeka, U., Dasa, U., and Karmakarb, P. (2022). The pollination efficiency of a pollinator depends on its foraging strategy, flowering phenology, and the flower characteristics of a plant species. J. Asia-Pacific Entomol. 25, 101882. doi: 10.1016/j.aspen.2022.101882
Lee, C., and Jung, C. (2019). Flower habitat supplementation can conserve pollinators and natural enemies in agricultural ecosystem: case study in the pepper field. J. Apic. 34, 141. doi: 10.17519/apiculture.2019.06.34.2.141
Lee, S-H., Choe, D-H., and Lee, C-Y. (2021). The impact of artificial sweeteners on insects. J. Econ. Entomol. 114, 1–13. doi: 10.1093/jee/toaa244
Locatelli, F., Bundrock, G., and Muller, U. (2005). Focal and temporal release of glutamate in the mushroom bodies improves olfactory memory in Apis mellifera. J. Neurosci. 25, 11614–11618. doi: 10.1523/JNEUROSCI.3180-05.2005
Margoan, R., Marghitas, L., Dezmirean, D. S., Bobis, O., and Mihai, C. M. (2012). Physical-chemical composition of fresh bee pollen from Transylvania. Bull. UASVM Anim. Sci. Biotech. 69, 351–355.
Mondal, A. K., Mondal, S., and Mandal, S. (2009). The free amino acids of pollen of some angiospermic taxa as taxonomic markers for phylogenetic interrelationships. Curr. Sci. 96, 1071–1081.
Nicholls, E., Rossi, M., and Niven, J. E. (2021). Larval nutrition impacts survival to adulthood, body size and the allometric scaling of metabolic rate in adult honeybees. J. Exp. Biol. 224, 14. doi: 10.1242/jeb.242393
Nicolson, S. W., and Human, H. (2013). Chemical composition of the ‘low quality' pollen of sunflower (Helianthus annuus L., Asteraceae). Apidologie . 44, 144–152. doi: 10.1007/s13592-012-0166-5
Nijhout, H. F. (2003). The control of body size in insects. Dev. Biol. 261, 1–9. doi: 10.1016/S0012-1606(03)00276-8
Pirk, C. W. W., Boodhoo, C., Human, H., and Nicolson, S. W. (2010). The importance of protein type and protein to carbohydrate ratio for survival and ovarian activation of caged honeybees (Apis mellifera scutellata). Apidologie 41, 62–72. doi: 10.1051/apido/2009055
Ramirez, I. (1990). Why do sugars taste good? Neurosci. Biobehav. Rev. 14, 125–134. doi: 10.1016/S0149-7634(05)80213-1
Roulston, T. H., Cane, J. H., and Buchmann, S. L. (2000). What governs protein content of pollen: pollinator preferences, pollen–pistil interactions, or phylogeny? Ecol. Monogr. 70, 617–643. doi: 10.1890/0012-9615(2000)070[0617:WGPCOP]2.0.CO;2
Salvucci, M. E., Stecher, D. S., and Henneberry, T. J. (2000). Heat shock proteins in whiteflies, an insect that accumulates sorbitol in response to heat stress. J. Therm. Bio. 25, 363–371. doi: 10.1016/S0306-4565(99)00108-4
Schmehl, D.R., Tomé, H. V. V., Mortensen, A. N., Martins, G. F., and Ellis, J. D. (2016). Protocol for the in vitro rearing of honey bee (Apis mellifera L.) workers. J. Apic. Res. 55, 113–129. doi: 10.1080/00218839.2016.1203530
Schmidt, J. O., Thoenes, S. C., and Levin, M. D. (1987). Survival of honey bees, Apis mellifera (Hymenoptera: Apidae), fed various pollen sources. J. Econ. Entomol. 80, 176–183. doi: 10.1093/aesa/80.2.176
Simpson, S. J., Clissold, F. J., Lihoreau, M., Ponton, F., Wilder, S. M., and Raubenheimer, D. (2015). Recent advances in the integrative nutrition of arthropods. Ann. Rev. Entomol. 60, 293–311. doi: 10.1146/annurev-ento-010814-020917
Simpson, S. J., and Raubenheimer, D. (2011). The nature of nutrition: a unifying framework. Aust. J. Zool. 59, 350–368. doi: 10.1071/ZO11068
Smart, M. D., Cornman, R. S., Iwanowicz, D. D., McDermott-Kubeczko, M., Pettis, J. S., Spivak, M. S., et al. (2017). A comparison of honey bee-collected pollen from working agricultural lands using light microscopy and ITS metabarcoding. Environ. Entomol. 46, 38–49. doi: 10.1093/ee/nvw159
Stanley, D. A., Msweli, S. M., and Johnson, S. D. (2020). Native honeybees as flower visitors and pollinators in wild plant communities in a biodiversity hotspot. Ecosphere 11, 1–12. doi: 10.1002/ecs2.2957
Storey, K. B., and Storey, J. M. (1992). Biochemical adaptations for winter survival in insects. Adv. Low. Temp. Biol. 1, 101–140. doi: 10.1098/rstb.1990.0036
Sun, X., Small, G. E., Zhou, X., Wang, D., Li, H., and Liu, C. (2015). Variation in C:N:S stoichiometry and nutrient storage related to body size in a holometabolous insect (Curculio davidi) (Coleoptera: curculionidae) larva. J. Insect Sci. 15, 1–6. doi: 10.1093/jisesa/iev004
Szczesna, T. (2006). Protein content and amino acid composition of bee-collected pollen from selected botanical origins. J. Apic. Sci. 50, 81–90. doi: 10.16/j.sjbs.2017.06.003
Taha, E. K. A. (2015). Chemical composition and amounts of mineral elements in honeybee-collected pollen in relation to botanical origin. J. Apic Sci. 59, 75–81. doi: 10.1515/jas-2015-0008
Taha, E. K. A., Al-Kahtani, S. N., and Taha, R. (2019). Protein content and amino acids composition of bee-pollens from major floral sources in Al-Ahsa, Eastern Saudi Arabia. Saudi J. Biol. Sci. 26, 232–237.
Tasei, J. N., and Aupinel, P. (2008). Nutritive value of 15 single pollens and pollen mixes tested on larvae produced by bumblebee workers (Bombus terrestris, Hymenoptera: Apidae). Apidologie 39, 397–409.
Vaudo, A. D., Tooker, J. F., Grozinger, C. M., and Patch, H. M. (2015). Bee nutrition and floral resource restoration. Curr. Opin. Insect Sci. 10, 133–141. doi: 10.1016/j.cois.2015.05.008
Vaulont, S., Vasseur-Cognet, M., and Kahn, A. F. (2000). Glucose regulation of gene transcription. J. Biol. Chem. 275, 555–558. doi: 10.1074/jbc.R000016200
Waldbauer, G., and Friedman, S. (1991). Self-selection of optimal diets by insects. Annu. Rev. Entomol. 36, 43–63. doi: 10.1146/annurev.en.36.010191.000355
Keywords: amino acids, brood development, brood survival, brood weight, pollen
Citation: Noor-ul-Ane M and Jung C (2022) Effect of non-essential amino acids (proline and glutamic acid) and sugar polyol (sorbitol) on brood of honey bees. Front. Ecol. Evol. 10:1009670. doi: 10.3389/fevo.2022.1009670
Received: 02 August 2022; Accepted: 20 September 2022;
Published: 10 November 2022.
Edited by:
Todd Jason McWhorter, University of Adelaide, AustraliaCopyright © 2022 Noor-ul-Ane and Jung. This is an open-access article distributed under the terms of the Creative Commons Attribution License (CC BY). The use, distribution or reproduction in other forums is permitted, provided the original author(s) and the copyright owner(s) are credited and that the original publication in this journal is cited, in accordance with accepted academic practice. No use, distribution or reproduction is permitted which does not comply with these terms.
*Correspondence: Chuleui Jung, Y2p1bmdAYW5kb25nLmFjLmty