- Department of Biological Sciences, University of Calgary, Calgary, AB, Canada
Selection for specialized coevolutionary relationships can arise if generalized opportunistic pollinators, while still delivering some pollen, operate as less effective pollen delivery agents. Nevertheless, generalization could buffer high-latitude communities from loss of specialist pollinator species by providing some pollination service. Currently, there is limited understanding of the ecosystem services provided by generalized pollinators and whether they increase the fitness of the plants they visit. Network data and thorough observations of floral visitors, paired with estimates of seed set, offer some insight into the role of generalists, which in turn can inform us about how plants are likely to respond to ecosystem disturbances, such as losses of some pollinators, or changes in land cover. Here, we report on plant-pollinator visitation networks in Canada with high levels of generalization and examine the effects of generalization on seed set under different disturbance histories. We also then take a case study of one crop wild relative, Rubus arcticus or Arctic raspberry, and report on a near-complete characterization of pollinator interactions in different environmental conditions. Our findings indicate that generalized pollinators, though frequent and robust to variable temperatures and moisture conditions, do not appear to play a strong role in increasing the reproductive output of many plant species, and may provide only a weak buffer against the stronger effects of disturbance.
Introduction
While it is well documented that plant species can vastly differ in the number of pollinator species that visit, there are still only a few studies providing evidence on whether the degree of pollinator specialization affects the relative proportion of ovules that develop into seeds (Knight et al., 2005; Bennett et al., 2020). It is currently unclear whether specialist plant lineages experience higher extinction rates, in part due to incomplete understanding of the extent to which species depend on their coevolved partnerships with key functional groups of pollinators (Fenster et al., 2004; Colles et al., 2009). In mutualistic networks, generalists may be buffered from the effects of decline of some mutualistic partners because those functions lost could be supplied by other species with similar preferences (Sonne et al., 2022). However, a high level of pollinator specialization can promote increased precision in pollen deposition and consequently increase seed production (Armbruster, 2017). In other words, although a pollinator deficit might lead to poor seed set over longer time scales, enhanced pollen deposition can lead to selection for specialization through plant reproductive success over shorter time scales (Potts et al., 2010).
Approximately 87.5% of flowering plant species are pollinated to some degree by animals (Ollerton et al., 2011), with roughly half of these poised to experience ≥80% reduction in seed set should pollinators become unavailable (Rodger et al., 2021). There have been increasing global trends of pollinator decline with climate change as a contributing factor (Potts et al., 2010). Pollination is considered an ecosystem service that can be positively influenced by diversity (Albrecht et al., 2012), although the mechanism of niche complementarity appears complex and context-dependent (Davila et al., 2012). Although diverse and generalist systems may be able to withstand the loss of some species when the impacts of threats such as climate change vary between functional groups of species (Hoehn et al., 2008), we do not currently have a thorough understanding of how the distributions of many pollinating functional groups change with environmental conditions, especially in areas relevant to cold-adapted species poised to be impacted by climate change (Abeli et al., 2018). For instance, the range size of bumblebees (Bombus spp.) has been steadily compressing since 2000 (Williams et al., 2007), with losses as large as ~300 km in the southern range limits of both European and North American bees (Kerr et al., 2015). On the other hand, butterfly ranges have shifted 14–240 km northward within the past 100 years (Parmesan et al., 1999) with butterflies in western Canada exhibiting low abilities to track climate (Lewthwaite et al., 2018). Although often overlooked, flies are also important pollinators as they are key pollinators for many northern plant species, such as Dryas spp. (Tiusanen et al., 2016), and they have also been experiencing sizable declines with increased climate warming (Høye et al., 2013).
Whether different pollinator guilds will respond to climate change and other forms of disturbance in ways that will allow the loss of one guild to be compensated for by another is currently unknown for many generalist species. With network approaches, the data collected can usually only represent a minimal sampling of the floral visitors and the effects of the environment on the visitors cannot be determined without more detailed pollen analysis (Barker and Arceo-Gomez, 2021). Ambient air temperature and solar radiation affect the minimum temperature thresholds in which the thoracic muscles can stimulate flight and are therefore thought to be the most important microclimate factors for pollinators. Canopy openness can directly alter the microclimate for pollinators and receptive flowers. Canopy gaps not only alter the understory moisture and temperature regimes (Herrera, 1995), but they can improve the visibility of flowers below for pollinators due to the greater light penetration (Walters and Stiles, 1996). Consequently, many insects forage only within specific “microclimate” windows (Corbet et al., 1993), constrained by various temperature and irradiance regimes. Relative humidity has less of a direct effect on insects because of the impermeability of their exoskeletons, yet has an effect on nectar concentration (Corbet et al., 1979), which may alter the feeding tendencies of prospective pollinators. Conversely, wind speed can directly affect the foraging behavior of insects as it not only influences their flight navigation and landing orientation (Chang et al., 2016), but can also negate the effects of temperature and irradiance via convective cooling (Unwin and Corbet, 1991). While microclimate can have implications for feeding and pollinating activities, little is known on the differences between the major guilds of pollinators, how these factors play a role in the level of pollinator specialization that is observed, or how these differences could impact reproductive success of the plants they visit.
Measuring the proportion of ovules that develop into fruit or seed (fruit set and seed set respectively) is one common measure of plant reproductive success, yet it has been noted that these metrics are conditional on environment as well as pollination (Biesmeijer et al., 2006). Pollen limitation is a metric devised to account for variability in environmental contributions to seed and fruit production and can simply be defined as a deficiency in pollen quality or quantity that limits the reproductive fitness of a plant (Knight et al., 2005). Both metrics have been used extensively to study plant reproduction and yet few studies have concentrated in northern regions of North America (Bennett et al., 2018). We examine here the influence of diversity of floral visitors on reproductive output, while also examining environmental features that influence the level of specialization such as the degree of landscape transformation (disturbed vs. undisturbed sites) and other microsite characteristics (e.g., temperature, canopy cover) at two different sites in western Alberta.
Recent evidence suggests that conservation of wild food resources in northern regions may be challenging due to pollinator decline (Vanbergen et al., 2013) and therefore the knowledge gap of determinants of fruit and seed set of wild harvested plant species is of some concern. Thus, we specifically turn the focus to examine a northern wild harvested plant species that may be affected by pollen limitation and microclimate, the Arctic/dwarf raspberry (Rubus arcticus L.). R. arcticus is an exclusively insect pollinated plant found in a variety of different habitat types (i.e., arctic, alpine, tundra, wetlands and boreal forests; Johnson et al., 1995), and this wide array of corresponding climatic conditions could influence the efficacy and availability of pollinators. Further, members of the genus Rubus have been observed exhibiting damaged styles when too many pollinating insects, particularly invasive species, have visited flowers (Sáez et al., 2014), suggesting that species within Rubus may not benefit from increased generalization. As well, Rubus can suffer from low drupelet number or “crumbly fruit,” which is thought to arise if heat stress occurs during pollination (Graham et al., 2015). Here, we examine the pollen limitation in fruit production and seed set in R. arcticus, with the expectation that pollen limitation is likely because, the species is self-incompatible (Tammisola and Ryynanen, 1970) with the dominant pollinators being bees (Kangasjärvi and Oksanen, 1989). As R. arcticus was observed to have fragile stigmas, we further investigated (1) how the handling efficiency differs in the dominant visitors of this species in Canada and (2) whether microclimate would affect the visitation by the more efficient pollinators. These assessments of the quality of pollinator visits relate to the potential impact of pollinator loss with biodiversity declines, and selection pressures for coevolved plant-pollinator relationships in northern ecosystems.
Materials and methods
Generalization in networks: Seed set and specialization in flowering plant communities in Glenbow Ranch Provincial Park
We used data collected from six transects (three sites with high disturbance; three sites with low disturbance) within Glenbow Ranch Provincial Park described in Villalobos et al. (2019), where, at each transect, five 1-m2 plots were spaced at 5 m intervals and were observed for pollinator visitation. Disturbed sites were in areas of the park that were heavily grazed until 2008, while undisturbed sites were free of grazing and corresponded to deciduous and mixedwood forests (see Villalobos et al., 2019). Within each plot, flower counts and pollinator visit counts were conducted on each individual plant at each site during each sample day to calculate floral specialization metrics (see description in following paragraph; Villalobos et al., 2019). For this study, we analyze, for each species in the quadrat, the ripe fruits collected at the end of the season. The number of undeveloped seeds, aborted seeds and fully developed seeds of these fruit units were counted. Aborted or undeveloped seeds usually had dark color, empty cavities and lower weight compared to full developed seeds. The number of seeds per fruit per individual plant was calculated and, from that, the proportion of the total ovules that developed into seeds was calculated by dividing the total number of seeds by the total number of ovules produced by plant.
To measure the specialization level of species, we used the species specialization index (d′), which measures the reciprocal specialization index of the species within the network (for a detailed description of data collection and methodology and plant and pollinator data, see Villalobos et al., 2019). The d′ index considers the abundance of species in the community, is highly robust to sample size, and ranges from 0 (no specialization) to 1 (perfect specialists). To examine the influence of plant specialization on seed set, we performed a Generalized Linear Mixed Model (package “lme4”; Bates et al., 2015). We explored four models with the response variable as seeds per ovule and the explanatory variable(s) as plant specialization index (d’), disturbance zone, and/or the presence of an interaction term between these two variables. We then used the package “AICcmodavg” to perform model selection to compare the fit of these four models. We included the effects of plant species identity and quadrats within site type as random effects with a binomial error structure. All analyses were conducted in R 3.4.3 (R Development Core Team, 2018).
Generalization at the species level: Study site and species
Rubus arcticus is a long-lived perennial with a circumpolar distribution (Tammisola, 1988), displaying bisexual flowers that are completely self-incompatible (Tammisola and Ryynanen, 1970). The species is also capable of asexual reproduction via rhizomes, which produce dense aggregations of clonal patches (Tammisola, 1988). The flowers of R. arcticus are entomophilous (insect pollinated) and reported to be primarily pollinated by honeybees, bumblebees and to a lesser extent syrphid flies (Ryynänen, 1973). When sufficiently pollinated, R. arcticus produces raspberry-like fruit with (10-)15–30 drupelets (individual aggregate units where each drupelet contains a single seed) bound together by a centre receptacle (Ryynänen, 1973; Alice et al., 1993; Figure 1).
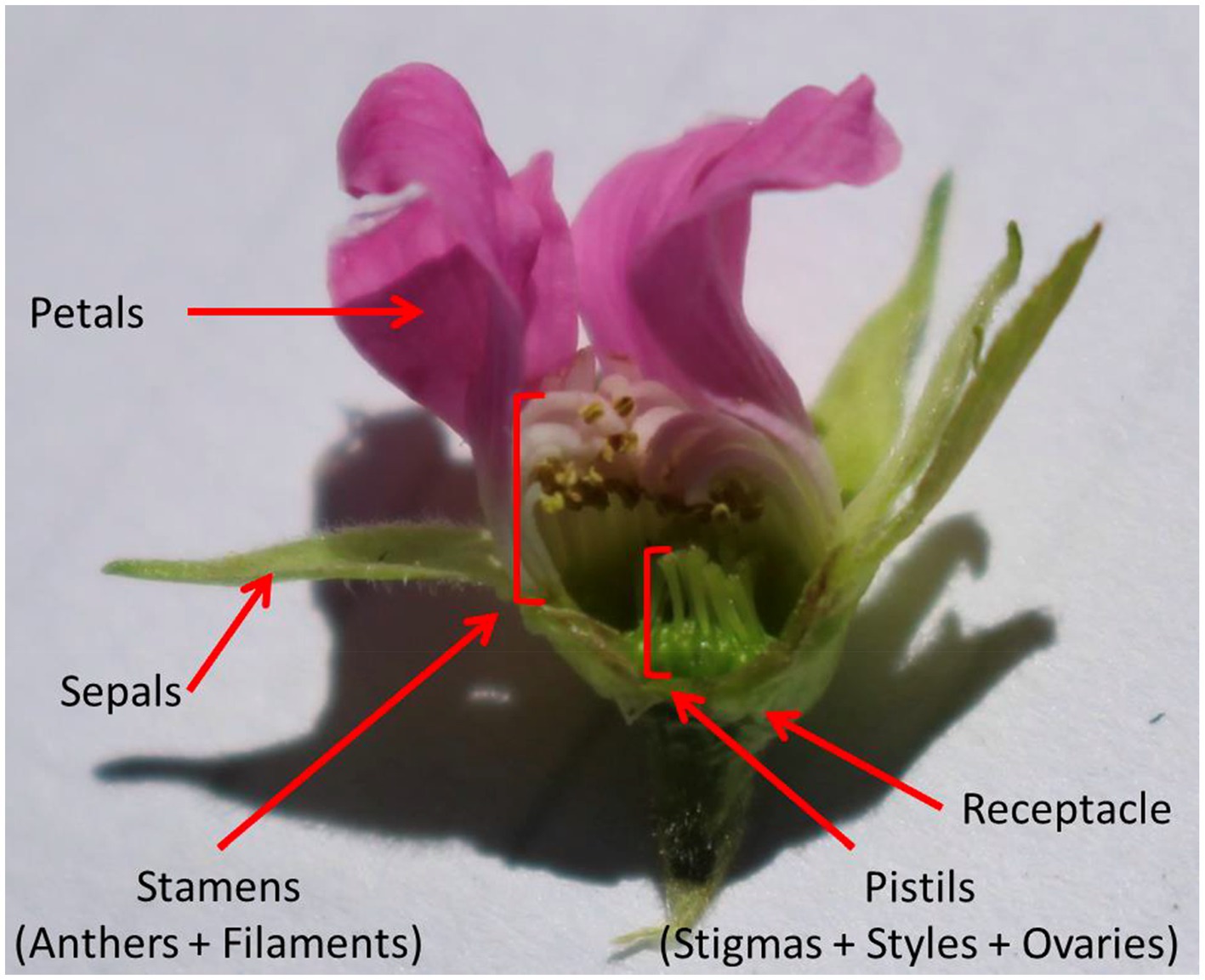
Figure 1. Longitudinal section of a Rubus arcticus flower showing restrictive morphology of receptacle.
This study site was in the north loop of the Jumpingpound Demonstration Forest (51.04794°N, −114.79494°W; NE-17-24-6-W5M; 1,406 m elevation), near Kananaskis, Alberta. We subdivided the site into two subsites: the “Manipulated Treatment Site” where pollen supplementation treatments occurred; and the “Natural Undisturbed Site” where pollinator observations occurred. We divided the site into two subsites to avoid disturbing the equipment needed for pollinator observations. Both subsites were very similar in composition and stand type. Two subspecies were present within the “Manipulated Treatment Site”: R. arcticus spp. arcticus Linnaeus, and R. arcticus spp. acaulis (Michaux) Focke. Because the genetics of the different subspecies have not received adequate study, only R. arcticus spp. arcticus specimens were used for this study. Voucher specimens for R. arcticus spp. arcticus and R. arcticus spp. acaulis were deposited into the University of Calgary’s herbarium.
Generalization at the species level: Pollen supplementation experiment
To assess the effects of pollen limitation on R. arcticus fruit production, a pollen supplementation experiment was used to compare the difference in fruit set between naturally pollinated control flowers and flowers supplemented with outcrossed pollen (Knight et al., 2005). An additional treatment group of bagged flowers supplemented with selfed pollen was included in the experiment to affirm the importance of pollen quality. All control flowers were left undisturbed and open for natural pollination only. After the flowers had fully opened and anthers had begun dehiscing, the pure-selfed flowers were supplemented with their own pollen by having the anthers gently pushed inward toward the stigmas with a Q-tip. Outcrossed supplemented flowers received xenogamous pollen from at least two donor flowers and hand pollination was performed by gently rubbing open dehiscing anthers of collected flowers directly on the receptive stigmas of supplemented flowers. As rhizomes and clonal networks of R. arcticus spp. arcticus have been observed to spread several meters (Ryynänen, 1973), the collected flowers for hand pollination were ≥ 10 m away from the supplemented individuals. There were 30 replicates (i.e., stems) for each of the three treatments and, because R. arcticus spp. arcticus can produce up to three flowers per stem (Ryynänen, 1973), all flowers per each stem received the respective treatment. To prevent herbivory from small rodents and birds, open and outcrossed flowers were also covered with bridal veil bags following the senescence of flowers. Berries were collected in late July to early August. The number of drupelets per berry was counted in the field and a portable scale was used to measure the fresh mass of harvested berries, which included the receptacle.
To examine pollen tube success, the same three treatments described previously (open-pollinated control, pure-selfed, and outcross supplemented) were conducted on three more groups of plants (Chacoff et al., 2008), with each group consisting of at least 10 individual stems. As above, selfed and outcrossed flowers remained covered with bridal veil bags until collection, when treatments were applied upon opening, while control flowers had bags removed immediately following opening. After receiving the respective treatment, all flowers were left undisturbed for at least 72 h and then placed in microtubes filled ethanol-acetic acid (3:1 v/v) for 24 h to fix floral tissues (Kearns and Inouye, 1993).
Pollen staining techniques were then used to compare whether selfed-and outcrossed-supplemented flowers had in fact received more pollen than the control treatments, as well as inspect for stigma damage. Forty-two flowers were collected in total for the pollen tube analysis experiment, with six test flowers (two flowers per treatment) used in the staining trials, and the remaining amount for pollen quantity analysis: 10 controls, 13 selfed and 13 outcrossed flowers. Following Sáez et al. (2014), 10 pistils from each flower were randomly selected for staining and pollen grain counts, making a total of 360 pistils. These pistils were then stained with 1% basic fuchsin, rinsed in distilled water, and then mounted in 50% glycerin (Thomson et al., 1989) on glass slides with coverslips to be viewed under a compound microscope. Both stigmas and all pollen grains (including heterospecific pollen) stained a pinkish to red hue, but R. arcticus grains appeared as bright pink (Supplementary Figure S1). In addition to the brighter hue, R. arcticus grains could also be identified by their tricolporate structure, which is a characteristic shared among members of the genus (Hebda and Chinnappa, 1990), allowing R. arcticus grains to be easily counted under 400X magnification. All stigmas were examined for breakage and classified into a binary system where stigmas were assessed as “completely damaged” (Supplementary Figure S1) or “not fully damaged” if at least some portion of the lobe was left intact.
For the pollen limitation analysis, we determined the difference between mean number of drupelets per berry and mean fresh berry mass for control and outcross supplemented stems (pure selfed stems yielded no fruit) with a two tailed t-test. For the pollen quantity analysis, we used a general linear mixed-effects model (GLMM) using the ‘lme’ function in the ‘nlme’ package (Pinheiro et al., 2017) to assess if there were significant differences in pollen grain counts between the pollination treatment groups, with the response variable as pollen grain count, treatment group and stigma breakage as fixed effects and the flower/plant as a random effect. Pollen grain count was square root transformed, assuming a Gaussian error distribution.
Generalization at the species level: Microclimate influences on pollination
Pollinator surveys for this experiment took place at the “Natural Undisturbed Site” from June 7 to July 10, 2017, using time-lapse photography with five Brinno TLC200 HD time lapse cameras (Brinno, Taipei City, Taiwan). Each camera was mounted in Brinno ATH110 weather resistant housing cases (Brinno, Taipei City, Taiwan) to protect the lenses from moisture as well as dust from the nearby road <25 m from the subsite. Anti-desiccant silica packs were placed inside each housing case to limit any potential fogging or condensation within. The five cameras were placed on flexible octopus tripods with a max height of 25 cm, which allowed for cameras to be placed at an optimal angle as R. arcticus stems can grow up to 15 cm high (Johnson et al., 1995). To capture the maximal pollinator activity, the time lapse cameras recorded from 09:00 to 17:00 (MST) for a total of 8 h each observation day. All cameras were set to record on the default “Better” image quality option to balance file size with resolution and recorded at 10 frames-per-second (FPS) and with an image capture rate of every 3 s.
A random sampling protocol was used for the positioning of these five cameras on different flowering individuals within the site, which was divided into 5 × 5 m grid cells. Any of the sampling grid cells containing open R. arcticus spp. arcticus flowers were randomly selected each day using a random number generator, such that each of the five cameras were in separate grid cells. Flowers were then randomly selected within inside each grid cell. However, during the beginning and end of the flowering season for the subsite, only two to three of the 25 grid cells would contain open flowers and thus, there would be more than one camera per grid cell for some observation days. Cameras were positioned to fit as many open flowers within the field of view (FOV) as possible (ranging from 1 to 6 flowers in the FOV), allowing for better visitation records.
From the 23 observation days, 866.39 useable observation hours were collected (time-lapse footage with a 10 FPS framerate and 3 s capture rate, resulting in ~30 h of actual footage). QuickTime Version 7.7.9 was used to score through the videos on a frame-by-frame basis (Edwards et al., 2015). Each time an insect had landed on a focal flower or probed it, it was considered to be a pollinator. Furthermore, the time of first contact was recorded because the footage was time-stamped with the date/exact time. Since the capture rate of the cameras was every 3 s and an insect could have landed anywhere within that time period, the total number of frames that the insect spent in contact in flower was also recorded as a measure of handling/foraging time. The number of flowers within each camera’s FOV was also documented. Each insect was classified into recognizable taxonomic units (RTUs) or morphospecies based on body size, taxonomy, and obvious morphological features because the resolution of the TLC200 HD cameras was not high enough to permit identification to species. RTU classification allows for rapid identification of large quantities of specimens while simultaneously offering a fair estimate of species richness (Oliver and Beattie, 1993).
Each camera was also paired with an Omega OM-92 temperature/RH data logger (Spectris Canada, Larval, Quebec, Canada) to measure ambient temperature and relative humidity (RH). The internal clock of each data logger was synced each day with Mountain Standard Time prior to recording measurements and was set to record at 1 s intervals so the precise temperature and RH could be applied to the corresponding time of a visiting insect. However, these data loggers exhibited sensitivity to excessive sun exposure. If left in the open sun, these data loggers would give spikes in temperature and RH that were most likely inaccurate. Similar to Karbassioon (2017), we attempted to mitigate these effects by placing the data loggers on wooden sticks 5–15 cm off the ground (depending on the height of the focal flower) with shelter from a white (colour selected for high albedo value) plastic bowl in attempt to reduce surface heat transfer from the soil as well as excessive radiation exposure skewing measurements. The data loggers were placed at least 15 cm away from flowers to allow accurate representation of local temperature/RH and to limit the interference of visiting insects. As an additional measure, we included the presence of spikes in temperature as an effect in the model.
A HoldPeak 866B digital anemometer (Zhuhai Jida Huapu Instrument Co.,Ltd., Zhuhai, China) was used to measure wind speed at each of the five focal flower/camera positions every 30 min, starting at 9:00 and ending at 17:00 MST. As recommended by Kearns and Inouye (1993), the anemometer was held at the same level as the flower and facing the direction of oncoming wind to provide the most accurate data. We used the built-in “average” function of the unit to calculate the mean wind speed during 10 s of recording for our wind speed measurements.
Hemispherical photography was used to measure the irradiance received by the focal flowers using a Canon EOS Rebel SL1 digital SLR camera with a 15-58 mm lens mounted with an Opteka HD2 0.20X professional super AF fisheye adapter. Hemispherical photographs (with a FOV of at least 180° as specified by the manufacturer) were captured at each focal flower/time-lapse camera position for each observation day. At each of the five positions, the camera was positioned with the lens directly facing the canopy vertically and with the top of the camera facing true north, as determined by a compass with the corrected declination for the region as a reference (14° 20.10′ East, Natural Resources Canada 2017) and the camera positioned as close to the focal R. arcticus flowers as possible (Hunter et al., 2013). The slope, aspect, elevation, and geographical position was recorded daily for each of the five focal flower positions, as these were additional input parameters required for the software package to simulate solar radiation exposure. Slope was calculated using a Suunto PM-5/360 PC Clinometer, aspect was collected using a Suunto MC-2/360/D/CM/IN/NH compass, and the exact field coordinates and elevation of focal flower positions was recorded using a Garmin eTrex® 30 GPS navigator. These hemispherical images were either taken in the early morning, late afternoon or during cloudy periods to limit the amount of direct sunlight entering the camera and the pinhole effect (Weiss et al., 2004). To limit the amount of over-exposure from the canopy vegetation, the exposure was adjusted until the “high light alert” warning had disappeared and then images were subsequently captured.
Solar radiation exposure in all the reference hemispherical photographs for each focal flower position was assessed using Gap Light Analyzer (GLA; Frazer et al., 1999). Using a reference hemispherical photograph, GLA computes the total amount of solar radiation received by simulating the sun’s path through the sky regions (Supplementary Figure SA1) and then estimates the amount of light transmitted by creating a binary image threshold in which the sky pixels are white whereas the canopy pixels are black (Supplementary Figure SA1). For the other input parameters, GLA also requires the solar radiation transmitted through the canopy and characterize understory light regimes; see descriptions in the Supplementary Appendix. From each reference hemispherical photo for each focal flower position, “% Canopy Openness” and “Trans Total” (Total transmitted radiation which is the sum of direct and diffuse radiation) were recorded from the output summary. To account for the possibility that the greater light penetration from these gaps results in changes in plant resources and floral density, and consequently influences the foraging behavior of pollinators (Ishii et al., 2008), the number of open flowers in each of the sampling grid cells was recorded for each observation day. Flower counts were performed at the end of the monitoring period (17:00) to account for any flowers that may have opened while pollinator surveys and microclimate monitoring was in progress.
Linear mixed models were constructed using R Version 3.4.3 (R Development Core Team, 2018) to determine if microclimate influences the activity and availability of R. arcticus pollinators. Model selection was performed to identify which predictors influenced the following response variables: handling time and richness. Because the time-lapse cameras had insufficient image quality to permit identification to species level, the total number of RTUs or RTU richness was used a means to characterize the diversity of R. arcticus pollinators (Lortie et al., 2012). RTU richness was assessed on a daily scale since the range of different RTUs on an hourly scale was too small of a response to model. Therefore, the response variable of the pollinator diversity model was the daily RTU richness as measured by each time-lapse camera. Fixed factors for the model included: an interaction between flowers in the field of view for each camera (FFOV) and flower density per sampling grid cell (FDPC), an interaction between daily average temperature of visits (DTOV) and daily average relative humidity of visits (DRHOV), daily average wind speed of visits (DWSOV), presence of daily erroneous data logger readings due to excessive sun exposure (DESE), an interaction between percent canopy openness (%CanOpen) and transmitted total radiation per day (TransTot). The only random effect for this model was the date of the observation day to account for seasonality and temporal oscillation (Karbassioon, 2017). Interactions between FFOV and FDPC are possible as pollinators could be attracted to a local patch or a focal flower. As well, the interaction between temperature and RH could have interactive effects depending on how RH interacts with air temperature and dewpoint temperature (Lawrence, 2005). However, initial models revealed no interactions in the reduced predictor sets and therefore interactions were excluded from further analyses. DESE was included in the model to account for the sun exposure issue of the data loggers and was a binary factor in which “0” corresponded with no inaccurate readings throughout the day and “1” noted there were some inaccurate readings present. The nearby Barrier Lake Research Station’s climate data served as a reference for characterizing this factor. If a data logger had any irregular temperature and RH readings during a given observation day in contrast to the station’s climate data, it was assigned a 1 for DESE for that day. All possible combinations of these fixed effects were run using the ‘MuMIn’ package (Barton, 2020). We calculated the relative Akaike weight of each predictor variable and the average parameter estimate using model averaging of models with ΔAICc <2 (Burnham and Anderson, 2002).
Handling time was simply characterized as the number of frames in which an insect was observed interacting with a flower. Since the capture rate of the cameras was every three seconds and an insect could have entered the FOV anywhere within that period, frame count is a more accurate index of time. Although it is possible that an insect could have entered the FOV, left and subsequently been replaced by another insect that was captured in the frame, the average rate of visitation of insects suggests that the frequency of these events (Supplementary Figure S3) was very low. Because handling time was characterized on such a fine scale, the temporal resolution differed between the fixed variables. For instance, because the data loggers recorded temperature and RH every second during the monitoring period, these measurements correspond to a pollinator’s exact foraging period within the same time scale. On the other hand, wind speed was measured every 30 min and GLA could only compute transmitted solar radiation for an entire day at the minimum. Therefore, these two variables could not be interpolated down to same scale as temperature and RH, operating as very restricted linear predictors of handling time. This model also had pollinators grouped by functional group/clade rather than RTU. To examine how biotic and abiotic predictors influenced handling time over the phenological variation throughout the flowering season (Karbassioon, 2017), model selection was performed with the fixed factors for this model being: FFOV, FDPC, instantaneous temperature of visit (average temperature across all frames an insect was present; ITOV) and instantaneous relative humidity of visit (average RH across all frames an insect was present; IRHOV), hourly average wind speed of visit (HWSOV), presence of instantaneous erroneous data logger readings due to excessive sun exposure (IESE), %CanOpen and TransTot. Lastly, the ‘lsmeans’ package (Lenth, 2016) was used to perform post-hoc pairwise comparisons for the handling times between each clade to confirm whether they were significantly different from one another.
The final sets of models were made with hourly visitation counts as the response variable. Separate visitation models were made for each clade/functional group. However, only the dominant clades (bumblebees, mosquitoes/Culicids, Syrphid flies and other Brachycerans) had their hourly visitation count modelled whereas Lepidopterans/butterflies were excluded because they were very rare/infrequent pollinators. Each model had the exact same equation, with date and the hour of the day as random factors to account for differences in temporal structure (Karbassioon, 2017) across the daily monitoring period and observation days. The fixed factors for each visitation were: an interaction between FFOV and FDPC, an interaction between hourly average temperature of visits (HTOV) and hourly average relative humidity of visits (HRHOV), hourly wind speed of visits (HWSOV), presence of hourly erroneous data logger readings due to excessive sun exposure (HESE), and an interaction between %CanOpen and TransTot. Each model was created using the ‘glmmTMB’ package with a Poisson distribution. Furthermore, each model equation had the “ziformula” parameter set to “~1,” which allows the model to run assuming zero inflation (Brooks et al., 2017). Accounting for zero inflation in this model was necessary as there were several hours in which the visitation count was zero for a given clade. To determine if the number of recording hours was adequate to sufficiently estimate diversity, species accumulation curves were constructed. The ‘specaccum’ function in the ‘vegan’ package (Oksanen et al., 2018) in R Development Core Team (2018) was used to create species accumulation curves with RTU richness as a function of both the total number of recording hours by the time-lapse cameras and the number of individual insect visitation events processed, similar to Edwards et al. (2015), to ascertain whether sampling effort was sufficiently complete.
Results
Generalization in networks: Seed set and specialization in flowering plant communities in Glenbow Ranch Provincial Park
We collected a total of 1,381 fruits belonging to 13 plant species in undisturbed plots, and a total of 2020 fruits belonging to 11 plant species in disturbed plots (Table 1). We counted a total of 24,293 ovules and 17,226 seeds in undisturbed plots and 29,353 ovules and 20,477 fertilized seed in disturbed plots. Despite previous findings of lower specialization in disturbed sites (see Villalobos et al., 2019), we find that, for species that occurred in both types of sites, there are no significant differences in the seed/ovule ratio produced in disturbed versus undisturbed sites (t = 0.9925, df = 3,319, p = 0.32). At the community level, the proportion of the total ovules that developed into seeds in both, undisturbed and disturbed zones was similar with approximately 70% of available ovules fertilized. In the undisturbed sites, the species with the highest seed/ovule proportions were Allium cernuum and Symphoricarpos albus (Table 1). In disturbed sites, the species with highest seed/ovule proportions were Achillea millefolium, Erigeron caespitosus, Gaillardia aristate, and Anemone cylindrica (Table 1). The results of the generalized linear mixed model showed that in disturbed sites, species with low specialization index produced fewer seeds per ovule than those with high specialization index, yet the same was not true for species in undisturbed sites (Table 2; Figure 2). Model selection approaches to examine the effects of dropping the interaction term between disturbance and the specialization index indicated that including the interaction term led to greater explanatory power in the model (ΔAICc >2; Supplementary Table S1).
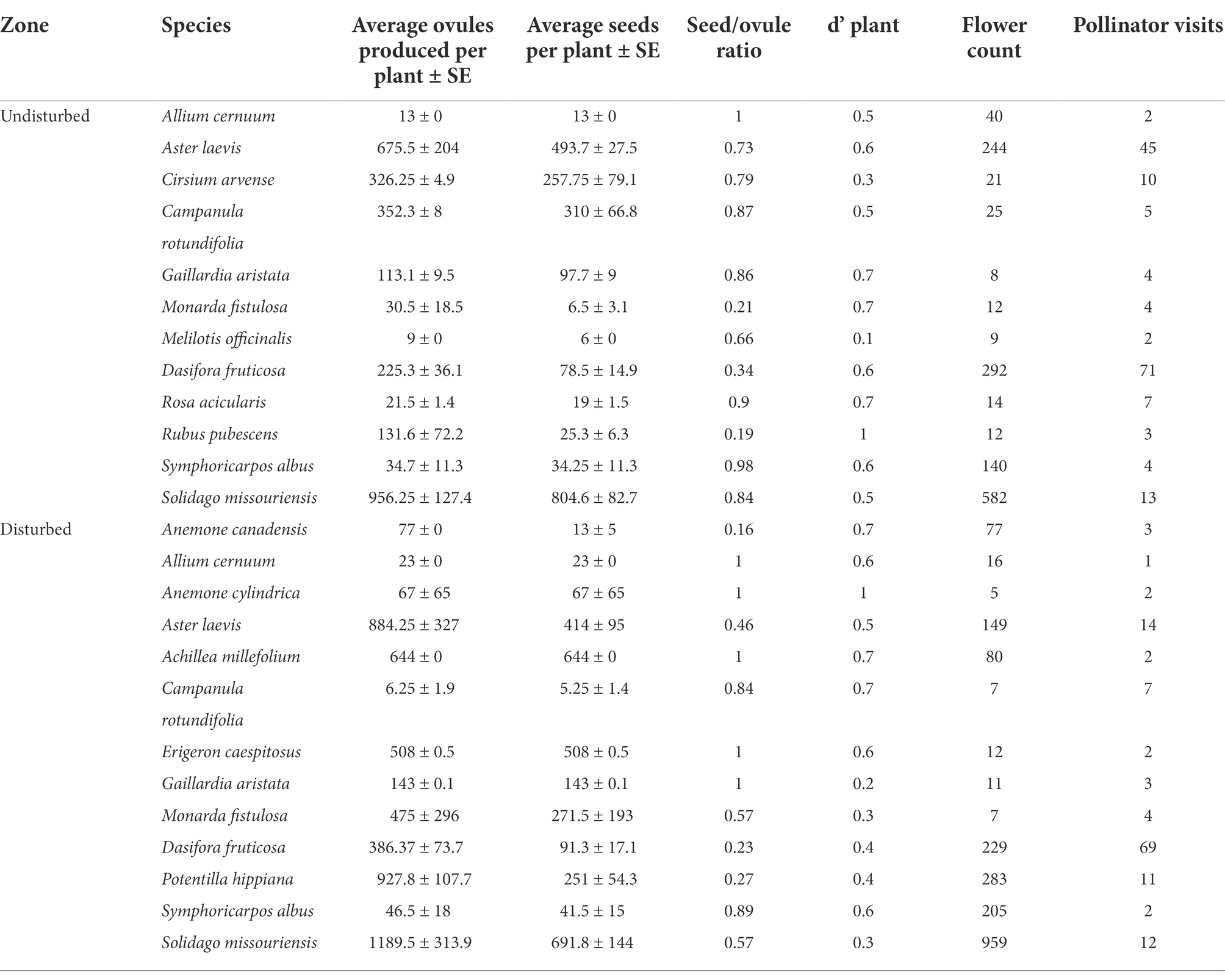
Table 1. Seed set production for 25 species recorded in undisturbed and disturbed zones in Glenbow Ranch Park.
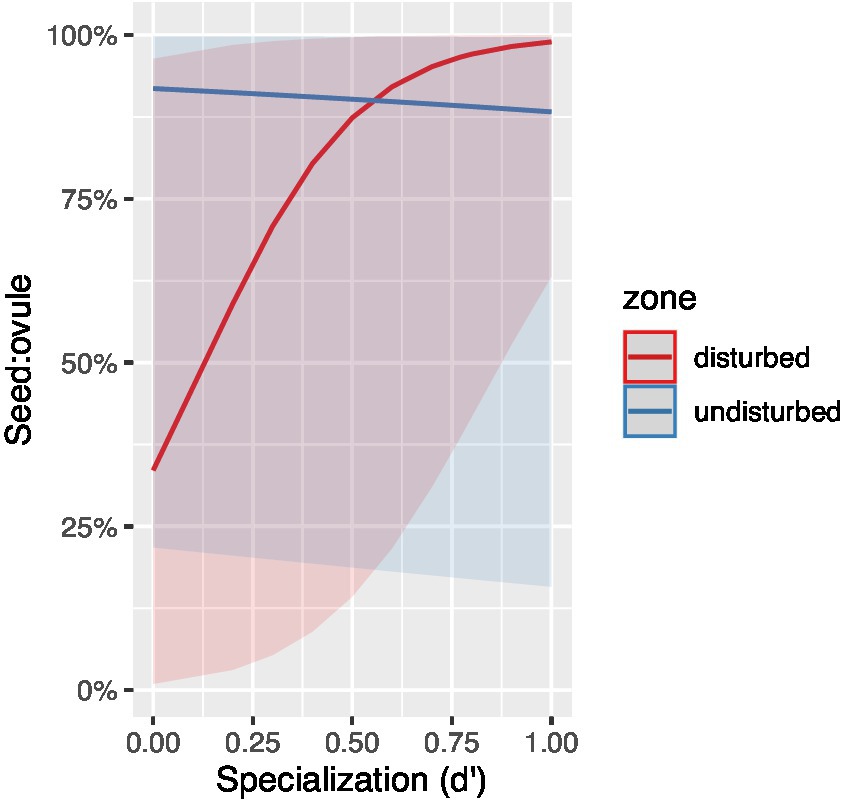
Figure 2. Generalized linear mixed model of the relationship between seeds per ovule and the specialization index (d’) for species found within quadrats in the disturbed and undisturbed zones.
Generalization at the species level: Pollen supplementation experiment
A total of 161 flowers were included among the 90 stems across the three treatments. However, the pollen supplementation experiment only yielded 21 berries in total: 14 from the control replicates, zero from the pure-self replicates and seven from the outcross supplemented replicates. Despite some stems producing up to three flowers, all fruit produced was the result of a single flower per stem and the other remaining flowers completely desiccated. Between the control and outcross supplemented groups, there was little variation in the number of drupelets per berry (Figure 3) with the most common number of drupelets produced being two. Only two berries produced the maximum amount of seven drupelets (see Supplementary Figure S2) and four berries yielded the minimum amount of one drupelet. There was no significant difference between the mean number of drupelets per berry for controls (2.64 drupelets) and outcross supplemented replicates (2.71 drupelets; t = −0.0739, df = 11.315, p = 0.9424). Regarding mean fresh berry mass, there was also little variation between the control and outcross supplemented groups (Supplementary Figure S2). There was also no significant difference between the mean fresh berry mass for controls (0.179 g) and outcross supplemented replicates, (0.171 g; t = 0.15133, df = 9.233, p = 0.883). Our pollen count analysis did reveal that the effect of treatment applied had a weak effect on pollen grain counts (Supplementary Table S2) with control stigmas generally having pollen grains well in excess of the ovule (Supplementary Figure S1), yet the interaction between stigma breakage and treatment suggests that the stigma breakage influenced treatment effects such that stigma breakage could have counteracted the addition of outcross pollen.
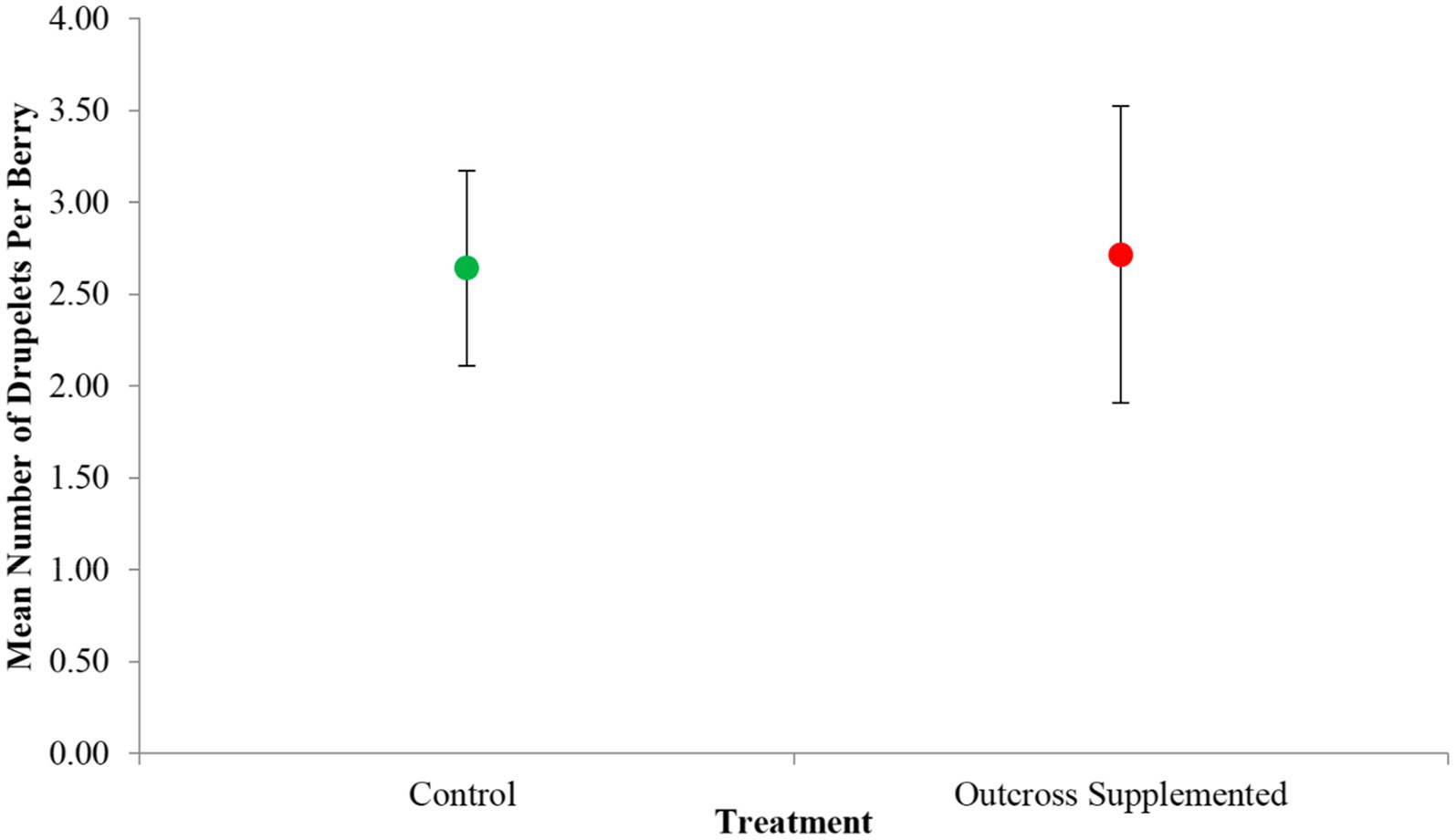
Figure 3. The mean number of drupelets per berry for the control (N = 14) and outcross supplemented (N = 7) treatment groups in the pollination supplementation experiment. Error bars represent standard error.
Generalization at the species level: Microclimate influences on pollination
The field season spanned 23 observation days resulting in 866.39 observation hours and 112 different time-lapse camera/focal flower position iterations within the 625m2 sampling area. Flower density across the entire sampling grid ranged from 1 to 128 flowers and the number of flowers in an individual grid cell ranged from 1 to 44. From the pollinator surveys, the time-lapse cameras captured a total of 1,497 visitation events (Supplementary Figure S3) in which syrphid flies were the most frequent visitor measured as both hourly visitation rate (0.967 visits/h) and total number of visits (844), comprising ~56% of all visits. Other brachyceran flies were the next most frequent pollinator group at ~26%, followed by bumblebees at ~10% and mosquitoes at ~7% of all visits. Clade/functional group of the pollinators was a strong predictor of handling time (Table 3), with bumblebees having the lowest handling time (see Supplementary Figure S4).
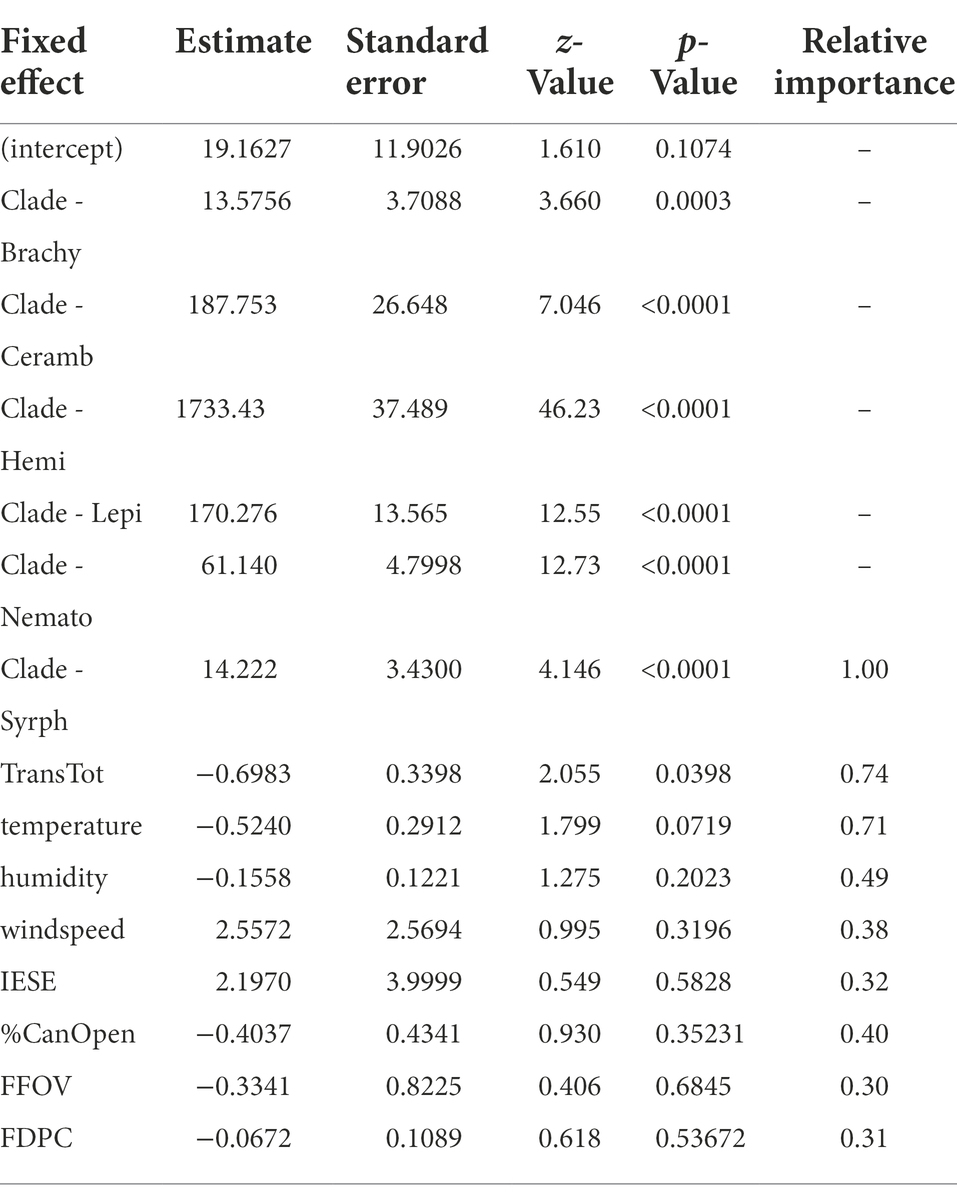
Table 3. Model-averaged values for predictors of handling time. FFOV=Flowers in the camera field of view; FDPC=Flower density per sampling grid cell; IESE = presence (1/0) of erroneous spike in temperature.
Generalization level was positively influenced by FFOV, FDPC and %CanOpen individual as well as the interaction between FFOV and FDPC (Table 4). Of the abiotic predictors, only total radiation (TransTot) and temperature had importance values >0.5 in their effects on handling time (Table 3). Temperature and humidity had similar effects on the visitation rates between syrphid flies and bumblebees (Supplementary Figure S5) although syrphid flies were observed visiting during periods of higher temperature and lower humidity.
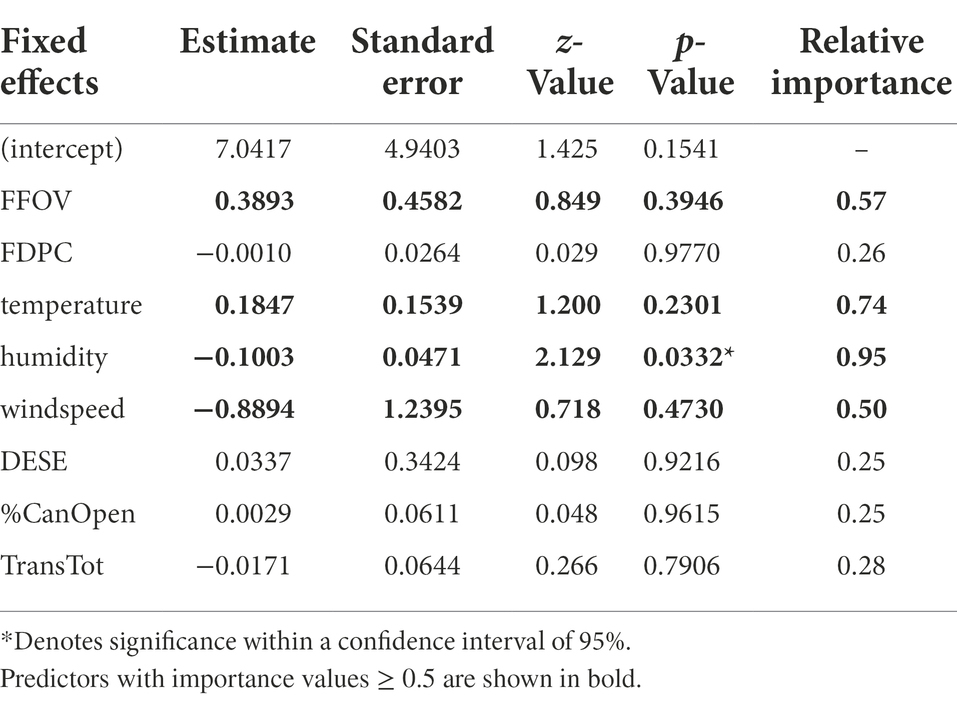
Table 4. Model-averaged values for predictors of daily pollinator diversity/RTU Richness throughout the flowering season.
Syrphid flies had the highest visitation frequency at the start of the flowering season (early June) and their maximal visitation coincided with the peak period in flowering (Figure 4). However, later in the flowering season (late June/early July), the frequency of syrphid fly visits dropped substantially. Finally, species accumulation curves display logarithmic trends (Supplementary Figure S3). There is no asymptote observed for either curve, despite the high number of RTUs recorded.
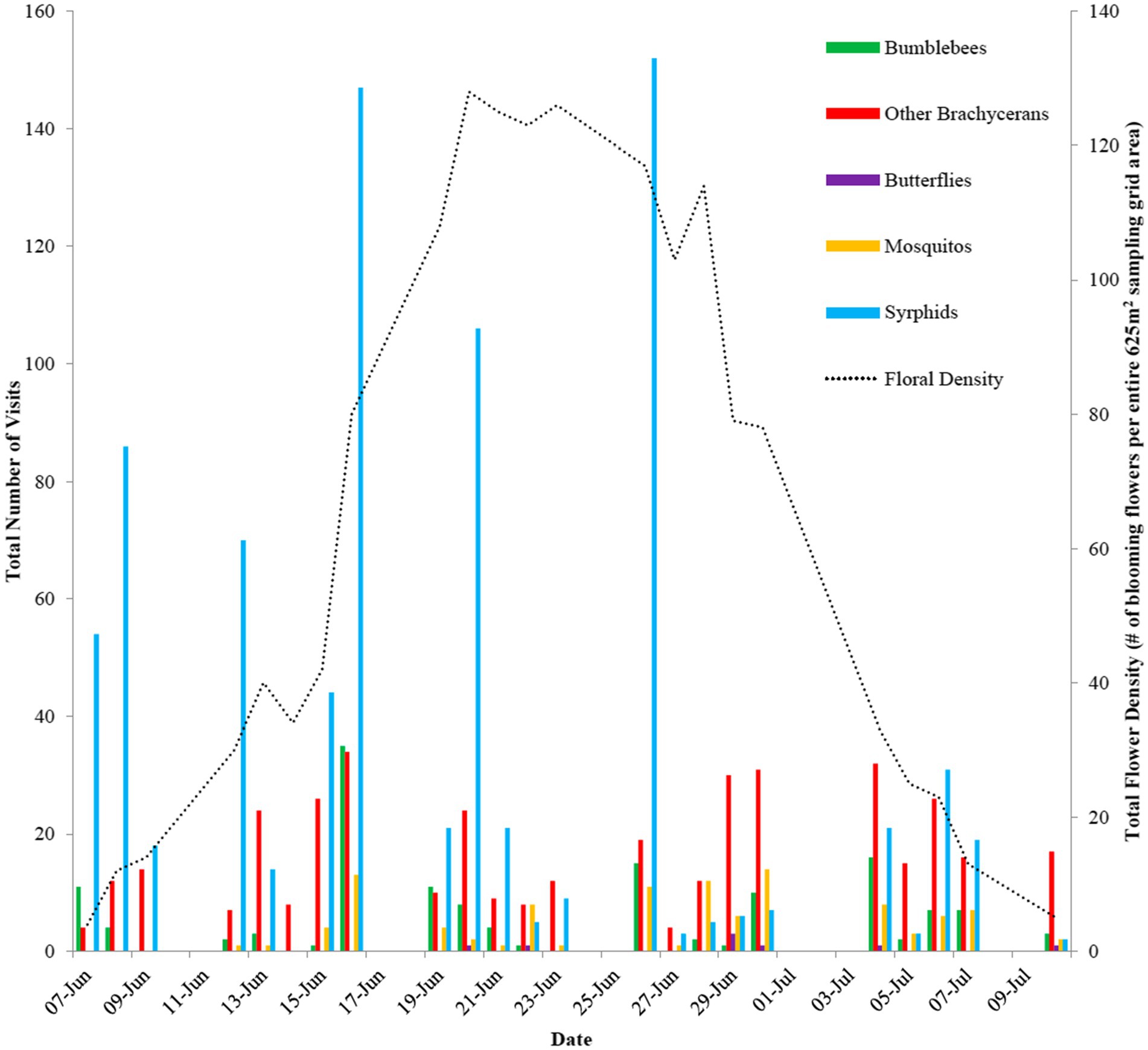
Figure 4. The changes in pollinator visits and flower density within the sampling grid across the entire observation period.
Discussion
Seed production depends in part on pollination services (Rodger et al., 2021), and therefore pollinator decline is a potential threat to plant reproduction. However, how environmental changes result in specific pollinators declining and which specific plant species will be impacted is still largely unknown. Network approaches used here show that: (1) disturbed sites produce more fruits and more seeds, yet the proportion of ovules that develop into seeds produced was equivalent to that in undisturbed sites, and (2) the ability to attract many pollinating species (high generalization) in disturbed sites resulted in decreased fitness as measured through seeds per ovule, a pattern not seen in undisturbed sites. A close examination of a wild-harvested species that has been suspected of experiencing declines in fruit and seed set with the loss of bees, indicates that specialization cannot be simplified to floral visitation observations and that the environment may strongly influence specialization and investment in fruit production. Rubus arcticus was observed here to be an extreme generalist in an undisturbed forest setting. While very few seeds were set, this species exhibited no pollinator limitation, and the low fruit production is more likely due to low genetic diversity and heavy investment in vegetative reproduction.
The loss of the most effective pollinators due to land use changes have been hypothesized to cause low rates of seed production (Memmott et al., 2005) by estimating fitness with visitation rates. We see here that visitation is not a perfect proxy for robustness as plants that receive fewer visiting taxa produce more seeds per ovule. The most fragmented sites in Glenbow Ranch corresponded to areas intensively used for grazing cattle until 2008. The disturbed areas exhibit high levels of erosion and soil compaction due to the grazing activities that have occurred for more than 20 years (see Villalobos et al., 2019). Many species in these areas would be characterized as ruderal, with a disproportionate number of species in Asteraceae, which exhibit dense arrays of flowers arranged in discs inflorescences. The high prevalence of Asteraceae could contribute to the higher counts of seed and ovule number in more eroded zones compared to undisturbed areas where species with solitary flowers were more common (e.g., Campanulaceae). These changes in composition likely contributed to the increase in seed production with disturbance as the results of the GLMM analysis showed that increasing plant specialization increases reproductive success.
Specialization was higher in the sites with lower land use impacts, consistent with previous studies (Classen et al., 2020). Plant-pollinator networks have been previously described as highly generalized and reciprocally redundant (Memmott et al., 2005). From this idea, we might conclude that more generalist species could consistently receive sufficient pollination from their sheer number of diverse visitors, and this should be reflected with consistently high seed set. However, this analysis detected that generalist species experienced high rates of insect visitation, yet fewer of the ovules developed into seeds. Although higher visitor abundance may reflect more chances of pollination, the composition or behavior of the visitors may be of lower quality such that these generalists experience little fitness benefit from additional visitor diversity, similar to that seen in previous studies (Morales and Traveset, 2008).
From simple network metrics, it is difficult to provide insight on pollination quality, such that more detailed studies on the impacts of pollinator visits are needed. Assessing these effects on R. arcticus, we can provide more insights on the effects of pollinator visitation. The results of the pollen supplementation experiment indicate that fruit set of R. arcticus is not pollinator limited as there was no significant difference between the control and outcrossed supplemented treatments in terms of mean number of drupelets per berry. Observations of pollen quantity further revealed that control stigmas often had an abundance of conspecific grains present, well above the number of ovules. Therefore, taken together these results suggest that naturally pollinated flowers of R. arcticus experience sufficient quantities of pollen delivered by the diversity of insects seen visiting. However, it is worth noting how easily the stigma experiences breakage, which may occur with inefficient pollinators with long handling times. The cause of the small berry yield may also have been the result of selection for asexual reproduction in this understory herb, as the maximum daily floral density for the entire subsite was 128 flowers, and only 11 berries (each from separate individual stems) were produced by the entire patch. This population thus represents an example where seed set is low, yet this low reproduction is not due to a lack of pollinators. The low number of drupelets observed at our site may suggest that self-pollen was being delivered between members of the same clone, or that fruit development was impeded due to heat stress (Graham et al., 2015).
We find that R. arcticus receives numerous visits from many functional groups of pollinators. Further R. arcticus also received visits from pollinators in a wide variety of climatic conditions. The relative differences in handling times between each functional group have been noted in earlier studies (Herrera, 1989), which further reinforces the idea that intrinsic differences within taxa is more influential than the direct effects of microclimate on foraging duration. In this study, syrphid fly handling times were 10X greater than that of bumblebees, butterfly handling times were 105X greater than that of bumblebees. There was support for the hypothesis that microclimate does influence the diversity, foraging time, and visitation frequency of R. arcticus pollinators. Firstly, humidity had a significant effect on pollinator handling times, while total radiation affected RTU richness. Further, the hourly visitation of the most frequent visitors, syrphid flies, tended to occur at higher temperatures and lower humidity compared to bumblebees. Considering the high pollen counts on intact stigmas, it seems unlikely that R. arcticus is a species with reproduction limited by pollinator visits. Determining whether some visitors could be responsible more than others for stigma breakage or visiting during times of heat stress, will require further study, and yet it seems clear that other factors play a greater role than lack of pollinators in causing fruiting failure in this species.
Syrphid flies being the dominant visitor of R. arcticus flowers for this population is a novel finding as previous studies found either honeybees or bumblebees comprising the majority of floral visits (Ryynänen, 1973; Tammisola, 1988; Kangasjärvi and Oksanen, 1989). This finding further supports previous studies finding increased prevalence of syrphid flies and reduced activity of bees in alpine/montane communities (Larson et al., 2001; McCabe and Cobb, 2021). Although bumblebees were far less frequent visitors in this study, they were likely efficient and higher quality pollinators. For instance, Vool et al. (2003) contends that syrphid flies are lower quality pollinators of R. arcticus because they do not weigh enough to successfully navigate past the densely packed anthers. As the pollen experiment showed, open pollinated flowers were rarely damaged compared to manipulated flowers. Nevertheless, many pistils could be seen not developing into drupelets, making for smaller and fewer berries throughout the patch that was not caused by a lack of pollinators. While it is unlikely that syrphids or bumblebees greatly damage the stigmas of R. arcticus, the effects of heat stress, drought, and low genetic diversity may represent threats to the long-term persistence of Arctic raspberry in extreme environments. Thus, while generalist pollinators could sometimes provide a safety net to pollination success for some species, any contribution they make is dependent on conditions being inhospitable to more specialized pollinators. It has been suggested by R. arcticus is pollinator limited in other parts of its range (Ladyman, 2006) due to low visitation by bees (Tammisola, 1988). Our data here suggest that the more specialized pollinating functional group (here, bumblebees) is robust to the microclimate conditions seen in this particular year and setting.
While the floral form of R. arcticus is suggestive of diffuse coevolution towards a generalist floral shape (Figure 1), this study indicates that selection to maintain a generalist floral shape to accommodate a wide variety of pollinators is likely weak and that selection towards increasing vegetation reproduction has been stronger and will likely be the main force buffering this species from declines in habitat quality. Producing seed (and fruit) by attracting a wide variety of pollinators may rarely act as a safeguard against extinction in flowering plants. The differences in strategies between the two communities examined here highlight the differences observed in investment in sexual reproduction between open grasslands and mature forests. Our examination of seeds produced in grasslands using network approaches provide insight into visitors that can or will gain access to a given flower in a community, and more detailed studies reveal how extensive generalization can be throughout the flowering season of some plant species. In areas with low disturbance, the environment may be benign enough that efficient and less efficient pollinators are present, yet investment in clonal reproduction prevails. In more open and disturbed habitat, reproduction through seeds is more important (Kanno and Seiwa, 2004), and it is in these settings where pollen limitation is highest (Bennett et al., 2020) and selection pressures for coevolution between plant-pollinator specialization will be most commonly observed.
Data availability statement
The raw data supporting the conclusions of this article will be made available by the authors, without undue reservation.
Author contributions
CB and SV were responsible for data collection and analysis as well as manuscript preparation. JV was responsible for manuscript oversight, writing, and editing, as well as study design. All authors contributed to the article and approved the submitted version.
Funding
This study was funded by an NSERC Discovery Grant 2019-04141 to JV, an NSERC Queen Elizabeth II Master’s Scholarship, Delia Gray Memorial Métis Scholarship, Alberta Indigenous Graduate Award, BluEarth ACES Award, and Indspire Post-Secondary Education Scholarship to CB.
Acknowledgments
We thank R. Cartar and S. Robinson for help with statistics and study design, R. Longair for help with insect identification, N. D’Souza and B. Ahmad for fieldwork assistance and two reviewers for their helpful suggestions.
Conflict of interest
The authors declare that the research was conducted in the absence of any commercial or financial relationships that could be construed as a potential conflict of interest.
Publisher’s note
All claims expressed in this article are solely those of the authors and do not necessarily represent those of their affiliated organizations, or those of the publisher, the editors and the reviewers. Any product that may be evaluated in this article, or claim that may be made by its manufacturer, is not guaranteed or endorsed by the publisher.
Supplementary material
The Supplementary material for this article can be found online at: https://www.frontiersin.org/articles/10.3389/fevo.2022.1012809/full#supplementary-material
References
Abeli, T., Vamosi, J. C., and Orsenigo, S. (2018). The importance of marginal population hotspots of cold-adapted species for research on climate change and conservation. J. Biogeogr. 45, 977–985. doi: 10.1111/jbi.13196
Albrecht, M., Schmid, B., Hautier, Y., and Müller, C. B. (2012). Diverse pollinator communities enhance plant reproductive success. Proc. R. Soc. B Biol. Sci. 279, 4845–4852. doi: 10.1098/rspb.2012.1621
Alice, L., Goldman, D., Macklin, J., and Moore, G. (1993). Rubus arcticus Linnaeus in FNA New York and Oxford: Flora of North America North of Mexico.
Armbruster, W. S. (2017). The specialization continuum in pollination systems: diversity of concepts and implications for ecology, evolution and conservation. Funct. Ecol. 31, 88–100. doi: 10.1111/1365-2435.12783
Barker, D. A., and Arceo-Gomez, G. (2021). Pollen transport networks reveal highly diverse and temporally stable plant–pollinator interactions in an Appalachian floral community. AoB PLANTS 13. doi: 10.1093/aobpla/plab062
Bates, D., Mächler, M., Bolker, B., and Walker, S. (2015). Fitting linear mixed-effects models using lme4. J. Stat. Softw. 67, 1–48. doi: 10.18637/jss.v067.i01
Bennett, J. M., Steets, J. A., Burns, J. H., Burkle, L. A., Vamosi, J. C., and Wolowski, M. (2020). Land use and pollinator dependency drives global patterns of pollen limitation in the Anthropocene. Nat. Commun. 11:3999. doi: 10.1038/s41467-020-17751-y
Bennett, J. M., Steets, J. A., Burns, J. H., Durka, W., Vamosi, J. C., Arceo-Gómez, G., et al. (2018). GloPL, a global data base on pollen limitation of plant reproduction. Sci. Data 5:180249. doi: 10.1038/sdata.2018.249
Biesmeijer, J. C., Roberts, S. P., Reemer, M., Ohlemüller, R., Edwards, M., Peeters, T., et al. (2006). Parallel declines in pollinators and insect-pollinated plants in Britain and the Netherlands. Science 313, 351–354. doi: 10.1126/science.1127863
Brooks, M. E., Kristensen, K., van Benthem, K. J., Magnusson, A., Berg, C. W., Nielsen, A., et al. (2017). glmmTMB balances speed and flexibility among packages for Zero-inflated Generalized Linear Mixed Modeling. The R Journal 9, 378–400. doi: 10.32614/RJ-2017-066
Burnham, K. P., and Anderson, D. L. (2002). Model Selection and Multimodel Inference: A Practical-theoretic Approach. Berlin: Springer-Verlag.
Chacoff, N., García, D., and Obeso, J. R. (2008). Effects of pollen quality and quantity on pollen limitation in Crataegus monogyna (Rosaceae) in NW Spain. Flora 203, 499–507. doi: 10.1016/j.flora.2007.08.005
Chang, J. J., Crall, J. D., and Combes, S. A. (2016). Wind alters landing dynamics in bumblebees. J. Exp. Biol. 219, 2819–2822. doi: 10.1242/jeb.137976
Classen, A., Eardley, C. D., Hemp, A., Peters, M. K., Peters, R. S., Ssymank, A., et al. (2020). Specialization of plant–pollinator interactions increases with temperature at Mt Kilimanjaro. Ecol. Evol. 10, 2182–2195. doi: 10.1002/ece3.6056
Colles, A., Liow, L. H., and Prinzing, A. (2009). Are specialists at risk under environmental change? Neoecological, paleoecological and phylogenetic approaches. Ecol. Lett. 12, 849–863. doi: 10.1111/j.1461-0248.2009.01336.x
Corbet, S. A., Fussell, M., Ake, R., Fraser, A., Gunson, C., Savage, A., et al. (1993). Temperature and the pollinating activity of social bees. Ecol. Entomol. 18, 17–30. doi: 10.1111/j.1365-2311.1993.tb01075.x
Corbet, S. A., Unwin, D. M., and Prys-Jone, O. E. (1979). Humidity, nectar and insect visits to flowers, with special reference to Crataegus. Tilia and Echium. Ecological Entomology 4, 9–22. doi: 10.1111/j.1365-2311.1979.tb00557.x
Davila, Y. C., Elle, E., Vamosi, J. C., Hermanutz, L., Kerr, J. T., Lortie, C. J., et al. (2012). Ecosystem services of pollinator diversity: a review of the relationship with pollen limitation of plant reproduction. Botany 90, 535–543. doi: 10.1139/b2012-017
Edwards, J., Smith, G. P., and McEntee, M. H. F. (2015). Long-term time-lapse video provides near complete records of floral visitation. J. Pollinat. Ecol. 16, 91–100. doi: 10.26786/1920-7603(2015)16
Fenster, C. B., Armbruster, W. S., Wilson, P., Thomson, J. D., and Dudash, M. R. (2004). Pollination syndromes and floral specialization. Ann. Rev. Ecol. Evol. Syst. 35, 375–403. doi: 10.1146/annurev.ecolsys.34.011802.132347
Frazer, G., Canham, C., and Lertzman, K. (1999). Gap Light Analyzer (GLA): Imaging Software to Extract Canopy Structure and Gap Light Transmission Indices from True-colour sheye Photographs, users Manual and Program Documentation. Burnaby: Simon Fraser University.
Graham, J., Smith, K., McCallum, S., Hedley, P. E., Cullen, D. W., Dolan, A., et al. (2015). Towards an understanding of the control of 'crumbly' fruit in red raspberry. Springerplus 4:223. doi: 10.1186/s40064-015-1010-y
Hebda, R. J., and Chinnappa, C. C. (1990). Studies on pollen morphology of Rosaceae in Canada. Rev. Palaeobot. Palynol. 64, 103–108. doi: 10.1016/0034-6667(90)90123-Z
Herrera, C. M. (1989). Pollinator abundance, morphology, and flower visitation rate: analysis of the “quantity” component in a plant-pollinator system. Oecologia 80, 241–248. doi: 10.1007/BF00380158
Herrera, C. M. (1995). Microclimate and individual variation in Pollinators: flowering plants are more than their flowers. Ecology 76, 1516–1524. doi: 10.2307/1938153
Hoehn, P., Tscharntke, T., Tylianakis, J. M., and Steffan-Dewenter, I. (2008). Functional group diversity of bee pollinators increases crop yield. Proc. R. Soc. B Biol. Sci. 275, 2283–2291. doi: 10.1098/rspb.2008.0405
Høye, T. T., Post, E., Schmidt, N. M., Trøjelsgaard, K., and Forchhammer, M. C. (2013). Shorter flowering seasons and declining abundance of flower visitors in a warmer Arctic. Nat. Clim. Chang. 3, 759–763. doi: 10.1038/nclimate1909
Hunter, K., Zorer, M., Strever, A., and Hunter, J. J. (2013). Hourly simulation of grape bunch light microclimate using hemispherical photography. Ciência e Técnica Vitivinícola 28, 1031–1034.
Ishii, H. S., Hirabayashi, Y., and Kudo, G. (2008). Combined effects of inflorescence architecture, display size, plant density and empty flowers on bumble bee behaviour: experimental study with artificial inflorescences. Oecologia 156, 341–350. doi: 10.1007/s00442-008-0991-4
Johnson, D., Kershaw, L., MacKinnon, A., and Pojar, J. (1995). Plants of the Western Boreal Forest and Aspen Parkland. Lone Pine Publishing and the Canadian Forest Service: Edmonton, Alberta, Canada.
Kangasjärvi, J., and Oksanen, J. (1989). Pollinator behaviour in cultivated and wild Arctic bramble (Rubus arcticus L.). Agric. Food Sci. 61, 33–38. doi: 10.23986/afsci.72349
Kanno, H., and Seiwa, K. (2004). Sexual vs. vegetative reproduction in relation to Forest dynamics in the Understorey shrub, Hydrangea paniculata (Saxifragaceae). Plant Ecol. 170, 43–53. doi: 10.1023/B:VEGE.0000019027.88318.54
Karbassioon, A. (2017). Pollinator activity along an environmental gradient. MSc thesis University of Oslo.
Kearns, C. A., and Inouye, D. W. (1993). Techniques for Pollination Biologists. Niwot, Colorado, USA: University Press of Colorado.
Kerr, J. T., Pindar, A., Galpern, P., Packer, L., Potts, S. G., Roberts, S. M., et al. (2015). Climate change impacts on bumblebees converge across continents. Science 349, 177–180. doi: 10.1126/science.aaa7031
Knight, T. M., Steets, J. A., Vamosi, J. C., Mazer, S. J., Burd, M., Campbell, D. R., et al. (2005). Pollen limitation of plant reproduction: pattern and process. Ann. Rev. Ecol. Evol. Syst. 36, 467–497. doi: 10.1146/annurev.ecolsys.36.102403.115320
Ladyman, J. A. R. (2006). Rubus arcticus L. ssp. acaulis (Michaux) Focke (dwarf raspberry): a technical conservation assessment. USDA Forest Service, Rocky Mountain region Available at: https://www.fs.usda.gov/detail/r2/landmanagement/?cid=stelprdb5206999
Larson, B. M. H., Kevan, P. G., and Inouye, D. W. (2001). Flies and flowers: taxonomic diversity of anthophiles and pollinators. Can. Entomol. 133, 439–465. doi: 10.4039/Ent133439-4
Lawrence, M. G. (2005). The relationship between relative humidity and the Dewpoint temperature in moist air: a simple conversion and applications. Bull. Am. Meteorol. Soc. 86, 225–234. doi: 10.1175/BAMS-86-2-225
Lenth, R. V. (2016). Least-squares means: the R package lsmeans. J. Stat. Softw. 69, 1–33. doi: 10.18637/jss.v069.i01
Lewthwaite, J. M. M., Angert, A. L., Kembel, S. W., Goring, S. J., Davies, T. J., Mooers, A. Ø., et al. (2018). Canadian butterfly climate debt is significant and correlated with range size. Ecography 41, 2005–2015. doi: 10.1111/ecog.03534
Lortie, C. J., Budden, A., and Reid, A. (2012). From birds to bees: applying video observation techniques to invertebrate pollinators. J. Poll. Ecol. 6. doi: 10.26786/1920-7603(2011)20
McCabe, L. M., and Cobb, N. S. (2021). From bees to flies: global shift in pollinator communities along elevation gradients. Front. Ecol. Evol. 8:626124. doi: 10.3389/fevo.2020.626124
Memmott, J., Waser, N., and Price, M. (2005). Tolerance of pollinator networks to species extinctions. Proc. Biol. Sci. R. Soc. 271, 2605–2611. doi: 10.1098/rspb.2004.2909
Morales, C. L., and Traveset, A. (2008). Interspecific pollen transfer: magnitude, prevalence, and consequences for plant fitness. Crit. Rev. Plant Sci. 27, 221–238. doi: 10.1080/07352680802205631
Oksanen, J., Blanchet, F. G., Friendly, M., Kindt, R., Legendre, P., McGlinn, D., et al. (2018). Vegan: community ecology package. R Package Version 2, 4–6.
Oliver, I., and Beattie, A. J. (1993). A possible method for the rapid assessment of biodiversity. Conserv. Biol. 7, 562–568. doi: 10.1046/j.1523-1739.1993.07030562.x
Ollerton, J., Winfree, R., and Tarrant, S. (2011). How many flowering plants are pollinated by animals? Oikos 120, 321–326. doi: 10.1111/j.1600-0706.2010.18644.x
Parmesan, C., Ryrholm, N., Stefanescu, C., Hill, J. K., Thomas, C. D., Descimon, H., et al. (1999). Poleward shifts in geographical ranges of butterfly species associated with regional warming. Nature 399, 579–583. doi: 10.1038/21181
Pinheiro, J., Bates, D., DebRoy, S., and Sarkar, D., Core Team R. (2017). Linear and Non linear Effects Models Manhattan: New Prairie Press.
Potts, S. G., Biesmeijer, J. C., Kremen, C., Neumann, P., Schweiger, O., and Kunin, W. E. (2010). Global pollinator declines: trends, impacts and drivers. Trends Ecol. Evol. 25, 345–353. doi: 10.1016/j.tree.2010.01.007
R Development Core Team. (2018). R: A Language and Environment for Statistical Computing. Vienna: R Foundation for Statistical Computing.
Rodger, J. G., Bennett, J. M., Razanajatovo, M., Knight, T. M., Mv, K., Ashman, T.-L., et al. (2021). Widespread vulnerability of flowering plant seed production to pollinator declines. Science. Science Advances 7:eabd3524. doi: 10.1126/sciadv.abd3524
Sáez, A., Morales, C. L., Ramos, L. Y., and Aizen, M. A. (2014). Extremely frequent bee visits increase pollen deposition but reduce drupelet set in raspberry. J. Appl. Ecol. 51, 1603–1612. doi: 10.1111/1365-2664.12325
Sonne, J., Maruyama, P. K., Martín González, A. M., Rahbek, C., Bascompte, J., and Dalsgaard, B. (2022). Extinction, coextinction and colonization dynamics in plant–hummingbird networks under climate change. Nat. Ecol. Evol. 6, 720–729. doi: 10.1038/s41559-022-01693-3
Tammisola, J. (1988). Incompatibility classes and fruit set in natural populations of arctic bramble (Rubus arcticus L.) in Finland. Agric. Food Sci. 60, 327–446. doi: 10.23986/afsci.72299
Tammisola, J., and Ryynanen, A. (1970). Incompatibility in Rubus arcticus L. Hereditas 66, 269–277. doi: 10.1111/j.1601-5223.1970.tb02350.x
Thomson, J. D., McKenna, M. A., and Cruzan, M. B. (1989). Temporal patterns of nectar and pollen production in Aralia hispida: implication for reproductive success. Ecology 70, 1061–1068. doi: 10.2307/1941375
Tiusanen, M., Hebert, P. D. N., Schmidt, N. M., and Roslin, T. (2016). One fly to rule them all; muscid flies are the key pollinators in the Arctic. Proc. R. Soc. B Biol. Sci. 283:20161271. doi: 10.1098/rspb.2016.1271
Unwin, D. M., and Corbet, S. A. (1991). Insects, Plants and Microclimate. Slough, UK: Richmond Publishing.
Vanbergen, A. J., Initiative Insect Pollinators (2013). Threats to an ecosystem service: pressures on pollinators. Front. Ecol. Environ. 11, 251–259. doi: 10.1890/120126
Villalobos, S., Sevenello-Montagner, J. M., and Vamosi, J. C. (2019). Specialization in plant–pollinator networks: insights from local-scale interactions in Glenbow ranch Provincial Park in Alberta. Canada. BMC Ecol. 19:34. doi: 10.1186/s12898-019-0250-z
Vool, E., Noormets, M., Karp, K., Mand, R., and Starast, M. (2003). Pollinators and their foraging behavior in arctic bramble (Rubus arcticus L.) plantations in Estonia. Integ. Approach. Baltic Sea Reg. 15, 481–489.
Walters, B. B., and Stiles, E. W. (1996). Effect of canopy gaps and flower patch size on pollinator visitation of Impatiens capensis. Bull. Torrey. Bot. Club 123, 184–188. doi: 10.2307/2996793
Weiss, M., Frederic, B., Smith, G. J., Jonckheere, I., and Coppin, P. (2004). Review of methods for in situ leaf area index (LAI) determination: part II. Estimation of LAI, errors and sampling. Agric. For. Meteorol. 121, 37–53. doi: 10.1016/j.agrformet.2003.08.001
Keywords: pollination, coevolution, generalized pollination, pollen limitation, seed set
Citation: Burns C, Villalobos S and Vamosi JC (2022) When less is more: Visitation by generalist pollinators can have neutral or negative effects on plant reproduction. Front. Ecol. Evol. 10:1012809. doi: 10.3389/fevo.2022.1012809
Edited by:
Vera Tai, Western University, CanadaReviewed by:
Kelly Ksiazek-Mikenas, Elmhurst College, United StatesAlyssa Stewart, Mahidol University, Thailand
Copyright © 2022 Burns, Villalobos and Vamosi. This is an open-access article distributed under the terms of the Creative Commons Attribution License (CC BY). The use, distribution or reproduction in other forums is permitted, provided the original author(s) and the copyright owner(s) are credited and that the original publication in this journal is cited, in accordance with accepted academic practice. No use, distribution or reproduction is permitted which does not comply with these terms.
*Correspondence: Jana C. Vamosi, anZhbW9zaUB1Y2FsZ2FyeS5jYQ==
†These authors have contributed equally to this work