- U.S. Geological Survey, Wetland and Aquatic Research Center, Fort Lauderdale, FL, United States
Biodiversity conservation under a changing climate is a challenging endeavor. Landscapes are shifting as a result of climate change and sea level rise but plant communities in particular may not keep up with the pace of change. Predictive ecological models can help decision makers understand how species are likely to respond to change and then adjust management actions to align with desired future conditions. Florida’s Everglades is a wetland ecosystem that is host to many species, including a large number of endangered and endemic species. Everglades ecosystem restoration has been ongoing for decades, but consideration of sea level rise impacts in restoration planning is more recent. Incorporating potential impacts from sea level rise into restoration planning should benefit species and their coastal habitats, most notably at the southern Florida peninsula. The endangered Cape Sable seaside sparrow (Ammospiza maritima mirabilis) occurs in marl prairie habitat at the southern end of the Everglades. The locations of three of its six subpopulations are proximate to the coast. We used a spatially explicit predictive model, EverSparrow, to estimate probability of sparrow presence considering both hydrologic change from restoration and sea level rise. We found that the probability of sparrow presence decreased with increasing sea level rise. Within approximately 50 years, probability of presence significantly decreased for all three coastal subpopulation areas, with areas above 40% probability increasingly limited. Given the exceptionally low dispersal ability of this species and the geographic restrictions for habitat expansion, our results highlight the importance of freshwater flow into the southern Everglades marl prairie for habitat conservation.
Introduction
Although the conservation approach has shifted over time from a single species focus toward a suite of species that inhabit a landscape, endangered species still receive special attention given their legal protection under the Endangered Species Act (16 U.S.C. §1531 et seq., 1973). Many approaches to biodiversity conservation have been tested over recent decades such as using umbrella or indicator species to represent communities (Noss, 1990) and implementing an ecosystem-based management approach to promote ecosystem function at the landscape scale (Kaufmann et al., 1994). Although endangered species are afforded legal protection, in some cases habitat management targeted toward one endangered species causes further population declines of other endangered species in the ecosystem, suggesting that approaches that improve ecosystem function as a whole could benefit multiple species within the ecosystem, including the endangered species that inhabit it (Simberloff, 1998).
Determining the most effective approaches for biodiversity conservation is even more challenging with changing environmental conditions. Management actions that are effective today might not be feasible in the future with a changing landscape. Further, plants may not be able to shift their ranges in response to changing climatic conditions as well as more mobile animals. Some vegetative communities would need to shift at a rate of 1 km/year to keep pace with climate change (Loarie et al., 2009). In areas with high human population growth and urban encroachment like Florida, these conservation challenges are even greater (Terando et al., 2014) and can result in habitat fragmentation, which is particularly concerning for Florida’s high number of endemic and endangered species (Benscoter et al., 2013).
Predictive ecological modeling can help conservation practitioners identify management actions to achieve desired future ecological conditions. The field of spatial ecology has grown tremendously with many spatially explicit approaches available to address conservation challenges (Fletcher and Fortin, 2018). Alongside these advances, projections of climatic conditions have been refined and downscaled to finer resolutions for more meaningful evaluations of potential future conditions (Tabor and Williams, 2010; Wang et al., 2016). Florida, and in particular the Greater Everglades ecosystem (hereafter “Everglades”), has the advantage of being data-rich, providing strength to analyses on the ecological relationships within. Many of these well-understood relationships have been turned into ecological models to assist with restoration and conservation planning in the Everglades (Romañach and Pearlstine, 2022).
Decades of environmental degradation in the Everglades have resulted in one of the largest restoration programs in the world, authorized by Congress in 2000 (Public Law 106-541 – Water Resources Development Act of 2000). The Everglades wetland was once a large “river of grass” with freshwater slowly flowing southward across the landscape toward the coast (Douglas, 1947). Residential and agricultural development beginning in the 1880s degraded and divided the wetland using a system of canals and levees (Light and Dineen, 1994). Further, the geographical position of the Everglades at the southern end of the Florida peninsula makes rising seas a threat that is already resulting in habitat shifts (Krauss et al., 2011). Rapidly advancing seas led the federal partner in Everglades restoration, the U.S. Army Corps of Engineers, to call for the consideration of sea level rise in all future project planning (United States Army Corps of Engineers Regulation 1100-2-8162, 2019). Everglades restoration is aimed at “getting the water right” for the suite of species that inhabit the wetland such as wading birds (Ciconiiformes) and the American alligator (Alligator mississippiensis). However, hydrologic and habitat needs vary by species and engendered species are prioritized to avoid further jeopardizing their populations and habitat (Romañach et al., 2022b).
The endangered Cape Sable seaside sparrow (Ammospiza maritima mirabilis; hereafter “sparrow”) is an endemic species in the Everglades that inhabits marl prairie, along with other listed species such as the Florida panther (Puma concolor coryi) and the wood stork (Mycteria americana). Marl prairie habitat is limited in its spatial range and bounded by coastlines to the south and west and infrastructure to the north and east (Benscoter and Romañach, 2022). The sparrow population has declined by approximately 63% since the early 1990s to fewer than 2,500 birds in 2021 (Virzi and Tafoya, 2021; Benscoter and Romañach, 2022). They are found in six subpopulations (named A–F) in the southern Everglades and are relatively sedentary with limited movements within and between subpopulations (Lockwood et al., 2001). The sparrow is a ground nesting bird that requires treeless short-hydroperiod wetland conditions that result in marl prairie to reproduce successfully (Davis et al., 2005). There are many threats to this species including nest flooding, water management regimes, breeding season fires, and woody vegetation encroachment (Benscoter et al., 2021; Benscoter and Romañach, 2022). A steep decline in bird count for subpopulation A was observed over the last 30 years, down to an estimated 0 birds observed in the range-wide point count surveys in 2021 (Benscoter and Romañach, 2022). The number of birds in subpopulations C, D, and F is relatively low and has declined since 1981 (although subpopulation D has shown a recent increase), while subpopulations B and E have relatively stable and higher number of birds (Benscoter et al., 2021; Benscoter and Romañach, 2022). Subpopulation estimates for 2021 are: A, 0; B, 1,488; C, 112; D, 288; E, 528; and F, 32 (Benscoter and Romañach, 2022). Given the severe declines in population size of this endangered species, and spatial limitations for expansion of habitat, questions remain about the spatial extent of habitat in the future.
Model development in the Everglades has advanced over the last few years and can provide insight into future conditions for the sparrow. The sparrow is difficult to observe and requires costly helicopter transport to access remote wilderness areas for population monitoring (Walters et al., 2000; Pimm et al., 2002). Predictive modeling can leverage these existing data to target outputs toward management questions for this declining species. Predictive models for sparrow habitat are used in Everglades restoration planning (Romañach and Pearlstine, 2022) but less utilized for conservation planning. EverSparrow is a spatially explicit model that provides estimates of potential sparrow habitat via probability of sparrow presence that can help natural resource managers target conservation action (Haider et al., 2021). EverSparrow models a range of environmental factors that are related to sparrow presence including water depth, fire history, and vegetation structure. Although hydrologic projections do not exist to examine future conditions with climate change, hydrologic projections for ecosystem response to restoration exist, as well as sea level rise projections.
Our research examines the current and future habitat conditions for the Cape Sable seaside sparrow and considers the ongoing ecosystem restoration and potential impacts from sea level rise. Everglades restoration will take decades to implement (National Research Council, 2003), therefore, natural resource managers want to know where suitable areas for sparrows will be in 50 years, after full implementation of Everglades restoration projects, and projections showing sea levels continuing to rise. Here we use the predictive model EverSparrow to gain insight into current and future potential habitat areas for the sparrow. The outputs of this study can help inform the National Park Service’s resist-accept-direct (RAD) framework to make strategic decisions about sparrow habitat management under changing conditions (Schuurman et al., 2020).
Materials and methods
Study area
Our study area covers the geographic extent of six sparrow subpopulations (A–F), located mainly in Everglades National Park and Big Cypress National Preserve (Figure 1). The extent of the study area was determined by the footprint of available hydrology. The western and southern edges of the study area approach the coast along the Gulf of Mexico and Florida Bay, respectively. We used a 400 m × 400 m grid cell size for all modeling, matching the grid cell size used in EverSparrow development.
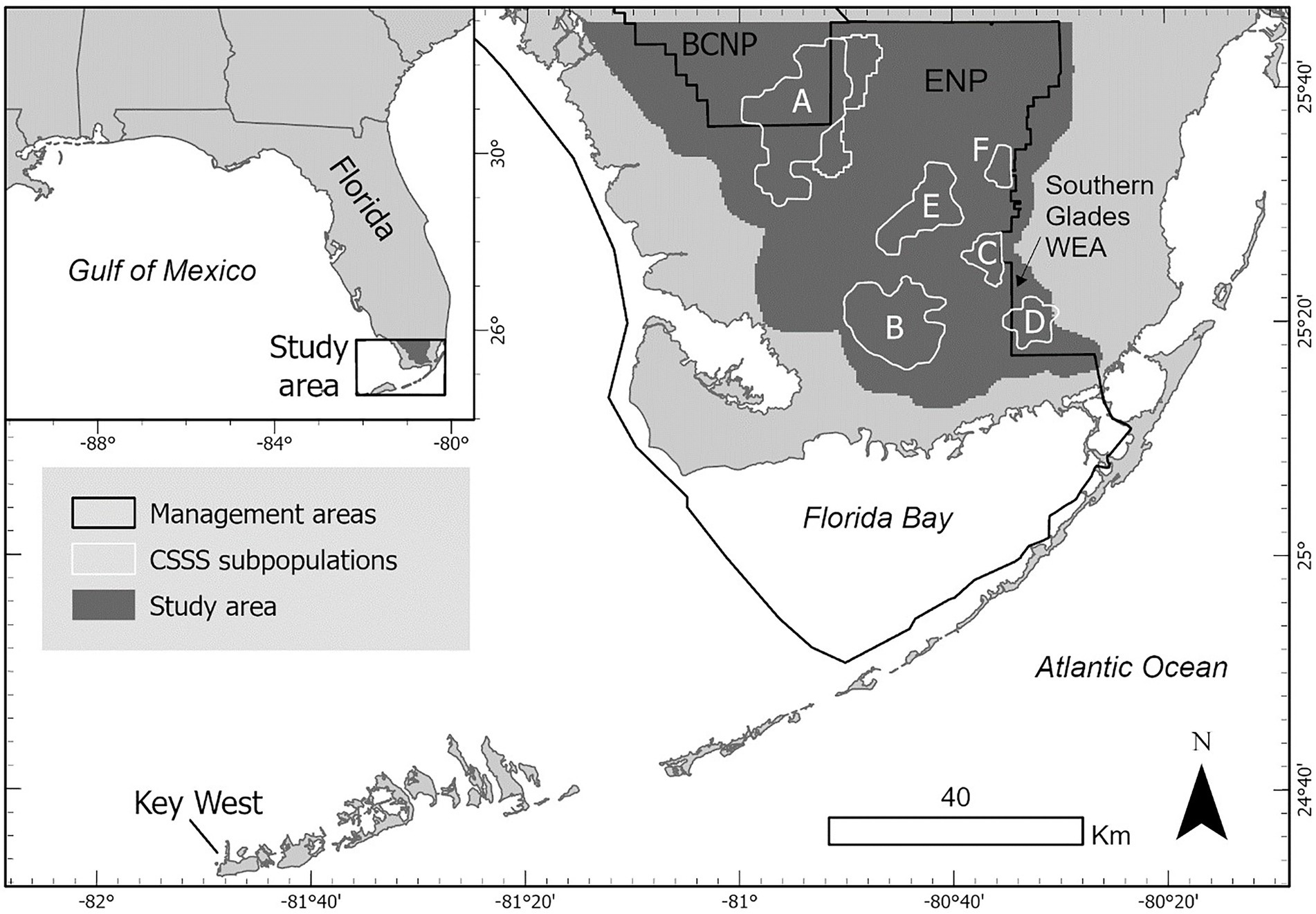
Figure 1. Map of the study area and overlap with management areas Everglades National Park (ENP), Big Cypress National Preserve (BCNP), and Southern Glades Wildlife and Environmental Area (WEA).
Environmental variables
EverSparrow requires four environmental variables as model inputs: years since last fire, percent burned at last fire, percent canopy cover, and percent woody vegetation. Fire history is incorporated into the model due to its importance for marl prairie vegetation and relationship to sparrow occupancy and bird count (Benscoter et al., 2019; Benscoter and Romañach, 2022). Previous studies indicate that fire every 4–11 years is related to the highest levels of sparrow occupancy or bird count (La Puma, 2010; Benscoter et al., 2019). Marl prairie vegetation can recover from fire within 2–4 years, although vegetation recovery can take longer if flooding occurs post-fire (La Puma et al., 2007; Sah et al., 2015). We calculated fire history from the Everglades National Park fire database which contains fire locations and extent from 1983 through 2020 (available by request from Everglades National Park). We determined the number of years since last fire and percent of cell burned in each 400 × 400 m grid cell. Because we calculate up to 10 years since the last fire, fire history available to EverSparrow begins in 1993.
Sparrows reside in areas with low tree cover or woody vegetation (Benscoter et al., 2021; Benscoter and Romañach, 2022), and the probability of sparrow presence is highest where percent canopy cover is lower than 10% and percent woody vegetation is lower than 25% (Haider et al., 2021); these variables are included in the model to spatially indicate their absence. We calculated canopy cover from the Multi-Resolution Land Characteristics Consortium percent tree canopy cover data set (30 m resolution) in the 2011 National Land Cover Database (U.S. Forest Service, 2019). For woody vegetation, we used the Cooperative Land Cover Map version 1.1 from the Florida Natural Areas Inventory and Florida Fish and Wildlife Conservation Commission (2010) (re-sampled to 400 × 400 m). More detail about the environmental variables can be found in Haider et al. (2021).
Hydrology
In addition to environmental variables, EverSparrow requires two hydrologic variables that are derived from daily water depths: hydroperiod and days since last dry. Hydroperiod is the number of discontinuous wet days in a climatic year (May 1–April 30) averaged over 4 years. Days since dry is a count of how long a grid cell has been wet, counting backwards to a maximum of 3 years or until the cell dries; it is calculated on a weekly time step using the first day of the seven-day time step. These variables are included in the model due to their impact on vegetation type and sparrow habitat (Benscoter et al., 2021; Benscoter and Romañach, 2022).
Water management in the Everglades changes over the years and follows a regulation schedule, which is a document that defines operational plans for water infrastructure (such as pumps and other constructed features). Because operational plans change over time, it would be inaccurate to use historical hydrology to estimate future conditions. Therefore, we used a hydrologic model of the current operations plan as the baseline of our model projection. At the time of writing, water operations in the Everglades study area are managed according to a regulation schedule named “ALTQ” from the Combined Operational Plan (COP). COP is aimed at restoring hydrologic conditions in Everglades National Park through changes to timing, location, and volume of water deliveries from the north. Water deliveries should benefit the Park broadly, including hydrologic restoration of marl prairie (U.S. Fish and Wildlife Service, 2020). The South Florida Water Management District developed the Regional Simulation Model and uses it to produce historical hydrologic scenarios but with current regulation schedules implemented. We selected their hydrologic scenario No Action 2022 (NA22f) as the baseline hydrology to estimate current sparrow conditions. NA22f includes the ALTQ water regulation schedule and has a period of record modeling daily water depths from 1965 to 2016. We resampled NA22f to the 400 m × 400 m grid and calculated hydroperiod and days since dry from 1969 to 2016.
Sea level rise
We used sea level rise (SLR) projections from the University of Florida’s GeoPlan Center. The GeoPlan Center used the U.S. Army Corps of Engineers (USACE) Sea-Level Change Curve Calculator (version 2019.21; SLCCC) which determines the annual value of SLR at tidal gauges, the nearest of which was Key West for our study area.1 The GeoPlan Center used these values to model inundation surfaces (5.4 m resolution) on the decadal time scale for all coastal counties of Florida using a modified bathtub approach with a hydrologic connectivity filter that removed areas isolated from major waterways.2 Modeled outputs include estimations of extent and depth of inundation due to SLR. We selected the USACE intermediate and high SLR projections which show values of approximately 0.24 m (0.8 ft) and 0.73 m (2.4 ft), respectively, in 50 years (in the year 2066; USACE, 2013).
At the time of writing, no hydrologic models exist of daily water depth that incorporate SLR in our study area. Therefore, to examine potential future conditions for the sparrow we combined an existing hydrologic model (NA22f) with the GeoPlan Center’s decadal SLR projections. Our approach was to add GeoPlan’s spatially explicit inundation depths of SLR to the water levels in NA22f. First, we resampled the SLR inundation surfaces to the 400 m × 400 m grid by calculating mean water depth per grid cell using the Spatial Analyst tools in ArcGIS Pro 2.9.1. Next, using the statistical software program R (R Core Team, 2021), we translated the spatially explicit GeoPlan Center’s SLR inundation layers, which are on a decadal scale, to an annual scale. The USACE SLCCC provides annual estimates of SLR for south Florida which we used to calculate the relative proportion of sea level increase annually because SLR does not increase linearly with time. We used this proportional fraction to temporally disaggregate the GeoPlan Center’s decadal surfaces into annual inundation surfaces. For example, if the USACE SLCCC projected a rise of 0.01 m from year 1 to year 2 and an increase of 0.30 m from year 1 to year 10, then we calculated that the proportion of SLR in the first year of a 10-year time span was 0.01 m/0.30 m = 0.03. We then multiplied the decadal SLR inundation surface by 0.03 to calculate the annual level of SLR for that first year. Last, we added the annual sea level increase to the NA22f water levels, using this process for both the USACE intermediate and high projections.
Statistical analysis
EverSparrow is a spatially explicit Bayesian logistic regression model that estimates sparrow probability of presence by determining relationships between sparrow presence from point count survey data and environmental variables, described in detail in Haider et al. (2021) We ran the EverSparrow model on three hydrologic scenarios: (1) NA22f (baseline without SLR), (2) NA22f plus USACE intermediate SLR inundation (0.24 m SLR in 50 years), and (3) NA22f plus USACE high SLR inundation (0.73 m SLR in 50 years). We evaluated the differences in predicted probability of sparrow presence within the areas of subpopulations A, B, and D. We selected these three areas because they are closest to the coast and most likely to be impacted by SLR. To compare the scenarios, we calculated the mean probability of sparrow presence per grid cell over the breeding season (March–June) for each year. We also calculated the 75th quantile over the breeding season, per grid cell for each year. We chose the 75th quantile to examine changes in higher suitability CSSS habitat, while not too high as to be impacted by outlier values. We tested differences using R (R Core Team, 2021) by running a two-way analysis of variance (ANOVA) with hydrologic scenarios and subpopulations as interactive factors. For pair-wise comparisons, we ran a Tukey’s Honest Significant Difference (HSD).
Results
For all three sparrow subpopulations (A, B, and D), the mean EverSparrow probability of presence decreased with increasing SLR (Figure 2). All outputs are available online as a U.S. Geological Survey data release (Romañach et al., 2022a). The two-way ANOVA showed that the interaction of subpopulation and hydrologic scenario had a statistically significant effect on mean probability of sparrow presence (F(4, 234,423) = 4,754, p < 2−16). Main effects of both subpopulation and scenario on probability of presence were significant (p < 2−16). The Tukey’s HSD test showed that the mean probability of presence values between the baseline hydrologic (NA22f) and USACE intermediate SLR scenarios were significantly different for subpopulation A (p = 0, 95% CI = [−0.009, −0.003]) and subpopulation B (p = 0, 95% CI = [−0.078, −0.070]). Between the USACE intermediate and high scenarios, the Tukey’s HSD test also showed that the mean probability of presence was significantly different for subpopulation A (p = 0.01, 95% CI = [−0.006, −0.001]), subpopulation B (p = 0, 95% CI = [−0.155, −0.147]), and subpopulation D (p = 0, 95% CI = [−0.119, −0.103]).
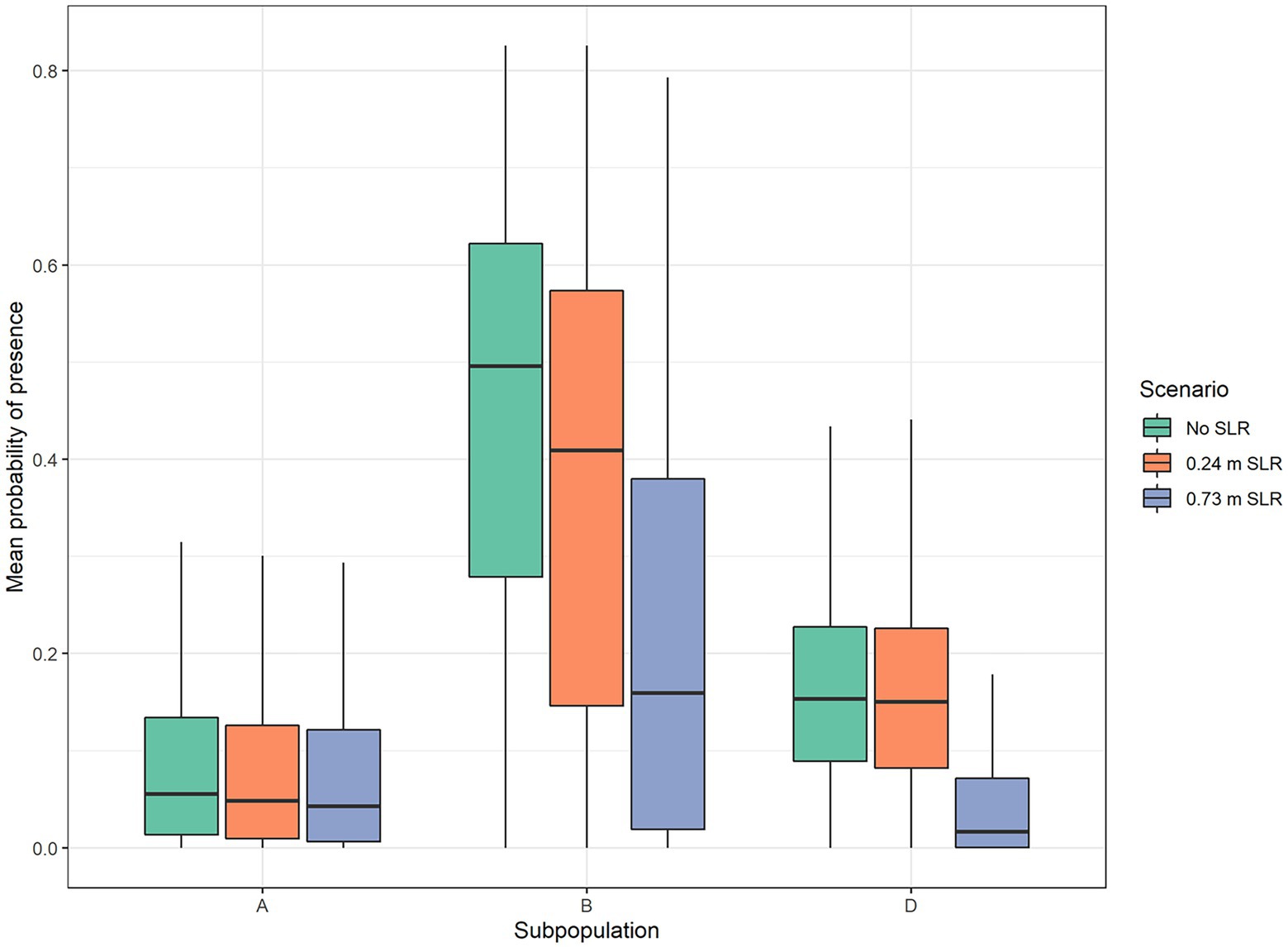
Figure 2. Boxplot showing the EverSparrow mean probability of presence for the Cape Sable seaside sparrow (Ammospiza maritima mirabilis) for the areas of subpopulations A, B, and D for three hydrologic scenarios: a baseline (NA22f) without sea level rise (SLR), the U.S. Army Corps of Engineers (USACE) intermediate SLR scenario (0.24 m in 50 years), and the USACE high SLR scenario (0.73 m in 50 years). Mean probability of presence values were averaged over the breeding season (March–June).
For the last year of the three scenarios, we calculated the mean probability of presence during the breeding season (March–June) over the study area to examine the spatial distribution of the impact of SLR on potential sparrow habitat (Figure 3). The mean sparrow probability of presence in the areas of subpopulations A and B decreases from the baseline to the 0.24 m SLR, and also decreases in the areas of subpopulations A, B, and D from 0.24 m SLR to 0.73 m SLR. With 0.73 m SLR, almost all areas within subpopulation D have less than 10% probability of sparrow presence, while the area of subpopulation B only has probabilities of presence above 10% in the northcentral and northeastern areas. Although the more inland subpopulations were not a focus of this study, we report little change in probability of presence values in the locations of subpopulations C, E, and F for the SLR scenarios, with the exception of the southwestern portion of subpopulation E in the 0.73 m SLR scenario.
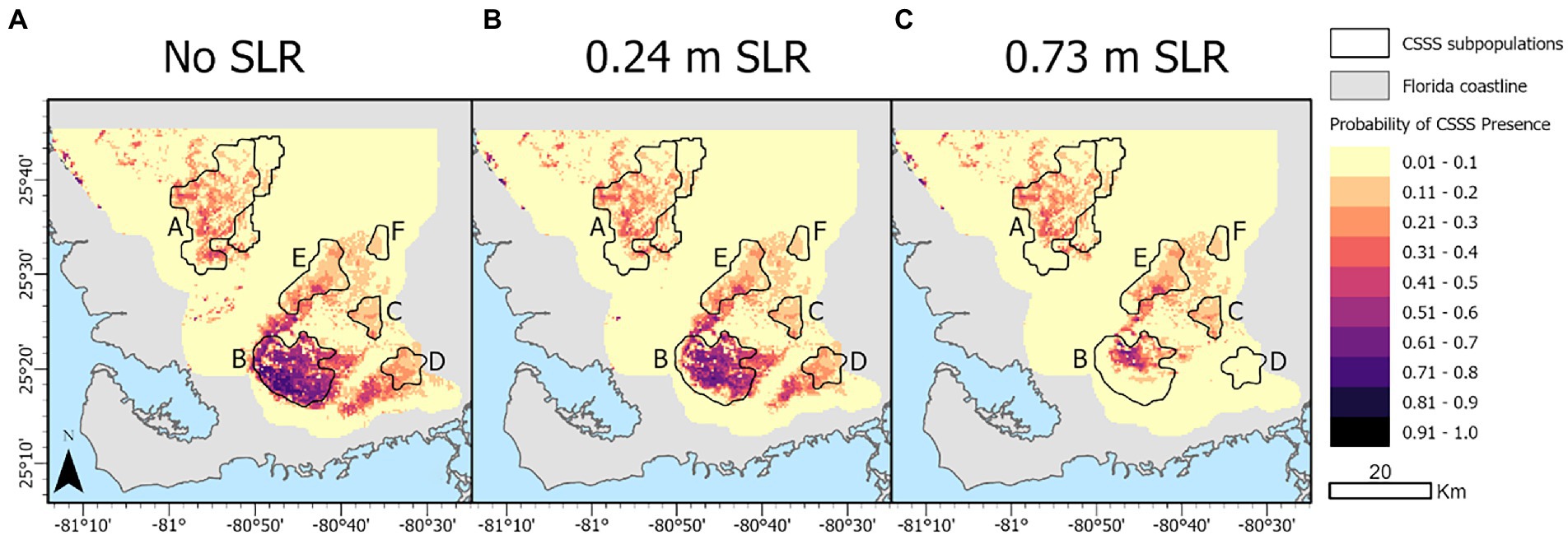
Figure 3. Maps showing the EverSparrow mean probability of presence for the Cape Sable seaside sparrow (Ammospiza maritima mirabilis; CSSS) during the breeding season (March – June) for the last year of three hydrologic scenarios: (A) a baseline (NA22f) without sea level rise (SLR), (B) the U.S. Army Corps of Engineers (USACE) intermediate SLR scenario (0.24 m in 50 years), and (C) the USACE high SLR scenario (0.73 m in 50 years).
Discussion
The Cape Sable seaside sparrow is endemic to the marl prairie of the Everglades and its range is unlikely to expand given the hard boundaries of coastline to the south and west, urbanization to the east, and water retention areas to the north. Our results show that the probability of presence for the sparrow is likely to decline in the coastal subpopulations with increasing sea level rise. Our findings are in line with previous studies showing a reduction in available habitat for salt marsh sparrows due to sea level rise (Ammodramus maritimus; Shriver and Gibbs, 2004; Kern and Shriver, 2014; Hunter et al., 2015). As the delineation between freshwater habitats and the sea becomes less clear, species distributions are changing as a result (Pressey et al., 2007). This challenge to adapt is greater for species with limited ranges, and even more so for those with limited dispersal abilities like the sparrow.
All subspecies of seaside sparrows tend to show low movement and dispersal, making it critical to conserve the habitats they currently occupy (Rising, 2005). The Cape Sable seaside sparrow occupies six subpopulation areas with half of those vulnerable to sea level rise because of their proximity to the coast. Although juveniles disperse up to 1 km, they tend to move under 600 m from their natal sites (Lockwood et al., 2001). For context, the distance between the boundaries of subpopulation areas B and D is approximately 9 km, and most subpopulations are at least 4 km apart. The average movement distance of 14 banded adult sparrows was 277 m from one breeding season to the next (Pimm et al., 2002). A study using mark-resight data from 1997 to 2007 found only eight instances of juveniles or adults moving among subpopulations (Boulton et al., 2009). Although longer distance movements are rare, sparrows have settled in new areas 3–7 km away (Dean and Morrison, 2001). However, because of their low dispersal ability, sparrows may not re-colonize new suitable areas (Jenkins et al., 2003). Translocation is an option listed in an emergency management action plan for the sparrow to help reduce the risk of extirpation (Slater et al., 2009). However, successful translocation is contingent upon the reduction or elimination of threats leading to population declines, to allow for persistence (IUCN/SSC, 2013).
In addition to range constraints, hydrologic requirements for this ground nesting bird pose conservation challenges to water managers. Because the sparrow is protected by the Endangered Species Act, water management decisions must consider impacts to sparrows and their habitat. The area for subpopulation A was excluded from critical habitat designation so that water levels would not require maintenance at “unnaturally low” conditions for the potential benefit of sparrows, and instead allow for broader ecosystem level benefits from restoration (Federal Register, 2007). When the water structures on the northern boundary of subpopulation A are closed to maintain low water levels for the benefit of the sparrow, water levels can become too high in the area to the north and lead to suboptimal conditions for other species, including another endangered bird, the Everglade snail kite [Rostrhamus sociabilis plumbeus; Kitchens et al., 2002; USACE (U.S. Army Corps of Engineers), 2020].
Although water depth is not the only factor impacting these endangered species, restoration and water management can influence recovery, including for the sparrow. Under the broad Everglades restoration objective to “get the water right,” one aim is to send more water southward across the landscape as once occurred naturally before water was diverted away from the central and southern Everglades through a system of canals (Davis and Ogden, 1994). The Everglades is heavily managed whereby water is moved across wetland compartments using infrastructure such as canals, pumps, and gates. Because of the narrow hydrologic band to create and sustain marl prairie habitat conditions for sparrows during the breeding season, the current water operations plan, COP, provides flexibility for operations, for example delayed opening or closing of water control structures to the north of sparrow habitat (U.S. Fish and Wildlife Service, 2020). Although not all subpopulation areas will benefit evenly, overall, sparrow habitat is expected to benefit from the current water operations plan (U.S. Fish and Wildlife Service, 2020). Under COP, the area housing subpopulation E is becoming wetter (Sah et al., 2021) and is expected to become less suitable for sparrows as a result (U.S. Fish and Wildlife Service, 2020). The area for subpopulation E holds the second highest sparrow population numbers (after subpopulation B; U.S. Fish and Wildlife Service, 2020) and is situated inland from the coastal subpopulations that we considered in our analysis. Implementing water management and restoration strategies that provide appropriate hydrologic conditions for the sparrow in subpopulation E could have an added benefit of providing a refuge from SLR.
Impacts from sea level rise are already evident in southern Florida through vegetative shifts and landscape level change (Ross et al., 2000; Krauss et al., 2011; Smith et al., 2013). Many studies have investigated the effects of increased salinity on freshwater marsh communities, which are wetter than the freshwater prairie the sparrow occupies (Ross et al., 2006). Studies have examined salinity tolerances of freshwater marsh species and mechanisms for movement of mangroves into marshes (McKee and Mendelssohn, 1989; Howard and Mendelssohn, 2000; Jiang et al., 2014). The movement and intrusion of salt-tolerant mangroves into freshwater marsh communities with increased salinity is well documented in south Florida (Ross et al., 2000; Krauss et al., 2011; Smith et al., 2013). Additional studies are warranted on the effects of saltwater intrusion on the freshwater prairie communities (e.g., Muhlenbergia) occupied by the sparrow. However, mangroves are already reported as encroaching into the southern portions of subpopulations B and D (Sah et al., 2020; Benscoter et al., 2021; Benscoter and Romañach, 2022). Continued sea level rise could also make freshwater marsh and wet prairie communities more susceptible to negative effects of hurricanes (e.g., Alexander, 1967), especially if there are severe storm surges that transport large amounts of saltwater inland (Jiang et al., 2014). As the presence of breeding sparrows is considered an indicator of marl prairie condition (Elderd and Nott, 2008), integrating marl prairie succession and underlying marl substrate dynamics with SLR has the potential to help natural resources managers gain insight toward water and habitat management for the sparrow.
Achieving freshwater flow and water depths for successful breeding may allow for the persistence of the Cape Sable seaside sparrow in the near term. However, at the current rate of SLR, sparrow habitat is projected to continue to shrink, and our results show a significant projected decline in probability of sparrow presence within 50 years. Given rates of SLR, the effectiveness of freshwater flow into Everglades National Park to reduce salinity depends on the timing of water releases from the retention areas to the north of Everglades National Park, whereby releases earlier in the dry season are better able to reduce salinity (Dessu et al., 2018). Although climate change mitigation and increased freshwater flow can reduce undesired impacts from SLR, vegetation communities are already changing on the landscape. With these changes already evident and climate change not explicitly addressed in the Endangered Species Act, natural resource agencies may face a short timescale to determine best practices for endangered species management under changing environmental conditions.
Data availability statement
The raw data supporting the conclusions of this article will be made available by the authors, without undue reservation.
Author contributions
SR conceived of the research and led the writing. SH conducted the analyses. AB conducted the literature review from which the idea was conceived. All authors contributed to the article and approved the submitted version.
Funding
Funding for this work was provided by the U.S. Geological Survey’s Greater Everglades Priority Ecosystems Science program.
Acknowledgments
We thank T. Hopkins and K. Palmer at the U.S. Fish and Wildlife Service for their review comments to improve the manuscript. Any use of trade, firm, or product names is for descriptive purposes only and does not imply endorsement by the U.S. Government.
Conflict of interest
The authors declare that the research was conducted in the absence of any commercial or financial relationships that could be construed as a potential conflict of interest.
The handling editor RW declared a past collaboration with the author SR.
Publisher’s note
All claims expressed in this article are solely those of the authors and do not necessarily represent those of their affiliated organizations, or those of the publisher, the editors and the reviewers. Any product that may be evaluated in this article, or claim that may be made by its manufacturer, is not guaranteed or endorsed by the publisher.
Footnotes
References
Alexander, T. R. (1967). Effect of hurricane Betsy on the southeastern Everglades. Q. J. Fla. Acad. Sci. 30, 10–24.
Benscoter, A. M., Beerens, J. M., Pearlstine, L. G., and Romañach, S. S. (2019). Fire disturbance influences endangered cape sable seaside sparrow (Ammospiza maritima mirabilis) relative bird count. Conserv. Sci. Pract. 1:e130. doi: 10.1111/csp2.130
Benscoter, A. M., Haider, S. M., Guilbeau, K. G., and Romañach, S. S. (2021). Knowledge synthesis of cape sable seaside sparrow science. Report to the U.S. Fish and Wildlife Service, Florida Ecological Services Field Office.
Benscoter, A. M., Reece, J. S., Noss, R. F., Brandt, L. A., Mazzotti, F. J., Romañach, S. S., et al. (2013). Threatened and endangered subspecies with vulnerable ecological traits also have high susceptibility to sea level rise and habitat fragmentation. PLoS One 8:e70647. doi: 10.1371/journal.pone.0070647
Benscoter, A. M., and Romañach, S. S. (2022). Endangered cape sable seaside sparrow ecology: actions towards recovery through landscape-scale ecosystem restoration. Endanger. Species Res. 49, 199–215. doi: 10.3354/esr01212
Boulton, R. L., Lockwood, J. L., Davis, M. J., Pedziwilk, A., Boadway, K. A., Boadway, J. J. T., et al. (2009). Endangered cape sable seaside sparrow survival. J. Wildl. Manag. 73, 530–537. doi: 10.2193/2007-467
Davis, S. M., and Ogden, J. C. (1994). Everglades: The Ecosystem and Its Restoration. Delray Beach, FL: St Lucie Press.
Davis, S. M., Gaiser, E. E., Loftus, W. F., and Huffman, A. E. (2005). Southern marl prairies conceptual ecological model. Wetlands 25, 821–831. doi: 10.1672/0277-5212(2005)025[0821:SMPCEM]2.0.CO;2
Dean, T. F., and Morrison, J. L. (2001). Non-breeding season ecology of the cape sable seaside sparrow (Ammodramus maritimus mirabilis). Final Report. Hartford, CT: Eagle Environmental, Inc. Submitted to Vero Beach, FL: U.S. Fish and Wildlife Service, South Florida Ecosystem Office.
Dessu, S. B., Price, R. M., Troxler, T. G., and Kominoski, J. S. (2018). Effects of sea-level rise and freshwater management on long-term water levels and water quality in the Florida Coastal Everglades. J. Environ. Manag. 211, 164–176. doi: 10.1016/j.jenvman.2018.01.025
Douglas, M. S. (1947). The Everglades: River of Grass, America’s Unique Natural Treasure. Marietta, GA: Mockingbird Books.
Elderd, B. D., and Nott, M. P. (2008). Hydrology, habitat change and population demography: an individual-based model for the endangered cape sable seaside sparrow Ammodramus maritimus mirabilis. J. Appl. Ecol. 45, 258–268. doi: 10.1111/j.1365-2664.2007.01369.x
Federal Register (2007). Endangered and Threatened Wildlife and Plants; Critical Habitat Revised Designation for the Cape Sable Seaside Sparrow; Final Rule. 50 CFR Part 17. November 6, 2007.
Fletcher, R., and Fortin, M. (2018). Spatial Ecology and Conservation Modeling. Applications with R. Cham, Switzerland: Springer International Publishing.
Florida Natural Areas Inventory and Florida Fish and Wildlife Conservation Commission (2010). Development of a Cooperative Land Cover Map: Final Report. Florida’s Wildlife Legacy Initiative Project 08009. Florida Natural Areas Inventory.
Haider, S. M., Benscoter, A. M., Pearlstine, L., D’Acunto, L. E., and Romañach, S. S. (2021). Landscape-scale drivers of endangered cape sable seaside sparrow (Ammospiza maritima mirabilis) presence using an ensemble modeling approach. Ecol. Model. 461:109774. doi: 10.1016/j.ecolmodel.2021.109774
Howard, R. J., and Mendelssohn, I. A. (2000). Structure and composition of oligohaline marsh plant communities exposed to salinity pulses. Aquat. Bot. 68, 143–164. doi: 10.1016/S0304-3770(00)00108-X
Hunter, E. A., Nibbelink, N. P., Alexander, C. R., Barrett, K., Mengak, L. F., Guy, R. K., et al. (2015). Coastal vertebrate exposure to predicted habitat changes due to sea level rise. Environ. Manag. 56, 1528–1537. doi: 10.1007/s00267-015-0580-3
IUCN/SSC (2013). Guidelines for Reintroductions and Other Conservation Translocations. Version 1.0. Gland, Switzerland: IUCN Species Survival Commission, viiii + 57 pp.
Jenkins, C. N., Powell, R. D., Bass, O. L. Jr., and Pimm, S. L. (2003). Why sparrow distributions do not match model predictions. Anim. Conserv. 6, 39–46. doi: 10.1017/S1367943003003068
Jiang, J., DeAngelis, D. L., Anderson, G. H., and Smith, T. J. III. (2014). Analysis and simulation of propagule dispersal and salinity intrusion from storm surge on the movement of a marsh–mangrove ecotone in South Florida. Estuaries Coast 37, 24–35. doi: 10.1007/s12237-013-9666-4
Kaufmann, M. R., Graham, R. T., Boyce, D. A. Jr., Moir, W. H., Perry, L., Reynolds, R. T., et al. (1994). An Ecological Basis for Ecosystem Management. Gen. Tech. Rep. RM 246. Fort Collins, CO: U.S. Department of Agriculture, Forest Service, Rocky Mountain Forest and Range Experiment Station.
Kern, R. A., and Shriver, W. G. (2014). Sea level rise and prescribed fire management: implications for seaside sparrow population viability. Biol. Conserv. 173, 24–31. doi: 10.1016/j.biocon.2014.03.007
Kitchens, W. M., Bennetts, R. E., and DeAngelis, D. L. (2002). “Linkages between the snail kite population and wetland dynamics in a highly fragmented South Florida hydroscape” in The Everglades, Florida Bay, and Coral Reefs of the Florida Keys: An Ecosystem Sourcebook. eds. J. W. Porter and K. G. Porter (Delray Beach, FL: CRC/St. Lucie Press), 183–203.
Krauss, K. W., From, A. S., Doyle, T. W., Doyle, T. J., and Barry, M. J. (2011). Sea-level rise and landscape change influence mangrove encroachment onto marsh in the ten Thousand Islands region of Florida, USA. J. Coast. Conserv. 15, 629–638. doi: 10.1007/s11852-011-0153-4
La Puma, D. A. (2010). Come hell or high water: conservation of the federally endangered cape sable seaside sparrow (Ammodramus maritimus mirabilis) in the dynamic Florida Everglades. PhD dissertation. New Brunswick, NJ: Rutgers University.
La Puma, D. A., Lockwood, J. L., and Davis, M. J. (2007). Endangered species management requires a new look at the benefit of fire: the cape sable seaside sparrow in the Everglades ecosystem. Biol. Conserv. 136, 398–407. doi: 10.1016/j.biocon.2006.12.005
Light, S. S., and Dineen, J. W. (1994). “Water control in the Everglades: a historical perspective” in Everglades: The Ecosystem and Its Restoration. eds. S. M. Davis and J. C. Ogden (Delray Beach, Florida: St Lucie Press), 47–84.
Loarie, S. R., Duffy, P. B., Hamilton, H., Asner, G. P., Field, C. B., and Ackerly, D. D. (2009). The velocity of climate change. Nature 462, 1052–1055. doi: 10.1038/nature08649
Lockwood, J. L., Fenn, K. H., Caudill, J. M., Okines, D., Bass, O. L., Duncan, J. R., et al. (2001). The implications of cape sable seaside sparrow demography for Everglades restoration. Anim. Conserv. 4, 275–281. doi: 10.1017/S1367943001001329
McKee, K. L., and Mendelssohn, I. A. (1989). Response of a freshwater marsh plant community to increased salinity and increased water level. Aquat. Bot. 34, 301–316. doi: 10.1016/0304-3770(89)90074-0
National Research Council (2003). Adaptive monitoring and assessment for the comprehensive Everglades restoration plan. Washington, D.C.: The National Academies Press.
Noss, R. F. (1990). Indicators for monitoring biodiversity: a hierarchical approach. Conserv. Biol. 4, 355–364. doi: 10.1111/j.1523-1739.1990.tb00309.x
Pimm, S. L., Lockwood, J. L., Jenkins, C. N., Curnutt, J. L., Nott, M. P., Powell, R. D., et al. (2002). Sparrow in the Grass. Homestead, FL: National Park Service, Everglades National Park.
Pressey, R. L., Cabeza, M., Watts, M. E., Cowling, R. M., and Wilson, K. A. (2007). Conservation planning in a changing world. Trends Ecol. Evol. 22, 583–592. doi: 10.1016/j.tree.2007.10.001
R Core Team (2021). R: A language and environment for statistical computing. Vienna, Austria: R Foundation for Statistical Computing. Available at: https://www.R-project.org/ (Accessed February 28, 2022).
Romañach, S. S., and Pearlstine, L. G. (2022). “Forecasting ecological responses for wetland restoration planning in Florida’s Everglades,” in Imperiled: The Encyclopedia of Conservation. Vol. 2. eds. D. A. DellaSala and M. I. Goldstein (Elsevier), 371–380.
Romañach, S. S., Haider, S. M., and Benscoter, A. M. (2022a). Sea level rise scenarios for the Cape Sable seaside sparrow: U.S. Geological Survey data release.doi: 10.5066/P9KJDZXZ
Romañach, S. S., Haider, S. M., Hackett, C., McKelvy, M., and Pearlstine, L. G. (2022b). Managing multiple species with conflicting needs in the Greater Everglades. Ecol. Indic. 136:108669. doi: 10.1016/j.ecolind.2022.108669
Ross, M. S., Meeder, J. F., Sah, J. P., Ruiz, P. L., and Telesnicki, G. J. (2000). The southeast saline Everglades revisited: 50 years of coastal vegetation change. J. Veg. Sci. 11, 101–112. doi: 10.2307/3236781
Ross, M. S., Sah, J. P., Ruiz, P. L., Jones, D. T., Cooley, H., Travieso, R., et al. (2006). Effect of hydrologic restoration on the habitat of the cape sable seaside sparrow. Annual Report of 2004–2005. Submitted to National Park Service, Everglades National Park. Miami, FL: Florida International University and Ochopee, FL: U.S. Geological Survey, Ochopee, FL. Available at: https://digitalcommons.fiu.edu/sercrp/85/ (Accessed May 15, 2022).
Sah, J. P., Ross, M. S., Pulido, C., Stoffella, S., and Vidales, R. (2020). Landscape pattern – marl prairie/Slough gradient; patterns and trends in shark Slough marshes and associated marl prairies. Year 5 report (2014–2019). Submitted to U.S. Army Engineer Research and Development Center, Vicksburg, MS. Miami, FL: Florida International University. Available at: http://softel.fiu.edu/reports/MAP_MP-S_Year5_2020.pdf (Accessed July 5, 2022).
Sah, J. P., Ross, M. S., Snyder, J. R., Stofella, S., Blanco, J., and Freixa, J. (2015). Re-sampling of vegetation survey sites within cape sable seaside sparrow habitat. Submitted to homestead, FL: South Florida Natural Resources Center, National Park Service. Available at: http://softel.fiu.edu/reports/CSSS_Veg_2015.pdf (Accessed June 13, 2022).
Sah, J. P., Snyder, J. R., Ross, M. S., Stoffella, S., Constant, B., Castaneda, S., et al. (2021). Evaluation of vegetation response to changes in hydrologic parameters within Cape Sable seaside sparrow habitat, Everglades National Park, Florida. Annual report—2020 (Year 4). Submitted to US Army Engineer Research and Development Center, Vicksburg, MS, and South Florida Natural Resources Center, National Park Service, Homestead, FL. Available at: https://softel.fiu.edu/reports/CSSS_USACE_ENP_Yr4_2021.pdf (Accessed June 13, 2022).
Schuurman, G. W., Hoffman, C. H., Cole, D. N., Lawrence, D. J., Morton, J. M., Magness, D. R., et al. (2020). Resist-accept-direct (RAD)-a framework for the 21st-century natural resource manager (No. 2020/2213). National Park Service. Available at: https://irma.nps.gov/DataStore/DownloadFile/654543 (Accessed August 3, 2022).
Shriver, W. G., and Gibbs, J. P. (2004). “Seaside sparrows (Ammodramus maritimus) in Connecticut; projected effects of sea-level rise” in Species Conservation and Management: Case Studies. eds. R. Akcakaya, M. A. Burgman, O. Kindwall, C. C. Wood, P. Sjorgren-Gulve, and J. S. Hatfield, et al. (New York, NY: Oxford University Press), 397–409.
Simberloff, D. (1998). Flagships, umbrellas, and keystones: is single-species management passé in the landscape era? Biol. Conserv. 83, 247–257. doi: 10.1016/S0006-3207(97)00081-5
Slater, G. L., Boulton, R. L., Jenkins, C. N., Lockwood, J. L., and Pimm, S. L. (2009). Emergency management action plan for the endangered Cape Sable seaside sparrow Ammodramus maritimus mirabilis. Report submitted to US Fish and Wildlife Service, Vero Beach, FL. Available at: https://www.ecoinst.org/wp-content/uploads/2014/03/Slater_etal_2009_CSSS_EMAP.pdf (Accessed June 1, 2022).
Smith, T. J. III, Foster, A. M., Tiling-Range, G., and Jones, J. W. (2013). Dynamics of mangrove-marsh ecotones in subtropical coastal wetlands: fire, sea-level rise, and water levels. Fire Ecol. 9, 66–77. doi: 10.4996/fireecology.0901066
Tabor, K., and Williams, J. W. (2010). Globally downscaled climate projections for assessing the conservation impacts of climate change. Ecol. Appl. 20, 554–565. doi: 10.1890/09-0173.1
Terando, A. J., Costanza, J., Belyea, C., Dunn, R. R., McKerrow, A., and Collazo, J. A. (2014). The southern megalopolis: using the past to predict the future of urban sprawl in the southeast U.S. PLoS One 9:e102261. doi: 10.1371/journal.pone.0102261
USACE (U.S. Army Corps of Engineers) (2013). ER 1100-2-8162: incorporating sea level change in civil works programs. Washington, D.C. Available at: https://www.publications.usace.army.mil/Portals/76/Publications/EngineerRegulations/ER_1100-2-8162.pdf (Accessed June 5, 2022).
USACE (U.S. Army Corps of Engineers) (2020). Final Environmental Impact Statement Combined Operational Plan.
U.S. Fish and Wildlife Service (2020). Biological Opinion for the Combined Operational Plan. Vero Beach, FL: U.S. Fish and Wildlife Service.
U.S. Forest Service (2019). NLCD 2011 Tree Canopy Cover (CONUS). Salt Lake City, Utah. Available at: https://www.mrlc.gov/data/nlcd-2011-usfs-tree-canopy-cover-conus (Accessed November 10, 2020).
Virzi, T., and Tafoya, D. (2021). Recovery of the Cape Sable Seaside Sparrow. Annual Report. Submitted to U.S. Fish and Wildlife Service, South Florida Ecological Services Office, and National Park Service, Everglades National Park. Happy Valley, OR: Conservation InSight.
Walters, J. R., Beissinger, S. R., Fitzpatrick, J. W., Greenberg, R., Nichols, J. D., Pulliam, H. R., et al. (2000). The AOU conservation committee review of the biology, status, and management of cape sable seaside sparrows. Auk 117, 1093–1115. doi: 10.1093/auk/117.4.1093
Keywords: Ammospiza maritima mirabilis, climate change, Everglades, ecosystem restoration, marl prairie, Florida (United States)
Citation: Romañach SS, Haider SM and Benscoter AM (2023) Sea level rise may pose conservation challenges for the endangered Cape Sable seaside sparrow. Front. Ecol. Evol. 10:1085970. doi: 10.3389/fevo.2022.1085970
Edited by:
Ruscena P. Wiederholt, Everglades Foundation, United StatesReviewed by:
Greg Shriver, University of Delaware, United StatesJamed Allan Cox, Tall Timbers Research Station and Land Conservancy, United States
Copyright © 2023 Romañach, Haider and Benscoter. This is an open-access article distributed under the terms of the Creative Commons Attribution License (CC BY). The use, distribution or reproduction in other forums is permitted, provided the original author(s) and the copyright owner(s) are credited and that the original publication in this journal is cited, in accordance with accepted academic practice. No use, distribution or reproduction is permitted which does not comply with these terms.
*Correspondence: Stephanie S. Romañach, ✉ c3JvbWFuYWNoQHVzZ3MuZ292