- 1State Key Laboratory of Water Environment Simulation, School of Environment, Beijing Normal University, Beijing, China
- 2College of Water Sciences, Beijing Normal University, Beijing, China
- 3Guangdong Provincial Key Laboratory of Water Quality Improvement and Ecological Restoration for Watersheds, School of Ecology, Environment and Resources, Guangdong University of Technology, Guangzhou, China
Macrobenthos taxonomic and functional diversity are key indicators of ecosystem health. River–lake ecotones are key macrobenthos habitats. However, we don’t fully understand macrobenthos biodiversity patterns in these ecotones. We studied water environment, sediment heavy metal contents, and macrobenthos community, which we sampled simultaneously from 29 sampling sites along the Fu River–Baiyangdian Lake gradient in Northern China with five field surveys from 2018 to 2019. Six trait classes resolved into 25 categories were allocated to macrobenthos through a binary coding system. We used the RLQ framework (R, environmental variables; L, species of taxa; Q, traits) and fourth-corner analyses to evaluate the relationship between environmental variables and macrobenthos traits. Finally, we carried out variance partitioning to assess the contributions of environmental variables to variation of macrobenthos diversities. As the results, TN and TP contents in the river and lake mouths were lower than those in the adjacent river and lake, indicating that the river–lake ecotones played a role in purifying the water and buffering pollution. High taxonomic diversity of macrobenthos in the lake mouth and the presence of unique taxa in the two ecotones revealed edge effects, but the macrobenthos abundance and biomass were extremely low compared with those in the adjacent river and lake. We found no significant correlation between the taxonomic and functional diversity indices in the river and lake mouths. Water depth, water transparency, TN, and TP were the main water environmental drivers of macrobenthos taxonomic and functional diversity, explaining up to 45.5% and 56.2% of the variation, respectively. Sediment Cd, Cr, Cu, Pb, and Zn contents explained 15.1% and 32.8%, respectively, of macrobenthos taxonomic and functional diversity. Our results suggest that functional diversity approaches based on biological traits can complement taxonomic approaches in river–lake ecotones. Furthermore, improving water depth, transparency, eutrophication, and heavy metal pollution will improve macrobenthos diversity in these ecotones and maintain ecosystem health.
Introduction
River–lake systems (i.e., rivers flowing through or into the lakes) can be regarded as continuous aquatic ecosystem, and are affected by the hydraulic exchanges and hydrological connectivity (Hillbricht-Ilkowska, 1999). As a result, they provide multiple ecological functions, such as material cycling, energy flows, and biotic exchanges (Zhang et al., 2020). Ecotones are zones of transition between rivers and lakes, and are key parts of a river–lake system (Holland, 1988; Gosz, 1993; Kolasa and Zalewski, 1995). They can exhibit edge effects (Odum, 1971; Murcia, 1995), with elevated species abundance and biodiversity in some parts of the ecotone (Winterbourn, 1971; Willis and Magnuson, 2000) and the presence of taxa specific to different parts of the ecotones (Poznańska et al., 2010). In addition, ecotones are sensitive to environmental variations (Risser, 1995; Allen and Breshears, 1998), which play important role in purifying water and buffering pollution (Risser, 1995; Ward and Tockner, 2001).
The macrobenthos community show a range of life history characteristics (e.g., relatively long lifespans, sedentary existence), and play a central role in energy flows and material circulation in aquatic ecosystems (Li et al., 2019a; Mosbahi et al., 2019). In general, the macrobenthos have been regarded as key biological indicators of freshwater ecosystem health because these organisms are in direct contact with the sediment and are highly sensitive to changes in physiochemistry of the environment (Soldner et al., 2004; O’Brien et al., 2016; Piló et al., 2016; Eriksen et al., 2021). Most recent studies that documented the macrobenthos biodiversity have focused solely on rivers (Azrina et al., 2006; Gonzalo and Camargo, 2013; Li Z. F. et al., 2020; Buffagni, 2021) or on lakes (Van De Meutter et al., 2006; Li et al., 2016; Cai et al., 2017; Zhang et al., 2019). Only few studies have accounted for both the river and lake components of these systems (Obolewski et al., 2014; Jakubik et al., 2015). However, to date, limited work has been conducted on the macrobenthos community structure and diversity in river–lake ecotones (Szkokan-Emilson et al., 2011; Patrick, 2014). These results have suggested that the environmental characteristics and macroinvertebrate assemblages of ecotones differ from those in adjacent lakes and tributaries (Krebs et al., 2018; Salvo et al., 2020).
Previous studies on macrobenthos biodiversity typically focused on species richness and taxonomic diversity (Li et al., 2016; Wu et al., 2019; Lento et al., 2020), but such a focus conceals ecological differences among species and ignores specific roles they play in the ecosystem (Heino et al., 2007). As a result, they cannot effectively guide biodiversity conservation (Cadotte et al., 2011; Gagic et al., 2015). Each species of macrobenthos is a collection of individuals with multiple functional traits (including phenotypic and behavioral traits) that determine their performance and fitness (Steneck and Dethier, 1994; Violle et al., 2007). More generally, these characteristics determine the compatibility of species with the characteristics of their environment (Martello et al., 2018; Brumm et al., 2021). Recent studies have considered the role of species functional diversity based on biological traits (e.g., body morphology, physiology, trophic habits, and life-history strategies) in a community (Schmera et al., 2017; Espinoza-Toledo et al., 2021; Paz et al., 2022), which may provide a more mechanistic perspective on the community–environment relationships than is possible with approaches based purely on taxonomic diversity (Violle et al., 2007; Cadotte et al., 2011). More importantly, some studies have shown that macrobenthos taxonomic and functional diversity indices respond differently to the same environmental gradients (Heino and Tolonen, 2017; Li et al., 2019b,c; Baker et al., 2021). This suggests that functional diversity metrics complement the information provided by classical taxonomic diversity metrics (England and Wilkes, 2018; Zhang et al., 2021).
Baiyangdian Lake is the largest shallow lake in Northern China. Most previous studies of the lake’s macrobenthos communities focused on the lake, with few studies in the tributaries. These studies showed that the macrobenthos richness and biodiversity in Baiyangdian Lake and its rivers have decreased significantly since the 1960s (Zhang et al., 2018; Yang et al., 2020). In the present study, we selected China’s Baiyangdian Lake–Fu River system as a case study, with the goals being to (1) compare the spatial distributions of the macrobenthos community structure among the river, river-lake ecotones, and lake; (2) explore the spatial patterns and differences of multiple aspects of macrobenthos biodiversity (including taxonomic and functional diversity) in different ecosystem types (the river, river and lake mouths, and lake); and (3) determine which functional traits respond to specific environmental factors and quantify the effects of the water environment and sediment heavy metal contents on the macrobenthos taxonomic and functional diversity in the river–lake system. We hypothesized that macrobenthos community characteristics would differ significantly along gradients in the above mentioned environmental factors.
Materials and methods
Study area
Baiyangdian Lake (38°43′N to 39°02′N, 115°45′E to 116°07′E) is the largest shallow lake in the North China Plain (Figure 1). The region has a typical warm-temperate monsoon climate, with monthly mean water temperatures of 2–29°C; the average annual precipitation and pan evaporation are approximately 550 and 1,637 mm, respectively. The lake plays an important role in providing habitats for native plants and animals, in water purification, as well as in protecting against floods. Historically, nine upstream rivers flowed into Baiyangdian Lake. Prior to 1960, the lake was dominated by natural flows. However, upstream dams and reservoirs have greatly reduced inflows to the lake, and left insufficient water to sustain the lake’s environmental flows. In recent years, the inflows of Baiyangdian Lake have come from three main rivers: Fu River, Xiaoyi River, and Baigouyin River. The Fu River is the main source of inflows, and includes water from upstream rivers, reclaimed city water, and storm runoff.
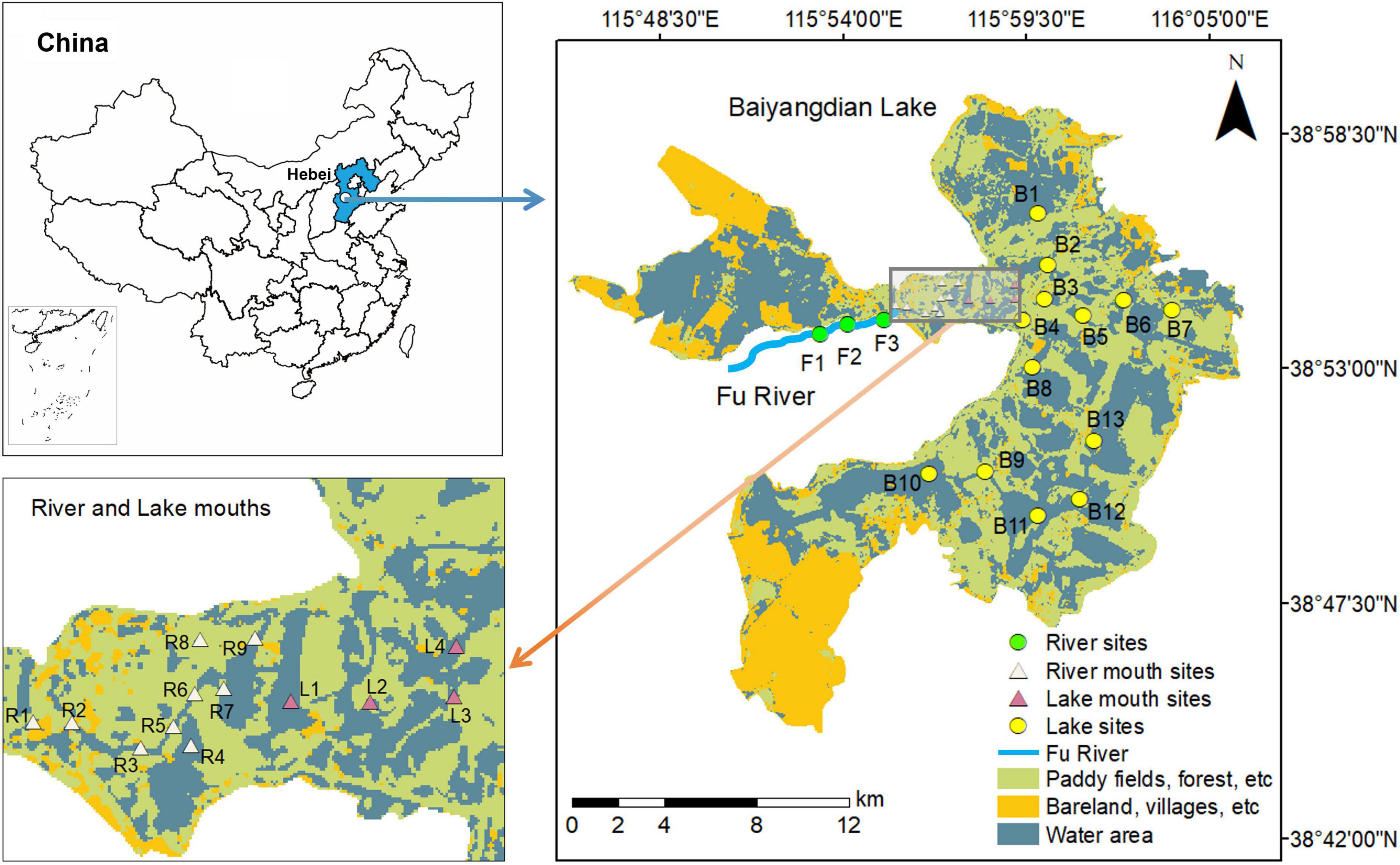
Figure 1. Location of Baiyangdian Lake in China and distribution of sampling sites in the study area.
Our study focused on quantifying the spatial differences of macrobenthos diversity among the lake, the lake–river ecotones, and the main tributary (the Fu River). Based on our preliminary field investigations, combined with measurements of the distribution of the water area in Baiyangdian Lake, we chose 13 sample sites (B1 to B13) that covered the entire lake. We chose three representative sampling sites (F1 to F3) in the downstream of the Fu River. In addition, we chose nine (R1 to R9) and four (L1 to L4) sampling sites from the river mouth and lake mouth zones, respectively (Figure 1), with the category defined by using the hydrologic boundaries of the ecotone between the Fu River and Baiyangdian lake (Tian et al., 2020). We performed five field surveys: in July and November 2018, and in April, June, and September 2019. We simultaneously collected samples of the water, sediments, and macrobenthos from the 29 sampling sites for each field survey in the river–lake system.
Samples for environmental factors
Water environmental factors
Water depth (H) and water transparency (the Secchi depth, SD) were measured on-site using a plumb-line (measured in centimeter) and a Secchi disk, respectively. We also measured the water temperature (Tw), dissolved oxygen (DO), and pH in situ with a multiparameter handheld probe (YSI Professional Plus; YSI, Yellow Springs, OH, United States). Moreover, we collected 2-L water samples for physicochemical analysis from the surface water (50 cm below the water surface) and then preserved the samples in pre-cleaned polyethylene bottles according to standard protocols (China Bureau of Environmental Protection [CBEP], 2002). These samples were transported in a portable refrigerator, and were carried back to the laboratory for further analysis. We measured total suspended solids (TSS), chemical demand oxygen (COD), total nitrogen (TN), total phosphorus (TP), phosphate (PO43–) and ammonium nitrogen (NH4+) in the laboratory. Total suspended solids (TSS) were measured by gravimetric methods. Chemical oxygen demand (COD), total nitrogen (TN) and ammonium nitrogen (NH4+) were determined using the potassium dichromate method, the alkaline potassium persulfate digestion UV spectrophotometric method and the Nessler’s reagent spectrophotometry, respectively. Total phosphorus (TP) and phosphate (PO43–) were both measured by means of ammonium molybdate tetrahydrate spectrophotometry.
Heavy metals in sediments
We collected three random sediments samples (to a depth of 10 cm) at each sampling site using a Van Veen grab sampler, and composited them into a single bulk sample. The composited samples were sealed in pre-labeled polyethylene zipper bags and stored in a portable refrigerator, and then brought back to the laboratory to determine the concentrations of As, Cd, Cr, Pb, Cu, and Zn. The sediment samples were air-dried and ground to a fine powder in the laboratory. We digested about 50 mg subsample of sediment samples using HCl–HNO3 solution (v/v, 3:1) to determine the total As content using reduction gasification–atomic fluorescence spectroscopy method according to standard protocol (HJ 680-2013). To determine the Cd, Cr, Pb, Cu, and Zn contents, we completely digested sediment subsamples (approximately 100 mg) with a 1:2:1:1 mixed acid solution (HCl–HNO3–HF–HClO4) in closed Teflon bombs, evaporated to near dryness (DigiPrep HotBlock; SCP Science, Baie d’Urfé, QC, Canada), and then diluted to 50 mL with Milli-Q water for further analysis. We determined the heavy metal contents (Cd, Cr, Pb, Cu, and Zn) in the diluted extracts using inductively coupled plasma mass spectrometry (X Series; Thermo Electron, Waltham, MA, United States) according to standard protocol (DB37/T 4435-2021).
Macrobenthos samples
We collected three random subsamples of the macrobenthos at each sampling site using a Van Veen grab sampler with a mouth area of 1/16 m2 following the general protocol by Chiasson and Williams (1999). Three subsamples were grouped into one composite sample to describe the macrobenthos community of each sampling site. Samples were sieved through a 0.425-mm mesh, and we manually removed impurities such as aquatic plants and gravel in the field. The sieve contents were then placed in white trays to let us manually remove the organisms. All macrobenthos were preserved in 75% ethanol. The organisms were identified to the lowest possible taxonomic level (i.e., species or genus) in the laboratory using a stereomicroscope (Nikon SM2, Nikon Ltd., Japan) based on appropriate identification guides (e.g., Liu et al., 1979; Morse et al., 1984; Tong, 1996; Wang, 2002; Tang, 2006), and were then counted and weighed to determine the fresh weight.
Taxonomic and functional diversity indices
Taxonomic diversity
To describe the taxonomic diversity of the macrobenthos community, we used the Shannon–Weiner diversity (H’), Simpson diversity (D), Pielou evenness (E), and Margalef richness (d) indices. The four indices were calculated as follows:
where Pi is the probability of occurrence of the ith species in a community; S is the total number of species in a community; and N is the total number of individuals in a community.
Functional diversity
We considered six functional trait classes to characterize macrobenthos, consisting of adult body size, longevity, voltinism, swimming ability, locomotion, and feeding habit (Supplementary Table 1), which have been widely used to characterize functional diversity (Coccia et al., 2021; Edegbene et al., 2021; Akamagwuna et al., 2022). For each trait, a set of attributes were used to categorize the macrobenthos species (Supplementary Table 1). These trait classes and their associated attributes are related to morphology, life history, and behavior of macrobenthos (Usseglio-Polatera et al., 2000; Akamagwuna et al., 2022), and showed sensitively response to various environmental stress (Li et al., 2019c; Li Z. F. et al., 2020; Juvigny-Khenafou et al., 2021). Additionally, they reflect the macrobenthos-related ecological functions, such as secondary production, energetic transference, and the nutrients cycle (Hébert et al., 2016; Braghin et al., 2018). We further used a binary coding approach (i.e., 1 if a species displays a trait attribute, 0 if not) to encode trait attributes of the macrobenthos collected in the river–lake system (Supplementary Table 2; Li et al., 2019a,c; Li S. H. et al., 2020; Juvigny-Khenafou et al., 2021). Information regarding the functional traits of macrobenthos was mainly obtained from the published literature on freshwater ecosystem (Li et al., 2019a; Zhang et al., 2019; Li Z. F. et al., 2020; Dolédec et al., 2021; Juvigny-Khenafou et al., 2021; Peng et al., 2021). Where the trait attributes of a given species were not available, we used information from other species within the same genus or family (Sivadas et al., 2021; Paz et al., 2022).
We used the following functional diversity indices: functional richness (FRic), functional evenness (FEve), functional divergence (FDiv), and Rao’s quadratic entropy index (RaoQ). FRic indicates the functional niche space occupied by a given community, and in general, a lower value implies low utilization of the ecological space (Villéger et al., 2008; Liu et al., 2021). FEve corresponds to the evenness of the distribution of species abundance within the functional trait space, and higher FEve indicates high utilization efficiency of the niche space (Mason et al., 2005; Villéger et al., 2008). FDiv (the functional divergence) describes the distribution of species abundance across the niche space, which reflects the degrees of niche differentiation and resource competition within the community. High FDiv entails a high degree of species niche complementarity and low resource competition (Villéger et al., 2008). RaoQ measures pairwise differences in trait composition between taxa weighted by their relative abundance (Botta-Dukát, 2005). It combines both FRic and FDiv, and therefore considers potential redundancy or complementarity between these diversity indices (Péru and Dolédec, 2010).
RLQ and fourth-corner analysis methods
We combined the RLQ method (Dolédec et al., 1996), which uses environmental variables (R), species of taxa (L), and traits (Q), with the fourth-corner method (Kleyer et al., 2012; Dray et al., 2014) to assess the responses of macrobenthos traits to environmental gradients. The RLQ method is a three-table ordination analysis that accounts for environmental variables in the R table (environment × site), for species abundance in the L table (species × site), and for functional traits in the Q table (trait × species). First, we conducted correspondence analysis (function dudi.coa) on the L table, and principal-components analysis (function dudi.pca) on the R and Q tables. We then combined these separate analyses using RLQ analysis (function rlq in R package ade4, Dolédec et al., 1996). We assessed the overall significance of this relationship using a global Monte Carlo test with 49,999 random permutations of both model 2 (H1: assuming no relationship between R and L) and model 4 (H2: assuming no relationship between L and Q). Since the global test could not test the bivariate relationship between individual environmental variables and functional traits, we used fourth-corner analysis with an adjusted p value (i.e., p-values were corrected using the false discovery rate adjustment method to limit bias due to multiple-test comparisons) to test their relationships (function fourthcorner in R package ade4, Benjamini and Hochberg, 1995; Dong et al., 2021). Furthermore, we used a combination of RLQ and fourth-corner analyses to evaluate the significance of the associations between environmental variables (or functional traits) and the RLQ axes (Dray et al., 2014).
Data analysis
We calculated the taxonomic and functional diversity indices of the macrobenthos community in each of the four ecosystem types (river, river mouth, lake mouth, lake) using version 2.5-7 of the ‘‘vegan’’ package1 and version 1.0-12 of the ‘‘FD’’ package2 for the R software (version 4.1.23), respectively. We used version 1.7-18 of the ‘‘ade4’’ package4 for the RLQ and fourth-corner analysis. In addition, to evaluate the contributions of the water environmental variables and sediment heavy metals to variation of the taxonomic and functional diversities, we carried out variance partitioning analysis using the “vegan” package.
Our primary goal was to detect relationships between environmental factors and macrobenthos diversity; because of this goal and limitations on our budget, we did not collect large enough samples on different dates to robustly test for changes in macrobenthos communities between sample dates. We used one-way ANOVA to test whether there were significant spatial differences in environmental variables, taxonomic diversity indices, and functional diversity indices. If the result was significant, we used a post hoc test (the least-significant-difference test) for pairwise comparisons of variables between pairs of ecosystem types. To identify relationships between the taxonomic and functional diversity metrics, we calculated Pearson’s correlation coefficient (r) for each ecosystem type. These statistical analyses were conducted using version 26.0 of the SPSS software5.
Results
Spatial variations of water environmental variables and sediment heavy metals
Table 1 shows the values (mean ± SE) of the water environmental variables and sediment heavy metals in the four ecosystem types. There were significant differences among the four ecosystem types in SD (F = 4.681, p < 0.05), TSS (F = 7.872, p < 0.05), DO (F = 3.095, p < 0.05), TN (F = 11.428, p < 0.05), and TP (F = 7.617, p < 0.05). Water transparency (SD) increased moving downstream from the river (with an average depth of 0.63 ± 0.06 m) to the lake (with an average depth of 0.88 ± 0.03 m), but was only significantly lower in the river than in the other sites. Mean DO was much greater in the lake mouth (8.39 ± 1.20 mg/L) and river mouth (8.39 ± 0.85 mg/L) than in the river (5.29 ± 0.61 mg/L) and lake (6.87 ± 0.43 mg/L), and the differences were significant. The highest and lowest values of TSS, TN, and TP were observed in the river and lake mouth, respectively, and the differences were significant. In addition, TN concentrations exceeded the fourth grade (IV) in the environmental quality standards for surface water of China (i.e., had water quality sufficiently bad that it was only suitable for industrial water supply and recreation in which there is no direct human contact with the water) at 80.0%, 55.6%, 54.6%, and 53.8% of sampling sites in the river, river mouth, lake mouth, and lake, respectively. The average TP ranged from 0.05 mg/L to 0.21 mg/L, and the concentrations of 88.2%, 75.9%, 66.7%, and 67.3% of the sampling sites along the river–lake gradient, respectively, exceeded grade IV.
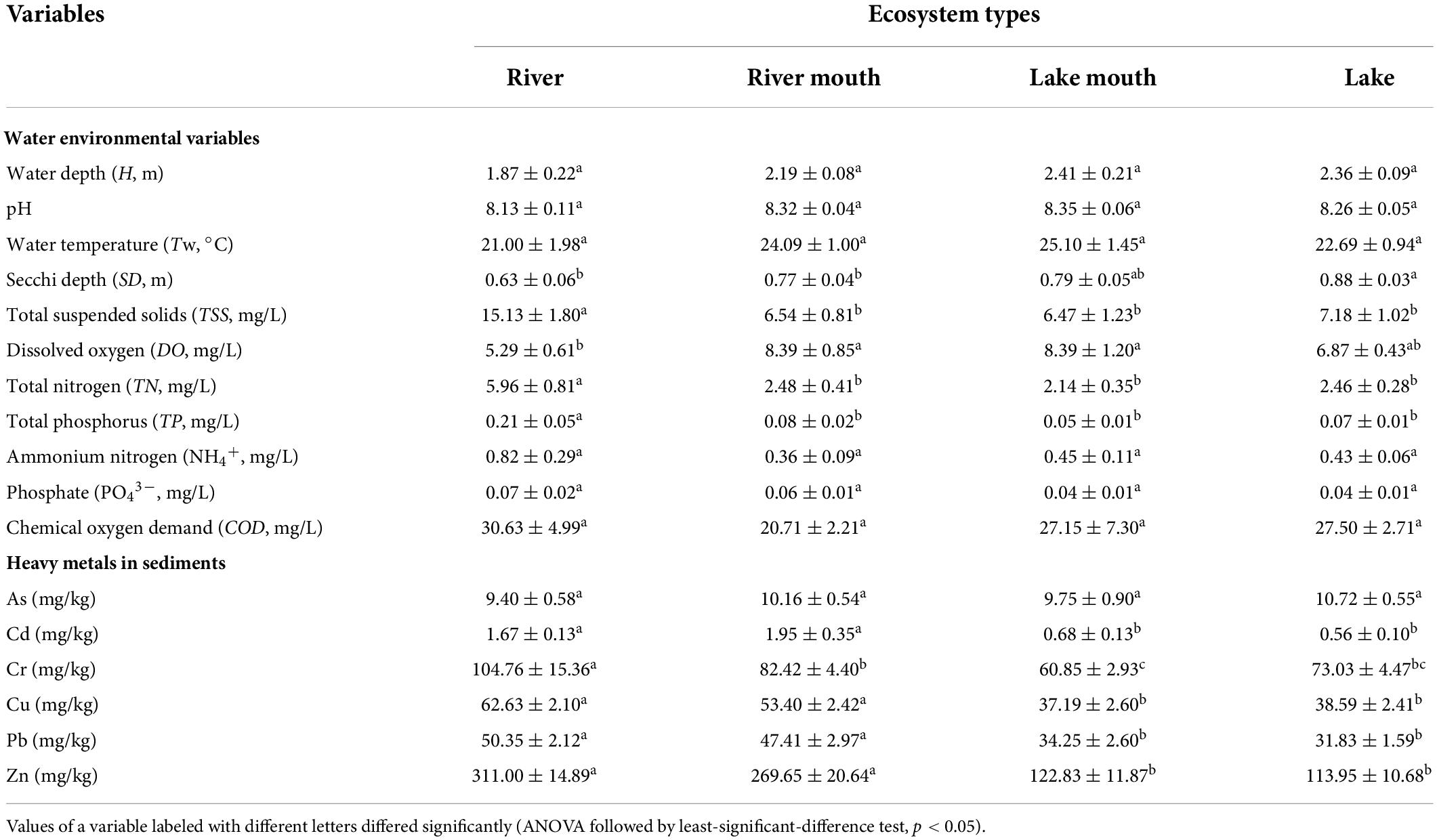
Table 1. Mean and standard error (mean ± SE) of the water environmental variables and sediment heavy metals in the four ecosystem types.
The values of Cd (F = 9.473, p < 0.05), Cr (F = 4.941, p < 0.05), Cu (F = 12.539, p < 0.05), Pb (F = 13.205, p < 0.05), and Zn (F = 29.733, p < 0.05) differed significantly among the four ecosystem types. The average Cd, Cr, Cu, Pb, and Zn concentrations were significantly higher in the river and river mouth than in the lake mouth and lake.
Macrobenthos community structure
Macrobenthos abundance and biomass
We identified a total of 30 macrobenthos taxa across all sampled areas (Supplementary Table 3): 12 Insecta (40.0%), 11 Gastropoda (36.7%), three Oligochaeta (10%), two Bivalvia (6.7%), one Crustacea (3.3%), and one Hirudinea (3.3%). The numbers of species in the different ecosystems were 13, 17, 12, and 25 in the river, river mouth, lake mouth, and lake, respectively. Among these taxa, only eight species (Limnodrilus hoffmeisteri, Bellamya aeruginosa, Bellamya purificata, Bithynia fuchsiana, Bithynia misella, Einfeldia dissidens, Propsilocerus kamusi, and Orthocladius sp.) appeared in all the four ecosystem types. Tubifex tubifex was only observed in the river. Seven species (Cipangopaludina cahayensis, Parafossarulus eximius, Semisulcospira cancellate, Stenothyra glabra, Unio dongtasiae, Glyptotendipes tokunagai, Microchironomus tener) were found only in the lake, but not in the other three study areas. Glossiphonia sp. and Polypedilum sp. were found only in the river mouth, and Dicrotendipes nervosus and Hyale changi were found only in the lake mouth.
Figure 2A shows that the total of the average abundance of all macrobenthos taxa in the lake (373.91 ± 77.79 ind./m2, mean ± SE) was significantly greater than that in the river mouth (142.48 ± 28.68 ind./m2) and lake mouth (110.55 ± 35.74 ind./m2), but not significantly different from the abundance in the river (327.38 ± 92.56 ind./m2). Limnodrilus hoffmeisteri was the most abundant species in the river (153.85 ± 54.91 ind./m2) and river mouth (64.00 ± 16.49 ind./m2), whereas Propsilocerus kamusi and Bithynia misella were the most abundant taxa in the lake mouth (37.82 ± 23.26 ind./m2) and lake (155.13 ± 44.15 ind./m2), respectively (Supplementary Table 4). The highest average macrobenthos biomass occurred in the river (164.64 ± 124.78 g/m2), followed by the lake (132.06 ± 38.58 g/m2), with the lowest biomass in the river mouth (8.41 ± 5.52 g/m2, Figure 2B); however, due to high variation, these differences were not significant. Bellamya aeruginosa was the species with the highest biomass both in the river and lake mouths (4.98 ± 3.89 g/m2 and 23.44 ± 16.09 g/m2, respectively), whereas Anodonta woodiana and Bithynia misella were the species with the highest biomass in the river (75.23 ± 75.23 g/m2) and lake (58.29 ± 23.89 g/m2), respectively (Supplementary Table 5). In addition, Supplementary Figure 1 shows the temporal changes in the species richness of macrobenthos in Baiyangdian Lake.
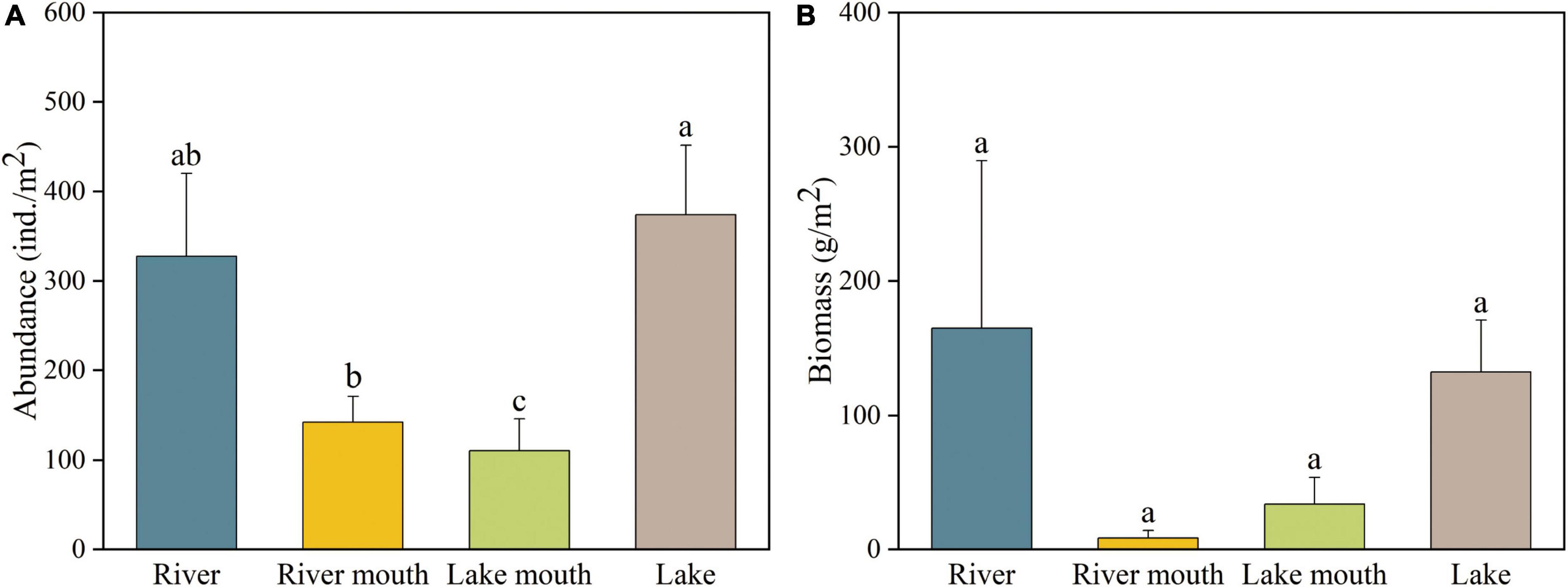
Figure 2. (A) Abundance and (B) biomass of macrobenthos communities in the four study areas. Values are mean ± SE. Values of a variable labeled with different letters differed significantly (ANOVA followed by least-significant-difference test, p < 0.05).
Macrobenthos biological traits
Figure 3 shows the proportions of the macrobenthos communities in the four study areas. The macrobenthos functional traits in the river–lake system was mainly characterized by very small body size (≤ 10 mm, BS1). The proportions of short-lived taxa (AL1) were higher than those of long-lived taxa (AL2) in the river, river mouth, and the lake, but equal in the lake mouth. The proportions of taxa with a univoltine life cycle (V2) were much higher than those of bi- or multivoltine (V3) and semivoltine (V1) taxa, and the proportion of univoltine taxa increased along the gradient from the river to the lake, with proportions of 61.5%, 64.7%, 66.7%, and 68.0%, respectively. More taxa were characterized as having no mobility (SA1) and weak mobility (SA2) in all four ecosystem types, but a few taxa with strong mobility (SA3) occurred in the lake mouth. Higher proportions of burrowers (H1, > 50%) appeared in the river and river mouth ecosystems, whereas climbers (H2) dominated the lake mouth. We found the same proportions (48%) of burrowers (H1) and climbers (H2) in the lake. Most taxa were scrapers (FH3) and collector-filterers (FH2) in all four ecosystem types. Among the 25 biological trait categories, we observed no semivoltine taxa (V1), swimming taxa (H5), and parasitic taxa (FH6) in the four ecosystem types.
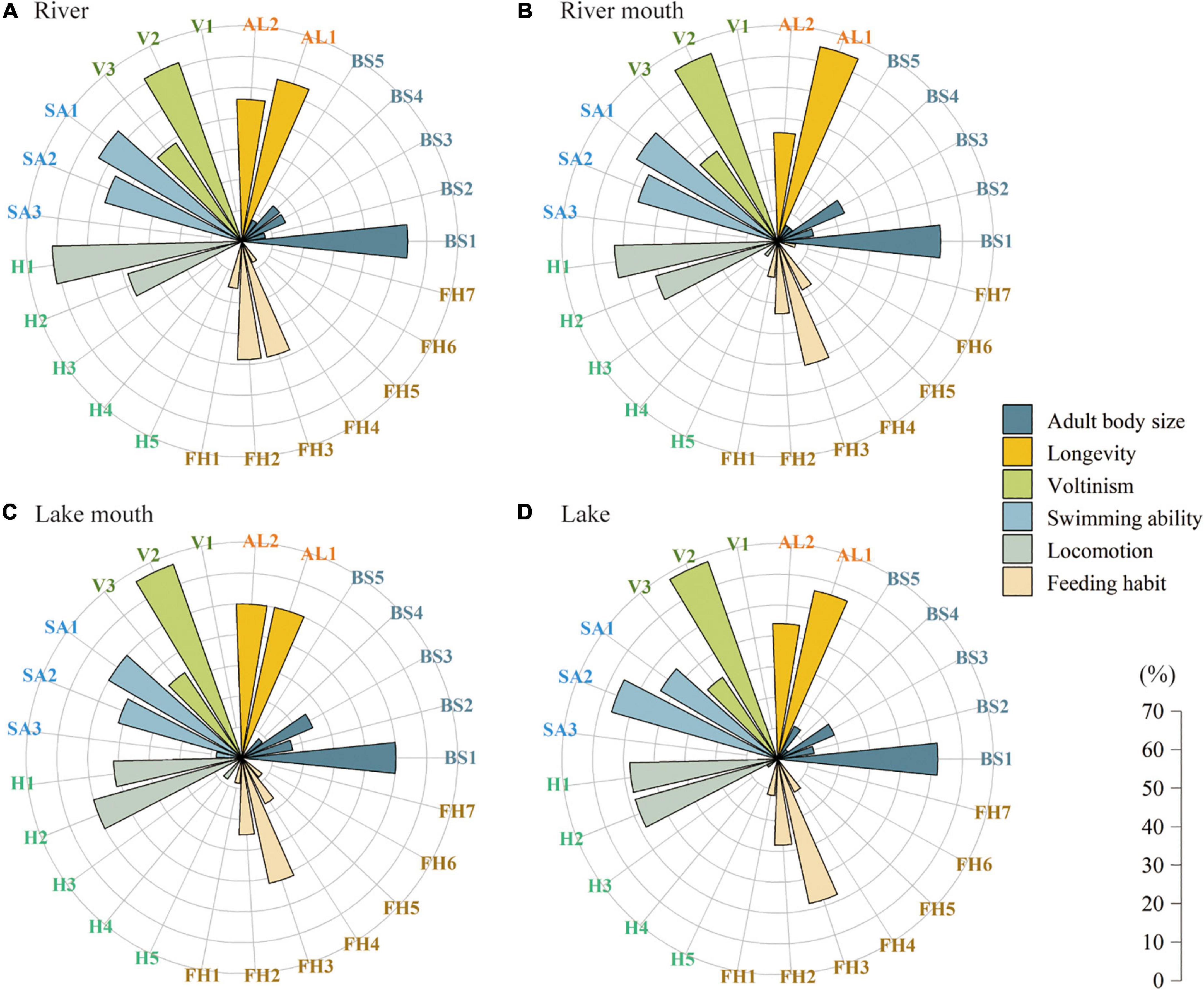
Figure 3. Proportion of the trait values for the macrobenthos communities in the four ecosystem types: (A) River, (B) River mouth, (C) Lake mouth, and (D) Lake. Trait values are defined in Supplementary Table 1.
Macrobenthos taxonomic and functional diversity
Taxonomic diversity
We found significant differences among the four ecosystem types in the Pielou evenness (F = 4.149, p < 0.05, Figure 4C). The peak and minimum values of the Pielou evenness were recorded at the lake mouth (0.99 ± 0.01, mean ± SE) and the river mouth (0.67 ± 0.07), respectively, and this difference was significant. The highest values of the Shannon–Wiener diversity and Simpson diversity both occurred at the lake mouth, followed by the lake, with the lowest values in the river mouth (Figures 4A,B); however, these differences were not significant. The average value of the Margalef richness was higher in the lake and river than that of in the river mouth and lake mouth (Figure 4D); however, these differences were not significant.
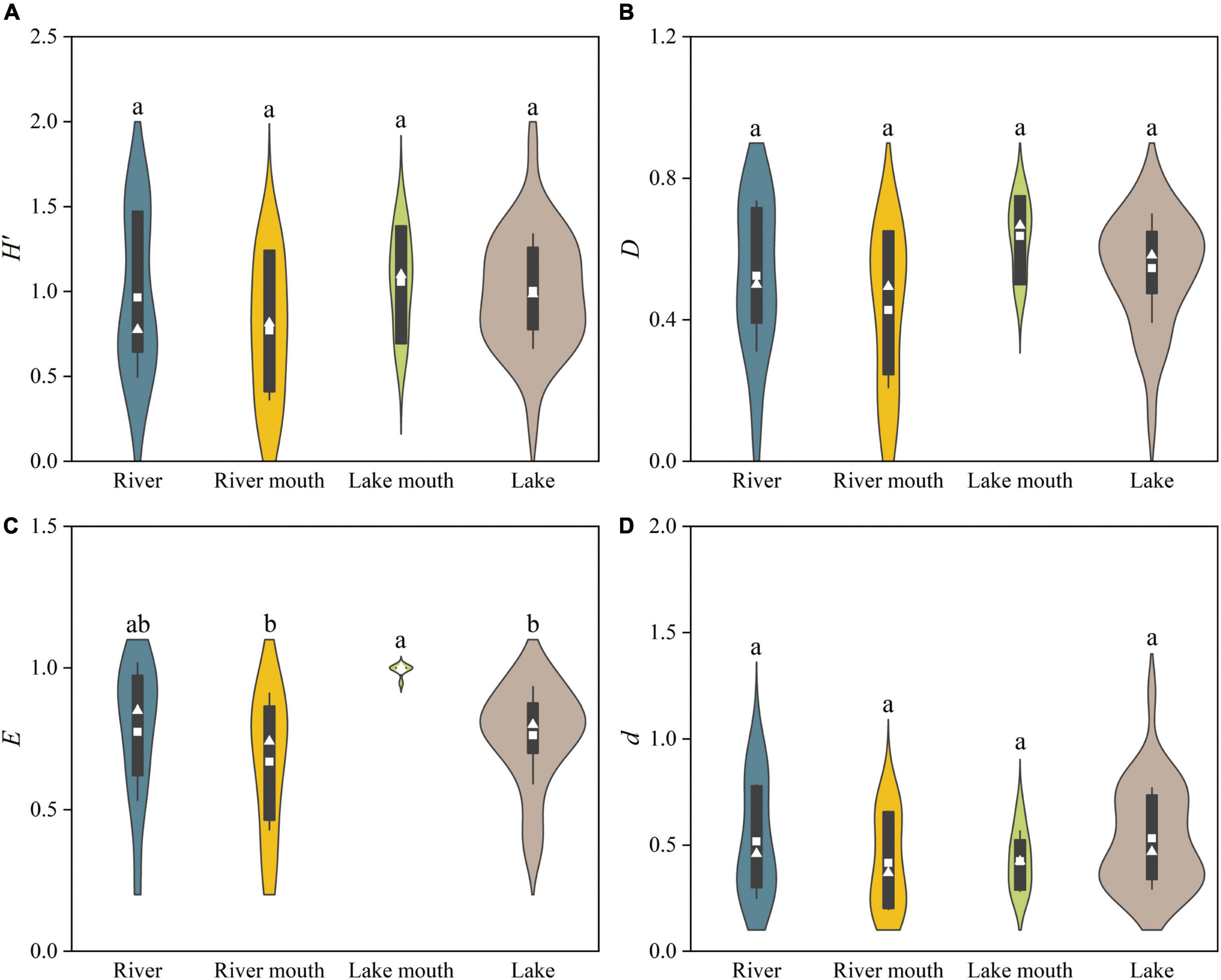
Figure 4. Taxonomic diversity indices for the macrobenthos communities in the four ecosystem types: (A) Shannon–Wiener diversity (H’), (B) Simpson diversity (D), (C) Pielou evenness (E), and (D) Margalef richness (d). Values are: Mean, white squares; standard deviation, vertical black bars; median, white triangles. The shaded areas correspond to the kernel density shapes. Distributions labeled with different letters differ significantly (ANOVA followed by least-significant-difference test, p < 0.05).
Functional diversity
FRic differed significantly among the four studied ecosystem types (F = 10.381, p < 0.05), with significantly higher values in the river (3.13 ± 0.54, mean ± SE) than in the lake mouth (0.94 ± 0.46) or the lake (0.87 ± 0.17, Figure 5A). FEve in the lake mouth and river mouth (0.68 and 0.71, respectively) were slightly greater than those in the river and lake (both about 0.62), but did not differ significantly among the locations (Figure 5B). FDiv showed the opposite pattern compared with FEve, and the differences were not significant (Figure 5C). For RaoQ, the greatest value (1.15 ± 0.23) was observed in the river, and the lowest value (0.61 ± 0.18) was in the river mouth (Figure 5D), but the differences were not significant.
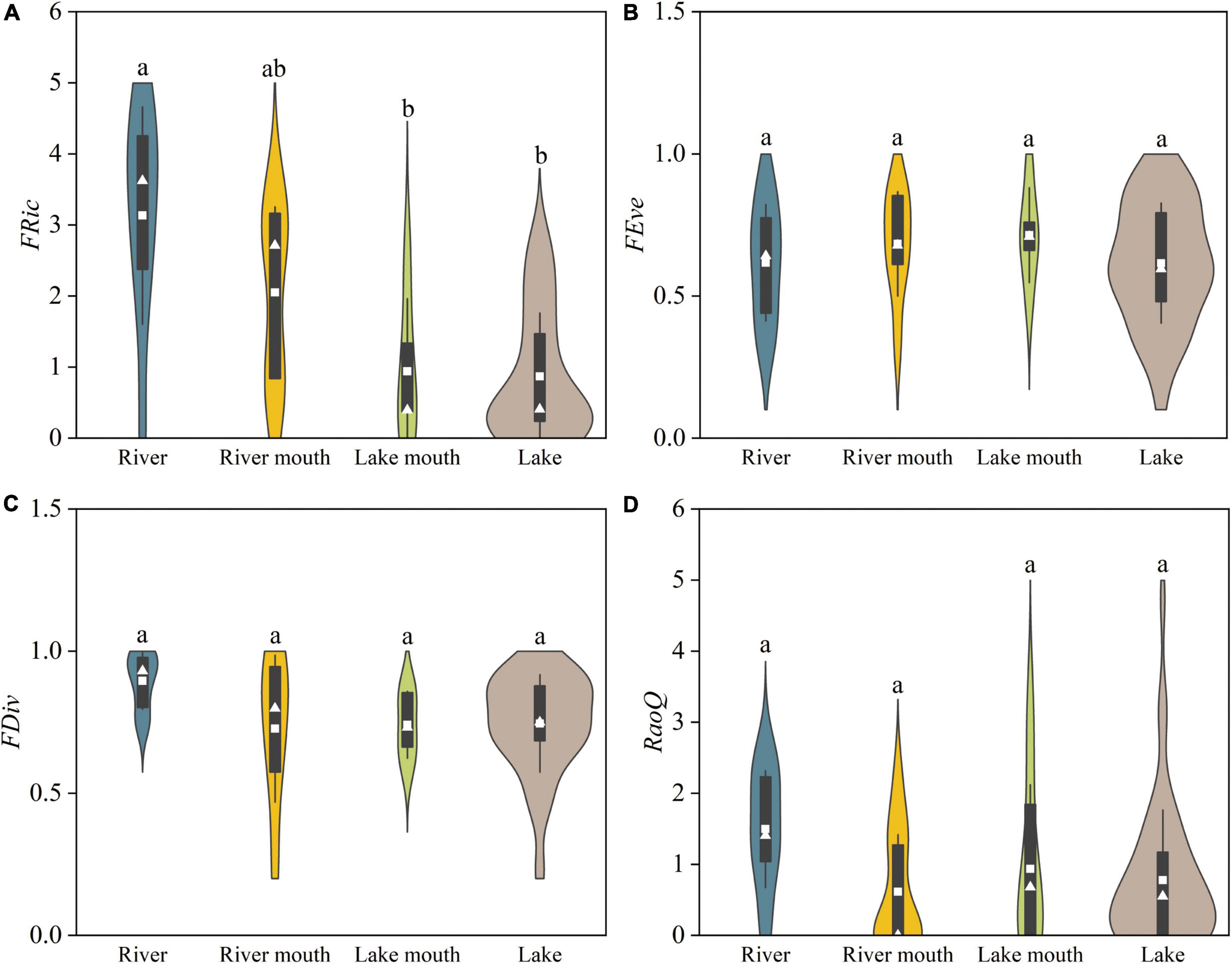
Figure 5. Functional diversity indices for macrobenthos communities in the four ecosystem types: (A) FRic, functional richness; (B) FEve, functional evenness; (C) FDiv, functional divergence; (D) RaoQ, Rao’s quadratic entropy. Values are: Mean, white squares; standard deviation, vertical black bars; median, white triangles. The shaded areas correspond to the kernel density shapes. Distributions labeled with different letters differ significantly (ANOVA followed by least-significant-difference test, p < 0.05).
Correlation between taxonomic and functional diversity indices
Figure 6 shows the significant correlations between each pair of biodiversity indices for the four ecosystem types. Overall, the taxonomic diversity indices had stronger correlations with each other than with the functional diversity indices. There were significant positive relationships between the Shannon–Wiener diversity and the other three taxonomic diversity indices in the river, river mouth, and lake, whereas Simpson diversity was significantly positively correlated with the Shannon–Wiener diversity and Margalef richness in the lake mouth. In addition, the Simpson diversity was positively correlated with all other taxonomic diversity indices in the river and lake. Among the functional diversity indices, RaoQ was significantly positively correlated with FEve in the river, FDiv was significantly positively correlated with FRic and RaoQ in the river mouth, and FRic was significantly negatively correlated with FEve in the lake. There was no significant relationship between pairs of functional diversity indices in the lake mouth. RaoQ was significantly positively correlated with all taxonomic diversity indices in the river. Pielou evenness and FDiv were significantly negatively and positively correlated in the river and lake, respectively. Among the taxonomic diversity indices, Simpson diversity and Pielou evenness were significantly positively correlated with FEve in the river but not in the lake, whereas Pielou evenness and Margalef richness were significantly positively and negatively correlated (respectively) with FEve in the lake. We found no significant correlation between the taxonomic and functional diversity indices in the river mouth and lake mouth.
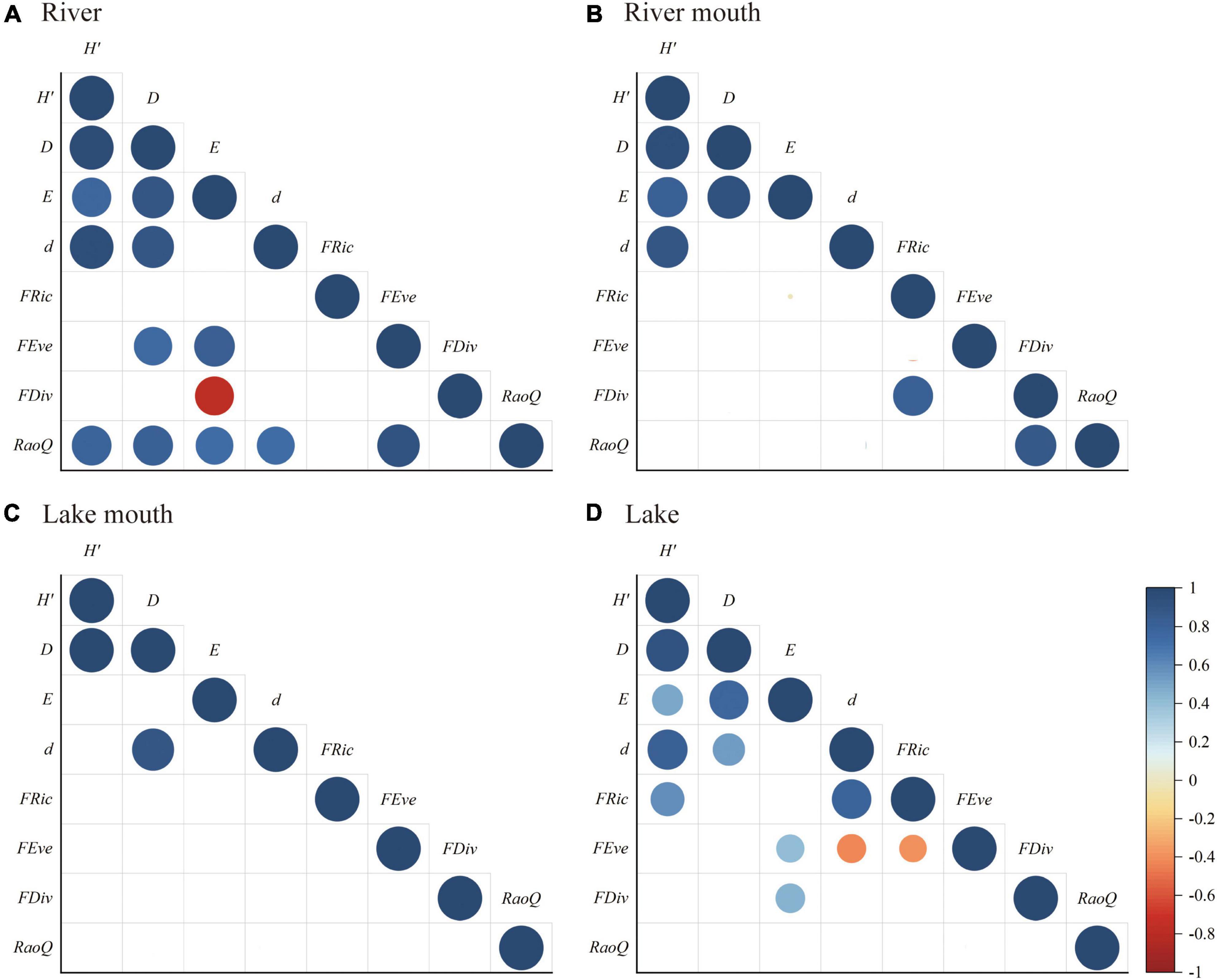
Figure 6. Pearson’s correlation coefficients (r) for the relationships between pairs of macrobenthos taxonomic and functional diversities in the (A) River, (B) River mouth, (C) Lake mouth, (D) Lake: H’, Shannon–Wiener diversity; D, Simpson diversity; E, Pielou evenness; d, Margalef richness; FRic, functional richness; FEve, functional evenness; FDiv, functional divergence; RaoQ, Rao’s quadratic entropy. The actual r values are presented in Supplementary Tables 6–9. Lower blank cells indicate insignificant correlation (p > 0.05).
Relationships between macrobenthos traits and environmental variables
Figure 7 and Supplementary Table 10 show the relationships between the macrobenthos traits and the environmental variables. Overall, these were significant (Model 2, p < 0.01; Model 4, p < 0.01). The first two axes of the RLQ multivariate analysis explained 98.9% of the total inertia of the three tables, with the first and second RLQ axes explaining 93.4% and 5.5% of the total variance, respectively.
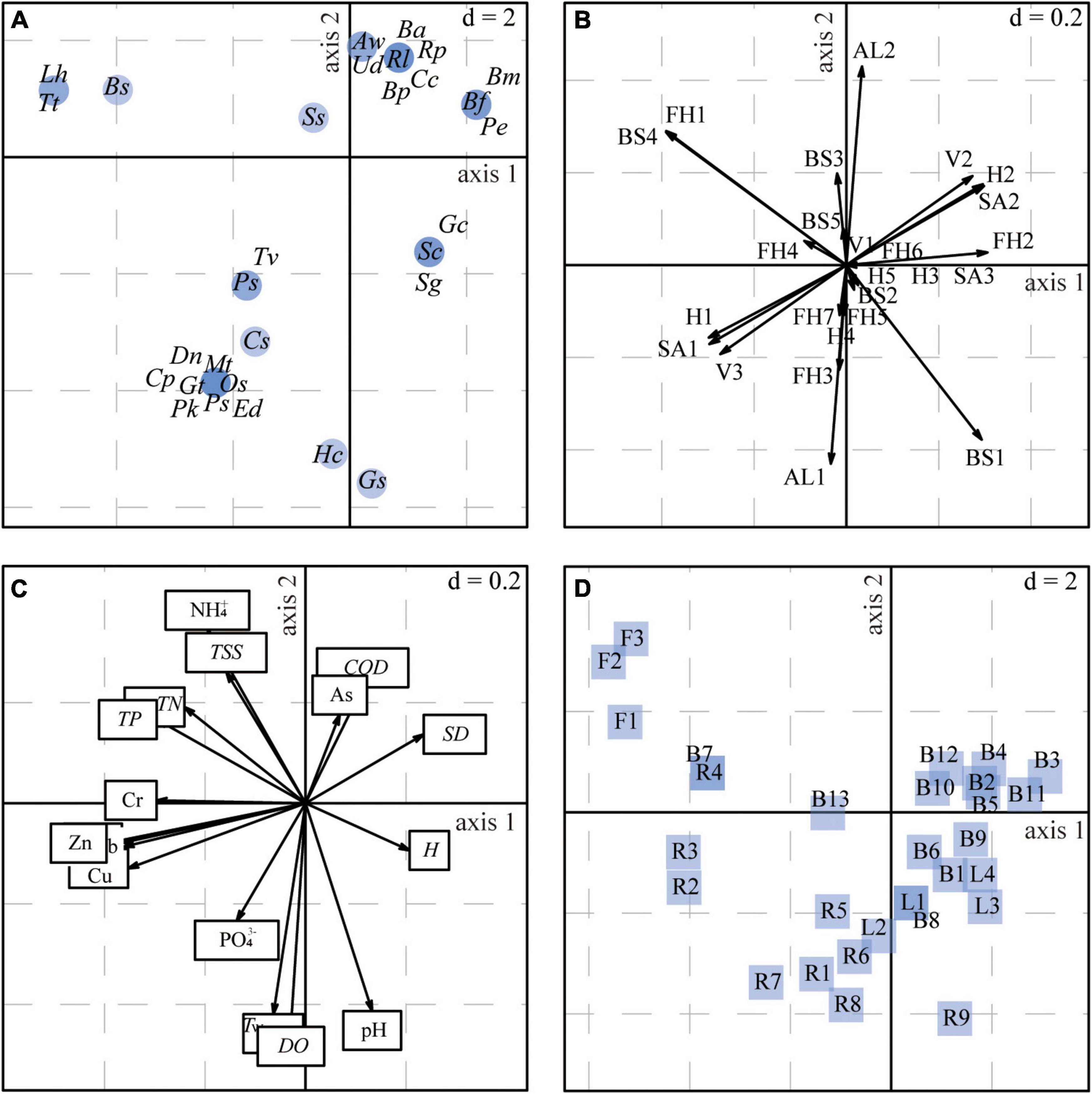
Figure 7. Results of the RLQ analysis represented by the first and second axes based on data for the four ecosystem types combined: (A) species scores, (B) trait states, (C) coefficients for the environmental variables, (D) projection of sampling sites. The d value represents the grid size of the graph. Species codes in panel (A) are defined in Supplementary Table 3; trait codes in panel (B) are defined in Supplementary Table 1.
The left (negative) part of the first RLQ axis (Figure 7A) included Oligochaeta species (i.e., Limnodrilus hoffmeisteri, Branchiura sowerbyi, and Tubifex tubifex), which were with characteristics of large body size and collector-gatherers (Figure 7B). These taxa were mostly found in the river (Figure 7D), with higher TN, TP, and Cr concentrations (Figure 7C). The left (negative) part of the first RLQ axis also showed that the trait states (e.g., burrower, no mobility) largely matched the characteristics of the Insecta species (e.g., Tanypus villipennis, Chironomus plumosus, Polypedilum sp.), which was related to the increasing heavy metal concentrations for Pb, Zn, Cu, and Cd, and tended to emerge in the river mouth. The right (positive) part of the first RLQ axis indicated trait states (e.g., univoltine life cycle, climber, weak mobility) were associated with the physical variables H and SD. The most representative species were Gastropoda (e.g., Semisulcospira cancellata, Stenothyra glabra, Gyraulus convexiusculus) and tended to emerge in the lake. The positive (top) part of the second RLQ axis showed trait states (e.g., medium body size and long life) associated with higher concentrations of NH4+, TSS, COD, and As. These trait states largely matched the characteristics of the Gastropoda and Bivalvia. Furthermore, the negative (bottom) part of the second RLQ axis illustrated that Hirudinea (Glossiphonia sp.) and Insecta (e.g., Propsilocerus kamusi, Procladius sp., Dicrotendipes nervosus) with a short life and a scraper feeding mode were more susceptible to PO, Tw, DO, and pH, and tended to emerge in the river mouth and lake mouth areas.
The fourth corner analysis showed no significant bivariate associations between a single trait state and a single environmental factor (p-adjusted < 0.05). Therefore, we further examined the relationships between individual trait states and the two RLQ environmental axes, as well as those between individual environmental variables and the two RLQ trait axes (Supplementary Figure 2). The first RLQ trait axis (AxcQ1) was significantly positively correlated with two physical variables (H and SD, p-adjusted < 0.05) and significantly negatively correlated with TN and TP (p-adjusted < 0.05). In addition, all heavy metals except As were significantly negatively correlated with the first RLQ trait axis (p-adjusted < 0.05). We found no other significant relationships between the two RLQ environmental axes and individual trait states.
Partitioning of the influences of environmental variables on macrobenthos diversities
Variance partitioning revealed that the water environmental variables and sediment heavy metals both played important roles in the macrobenthos taxonomic and functional diversity in the river–lake system (Supplementary Figure 3). Water environmental variables accounted for a larger proportion of the variation than sediment heavy metals for both taxonomic and functional diversity. The combination of both variable categories explained the taxonomic diversity well (66.8% of the variance; Supplementary Figure 3A), which was higher than the sum for the individual environmental variables (60.5%). The variability of taxonomic diversity explained by the interaction between water environmental variables and sediment heavy metals was 6.2%. For functional diversity (Supplementary Figure 3B), the sum of the individual environmental variables (89.0%) explained more than the combination of water environmental variables and sediment heavy metals (77.6%). The variability of functional diversity explained by the interaction between water environmental variables and sediment heavy metals was −11.4%. Remarkably, the amount of the variation explained by water environmental variables and sediment heavy metals were both higher for functional diversity than for taxonomic diversity.
Discussion
Spatial patterns of macrobenthos taxonomic and functional diversity
Functional diversity measures are increasingly being incorporated into making better conservation and restoration decisions for freshwater ecosystems (Cadotte et al., 2011; Schmera et al., 2017; Coccia et al., 2021). Using several functional trait indices is likely to provide more insight into functional attributes of the ecosystem than relying only on species-based indices (Gagic et al., 2015; Sotomayor et al., 2021). In the present study, we investigated the spatial patterns of macrobenthos taxonomic and functional diversity along the gradient from the Fu River to Baiyangdian Lake. Our results support our hypothesis that macrobenthos community characteristics would differ significantly along gradients in the environmental factors. The average abundance of all macrobenthos taxa was significantly greater in the lake than that in the river and lake mouths. Pielou evenness was significantly higher in the lake mouth than in the river mouth and lake. Similarly, Poznańska et al. (2010) found that the higher taxonomic diversity (e.g., Pielou eveness) but extremely lower abundance of macrobenthos in a transition zone (i.e., the sandy substrata) compared with that found at the adjacent reservoir ecosystem. Functional richness was significantly higher in the river than in the lake mouth and lake, which can be interpreted as a sign of functional redundancy in the lake mouth and lake (Arenas-Sánchez et al., 2021). In addition, greater functional evenness in the river and lake mouths indicated that functional traits were distributed more evenly across the trait space than that in the river and lake, which was associated with greater response diversity and resilience in aquatic ecosystems (Gagic et al., 2015; Schriever et al., 2015; England and Wilkes, 2018). Differences other three taxonomic diversity indices (i.e., Shannon–Weiner diversity, Simpson diversity, and Margalef richness) were not significant among the four ecosystem types. These can be related to the fact that the collected communities were dominated by few taxa with high abundances in each ecosystem type (Supplementary Table 4; Arenas-Sánchez et al., 2021).
We found significant positive correlations among the taxonomic diversity indices in our study area, which was consistent with previous studies (Heino et al., 2007; Gascón et al., 2009; Tolonen et al., 2017). Moreover, there was significant positive relationship between the Rao’s quadratic entropy and functional evenness in the river, whereas Rao’s quadratic entropy was significantly positively correlated with functional divergence in the river mouth. In contrast, De Castro-Català et al. (2020) indicated that Rao’s quadratic entropy was negatively correlated with functional evenness and functional divergence of macrobenthos in European river basins. In addition, the relationships between the taxonomic and functional diversity indices were complex and differed among the four ecosystem types. In the river, Rao’s quadratic entropy was significantly positively correlated with the four taxonomic diversity indices, which corresponded with findings of De Castro-Català et al. (2020). Similarly, Feld et al. (2014) revealed a strong positive relationship between Rao’s quadratic entropy and three taxonomic diversity indices (Shannon–Weiner diversity, Simpson diversity, and Pielou evenness) of benthic macroinvertebrates along a gradient of hydromorphological alteration across 65 lowland river sites. In the lake, Pielou evenness was significantly positively correlated with functional divergence and functional evenness. Similar results have been reported for macroinvertebrate communities in a large lake undergoing eutrophication, where Pielou evenness was found to be significantly positively correlated with functional divergence and functional evenness (Tolonen et al., 2017).
Some studies on the relationships between taxonomic and functional diversity indices of freshwater macrobenthos suggest that even if some taxonomic and functional diversity indices are correlated, the correlation is not sufficient to guarantee that they can replace each other (Heino et al., 2008; Cadotte et al., 2011; Gallardo et al., 2011; Feld et al., 2014). In particular, despite these significant correlations between macrobenthos taxonomic and functional diversity indices, this trend is not universally supported. We found no significant correlation between taxonomic and functional diversity indices in the river mouth and lake mouth ecosystems. Some studies on macrobenthos also indicated that functional diversity fluctuates independently from taxonomic diversity (Cadotte et al., 2011; Reynaga and Dos Santos, 2013). These results highlight and support the importance of functional diversity as complementary to, rather than a replacement for, taxonomic biodiversity in river–lake ecotones. The different indices quantify different and unique aspects of biodiversity, so using complementary and uncorrelated diversity indices can provide a more thorough understanding of the multiple facets of biodiversity (Wilsey et al., 2005; Gallardo et al., 2011).
Effects of water environmental variables and sediment heavy metals on the macrobenthos community
In the present study, ecotones are transitional areas between a river (a lotic environment) and a lake (a lentic environment). In such river–lake systems, ecotones can function as water purification areas and pollution buffers. Nutrient concentrations (i.e., TN and TP) in the river mouth and lake mouth were both lower than those in the adjacent river, and the lake mouth values were lower than those in the adjacent lake. The sediment Cr, Cu, Pb, and Zn concentrations in the river mouth were generally lower than those in the adjacent river but higher than those in the adjacent lake mouth. The RLQ and fourth-corner results clarified the relationships between environmental factors and functional traits in the four ecosystem types. They not only indicated that the functional traits of macrobenthos communities can respond to different environmental variables, but also support previous research, in which the functional traits of these communities are not randomly spatially distributed (Piló et al., 2016; Kuzmanovic et al., 2017; Hu et al., 2019).
The effects of water environmental variables on macrobenthos community might be complex and indirect, which might work by the alterations in their water habitat conditions. Even so we still tried to explore the statistical relationship between water environmental variables and macrobenthos community from the field survey. We found that physical conditions (H and SD) strongly correlated with the abundance and traits of many of the macrobenthos in Baiyangdian Lake, which confirms previous studies in this region (Yi et al., 2018; Yang et al., 2020). In addition, the RLQ analysis showed that with increasing eutrophication as a result of high TN and TP, a community dominated by macrobenthos with climber, scraper, and weak mobility functional traits changed to a community dominated by burrowers, collector-gatherers, and organisms with no mobility. These results support the hypothesis that spatial variation of the macrobenthos community structure can be largely attributed to a gradient from eutrophic to oligotrophic conditions in a freshwater ecosystem (Birk et al., 2020; Faghihinia et al., 2021). In addition, semivoltine and univoltine species were more common in Baiyangdian Lake than in the ecotones and the Fu River, which were lotic environments and were constantly disturbed by hydraulic exchanges. The semivoltine and univoltine macrobenthos require a longer period with a stable water environment to reach maturity and reproduce, whereas bi- or multivoltine species can reach maturity faster and maintain their populations despite constant disturbance (Weiher, 2011; Saito et al., 2015; Dolédec et al., 2021).
Our variance partitioning analysis results revealed that sediment heavy metals contributed more to the variation of functional diversity than to the variation of taxonomic diversity, which suggests that the functional diversity can serve as a good indicator for monitoring heavy metal pollution. In particular, the dominant trait modalities (burrower, no swimming ability, bi- or multivoltine life cycles) may be indicators of the effects of heavy metal pollution. Our results therefore provide information on the responses of functional traits to heavy metal pollution in river–lake ecotones. In addition, adult body size has been proven to be important in previous studies of the effects of heavy metal pollution on changes in macrobenthos functional traits (Gusmao et al., 2016; Odume, 2020; Dong et al., 2021). Adult body size is a direct and intuitive trait of macrobenthos (Edegbene et al., 2020; Odume, 2020) and is usually associated with environmental gradients. The present results indicated that a large adult body size (BS4) was a dominant trait state in areas with high sediment heavy metal contents (e.g., Cr, Pb, Zn, Cu, and Cd). These results support the common hypothesis that large adult body size is a more prevalent functional trait than small or very small adult sizes in areas contaminated with heavy metals (Gusmao et al., 2016; Dong et al., 2021). This may be because of a higher content of organic matter with high concentrations of heavy metals (Zhang et al., 2014). Areas rich in organic matter may represent a large food resource and, therefore, may increase the potential for certain organisms that feed on organic detritus to grow and attain greater body size, despite any toxic effects from heavy metals (Ryu et al., 2011; Hu et al., 2019).
Management insights
Eutrophication and heavy metal contamination have attracted much attention in Baiyangdian Lake (Zhao et al., 2011; Ji et al., 2019a,b). Although ecotones between the Fu River and Baiyangdian Lake functioned as a water purification and pollution buffer, the contents of nutrients (i.e., TN and TP) and heavy metals in the ecotones were still high. In addition, the macrobenthos abundance and biomass in the two ecotones were extremely low compared with those in the adjacent river and lake. A current dredging project aims to reduce the load of endogenous pollution (heavy metals and nutrients) in the sediments and thereby improve water quality in Baiyangdian Lake (Li et al., 2021; Zhou et al., 2021). However, previous studies of other shallow lakes (Da̧bkowski et al., 2016; Meng et al., 2018, 2020; Zou et al., 2019) showed that dredging can cause a significant reduction in the macrobenthos species richness, abundance, biomass, and taxonomic and functional diversity indices. These negative consequences could be due to the direct removal of macrobenthos in the sediment (Meng et al., 2018, 2021; Piló et al., 2019) combined with alterations of physical environmental conditions (e.g., water depth and turbidity), thereby making the environment less suitable for these organisms (Robinson et al., 2005; Meng et al., 2018). We therefore recommend that dredging be stopped until studies can be conducted to confirm that this activity will not adversely affect the macrobenthos community in the long term.
Our results show that macrobenthos taxonomic and functional diversity responded strongly to water depth, water transparency, TN, TP, and sediment heavy metals in the river–lake system. Therefore, we recommend that if dredging continues, managers define an appropriate dredging depth to protect the macrobenthos and maintain high water transparency, thereby mitigating the consequences for the macrobenthos community composition. Furthermore, most studies indicated that macrobenthos species richness, abundance, and taxonomic diversity can eventually recover after dredging, and may even surpass the pre-dredging levels during the recovery period (Aldridge, 2000; Da̧bkowski et al., 2016; Zawal et al., 2016; Piló et al., 2019; Meng et al., 2021). Thus, it will be necessary to provide a recovery period to promote restoration of the macrobenthos taxonomic and functional diversity.
Conclusion
In this study, we investigated the spatial patterns of macrobenthos taxonomic and functional diversity along a river–lake gradient for a shallow lake in Northern China. We found that nutrient concentrations (i.e., TN and TP) in the river mouth and lake mouth were both lower than those in the adjacent river and lake. Cr, Cu, Pb, and Zn contents in the river mouth and lake mouth were generally lower than those in the river. These results indicated that the river–lake ecotones functioned as a water purification and pollution buffer. The macrobenthos abundance and biomass in the two ecotones (river mouth and lake mouth) were both lower than those in the river and lake. The highest values of three macrobenthos taxonomic diversity indices (Shannon–Wiener diversity, Simpson diversity, and Pielou evenness) occurred in the lake mouth, with the lowest in the river mouth; however, the differences between ecosystem types were only significant for Pielou evenness. Moreover, the highest macrobenthos functional evenness occurred in the lake mouth, whereas the lowest values of functional divergence and Rao’s quadratic entropy index occurred in the river mouth. We also found that the first RLQ trait axis was significantly positively correlated with two physical variables (H and SD) and significantly negatively correlated with TN and TP. All heavy metals except for As were significantly negatively correlated with the first RLQ trait axis. Furthermore, the proportions of variation explained by the taxonomic and functional diversity were 66.8% and 77.6%, respectively, which reflect the combined effects of water environmental variables and sediment heavy metals. Overall, our results suggest that studies of macrobenthos functional diversity in ecotones will complement the information provided by classical taxonomic diversity indices, and can therefore provide useful data to support the management of river–lake systems. Because we combined samples from multiple dates in our analysis, it was not possible to test whether the environmental values and macrobenthos community varied over time. Improving our understanding of these variations will be an important area of future research. In addition, the macrobenthos are only one component of biodiversity. In future research, it will be important to sample other components of biodiversity, such as the plant and fish communities.
Data availability statement
The original contributions presented in this study are included in the article/Supplementary material, further inquiries can be directed to the corresponding author/s.
Author contributions
XF, DL, and LZ conducted the field sampling. XF and DL processed samples in the laboratory. XF and LZ analyzed and visualized the data. WY and XF wrote the first draft of this manuscript. WY obtained the funding. XL and LZ revised the manuscript. All authors conceived and designed the study.
Funding
This study was supported by the National Natural Science Foundation of China (no. 52079006), the National Key R&D Program of China (no. 2017YFC0404505), and the Beijing Advanced Innovation Program for Land Surface Science.
Acknowledgments
We would like to thank Geoffrey Hart for providing language help during the writing of this manuscript.
Conflict of interest
The authors declare that the research was conducted in the absence of any commercial or financial relationships that could be construed as a potential conflict of interest.
Publisher’s note
All claims expressed in this article are solely those of the authors and do not necessarily represent those of their affiliated organizations, or those of the publisher, the editors and the reviewers. Any product that may be evaluated in this article, or claim that may be made by its manufacturer, is not guaranteed or endorsed by the publisher.
Supplementary material
The Supplementary Material for this article can be found online at: https://www.frontiersin.org/articles/10.3389/fevo.2022.922539/full#supplementary-material
Footnotes
- ^ https://cran.r-project.org/web/packages/vegan/index.html
- ^ https://cran.r-project.org/web/packages/FD/index.html
- ^ http://www.r-project.org/
- ^ https://cran.r-project.org/web/packages/ade4/index.html
- ^ https://www.ibm.com/analytics/spss-statistics-software
References
Akamagwuna, F. C., Odume, O. N., and Richoux, N. B. (2022). Exploring the community structure of Afrotropical macroinvertebrate traits and ecological preferences along an agricultural pollution gradient in the Kat River, Eastern Cape, South Africa. Ecol. Indic. 135:108570. doi: 10.1016/j.ecolind.2022.108570
Aldridge, D. C. (2000). The impacts of dredging and weed cutting on a population of freshwater mussels (Bivalvia: Unionidae). Biol. Conserv. 95, 247–257. doi: 10.1016/S0006-3207(00)00045-8
Allen, C. D., and Breshears, D. D. (1998). Drought-induced shift of a forest–woodland ecotone: Rapid landscape response to climate variation. Proc. Natl. Acad. Sci. U.S.A. 95, 14839–14842. doi: 10.1073/pnas.95.25.14839
Arenas-Sánchez, A., Dolédec, S., Vighi, M., and Rico, A. (2021). Effects of anthropogenic pollution and hydrological variation on macroinvertebrates in Mediterranean rivers: A case-study in the upper Tagus river basin (Spain). Sci. Total Environ. 766:144044. doi: 10.1016/j.scitotenv.2020.144044
Azrina, M. Z., Yap, C. K., Rahim Ismail, A., Ismail, A., and Tan, S. G. (2006). Anthropogenic impacts on the distribution and biodiversity of benthic macroinvertebrates and water quality of the Langat River, Peninsular Malaysia. Ecotoxicol. Environ. Saf. 64, 337–347. doi: 10.1016/j.ecoenv.2005.04.003
Baker, N. J., Pilotto, F., Haubrock, P. J., Beudert, B., and Haase, P. (2021). Multidecadal changes in functional diversity lag behind the recovery of taxonomic diversity. Ecol. Evol. 11, 17471–17484. doi: 10.1002/ece3.8381
Benjamini, Y., and Hochberg, Y. (1995). Controlling the false discovery rate: A practical and powerful approach to multiple testing. J. R. Stat. Soc. Ser. B 57, 289–300. doi: 10.1111/j.2517-6161.1995.tb02031.x
Birk, S., Chapman, D., Carvalho, L., Spears, B. M., Andersen, H. E., Argillier, C., et al. (2020). Impacts of multiple stressors on freshwater biota across spatial scales and ecosystems. Nat. Ecol. Evol. 4, 1060–1068. doi: 10.1038/s41559-020-1216-4
Botta-Dukát, Z. (2005). Rao’s quadratic entropy as a measure of functional diversity based on multiple traits. J. Veg. Sci. 16, 533–540. doi: 10.1111/j.1654-1103.2005.tb02393.x
Braghin, L. S. M., Almeida, B. A., Amaral, D. C., Canella, T. F., Gimenez, B. C. G., and Bonecker, C. C. (2018). Effects of dams decrease zooplankton functional β−diversity in river-associated lakes. Freshw. Biol. 63, 721–730. doi: 10.1111/fwb.13117
Brumm, K. J., Daniel Hanks, R., Baldwin, R. F., and Peoples, B. K. (2021). Accounting for multiple dimensions of biodiversity to assess surrogate performance in a freshwater conservation prioritization. Ecol. Indic. 122:107320. doi: 10.1016/j.ecolind.2020.107320
Buffagni, A. (2021). The lentic and lotic characteristics of habitats determine the distribution of benthic macroinvertebrates in Mediterranean rivers. Freshw. Biol. 66, 13–34. doi: 10.1111/fwb.13596
Cadotte, M. W., Carscadden, K., and Mirotchnick, N. (2011). Beyond species: Functional diversity and the maintenance of ecological processes and services. J. Appl. Ecol. 48, 1079–1087. doi: 10.1111/j.1365-2664.2011.02048.x
Cai, Y. J., Zhang, Y., Wu, Z. S., Chen, Y. W., Xu, J., and Gong, Z. J. (2017). Composition, diversity, and environmental correlates of benthic macroinvertebrate communities in the five largest freshwater lakes of China. Hydrobiologia 788, 85–98. doi: 10.1007/s10750-016-2989-y
Chiasson, A., and Williams, C. (1999). Protocols for assessing water quality and aquatic biodiversity using macroinvertebrates. Lower Cove: Fundy Model Forest.
China Bureau of Environmental Protection [CBEP] (2002). Methods for monitoring and analysis of water and wastewater, 4th Edn. Beijing: China Environmental Science Press.
Coccia, C., Almeida, B. A., Green, A. J., Gutiérrez, A. B., and Carbonell, J. A. (2021). Functional diversity of macroinvertebrates as a tool to evaluate wetland restoration. J. Appl. Ecol. 58, 2999–3011. doi: 10.1111/1365-2664.14038
Da̧bkowski, P., Buczyński, P., Zawal, A., Stêpień, E., Buczyńska, E., Stryjecki, R., et al. (2016). The impact of dredging of a small lowland river on water beetle fauna (Coleoptera). J. Limnol. 75, 472–487. doi: 10.4081/jlimnol.2016.1270
De Castro-Català, N., Dolédec, S., Kalogianni, E., Skoulikidis, N. T., Paunovic, M., Vasiljeviæ, B., et al. (2020). Unravelling the effects of multiple stressors on diatom and macroinvertebrate communities in European river basins using structural and functional approaches. Sci. Total Environ. 742:140543. doi: 10.1016/j.scitotenv.2020.140543
Dolédec, S., Chessel, D., ter Braak, C. J. F., and Champely, S. (1996). Matching species traits to en- vironmental variables: A new three-table ordination method. Environ. Ecol. Stat. 3, 143–166. doi: 10.1007/BF02427859
Dolédec, S., Simon, L., Blemus, J., Rigal, A., Robin, J., and Mermillod-Blondin, F. (2021). Multiple stressors shape invertebrate assemblages and reduce their trophic niche: A case study in a regulated stream. Sci. Total Environ. 773:145061. doi: 10.1016/j.scitotenv.2021.145061
Dong, J. Y., Zhao, L., Sun, X., Hu, C., Wang, Y., Li, W. T., et al. (2021). Response of macrobenthic communities to heavy metal pollution in Laoshan Bay, China: A trait-based method. Mar. Pollut. Bull. 167:112292. doi: 10.1016/j.marpolbul.2021.112292
Dray, S., Choler, P., Dolédec, S., Peres-Neto, P. R., Thuiller, W., Pavoine, S., et al. (2014). Combining the fourth-corner and the RLQ methods for assessing trait responses to environmental variation. Ecology 95, 14–21. doi: 10.1890/13-0196.1
Edegbene, A. O., Arimoro, F. O., and Odume, O. N. (2020). Exploring the distribution patterns of macroinvertebrate signature traits and ecological preferences and their responses to urban and agricultural pollution in selected rivers in the Niger Delta ecoregion, Nigeria. Aquat. Ecol. 54, 553–573. doi: 10.1007/s10452-020-09759-9
Edegbene, A. O., Odume, O. N., Arimoro, F. O., and Keke, U. N. (2021). Identifying and classifying macroinvertebrate indicator signature traits and ecological preferences along urban pollution gradient in the Niger Delta. Environ. Pollut. 281:117076. doi: 10.1016/j.envpol.2021.117076
England, J., and Wilkes, M. A. (2018). Does river restoration work? Taxonomic and functional trajectories at two restoration schemes. Sci. Total Environ. 618, 961–970. doi: 10.1016/j.scitotenv.2017.09.014
Eriksen, T. E., Brittain, J. E., Søli, G., Jacobsen, D., Goethals, P., and Friberg, N. (2021). A global perspective on the application of riverine macroinvertebrates as biological indicators in Africa, South-Central America, Mexico and Southern Asia. Ecol. Indic. 126:107609. doi: 10.1016/j.ecolind.2021.107609
Espinoza-Toledo, A., Mendoza-Carranza, M., Castillo, M. M., Barba-Macías, E., and Capps, K. A. (2021). Taxonomic and functional responses of macroinvertebrates to riparian forest conversion in tropical streams. Sci. Total Environ. 757:143972. doi: 10.1016/j.scitotenv.2020.143972
Faghihinia, M., Xu, Y., Liu, D., and Wu, N. (2021). Freshwater biodiversity at different habitats: Research hotspots with persistent and emerging themes. Ecol. Indic. 129:107926. doi: 10.1016/j.ecolind.2021.107926
Feld, C. K., de Bello, F., and Dolédec, S. (2014). Biodiversity of traits and species both show weak responses to hydromorphological alteration in lowland river macroinvertebrates. Freshw. Biol. 59, 233–248. doi: 10.1111/fwb.12260
Gagic, V., Bartomeus, I., Jonsson, T., Taylor, A., Winqvist, C., Fischer, C., et al. (2015). Functional identity and diversity of animals predict ecosystem functioning better than species-based indices. Proc. R. Soc. B Biol. Sci. 282:20142620. doi: 10.1098/rspb.2014.2620
Gallardo, B., Gascón, S., Quintana, X., and Comín, F. A. (2011). How to choose a biodiversity indicator—redundancy and complementarity of biodiversity metrics in a freshwater ecosystem. Ecol. Indic. 11, 1177–1184. doi: 10.1016/j.ecolind.2010.12.019
Gascón, S., Boix, D., and Sala, J. (2009). Are different biodiversity metrics related to the same factors? A case study from Mediterranean wetlands. Biol. Conserv. 142, 2602–2612. doi: 10.1016/j.biocon.2009.06.008
Gonzalo, C., and Camargo, J. A. (2013). The impact of an industrial effluent on the water quality, submersed macrophytes and benthic macroinvertebrates in a dammed river of central Spain. Chemosphere 93, 1117–1124. doi: 10.1016/j.chemosphere.2013.06.032
Gusmao, J. B., Brauko, K. M., Eriksson, B. K., and Lana, P. C. (2016). Functional diversity of macrobenthic assemblages decreases in response to sewage discharges. Ecol. Indic. 66, 65–75. doi: 10.1016/j.ecolind.2016.01.003
Hébert, M. P., Beisner, B. E., and Maranger, R. (2016). A meta-analysis of zooplankton functional traits influencing ecosystem function. Ecology 97, 1069–1080. doi: 10.1890/15-1084.1
Heino, J., Mykrä, H., Hämäläinen, H., Aroviita, J., and Muotka, T. (2007). Responses of taxonomic distinctness and species diversity indices to anthropogenic impacts and natural environmental gradients in stream macroinvertebrates. Freshw. Biol. 52, 1846–1861. doi: 10.1111/j.1365-2427.2007.01801.x
Heino, J., Mykrä, H., and Kotanen, J. (2008). Weak relationships between landscape characteristics and multiple facets of stream macroinvertebrate biodiversity in a boreal drainage basin. Landsc. Ecol. 23, 417–426. doi: 10.1007/s10980-008-9199-6
Heino, J., and Tolonen, K. T. (2017). Ecological drivers of multiple facets of beta diversity in a lentic macroinvertebrate metacommunity. Limnol. Oceanogr. 62, 2431–2444. doi: 10.1002/lno.10577
Hillbricht-Ilkowska, A. (1999). Shallow lakes in lowland river systems: Role in transport and transformations of nutrients and in biological diversity. Hydrobiologia 408, 349–358. doi: 10.1007/978-94-017-2986-4_39
Holland, M. M. (1988). “SCOPE/MAB technical consultations on landscape boundaries. Report of a SCOPE/MAB workshop on ecotones,” in A new look at ecotones: Emerging international projects on landscape boundaries, Vol. 17, eds F. di Castri, A. J. Hansen, and M. M. Holland (Paris: International Union of Biological Sciences), 47–106.
Hu, C. Y., Dong, J. Y., Gao, L. J., Yang, X. L., Wang, Z., and Zhang, X. M. (2019). Macrobenthos functional trait responses to heavy metal pollution gradients in a temperate lagoon. Environ. Pollut. 253, 1107–1116. doi: 10.1016/j.envpol.2019.06.117
Jakubik, B., Koperski, P., and Lewandowski, K. (2015). Diversity of mollusca in lowland river–lake system: Lentic versus lotic patches. Pol. J. Ecol. 62, 335–348. doi: 10.3161/104.062.0212
Ji, Z. H., Zhang, H., Zhang, Y., Chen, T., Long, Z. W., Li, M., et al. (2019a). Distribution, ecological risk and source identification of heavy metals in sediments from the Baiyangdian Lake, Northern China. Chemosphere 237:124425. doi: 10.1016/j.chemosphere.2019.124425
Ji, Z. H., Zhang, Y., Zhang, H., Huang, C. X., and Pei, Y. S. (2019b). Fraction spatial distributions and ecological risk assessment of heavy metals in the sediments of Baiyangdian Lake. Ecotoxicol. Environ. Saf. 174, 417–428. doi: 10.1016/j.ecoenv.2019.02.062
Juvigny-Khenafou, N. P. D., Piggott, J. J., Atkinson, D., Zhang, Y., Macaulay, S. J., Wu, N., et al. (2021). Impacts of multiple anthropogenic stressors on stream macroinvertebrate community composition and functional diversity. Ecol. Evol. 11, 133–152. doi: 10.1002/ece3.6979
Kleyer, M., Dray, S., Bello, F., Lepš, J., Pakeman, R. J., Strauss, B., et al. (2012). Assessing species and community functional responses to environmental gradients: Which multivariate methods? J. Veg. Sci. 23, 805–821. doi: 10.1111/j.1654-1103.2012.01402.x
Kolasa, J., and Zalewski, M. (1995). Notes on ecotone attributes and functions. Hydrobiologia. 303, 1–7. doi: 10.1007/BF00034039
Krebs, R. A., Prescott, T. J., Clapham, W. B., and Klarer, D. M. (2018). Freshwater mussel assemblages at the lotic-lentic interface along Lake Erie. Am. Malacol. Bull. 36, 31–41. doi: 10.4003/006.036.0104
Kuzmanovic, M., Dolédec, S., de Castro-Catala, N., Ginebreda, A., Sabater, S., Muñoz, I., et al. (2017). Environmental stressors as a driver of the trait composition of benthic macroinvertebrate assemblages in polluted Iberian rivers. Environ. Res. 156, 485–493. doi: 10.1016/j.envres.2017.03.054
Lento, J., Laske, S. M., Lavoie, I., Bogan, D., Brua, R. B., Campeau, S., et al. (2020). Diversity of diatoms, benthic macroinvertebrates, and fish varies in response to different environmental correlates in Arctic rivers across North America. Freshw. Biol. 67, 95–115. doi: 10.1111/fwb.13600
Li, D., Erickson, R. A., Tang, S., Zhang, Y., Niu, Z., Liu, H., et al. (2016). Structure and spatial patterns of macrobenthic community in Tai Lake, a large shallow lake, China. Ecol. Indic. 61, 179–187. doi: 10.1016/j.ecolind.2015.08.043
Li, X. X., Yang, W., Sun, T., and Su, L. Y. (2019a). Framework of multidimensional macrobenthos biodiversity to evaluate ecological restoration in wetlands. Environ. Res. Lett. 14:054003. doi: 10.1088/1748-9326/ab142c
Li, Z. F., Jiang, X. M., Wang, J., Meng, X. L., Heino, J., and Xie, Z. C. (2019b). Multiple facets of stream macroinvertebrate alpha diversity are driven by different ecological factors across an extensive altitudinal gradient. Ecol. Evol. 9, 1306–1322. doi: 10.1002/ece3.4841
Li, Z. F., Wang, J., Liu, Z. Y., Meng, X. L., Heino, J., Jiang, X. K., et al. (2019c). Different responses of taxonomic and functional structures of stream macroinvertebrate communities to local stressors and regional factors in a subtropical biodiversity hotspot. Sci. Total Environ. 655, 1288–1300. doi: 10.1016/j.scitotenv.2018.11.222
Li, Y. L., Lv, J. X., and Li, L. J. (2021). Coordinated development of water environment protection and water ecological carbon sink in Baiyangdian Lake. Processes 9:2066. doi: 10.3390/pr9112066
Li, Z. F., Liu, Z. Y., Heino, J., Jiang, X. M., Wang, J., Tang, T., et al. (2020). Discriminating the effects of local stressors from climatic factors and dispersal processes on multiple biodiversity dimensions of macroinvertebrate communities across subtropical drainage basins. Sci. Total Environ. 711:134750. doi: 10.1016/j.scitotenv.2019.134750
Li, S. H., Sun, T., Yang, W., Cui, B. S., and Yin, X. A. (2020). A biodiversity evaluation framework for restoration of aquatic macrophyte communities in shallow lakes driven by hydrological process management. Hydrol. Process. 35:e13983. doi: 10.1002/hyp.13983
Liu, Y., Zhang, M., Peng, W. Q., Qu, X. D., Zhang, Y. H., Du, L. F., et al. (2021). Phylogenetic and functional diversity could be better indicators of macroinvertebrate community stability. Ecol. Indic. 129:107892. doi: 10.1016/j.ecolind.2021.107892
Liu, Y. Y., Zhang, W. Z., and Wang, Y. X. (1979). China economic animal: Freshwater mollusks. Beijing: Science Press.
Martello, F., De Bello, F., De Castro Morini, M. S., Silva, R. R., De Souza-Campana, D. R., Ribeiro, M. C., et al. (2018). Homogenization and impoverishment of taxonomic and functional diversity of ants in Eucalyptus plantations. Sci. Rep. 8:3266. doi: 10.1038/s41598-018-20823-1
Mason, N. W. H., Mouillot, D., Lee, W. G., and Wilson, J. B. (2005). Functional richness, functional evenness and functional divergence: The primary components of functional diversity. Oikos 111, 112–118. doi: 10.1111/j.0030-1299.2005.13886.x
Meng, X. L., Chen, J. J., Li, Z. F., Liu, Z. Y., Jiang, X. K., Ge, Y. H., et al. (2020). Degraded functional structure of macroinvertebrates caused by commercial sand dredging practices in a flood plain lake. Environ. Pollut. 263:114415. doi: 10.1016/j.envpol.2020.114415
Meng, X. L., Cooper, K. M., Liu, Z., Li, Z. Y., Chen, J. J., Jiang, X. K., et al. (2021). Integration of α, β and γ components of macroinvertebrate taxonomic and functional diversity to measure of impacts of commercial sand dredging. Environ. Pollut. 269:116059. doi: 10.1016/j.envpol.2020.116059
Meng, X. L., Jiang, X. M., Li, Z. F., Wang, J., Cooper, K. M., and Xie, Z. C. (2018). Responses of macroinvertebrates and local environment to short-term commercial sand dredging practices in a flood-plain lake. Sci. Total Environ. 631-632, 1350–1359. doi: 10.1016/j.scitotenv.2018.03.086
Morse, J. C., Yang, L., and Tian, L. (1984). Aquatic insects of China useful for monitoring water quality. Nanjing: Hohai University Press.
Mosbahi, N., Serbaji, M. M., Pezy, J. P., Neifar, L., and Dauvin, J. C. (2019). Response of benthic macrofauna to multiple anthropogenic pressures in the shallow coastal zone south of Sfax (Tunisia, central Mediterranean Sea). Environ. Pollut. 253, 474–487. doi: 10.1016/j.envpol.2019.06.080
Murcia, C. (1995). Edge effects in fragmented forests: Implications for conservation. Trends Ecol. Evol. 10, 58–62. doi: 10.1016/S0169-5347(00)88977-6
Obolewski, K., Glińska-Lewczuk, K., Jarząb, N., Burandt, P., Kobus, S., Kujawa, R., et al. (2014). Benthic invertebrates in floodplain lakes of a Polish River: Structure and biodiversity analyses in relation to hydrological conditions. Pol. J. Environ. Stud. 23, 1679–1689.
O’Brien, A., Townsend, K., Hale, R., Sharley, D., and Pettigrove, V. (2016). How is ecosystem health defined and measured? A critical review of freshwater and estuarine studies. Ecol. Indic. 69, 722–729. doi: 10.1016/j.ecolind.2016.05.004
Odume, O. N. (2020). Searching for urban pollution signature and sensitive macroinvertebrate traits and ecological preferences in a river in the Eastern Cape of South Africa. Ecol. Indic. 108:105759. doi: 10.1016/j.ecolind.2019.105759
Patrick, C. J. (2014). Macroinvertebrate communities of ecotones between the boundaries of streams, wetlands, and lakes. Fundam. Appl. Limnol. 185, 223–233. doi: 10.1127/fal/2014/0645
Paz, L. E., Rodriguez, M., Gullo, B., and Rodrigues Capítulo, A. (2022). Impacts of urban and industrial pollution on functional traits of benthic macroinvertebrates: Are some traits advantageous for survival? Sci. Total Environ. 807:150650. doi: 10.1016/j.scitotenv.2021.150650
Peng, F. J., ter Braak, C. J. F., Rico, A., and Van den Brink, P. J. (2021). Double constrained ordination for assessing biological trait responses to multiple stressors: A case study with benthic macroinvertebrate communities. Sci. Total Environ. 754:142171. doi: 10.1016/j.scitotenv.2020.142171
Péru, N., and Dolédec, S. (2010). From compositional to functional biodiversity metrics in bioassessment: A case study using stream macroinvertebrate communities. Ecol. Indic. 10, 1025–1036. doi: 10.1016/j.ecolind.2010.02.011
Piló, D., Ben-Hamadou, R., Pereira, F., Carriço, A., Pereira, P., Corzo, A., et al. (2016). How functional traits of estuarine macrobenthic assemblages respond to metal contamination? Ecol. Indic. 71, 645–659. doi: 10.1016/j.ecolind.2016.07.019
Piló, D., Carvalho, A. N., Pereira, F., Coelho, H. E., and Gaspar, M. B. (2019). Evaluation of macrobenthic community responses to dredging through a multimetric approach: Effective or apparent recovery? Ecol. Indic. 96, 656–668. doi: 10.1016/j.ecolind.2018.08.064
Poznańska, M., Kobak, J., Wolnomiejski, N., and Kakareko, T. (2010). Macrozoobenthos communities from two types of land-water transition zones in a European lowland dam reservoir. Fundam. Appl. Limnol. 176, 115–126. doi: 10.1127/1863-9135/2010/0176-0115
Reynaga, M. C., and Dos Santos, D. A. (2013). Contrasting taxonomical and functional responses of stream invertebrates across space and time in a Neotropical basin. Fundam. Appl. Limnol. 183, 121–133. doi: 10.1127/1863-9135/2013/0501
Risser, P. G. (1995). The status of the science examining ecotones. Bioscience 45, 318–325. doi: 10.2307/1312492
Robinson, J. E., Newell, R. C., Seiderer, L. J., and Simpson, N. M. (2005). Impacts of aggregate dredging on sediment composition and associated benthic fauna at an offshore dredge site in the southern North Sea. Mar. Environ. Res. 60, 51–68. doi: 10.1016/j.marenvres.2004.09.001
Ryu, J., Khim, J. S., Kang, S. G., Kang, D., Lee, C. H., and Koh, C. H. (2011). The impact of heavy metal pollution gradients in sediments on benthic macrofauna at population and community levels. Environ. Pollut. 159, 2622–2629. doi: 10.1016/j.envpol.2011.05.034
Saito, V. S., Siqueira, T., and Fonseca-Gessner, A. A. (2015). Should phylogenetic and functional diversity metrics compose macroinvertebrate multimetric indices for stream biomonitoring? Hydrobiologia 745, 167–179. doi: 10.1007/s10750-014-2102-3
Salvo, J., Valdovinos, C., and Fierro, P. (2020). Benthic macroinvertebrate assemblages of a stream-lake network in the upper zone of the trans-Andean basin of the Valdivia River (Chile). N. Z. J. Mar. Freshw. Res. 55, 375–392. doi: 10.1080/00288330.2020.1784239
Schmera, D., Heino, J., Podani, J., Erõs, T., and Dolédec, S. (2017). Functional diversity: A review of methodology and current knowledge in freshwater macroinvertebrate research. Hydrobiologia 787, 27–44. doi: 10.1007/s10750-016-2974-5
Schriever, T. A., Bogan, M. T., Boersma, K. S., Cañedo-Argüelles, M., Jaeger, K. L., Olden, J. D., et al. (2015). Hydrology shapes taxonomic and functional structure of desert stream invertebrate communities. Freshw. Sci. 34, 399–409. doi: 10.1086/680518
Sivadas, S. K., Gupta, G. V. M., Kumar, S., and Ingole, B. S. (2021). Trait-based and taxonomic macrofauna community patterns in the upwelling ecosystem of the southeastern Arabian sea. Mar. Environ. Res. 170:105431. doi: 10.1016/j.marenvres.2021.105431
Soldner, M., Stephen, I., Ramos, L., Angus, R., Wells, N. C., Grosso, A., et al. (2004). Relationship between macroinvertebrate fauna and environmental variables in small streams of the Dominican Republic. Water Res. 38, 863–874. doi: 10.1016/S0043-1354(03)00406-8
Sotomayor, G., Hampel, H., Vázquez, R. F., Forio, M. A. E., and Goethals, P. L. M. (2021). Implications of macroinvertebrate taxonomic resolution for freshwater assessments using functional traits: The Paute River Basin (Ecuador) case. Divers. Distrib. 28, 1735–1747. doi: 10.1111/ddi.13418
Steneck, R. S., and Dethier, M. N. (1994). A functional group approach to the structure of algal-dominated communities. Oikos 69, 476–498. doi: 10.2307/3545860
Szkokan-Emilson, E. J., Wesolek, B. E., and Gunn, J. M. (2011). Terrestrial organic matter as subsidies that aid in the recovery of macroinvertebrates in industrially damaged lakes. Ecol. Appl. 21, 2082–2093. doi: 10.1890/10-1967.1
Tang, H. Q. (2006). Biosystematic study on the chironomid larvae in China (Diptera: Chironomidae). TianJing: Nankai University, 945.
Tian, K., Yang, W., Zhao, Y. W., Yin, X. A., Cui, B. S., and Yang, Z. F. (2020). Development of a hydrological boundary method for the river–lake transition zone based on flow velocity gradients, and case study of Baiyangdian Lake transition zones, China. Water 12:674. doi: 10.3390/w12030674
Tolonen, K. T., Vilmi, A., Karjalainen, S. M., Hellsten, S., and Heino, J. (2017). Do different facets of littoral macroinvertebrate diversity show congruent patterns in a large lake system? Commun. Ecol. 18, 109–116. doi: 10.1556/168.2017.18.1.12
Usseglio-Polatera, P., Bournaud, M., Richoux, P., and Tachet, H. (2000). Biological and ecological traits of benthic freshwater macroinvertebrates relationships and definition of groups with similar traits. Freshw. Biol. 43, 175–205. doi: 10.1046/j.1365-2427.2000.00535.x
Van De Meutter, F., Stoks, R., and De Meester, L. (2006). Rapid response of macroinvertebrates to drainage management of shallow connected lakes. J. Appl. Ecol. 43, 51–60. doi: 10.1111/j.1365-2664.2005.01115.x
Villéger, S., Mason, N. W. H., and Mouillot, D. (2008). New multidimensional functional diversity indices for a multifaceted framework in functional ecology. Ecology 89, 2290–2301. doi: 10.1890/07-1206.1
Violle, C., Navas, M.-L., Vile, D., Kazakou, E., Fortunel, C., Hummel, I., et al. (2007). Let the concept of trait be functional! Oikos 116, 882–892. doi: 10.1111/j.2007.0030-1299.15559.x
Wang, H. Z. (2002). Studies on taxonomy, distribution and ecology of microdrile oligochaetes of China, with descriptions of two new species from the vicinity of the great wall station of China, Antarctica. Beijing: Higher Education Press.
Ward, J. V., and Tockner, K. (2001). Biodiversity: Towards a unifying theme for river ecology. Freshw. Biol. 46, 807–819. doi: 10.1046/j.1365-2427.2001.00713.x
Weiher, E. (2011). “A primer of trait and functional diversity,” in Biological diversity: Frontiers in measurement and assessment, eds A. E. Magurran and B. J. McGill (Oxford: Oxford University Press), 175–193.
Willis, T. V., and Magnuson, J. J. (2000). Patterns in fish species composition across the interface between streams and lakes. Can. J. Fish. Aquat. Sci. 57, 1042–1052. doi: 10.1139/cjfas-57-5-1042
Wilsey, B. J., Chalcraft, D. R., Bowles, C. M., and Willig, M. R. (2005). Relationships among indices suggest that richness is an incomplete surrogate for grassland biodiversity. Ecology 86, 1178–1184. doi: 10.1890/04-0394
Winterbourn, M. J. (1971). The life histories and trophic relationships of the trichoptera of Marion Lake, British Columbia. Can. J. Zool. 49, 623–635. doi: 10.1139/z71-100
Wu, H. P., Chen, J., Xu, J. J., Zeng, G. M., Sang, L. H., Liu, Q., et al. (2019). Effects of dam construction on biodiversity: A review. J. Clean. Prod. 221, 480–489. doi: 10.1016/j.jclepro.2019.03.001
Yang, Y. F., Yi, Y. J., Zhou, Y., Wang, X., Zhang, S. H., and Yang, Z. F. (2020). Spatio-temporal variations of benthic macroinvertebrates and the driving environmental variables in a shallow lake. Ecol. Indic. 110:105948. doi: 10.1016/j.ecolind.2019.105948
Yi, Y. J., Sun, J., Yang, Y. F., Zhou, Y., Tang, C. H., Wang, X., et al. (2018). Habitat suitability evaluation of a benthic macroinvertebrate community in a shallow lake. Ecol. Indic. 90, 451–459. doi: 10.1016/j.ecolind.2018.03.039
Zawal, A., Czachorowski, S., Stępień, E., Buczyńska, E., Szlauer-Łukaszewska, A., Buczyński, P., et al. (2016). Early post-dredging recolonization of caddisflies (Insecta: Trichoptera) in a small lowland river (NW Poland). Limnology 17, 71–85. doi: 10.1007/s10201-015-0466-3
Zhang, C., Shan, B. Q., Zhao, Y., Song, Z. X., and Tang, W. Z. (2018). Spatial distribution, fractionation, toxicity and risk assessment of surface sediments from the Baiyangdian Lake in Northern China. Ecol. Indic. 90, 633–642. doi: 10.1016/j.ecolind.2018.03.078
Zhang, C., Yu, Z. G., Zeng, G. M., Jiang, M., Yang, Z. Z., Cui, F., et al. (2014). Effects of sediment geochemical properties on heavy metal bioavailability. Environ. Int. 73, 270–281. doi: 10.1016/j.envint.2014.08.010
Zhang, C., Zhu, R., Sui, X. Y., Li, X. Q., and Chen, Y. F. (2021). Understanding patterns of taxonomic diversity, functional diversity, and ecological drivers of fish fauna in the Mekong River. Glob. Ecol. Conserv. 28:e01711. doi: 10.1016/j.gecco.2021.e01711
Zhang, W. L., Zhu, M. J., Li, Y., Wang, C., Qian, B., Niu, L. H., et al. (2020). How fluvial inputs directly and indirectly affect the ecological status of different lake regions: A bio-assessment framework. J. Hydrol. 582:124502. doi: 10.1016/j.jhydrol.2019.124502
Zhang, Y., Cheng, L., Li, K. Y., Zhang, L., Cai, Y. J., Wang, X. L., et al. (2019). Nutrient enrichment homogenizes taxonomic and functional diversity of benthic macroinvertebrate assemblages in shallow lakes. Limnol. Oceanogr. 64, 1047–1058. doi: 10.1002/lno.11096
Zhao, Y., Xia, X. H., Yang, Z. F., and Xia, N. (2011). Temporal and spatial variations of nutrients in Baiyangdian Lake, North China. J. Environ. Inf. 17, 102–108. doi: 10.3808/jei.201100192
Zhou, H., Zhang, W. J., Li, L. Q., Zhang, M. Y., and Wang, D. S. (2021). Environmental impact and optimization of lake dredged-sludge treatment and disposal technologies based on life cycle assessment (LCA) analysis. Sci. Total Environ. 787:147703. doi: 10.1016/j.scitotenv.2021.147703
Keywords: taxonomic diversity, functional diversity, macrobenthos, environmental factors, river-lake ecotone, Baiyangdian lake-river system
Citation: Fu X, Yang W, Zheng L, Liu D and Li X (2022) Spatial patterns of macrobenthos taxonomic and functional diversity throughout the ecotones from river to lake: A case study in Northern China. Front. Ecol. Evol. 10:922539. doi: 10.3389/fevo.2022.922539
Received: 18 April 2022; Accepted: 22 July 2022;
Published: 10 August 2022.
Edited by:
Celso Santos, Federal University of Paraíba, BrazilReviewed by:
Augustine Ovie Edegbene, Federal University of Health Sciences, NigeriaLinlin Zhao, Ministry of Natural Resources, China
Copyright © 2022 Fu, Yang, Zheng, Liu and Li. This is an open-access article distributed under the terms of the Creative Commons Attribution License (CC BY). The use, distribution or reproduction in other forums is permitted, provided the original author(s) and the copyright owner(s) are credited and that the original publication in this journal is cited, in accordance with accepted academic practice. No use, distribution or reproduction is permitted which does not comply with these terms.
*Correspondence: Wei Yang, eWFuZ3dlaUBibnUuZWR1LmNu