- 1Bordeaux Sciences Agro, INRAE, SAVE, ISVV, Bordeaux, France
- 2Institute of Plant Protection and Institute of Integrative Nature Conservation Research, University of Natural Resources and Life Sciences Vienna (BOKU), Vienna, Austria
- 3Department of Agricultural, Forest and Food Sciences, University of Turin, Grugliasco, Italy
- 4UMR 6553 EcoBio, Station Biologique de Paimpont, Université Rennes 1, Paimpont, France
- 5Living Lab CLEF, Plélan-le-Grand, France
- 6Research Center for Agriculture and Environment – Council for Agricultural Research and Economics (CREA- AA), Florence, Italy
- 7UMR CNRS ECOBIO, OSUR, Station Biologique, Université Rennes 1, Paimpont, France
- 8Viti-Oeno Conseil, Perpignan, France
- 9UMR CEFE 5175, University of Montpellier, EPHE, University Paul-Valéry Montpellier, Montpellier, France
- 10Institut Agro, INRAE, SAS, Rennes, France
- 11Department of Agricultural Sciences, University of Naples Federico II, Portici, Italy
- 12CNR – Istituto per la Protezione Sostenibile delle Piante (IPSP), Portici, Italy
- 13Department of Crop Sciences, Institute of Viticulture and Pomology, University of Natural Resources and Life Sciences, Vienna, Austria
- 14Research Centre for Plant Protection and Certification-Council for Agricultural Research and Economics (CREA–DC), Palermo, Italy
- 15LISAH, Univ Montpellier, INRA, IRD, Montpellier SupAgro, Montpellier, France
- 16Faculty of Natural and Agricultural Sciences, Universitatii Alley, Ovidius University of Constanta, Constanta, Romania
- 17Dipartimento di Scienze Agrarie e Forestali (DAFNE), Università degli Studi della Tuscia, Viterbo, Italy
- 18Department of Crop Protection, Geisenheim University, Geisenheim, Germany
- 19INRAE, UMR 1065 Santé et Agroécologie du Vignoble, ISVV, Université de Bordeaux, Bordeaux Sciences Agro, Bordeaux, France
- 20Agricultural Institute of Slovenia, Ljubljana, Slovenia
- 21Ecology and Evolution Unit, Department of Biology, University of Fribourg, Fribourg, Switzerland
- 22University of Agricultural Sciences and Veterinary Medicine Cluj-Napoca, Cluj-Napoca, Romania
- 23CNR-IBE – Department of Biology, Agriculture and Food Sciences, Sesto Fiorentino, Italy
- 24Institute of Zoology, University of Natural Resources and Life Sciences Vienna (BOKU), Vienna, Austria
- 25Department of Applied Ecology, Geisenheim University, Geisenheim, Germany
Healthy soils form the basis of sustainable viticulture, where soil characteristics have a direct impact on wine quantity and quality. Soil not only provides water and nutrients to vines, but is also a living medium containing micro- and macroorganisms that perform many ecological functions and provide ecosystem services. These organisms are involved in many processes, from decomposing organic matter to providing minerals to vine roots. They also control diseases, pests, and weeds, in addition to improving the soil structure in terms of its capacity to retain water and nutrients. Related to decomposition processes, the carbon content of vineyard soils influences fertility, erosion and biogeochemical cycles, with significant implications for the global climate. However, common agricultural practices represent strong threats to biodiversity and associated ecosystem services provided by vineyard soils. As consumers increasingly consider environmental aspects in their purchase decisions, winegrowers have to adapt their vineyard management strategies, raising the demand for sustainable pest- and weed-control methods. This article presents a comprehensive review of the impacts of vineyard practices on the soil ecosystem, biodiversity, and biodiversity-based ecosystem services, and provides future prospects for sustainable viticulture.
Introduction: The Importance of Soil in Viticulture
Vineyards are planted all over the world, mostly between 30 and 50 degrees latitude, in both the Northern and Southern hemispheres. In 2020, the total area under vines was about 7.3 million hectares (wine grapes, table grapes or dried grapes) and total wine production was 260 million hectoliters. The global market (total exports of all countries) reached 105.8 million hectoliters and 29.6 billion Euro in terms of value (Organisation Internationale de la Vigne et du Vin [Oiv]., 2021). Despite this significant economy, there is a growing awareness and concern among winegrowers and consumers that some agricultural practices, and in particular the use of pesticides and intensive tillage, can have detrimental impacts on biodiversity (Paiola et al., 2020), soil quality and soil associated biodiversity and on sustainable wine production (Viers et al., 2013). Hence, winegrowers must consider and promote soil quality in their vineyards, defined as “the capacity of a soil to function within ecosystem boundaries, sustain biological productivity, maintain environmental quality and promote plant and animal health” (Doran and Zeiss, 2000; Riches et al., 2013).
However, knowledge about the threats to the soil in vineyards and their consequences on wine quality is scarce. Thus, efforts are required to better understand and protect soil to maintain respective ecosystem services. Interactions between soil biological communities as well as chemical and physical properties of the soil environment are fundamental to many soil processes, functions and services, such as carbon storage and cycling, nutrient cycling, soil structure formation, and pest regulation (Pulleman et al., 2012). Soils also play a key role in climate regulation and, thus, in mitigation and adaptation to climate-change, particularly in regulating greenhouse gases (Pulleman et al., 2012). This contrasts with the considerable body of knowledge on the relationships between soil characteristics and wine quality, driven by the prestige and profitability of high-quality wine production. Future research must focus on a better understanding of threats associated with viticultural management practices, as well as options for protecting soil biodiversity, functions and services while maintaining high-quality wine production and the aesthetic value of vineyard landscapes. The current work summarizes existing knowledge concerning the effects of vineyard practices on soil biodiversity, and how related ecosystem functions and services may enhance vine growth and yield. Subsequently, it offers some prospects for mitigating threats to the soil ecosystem and improving conditions for biodiversity, drawn from current research projects on these topics.
Relationship Between Terroir Characteristics, Soil Management, and Wine Production
The economic importance of wine production within a particular territory is one of the most effective motivations for protecting wine typicity (Costantini et al., 2012; Vaudour et al., 2015). Consumer perception that wine quality is closely linked to its geographical provenance creates the basis for preserving rural environments, not only in terms of productivity, but also in terms of landscape aesthetics and lifestyle. Consequently, winegrowers in geographically defined production areas place particular importance on preserving the quality of the land, thus adding further value to the wine. Wine marketing often uses the beauty of the vineyard landscape as an effective medium for promoting sales and increasing the market value of the product, developed through oenotourism (Tempesta et al., 2010). The use of the expressions “terroir,” “typical,” “identity,” and “sense of place,” mostly used in the wine sector, strictly connect the value of geographic origin and the landscape to the intrinsic value of a wine (van Leeuwen et al., 2004). In contrast, viticulture is often practiced as an extensive monoculture within a region, reducing the cover and quality of natural or semi-natural habitats. This has a negative impact on landscape aesthetics and associated biodiversity (Costantini and Barbetti, 2008). Management of the entire agroecosystem should be integrated, including ecological and cultural practices, to improve sustainability and habitat protection, as well as biodiversity and the associated ecosystem services (Viers et al., 2013; Chrysargyris et al., 2018). Most of these conservation actions concern the soil, which is not only one of the most threatened habitats in vineyards (Costantini et al., 2015, 2018), but also provides fundamental services to wine production.
Terroir may be defined as the interaction of all ecosystem characteristics in a given place that affect the crop phenotype, including vines (grape varieties and rootstocks), climate, and soil (van Leeuwen and Seguin, 2006). Grapevines are planted in a huge variety of different soils throughout the world, but soil is one of the most important factors in wine quality (van Leeuwen and Seguin, 2006). The wine economy of an area, including the focus on marketing high- or low-priced wine, is associated with soil characteristics, as well as environmental factors such as climate, geomorphology, and landscape quality (Costantini et al., 2016). However, only rarely the impact of soil management on biodiversity is taken into account.
Based on the most recent classification proposed by the Common International Classification of Ecosystem Services (CICES - La Notte et al., 2017), many soil processes contribute to functions associated with Ecosystem Services (ESs). For example, grape production and the decomposition of organic matter are related to provisioning and regulating ESs. According to Lal (2001), any soil degradation impairs soil quality or deteriorates the functions that contribute to ESs provision. It is, therefore, essential to consider the effects of soil management practices in vineyards on ecosystem functions (Herrick, 2000), particularly in the context of climate change (Chrysargyris et al., 2018).
In the Mediterranean or comparable water-limited climates, rainfall is generally concentrated in the winter season and does not usually exceed 400–500 mm per year. In this context, where water is a very limited resource, traditional management is based on intensive weed control to avoid competition with grapevines for water and nutrients. Herbicide use increased considerably with the global intensification of pesticide use in the 1950–1960s. However, nowadays, tillage and herbicide use are the two most-widespread systems under water-limited conditions (e.g., Biarnès et al., 2004). These practices are associated with a decline in soil quality, e.g., soil erosion, soil compaction, and loss of organic matter (Steenwerth and Belina, 2008; Salome et al., 2014, 2016; Biddoccu et al., 2016). Herbicide use also negatively influences biodiversity through the reduction of resources (Kazakou et al., 2016; Hall et al., 2020) or direct effects on the metabolism of organisms and groundwater quality (Louchart et al., 2001).
In temperate regions, especially under maritime influence, the climate is characterized by higher rainfalls, more evenly spread throughout the year, and soils are more fertile with higher nutrient availability (Peregrina et al., 2012). Hence, tillage or herbicides to reduce competition between vines and weeds is unnecessary and may even be undesirable, due to the risk of intensified erosion and nutrient leakage from bare soil in heavy rainfall (Biddoccu et al., 2016). In addition, it may be difficult to drive machinery for other pesticide applications. Permanent plant cover between rows has been widely adopted, at least during winter and spring, since vineyards are more often prone to precipitation-related soil erosion than weed competition. A further possibility is to adopt a permanent grass cover in alternate inter-rows, while the intervening inter-rows are tilled one or more times a year, depending on precipitation conditions and any potential negative competitive effects of weed species on grape yields or quality (Peregrina et al., 2012).
Another intermediate management technique, called “green manure,” combines tillage or herbicide and permanent cover, with sown cover crops in winter or spring. These cover crops help to improve nutrient supply to the vines (especially nitrogen fixation by legumes species), store carbon, and mitigate soil erosion during winter. This practice includes the cultivation of sown cover crops destroyed by tillage before it starts competing with the vines for nutrients and water. Nevertheless, the soil type and, in particular, its lime content and texture, strongly influence the effectiveness of cover crops in providing these benefits (Ruiz-Colmenero et al., 2011; Salome et al., 2016). Consequently, strategies combining various management practices are more effective for maintaining and improving soil quality (Ruiz-Colmenero et al., 2011). Therefore, flexible, innovative practices are required to face the key issues of vineyard complexity and counteract the negative effects of climate change on soil fertility (Salome et al., 2014, 2016).
Wine-growing areas are more exposed to several environmental risks, as other agricultural landscapes: soil erosion, depletion of biodiversity, as well as water and air pollution due to the use of pesticides (Viers et al., 2013). In addition, vineyard topography (especially steep slopes), the abundance and distribution of precipitation (modified by climate change), and anthropogenic factors increase soil exposure to various threats, particularly erosion and landslides (Arnaez et al., 2007; Martínez-Casasnovas and Ramos, 2009; Biddoccu et al., 2016; Chrysargyris et al., 2018). As highlighted by the analysis of long-term soil-loss measurements under natural rainfall (Cerdan et al., 2010), vineyards exhibited the highest soil losses in Europe, compared to other crops and land uses. Vineyard planting also strongly impacts soil and involves slope reshaping, deep plowing, which mixes topsoil and subsoil, as well as stone crushing and removal. These operations entail a high risk of decreasing soil functionality and biodiversity, as well as degrading the beauty of the landscape and long term sustainability of the vineyard (Le Bissonnais et al., 2002; Costantini and Barbetti, 2008; Martínez-Casasnovas and Ramos, 2009; Costantini et al., 2015). During high-intensity rainfall events, the accelerated erosion damages fields, reduces organic carbon stocks and soil fertility, exposes roots, and causes rill erosion, as well as downstream damage, with flooding, mudslides, and pollution (Le Bissonnais et al., 2002; Arnaez et al., 2007). As this accelerated erosion modifies the carbon dynamics, it may also have a broader environmental impact, such as reducing carbon sequestration and biodiversity (Lal, 2001). Furthermore, climate change may lead to the extension of viticulture at higher altitudes and latitudes, potentially impacting upland ecosystems and eliminating natural or semi-natural vegetation (Hannah et al., 2013).
Soil Biodiversity in Vineyards: Trophic Interactions and Ecological Services
Soils are living environments and include a large variety of microhabitats, characterized by different physicochemical properties (Coleman et al., 2004). A countless diversity of organisms lives in these habitats, generally divided into 4 categories according to their body size (Lavelle and Spain, 2001): microorganisms (Fungi, Bacteria, Protists, and Archaea), microfauna (e.g., Collembola, Acari, Amoeba, Nematoda, Annelida Enchytraeids) and macrofauna (e.g., earthworms, gastropods and larger arthropods).
All these organisms have a textural (changes in the distribution of mineral and organic particles) or structural (formation of aggregates and soil horizons) influence on soil functions. Soil texture and structure influence above-ground vegetation and animal communities through changes in fertility, related to biogeochemical cycles and plant nutrition (Coleman et al., 2004; Blouin et al., 2013). Soils provide nutrients and water to support the growth of both crop and spontaneous plant species: the latter are often considered weeds (Figure 1). The provisioning service of soil fertility associated with the regulation service of nutrient cycling is directly or indirectly associated with below-ground organisms, from macro- or mesofauna, the primary decomposers of organic matter, to microorganisms (Figure 1). Agricultural practices, such as tillage, pesticide and heavy machinery use, interfere with vegetation diversity and cover and are of primary importance for the soil trophic networks that drive the diversity and abundance of all taxa.
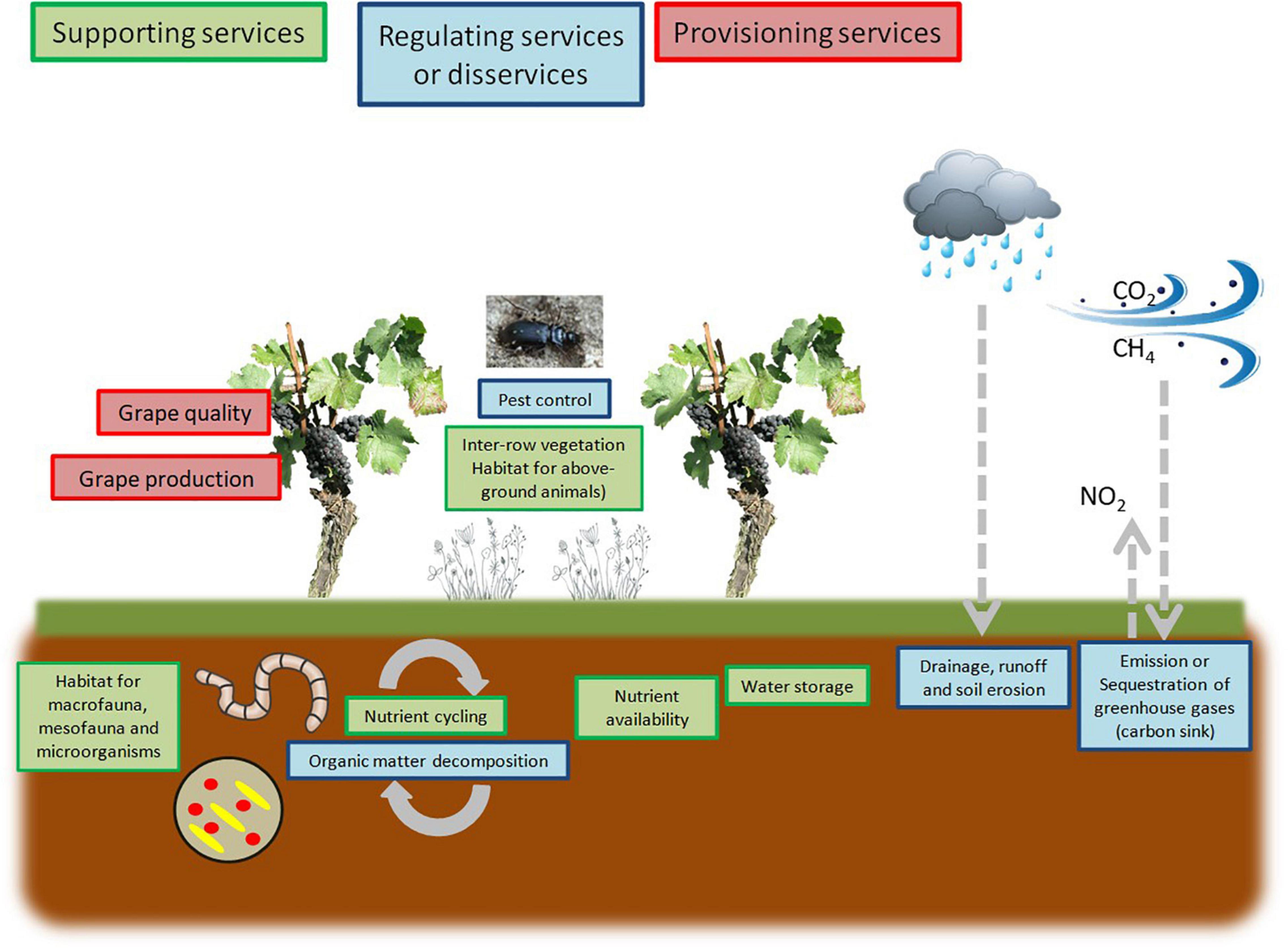
Figure 1. Ecosystem services provided by vineyard soils. Biodiversity is strongly linked to habitats availability in this agroecosystem: soil and plant cover above-ground. In turn, biodiversity strongly drives several regulating services (blue boxes), related to supporting services (green boxes), and influences some provisioning services (red boxes).
Most of the studies exploring the relationship between soil biodiversity and its functions in agricultural landscapes have focused on arable crops, but less on vineyards. The following paragraphs examine aboveground biodiversity that is strongly linked to soil conditions, firstly represented by vascular plant communities in vineyards and the services they provide. Organisms will be then considered in order of size, from the larger taxa of animals, macrofauna and mesofauna living in soil or on the ground surface, to microorganisms. We describe the state of the art concerning these taxa, as well as the services provided in vineyards, particularly nutrient cycling and pest regulation, as well as the influence of agricultural practices and soil parameters on the diversity and abundance of these taxa.
Very little research exists on larger soil organisms such as small mammals (moles and small rodents), and focuses mostly on their negative effects on vine yield, and their biological control by predatory birds such as owls in Napa valley using next boxes in vineyards (e.g., Wendt and Johnson, 2017).
Vascular Plants
Herbicides and tillage are still the most common techniques for soil management in viticulture as they are supposed to eliminate the competition between weeds and vines, thus improving grape yields and quality in water-limited conditions (Peregrina et al., 2012). However, no significant competitive effects of inter-row vegetation cover have been observed in oceanic climates or irrigated vineyards, especially where soil water-holding capacity was high (Wheeler et al., 2005; Monteiro and Lopes, 2007; Baumgartner et al., 2008; Steenwerth et al., 2013; Irvin et al., 2016). Nowadays, many winegrowers are aware of the benefits of plant cover for the vines and the vineyard agroecosystem. The vineyard should be considered as a consociation, formed by the vines and the underlying grasses, both complementing the functions and ecosystem services of the vineyard. Associated plant diversity in vineyards may contribute to multiple ESs, such as mitigating soil erosion and, preserving soil fertility, biocontrol of vine pests by promoting colonization of vine rows by their natural predators (see in the following paragraphs and Nicholls et al., 2000), aesthetic value of viticultural landscape (Hervé et al., 2020), and conservation of endemic species (Gillespie and Wratten, 2012). Studying the benefits and ecosystem services supported by spontaneous flora in vineyard agroecosystems is a quite novel topic (Garcia et al., 2018), but findings suggest that vegetation cover should be used wherever soil and climate conditions are favorable.
Vineyards are mostly associated with a moderate level of plant diversity; nevertheless, like other permanent, heterogeneous systems with a multi-strata design such as orchards (Simon et al., 2010), they have a high potential for preserving agroecosystem biodiversity and providing habitats for xerothermic species. In recent years several studies have evaluated plant diversity in vineyard ecosystems. Examining varying numbers of sampling plots and environmental conditions, 32 plant species were found in one vineyard in Napa Valley (Baumgartner et al., 2008), 86 species in 25 vineyards (averaging 22.2 ± 2.9 per site) in northern Switzerland (Bruggisser et al., 2010), 177 species in 33 vineyards (ranging from 24 to 46 average number of plant species per vineyard) in the Piemonte region of north-western Italy (Mania et al., 2015), 211 species in 18 vineyards (averaging 32.8 in organic and 25.1 in conventional vineyards) in the Veneto region in north-eastern Italy (Nascimbene et al., 2012), over than 200 species in 120 vineyards of Dão winegrowing region of Central Portugal (Monteiro et al., 2012), and 259 species in 48 vineyards in southern Switzerland (Trivellone et al., 2014).
Soil conditions and cultural practices selected several physiological, morphological, and dispersal traits among plant communities (Kazakou et al., 2016), e.g., the proportion of perennial species increased with less-intense management (Gago et al., 2007; Kazakou et al., 2016). Plant diversity and community composition depend on inter-row plant management, i.e., mowing or mulching frequency, fertilization and tillage intensity, chemical weed control, and sowing of a mixed cover crop or fostering spontaneous vegetation (Gago et al., 2007; Bruggisser et al., 2010; Nascimbene et al., 2013; Trivellone et al., 2014), as well as the management and characteristics of adjacent areas (Thomson and Hoffmann, 2009; Simon et al., 2010; Mania et al., 2015). Weed-control techniques influence species richness and composition of plant communities. For example, shredding inter-row vegetation had a more negative effect on plant diversity than mowing but this effect was lower than local abiotic conditions, such as vineyard altitude and slope that drive the community composition (Bruggisser et al., 2010). In a landscape with small vineyard plots, the diversity of microhabitats also enhances the conservation of rare species. For example, 9% of sampled species in Swiss vineyards were regarded as near threatened or vulnerable, according to the national red list (Bruggisser et al., 2010). Extensive management practices in vineyards, like organic farming, have been found to increase plant diversity in Italy (e.g., Nascimbene et al., 2012), probably due to the ban on herbicide use. The negative effect of herbicides was also confirmed by Sanguankeo and León (2011) in California. However, Bruggisser et al. (2010) and Kehinde and Samways (2014) did not find any significant effect of organic farming on plant diversity in a set of South African and Swiss vineyards, where no herbicides were used, even in the conventional plots. Inter-row vegetation management also influences dominant plant traits, such as basal buds or underground regeneration in case of frequent mowing (Gago et al., 2007) or a higher specific leaf area in tilled vineyards (Kazakou et al., 2016). Several studies identified a significant reduction in vascular plant diversity in vineyards with intensive soil tillage (Sanguankeo and León, 2011; Bagella et al., 2014; Kazakou et al., 2016). This technique benefits certain species that regenerate from plant fragments, like Spergula arvensis (segetal species), and annual plant species with short development cycle (Gago et al., 2007). Changing from mulching to mowing led to an increase in the proportion of plant species with basal rosettes (Bruggisser et al., 2010). Traditional species-rich communities, featuring bulbous geophytes, have become very rare due to changes in soil management in Central European vineyards (Bruggisser et al., 2010). Mowing frequency also modifies dominant plant traits and particularly enhances the cover of rosulate and reptant species (Nascimbene et al., 2012). Nevertheless, since the seed germination of ruderal species benefits from bare soil created by tillage, a low frequency of soil disturbance seems to benefit plant diversity (Gago et al., 2007), in accordance with the intermediate disturbance hypothesis (Grime, 2006).
First tested in annual crops, there is a growing interest in cover crops or sown vegetation in vineyards, at least in inter-rows (Garcia et al., 2018). As already mentioned, one common technique is to establish permanent vegetation in alternate inter-rows, while the intervening inter-rows are tilled several times a year, depending on precipitation or the sowing of cover crops. Annual cover crops may be divided into two main classes: winter cover crops, sown in autumn, adapted to winter conditions and removed by tillage in the following spring or dry season, and summer cover crops, sown in spring. Cereals and legumes are the two main categories of plants used as cover crops. Spontaneous as well as sown species may provide several services to winegrowers: their management and the choice of species are very important for simultaneously maintaining production and regulating services in the vineyard.
Although using resident natural vegetation or sowing cover crops is viewed partly critically due to potential competition effects on vines, this management is beneficial from an environmental point of view (Marques et al., 2010) and it is supported by agro-environmental schemes. This becomes especially obvious if a permanent vegetation cover is permanently maintained over the entire surface throughout the year to reduce soil erosion, one of the major ecosystem disservices in many sloping vineyards (Gyssels et al., 2005; Blavet et al., 2009; Marques et al., 2010). Plant species adapted to local conditions may also provide numerous other ESs for the agroecosystem, including buffering soil nitrogen availability (Martínez-Casasnovas and Ramos, 2009; Messiga et al., 2015; Pérez-Álvarez et al., 2015), increasing soil organic matter content (Steenwerth and Belina, 2008; Blavet et al., 2009; Mazzoncini et al., 2011; Salome et al., 2016), regulating water use (Monteiro and Lopes, 2007), soil temperature and vineyard microclimate (Fourie and Freitag, 2010), as well as in fertile soils, controlling vine vigor and enhancing grape quality (Wheeler et al., 2005; Monteiro and Lopes, 2007; Giese et al., 2014). Furthermore, fostering spontaneous vegetation or use of cover crops not only increases plant diversity (Hall et al., 2020) but also inhibits troublesome weeds (Monteiro et al., 2012; Kazakou et al., 2016; Steenwerth et al., 2016). For example, the use of cover crops reduced the cover and frequency of spontaneous, ruderal plant species, like Digitaria sanguinalis and Chenopodium album (Gago et al., 2007; Smith et al., 2015). Spontaneous vegetation enhances the biodiversity of the whole ecosystem at different trophic levels, as it provides the main energy source for decomposers and heterotrophic organisms (Danne et al., 2010; Kehinde and Samways, 2014; James et al., 2015; Buchholz et al., 2017; Geldenhuys et al., 2021).
Macrofauna: Earthworms
Among all the taxa, earthworms are a well-studied group of macroinvertebrates, representing the largest component in the animal biomass in soils (0.1–12 g dry weight per square meter) and are very important organisms for maintaining soil fertility (Jeffery et al., 2010). They are commonly known as “ecosystem engineers,” thanks to their burrowing, mixing and casting activities (Jones et al., 1994; Blouin et al., 2013). Earthworms can be divided into three ecological categories, based on their distribution within the soil: epigeics, anecics and endogeics (Bouché, 1977 in Blouin et al., 2013). Epigeic species, also known as litter or surface-dwelling species, live on the soil surface, in leaf litter and humus layers, and sometimes in the first few soil centimeters. Anecic species, also known as topsoil species or soil-dwelling species, live in permanent, vertical burrows, connected to the soil surface, which are important for soil drainage. Endogeic species, also known as subsoil or soil-dwelling species, live mainly within the soil and are important for maintaining its granular structure. All these groups and species are known to strongly influence plant growth by creating and connecting pores (burrows, aestivation or hibernation nests), that modify the physical matrix for roots, aggregating or disaggregating particles, and moving them within the soil profile (Wurst et al., 2018). These activities are conditioned by soil organic matter content and quality, soil compaction, species interactions, and seasons (Pérès et al., 1998; Capowiez et al., 2009; Blouin et al., 2013).
Ecological functions provided by earthworms are mainly pedogenesis, soil structure development, water regulation, nutrient cycling, primary production, climate regulation, and pollution remediation (Blouin et al., 2013). Soil management practices in vineyards have direct and indirect impacts on the ecology and physiology of earthworms (Schreck et al., 2012). While the impacts of tillage on earthworms have been studied intensively in arable systems, little is known about vineyards. However, the consequences of soil tillage are quite similar, as it severely reduces earthworm abundance, biomass, and variety (Paoletti et al., 1998). Conversely, Buchholz et al. (2017) showed a higher species diversity in infrequently tilled plots, which could be related to a higher plant diversity and soil fertility in these plots. Earthworm biomass was negatively, but only slightly, impacted by reduced tillage methods, such as grubbing, rotary hoeing and harrowing, particularly in spring under dry soil conditions. Furthermore, when soil is tilled only every second inter-row, rapid re-colonization may occur between disturbed rows and rows with permanent herbaceous cover (Faber et al., 2017).
Earthworms are useful bioindicators, as they respond quickly to environmental inputs, such as chemicals, and can therefore be used to monitor the effects of farming practices and soil contamination (Paoletti et al., 1998; Pérès et al., 2011). Earthworms are impacted by copper, used as fungicide, which decreases earthworm survival, growth, and behavior (measured as a decreasing burrowing rate, Eijsackers et al., 2005; Pelosi et al., 2014; Karimi et al., 2021). Herbicides may also affect the activity and reproduction of earthworms, especially anecic species (Gaupp-Berghausen et al., 2015). A laboratory experiment showed that fungicide and insecticide spraying was neurotoxic for endogeic earthworms (Schreck et al., 2008). In contrast, organic fertilization increased the abundance, biomass, and diversity of the earthworm community (Pérès et al., 1998).
Macrofauna: Gastropods
Unlike earthworms, gastropods are part of a less studied group of macroinvertebrates, including isopods and myriapods, comprising mainly litter- and topsoil-dwelling species, as well as a few endogeic ones. ESs provided by gastropods are mainly linked to the decomposition of organic matter and soil structure improvement (Wolters and Ekschmitt, 1997). By feeding on organic matter and promoting microflora through burrowing organic material, as well as producing enzymes and feces, gastropods contribute 1% to the total decomposition activity of soil biota (Schaefer, 1990).
In natural systems, gastropod richness and diversity decreased with lower soil pH values, due to lower calcium availability (Nekola, 2010). Soil moisture, texture, and vegetation composition are also strong drivers of their abundance and diversity (Dvořáková and Horsák, 2012; Hettenbergerová et al., 2013). In vineyards, as in agricultural fields in general, most gastropods are xerothermophilic (dry- and warm-adapted, mostly open-land species), but communities tend to be less species-rich than in grasslands. Cultivation involves several types of disturbance: (i) mechanical disturbance that increases exposure to predators and destroys microhabitats used for reproduction and shelter, (ii) simplification of vegetation that leads to loss of shelter, moisture, and food, (iii) use of mineral fertilizers that acidify the soil, such as nitrates, and (iv) biocides that reduce plant cover and/or are toxic to gastropods (Wolters and Ekschmitt, 1997).
The decrease in abundance and richness within gastropod communities may foster pest organisms: the simplification of plant cover favors pest species with corresponding feeding preferences and the loss of predators and competitors (Wolters and Ekschmitt, 1997). Most of the introduced gastropod species are considered vineyard pests, such as (i) the Cepaea nemoralis snails in Ontario, Canada, which use vines as a daytime roost and are picked with the grapes, thereby fouling the wine (Martinson, 1999), (ii) Meghimatium pictum slugs in Brazil, that feed on fruit pulp (Baronio et al., 2014), (iii) Bradybaena similaris snails in Taiwan, that destroy leaves and buds (Chang, 2002), and (iv) Cornu aspersum and Theba pisana snails in South Africa, which feed on developing foliage and leaving a mucus trail on grapes, deteriorating the aesthetics of table grapes, or contaminating dried-fruit products with their shells in Australia (Sanderson and Sirgel, 2002). The last two species may reach densities of up to 400 individuals per vine and cause economic losses of up to 25% (Sanderson and Sirgel, 2002).
Soil management practices that minimize the disturbance of gastropod communities, such as low tillage and the use of organic fertilizers, help to maintain and restore the gastropod community and reduce or eliminate pest problems (Wolters and Ekschmitt, 1997). The maintenance of a complex landscape in wine-growing regions may also favor the persistence of gastropod communities able to recolonize vineyards if soil management intensity decreases. For example, in Austria, two rare gastropod species, Zebrina detrita and Caucasotachea vindobonensis, had refugia in managed grassy stripes bordering vineyards (Duda, 2016). However, changing from intensive soil management to an extensive system may also attract pest species (e.g., in Australia - Sanderson and Sirgel, 2002). Vegetation or mulch cover provides shade and moisture advantageous for gastropod development and thus increasing pest slug densities (Baronio et al., 2014).
Macrofauna: Predatory Arthropods
Among the arthropod communities that provide ESs in agroecosystems, generalist predators may act as biological control agents of crop pests (Symondson et al., 2002). Several groups of generalist predators are found in vineyards. Spiders (Araneae), rove beetles (Staphylinidae), ground beetles (Carabidae) and ants (Formicidae) are among the most abundant taxa living on the ground or near the soil surface in vineyards (Thomson and Hoffmann, 2009). Soil dwelling spiders are excellent hunters and mostly belong to the Lycosidae and Gnaphosidae families. They prey on various vineyard pests, e.g., caterpillars (grapevine moths) or Hemipteran species living on vine leaves. Ground beetles such as carabids or rove beetles may also be abundant in vine plots. They are well-studied arthropod predators in agroecosystems and consume various insect pests and weed seeds (Holland, 2002). Moreover, they are well-adapted to open habitats and intensive agricultural landscapes, dominated by perennial cropping systems, such as vineyards (Rusch et al., 2016a). Ants may also exert top-down control of arthropods in natural and agricultural systems (e.g., Chong et al., 2010), but mealybug-tending ants could also hamper parasitoid biocontrol of certain pests such as vine mealybug (Cocco et al., 2021).
Several factors related to soil, vegetation diversity and management are known to affect generalist predators and regulation associated services (Rusch et al., 2010; Winter et al., 2018; Pfingstmann et al., 2019).
Firstly, habitats with higher plant diversity or a more complex structure feature more abundant predators, smaller herbivore populations, and less plant damage, compared with plant monocultures (Langellotto and Denno, 2004; Letourneau et al., 2011; Rusch et al., 2017). Vegetation diversity around vineyards and in-field grass cover is thus expected to favor the diversity of arthropod communities with a high level of resources (Nicholls et al., 2001; Hogg and Daane, 2010), and sustains biological pest control and seed predation (Rusch et al., 2017; Winter et al., 2018). Perennial agroecosystems provide important resources and functions for all these generalist natural enemies, such as overwintering sites, alternative hosts, and food sources. Rusch et al. (2017) demonstrated that inter-row plant cover is a strong driver of grape moth density in vineyards: it was significantly lower in vineyards with full compared to partial grass cover. Higher richness and activity-density of rove beetles, carabids, and hoverflies were found when natural vegetation was preserved, at least between the rows (Sanguankeo and León, 2011; Pétremand et al., 2017). However, several studies detected little or no effect of vegetation cover on carabid communities: the presence of surrounding vegetation may influence trapping success (Hanna et al., 2003; Sanguankeo and León, 2011). Community changes may also occur, with higher abundances of macropterous species with higher dispersal capacities than dimorphic or brachypterous species, which may be associated with an increase in tillage (Rusch et al., 2016b). The relationship between arthropod diversity and ES delivery is, however, variable, suggesting that rare species may contribute little to the agroecosystem functions. Thus dominant species are probably the main drivers of ES delivery such as pest control (Winfree et al., 2015; Winter et al., 2018).
Secondly, disturbance on the field scale, especially soil tillage, is known to be a key driver of the abundance and diversity of predatory arthropods (Holland, 2004). Several characteristics of soil tillage may affect aboveground arthropods, including intensity, frequency, the type of tool used, and the timing of the operation (Rusch et al., 2010; Pfingstmann et al., 2019). Larval stages of several predatory Coleopteran families are also strongly dependent on above-ground resources and disturbance intensity (Holland, 2002). Tillage may affect arthropods through indirect effects on habitat quality, by modifying their biochemical and biophysical characteristics or changing prey distribution and resource availability such as microarthropod prey (Pfingstmann et al., 2019). In addition, soil tillage may have direct lethal effects through mechanical damage, burying organisms or forcing them to migrate (Holland, 2004; Rusch et al., 2010).
Thirdly, it is reported that organic farming enhances the abundance and diversity of several taxa, from plants to birds, as compared to conventional arable farming (Tuck et al., 2014). However, somewhat contrasting results have been reported in viticulture: some studies reported a neutral effect (Bruggisser et al., 2010; Uzman et al., 2020) and others a positive effect of organic farming on certain predatory arthropods (Caprio et al., 2015; Ostandie et al., 2021). These may be due to the taxa considered, as well as differences in terms of farming practices labeled “organic” or “conventional”, which are highly variable (Ostandie et al., 2021). Brachypterous carabids, ambush spiders, ground-hunter spiders and other hunters preferred organic vineyards, whereas macropterous carabids, specialist spiders (mostly ant-eating spiders), and sheet web weavers selected conventional vineyards (Caprio et al., 2015). Landscape context is also known to be an important predictor of arthropod assemblages and the level of biological control in agroecosystems (Bianchi et al., 2006; Chaplin-Kramer et al., 2011; Rusch et al., 2016b). For example, it is well documented that semi-natural habitats are key habitats for many arthropods, as they foster individual fluxes, provide overwintering sites, refuges from disturbance, and alternative food and hosts (Thomson and Hoffmann, 2009; Hogg and Daane, 2010; Paiola et al., 2020).
Mesofauna: Collembolans and Mites
Soil mesofauna mediates soil functioning through a wide range of engineering processes such as distribution of organic matter, bioturbation, comminution, incorporation of litter into soil, determining structural porosity, and the formation of soil aggregates through burrowing, casting, and nesting activities, as well as feeding on microbial communities (Lavelle et al., 2006; Brussaard et al., 2007). These functions contribute to improving water infiltration, as well as mitigating soil erosion and nutrient provision. Collembola and some taxa in the mite (Acari) subclass, such as Oribatida are the most extensively studied microarthropods involved in detritivore food webs. The suborder Oribatida (order Sarcoptiformes) among the mites comprises over 10,000 species worldwide and is the most important group in providing decomposition ES in forests and grasslands (Culliney, 2013). They are also very abundant in vineyards (Gagnarli et al., 2015). They are involved in decomposition as direct consumers of organic matter, as well as indirectly, via a catalytic effect, by consuming saprophytic fungi and bacteria. This has a major impact on the composition of microorganism communities and makes a significant contribution to the fragmentation of plant residuals (Brennan et al., 2006). Generally, these organisms are quite sensitive to the quantity of resources in their habitat, i.e., the organic matter content of upper soil layers (Gagnarli et al., 2015).
Mesofauna abundance and biomass in soil differ among land use and habitat types. Few studies have identified microarthropods in soil samples at the species level and used standardized data on individuals per surface. However, a recent study in France compiling over 750 samples collected under different land-use conditions, including forests, grasslands, arable lands, vineyards, urban vegetable gardens, and urban soils, concluded that vineyard soils were by far the most negatively impacted by human activities (Joimel et al., 2017). Even if total microarthropod densities were higher in vineyards than in other agroecosystems, these soils presented the lowest Collembola species richness and evenness, the lowest Collembola ecomorphological index, and the highest Acari/Collembola ratio (Joimel et al., 2017). However, the samples were collected from Mediterranean vineyards (Languedoc and Banyuls appellations), where the dry climate may lead to generally low mesofauna density and diversity (Joimel et al., 2017). Renaud et al. (2004) found very wide variations in Collembola abundance, from a few hundred to over 12,000 individuals per square meter, depending on the soil treatment and season, with considerably smaller populations where herbicides were used. In undisturbed ecosystems, Oribatida densities easily reach several thousand individuals per m2 comprising between 20 and 50 different species. In agricultural fields, their density is about ten times lower, mainly because of the cultivation practices that have negative effects on these mites (Behan-Pelletier, 1999). In vineyards, maintaining herbaceous vegetation in inter-rows fosters Oribatida adult densities (Nannelli and Simoni, 2002). Other vineyards in Europe need to be sampled to extend our knowledge of these groups, particularly their diversity and abundance. The abundance of some species seem to be enhanced by organic management as observed by Seniczak et al. (2018). Furthermore, vineyard management includes many different levels of impact (i.e., fertilization, mowing frequency) that affect this mesofauna (Fiera et al., 2020). Surprisingly, springtail communities were more diverse in tilled inter-rows, and springtails were more abundant in the inter-rows with herbicide use in vineyard rows of Romanian vineyards (Fiera et al., 2020), which is probably due to disrupted competitor/predator relationships. In Bordeaux vineyards, Ostandie et al. (2021) also found that tillage combined with organic farming and the reduction of insecticide use fostered springtail abundances, however, not their diversity. Due to their sensitivity to changes in the environmental conditions, mesofauna is increasingly used as a quality bioindicator of quality in agroecosystems. The Soil Biological Quality index QBS-ar assumes that soils of higher quality will shelter more abundant, varied mesofauna (Parisi et al., 2005). The advantage of this method is that specimens are not classified on a species level, but grouped into specific taxa (Classes, Superorders and Orders). Furthermore biological forms are determined according to their morphological adaptation to soil environments and scored to calculate the QBS-ar index (Parisi et al., 2005). The few studies conducted in vineyards found QBS-ar values ranging from 137 to 230, indicating potential higher quality than in arable soils (e.g., Rüdisser et al., 2015). Values were lower in Portugal vineyards and higher in ground cover treatments (Gonçalves et al., 2020). For example, some biological forms symptomatic of undisturbed soil, such as Diplura, Protura, Pauropoda, and Chilopoda, were found in many vineyards. Organic management even recently adopted (3-year period after conversion) fostered microarthropod community diversity as observed by Ghiglieno et al. (2020) in Lombardy vineyards. Several other parameters influence microarthropod communities such as vegetation cover, soil characteristics, degree of compaction, and organic matter content related to weed and soil management. In agricultural fields, the long-term exposure to synthetic or organic pesticides and their accumulation in soils also contributes to the reduction of the abundance and the diversity of mesofauna, particularly euedaphic forms (Karimi et al., 2020, 2021; Ostandie et al., 2021). A comprehensive risk assessment of the degree of soil contamination (by heavy metals and pesticides; excess of nitrates and phosphates) and declining soil biodiversity is usually indirectly assessed.
Mesofauna: Nematodes
Nematodes are probably the most abundant multicellular animals on earth, occupying a broad range of trophic levels. Several taxa of nematodes occupy important trophic positions in the soil detritus food web; many graze on bacteria and fungi, thus regulating decomposition and nitrogen mineralization. Other free-living or plant-parasitic nematodes are useful bioindicators of soil health. Nematode assemblages act as disturbance indicators for assessing the effects of pollution on soil and studying food web dynamics (Ferris and Tuomisto, 2015). The following tools are used to measure the ecological status of soil communities: the Maturity Index (MI), an ecological measure of environmental disturbance based on nematode species composition (Bongers, 1990), and the Ferris indices, based on trophic levels and food web systems (Ferris et al., 2001). ESs provided by soil nematodes include nutrient cycling (supporting service) and controlling pest species (regulating service). Predatory and omnivorous nematodes are involved in these services through a process of predation. Recently, Ferris and Tuomisto (2015) developed a new index named “diversity-weighted abundance” index, to evaluate the efficiency of these ESs.
Overall, agricultural intensification affects nematode fauna causing important multitrophic effects (Yeates et al., 1999; Govaerts et al., 2007). Nematodes are not necessarily adversely affected by cultivation practices and are, for example, less sensitive to tillage than larger soil animals (Wardle, 1995). As reported by Coll et al. (2012), compaction reduces nematode abundance and disturbs omnivores and predators in vineyards.
Few studies have investigated the whole nematode community associated with vineyards. Overall, free-living nematodes: bacterial feeders, omnivores, and predators, are more dominant in shallow soil (0–10 cm), whereas plant-parasitic nematodes are mainly located deeply and in the immediate vicinity of the root surface (Malossini et al., 2011; Rahman et al., 2014). Most studies have focused on plant-parasitic nematodes that cause serious damage in vineyards. Most severe losses are caused by Xiphinema spp., Meloidogyne spp., Pratylenchus spp., Helicotylenchus spp. and Criconemoides spp. For example, Grapevine fanleaf virus (GFLV), transmitted by Xiphinema index, is responsible for a gradual degeneration of grapevines that occurs in most vineyards worldwide, causing yield losses of up to 80% (Andret-Link et al., 2004). Xiphinema indexis the sole natural vector of GFLV and the transmission process is characterized by a specific association between X. index and GFLV. Their actual management is preventive: infested grapevines including their roots should be removed at an early stage and destroyed to avoid contamination of replants (Villate et al., 2008; d’Errico et al., 2014). Generally, under uncultivated soil and set-aside management, the nematode colonizer species, mainly bacterivores, increased in abundance and richness while plant-parasitic nematodes and predators remained constant (Landi et al., 2018). Soil resting strategies following vine uprooting is therefore applied currently especially in infested vineyards to reduce abundances of plant-parasitic nematodes. Traditionally, the most effective control of plant-parasitic nematodes has been based on the use of chemical fumigants and nematicides, today banned for toxicological and environmental reasons (d’Errico et al., 2014).
Several studies have reported that increased soil organic matter content has a direct effect on nematode community, reducing plant-parasitic and increasing saprophytic species. Amendments, such as poultry-litter biochar, green manures, and compost, decreased the abundance of plant-parasitic nematodes (Rahman et al., 2014). In a long term study, Coll et al. (2012) estimated that organic practices promoted fungal-feeding nematodes, which are key species in organic matter turnover and nutrient cycling in soils. Cover crops or vegetation strips with plants attracting parasitic nematodes is also a considered solution, although not well-explored (Villate et al., 2008).
Microorganisms and Microfauna
Soil is described as being the most diverse environment but the least known habitat, in terms of microbial diversity and its role in ecosystem functioning (Nielsen et al., 2015; Orgiazzi et al., 2016). Recent advances in genomics have made sampling and monitoring more standardized and affordable1. The major challenge is still to assign species to ecosystem functions. Nevertheless, it is well known that soil microorganisms are key drivers of most of the main soil processes (Nannipieri et al., 2003; Wagg et al., 2014) and play diverse and often crucial roles in soil-related ESs. Microbial communities drive soil nutrient dynamics and any changes in their activities and functions may compromise soil biogeochemical cycles and nutrient availability to plants. Soils harbor an enormous microbial diversity, mainly represented by bacteria and fungi that represent the largest proportion of the total microbial soil biomass. Thus, maintaining a taxonomically and functionally diverse microbial community is a key factor in supporting sustainable agricultural management (García-Orenes et al., 2016).
Microbes have been collected throughout the soil profile, although they are more abundant in upper soil layers and the rhizosphere (Bundt et al., 2001; Fierer et al., 2007). Rhizodeposition affects both the structural and functional diversity of microbial communities (Mendes et al., 2011; Dias et al., 2013; Philippot et al., 2013). Notably, soil bacteria can live in plant tissues as endophytes and modulate plant growth and development, with direct implications on plant physiological status and its response to biotic and abiotic stress (Bulgarelli et al., 2013) or on the beneficial properties of medicinal plants (Chiellini et al., 2014). Plant-soil-microbe interactions are complex, with plant species (even specific genotypes or varieties), soil type, and agricultural practices exerting the most influence on the composition of the microbial communities (Wu et al., 2008; Dias et al., 2013; Burns et al., 2015; Vega-Avila et al., 2015). Repeated tillage is known for its long-lasting impacts on soil microbial communities (Buckley and Schmidt, 2001; López-Piñeiro et al., 2013) and Pinguel et al. (2018) observed a strong composition difference in both bacterial and fungal communities between tilled rows and those with permanent grass cover. Vega-Avila et al. (2015) also observed an increase in bacterial diversity in the rhizosphere of organically managed vineyards. Conversely, reduced tillage, nutrient application, and the reduction of pesticide inputs exert a positive effect on soil biology, resulting in a significantly higher level of biological activity (Mäder et al., 2002; Girvan and Bullimore, 2003; Morlat and Jacquet, 2003; López-Piñeiro et al., 2013; Martins et al., 2013). Moreover, long-term application of organic fertilizers compared to mineral fertilization induced a further increase (Treseder, 2008; Geisseler and Scow, 2014) or a slight decrease in microbial biomass with an increase in bacterial diversity (Francioli et al., 2016). Bokulich et al. (2014) proposed the term “microbial terroir” to emphasize the importance of regionally-specific microbial communities in fermentation processes that contribute to the specific characteristics of wine (see also Gilbert et al., 2014; Burns et al., 2015; Vaudour et al., 2015; Franco et al., 2021). Microbes colonizing roots may migrate through the plant to colonize aerial tissues (Bulgarelli et al., 2013), influencing plant traits and grape quality. Further studies are needed to elucidate the ecological role of specific soil- and plant-associated microbial taxa identified in vineyards. For example, soil protists are receiving increasing attention for their important and hitherto underestimated roles in soil food-web dynamics and nutrient cycling processes (Geisen et al., 2016). However, relatively few studies have investigated soil protists and none of them was conducted in vineyards.
Fungi and Mycorrhiza
Fungi drive ecological dynamics in soils as decomposers of organic biomass and nutrient recyclers. Macromolecules, including lignin and cellulose, are mostly depolymerized by fungi using peroxidases and laccases and are, therefore, essential for humification processes (Zavarzina et al., 2011). The filamentous nature of fungi and their production of polysaccharides contribute to aggregate formation and soil stability, especially in degraded soils (Rashid et al., 2016), and mineralization of nitrogen from organic biomass and rhizosphere depositions, thus recycling nitrogen for plant uptake. As a part of the food web, fungal hyphae provide a direct food source for numerous mesofaunal components, such as Collembola and mites (Jonas et al., 2007).
Numerous soil fungi are known for their opportunistic lifestyle, including strong saprotrophic compatibility and plant-related traits as endophytes or root associates. Certain Trichoderma species or strains activate plant defenses and induce root resistance to necrotic plant pathogenic fungi or restrict their proliferation through mycoparasitism. However, waterlogged situations or soil compaction in vineyards may trigger Blackfoot disease in grapevines, caused by other opportunistic species of the Nectriaceae with equally wide ecological amplitudes, including strong saprotrophic compatibility and plant-pathogenic abilities (Halleen et al., 2004). Permanent grass cover increased organic matter content in vineyard soils and, consequently, increased fungal counts (Whitelaw-Weckert et al., 2007). Furthermore, not only synthetic fungicides in conventional vineyards but also copper-based fungicides used in all vineyards including organic are used to control vine foliar pathogens and these can impact fungal soil communities and associated ESs (Probst et al., 2008; Karimi et al., 2020, 2021).
Among the most important soil fungi affecting the vineyard ecosystem are symbiotic arbuscular mycorrhizal fungi (AMF) (Trouvelot et al., 2015). AMF increase grapevine growth and nutrition by providing better access to soil nutrients and activating the regulation of plant transport proteins, particularly phosphate solubilization through acid phosphatases and the uptake of carbohydrates and lipids produced by plants and their dissemination into the soil (Trouvelot et al., 2015; Rich et al., 2017). The nutrient providing service of AMF is not the only one of primary interest in production systems where external inputs of fertilizers are limited (Gosling et al., 2006). It has also been demonstrated that AMF increase tolerance to abiotic stresses, such as water stress, soil salinity, iron chlorosis, and heavy metal toxicity, as well as protecting grapevines from root diseases (Trouvelot et al., 2015). Herbaceous plants used as cover crops may favor AMF in vineyards (Radic et al., 2012) whereas herbicides have been shown to decrease root mycorrhization (Zaller et al., 2018) but also soil AMF spore biomass, vesicles, and propagules in a model system (Zaller et al., 2014). AMF also induce resistance to root nematodes in grapevines (Li et al., 2006), as well as above-ground pathogens (Jung et al., 2012), as they alter biochemical pathways in above-ground grapevine tissue (Krishna et al., 2005). Surprisingly, AMF also alter the content and composition of secondary metabolites in grapevines, including phenols (Krishna et al., 2005).
Ecological Functions Associated With Soil Enzymes
Soil enzymes catalyze biochemical reactions and rate-limiting steps in organic matter decomposition and nutrient cycling in soil, thus controlling whether organic substances are decomposed or stored (Fansler et al., 2005) and influencing plant nutrient availability (Allison and Vitousek, 2005). Microorganisms mainly produce them, but plant debris, root exudates, and soil fauna also contribute to a lesser extent. Enzyme action may be intracellular or extracellular (both in the presence or absence of their originating cells), as well as free or immobilized (Gianfreda and Rao, 2004). Complex macromolecules (e.g., cellulose, lignin, pectin and hemicellulose) are not directly incorporated into cells, and need to be degraded by extracellular enzymes to yield small enough substrates (ca. 600 Da) for absorption into cell (Arnosti, 2011).
Extracellular enzymes, immobilized through association with clay minerals, humic acids, and particulate organic matter, retain significant levels of activity for prolonged periods (Burns, 1978; Dick, 1984; Fansler et al., 2005). The proportion of extracellular enzymes in soil largely varies depending on the type of enzyme and soil (reviewed by Burns et al., 2013). Anyhow, they represent a significant reservoir of potential activity and may even function as the first catalytic response to changes in substrate availability in soils, and they are also generating signal molecules for the microbial community (Caldwell, 2005; Fansler et al., 2005; Burns et al., 2013). Soil-bound enzymes may also be a source of substrate turnover during periods when microbial biomass is low or shut down due to stressed conditions (Stursova and Sinsabaugh, 2008). Their concentration in soils as well and microbial diversity and abundance is highly variable, as proposed by the use of “microbial hotspots and moments” by Kuzyakov and Blagodatskaya (2015). The concentration of substrates induces soil microbial synthesis of extracellular enzymes and one approach to assessing the physiological capabilities of microbial communities is to assay substrate degradation. In this context, measuring the activity of several soil enzymes may be useful for understanding organic matter turnover and inorganic nutrient availability (Dick, 1984).
Soil enzyme activities are a proximal driver of soil functioning, contributing to biogeochemical cycling, organic matter transformation, and nutrient availability. They are also widely recognized as indicators of soil health and candidate “sensors” of changes in soil management or fertility (Badiane et al., 2001; Vepsäläinen et al., 2001). In particular, they have been increasingly used to investigate changes in functions due to anthropogenic impacts (Dick, 1984; Vepsäläinen et al., 2001; Naseby and Lynch, 2002; Lagomarsino et al., 2008). The effectiveness of alternative vineyard strategies on higher enzyme hydrolytic activities has been demonstrated in several experiments applying green manure (Okur et al., 2009), no-tillage (Lagomarsino et al., 2008), grass-covering (Schreck et al., 2012), compost made from the organic fraction of municipal solid waste (Calleja-Cervantes et al., 2015), and biochar-compost mix (Mackie et al., 2015). The most extensively studied group of enzymes with ecological importance in soil are hydrolases, which are involved in the main biogeochemical cycling of elements and release of carbon, nitrogen, phosphorus and sulfur compounds (Caldwell, 2005). Other classes of enzymes exhibit oxidative activities such as laccases described by Theuerl and Buscot (2010); they can enhance the degradation of the most recalcitrant components of organic matter and are closely linked to soil carbon sequestration (Caldwell, 2005). Phenol oxidases and peroxidases, in particular, are key in cellulose and lignin degradation and further condensation of phenols, peptides and carbohydrates leading the formation of secondary humic compounds (Sinsabaugh, 2010). A final group of soil enzymes, poorly assessed, despite their importance for nutrient release, are lyases, which play a fundamental role in the detoxification of phenolics and reactive metals, antimicrobial defense, and the degradation of lignin, polysaccharides, and humic acids (Theuerl and Buscot, 2010).
Agricultural Practices in Vineyards and Threats to Biodiversity and Services
The soil ecosystem is one of the most complex habitats, due to the huge diversity of species, and the huge number of individuals present on a very small surface (Jeffery et al., 2010). A majority of studies mentions the following agricultural practices as threats to soil biodiversity: plowing or tillage, mineral fertilization, pesticide use, irrigation and drainage systems, and weed management (Brussaard et al., 2007; Holland et al., 2013; Tsiafouli et al., 2014; Paungfoo-Lonhienne et al., 2015). Perennial crops—particularly those planted as monocultures in large areas—are frequently associated with an increase in the use of machinery and pesticides, and with a decrease in vegetation cover. All these practices result in soil compaction, and a decrease in soil organic matter, that often is associated with structure disruption and erosion (Lal, 2001; Power, 2010; Tsiafouli et al., 2014).
In agroecosystems, intensive agricultural practices may cause soil degradation or deteriorate soil quality, leading to a decrease in the magnitude of one or more ESs (Lavelle et al., 2006). Agricultural practices increase the level of disturbance (e.g., tillage, mowing) and pollution (fertilizer and pesticide inputs), thereby not only changing physical soil properties but also affecting its biological communities and reducing the complexity of soil food webs and associated ESs (Bommarco et al., 2013; Tsiafouli et al., 2014). These reductions in biodiversity further modify soil functioning and, thus, the services it provides, as well as its resilience and ability to face the consequences of climate change, such as extreme weather events (Wall et al., 2015).
Soil erosion may represent losses of up to several millimeters of soil each year (Quiquerez et al., 2008; Rodrigo Comino et al., 2016). These values are much higher than those observed in natural systems and severely reduce the habitat suitability for a large number of taxa. A 4-year, field-scale experiment comparing four soil management systems revealed that permanent, inter-row grass cover reduced runoff and soil loss, compared to herbicide application resulting in bare soil, whereas results for superficial tillage and temporary grass cover with delayed herbicide treatment were intermediate (Le Bissonnais and Andrieux, 2007).
Chemical fertilizer and pesticide application are responsible for a global decrease in soil biodiversity, functions and services (Wall et al., 2015). The direct effects of mineral fertilizers on soil organisms have generally been described as limited, but their application may enhance soil biological activity through an increase in system productivity, crop residue return, and soil organic matter (Bünemann et al., 2006; Paungfoo-Lonhienne et al., 2015). However, nitrogen fertilization may lead to soil acidification, with considerable negative effects on soil organisms. In addition, a high input of mineral fertilizers negatively impacts plant diversity and community composition (Nascimbene et al., 2013; Paungfoo-Lonhienne et al., 2015). Organic amendments such as manure and compost provide a direct source of carbon for soil organisms and an indirect carbon source via increased plant growth and plant residue returns (Bünemann et al., 2006). For example, in Turkish vineyards, the application of green manure resulted in greater enzyme activity, and microbial biomass and activity (Okur et al., 2009). Compost applications or mulching were shown to have positive effects on soil structure comparable to permanent grass cover (Nachtergaele et al., 1998; Pinamonti, 1998). However, the costs involved in transport and the need for frequent applications may be high. The vineyard management techniques that are most efficient at preventing soil loss are also most favorable for preserving soil organic matter content and associated soil properties, such as aggregate stability (Le Bissonnais and Andrieux, 2007)and biodiversity (Duarte et al., 2014).
Water management in vineyards mainly consists of irrigation, but drainage may also be necessary in vineyards located in floodplains (Abbona et al., 2007). Water management aims to provide grapevines with a more amenable environment and prevent water stress. However, particularly in medium-dry environments, the implementation of water-saving irrigation techniques (e.g., partial rootzone drying) may lead to changes or decreases in the diversity and abundance of soil organisms that prefer a higher moisture content such as earthworms and generalist predators, spiders and ground beetles (Thomson, 2006; Holland et al., 2013).
Pest management techniques aim to reduce the competition between crops and weeds or reduce plant pest and pathogen densities. However, plant diversity and vegetation cover are important drivers of belowground processes: different plant species in crop rotation were shown to influence faunal, microbial, and soil organic matter dynamics via the diversity of root architecture, the quantity and quality of rhizodeposits, and residue biochemistry (Ingels et al., 2005). McDaniel et al. (2014) reported that introducing diversity with inter-row cover crops significantly increased microbial biomass. In addition, vegetation in vineyards also provides food and structure for many arthropod taxa (Altieri et al., 2005), and indirectly reduce pest abundance and support natural enemies (Lewis et al., 1997; Danne et al., 2010). Comparing the effects of pre- and post-emergence herbicide application, surface soil tillage, and no vegetation removal treatments, Collembola were most abundant in plots with vegetation cover, slightly less in post-emergence herbicide application and tillage plots, and least abundant in pre-emergence herbicide plots (Sturm et al., 2002; Renaud et al., 2004). However, herbicide application may increase mesofauna feeding activity in the short term, by providing dead plant material as food for detritivores (Reinecke et al., 2002). Due to the severe disturbance it causes, tillage may be more detrimental for mesofauna than herbicide application (Reinecke et al., 2002). Herbicide applications in vineyards can also affect soil biota with knock-on effects on other parts of the grapevine system, detectable even several months after its application (Mandl et al., 2018; Zaller et al., 2018).
Insecticide application generally reduces the abundance and, in the long term, the diversity not only of target pests but also of non-target species. For example, leafhopper abundance and diversity decreased strongly with insecticide use in Swiss vineyards (Trivellone et al., 2012). However, in a 2-year study comparing 5 vineyards in Arkansas (United States), carabids were more diverse and abundant in vineyards sprayed with insecticides than in abandoned, non-sprayed vineyards, while Hymenopteran parasitoids suffered from insecticide treatments (Williamson and Johnson, 2005). The authors hypothesized that, in the short term, insecticide spraying resulted in more food available for carabids on the vineyard floor.
Little research has been done into the effects of fungicides on biodiversity in vineyards. While some studies showed an effect of sulfur spraying on predacious mites, other studies did not find any negative effects (Walton et al., 2012). Fungicide application alters the grape surface microbial communities, and fungicides used in organic farming (sulfur, copper) have stronger effects than synthetic fungicides (Milanović et al., 2013). In particular, copper fungicides are considered to be among the most toxic, persistent fungicides (Bünemann et al., 2006) and impact several biodiversity groups (Korthals et al., 1996; Karimi et al., 2020, 2021; Ostandie et al., 2021). Copper residues are reported to be responsible for significant reductions in microbial biomass due to stressed microbes in orchards (Merrington et al., 2002) and cause long-term reductions of earthworm populations (Bünemann et al., 2006). Copper has also a major influence on nematodes and this effect was generally enhanced with decreasing soil pH. High copper contaminations significantly reduced the number of bacterial-feeding nematodes, whereas the number of hyphal-feeding nematodes increased (Korthals et al., 1996). Omnivorous and predacious nematodes showed the most sensitive response, until extinction. Plant-feeding nematodes also showed large variations in their abundances that could be related to the effects of copper and pH on primary production (Korthals et al., 1996). As copper accumulates in surface soils, these negative effects are likely to persist for many years.
One of the principles of organic farming states that it protects and benefits the common environment, including biodiversity. However, organic farming regulations do not oblige farmers to use inter-row vegetation cover and scientific evidence for the benefits of organic farming on biodiversity in vineyards is conflicting. In a large-scale study, including vineyards throughout Italy, predatory mite biodiversity was higher in organic and untreated vineyards than conventional ones (Peverieri et al., 2009). A slight increase of spider diversity has been observed in organic vineyards in Germany but no significant effect was reported on spider abundance (Kolb et al., 2020). However, other studies showed less biodiversity in organic vineyards: soil bacteria were less diverse on organic farms than conventional ones (Bonanomi et al., 2016). In a Swiss study, Bruggisser et al. (2010) also failed to detect an increase in plant and spider diversity in organic vineyards, while grasshopper diversity was even significantly lower in organic compared to conventional vineyards. Most of the benefits of organic farming for plants seem to be attributable to the absence of herbicide use (Sanguankeo and León, 2011; Nascimbene et al., 2012; Kehinde and Samways, 2014).
Suggestions for Managing Soil Biodiversity to Ensure Sustainable Viticulture
Trade-Offs Between Grape Production and Biodiversity
Viticulture has a long tradition and management practices have changed over the centuries. The economic importance of wine production, especially of high-quality wines, has also increased and winegrowers tended to focus on consistent production and consider that ecological aspects were of minor importance.
A range of farming practices that increase species diversity and associated functions and services have gradually been adopted by farmers in arable crops: conservation tillage, organic fertilization, natural vegetation cover or cover crops. These techniques can maintain higher levels of biodiversity by providing continuous plant cover and improving organic matter content (Bommarco et al., 2013). In addition, practices such as agroforestry, manure and residue inputs, and soil resting (a long period before replanting of vines) are gaining importance and may help to prevent soil degradation, reduce pesticide inputs and nutrient losses to adjacent habitats. They also indirectly promote the maintenance and conservation of biodiversity and multiple ESs such as pest control on a larger scale (Lewis et al., 1997; Bommarco et al., 2013). Such techniques, trade-offs between services they imply as well as the level of provided services are not well assessed and known for vineyards, nonetheless fundamental if we are to develop sustainable grapevine cropping systems.
Diversifying the management around viney ards or landscape diversification outside the actual production area influences biodiversity in vineyards. They can consist of buffer strips or riparian hedges. For example, remnants of natural habitats within vineyards promoted spider diversity in South African vineyards (Kehinde and Samways, 2014). However, islands or patches of beneficial plant species do not necessarily have to be in the vineyards (Altieri et al., 2005). Semi-natural areas in the surroundings of vineyards have a positive impact on habitat diversity but need to be connected by habitat corridors (Altieri et al., 2005). Alternating vegetation cover in inter-rows, when technically feasible, can maintain a high-biodiversity habitat within a single vineyard. This idea should be combined with current research on pesticide use adapted to local conditions, at the row or even vine-stock level (Llorens et al., 2010).
However, certain management practices require trade-offs between biodiversity and ESs (Bommarco et al., 2013). For example, the use of manure and agricultural compost increases organic matter content, but should be avoided close to water sources such as rivers and lakes, due to possible increased nitrate seepage, resulting in deteriorated water quality. At the same time, farmers may perceive the loss of certain ESs as negligible, while the reduction in yield or crop surface results in a decrease in provisioning ES (Verbruggen et al., 2012), directly linked to tangible economic losses. Such trade-offs, and especially, their consequences should be at the center of future research programs exploring links between biodiversity, ESs, and wine production.
Recently, the ecological aspects of wine production have received more attention, with higher numbers of organic and biodynamic winegrowers and greater awareness among consumers and politicians of the concept of sustainability and the negative effects of high-input viticulture. Sustaining ecosystem functions and services, as well as testing sustainable farming practices, are key issues in recent agroecological and biological conservation research and policy (Batáry et al., 2011). Winegrowers need to find a balance between ecological and economic aspects that may be symbiotic although contradictory in some parts of the production process.
However, environmental protection is often considered to impede the prosperity of enterprises, especially in the agricultural sector. Winegrowers tend to think of environmental-friendly actions as counterproductive for the quality of their wine and their earnings (Gemmrich and Arnold, 2007). Sustainability offers a solution for this conflict by integrating profitability with environmental and social issues. It offers a wholesome approach toward winegrowing and wine making (Gemmrich and Arnold, 2007). One of the major advances achieved by farmers in recent years is the perception that only a small percentage of the invertebrates present in their fields have a negative impact on crops (Lavelle et al., 2006).
There is also a gap between scientific literature and winegrowers’ definitions of the ES concept and its application to viticulture. Both farmers and scientists recognize biodiversity and genetic diversity conservation as important (Brussaard et al., 2010). Viers et al. (2013) linked the sensitivity of wine-makers to ESs with the importance that they seem to attach to the terroir concept and their understanding of soil characteristics. The challenge for winegrowers is to identify the aspects of biodiversity that are desirable to maintain and/or enhance in their vineyards, as they provide specific ecological services, and then determine the best practices for encouraging this biodiversity and the associated ESs (Altieri, 1995; Gliessman, 1998; Winkler et al., 2017).
Lack of Knowledge and Research Gaps in Vineyards
Some aspects of the interaction between biodiversity conservation, management practices, ecosystem functions, and grape quality/yield are obvious and clearly understood, while many others are uncertain or even unknown (see previous sections). For example, insect functional biodiversity is assessed on plot and field scales with a focus on natural enemies to tackle biological control of vine pests (e.g., Danne et al., 2010; Caprio et al., 2015), while others investigate functional micro-biodiversity in the soil, involved in plant health management (Whitelaw-Weckert et al., 2007; Trouvelot et al., 2015). Future research should focus on the impact of viticultural landscape diversification and natural vegetation or cover crop mixtures in vineyard inter-rows on overall biodiversity and associated ESs. The interrelations and trade-offs between the responses of different taxa on field and landscape scales and the consequences for provisioning ES are still largely unexplored. In addition, only a few studies have investigated multiple trophic levels and ESs in vineyards. Furthermore, studies implementing a standardized research protocol in different climate zones are important to assess possible trade-offs and synergies between ESs in vineyards. In addition, in order to promote biodiversity conservation measures, further research is required on the relationships between certain plant species and host-disease transmitting vectors, which may impede the positive effects on biodiversity (Costa et al., 2004).
Relevant topics include the ecological role of specific soil- and plant-associated microbial taxa in vineyards. Plant growth-promoting rhizobacteria increase growth, but may also induce system resistance, thereby enhancing plant defenses (Beneduzi et al., 2012). Some of these mechanisms have been described in model plants, but current knowledge of these aspects in vineyards is limited (Beneduzi et al., 2012). Recently, certain microorganisms have been promoted as soil quality enhancers and responsible for particular organoleptic qualities (Zarraonaindia et al., 2015). These aspects need to be investigated under different soil and climate conditions keeping an eye also on nutrient cycles and nutrient availability. This topic also nicely illustrates the complexity of research tasks and the different disciplines which need to get involved.
Future Management Options and Research Directions
Currently vineyard management range from no disturbance at all (permanent plant cover) to high disturbance (regular soil tillage or herbicide application). Permanent cover and cover crops provide numerous benefits in terms of ESs, which are beneficial for the vineyard environment and increase grape quality (Biddoccu et al., 2016; Chrysargyris et al., 2018; Garcia et al., 2018). Cover crops have been found to increase soluble solids, anthocyanins and other phenolic components of grapes (Guerra and Steenwerth, 2012), increase microbial biomass of soil (Ingels et al., 2005) while controlling grapevine canopy growth (Tesic et al., 2007). However, they may compete for nutrient supply and nitrogen as observed in several European vineyards by Griesser et al. (2022).
Cover crops could be also implemented for in-row weed control, even if knowledge about in-row cover crops is scarce (Garcia et al., 2018). Use of in-row vegetation cover would avoid herbicide applications or mechanical weed control (Garcia et al., 2018), thereby indirectly and positively affecting inter-rows through the reduction of soil compaction and the number of tractor passages. Winegrowers are concerned about cover crops that may compete with vines for nutrients and water, but specific mixtures may avoid these negative effects and could provide higher biodiversity within vineyards (Garcia et al., 2018; Griesser et al., 2022). Using native plants and selecting particular species and traits within the natural pool has been also proposed as a potential solution for managing the soil under the vine rows (Tompkins, 2010). Furthermore, the application of mulch under vines during winter has been demonstrated to disturb the cycle of the gray mould fungus, Botrytis cinerea, and reduce its pressure by up to 70% (Jacometti et al., 2007). Studies using different plant mixtures and evaluating their impact on vine physiology, as well as grape quality, are pending, but are likely to produce important findings for adapting in-row management toward increased sustainability, less disturbance, and, possibly, promoting biodiversity.
The combined need for consistent yields of high-quality grapes, a reduction in external inputs, and climatic challenges with prolonged periods of drought, heavy rainfall, and prolonged growing seasons, makes it difficult for winegrowers to make informed decisions (Chrysargyris et al., 2018). It is necessary to develop risk management tools and adapt them to local conditions, including aspects of soil preservation, plant physiology, and pesticide management. Existing models have proved their effectiveness for pest management, but do not provide a thorough evaluation of the effects of pesticide applications on soil organisms.
Furthermore, awareness of sustainable crop production has increased among consumers, as well as producers. Winegrowers need reliable, basic knowledge of the effects of their production systems on biodiversity and other ecosystem functions, and implementing new measures need to be knowledge based. This knowledge has to be provided by scientists in terms of cultivar selection (cultivars resistant to biotic and abiotic stresses), trellising systems, canopy and cover-crop management, soil cultivation, winemaking processes, storage, and the use of renewable energy. Due to the increasing influence of international wine organizations, threats linked to climate change and new market opportunities, the wine sector has become more aware of the concept of sustainability (Hannah et al., 2013; Viers et al., 2013; Litskas et al., 2020). The global environmental footprint of wine production could be quantified and other impacts of practices on the use of resources or human health have to be measured and compared to biodiversity and environmental impacts (Litskas et al., 2020). Growing numbers of sustainable winemaking projects on local, regional, and national scales identified a number of ESs provided by vineyard soils that led to the development of locally-adapted methods and educational programs (Viers et al., 2013; Santiago-Brown et al., 2014).
Author Contributions
BG, SW, SB, EAC, JZ, and IL conceived of the presented idea, based on European projects ReSolVe, PromESSinG and VineDivers. All authors contributed to the article and approved the submitted version.
Funding
The review formed part of the PromESSinG and VineDivers projects, funded by the 2013–2014 BiodivERsA/FACCE JPI joint call for research proposals with the national funders Bundesministerium für Bildung und Forschung (BMBF, Germany), Agence Nationale de la Recherche (ANR, France), Executive Agency for Higher Education, Research, Development and Innovation Funding (UEFISCDI, Romania), Ministerio des economía y competitividad (MINECO, Spain), FWF-Der Wissenschaftsfonds (Austria) and the Swiss National Science Foundation (SNSF, Switzerland Grant No: 40FA40_158390). The contract of BG was supported in 2017 by the European Union Seventh Framework Program via a grant from the PromESSinG project. Contributions to this review were also supported by the research project ReSolVe funded by transnational funding bodies, being partners of the FP7 ERA-net project, CORE Organic Plus, and the cofound from the European Commission; and by the research project SECBIVIT which was funded through the 2017–2018 Belmont Forum and BiodivERsA joint call for research proposals, under the BiodivScen ERA-Net COFUND program, and with the funding organizations: AEI/Spain, BMBF/Germany, ANR/France, NWO/Netherlands, UEFISCDI/Romania, FWF/Austria (Grant No: I 4025-B32) and the NSF/United States (Grant No: 1850943). Researches on soil biodiversity conducted in the SAVE unit (BG and ARu) were supported by the Conseil Interprofessionel des Vins de Bordeaux CIVB during the Phytae project (2018–2020) and the Endless project (2021–2024).
Conflict of Interest
The authors declare that the research was conducted in the absence of any commercial or financial relationships that could be construed as a potential conflict of interest.
Publisher’s Note
All claims expressed in this article are solely those of the authors and do not necessarily represent those of their affiliated organizations, or those of the publisher, the editors and the reviewers. Any product that may be evaluated in this article, or claim that may be made by its manufacturer, is not guaranteed or endorsed by the publisher.
Footnotes
References
Abbona, E. A., Sarandón, S. J., Marasas, M. E., and Astier, M. (2007). Ecological sustainability evaluation of traditional management in different vineyard systems in Berisso, Argentina. Agric. Ecosyst. Environ. 119, 335–345. doi: 10.1016/j.agee.2006.08.001
Allison, S. D., and Vitousek, P. M. (2005). Responses of extracellular enzymes to simple and complex nutrient inputs. Soil Biol. Biochem. 37, 937–944. doi: 10.1016/j.soilbio.2004.09.014
Altieri, M. A. (1995). Agroecology: the Science of Sustainable Agriculture. Boulder: Westview Press.
Altieri, M. A., Ponti, L., and Nichols, C. I. (2005). Manipulating vineyard biodiversity for improved insect pest management: case studies from northern California. Int. J. Biodivers. Sci. Manage. 1, 191–203. doi: 10.1080/17451590509618092
Andret-Link, P., Schmitt-Keichinger, C., Demangeat, G., Komar, V., and Fuchs, M. (2004). The specific transmission of Grapevine fanleaf virus by its nematode vector Xiphinema index is solely determined by the viral coat protein. Virology 320, 12–22. doi: 10.1016/j.virol.2003.11.022
Arnaez, J., Lasanta, T., Ruiz-Flano, P., and Ortigosa, L. (2007). Factors affecting runoff and soil erosion under simulated rainfall in Mediterranean vineyards. Soil Till. Res. 93, 324–334. doi: 10.1016/j.still.2006.05.013
Arnosti, C. (2011). Microbial extracellular enzymes and the marine carbon cycle. Ann. Rev. Mar. Sci. 3, 401–425. doi: 10.1146/annurev-marine-120709-142731
Badiane, N. N. Y., Chotte, J. L., Pate, E., Masse, D., and Rouland, C. (2001). Use of soil enzyme activities to monitor soil quality in natural and improved fallows in semi-arid tropical regions. Appl. Soil Ecol. 18, 229–238. doi: 10.1016/S0929-1393(01)00159-7
Bagella, S., Filigheddu, R., Caria, M. C., Girlanda, M., and Roggero, P. P. (2014). Contrasting land uses in Mediterranean agro-silvo-pastoral systems generated patchy diversity patterns of vascular plants and below-ground microorganisms. C.R. Biol. 337, 717–724. doi: 10.1016/j.crvi.2014.09.005
Baronio, C. A., Botton, M., Gomes, S. R., and Robinson, D. G. (2014). First record of qualitative losses caused by Meghimatium pictum in vineyards of Southern Brazil and the effects of two molluscicides for its control. Cienc. Rural. 44, 1715–1720. doi: 10.1590/0103-8478cr20130522
Batáry, P., Báldi, A., Kleijn, D., and Tscharntke, T. (2011). Landscape-moderated biodiversity effects of agri-environmental management – a meta-analysis. Proc. Biol. Sci. 278, 1894–1902. doi: 10.1098/rspb.2010.1923
Baumgartner, K., Steenwerth, K. L., and Veilleux, L. (2008). Cover-crop systems affect weed communities in a California vineyard. Weed Sci. 56, 596–605. doi: 10.1614/ws-07-181.1
Behan-Pelletier, V. M. (1999). Oribatid mite biodiversity in agroecosystems: role for bioindication. Agr. Ecosys. Env. 74, 411–423.
Beneduzi, A., Ambrosini, A., and Passaglia, L. M. P. (2012). Plant growth-promoting rhizobacteria (PGPR): their potential as antagonists and biocontrol agents. Genet. Mol. Biol. 35, 1044–1051. doi: 10.1590/S1415-47572012000600020
Bianchi, F. J. J., Booij, C. J., and Tscharntke, T. (2006). Sustainable pest regulation in agricultural landscapes: a review on landscape composition, biodiversity and natural pest control. Proc. Biol. Sci. 273, 1715–1727. doi: 10.1098/rspb.2006.3530
Biarnès, A., Rio, P., and Hocheux, A. (2004). Analyzing the determinants of spatial distribution of weed control practices in a Languedoc vineyard catchment. Agronomie 24, 187–196. doi: 10.1051/agro:2004018
Biddoccu, M., Ferraris, S., Opsi, F., and Cavallo, E. (2016). Long-term monitoring of soil management effects on runoff and soil erosion in sloping vineyards in Alto Monferrato (North-West Italy). Soil Till. Res. 155, 176–189. doi: 10.1016/j.still.2015.07.005
Blavet, D., De Noni, G., Le Bissonnais, Y., and Leonard, M. (2009). Effect of land use and management on the early stages of soil water erosion in French Mediterranean vineyards. Soil Till. Res. 106, 124–136. doi: 10.1016/j.still.2009.04.010
Blouin, M., Hodson, M. E., Delgado, E. A., Baker, G., Brussaard, L., Butt, K. R., et al. (2013). A review of earthworm impact on soil function and ecosystem services. Eur. J. Soil Sci. 64, 161–182. doi: 10.1111/ejss.12025
Bokulich, N. A., Thorngate, J. H., Richardson, P. M., and Mills, D. A. (2014). Microbial biogeography of wine grapes is conditioned by cultivar, vintage, and climate. Proc. Natl. Acad. Sci. U.S.A. 111, 139–148. doi: 10.1073/pnas.1317377110
Bommarco, R., Kleijn, D., and Potts, S. G. (2013). Ecological intensification: harnessing ecosystem services for food security. Trends Ecol. Evol. 28, 230–238. doi: 10.1016/j.tree.2012.10.012
Bonanomi, G., De Filippis, F., Cesarano, G., La Storia, A., Ercolini, D., and Scala, F. (2016). Organic farming induces changes in soil microbiota that affect agro-ecosystem functions. Soil Biol. Biochem. 103, 327–336. doi: 10.1016/j.soilbio.2016.09.005
Bongers, T. (1990). The maturity index: an ecological measure of environmental disturbance based on nematode species composition. Oecologia 83, 14–19. doi: 10.1007/BF00324627
Brennan, A., Fortune, T., and Bolger, T. (2006). Collembola abundances and assemblage structures in conventionally tilled and conservation tillage arable systems. Pedobiologia 50, 135–145. doi: 10.1016/j.pedobi.2005.09.004
Bruggisser, O. T., Schmidt-Entling, M. H., and Bacher, S. (2010). Effects of vineyard management on biodiversity at three trophic levels. Biol. Conserv. 143, 1521–1528. doi: 10.1016/j.biocon.2010.03.034
Brussaard, L., Carron, P., Campbell, B., Lipper, L., Mainka, S., Rabbinge, R., et al. (2010). Reconciling biodiversity conservation and food security: scientific challenges for a new agriculture. Curr. Opin. Environ. Sustain. 2, 34–42. doi: 10.1016/j.cosust.2010.03.007
Brussaard, L., De Ruiter, P. C., and Brown, G. G. (2007). Soil biodiversity for agricultural sustainability. Agric. Ecosys. Environ. 121, 233–244. doi: 10.1016/j.agee.2006.12.013
Buchholz, J., Querner, P., Paredes, D., Bauer, T., Strauss, P., Guernion, M., et al. (2017). Soil biota in vineyards are more influenced by plants and soil quality than by tillage intensity or the surrounding landscape. Sci. Rep. 7:17745. doi: 10.1038/s41598-017-17601-w
Buckley, D. H., and Schmidt, T. M. (2001). The structure of microbial communities in soil and the lasting impact of cultivation. Microb. Ecol. 42, 11–21. doi: 10.1007/s002480000108
Bulgarelli, D., Schlaeppi, K., Spaepen, S., Ver Loren van Themaat, E., and Schulze-Lefert, P. (2013). Structure and functions of the bacterial microbiota of plants. Annu. Rev. Plant Biol. 64, 807–838. doi: 10.1146/annurev-arplant-050312-120106
Bundt, M., Widmer, F., Pesaro, M., Zeyer, J., and Blaser, P. (2001). Preferential flow paths: biological ‘hot spots’ in soils. Soil Biol. Biochem. 33, 729–738.
Bünemann, E. K., Schwenke, G. D., and van Zwieten, L. (2006). Impact of agricultural inputs on soil organisms - a review. Aust. J. Soil Res. 44, 379–406.
Burns, K. N., Kluepfel, D. A., Strauss, S. L., Bokulich, N. A., Cantu, D., and Steenwerth, K. L. (2015). Vineyard soil bacterial diversity and composition revealed by 16S rRNA genes: differentiation by geographic features. Soil Biol. Biochem. 91, 232–247. doi: 10.1016/j.soilbio.2015.09.002
Burns, R. G., DeForest, J. L., Marxsen, J., Sinsabaugh, R. L., Stromberger, M. E., Wallenstein, M. D., et al. (2013). Soil enzymes in a changing environment: current knowledge and future directions. Soil Biol. Biochem. 58, 216–234. doi: 10.1016/j.soilbio.2012.11.009
Caldwell, B. A. (2005). Enzyme activities as a component of soil biodiversity: a review. Pedobiologia 49, 637–644. doi: 10.1016/j.pedobi.2005.06.003
Calleja-Cervantes, M. R., Fernández-González, A. J., Irigoyen, I., Fernández-López, M., Aparicio-Tejo, P. M., and Menéndez, S. (2015). Thirteen years of continued application of composted organic wastes in a vineyard modify soil quality characteristics. Soil Biol. Biochem. 90, 241.254. doi: 10.1016/j.soilbio.2015.07.002
Capowiez, Y., Cadoux, S., Bouchant, P., Ruy, S., Roger-Estrade, J., Richard, G., et al. (2009). The effect of tillage type and cropping system on earthworm communities, macroporosity and water infiltration. Soil Till. Res. 105, 209–216. doi: 10.1016/j.still.2009.09.002
Caprio, E., Nervo, B., Isaia, M., Allegro, G., and Rolando, A. (2015). Organic versus conventional systems in viticulture: comparative effects on spiders and carabids in vineyards and adjacent forests. Agr. Syst. 136, 61–69. doi: 10.1016/j.agsy.2015.02.009
Cerdan, O., Govers, G., Le Bissonnais, Y., Van Oostd, K., Poesen, J., Sabye, N., et al. (2010). Rates and spatial variations of soil erosion in Europe: a study based on erosion plot data. Geomorphology 122, 167–177. doi: 10.1016/j.geomorph.2010.06.011
Chang, C. (2002). “Bradybaena similaris (de Férussac) (Bradybaenidae) as a pest in grapevines of Taiwan,” in Molluscs as Crop Pests, ed. G. M. Barker (Wallingford: CABI Publishing), 241–245.
Chaplin-Kramer, R., O’Rourke, M. E., Blitzer, E. J., and Kremen, C. (2011). A meta-analysis of crop pest and natural enemy response to landscape complexity. Ecol. Lett. 14, 922–932. doi: 10.1111/j.1461-0248.2011.01642.x
Chiellini, C., Maida, I., Emiliani, G., Mengoni, A., Mocali, S., and Fabiani, A. (2014). Endophytic and rhizospheric bacterial communities isolated from the medicinal plants Echinacea purpurea and Echinacea angustifolia. Int. Microbiol. 17, 165–174. doi: 10.2436/20.1501.01.219
Chong, C.-S., D’Alberto, C. F., Thomson, L. J., and Hoffman, A. A. (2010). Influence of native ants on arthropod communities in a vineyard. Agr. Forest Entomol. 12, 223–232. doi: 10.1111/j.1461-9563.2010.00472.x
Chrysargyris, A., Xylia, P., Litskas, V., Mandoulaki, A., Antoniou, D., Boyias, T., et al. (2018). Drought stress and soil management practices in grapevines in Cyprus under the threat of climate change. J. Wat. Clim. Change 9, 703–714. doi: 10.2166/wcc.2018.135
Cocco, A., Pacheco, da Silva, V. C., Benelli, G., Botton, M., Lucchi, A., et al. (2021). Sustainable management of the vine mealybug in organic vineyards. J. Pest. Sci. 94, 153–185. doi: 10.1007/s10340-020-01305-8
Coleman, D. C., Crossley, D. A., and Hendrix, P. F. (2004). Fundamentals of Soil Ecology, 2nd Edn. Amsterdam: Elsevier Academic Press, doi: 10.1017/S0014479704232616
Coll, P., Le Cadre, E., and Villenave, C. (2012). How are nematode communities affected during a conversion from conventional to organic farming in southern French vineyards? Nematology 14, 665–676. doi: 10.1163/156854112X624195
Costa, H. S., Raetz, E., Pinckard, T. R., Gispert, C., Hernandez-Martinez, R., Dumenyo, C. K., et al. (2004). Plant hosts of Xylella fastidiosa in and near southern California vineyards. Plant Dis. 88, 1255–1261. doi: 10.1094/PDIS.2004.88.11.1255
Costantini, E. A. C., Agnelli, A. E., Fabiani, A., Gagnarli, E., Mocali, S., Priori, S., et al. (2015). Short-term recovery of soil physical, chemical, micro- and mesobiological functions in a new vineyard under organic farming. Soil 1, 443–457. doi: 10.5194/soil-1-443-2015
Costantini, E. A. C., and Barbetti, R. (2008). Environmental and visual impact analysis of viticulture and olive tree cultivation in the Province of Siena (Italy). Eur. J. Agron. 28, 412–426. doi: 10.1016/j.eja.2007.11.012
Costantini, E. A. C., Bucelli, P., and Priori, S. (2012). Quaternary landscape history determines the soil functional characters of terroir. Quatern. Int. 265, 63–73. doi: 10.1016/j.quaint.2011.08.021
Costantini, E. A. C., Lorenzetti, R., and Malorgio, G. (2016). A multivariate approach for the study of environmental drivers of wine economic structure. Land Use Policy 57, 53–63. doi: 10.1016/j.landusepol.2016.05.015
Costantini, E. A., Castaldini, M., Diago, M. P., Giffard, B., Lagomarsino, A., and Schroers, H. J. (2018). Effects of soil erosion on agro-ecosystem services and soil functions: a multidisciplinary study in nineteen organically farmed European and Turkish vineyards. J. Env. Manag. 223, 614–624. doi: 10.1016/j.jenvman.2018.06.065
Culliney, T. W. (2013). Role of arthropods in maintaining soil fertility. Agriculture 3, 629–659. doi: 10.3390/agriculture3040629
d’Errico, G., Crescenzi, A., and Landi, S. (2014). First report of the Southern Root Knot Nematode Meloidogyne incognita in the invasive weed Araujia sericifera in Italy. Plant Dis. 98, 1593–1594. doi: 10.1094/PDIS-06-14-0584-PDN
Danne, A., Thomson, L. J., Sharley, D. J., Penfold, C. M., and Hoffmann, A. A. (2010). Effects of native grass cover crops on beneficial and pest invertebrates in Australian vineyards. Environ. Entomol. 39, 970–978. doi: 10.1603/EN09144
Dias, A. C. F., Dini-Andreote, F., Hannula, S. E., Andreote, F. D., Pereira e Silva, M., and Falcäo Salles, J. (2013). Different selective effects on rhizosphere bacteria exerted by genetically modified versus conventional potato lines. PLoS One 8:e67948. doi: 10.1371/journal.pone.0067948
Dick, W. A. (1984). Influence of long-term tillage and crop rotation combinations on soil enzyme activities. Soil Sci. Soc. Am. J. 48, 569–574.
Doran, J. W., and Zeiss, M. R. (2000). Soil health and sustainability: managing the biotic component of soil quality. Appl. Soil Ecol. 15, 3–11. doi: 10.1016/S0929-1393(00).00067-6
Duarte, J., Farfán, M. A., Fa, J. E., and Vargas, J. M. (2014). Soil conservation techniques in vineyards increase passerine diversity and crop use by insectivorous birds. Bird Study 61, 193–203. doi: 10.1080/00063657.2014.901294
Duda, M. (2016). The efficiency of landscape management on selected thermophilous land snails - a small-scale case report from the vineyard area in northern Vienna. J. Prot. Mt Areas Res. 8, 22–32. doi: 10.1553/eco.mont-8-2s22
Dvořáková, J., and Horsák, M. (2012). Variation of snail assemblages in hay meadows: disentangling the predictive power of abiotic environment and vegetation. Malacologia 55, 151–162. doi: 10.4002/040.055.0110
Eijsackers, H., Beneke, P., Maboeta, M., Louw, J. P. E., and Reinecke, A. J. (2005). The implications of copper fungicide usage in vineyards for earthworm activity and resulting sustainable soil quality. Ecotox. Environ. Safe 62, 99–111. doi: 10.1016/jecoenv200502017
Faber, F., Wachter, E., and Zaller, J. G. (2017). Earthworms are little affected by reduced soil tillage methods in vineyards. Plant Soil Environ. 63, 257–263. doi: 10.17221/160/2017-PSE
Fansler, S. J., Smith, J. L., Bolton, H. J., and Bailey, V. L. (2005). Distribution of two C cycle enzymes in soil aggregates of a prairie chronosequence. Biol. Fert. Soils 42, 17–23. doi: 10.1007/s00374-005-0867-2
Ferris, H., and Tuomisto, H. (2015). Unearthing the role of biological diversity in soil health. Soil Biol. Biochem. 85, 101–109. doi: 10.1016/j.soilbio.2015.02.037
Ferris, H., Bongers, T., and de Goede, R. G. M. (2001). A framework for soil food web diagnostics: extension of nematode faunal analysis concept. Appl. Soil Ecol. 18, 13–29. doi: 10.1016/S0929-1393(01)00152-4
Fiera, C., Ulrich, W., Popescu, D., Buchholz, J., Querner, P., and Bunea, C.-I. (2020). Tillage intensity and herbicide application influence surface-active springtail (Collembola) communities in Romanian vineyards. Agric. Ecosys. Environ. 300:107006. doi: 10.1016/j.agee.2020.107006
Fierer, N., Bradford, M. A., and Jackson, R. B. (2007). Towards an ecological classification of soil bacteria. Ecology 88, 1354–1364. doi: 10.1890/05-1839
Fourie, J. C., and Freitag, K. (2010). Soil Management in the Breede River Valley Wine Grape Region, South Africa. 2. Soil Temperature. S. Afr. J. Enol. Vitic. 31, 165–168.
Francioli, D., Schulz, E., Lentendu, G., Wubet, T., Buscot, F., and Reitz, T. (2016). Mineral vs. organic amendments: microbial community structure, activity and abundance of agriculturally relevant microbes are driven by long-term fertilization strategies. Front. Microbiol. 7:1446. doi: 10.3389/fmicb.2016.01446
Franco, W., Benavides, S., Valencia, P., Ramírez, C., and Urtubia, A. (2021). Native yeasts and lactic acid bacteria isolated from spontaneous fermentation of seven grape cultivars from the Maule region (Chile). Foods 10:1737. doi: 10.3390/foods10081737
Gagnarli, E., Goggioli, D., Tarchi, F., Guidi, S., Nannelli, R., Vignozzi, N., et al. (2015). Study case of microarthropod communities to assess soil quality in different managed vineyards. Soil 1, 527–536. doi: 10.5194/soil-1-527-2015
Gago, P., Cabaleiro, C., and García, J. (2007). Preliminary study of the effect of soil management systems on the adventitious flora of a vineyard in northwestern Spain. Crop. Prot. 26, 584–591. doi: 10.1016/j.cropro.2006.05.012
Garcia, L., Celette, F., Gary, C., Ripoche, A., Valdés-Gómez, H., and Metay, A. (2018). Management of service crops for the provision of ecosystem services in vineyards: a review. Agr. Ecosyst. Environ. 251, 158–170. doi: 10.1016/j.agee.2017.09.030
García-Orenes, F., Roldán, A., Morugán-Coronado, A., Linares, C., Cerdà, A., and Caravaca, F. (2016). Organic fertilization in traditional mediterranean grapevine orchards mediates changes in soil microbial community structure and enhances soil fertility. Land. Degrad. Dev. 27, 1622–1628. doi: 10.1002/ldr.2496
Gaupp-Berghausen, M., Hofer, M., Rewald, B., and Zaller, J. G. (2015). Glyphosate-based herbicides reduce the activity and reproduction of earthworms and lead to increased soil nutrient concentrations. Sci. Rep. 5:12886. doi: 10.1038/srep12886
Geisen, S., Koller, R., Hünninghaus, M., Dumack, K., Urich, T., and Bonkowski, M. (2016). The soil food web revisited?: diverse and widespread mycophagous soil protists. Soil Biol. Biochem. 94, 10–18. doi: 10.1016/j.soilbio.2015.11.010
Geisseler, D., and Scow, K. M. (2014). Long-term effects of mineral fertilizers on soil microorganisms – A review. Soil Biol. Biochem. 75, 54–63. doi: 10.1016/j.soilbio.2014.03.023
Geldenhuys, M., Gaigher, R., Pryke, J. S., and Samways, M. J. (2021). Diverse herbaceous cover crops promote vineyard arthropod diversity across different management regimes. Agr. Ecosyst. Environ. 307:10722. doi: 10.1016/j.agee.2020.107222
Gemmrich, A. R., and Arnold, R. C. G. (2007). Sustainable winegrowing, is it sustainable or just another fad? - An international overview. Annals Agr. Sci. 5, 87–90.
Ghiglieno, I., Simonetto, A., Orlando, F., Donna, P., Tonni, M., Valenti, L., et al. (2020). Response of the Arthropod Community to Soil Characteristics and Management in the Franciacorta Viticultural Area (Lombardy. Italy). Agronomy 10:740. doi: 10.3390/agronomy10050740
Gianfreda, L., and Rao, M. A. (2004). Potential of extra cellular enzymes in remediation of polluted soils: a review. Enzyme Microb. Tech. 35, 339–354. doi: 10.1016/j.enzmictec.2004.05.006
Giese, G., Velasco-Cruz, C., Roberts, L., Heitman, J., and Wolf, T. K. (2014). Complete vineyard floor cover crops favorably limit grapevine vegetative growth. Sci. Hortic. 170, 256–266. doi: 10.1016/j.scienta.2014.03.011
Gilbert, J. A., van der Lelie, D., and Zarraonaindia, I. (2014). Microbial terroir for wine grapes. Proc. Natl. Acad. Sci. Biol.U.S.A. 111, 5–6. doi: 10.1073/pnas.1320471110
Gillespie, M., and Wratten, S. D. (2012). The importance of viticultural landscape features and ecosystem service enhancement for native butterflies in New Zealand vineyards. J. Insect. Conserv. 16, 13–23. doi: 10.1007/s10841-011-9390-y
Girvan, M., and Bullimore, J. (2003). Soil type is the primary determinant of the composition of the total and active bacterial communities in arable soils. Appl. Environ. Microb. 69, 1800–1809. doi: 10.1128/AEM.69.3.1800-1809.2003
Gliessman, S. R. (1998). Agroecology: Ecological Processes in Sustainable Agriculture. Boca Raton, FL: CRC Press.
Gonçalves, F., Nunes, C., Carlos, C., Lopez, A., Oliveira, I., Crespi, A., et al. (2020). Do soil management practices affect the activity density, diversity, and stability of soil arthropods in vineyards? Agr. Ecosyst. Envir. 294:106893. doi: 10.1016/j.agee.2020.106863
Gosling, P., Hodge, A., Goodlass, G., and Bending, G. D. (2006). Arbuscular mycorrhizal fungi and organic farming. Agr. Ecosyst. Environ. 113, 17–35. doi: 10.1016/j.agee.2005.09.009
Govaerts, B., Fuentes, M., Mezzalama, M., Nicol, J. M., Deckers, J., Etchevers, J. D., et al. (2007). Infiltration, soil moisture, root rot and nematode populations after 12 years of different tillage, residue and crop rotation managements. Soil Till. Res. 94, 209–219. doi: 10.1016/j.still.2006.07.013
Griesser, M., Steiner, M., Pingel, M., Uzman, D., Preda, C., Giffard, B., et al. (2022). General trends of different inter-row vegetation management affecting vine vigor and grape quality across European vineyards. Agric. Ecosyst. Environ. 338:108073. doi: 10.1016/j.agee.2022.108073
Grime, J. P. (2006). Trait convergence and trait divergence in herbaceous plant communities: mechanisms and consequences. J. Veg. Sci. 17, 255–260. doi: 10.1111/j.1654-1103.2006.tb02444.x
Guerra, B., and Steenwerth, K. (2012). Influence of floor management technique on grapevine growth, disease pressure, and juice and wine composition: a review. Am. J. Enol. Vitic. 63, 149–164. doi: 10.5344/ajev.2011.10001
Gyssels, G., Poesen, J., Bochet, E., and Li, Y. (2005). Impact of plant roots on the resistance of soils to erosion by water: a review. Prog. Phys. Geog. 29, 189–217. doi: 10.1191/0309133305pp443ra
Hall, R. M., Penke, N., Kriechbaum, M., Kratschmer, S., Jung, V., and Chollet, S. (2020). Vegetation management intensity and landscape diversity alter plant species richness, functional traits and community composition across European vineyards. Agric. Syst. 177:102706. doi: 10.1016/j.agsy.2019.102706
Halleen, F., Schroers, H.-J., Groenewald, J. Z., and Crous, P. W. (2004). Novel species of Cylindrocarpon (Neonectria) and Campylocarpon gen. nov. associated with black foot disease of grapevines (Vitis spp). Stud. Mycol. 50, 431–455.
Hanna, R., Zalom, F. G., and Roltsch, W. J. (2003). Relative impact of spider predation and cover crop on population dynamics of Erythroneura variabilis in a raisin grape vineyard. Entomol. Exp. Appl. 107, 177–191. doi: 10.1046/j.1570-7458.2003.00051.x
Hannah, L., Roehrdanz, P. R., Ikegami, M., Shepard, A. V., Shaw, M. R., Tabord, G., et al. (2013). Climate change, wine, and conservation. Proc. Natl. Acad. Sci. U.S.A. 110, 6907–6912. doi: 10.1073/pnas.1210127110
Herrick, J. E. (2000). Soil quality: an indicator of sustainable land management? Appl. Soil Ecol. 15, 75–83. doi: 10.1016/S0929-1393(00)00073-1
Hervé, M. E. T., Boudes, P., Cieslik, C., Montembault, D., Jung, V., Burel, F., et al. (2020). Landscape complexity perception and representation in a wine-growing region with the designation of origin in the Loire Valley (France): a cultural ecosystem service? Ren. Agr. Food Syst. 35, 77–89. doi: 10.1017/S1742170518000273
Hettenbergerová, E., Horsák, M., Chandran, R., Hájek, M., Zeleny, D., and Dvoøáková, J. (2013). Patterns of land snail assemblages along a fine-scale moisture gradient. Malacologia 56, 31–42. doi: 10.4002/040.056.0227
Hogg, B. N., and Daane, K. M. (2010). The role of dispersal from natural habitat in determining spider abundance and diversity in California vineyards. Agr. Ecosyst. Environ. 135, 260–267. doi: 10.1016/j.agee.2009.10.004
Holland, J. M. (2004). The environmental consequences of adopting conservation tillage in Europe: reviewing the evidence. Agr. Ecosyst. Environ. 103, 1–25. doi: 10.1016/j.agee.2003.12.018
Holland, T. C., Reynolds, A. G., Bowen, P. A., Bogdanoff, C. P., Marciniak, M., Brown, R. B., et al. (2013). The response of soil biota to water availability in vineyards. Pedobiologia 56, 9–14.
Ingels, C. A., Scow, K. M., Whisson, D. A., and Drenovsky, R. E. (2005). Effects of cover crops on grapevines, yield, juice composition, soil microbial ecology, and gopher activity. Am. J. Enol. Vitic. 56, 19–29.
Irvin, N. A., Bistline-East, A., and Hoddle, M. S. (2016). The effect of an irrigated buckwheat cover crop on grape vine productivity, and beneficial insect and grape pest abundance in southern California. Biol. Control 93, 72–83. doi: 10.1016/j.biocontrol.2015.11.009
Jacometti, M. A., Wratten, S. D., and Walter, M. (2007). Understorey management increases grape quality, yield and resistance to Botrytis cinerea. Agr. Ecosyst. Environ. 122, 349–356. doi: 10.1016/j.agee.2007.01.021
James, D. G., Seymour, L., Lauby, G., and Buckley, K. (2015). Beauty with benefits: butterfly conservation in Washington State. USA, wine grape vineyards. J. Insect Conserv. 19, 341–348. doi: 10.1007/s10841-015-9761-x
Jeffery, S., Gardi, C., Jones, A., Montanarella, L., Marmo, L., Miko, L., et al. (2010). European Atlas of Soil Biodiversity. Luxembourg: Publications Office of the European Union.
Joimel, S., Schwartz, C., Hedde, M., Kiyota, S., Krogh, P. H., Nahmani, J., et al. (2017). Urban and industrial land uses have a higher soil biological quality than expected from physicochemical quality. Sci. Total Environ. 584-585, 614–621. doi: 10.1016/j.scitotenv.2017.01.086
Jonas, J. L., Wilson, G. W. T., White, P. M., and Joern, A. (2007). Consumption of mycorrhizal and saprophytic fungi by Collembola in grassland soils. Soil Biol. Biochem. 39, 2594–2602. doi: 10.1016/j.soilbio.2007.05.004
Jones, C. G., Lawton, J. H., and Shachak, M. (1994). “Organisms as Ecosystem Engineers,” in Ecosystem Management, eds F. B. Samson and F. L. Knopf (New York, NY: Springer).
Jung, S. C., Martinez-Medina, A., Lopez-Raez, J. A., and Pozo, M. J. (2012). Mycorrhiza-induced resistance and priming of plant defenses. J. Chem. Ecol. 38, 651–664. doi: 10.1007/s10886-012-0134-6
Karimi, B., Cahurel, J.-Y., Gontier, L., Charlier, L., Chovelon, M., Mahé, H., et al. (2020). A meta-analysis of the ecotoxicological impact of viticultural practices on soil biodiversity. Environ. Chem. Lett. 18, 1947–1966.
Karimi, B., Masson, V., Guilland, V., Leroy, E., Pellegrinelli, S., Giboulot, E., et al. (2021). Ecotoxicity of copper input and accumulation for soil biodiversity in vineyards. Environ. Chem. Lett. 19, 2013–2030.
Kazakou, E., Fried, G., Richarte, J., Gimenez, O., Violle, C., and Metay, A. (2016). A plant trait-based response-and-effect framework to assess vineyard inter-row soil management. Bot. Lett. 163, 373–388. doi: 10.1080/23818107.2016.1232205
Kehinde, T., and Samways, M. J. (2014). Management defines species turnover of bees and flowering plants in vineyards. Agr. Forest Entomol. 16, 95–101. doi: 10.1111/afe.12038
Kolb, S., Uzman, D., Leyer, I., Reineke, A., and Entling, M. H. (2020). Differential effects of semi-natural habitats and organic management on spiders in viticultural landscapes. Agr. Ecosys. Environ. 287:106695. doi: 10.1016/j.agee.2019.106695
Korthals, G. W., Alexiev, A. D., Lexmond, T. M., Kammenga, J. E., and Bongers, T. (1996). Long-term effects of copper and pH on the nematode community in an agroecosystem. Environ. Tox. Chem. 15, 979–985.
Krishna, H., Singh, S. K., Sharma, R. R., and Khawale, R. N. (2005). Biochemical changes in micropropagated grape (Vitis vinifera L) plantlets due to arbuscular-mycorrhizal fungi (AMF) inoculation during ex vitro acclimatization. Sci. Hortic. Amsterdam 106, 554–567. doi: 10.1016/j.scienta.2005.05.009
Kuzyakov, Y., and Blagodatskaya, E. (2015). Microbial hotspots and hot moments in soil: concept & review. Soil Biol. Biochem. 83, 184–199. doi: 10.1016/j.soilbio.2015.01.025
La Notte, A., D’Amato, D., Mäkinen, H., Paracchini, M. L., Liquete, C., Egoh, B., et al. (2017). Ecosystem services classification: a systems ecology perspective of the cascade framework. Ecol. Ind. 74, 392–402. doi: 10.1016/j.ecolind.2016.11.030
Lagomarsino, A., Grego, S., Marhan, S., Moscatelli, M. C., and Kandeler, E. (2008). Soil management modifies micro-scale abundance and function of soil microorganisms in a Mediterranean ecosystem. Eur. J. Soil Sci. 60, 2–12.
Landi, S., Papini, R., d’Errico, G., Brandi, G., Rocchini, A., Roversi, P. F., et al. (2018). Effect of different set-aside management systems on soil nematode community and soil fertility in North, Central and South Italy. Agr. Ecosys. Env. 261, 251–260.
Langellotto, G. A., and Denno, R. F. (2004). Responses of invertebrate natural enemies to complex-structured habitats: a meta-analytical synthesis. Oecologia 139, 1–10. doi: 10.1007/s00442-004-1497-3
Lavelle, P., Decaëns, T., Aubert, M., Barot, S., Blouin, M., Bureau, F., et al. (2006). Soil invertebrates and ecosystem services. Eur. J. Soil Biol. 42, S3–S15. doi: 10.1016/j.ejsobi.2006.10.002
Le Bissonnais, Y., and Andrieux, P. (2007). Impact des modes d’entretien de la vigne sur le ruissellement, l’érosion et la structure des sols. Mécanismes et résultats expérimentaux. Prog. Agric. Vitic. 124, 191–196.
Le Bissonnais, Y., Montier, C., Jamagne, M., Daroussin, J., and King, D. (2002). Mapping erosion risk for cultivated soil in France. Catena 46, 207–220. doi: 10.1016/S0341-8162(01).00167-9
Letourneau, D. K., Armbrecht, I., Rivera, B. S., Lerma, J. M., Carmona, E. J., and Daza, M. C. (2011). Does plant diversity benefit agroecosystems? A synthetic review. Ecol. Appl. 21, 9–21. doi: 10.1890/09-2026.1
Lewis, W. J., van Lenteren, J. C., Phatak, S. C., and Tumlinson, J. H. (1997). A total system approach to sustainable pest management. Proc. Natl. Acad. Sci. U.S.A. 94, 12243–12248. doi: 10.1073/pnas.94.23.12243
Li, H. Y., Yang, G. D., Shu, H. R., Yang, Y. T., Ye, B. X., Nishida, I., et al. (2006). Colonization by the arbuscular mycorrhizal fungus Glomus versiforme induces a defense response against the root-knot nematode Meloidogyne incognita in the grapevine (Vitis amurensis Rupr), which includes transcriptional activation of the class III chitinase gene VCH3. Plant Cell. Physiol. 47, 154–163.
Litskas, V., Mandoulaki, A., Vogiatzakis, I. N., Tzortzakis, N., and Stavrinides, M. (2020). Sustainable viticulture: first determination of the environmental footprint of grapes. Sustainability 12:8812. doi: 10.3390/su12218812
Llorens, J., Gil, E., Llop, J., and Escolà, A. (2010). Variable rate dosing in precision viticulture: use of electronic devices to improve application efficiency. Crop Prot. 29, 239–248. doi: 10.1016/j.cropro.2009.12.022
López-Piñeiro, A., Muñoz, A., Zamora, E., and Ramírez, M. (2013). Influence of the management regime and phenological state of the vines on the physicochemical properties and the seasonal fluctuations of the microorganisms in a vineyard soil under semi-arid conditions. Soil Till. Res. 126, 119–126. doi: 10.1016/j.still.2012.09.007
Louchart, X., Voltz, M., Andrieux, P., and Moussa, R. (2001). Herbicide transport to surface waters at field and watershed scales in a mediterranean vineyard area. J. Environ. Qual. 30, 982–991. doi: 10.2134/jeq2001.303982x
Mackie, K. A., Marhan, S., Ditterich, F., Schmidt, H. P., and Kandeler, E. (2015). The effects of biochar and compost amendments on copper immobilization and soil microorganisms in a temperate vineyard. Agr. Ecosyst. Environ. 201, 58–69. doi: 10.1016/j.agee.2014.12.001
Mäder, P., Fliessbach, A., Dubois, D., Gunst, L., Fried, P., and Nuggli, U. (2002). Soil fertility and biodiversity in organic farming. Science 296, 1694–1697. doi: 10.1126/science.1071148
Malossini, U., d’Errico, G., Varner, M., d’Errico, F. P., and Soppelsa, O. (2011). The vertical and horizontal distribution of Mesocriconema xenoplax (Raski, 1952) in Trentino vineyards (Northern Italy). Redia 94, 153–157.
Mandl, K., Cantelmo, C., Gruber, E., Faber, F., Friedrich, B., and Zaller, J. G. (2018). Effects of Glyphosate-, Glufosinate- and Flazasulfuron-based herbicides on soil microorganisms in a vineyard. Bull. Env. Contam. Toxic. 101, 562–569. doi: 10.1007/s00128-018-2438-x
Mania, E., Isocrono, D., Pedullà, M. L., and Guidoni, S. (2015). Plant diversity in an intensively cultivated vineyard agroecosystem (Langhe, North-West Italy). S. Afr. J. Enol. Vitic. 36378–36388. doi: 10.21548/36-3-970
Marques, M. J., García-Muñoz, S., Muñoz-Organero, G., and Bienes, R. (2010). Soil conservation beneath grass cover in hillside vineyards under mediterranean climatic conditions (Madrid. Spain). Land. Degrad. Dev. 21, 122–131. doi: 10.1002/ldr.915
Martínez-Casasnovas, J. A., and Ramos, M. C. (2009). Soil alteration due to erosion, ploughing and levelling of vineyards in north east Spain. Soil Use Manage. 25, 183–192. doi: 10.1111/j.1475-2743.2009.00215.x
Martins, G., Lauga, B., Miot-Sertier, C., Mercier, A., Lonvaud, A., Soulas, M. L., et al. (2013). Characterization of epiphytic bacterial communities from grapes, leaves, bark and soil of grapevine plants grown, and their relations. PLoS One 8:e73013. doi: 10.1371/journal.pone.0073013
Martinson, T. E. (1999). Biology and Control of the Striped Garden Snail Cepaea Nemoralis. New York, NY: Finger Lakes Grape Program.
Mazzoncini, M., Sapkota, T. B., Barberi, P., Antichi, D., and Risaliti, R. (2011). Long-term effect of tillage, nitrogen fertilization and cover crops on soil organic carbon and total nitrogen content. Soil Till. Res. 114, 165–174. doi: 10.1016/j.still.2011.05.001
McDaniel, M. D., Tiemann, L. K., and Grandy, A. S. (2014). Does agricultural crop diversity enhance soil microbial biomass and organic matter dynamics? A meta-analysis. Ecol. Appl. 24, 560–570. doi: 10.1890/13-0616.1
Mendes, R., Kruijt, M., de Bruijn, I., Dekkers, E., van der Voort, M., Schneider, J. H. M., et al. (2011). Deciphering the rhizosphere microbiome for disease-suppressive bacteria. Science 332, 1097–1100. doi: 10.1126/science.1203980
Merrington, G., Rogers, S. L., and van Zwieten, L. (2002). The potential impact of long term copper fungicide usage on soil microbial biomass and microbial activity in an avocado orchard. Aust. J. Soil Res. 40, 749–759. doi: 10.1071/SR01084
Messiga, A. J., Sharifi, M., Hammermeister, A., Gallant, K., Fuller, K., and Tango, M. (2015). Soil quality response to cover crops and amendments in a vineyard in Nova Scotia. Can. Sci. Hortic. 188, 6–14. doi: 10.1016/j.scienta.2015.02.041
Milanović, V., Comitini, F., and Ciani, M. (2013). Grape berry yeast communities: influence of fungicide treatments. Int. J. Food Microbiol. 161, 240–246. doi: 10.1016/j.ijfoodmicro.2012.12.019
Monteiro, A., and Lopes, C. M. (2007). Influence of cover crop on water use and performance of vineyard in Mediterranean Portugal. Agric. Ecosyst. Environ. 121, 336–342. doi: 10.1016/j.agee.2006.11.016
Monteiro, A., Caetano, F., Vasconcelos, T., and Lopes, C. M. (2012). Vineyard weed community dynamics in the Dão winegrowing region. Cienc. Tec. Vitivinic. 27, 73–82.
Morlat, R., and Jacquet, A. (2003). Grapevine root system and soil characteristics in a vineyard maintained long-term with or without interrow sward. Am. J. Enol. Vitic. 54, 1–7.
Nachtergaele, J., Poesen, J., and Van Wesemael, B. (1998). Gravel mulching in vineyards of southern Switzerland. Soil Till. Res. 46, 51–59.
Nannelli, R., and Simoni, S. (2002). “Influence of different types of grass mulching on the communities of Oribatid mites in the vineyards,” in Acarid Phylogeny and Evolution: Adaptation in Mites and Ticks, eds F. Bernini, R. Nannelli, G. Nuzzaci, and E. De Lillo (Dordrecht: Springer), 363–371.
Nannipieri, P., Ascher, J., Ceccherini, M., Landi, L., Pietramellara, G., and Renella, G. (2003). Microbial diversity and soil functions.Eur. J. Soil Sci. 54, 655–670. doi: 10.1046/j.1351-0754.2003.0556.x
Nascimbene, J., Marini, L., and Paoletti, M. G. (2012). Organic farming benefits local plant diversity in vineyard farms located in intensive agricultural landscapes. Environ. Manage. 49, 1054–1060. doi: 10.1007/s00267-012-9834-5
Nascimbene, J., Marini, L., Ivan, D., and Zottini, M. (2013). Management intensity and topography determined plant diversity in vineyards. PLoS One 8:e76167. doi: 10.1371/journal.pone.0076167
Naseby, D. C., and Lynch, J. M. (2002). “Enzymes and microorganisms in the rhizosphere,” in Enzymes in the Environment. Activity, Ecology and Applications, eds R. G. Burns and R. P. Dick (New York, NY: Marcel Dekker), 109–123.
Nekola, J. C. (2010). Acidophilic terrestrial gastropod communities of North America. J. Mollus. Stud. 76, 144–156. doi: 10.1093/mollus/eyp053
Nicholls, C. I., Parella, M. P., and Altieri, M. A. (2000). Reducing the abundance of leafhoppers and thrips in a northern California organic vineyard through maintenance of full season floral diversity with summer cover crops. Agr. For. Entomol. 2, 107–113. doi: 10.1046/j.1461-9563.2000.00054.x
Nicholls, C. I., Parrella, M., and Altieri, M. A. (2001). The effects of a vegetational corridor on the abundance and dispersal of insect biodiversity within a northern California organic vineyard. Landscape Ecol. 16, 133–146. doi: 10.1023/A:1011128222867
Nielsen, U. N., Wall, D. H., and Six, J. (2015). Soil biodiversity and the Environment. Annu. Rev. Environ. Resour. 40, 63–90. doi: 10.1146/annurev-environ-102014-021257
Okur, N., Altindsli, A., Cengel, M., Gocmez, S., and Kayikcioglu, H. H. (2009). Microbial biomass and enzyme activity in vineyard soils under organic and conventional farming systems. Turk. J. Agric. For. 33, 413–423. doi: 10.3906/tar-0806-23
Organisation Internationale de la Vigne et du Vin [Oiv]. (2021). State of the Vitiviniculture world market. Available online at: https://www.oiv.int/public/medias/7909/oiv-state-of-the-world-vitivinicultural-sector-in-2020 (Accessed on Nov 18 2021)
Orgiazzi, A., Bardgett, R. D., Barrios, E., Behan-Pelletier, V., Briones, M. J. I., Chotte, J.-L., et al. (2016). Global Soil Biodiversity Atlas. Luxembourg, LUX: Publication Office of the European Union.
Ostandie, N., Giffard, B., Bonnard, O., Joubard, B., Richart-Cervera, S., Thiéry, D., et al. (2021). Multi-community effects of organic and conventional farming practices in vineyards. Sci. Rep. 11:11979. doi: 10.1038/s41598-021-91095-5
Paiola, A., Assandri, G., Brambilla, M., Zottini, M., Pedrini, P., and Nascimbene, J. (2020). Exploring the potential of vineyards for biodiversity conservation and delivery of biodiversity-mediated ecosystem services: a global-scale systematic review. Sci. Tot. Environ. 706:135839. doi: 10.1016/j.scitotenv.2019.135839
Paoletti, M. G., Sommaggio, D., Favretto, M. R., Petruzzelli, G., Pezzarossa, B., and Barbafieri, M. (1998). Earthworms as useful bioindicators of agroecosystem sustainability in orchards and vineyards with different inputs. Appl. Soil Ecol. 10, 137–150. doi: 10.1016/S0929-1393(98)00036-5
Parisi, V., Menta, C., Gardi, C., Jacomini, C., and Mozzanica, E. (2005). Microarthropod communities as a tool to assess soil quality and biodiversity: a new approach in Italy. Agr. Ecosyst. Environ. 105, 323–333. doi: 10.1016/j.agee.2004.02.002
Paungfoo-Lonhienne, C., Yeoh, Y. K., Kasinadhuni, N. R., Lonhienne, T. G., Robinson, N., Hugenholtz, P., et al. (2015). Nitrogen fertilizer dose alters fungal communities in sugarcane soil and rhizosphere. Sci. Rep. 5:8678. doi: 10.1038/srep08678
Pelosi, C., Barot, S., Capowiez, Y., Hedde, M., and Vandenbulcke, F. (2014). Pesticides and earthworms. A review. Agron. Sustain. Dev. 34, 199–228. doi: 10.1007/s13593-013-0151-z
Peregrina, F., Pérez-Álvarez, E. P., Colina, M., and García-Escudero, E. (2012). Cover crop and tillage influence soil organic matter and nitrogen availability in a semi-arid vineyard. Arch. Agron. Soil Sci. 58, S95–S102. doi: 10.1080/03650340.2011.648182
Pérès, G., Cluzeau, D., Curmi, P., and Hallaire, V. (1998). Earthworm activity and soil structure changes due to organic enrichments in vineyard systems. Biol. Fert. Soils 27, 417–424. doi: 10.1007/s003740050452
Pérès, G., Vandenbulcke, F., Guernion, M., Hedde, M., Beguiristain, T., Douay, F., et al. (2011). Earthworm indicators as tools for soil monitoring, characterization and risk assessment. An example from the national Bioindicator programme (France). Pedobiologia 54, S77–S87. doi: 10.1016/jpedobi201109015
Pérez-Álvarez, E. P., Garde-Cerdán, T., Santamaría, P., García-Escudero, E., and Peregrina, F. (2015). Influence of two different cover crops on soil N availability, N nutritional status, and grape yeast assimilable N (YAN) in a cv. Tempranillo vineyard. Plant Soil 390, 143–156. doi: 10.1007/s11104-015-2387-7
Pétremand, G., Speight, M. C. D., Fleury, D., Castella, E., and Delabays, N. (2017). Hoverfly diversity supported by vineyards and the importance of ground cover management. Bull. Insectol. 70, 147–155.
Peverieri, G. S., Simoni, S., Goggioli, D., Liguori, M., and Castagnoli, M. (2009). Effects of variety and management practices on mite species diversity in Italian vineyards. Bull. Insectol. 62, 53–60.
Pfingstmann, A., Paredes, D., Buchholz, J., Querner, P., Bauer, T., Strauss, P., et al. (2019). Contrasting effects of tillage and landscape structure on spiders and springtails in vineyards. Sustainability 11:2095. doi: 10.3390/su11072095
Philippot, L., Raaijmakers, J. M., Lemanceau, P., and van der Putten, W. H. (2013). Going back to the roots: the microbial ecology of the rhizosphere. Nat. Rev. Microbiol. 11, 789–799. doi: 10.1038/nrmicro3109
Pinamonti, F. (1998). Compost mulch effects on soil fertility, nutritional status and performance of grapevine. Nutr. Cycl. Agroecosys. 51, 239–248. doi: 10.1023/A:1009701323580
Pinguel, M., Reineke, A., and Leyer, I. (2018). A 30-years vineyard trial: plant communities, soil microbial communities and litter decomposition respond more to soil treatment than to N fertilization. Agr. Ecosyst. Env. 272:114–125.
Power, A. G. (2010). Ecosystem services and agriculture: tradeoffs and synergies. Phil. Trans. R. Soc. B 365, 2959–2971. doi: 10.1098/rstb.2010.0143
Probst, B., Schüler, C., and Joergensen, R. G. (2008). Vineyard soils under organic and conventional management—microbial biomass and activity indices and their relation to soil chemical properties. Biol. Fert. Soils 44, 443–450. doi: 10.1007/s00374-007-0225-7
Pulleman, M., Creamer, R., Hamer, U., Helder, J., Pélosi, C., Pérès, G., et al. (2012). Soil biodiversity, biological indicators and soil ecosystem services—an overview of European approaches. Curr. Opin. Env. Sust. 4, 529–538. doi: 10.1016/j.cosust.2012.10.009
Quiquerez, A., Brenot, J., Garcia, J.-P., and Petit, C. (2008). Soil degradation caused by a high-intensity rainfall event: implications for medium-term soil sustainability in Burgundian vineyards. Catena 73, 89–97. doi: 10.1016/j.catena.2007.09.007
Radic, T., Hancevic, K., Likar, M., Protega, I., and Jug-Dujakoviæ, M. (2012). Neighbouring weeds influence the formation of arbuscular mycorrhiza in grapevine. Symbiosis 56, 111–120. doi: 10.1007/s13199-012-0165-3
Rahman, L., Whitelaw-Weckert, M. A., and Orchard, B. (2014). Impact of organic soil amendments, including poultry-litter biochar, on nematodes in a Riverina. New South Wales, vineyard. Soil Res. 52, 604–619. doi: 10.1071/SR14041
Rashid, M. I., Mujawar, L. H., Shahzad, T., Almeelbi, T., Ismail, I. M., and Oves, M. (2016). Bacteria and fungi can contribute to nutrients bioavailability and aggregate formation in degraded soils. Microbiol. Res. 183, 26–41. doi: 10.1016/j.micres.2015.11.007
Reinecke, A. J., Helling, B., Louw, K., Fourie, J., and Reinecke, S. A. (2002). The impact of different herbicides and cover crops on soil biological activity in vineyards in the Western Cape. South Africa. Pedobiologia 46, 475–484. doi: 10.1078/0031-4056-00153
Renaud, A., Poinsot-Balaguer, N., Cortet, J., and Le Petit, J. (2004). Influence of four soil maintenance practices on Collembola communities in a Mediterranean vineyard. Pedobiologia 48, 623–630. doi: 10.1016/j.pedobi.2004.07.002
Rich, M. K., Nouri, E., Courty, P. E., and Reinhardt, D. (2017). Diet of Arbuscular Mycorrhizal Fungi: bread and Butter? Trends Plant. Sci. 22, 652–660. doi: 10.1016/j.tplants.2017.05.008
Riches, D., Porter, I. J., Oliver, D. P., Bramley, R. G. V., Rawnsley, B., Edwards, J., et al. (2013). A review of soil biological properties as indicators for soil quality in Australian viticulture. Aust. J. Grape Wine Res. 19, 311–323. doi: 10.1111/ajgw.12034
Rodrigo Comino, J., Quiquerez, A., Follain, S., Raclot, D., Le Bissonais, Y., and Casali, J. (2016). Soil erosion in sloping vineyards assessed by using botanical indicators and sediment collectors in the Ruwer-Mosel valley. Agr. Ecosyst. Env. 233, 158–170. doi: 10.1016/j.agee.2016.09.009
Rüdisser, J., Tasser, E., Peham, T., Meyer, E., and Tappeiner, U. (2015). The dark side of biodiversity: spatial application of the biological soil quality indicator (BSQ). Ecol. Indic. 53, 240–246. doi: 10.1016/j.ecolind.2015.02.006
Ruiz-Colmenero, M., Bienesa, R., and Marques, M. J. (2011). Soil and water conservation dilemmas associated with the use of green cover in steep vineyards. Soil Till. Res. 117, 211–223. doi: 10.1016/j.still.2011.10.004
Rusch, A., Chaplin-Kramer, R., Gardiner, M. M., Hawro, V., Holland, J., and Landis, D. (2016a). Agricultural landscape simplification reduces natural pest control: a quantitative synthesis. Agr. Ecosyst. Environ. 221, 198–204. doi: 10.1016/j.agee.2016.01.039
Rusch, A., Binet, D., Delbac, L., and Thiéry, D. (2016b). Local and landscape effects of agricultural intensification on Carabid community structure and weed seed predation in a perennial cropping system. Landscape Ecol. 31, 2163–2174. doi: 10.1007/s10980-016-0390-x
Rusch, A., Delbac, L., and Thiéry, D. (2017). Grape moth density in Bordeaux vineyards depends on local habitat management despite effects of landscape heterogeneity on their biological control. J. Appl. Ecol. 54, 1794–1803. doi: 10.1111/1365-2664.12858
Rusch, A., Valantin-Morison, M., Sarthou, J. P., and Roger-Estrade, J. (2010). Biological control of insect pests in agroecosystems: effects of crop management, farming systems and semi-natural habitats at the landscape scale. A review. Adv. Agron. 109, 219–259. doi: 10.1016/S0065-2113(10).09006-1
Salome, C., Coll, P., Lardo, E., Metay, A., Villenave, C., Marsden, C., et al. (2016). The soil quality concept as a framework to assess management practices in vulnerable agroecosystems: a case study in Mediterranean vineyards. Ecol. Indic. 61, 456–465. doi: 10.1016/j.ecolind.2015.09.047
Salome, C., Coll, P., Lardo, E., Villenave, C., Blanchart, E., Hinsinger, P., et al. (2014). Relevance of use-invariant soil properties to assess soil quality of vulnerable ecosystems: the case of Mediterranean vineyards. Ecol. Indic. 43, 83–93. doi: 10.1016/j.ecolind.2014.02.016
Sanderson, G., and Sirgel, W. (2002). “Helicidae as pests in Australian and South African grapevines,” in Molluscs as Crop Pests, ed. G. M. Barker (Wallingford: CABI Publishing), 255–270.
Sanguankeo, P. P., and León, R. G. (2011). Weed management practices determine plant and arthropod diversity and seed predation in vineyards. Weed Res. 51, 404–412. doi: 10.1111/j.1365-3180.2011.00853.x
Santiago-Brown, I., Metcalfe, A., Jerram, C., and Collins, C. (2014). Transnational comparison of sustainability assessment programs for viticulture and a case-study on programs’ engagement processes. Sustainability 6, 2031–2066. doi: 10.3390/su6042031
Schaefer, M. (1990). The soil fauna of a beech forest on limestone: trophic structure and energy budget. Oecologia 82, 128–136. doi: 10.1007/BF00318544
Schreck, E., Geret, F., Gontier, L., and Treilhou, M. (2008). Neurotoxic effect and metabolic responses induced by a mixture of six pesticides on the earthworm Aporrectodea caliginosa nocturna. Chemosphere 71, 1832–1839. doi: 10.1016/jchemosphere.2008.02.003
Schreck, E., Gontier, L., Dumat, C., and Geret, F. (2012). Ecological and physiological effects of soil management practices on earthworm communities in french vineyards. Eur. J. Soil Biol. 52, 8–15. doi: 10.1016/j.ejsobi.2012.05.002
Seniczak, A., Seniczak, S., García-Parra, I., Ferragut, F., Xamaní, P., and Graczyk, R. (2018). Oribatid mites of conventional and organic vineyards in the Valencian Community. Spain. Acarologia 58, 119–133.
Simon, S., Bouvier, J. C., Debras, J. F., and Sauphanor, B. (2010). Biodiversity and pest management in orchard systems. A review. Agron. Sustain. Dev. 30, 139–152. doi: 10.1051/agro/2009013
Sinsabaugh, R. L. (2010). Phenol oxidase, peroxidase and organic matter dynamics of soil. Soil Biol. Biochem. 42, 391–404. doi: 10.1016/j.soilbio.2009.10.014
Smith, R. G., Atwood, L. W., Pollnac, F. W., and Warren, N. D. (2015). Cover-crop species as distinct biotic filters in weed community assembly. Weed Sci. 63, 282–295. doi: 10.1614/WS-D-14-00071.1
Steenwerth, K. L., McElrone, A. J., Calderón-Orellana, A., Hanifin, R. C., Storm, C., Collatz, W., et al. (2013). Cover crops and tillage in a mature Merlot vineyard show few effects on grapevines. Am. J. Enol. Vitic. 64, 515–521. doi: 10.5344/ajev.2013.12119
Steenwerth, K., and Belina, K. M. (2008). Cover crops enhance soil organic matter, carbon dynamics and microbiological function in a vineyard agroecosystem. Appl. Soil Ecol. 40, 359–369. doi: 10.1016/j.apsoil.2008.06.006
Steenwerth, K., Orellana-Calderón, A., Hanifin, R. C., Storm, C., and McElrone, A. J. (2016). Effects of various vineyard floor management techniques on weed community shifts and grapevine water relations. Am. J. Enol. Vitic. 67, 153–162. doi: 10.5344/ajev.2015.15050
Sturm, J. M., Sturm, M., and Eisenbeis, G. (2002). Recovery of the biological activity in a vineyard soil after landscape redesign: a three-year study using the bait-lamina method. Vitis 41, 43–45.
Stursova, M., and Sinsabaugh, R. L. (2008). Stabilization of oxidative enzymes in desert soil may limit organic matter accumulation. Soil Biol. Biochem. 40, 550–553. doi: 10.1016/j.soilbio.2007.09.002
Symondson, W. O. C., Sunderland, K. D., and Greenstone, M. H. (2002). Can generalist predators be effective biocontrol agents? Annu. Rev. Entomol. 47, 561–594. doi: 10.1146/annurev.ento.47.091201.145240
Tempesta, T., Giancristofaro, R. A., Corain, L., Salmaso, L., Tomasi, D., and Boatto, V. (2010). The importance of landscape in wine quality perception: an integrated approach using choice-based conjoint analysis and combination-based permutation tests. Food Qual. Prefer. 21, 827–836. doi: 10.1016/j.foodqual.2010.04.007
Tesic, D., Keller, M., and Hutton, R. J. (2007). Influence of vineyard floor management practices on grapevine vegetative growth, yield, and fruit composition. Am. J. Enol. Vitic. 58, 1–11.
Theuerl, S., and Buscot, F. (2010). Laccases: toward disentangling their diversity and functions in relation to soil organic matter cycling. Biol. Fertil. Soils 46, 215–225. doi: 10.1007/s00374-010-0440-5
Thomson, L. (2006). Influence of reduced irrigation on beneficial invertebrates in vineyards. Aust. J. Exp. Agr. 46, 1389–1395. doi: 10.1071/EA05139
Thomson, L., and Hoffmann, A. (2009). Vegetation increases the abundance of natural enemies in vineyards. Biol. Contr. 49, 259–269. doi: 10.1016/j.biocontrol.2009.01.009
Tompkins, J. (2010). Ecosystem services provided by native New Zealand plants in vineyards. PhD thesis.
Treseder, K. K. (2008). Nitrogen additions and microbial biomass: a meta-analysis of ecosystem studies. Ecol. Lett. 11, 1111–1120. doi: 10.1111/j.1461-0248.2008.01230.x
Trivellone, V., Paltrinieri, L. P., Jermini, M., and Moretti, M. (2012). Management pressure drives leafhopper communities in vineyards in Southern Switzerland. Insect Conserv. Divers. 5, 75–85. doi: 10.1111/j.1752-4598.2011.00151.x
Trivellone, V., Schoenenberger, N., Bellosi, B., Jermini, M., de Bello, F., Mitchell, E. A. D., et al. (2014). Indicators for taxonomic and functional aspects of biodiversity in the vineyard agroecosystem of southern Switzerland. Biol. Conserv. 170, 103–109. doi: 10.1016/j.biocon.2013.12.008
Trouvelot, S., Bonneau, L., Redecker, D., van Tuinen, D., Adrian, M., and Wipf, D. (2015). Arbuscular mycorrhiza symbiosis in viticulture: a review. Agron. Sustain. Dev. 35, 1449–1467. doi: 10.1007/s13593-015-0329-7
Tsiafouli, M. A., Thébault, E., Sgardelis, S. P., de Ruiter, P. C., van der Putten, W. H., and Birkhofer, K. (2014). Intensive agriculture reduces soil biodiversity across Europe. Glob. Change Biol. 21, 973–985. doi: 10.1111/gcb.12752
Tuck, S. L., Winqvist, C., Mota, F., Ahnström, J., Turnbull, L. A., and Bengtsson, J. (2014). Land-use intensity and the effects of organic farming on biodiversity: a hierarchical meta-analysis. J. Appl. Ecol. 51, 746–755. doi: 10.1111/1365-2664.12219
Uzman, D., Entling, M. H., Leyer, I., and Reineke, A. (2020). Mutual and Opposing Responses of Carabid Beetles and Predatory Wasps to Local and Landscape Factors in Vineyards. Insects 11:746. doi: 10.3390/insects11110746
van Leeuwen, C., and Seguin, G. (2006). The concept of terroir in viticulture. J. Wine Res. 17, 1–10. doi: 10.1080/09571260600633135
van Leeuwen, C., Friant, P., Choné, X., Tregoat, O., Koundouras, S., and Dubourdieu, D. (2004). Influence of climate, soil, and cultivar on terroir. Am. J. Enol. Vitic. 55, 207–217.
Vaudour, E., Costantini, E. A. C., Jones, G. V., and Mocali, S. (2015). An overview of the recent approaches to terroir functional modelling, footprinting and zoning. Soil 1, 287–312. doi: 10.5194/soil-1-287-2015
Vega-Avila, A. D., Gumiere, T., Andrade, P. A. M., Lima-Perim, J. E., Durrer, A., Baigori, M., et al. (2015). Bacterial communities in the rhizosphere of Vitis vinifera L. cultivated under distinct agricultural practices in Argentina. A. Van Leeuw. J. Microb. 107, 575–588. doi: 10.1007/s10482-014-0353-7
Vepsäläinen, M., Kukkonen, S., Vestberg, M., Sirviö, H., and Niemi, R. M. (2001). Application of soil enzyme activity test kit in a field experiment. Soil Biol. Biochem. 33, 1665–1672.
Verbruggen, E., Kiers, E. T., Bakelaar, P. N. C., Röling, W. F. M., and van der Heijden, M. G. A. (2012). Provision of contrasting ecosystem services by soil communities from different agricultural fields. Plant Soil 350, 43–55. doi: 10.1007/s11104-011-0828-5
Viers, J. H., Williams, J. N., Nicholas, K. A., Barbosa, O., Kotze, I., Spence, L., et al. (2013). Vinecology: pairing wine with nature. Conserv. Lett. 6, 287–299. doi: 10.1111/conl.12011
Villate, L., Fievet, V., Hanse, B., Delemarre, F., Plantard, O., Esmenjaud, D., et al. (2008). Spatial distribution of the dagger nematode Xiphinema index and its associated Grapevine fanleaf virus in French vineyard. Phytopathology 98, 942–948. doi: 10.1094/PHYTO-98-8-0942
Wagg, C., Bender, S. F., Widmer, F., and van der Heijden, M. G. A. (2014). Soil biodiversity and soil community composition determine ecosystem multifunctionality. Proc. Natl. Acad. Sci. U.S.A. 111, 5266–5270. doi: 10.1073/pnas.1320054111
Wall, D. H., Nielsen, U. N., and Six, J. (2015). Soil biodiversity and human health. Nature 528, 69–76. doi: 10.1038/nature15744
Walton, V. M., Daane, K. M., and Addison, P. (2012). “Biological control of arthropods and its application in vineyards,” in Arthropod Management in Vineyards, eds N. Bostanian, C. Vincent, and R. Isaacs (Dordrecht: Springer), 91–117.
Wardle, D. A. (1995). Impacts of disturbance on detritus food webs in agro-ecosystems of contrasting tillage and weed management practices. Adv. Ecol. Res. 26, 105–185. doi: 10.1016/S0065-2504(08).60065-3
Wendt, C. A., and Johnson, M. D. (2017). Multi-scale analysis of barn owl nest box selection on Napa Valley vineyards. Agr. Ecosys. Environ. 247, 75–83. doi: 10.1016/j.agee.2017.06.023
Wheeler, S. J., Black, A. S., and Pickering, G. J. (2005). Vineyard floor management improves wine quality in highly vigorous Vitis vinifera ‘Cabernet Sauvignon’ in New Zealand. J. Crop. Hort. 33, 317–328. doi: 10.1080/01140671.2005.9514365
Whitelaw-Weckert, M. A., Rahman, L., Hutton, R. J., and Coombes, N. (2007). Permanent swards increase soil microbial counts in two Australian vineyards. Appl. Soil Ecol. 36, 224–232. doi: 10.1016/j.apsoil.2007.03.003
Williamson, J. R., and Johnson, D. T. (2005). Effects of grape berry moth management practices and landscape on arthropod diversity in grape vineyards in the southern United States. Hort. Tech. 15, 232–238.
Winfree, R., Fox, J. W., Williams, N. M., Reilly, J. R., and Cariveau, D. P. (2015). Abundance of common species, not species richness, drives delivery of a real-world ecosystem service. Ecol. Lett. 18, 626–635. doi: 10.1111/ele.12424
Winkler, K. J., Viers, J. H., and Nicholas, K. A. (2017). Assessing Ecosystem Services and Multifunctionality for Vineyard Systems. Front. Environ. Sci. 5:15. doi: 10.3389/fenvs.2017.00015
Winter, S., Bauer, T., Strauss, P., Kratschmer, S., Paredes, D., Landa, B., et al. (2018). Effects of vegetation management intensity on biodiversity and ecosystem services in vineyards: a meta-analysis. J. Appl. Ecol. 55, 2484–2495. doi: 10.1111/1365-2664.13124
Wolters, V., and Ekschmitt, K. (1997). “Gastropods, Isopods, Diplopods, and Chilopods: Neglected groups of the decomposer food webs,” in Fauna in Soil Ecosystems: Recycling Processes, Nutrient Fluxes, and Agricultural Production, ed. G. Benckiser (Basel: Marcel Dekker), 265–306.
Wu, T., Chellemi, D. O., Graham, J. H., Martin, K. J., and Rosskopf, E. N. (2008). Comparison of soil bacterial communities under diverse agricultural land management and crop production practices. Microb. Ecol. 55, 293–310. doi: 10.1007/s00248-007-9276-4
Wurst, S., Sonnemann, I., and Zaller, J. G. (2018). “Soil Macro-Invertebrates: Their Impact on Plants and Associated Aboveground Communities in Temperate Regions,” in Aboveground–Belowground Community Ecology. Ecological Studies (Analysis and Synthesis), eds T. Ohgushi, S. Wurst, and S. Johnson (Cham: Springer), 234. doi: 10.1007/978-3-319-91614-9_8
Yeates, G. W., Wardle, D. A., and Watson, R. N. (1999). Response of soil nematode populations, community structure, diversity and temporal variability to agricultural intensification over a 7-year period. Soil Biol. Biochem. 31, 1721–1733. doi: 10.1016/S0038-0717(99).00091-7
Zaller, J. G., Cantelmo, C., Dos Santos, G., Muther, S., Gruber, E., and Pallua, P. (2018). Herbicides in vineyards reduce grapevine root mycorrhization and alter soil microorganisms and the nutrient composition in grapevine roots, leaves, xylem sap and grape juice. Environ. Science Pollut. Res. 25, 23215–23226. doi: 10.1007/s11356-018-2422-3
Zaller, J. G., Heigl, F., Ruess, L., and Grabmaier, A. (2014). Glyphosate herbicide affects belowground interactions between earthworms and symbiotic mycorrhizal fungi in a model ecosystem. Sci. Rep. 4:5634. doi: 10.1038/srep05634
Zarraonaindia, I., Owens, S. M., Weisenhorn, P., West, K., Hampton-Marcell, J., Lax, S., et al. (2015). The soil microbiome influences grapevine-associated microbiota. mBio 6:e02527–e02514. doi: 10.1128/mBio.02527-14
Keywords: microarthropods, earthworms, gastropods, nematodes, plants, predatory arthropods, microflora, pest control
Citation: Giffard B, Winter S, Guidoni S, Nicolai A, Castaldini M, Cluzeau D, Coll P, Cortet J, Le Cadre E, d’Errico G, Forneck A, Gagnarli E, Griesser M, Guernion M, Lagomarsino A, Landi S, Bissonnais YL, Mania E, Mocali S, Preda C, Priori S, Reineke A, Rusch A, Schroers H-J, Simoni S, Steiner M, Temneanu E, Bacher S, Costantini EAC, Zaller J and Leyer I (2022) Vineyard Management and Its Impacts on Soil Biodiversity, Functions, and Ecosystem Services. Front. Ecol. Evol. 10:850272. doi: 10.3389/fevo.2022.850272
Received: 07 January 2022; Accepted: 23 June 2022;
Published: 22 July 2022.
Edited by:
Christopher Williams, Liverpool John Moores University, United KingdomReviewed by:
Menelaos Stavrinides, Cyprus University of Technology, CyprusAndrea Sciarretta, University of Molise, Italy
Copyright © 2022 Giffard, Winter, Guidoni, Nicolai, Castaldini, Cluzeau, Coll, Cortet, Le Cadre, d’Errico, Forneck, Gagnarli, Griesser, Guernion, Lagomarsino, Landi, Bissonnais, Mania, Mocali, Preda, Priori, Reineke, Rusch, Schroers, Simoni, Steiner, Temneanu, Bacher, Costantini, Zaller and Leyer. This is an open-access article distributed under the terms of the Creative Commons Attribution License (CC BY). The use, distribution or reproduction in other forums is permitted, provided the original author(s) and the copyright owner(s) are credited and that the original publication in this journal is cited, in accordance with accepted academic practice. No use, distribution or reproduction is permitted which does not comply with these terms.
*Correspondence: Brice Giffard, YnJpY2UuZ2lmZmFyZEBnbWFpbC5jb20=