- 1Institute of Zoology, University of Regensburg, Regensburg, Germany
- 2Department of Chemistry, Kenyon College, Gambier, OH, United States
The use of sex pheromones for the attraction of potential mating partners is widespread in insects. Species-specificity of these chemical signals is essential, particularly in closely related species with overlapping habitats. In parasitoid wasps of the genus Nasonia, it is the males that produce sex pheromones in their rectal vesicles. The genus consists of four species: N. vitripennis (Nv), N. giraulti (Ng), N. oneida (No), and N. longicornis (Nl). The cosmopolitan species Nv is sympatric with Ng and No in eastern North America and with Nl in the west. Interspecific courtship is common in Nasonia although hybridization is prevented in most combinations by Wolbachia-mediated cytoplasmic incompatibility. The pheromone of Nv males differs from all Nasonia congeners by an additional component that is believed to ensure precopulatory isolation from the sympatric congeners. Detailed investigations on these interactions, however, exist only for the species combination Nv/Ng. Here, we report the results of chemical and behavioral investigations on the sex pheromone of Nl males. The pheromone consists of (4R,5S)-5-hydroxy-4-decanolide (RS) and 4-methylquinazoline (MQ) as a minor component which are produced only after eclosion. Pheromone titers peaked 2 days after eclosion and remained constant on day three. The pheromone is deposited by abdomen dipping which males increasingly exhibited after mating or brief contact with a female. The presence of hosts containing females about to emerge did not increase marking behavior. Site fidelity of males at their own pheromone markings is mediated by MQ. Both natural and synthetic pheromone attracted virgin but not mated females and both RS and MQ are required for female attraction. Females collected during emergence from the host responded likewise to the pheromone and 84% of them produced mixed-sex offspring showing that most of them emerge unmated. Nl females preferred pheromone extract from conspecific males to extract from Nv males, and the addition of the Nv-specific component (4R,5R)-5-hydroxy-4-decanolide (RR) to the Nl pheromone made them unresponsive. The present study demonstrates that Nl uses the male sex pheromone in a similar way as previously shown for Nv and Ng. Furthermore, it shows that Nl females use the Nv-specific pheromone component RR to avoid costly sexual interactions with Nv males in regions of sympatry.
Introduction
In most insects, the mate finding process is mediated by volatile sex pheromones, chemical signals that are released by one sex to attract the other (Wyatt, 2014). To guide the signal recipient effectively and reliably to the sender, sex pheromones need to be species-specific. Being species-specific, sex pheromones provide long-range precopulatory isolation even in sympatric species with overlapping mate recognition signals (Smadja and Butlin, 2009).
An excellent model system to study such processes is the parasitic wasp genus Nasonia (Ashmead, 1904). During the past decades, the genus has been frequently used to answer questions concerning all aspects of parasitic wasp biology (Werren and Loehlin, 2009; Werren et al., 2010; Lynch, 2015; Mair and Ruther, 2019; Wang et al., 2020). It consists of four species: N. vitripennis (Nv), N. giraulti (Ng), N. oneida (No) and N. longicornis (Nl; Darling and Werren, 1990; Raychoudhury et al., 2010; Werren et al., 2010). All species parasitize the pupae of various cyclorraphous fly species. The typical habitat of Nasonia wasps is in the nests of cavity-nesting birds. Nv is cosmopolitan and sympatric with Ng and No in eastern North America and with Nl in the west. In areas of sympatry, Nasonia species occur often microsympatrically, i.e., heterospecific offspring develop within the same host individuals (Darling and Werren, 1990; Grillenberger et al., 2009; Raychoudhury et al., 2010). Hence, interspecific interactions between males and females of sympatric species are likely to occur. Interspecific mating in Nasonia occurs in the lab with varying frequency but does not lead to hybrid offspring in most combinations of species due to Wolbachia-mediated cytoplasmic incompatibility (Breeuwer and Werren, 1990; Bordenstein et al., 2001; Giesbers et al., 2013). Hence, fertilized eggs of females that mated with a heterospecific male die or develop into male offspring due to the haplodiploid sex determination in Hymenoptera (Tram et al., 2006). The resulting all-son-offspring impose evolutionary costs on the respective females because the mating system of Nasonia is characterized by local mate competition and female-biased offspring sex ratios are common (Werren, 1983; Grillenberger et al., 2009; Ivens et al., 2009). Given that Nasonia females typically mate only once in their life, they depend on reliable information to reduce the probability of interspecific mating.
Males of all Nasonia species produce sex pheromones in their rectal vesicle (Abdel-Latief et al., 2008). Three pheromone components have been identified in Nasonia males: the two diastereomers (4R,5S)- and (4R,5R)-5-hydroxy-4-decanolide (RS and RR; Ruther et al., 2007) and 4-methylquinazoline (MQ) as a minor component (Ruther et al., 2008; Figure 1A). RS and MQ are produced by males of all Nasonia species as well as by males of the closely related species Trichomalopsis sarcophagae, whereas RR is specific for Nv. This suggests that the ancestral sex pheromone of Nasonia males consisted solely of RS and MQ and that the additional occurrence of RR is a derived state that evolved in the N. vitripennis lineage (Niehuis et al., 2013). RR has been shown to be synthesized from RS by short-chain dehydrogenases/reductases (SDRs; Niehuis et al., 2013; Ruther et al., 2016). The chemical identities of the pheromones have been established in all Nasonia species, but detailed analyses of the biological function are so far available only for the model organism Nv and, to a lesser extent, for Ng (Ruther et al., 2014; Mair and Ruther, 2019). Nv males eclose without pheromone, but pheromone titers increase within the first 2 days after eclosion and remain constant on day three (Ruther et al., 2007). Males deposit pheromone by dragging their abdominal tips over substrates, a behavior referred to as ‘abdomen dipping’ (Barrass, 1969; van den Assem et al., 1980). Nv males economize pheromone deposition by engaging in abdomen dipping more frequently after contact or mating with virgin females (Steiner and Ruther, 2009), i.e., if the chance of mating with (additional) females is high. The mating system of Nv is characterized by territorial males that monopolize parasitized hosts containing females about to emerge. Apart from showing aggressiveness towards male competitors (Leonard and Boake, 2006), dominant males often mark monopolized hosts to prevent emerging females from dispersing unmated (Mair and Ruther, 2018). Nv males are able to identify intact hosts (without exit holes) containing females about to emerge (Prazapati et al., 2022). It is unknown, however, whether the presence of those hosts also affects male marking.
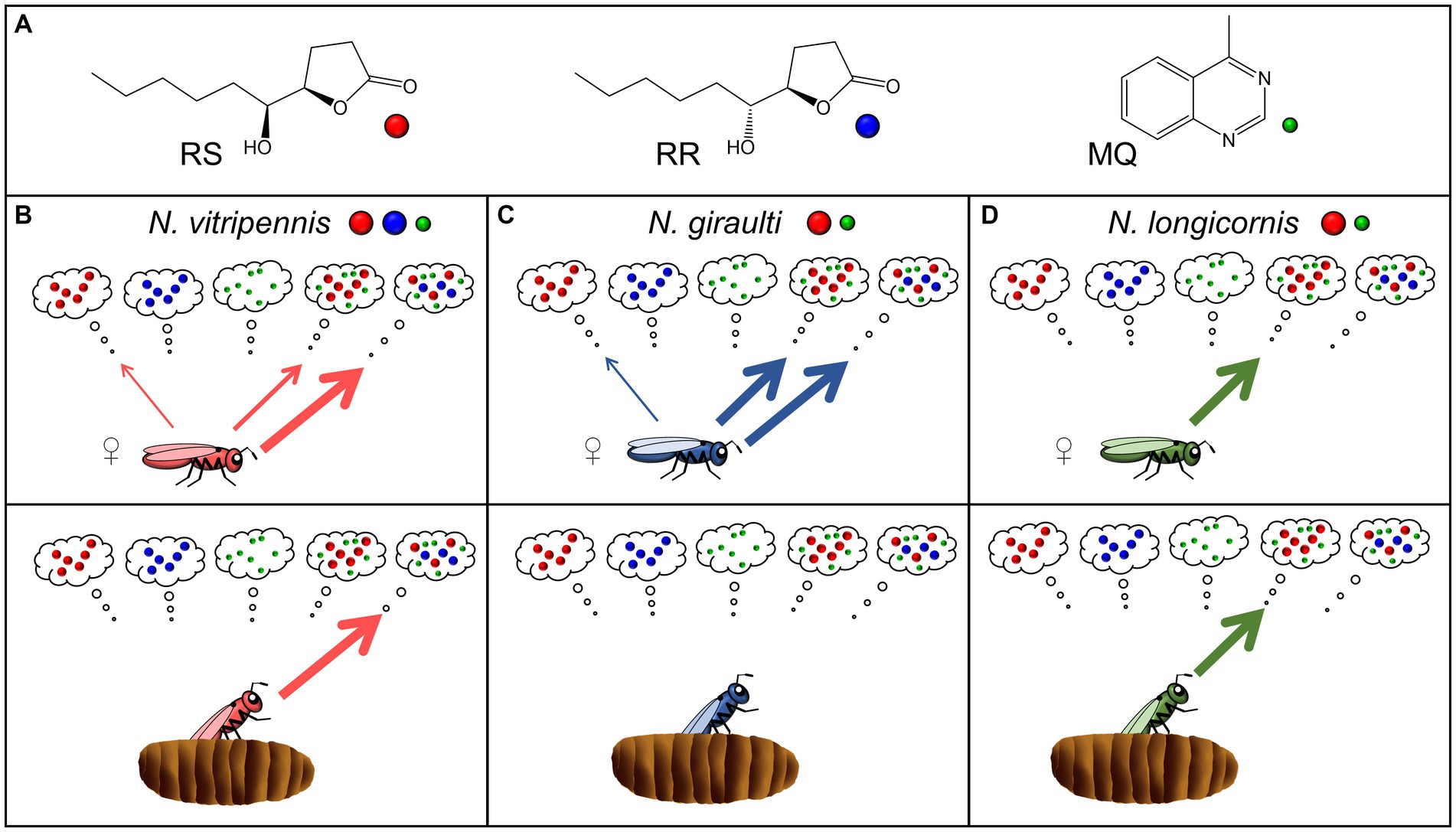
Figure 1. (A) Structures of the components of the male abdominal sex pheromones in the genus Nasonia, (4R,5S)-5-hydroxy-4-decanolide (RS), (4R,5R)-5-hydroxy-4-decanolide (RR), and 4-methylquinazoline (MQ). (B–D) Scheme summarizing the female responses of the hitherto investigated species N. vitripennis (Niehuis et al., 2013; Ruther et al., 2014), N. giraulti (Niehuis et al., 2013; Ruther et al., 2014), and N. longicornis (this study) to the male sex pheromone components and combinations thereof. Pheromone composition of the different species is indicated by colored dots near the species names. The upper panels show the response of 2-d-old virgin females excised from the hosts, the lower panels refer to females collected at the moment of emergence from the host (for more details see text).
Pheromone markings, abdomen extracts and synthetic blends of the ternary Nv pheromone (RS + MQ + RR) and the binary Ng pheromone (RS + MQ) all attract conspecific females (Niehuis et al., 2013). The exclusive occurrence of RR in Nv strongly suggests that this compound has a function in precopulatory isolation and reduces the risk of interspecific sexual interactions in sympatric Nasonia species both in eastern and western North America. The role of individual pheromone components, however, has so far only been studied in the eastern species pair Nv/Ng. In the initial study addressing this question (Niehuis et al., 2013), virgin females of both species were attracted to RS when presented alone (Figures 1B,C). The attractiveness of RS was synergized by the minor component MQ in both Nv and Ng, whereas MQ had no effect when presented alone. Likewise, RR was neither attractive for Nv nor for Ng virgin females when presented alone, but the addition of RR to the binary Ng blend (RS + MQ) synergized the attractiveness of this blend for Nv whereas this modification had no effect on Ng females. Hence, females of both species responded to the pheromone of heterospecific males, but Nv females preferred the ternary conspecific pheromone blend over the binary heterospecific one (Niehuis et al., 2013). The preference for the heterospecific pheromone blend in either species raised the question whether this is associated with evolutionary costs. In the case of Ng, this is unlikely, because Ng differs from its Nasonia congeners by showing a very high within-host-mating (WHM) rate, i.e., mating in Ng occurs already inside the host prior to female emergence (Drapeau and Werren, 1999). Given that mating makes Nv and Ng females unresponsive to the male sex pheromone (Ruther et al., 2010, 2014; Ruther and Hammerl, 2014) it is unlikely that the response to the ternary Nv pheromone blend has fitness consequences for them. In fact, females collected at the moment of emergence from the host were not even attracted to the conspecific pheromone (Ruther et al., 2014; Figure 1C). As for Nv, mating occurs almost exclusively outside the host within a few minutes after emergence of the females (Mair and Ruther, 2018) and thus, the attraction of females to the binary Ng blend might be problematic. However, a second study addressing the Nv/Ng pheromone interaction in more detail revealed that the attraction of virgin Nv females to the binary Ng blend is age-dependent (Ruther et al., 2014). Newly eclosed females and those collected at the moment of emergence from the host did not respond to the binary Ng blend but strongly preferred the conspecific ternary blend (Figure 1B). Only with increasing age (≥1d), when most females would have been mated and dispersed under natural conditions anyway (Mair and Ruther, 2018), do Nv females became less choosy and responsive to the heterospecific binary pheromone blend (Ruther et al., 2014). Nothing is known about the pheromone response and the role of individual pheromone components in Nl females. This species shows intermediate WHM rates (Drapeau and Werren, 1999; Trienens et al., 2021) and thus many females emerge as virgins and mate outside the host. Therefore, Nl females should discriminate more strongly against the ternary Nv pheromone blend than Ng females to avoid costly sexual interactions with Nv males in regions of sympatry.
The abdominal sex pheromone of Nv males is not only attractive for virgin females but also mediates site fidelity of the pheromone-marking males using their own pheromone markings (van den Assem et al., 1980). This behavior could also be interpreted as an economization of pheromone use because males have a way to stay near and return to their own pheromone markings rather than needing to deposit a new one. The minor component MQ has been shown to mediate this phenomenon in Nv (Ruther et al., 2011), whereas nothing is known about the male response to the pheromones in the other Nasonia species.
In the present study, we fill a knowledge gap about pheromone communication in the genus Nasonia by investigating chemical and behavioral aspects of the male Nl sex pheromone. We study the age dependence of pheromone titers in males, examine the context of pheromone deposition and elucidate the role of the different pheromone components in mediating the attractiveness of the pheromone for both females and males. We hypothesize that the sex pheromone of Nl males attracts virgin females and mediates site fidelity in males whereas the Nv-specific pheromone component RR contributes to precopulatory isolation between the sympatric species Nl and Nv.
Materials and methods
Insects
The Nl strain NLMN8510 used in this study was kindly provided by B. Pannebakker (University of Wageningen). Wasps were reared on freeze-killed pupae of the green bottle fly Lucilia caesar at 25°C under a 16:8 light:dark regime. To obtain wasps of known age and mating status, fully melanized pupae were isolated from host puparia and kept singly in 1.5-mL microcentrifuge tubes. Tubes were checked daily in the morning and newly eclosed wasps were defined as being 0 day old. Mated females were obtained by observing couples under a stereomicroscope until copulation was completed. To obtain enough males for the pheromone isolation, all-male broods were produced by using virgin females for parasitization. Because Nasonia wasps are haplodiploid, virgin females produce all-son offspring. In all experiments, wasps were used only once.
Pheromone extraction
Sex pheromone extracts of Nl and Nv males for bioassays were obtained by extracting batches of 30 abdomens from 2-d-old freeze-killed males with 30 μl of dichloromethane (DCM) per abdomen. After 30 min, the abdomens were removed, and raw extracts were cleaned by solid phase extraction as described previously for Nv (Ruther et al., 2007). One microliter aliquots of cleaned extracts (representing one male equivalent) were used in the bioassays.
To investigate the age dependence of male pheromone titers, the abdomens of 0-, 1-, 2-, and 3-d-old males (n = 10 per age) were dissected and extracted singly with 30 μl DCM containing 10 ng μl−1 methyl undecanoate (Sigma-Aldrich, Deisenhofen, Germany) as an internal standard. These extracts were analyzed by coupled gas chromatography/mass spectrometry (GC/MS, see “GC/MS analysis”).
Bioassays
The response of Nl to pheromone extracts and synthetic pheromone components was observed using a still-air, dual-choice olfactometer described previously (Ruther et al., 2014). It consists of a round glass arena (9 cm diameter) with a hole (3 mm diameter) in the center from which the wasps were released. Four symmetrically arranged spherical cavities (1 cm diameter, 4 mm depth, 3 cm distance from the central hole) in the arena served for sample storage and a plastic rim (2 mm height) was used as spacer for a glass plate that covered the arena from above. Natural and synthetic pheromone samples (1 μl) or equal amounts of the pure solvent (control) were applied to discs of filter paper (5 mm diameter). After 1 min of solvent evaporation, paper discs were placed into two opposite cavities of the olfactometer, whereas the two remaining cavities were left empty. A wasp was released into the central hole, and the arena was covered with the glass plate. Finally, an open gray plastic cylinder (11 cm diameter, 6 cm height) was put on the upper glass plate to exclude visual stimuli. The experimental set-up was illuminated from above with a desk light (60 W incandescent lamp, distance 50 cm, centered by eye). The residence times spent by the responding wasps (n = 25 for each test if not stated otherwise) in the pheromone-baited and the unbaited control cavity was recorded for 5 min (live observation) using The Observer XT 15.0 observational software (Noldus, Wageningen, The Netherlands). After each observation, the arena was rotated by 180°C to avoid positional bias. After every five observations, the arenas were cleaned with ethanol and deionized water and dried at 60°C. Doses and relative proportions of synthetic pheromones used in the experiments were matched to the amounts found in natural pheromone extracts and pheromone markings deposited by live Nl males (see results of this paper) and Nv (Blaul and Ruther, 2011), respectively.
Responses of females to natural and synthetic pheromones
In the first series of experiments, we tested responses of Nl females (1–2 day old) to the conspecific pheromone and synthetic analogues thereof (Table 1). Virgin females were exposed in the olfactometer to (a) natural pheromone extract of conspecific males (one male equivalent), (b) the synthetic pheromone components RS (200 ng), (c) MQ (0.5 ng) and (d) a combination of RS (200 ng) + MQ (0.5 ng). To investigate whether the Nv-specific pheromone component RR alters the behavior of virgin Nl females, we also tested their responses to (e) natural pheromone extract from Nv against extract from Ng males, (f) the ternary heterospecific combination RS (200 ng) + MQ (0.5 ng) + RR (100 ng) against the solvent control, and (g) the ternary blend directly against the binary conspecific combination RS (200 ng) + MQ (0.5 ng). To investigate the impact of the mating status on the pheromone responses of Nl females, we tested the responses of (h) mated females to the natural pheromone extract (one male equivalent). Finally, we tested the responses of (i) females collected at the moment of emergence from the host to a combination of RS (200 ng) and MQ (0.5 ng; n = 39). The last experiment of this series was performed to investigate whether emerging females are responsive to the male pheromone despite having been exposed to males inside the host thus having had the opportunity of WHM. We additionally controlled the WHM rate of the tested females by offering them hosts for 2 days after the experiment and checking whether they produced daughters. All-son offspring would indicate virginity of the respective female because of the haplodiploid sex determination system in Nasonia.
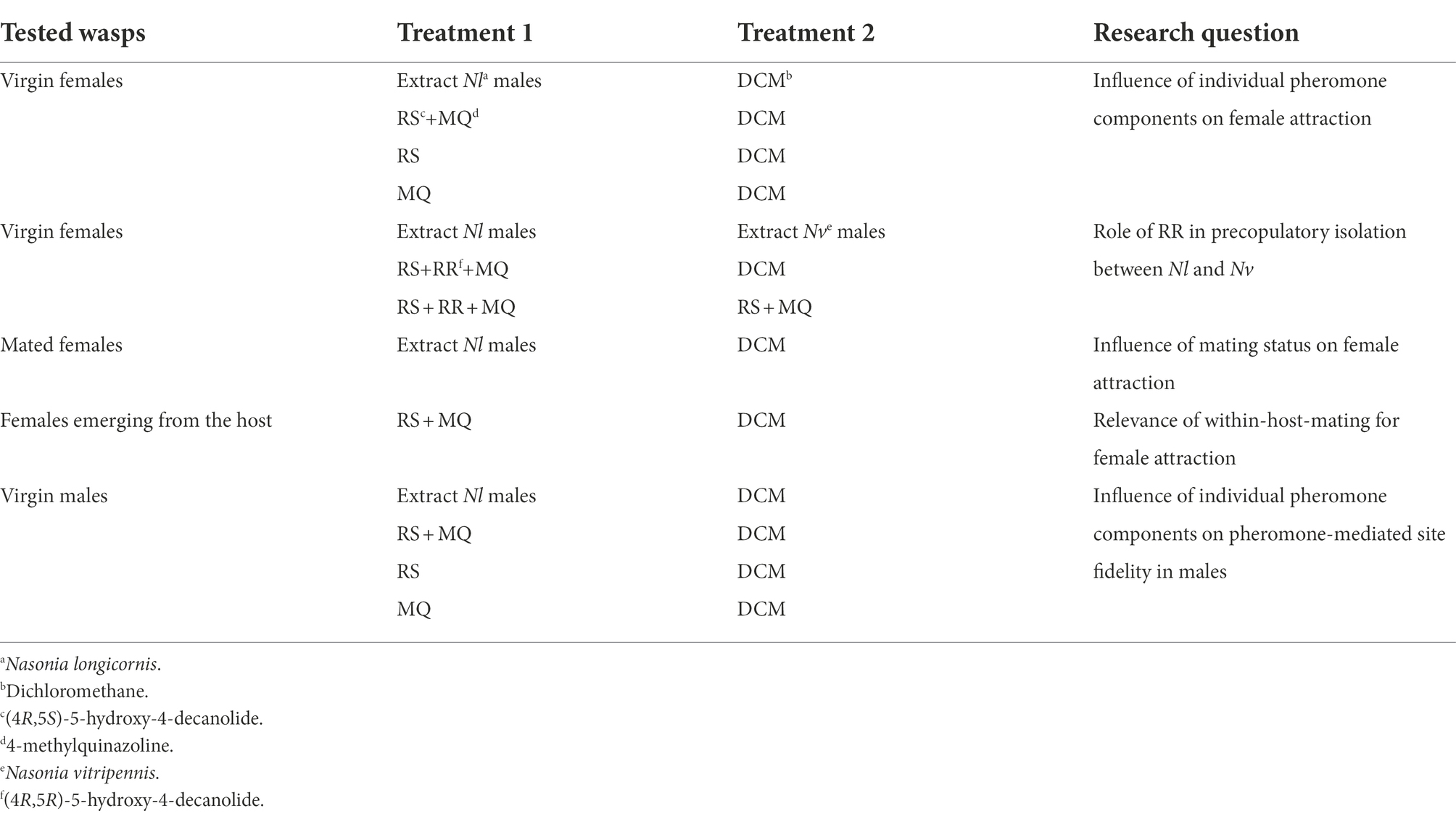
Table 1. Overview of the behavioral bioassays testing the responses of N. longicornis to natural extracts and synthetic pheromone components (for more details see text).
Responses of males to natural and synthetic pheromones
In the second series of experiments, we tested the responses of naïve Nl males to their own pheromone and synthetic constituents thereof. Unmated males were exposed in the olfactometer to (a) natural pheromone extract (one male equivalent), (b) RS (200 ng), (c) MQ (0.5 ng) and (d) a combination of RS (200 ng) + MQ (0.5 ng).
Male abdomen dipping behavior
To study the behavioral context of abdomen dipping, individual males were placed into a glass observation chamber (3 cm diameter, 12 mm high) and the frequency of abdomen dipping was recorded for 10 min under a stereomicroscope. Two-day-old males were observed (a) without any additional cue being present, (b) immediately after having had contact with a female but without having copulated, (c) immediately after having mated with a female. Additionally, we observed males (d) in the presence of a parasitized host containing females about to emerge to investigate whether the upcoming emergence of females has a preemptive effect on pheromone deposition behavior. Under our rearing conditions, Nl develops within 14 days. Thus, the hosts offered in the last experiment had been parasitized 14 days prior to the experiment but did not yet show any visible exit holes.
Quantification of deposited pheromone
RS and MQ deposited by abdomen dipping of individual males were quantified by GC/MS using thermal desorption (TD) sampling as described previously for Nv (Blaul and Ruther, 2011). To this end, empty 89 mm x 5 mm inner diameter TD glass tubes that had been filled at one end with 50 mg of Tenax TA (Supelco, Bellefonte, PA) were used. The adsorbent layer was fixed using fine mesh metal screens (Supelco). One μL of an internal standard solution containing 100 ng μL−1 methyl undecanoate (Sigma-Aldrich, Deisenhofen, Germany) in methanol was applied to the adsorbent, and the tube was purged for 5 min with a nitrogen flow of 60 ml min−1 to remove the solvent. Subsequently, 2-day-old males were released into the open end of the adsorption tubes and kept there for 10 min. Analogous to the behavioral observations in “Male abdomen dipping behavior,” the 2-day-old males were either (a) naïve (unmated), (b) had contact with a female but were prevented from mating or (c) were sampled immediately after having mated with a female (n = 20 per treatment). After removing the male, TD tubes were purged for 10 min with charcoal filtered air at a flow rate of 60 ml min−1 (adsorbent upstream) to trap the volatilized proportions of RS and MQ. By this means, losses of RS and MQ during sampling were kept to a minimum and both the pheromone adsorbed to the glass surface and the volatilized proportion could be sampled. TD was accomplished using an automated Shimadzu TD20 sampler coupled to a QP2010 Plus GC/MS system (Shimadzu GmbH, Duisburg, Germany) as described previously (Blaul and Ruther, 2011).
GC/MS analysis
Aliquots (1 μl) of pheromone extracts were analyzed on a Shimadzu QP2010 Plus GC/MS system fitted with a non-polar BPX5 capillary column (60 m × 0.25 mm inner diameter, 0.25 μm film thickness, SGE Analytical Science Europe, Milton Keynes, UK). Samples were injected at 280°C in splitless mode using an AOC 20i auto sampler. Helium was used as carrier gas at a linear velocity of 37.8 cm s−1. The initial oven temperature of 80°C was increased at 5°C min−1 to 280°C and held at this temperature for 15 min. The mass spectrometer was operated in electron ionization (EI) mode at 70 eV, and the mass range was m/z 35–600. RS and MQ were identified by comparison of mass spectra and retention times with those of authentic reference chemicals that were synthesized as described previously (Ruther et al., 2008, 2016). For pheromone quantification, peak areas of RS and MQ were related to the peak area of the internal standard.
Statistical analysis
Data did not meet the assumptions of parametric statistical analysis. Therefore, non-parametric tests were applied using Past 4.05 statistical software (Hammer et al., 2001). Pheromone titers of males of different age, frequencies of abdomen dipping by differently treated males and deposited amounts of RS and MQ were compared by a Kruskal-Wallis H-test followed by multiple pairwise Mann–Whitney U-tests with Bonferroni correction. Residence times in the test and control cavities of the olfactometer were analyzed by a Wilcoxon matched pairs test.
Results
Male pheromone titers were almost zero in newly eclosed males, increased significantly within the first 2 days after eclosion and remained constant on day three (median RS/MQ 0d: 0/0 ng; 1d: 14/0.1 ng; 2d: 302/0.4 ng; 3d: 318/0.4 ng; Figures 2A,B). In the behavioral bioassays, virgin Nl females were attracted to filter paper disks treated with purified abdomen extract or the binary conspecific blend consisting of synthetic RS + MQ (Figures 3A,D). However, they neither preferred RS nor MQ over the solvent controls when these chemicals were offered alone (Figures 3B,C). Virgin females preferred purified extract of conspecific males over extract of Nv males. They did not prefer the ternary heterospecific pheromone blend consisting of synthetic RS + MQ + RR when tested against the solvent control. When given the choice between the ternary hetero- and the binary conspecific pheromone blends, Nl females preferred the latter (Figures 3E–G). Mated Nl females were no longer attracted to the male sex pheromone (Figure 4A). However, females collected at the moment of emergence from the host were attracted to the conspecific binary pheromone blend indicating that most of them had emerged unmated (Figure 4B). This was confirmed by the analysis of the offspring’s sex, because following olfactometer bioassays most (84.4%) offspring-producing females had only sons, indicative of their virgin mating status.
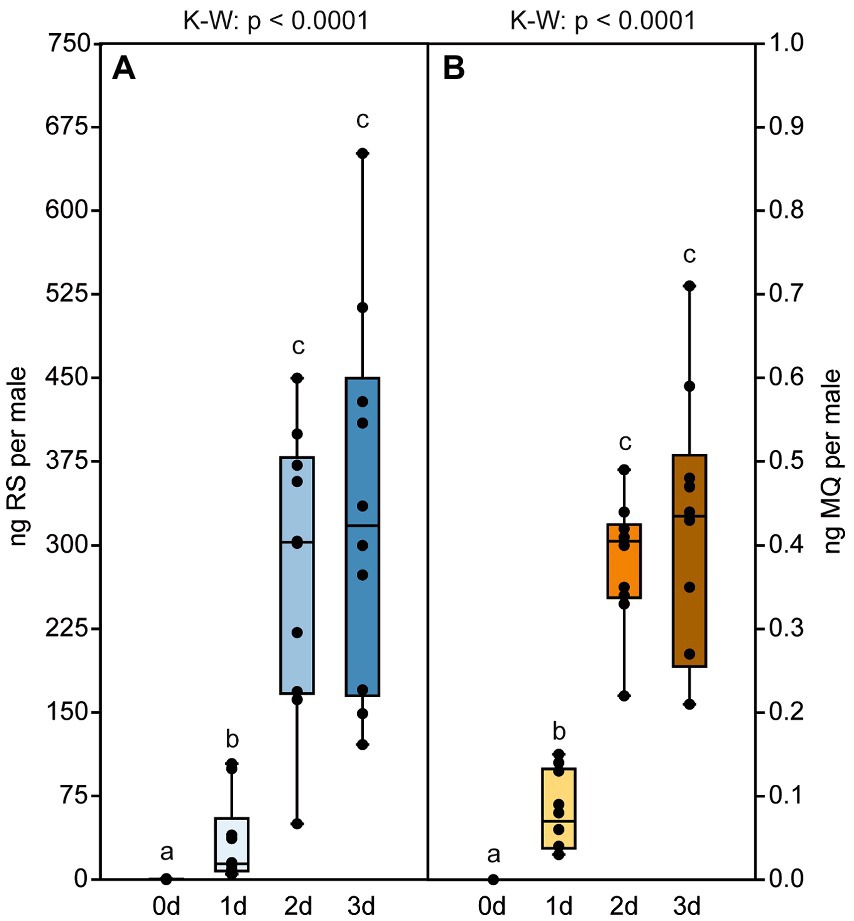
Figure 2. Pheromone titers of N. longicornis males are age dependent. Amounts of (A) (4R,5S)-5-hydroxy-4-decanolide (RS) and (B) 4-methylquinazoline (MQ) in abdomen extracts from N. longicornis males of various age. Box and whisker plots show median (horizontal line), 25%–75% quartiles (box), maximum/minimum range (whiskers) and outliers (°>1.5 × above box height; *>3 × above box height). Different lowercase letters indicate significant differences between the ages at p < 0.001 [n = 10, data analysis by a Kruskal–Wallis H-test (K–W) followed by multiple Bonferroni-corrected Mann–Whitney U-tests].
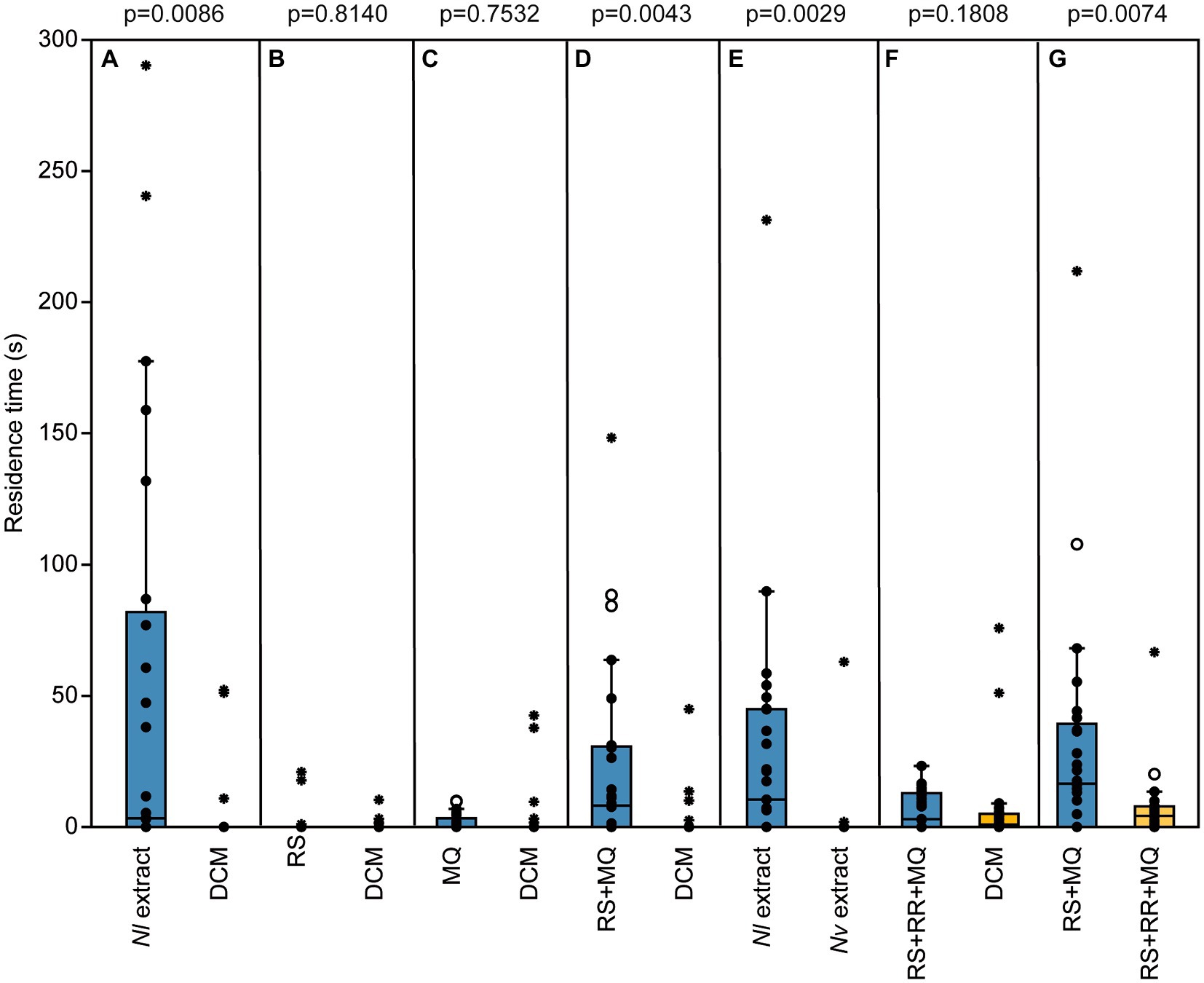
Figure 3. The male sex pheromone attracts virgin Nasonia longicornis (Nl) females species-specifically. Residence time of virgin Nl females in the two odor fields of a static two-choice olfactometer when given the choice between dichloromethane (DCM, control) and (A) one male equivalent of a natural Nl pheromone extract, (B) 200 ng of synthetic (4R,5S)-5-hydroxy-4-decanolide (RS), (C) 0.5 ng of synthetic 4-methylquinazoline (MQ), (D) the synthetic pheromone blend of Nl males (200 ng RS + 0.5 ng MQ), and (F) the synthetic pheromone blend of N. vitripennis (Nv) males (200 ng RS + 0.5 ng MQ + 100 ng (4R,5R)-5-hydroxy-4-decanolide (RR)). Furthermore, virgin females were given direct choice between (E) natural pheromone extracts from Nl and Nv males and (G) the synthetic pheromone blends of Nl and Nv males [treatments (D) and (E)]. Box and whisker plots show median (horizontal line), 25%–75% quartiles (box), maximum/minimum range (whiskers) and outliers (°>1.5 × above box height; *>3 × above box height; n = 25, data analysis by a Wilcoxon matched-pairs test).
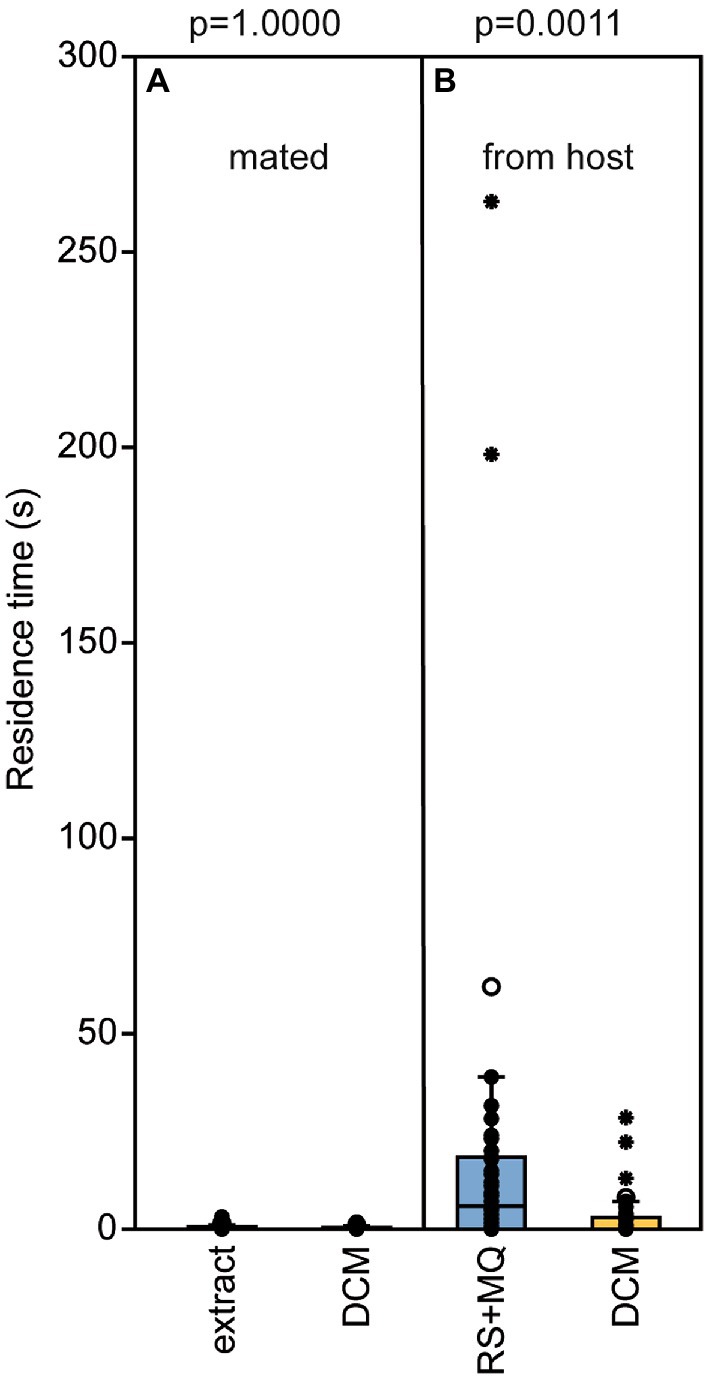
Figure 4. Newly emerged, but not mated, Nasonia longicornis females are attracted by the male pheromone. Residence time of N. longicornis females in the two odor fields of a static two-choice olfactometer when given the choice between dichloromethane (DCM, control) and (A) one male equivalent of a natural N. longicornis pheromone extract (mated females) or (B) a combination of synthetic (4R,5S)-5-hydroxy-4-decanolide (RS) + 0.5 ng 4-methylquinazoline (MQ; females were collected during the process of emergening from the host). Box and whisker plots show median (horizontal line), 25%–75% quartiles (box), maximum/minimum range (whiskers) and outliers (°>1.5 × above box height; *>3 × above box height; n = 25, data analysis by a Wilcoxon matched-pairs test).
Nl males were attracted to their own pheromone in the olfactometer tests. They preferred both the natural pheromone and the binary blend consisting of synthetic RS + MQ over the solvent control. When given the choice between RS or MQ alone and the solvent control, males preferred MQ but not RS indicating that MQ underlies the preference of males for their own pheromone (Figures 5A–D).
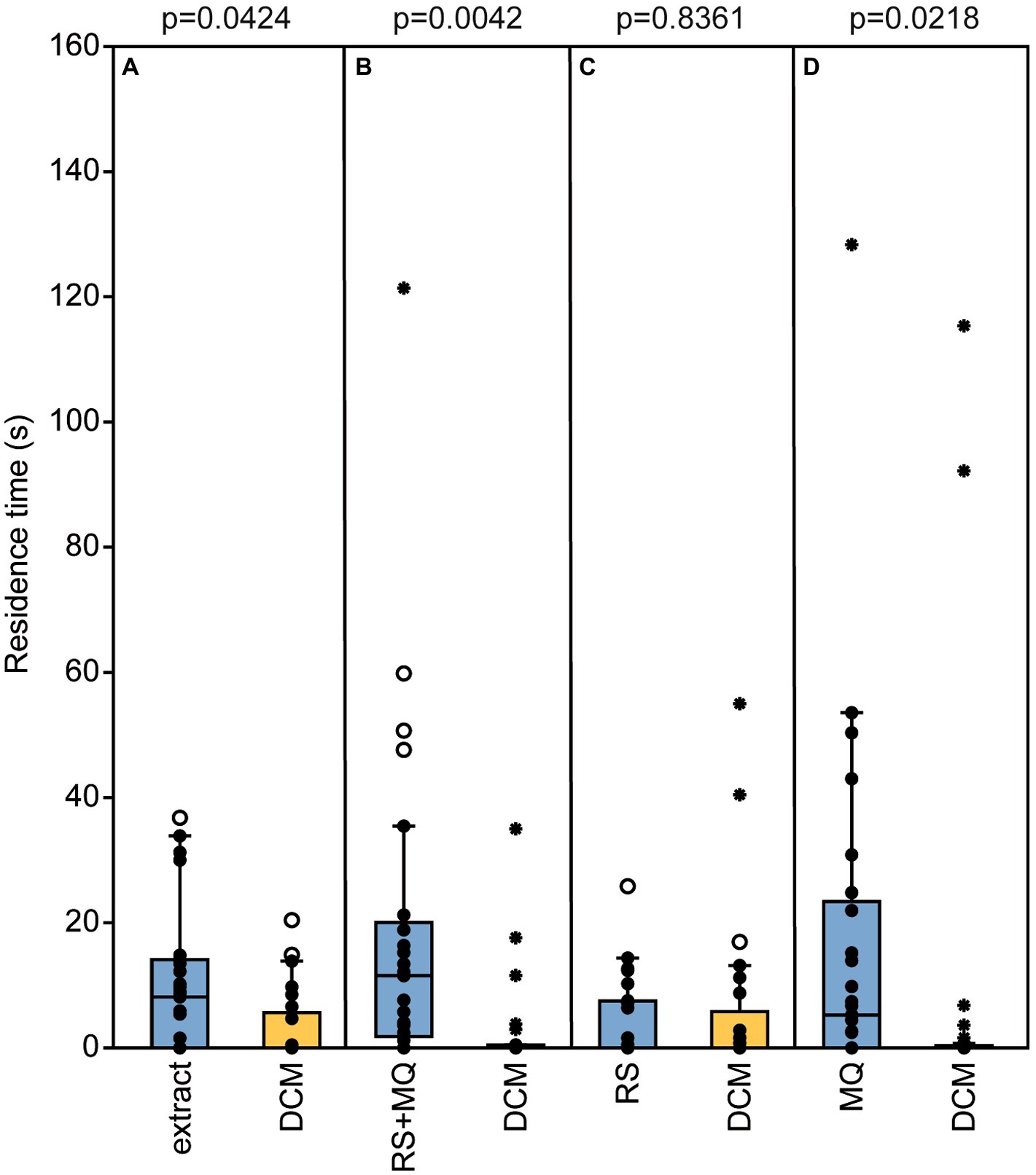
Figure 5. Nasonia longicornis males are arrested by their own pheromone. Residence time of unmated N. longicornis males in the two odor fields of a static two-choice olfactometer when given the choice between dichloromethane (DCM, control) and (A) one male equivalent of a natural N. longicornis pheromone extract, (B) a combination of 200 ng of synthetic (4R,5S)-5-hydroxy-4-decanolide (RS) + 0.5 ng 4-methylquinazoline (MQ), (C) 200 ng RS, and (D) 0.5 ng MQ. Box and whisker plots show median (horizontal line), 25%–75% quartiles (box), maximum/minimum range (whiskers) and outliers (°>1.5 × above box height; *>3 × above box height; n = 25, data analysis by a Wilcoxon matched-pairs test).
Naïve, 2-d-old males exhibited the typical abdomen dipping behavior that has been shown to serve pheromone deposition in Nv males (Steiner and Ruther, 2009). Both contact with a female and successful mating equally increased abdomen dipping frequency in Nl males (Figure 6A). In contrast, the presence of a host containing females about to emerge did not increase male abdomen dipping frequency (Figure 7A) and males showed this marking behavior almost exclusively in the vicinity of a host but hardly on a host itself (Figure 7B). Quantification of deposited pheromone after abdomen dipping revealed that 2-day-old, mated males deposited significantly more RS than naïve ones of the same age, while males with only female contact released intermediate amounts (median RS unmated: 1.5 ng; contact: 12 ng; mated: 176 ng; Figure 6B). As for the minor component MQ, deposited amounts showed a similar pattern (Figure 6C), however, statistical analysis did not reveal any significant differences between treatments (median MQ unmated: 0 ng; contact: 0.01 ng; mated: 0.2 ng).
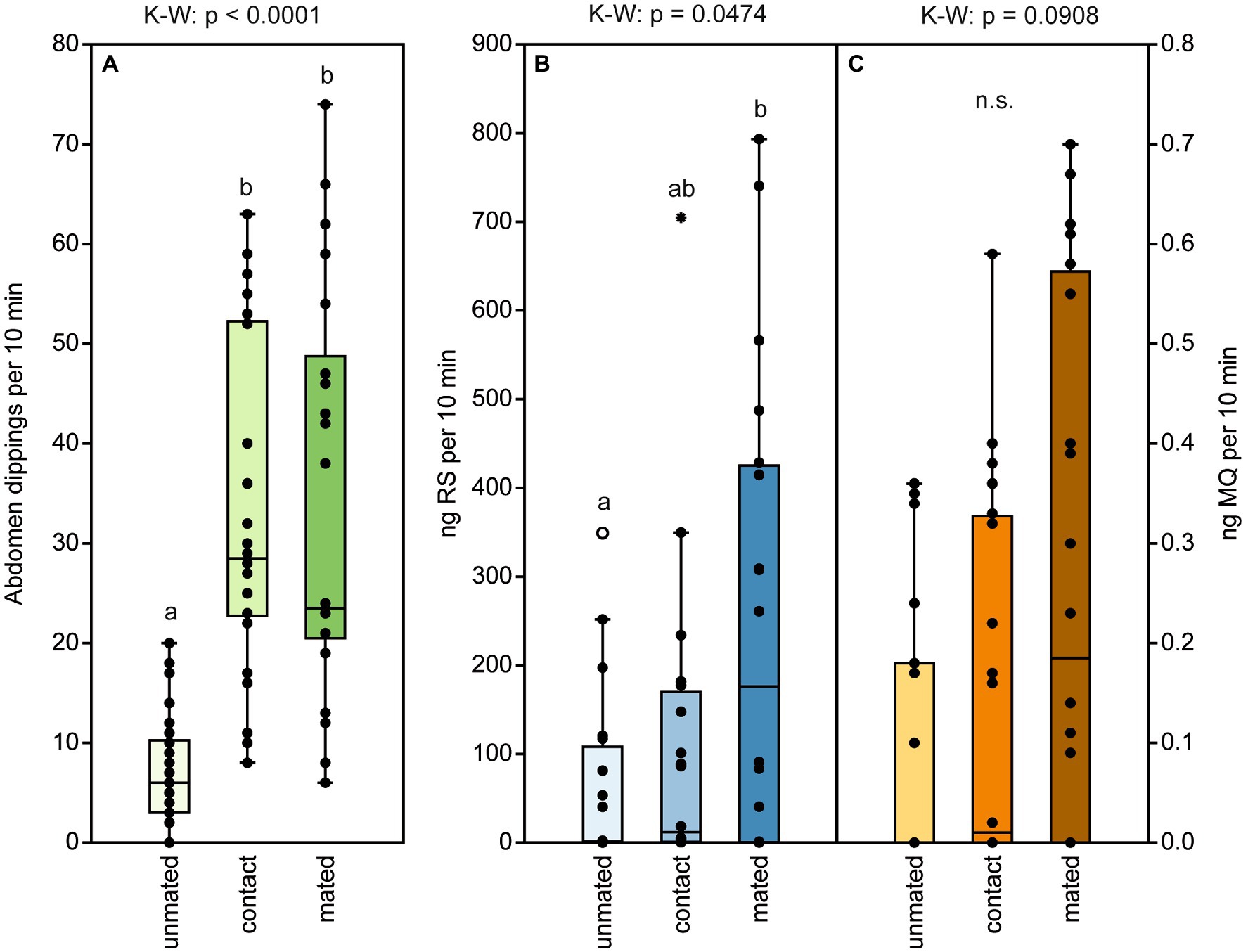
Figure 6. Male abdomen dipping serves to deposit pheromone and increases in frequency after mating and contact with a female. (A) Frequency of abdomen dipping shown by males within a 10-min observation time. Males were either unmated (naïve), had contact with a female, or were allowed to mate with a female. (Β) Amounts of (4R,5S)-5-hydroxy-4-decanolide (RS) and (C) 4-methylquinazoline (MQ) deposited by males of the three treatments within 10 min in a thermal desorption tube. Box and whisker plots show median (horizontal line), 25%–75% quartiles (box), maximum/minimum range (whiskers) and outliers (°>1.5 × above box height; *>3 × above box height). Different lowercase letters indicate significant differences between the treatments at p < 0.05 [n.s. = non significant, n = 10, data analysis by a Kruskal–Wallis H-test (K–W) followed by multiple Bonferroni-corrected Mann–Whitney U-tests].
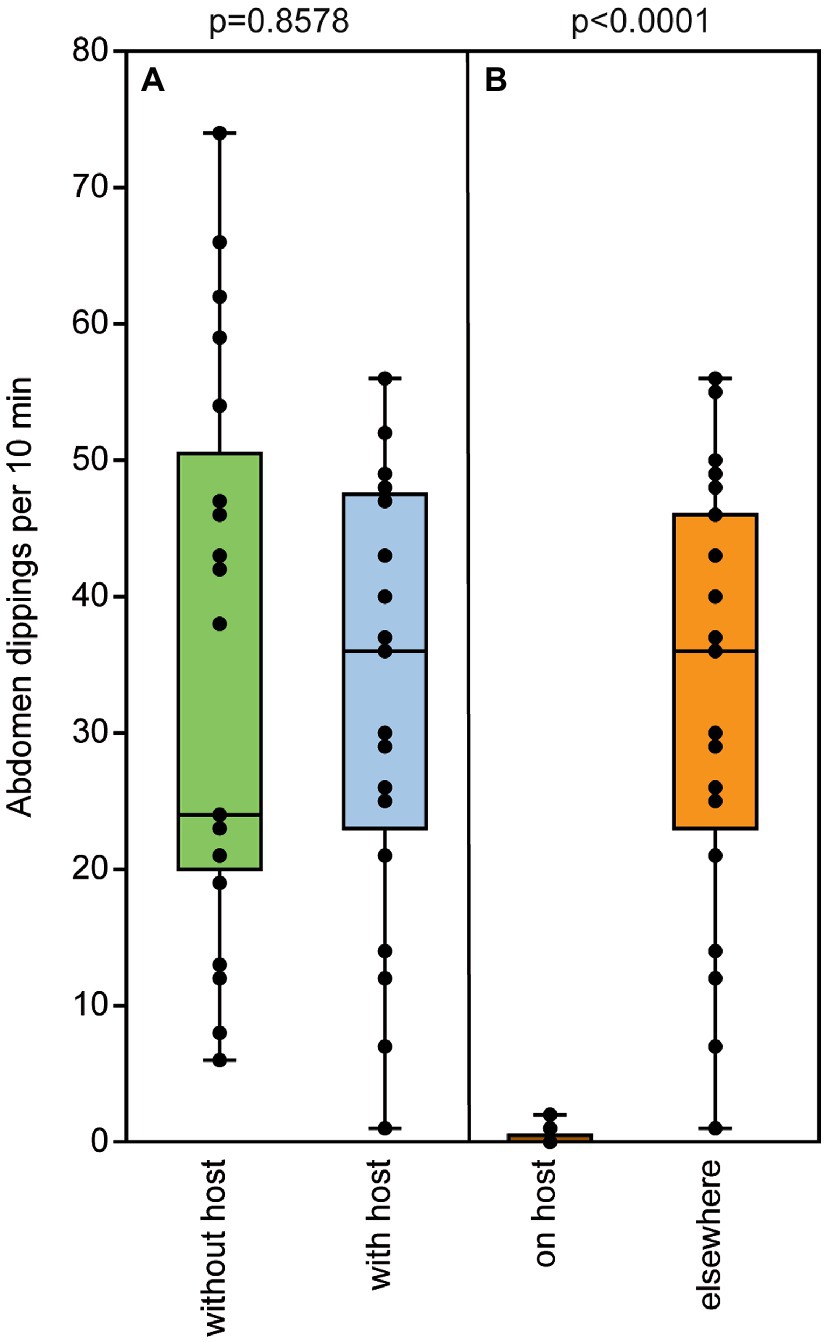
Figure 7. The presence of a parasitized host does not influence male marking behavior. (A) Frequency of abdomen dipping shown by mated males within a 10-min observation time in the absence or presence of a host containing females about to emerge. (Β) Location of abdomen dipping by mated males when a host was present. Box and whisker plots show median (horizontal line), 25%–75% quartiles (box), maximum/minimum range [whiskers; n = 25, data analysis by a (A) Mann–Whitney U-test and (B) Wilcoxon matched-pairs test].
Discussion
The present study demonstrates that Nl uses the male abdominal sex pheromone in a similar way as previously described in the congeneric species Nv and Ng. Furthermore, it shows that the Nv-specific pheromone component RR mediates precopulatory isolation not only in the eastern North American species pair Nv/Ng but also in the western pair Nv/Nl. Like in Nv and Ng (Ruther et al., 2007, 2010, 2014), exclusively virgin Nl females were attracted to the conspecific male pheromone. The vast majority of Nl females emerged from the host as virgins showing that mating occurs predominantly outside the host and that the male pheromone is relevant for finding an adequate mating partner. In contrast to Nv and Ng (Niehuis et al., 2013), RS is not attractive for Nl females when presented alone. Rather, the presence of synergistic MQ is needed to attract Nl females. The addition of the Nv-specific pheromone component RR to the conspecific binary blend RS + MQ made Nl females unresponsive, and they preferred both natural and synthetic conspecific binary blends over the respective ternary heterospecific blends when exposed to them simultaneously. This demonstrates that Nl females discriminate more strongly against the Nv-specific pheromone component RR than previously reported for Ng (Niehuis et al., 2013). This might be explained by the lower WHM rate in Nl (Drapeau and Werren, 1999; Trienens et al., 2021) that makes encounters between virgin Nl females and Nv males in shared habitats more likely than in the eastern species pair Nv/Ng. The high WHM rate in Ng has been shown to be due to the males refraining from gnawing exit holes through which virgin females might escape. A recent study, however, has demonstrated that Ng shows much lower WHM rates when developing in microsympatry with Nv, because virgin Ng females emerge through exit holes gnawed by Nv males (Trienens et al., 2021). This suggests that, under conditions of microsympatry, Ng females are prone to get involved in costly sexual interactions with Nv males if they do not discriminate against the ternary pheromone blend produced by these sympatric heterospecific males. Therefore, it remains to be investigated whether Ng females having developed in microsympatry with Nv discriminate more strongly against the Nv-specific pheromone component RR than those having developed in the absence of Nv. The strong discrimination of Nl females against the Nv-specific pheromone component RR comes along with a low discriminatory ability shown by these females during courtship. In a previous study, Nl females accepted more than 80% Nv males as mates while vice versa Nv females accepted less than 30% of Nl males (Giesbers et al., 2013). This suggests that Nl females rely mainly on precopulatory isolation based on the male abdominal sex pheromone to avoid costly interspecific mating with Nv males, while Nv females use both the species-specific composition of the male pheromones (Niehuis et al., 2013; Ruther et al., 2014) as well as courtship-related cues (Giesbers et al., 2013) to decrease the risk of mismating with sympatric congeneric males.
Nl males economize both pheromone production and deposition. Like in Nv and Ng (Ruther et al., 2007, 2014), Nl males eclose without any pheromone in the rectal vesicle, and pheromone production starts only after eclosion. In Nv, newly eclosed males typically remain inside the host for approximately 1.5 days before emerging from the host and most males eclose and emerge before the females (protandry; Moynihan and Shuker, 2011). Assuming a similar scenario in Nl, emerging males should have produced sufficient pheromone to attract and arrest virgin females. Nl males show abdomen dipping increasingly if mating chances are high, i.e., after contact or successful mating with a female. Once an exit hole has been gnawed by protandrous Nasonia males, females may emerge from the host in rapid succession (Mair and Ruther, 2018). Hence, the presence of one female increases the probability of meeting others and pheromone deposition is likely to enable additional mating opportunities. This is supported by our TD analyses of deposited pheromone amounts: Mated Nl males deposited ca. two-thirds of their available pheromone which is probably a significant investment. The presence of hosts (without showing exit holes) containing females about to emerge is obviously not sufficient to trigger this investment. Pheromone markings remain attractive for virgin Nasonia females for a maximum of 2 h (Steiner and Ruther, 2009). Hence, males likely refrain from depositing higher pheromone amounts in the presence of female containing hosts, because they are unable to reliably estimate the time of female emergence when exit holes are missing. Dominant Nv males pheromone-marked monopolized hosts to prevent females from dispersing unmated. In these experiments, however, exit holes were already present (Mair and Ruther, 2018). Site fidelity at the sites of pheromone deposition is another strategy of economic pheromone use in Nasonia. As previously shown for Nv (Ruther et al., 2011), Nl males use the trace component MQ but not the major component RS to recognize the pheromone markings at which virgin females are likely to appear. In this context, 0.5 ng MQ was sufficient to mediate site fidelity in Nl males and to enhance responses of virgin Nl females. This falls into place with much lower MQ titers determined in Nl males (ca. 0.5 ng per male) when compared to values reported previously for Nv (ca. 3 ng per male; Ruther et al., 2008).
After this study, No remains the only Nasonia species to be investigated in detail with respect to responses of females and males to male sex pheromone. Given that No is sympatric with both Nv and Ng in eastern North America, No females likely encounter both the binary pheromone blend of Ng males and the ternary pheromone blend of Nv males, with the former blend being identical to that of conspecific No males. However, No has been reported to possess much lower titers of the binary pheromone blend (RS + MQ) than Ng (Diao et al., 2016) suggesting that No no longer uses the male abdominal sex pheromone in the context of mate finding. Further studies are needed to fully understand the role of male sex pheromones in precopulatory isolation of the three sympatric Nasonia species in eastern North America.
Data availability statement
The raw data supporting the conclusions of this article will be made available by the authors, without undue reservation.
Author contributions
JR designed the study, supervised AK, and wrote the first draft of the manuscript. AK performed the experiments and analyzed the data. JH synthesized and provided a sample of (4R,5S)-5-hydroxy-4-decanolide. All authors contributed to the article and approved the submitted version.
Acknowledgments
The authors thank B. Pannebakker for providing a starter culture of N. longicornis and J. Herman for rearing the insects.
Conflict of interest
The authors declare that the research was conducted in the absence of any commercial or financial relationships that could be construed as a potential conflict of interest.
Publisher’s note
All claims expressed in this article are solely those of the authors and do not necessarily represent those of their affiliated organizations, or those of the publisher, the editors and the reviewers. Any product that may be evaluated in this article, or claim that may be made by its manufacturer, is not guaranteed or endorsed by the publisher.
References
Abdel-Latief, M., Garbe, L. A., Koch, M., and Ruther, J. (2008). An epoxide hydrolase involved in the biosynthesis of an insect sex attractant and its use to localize the production site. Proc. Natl. Acad. Sci. U. S. A. 105, 8914–8919. doi: 10.1073/pnas.0801559105
Barrass, R. (1969). Preening and abdomen dipping by the male Mormoniella vitripennis (Walker) (Hymenoptera, Pteromalidae) after courtship. Behaviour 35, 304–312. doi: 10.1163/156853969X00279
Blaul, B., and Ruther, J. (2011). How parasitoid females produce sexy sons: a causal link between oviposition preference, dietary lipids and mate choice in Nasonia. Proc. R. Soc. B-Biol. Sci. 278, 3286–3293. doi: 10.1016/j.ibmb.2014.05.007
Bordenstein, S. R., O’hara, F. P., and Werren, J. H. (2001). Wolbachia-induced incompatibility precedes other hybrid incompatibilities in Nasonia. Nature 409, 707–710. doi: 10.1038/35055543
Breeuwer, J. A. J., and Werren, J. H. (1990). Microorganisms associated with chromosome destruction and reproductive isolation between two insect species. Nature 346, 558–560. doi: 10.1038/346558a0
Darling, D. C., and Werren, J. H. (1990). Biosystematics of Nasonia (Hymenoptera, Pteromalidae) - two new species reared from birds nests in North-America. Ann. Entomol. Soc. Am. 83, 352–370. doi: 10.1093/aesa/83.3.352
Diao, W. W., Mousset, M., Horsburgh, G. J., Vermeulen, C. J., Johannes, F., Van De Zande, L., et al. (2016). Quantitative trait locus analysis of mating behavior and male sex pheromones in Nasonia wasps. G3 Genes Genom. Genet. 6, 1549–1562. doi: 10.1534/g3.116.029074
Drapeau, M. D., and Werren, J. H. (1999). Differences in mating behaviour and sex ratio between three sibling species of Nasonia. Evol. Ecol. Res. 1, 223–234.
Giesbers, M. C. W. G., Gerritsma, S., Buellesbach, J., Diao, W. W., Pannebakker, B. A., Van Der Zande, L., et al. (2013). “Prezygotic isolation in the parasitoid wasp genus Nasonia,” in Speciation: Natural Processes, Genetics and Biodiversity. ed. P. Michalak (New York: Nova Biomedical), 165–191.
Grillenberger, B. K., Van De Zande, L., Bijlsma, R., Gadau, J., and Beukeboom, L. W. (2009). Reproductive strategies under multiparasitism in natural populations of the parasitoid wasp Nasonia (Hymenoptera). J. Evol. Biol. 22, 460–470. doi: 10.1111/j.1420-9101.2008.01677.x
Hammer, Ø., Harper, D. A. T., and Ryan, P. D. (2001). PAST: paleontological statistics software package for education and data analysis. Palaeontol. Electron. 4:4.
Ivens, A. B. F., Shuker, D. M., Beukeboom, L. W., and Pen, I. (2009). Host acceptance and sex allocation of Nasonia wasps in response to conspecifics and heterospecifics. Proc. R. Soc. B-Biol. Sci. 276, 3663–3669. doi: 10.1098/rspb.2009.0977
Leonard, J. E., and Boake, C. R. B. (2006). Site-dependent aggression and mating behaviour in three species of Nasonia (Hymenoptera: Pteromalidae). Anim. Behav. 71, 641–647. doi: 10.1016/j.anbehav.2005.07.010
Lynch, J. A. (2015). The expanding genetic toolbox of the wasp Nasonia vitripennis and its relatives. Genetics 199, 897–904. doi: 10.1534/genetics.112.147512
Mair, M. M., and Ruther, J. (2018). Territoriality and behavioural strategies at the natal host patch differ in two microsympatric Nasonia species. Anim. Behav. 143, 113–129. doi: 10.1016/j.anbehav.2018.07.015
Mair, M. M., and Ruther, J. (2019). Chemical ecology of the parasitoid wasp genus Nasonia (hymenoptera, Pteromalidae). Front. Ecol. Evol. 7:184. doi: 10.3389/fevo.2019.00184
Moynihan, A. M., and Shuker, D. M. (2011). Sexual selection on male development time in the parasitoid wasp Nasonia vitripennis. J. Evol. Biol. 24, 2002–2013. doi: 10.1111/j.1420-9101.2011.02343.x
Niehuis, O., Büllesbach, J., Gibson, J. D., Pothmann, D., Hanner, C., Navdeep, M., et al. (2013). Behavioural and genetic analyses of Nasonia shed light on the evolution of sex pheromones. Nature 494, 345–348. doi: 10.1038/nature11838
Prazapati, G., Yadav, A., Ambili, A., Sharma, A., and Raychoudhury, R. (2022). Males of the parasitoid wasp, Nasonia vitripennis, can identify which fly hosts contain females. Roy. Soc. Open Sci. 9:211865. doi: 10.1098/rsos.211865
Raychoudhury, R., Desjardins, C. A., Buellesbach, J., Loehlin, D. W., Grillenberger, B. K., Beukeboom, L., et al. (2010). Behavioral and genetic characteristics of a new species of Nasonia. Heredity 104, 278–288. doi: 10.1038/hdy.2009.147
Ruther, J., Hagström, Å. K., Brandstetter, B., Hofferberth, J., Bruckmann, A., Semmelmann, F., et al. (2016). Epimerisation of chiral hydroxylactones by short-chain dehydrogenases/reductases accounts for sex pheromone evolution in Nasonia. Sci. Rep. 6:34697. doi: 10.1038/srep34697
Ruther, J., and Hammerl, T. (2014). An oral male courtship pheromone terminates the response of Nasonia vitripennis females to the male-produced sex attractant. J. Chem. Ecol. 40, 56–62. doi: 10.1007/s10886-013-0372-2
Ruther, J., Mccaw, J., Böcher, L., Pothmann, D., and Putz, I. (2014). Pheromone diversification and age-dependent behavioural plasticity decrease interspecific mating costs in Nasonia. PLoS One 9:e89214. doi: 10.1371/journal.pone.0089214
Ruther, J., Stahl, L. M., Steiner, S., Garbe, L. A., and Tolasch, T. (2007). A male sex pheromone in a parasitic wasp and control of the behavioral response by the female's mating status. J. Exp. Biol. 210, 2163–2169. doi: 10.1242/jeb.02789
Ruther, J., Steiner, S., and Garbe, L. A. (2008). 4-methylquinazoline is a minor component of the male sex pheromone in Nasonia vitripennis. J. Chem. Ecol. 34, 99–102. doi: 10.1007/s10886-007-9411-1
Ruther, J., Thal, K., Blaul, B., and Steiner, S. (2010). Behavioural switch in the sex pheromone response of Nasonia vitripennis females is linked to receptivity signalling. Anim. Behav. 80, 1035–1040. doi: 10.1016/j.anbehav.2010.09.008
Ruther, J., Thal, K., and Steiner, S. (2011). Pheromone communication in Nasonia vitripennis: abdominal sex attractant mediates site fidelity of releasing males. J. Chem. Ecol. 37, 161–165. doi: 10.1007/s10886-010-9898-8
Smadja, C., and Butlin, R. K. (2009). On the scent of speciation: the chemosensory system and its role in premating isolation. Heredity 102, 77–97. doi: 10.1038/hdy.2008.55
Steiner, S., and Ruther, J. (2009). Mechanism and behavioral context of male sex pheromone release in Nasonia vitripennis. J. Chem. Ecol. 35, 416–421. doi: 10.1007/s10886-009-9624-6
Tram, U., Fredrick, K., Werren, J. H., and Sullivan, W. (2006). Paternal chromosome segregation during the first mitotic division determines Wolbachia-induced cytoplasmic incompatibility phenotype. J. Cell Sci. 119, 3655–3663. doi: 10.1242/jcs.03095
Trienens, M., Giesbers, M., Pannebakker, B. A., Van De Zande, L., and Beukeboom, L. W. (2021). A re-assessment of within-host mating behavior in the Nasonia species complex. Entomol. Exp. Appl. 169, 1081–1091. doi: 10.1111/eea.13106
Van Den Assem, J., Jachmann, F., and Simbolotti, P. (1980). Courtship behavior of Nasonia vitripennis (Hym., Pteromalidae): some qualitative, experimental evidence for the role of pheromones. Behaviour 75, 301–307. doi: 10.1163/156853980X00456
Wang, X. Z., Kelkar, Y. D., Xiong, X., Martinson, E. O., Lynch, J., Zhang, C., et al. (2020). Genome report: whole genome sequence and annotation of the parasitoid jewel wasp Nasonia giraulti laboratory strain RV2X[u]. G3 Genes Genom. Genet. 10, 2565–2572. doi: 10.1534/g3.120.401200
Werren, J. H. (1983). Sex-ratio evolution under local mate competition in a parasitic wasp. Evolution 37, 116–124. doi: 10.1111/j.1558-5646.1983.tb05520.x
Werren, J. H., and Loehlin, D. W. (2009). The parasitoid wasp Nasonia: an emerging model system with haploid male genetics. Cold Spring Harbor Protoc. 2009, pdb.emo134. doi: 10.1101/pdb.emo134
Werren, J. H., Richards, S., Desjardins, C. A., Niehuis, O., Gadau, J., Colbourne, J. K., et al. (2010). Functional and evolutionary insights from the genomes of three parasitoid Nasonia species. Science 327, 343–348. doi: 10.1126/science.1178028
Keywords: mate finding, parasitic wasp, precopulatory isolation, sex attractant, species-specificity, (4R,5S)-5-hydroxydecanolide, (4R,5R)-5-hydroxydecanolide, 4-methylquinazoline
Citation: Kurtanovic A, Hofferberth J and Ruther J (2022) Male sex pheromone in the parasitoid wasp Nasonia longicornis: Chemical and behavioral analyses. Front. Ecol. Evol. 10:1076398. doi: 10.3389/fevo.2022.1076398
Edited by:
Angel Guerrero, Spanish National Research Council, SpainReviewed by:
Gerhard Johannes Gries, Simon Fraser University, CanadaStephen A. Teale, SUNY College of Environmental Science and Forestry, United States
Lawrence Hanks, University of Illinois at Urbana-Champaign, United States
Copyright © 2022 Kurtanovic, Hofferberth and Ruther. This is an open-access article distributed under the terms of the Creative Commons Attribution License (CC BY). The use, distribution or reproduction in other forums is permitted, provided the original author(s) and the copyright owner(s) are credited and that the original publication in this journal is cited, in accordance with accepted academic practice. No use, distribution or reproduction is permitted which does not comply with these terms.
*Correspondence: Joachim Ruther, am9hY2hpbS5ydXRoZXJAdXIuZGU=