- 1School of Agriculture, Graphic Era Hill University, Dehradun, Uttarakhand, India
- 2Department of Biotechnology, Graphic Era Deemed to be University, Dehradun, Uttarakhand, India
- 3Uttarakhand Biotechnology Council, US Nagar, Uttarakhand, India
- 4Uttarakhand Space Application Centre, Dehradun, Uttarakhand, India
- 5Department of Environmental Science, Graphic Era Deemed to be University, Dehradun, Uttarakhand, India
- 6Botany Division, Forest Research Institute, Dehradun, Uttarakhand, India
- 7Department of Life Sciences, Graphic Era Deemed to be University, Dehradun, Uttarakhand, India
- 8Smart-Health Initiative (SHI) and Red Sea Research Center (RSRC), Division of Biological and Environmental Sciences and Engineering (BESE), King Abdullah University of Science and Technology (KAUST), Thuwal, Saudi Arabia
- 9Department of Pharmaceutics, Unaizah College of Pharmacy, Qassim University, Unaizah, Saudi Arabia
Himalayan mountain forests have been a potential candidate for the investigation of perturbations due to the complex geography in which they sustain and the sensitivity of the species toward human disturbance and climate change. Among various tree species, brown oak (Quercus semecarpifolia), a very important component of the Himalayan mountains, has been identified as a keystone species due to its substantial economic and ecological benefits. Maintenance of microclimate and suitable habitats with a rich source of natural resources makes Q. semecarpifolia the most preferred forest for luxuriant growth of ground flora, shelter for fauna, and multipurpose uses by the local people. In a climax community, it plays a critical role in environmental balance both at the local and regional levels. Unfortunately, it has become one of the most overexploited tree species of the Himalayan region over the last few decades due to its high demand for dry season fodder and firewood. The wide range of seedling distribution 348–4,663 individuals ha–1 is evidence of the disturbance accompanied by poor regeneration in Q. semecarpifolia forests. Moreover, litter accumulation and grass cover adversely affect seed germination. The ecological cost of oak forest degradation is perhaps more important and damage is irreversible. Thus, continuous demand and extensive threats accompanied by poor regeneration have drawn the attention of stakeholders to conserve this species. However, propagation protocol, especially the pre-sowing treatment of the species, has not been impressive for large-scale multiplication. This review is comprehensive information on distribution, phenology, regeneration pattern, human threat, conservation approaches, and management of Q. semecarpifolia in the Himalayan region.
Introduction
Quercus belongs to the family Fagaceae and is the biggest genus with 600 species worldwide (Tudge, 2006), commonly known as oak. Oaks are found in northern temperate, subtropical, and tropical Asia and the Andes of South America. Himalayan temperate oaks are evergreen, medium- to large-sized trees, mostly found in clusters. The distribution of oak plants occurs between 800 and 3,800 m asl throughout the Himalayan region. In this region, more than 35 species have been reported (Negi and Naithani, 1995), most of which are plentiful across the temperate region. The oak possesses greater economic and ecological values compared to other associated plant species. All the oak species of the Himalayan region serve as an important source of livelihood (fodder, fuelwood, charcoal, agricultural tools, and bee boxes) (Negi and Naithani, 1995). In addition to economic benefits, oak plants are of great ecological significance. Many oak plants have been identified as keystone species that play a vital role in the stability of a complex ecosystem web (Shrestha, 2003; Maren and Vetaas, 2007). Oak forests, being a climax community, attract important attention in ecological succession processes (Singh and Singh, 1992; Rawat, 2014) and also help in watershed protection by promoting the recharge of mountain springs (Valdia, 1998). Unfortunately, the regenerative capability of this important forest element is poor not only in the Himalayan region but also in North America (Lorimer et al., 1994) and Europe (Andersson, 1991) owing to its vulnerability to climate change (Bisht et al., 2013). Irregular seed production, frugivory, herbivory, animal browsing, and stem and leaf damage by insects and pests are the major reasons for poor seed production and germination (Singh and Singh, 1987; Andersson, 1991; Lorimer et al., 1994; Singh et al., 2021). In addition, forest fire, extensive lopping, and accumulation of thick litter with a slow decomposition rate further retard the regeneration rate of oak trees (Thadani and Ashton, 1995; Singh, 2014; Shekhar et al., 2021).
Of the total reported oak species in the Himalayan region, only five naturally occurring species are found in Western Himalayas, namely, Quercus glauca (harinj), Quercus leucotrichophora (banj), Quercus floribunda (moru), Quercus lanuginosa (rianj), and Quercus semecarpifolia (kharsu). There are several characteristics that make Q. semecarpifolia important compared with other Quercus species in the Himalayas such as (i) among all the oak species, Q. semecarpifolia forms the most extensive belt along the higher elevations between 2,400 and 3,800 m in the Western Himalayas. (ii) It is most abundant and widespread around 2,700–3,300 m, where it exhibits 80% dominance (based on relative basal area) while between 2,400 and 2,700 m it shares dominance with Q. floribunda. (iii) It is one of the most preferred tree species for multiple uses by local people in the Himalayan region (Orwa et al., 2009; Singh et al., 2021), such as fodder (the tree is lopped extensively in the hills of Himachal Pradesh, Uttarakhand, and Uttar Pradesh), food (bears are particularly fond of acorns, seed powder used for thickening in stews and mixed with cereals for making bread), fuel (it makes good firewood and excellent charcoal; the calorific value of sapwood is 4,879 kcal and of heartwood 4,815 kcal/kg of dry wood), timber (it is used only locally for building, door frames, beds steads, ploughs, mule saddles, substitute for imported oak for kegs in distilleries), other services (mulch of the leaves repels slugs, grubs; silkworms of Antheraea proylei are widely cultivated on the leaves of oaks, and the leaves contain 4.5% ash and 1.37% nitrogen). The closed canopy allows the growth of shade-loving ground vegetation. Vascular and non-vascular epiphytic plants grow luxuriantly on the trunks and branches of mature trees. (v) In a climax community, it is a keystone species, playing a critical role in environmental balance at both the local and also regional levels. (vi) Khasru oak forest supplies dry season fodder, manure, higher quality firewood, and durable timber. Supply of fodder allows the farmers continue the practice of keeping animals and ultimately supporting the crop production in the region (Shrestha and Paudel, 1996). (vii) A discernible better positive impact has been observed on the overall performance of goats fed on Q. semecarpifolia than other Quercus species-based diet (Singh et al., 1998; Singh and Todaria, 2012; Raju et al., 2015, 2018). Based on the preciousness of Q. semecarpifolia over other oak and associated species and failure of regeneration under human disturbances, it requires immediate attention for the conservation of this species. To achieve this, a detailed study of species distribution, composition, species biology, disturbance level, and various scientific interventions in Q. semecarpifolia is urgently required. Several studies have been conducted covering various aspects of the Q. semecarpifolia such as taxonomy and distribution (Naithani, 1984; Singh and Singh, 1986; Singh and Rawat, 2000), composition, structure, and function (Singh et al., 1997; Joshi and Samant, 2014; Rawat, 2014; Negi et al., 2018; Rawat and Negi, 2021; Rawat et al., 2021a; Singh et al., 2021), failure of regeneration (Negi and Naithani, 1995; Singh et al., 1997), and spatiotemporal changes (Rawat et al., 2021b; Shekhar et al., 2022); however, a detailed review is lacking, which is imperative for preparing long-term monitoring and management strategies of the species. In this article, the authors have tried to provide a comprehensive account of species distribution, plant biology, abundance, and present status with a special focus on regeneration patterns, human threat, and management interventions.
Considering the importance of this article, a detailed literature search was carried out. Various search engines, viz., Web of Science,1 ScienceDirect,2 ACS publications, Taylor and Francis Online, SpringerLink, Scopus,3 Web of Science, PubMed,4 National Library of Medicine,5 Wiley online library databases were used. Research papers, review papers, books and book chapters, reports, and conference and seminar proceedings on Q. semecarpifolia were used to compile the data. Different keywords like Quercus, Quercus semecarpifolia, Kharsu, Brown oak, Q. semecarpifolia habitat, Q. semecarpifolia geography, Q. semecarpifolia ecology, Q. semecarpifolia distribution, Q. semecarpifolia diversity, Q. semecarpifolia regeneration, Q. semecarpifolia conservation, and Q. semecarpifolia biotechnology were used to investigate the distribution, use, ecological, biotechnological, molecular, and conservation aspects of Q. semecarpifolia. An extensive database on various aspects of Q. semecarpifolia from 1921 until 2022 was searched using 169 references that were used to prepare this article.
Quercus semecarpifolia at a glance
In 1814, James Edward Smith described the soubriquet “semecarpifolia” denoting the resemblance of its leaves to those of an Indian plant Semecarpus anacardium, known as “marking nut” (Negi and Rawal, 2018).
Taxonomy, morphology, and phenology
The taxonomic classification of Q. semecarpifolia is as follows:
Kingdom: Plantae
Phylum: Spermatophyta
Subphylum: Angiospermae
Class: Dicotyledonae
Order: Fagales
Family: Fagaceae
Genus: Quercus
Species: Quercus semecarpifolia
Quercus semecarpifolia (local name Khasru) is a middle to large spread, evergreen tree with a height of 25–35 m (Shrestha, 2003; Shrestha et al., 2013) and a girth of 210 cm or more under favorable conditions. It is a climax community of the Himalayan region and has been identified as one of the oldest vegetation types in this region (Negi and Naithani, 1995; Negi and Rawal, 2018). The larger trees are generally hollow, with young parts covered with soft hairs. The bark of the trees is generally rough, dark gray with cracked rectangular scales. Leaves are 5–12 × 2.5–7.5 cm in size, elliptic or oblong-shaped, spinous-toothed in young trees often entire on older trees, coriaceous, and glabrous. The new leaves, subtended by brown deciduous stipules, are bright green above and nearly white beneath. The branches are often decorated with mosses and lichens. Male catkins are crowded, 5–10 cm long, and congested in seed years and 10–20 cm long in other years. The male catkins and female spikes appear in dense drooping clusters on the new shoots. The flowers have ciliated perianth and indefinite stamens. Acorn is 2.5 cm diameter, globose, and found as solitary, in pairs or clusters of 3–6 on previous year’s shoots. The oak nuts are black when ripe with soft and leathery cups enclosing approximately one-third of the nut (Orwa et al., 2009).
Phenological characteristics are depicted in Table 1. The falling of leaves in old trees begins in April–June (Shrestha, 2003; Orwa et al., 2009; Bisht et al., 2013; Shrestha et al., 2013; Singh et al., 2021), but all leaves do not fall until the new leaves are formed. The new shoots appear in April–May at lower elevations, whereas on the higher elevations the new shoots start appearing after June. In terms of acorn growth, minimum/no growth occurs in the first season. However, the speedy growth of green and dark brown-colored acorns of all sizes succeeds in the second season. The ripening of acorns takes approximately 15 months commencing from the flowering time (Shrestha, 2003). The ripening of the acorn takes place from June to August, and germination takes place immediately after the fruit falls. More than 95% of fresh seeds are viable and can germinate in normal conditions. Partial vivipary has also been reported in some seeds, where seeds start germination even before they fall on the ground (Negi and Naithani, 1995). Different foliar phenology of Khasru has been observed with the regions. In this sequence, completely leafless trees have been reported in Nepal during early September (Shrestha and Lekhak, 2002). Seed dispersal takes place between May and July followed by seedling emergence in July–August.
Climate and soil
Temperature, precipitation, and evapotranspiration are key determinants for the exchange of energy and mass in any ecosystem (Shenbin et al., 2006). Variation is relatively consistent in local or global weather conditions due to abiotic and biotic forces that may alter the biological clock of plants. Phenological events in the oaks growing in subalpine-timberline forests (i.e., Q. semecarpifolia) are triggered with the commencement of favorable growth seasons (Bisht, 2010; Singh et al., 2021). Soil is one of the most important factors for tree growth, which is governed by weathering and climatic conditions. The rate of growth and type of species depend upon the mineral composition of the soil (Raina and Gupta, 2009). Simultaneously, the influence of tree species on forest soil properties has also been investigated (Zinke, 1962; Singh et al., 1986; Vesterdal et al., 2008). Soil organic carbon, soil total nitrogen, and the ratio of carbon and nitrogen are very important parameters to understand the biogeochemical cycles in the ecosystem, but still, it is poorly studied and understood (Yang et al., 2010). Fartyal et al. (2022) reported that variation in species richness, distribution pattern, and regeneration potential is related to site characteristics, which is a very important aspect to check the ecosystem imbalance.
Studies on climatic and soil aspects in Q. semecarpifolia forests are lacking. In the past, few authors attempted to highlight the climate (Ralhan et al., 1982) and soil (Jha and Dimri, 1991; Thadani and Ashton, 1995; Vetaas, 1997) patterns in this forest. Climate and soil data become very important to predict the future compositional pattern of any forests when combined with phytosociological data. Keeping this in mind, authors working on Q. semecarpifolia forests have shown interest in the collection of meteorological (Tashi, 2004; Maren and Vetaas, 2007; Thakuri, 2010; Singh and Rawat, 2012; Singh et al., 2021) and soil (Kumar et al., 2004; Thakuri, 2010; Gairola et al., 2012) data in recent years. The mean annual temperature and rainfall in Q. semecarpifolia forests range between 0–30°C and 500–2500 mm, respectively (Orwa et al., 2009). However, the species prefers a mean annual rainfall of approximately 1,000–2,500 mm (CABI, 2002). It can also survive at an absolute minimum temperature of –15°C (Tashi, 2004). Singh and Rawat (2012) observed –4°C as the minimum temperature and 34°C as the maximum temperature in Q. semecarpifolia forest.
Quercus semecarpifolia generally grows in a deep fertile loamy soil with free soil drainage (Chittendon, 1951; Bean, 1981; CABI, 2002). It can grow in deep rich moist soil and poor rocky ground. For a balanced nutrient supply, the forest soils should be slightly acidic (Leskiw, 1998) with pH values between 5.5 and 7.2. The pH of Q. semecarpifolia forests has been recorded less acidic (5.8–6.87) compared to other pure forests (Gairola et al., 2012; Rawat, 2014) in the Himalayan region. It can be attributed to the high organic matter content (2.30–6.40%) and undisturbed nature of soils in other forests (Robertson and Vitousek, 1981; Adams and Sidle, 1987; Dimri et al., 1987). The pH values of Q. semecarpifolia forests are comparable with other oak forests in the Himalayan region (Table 2).
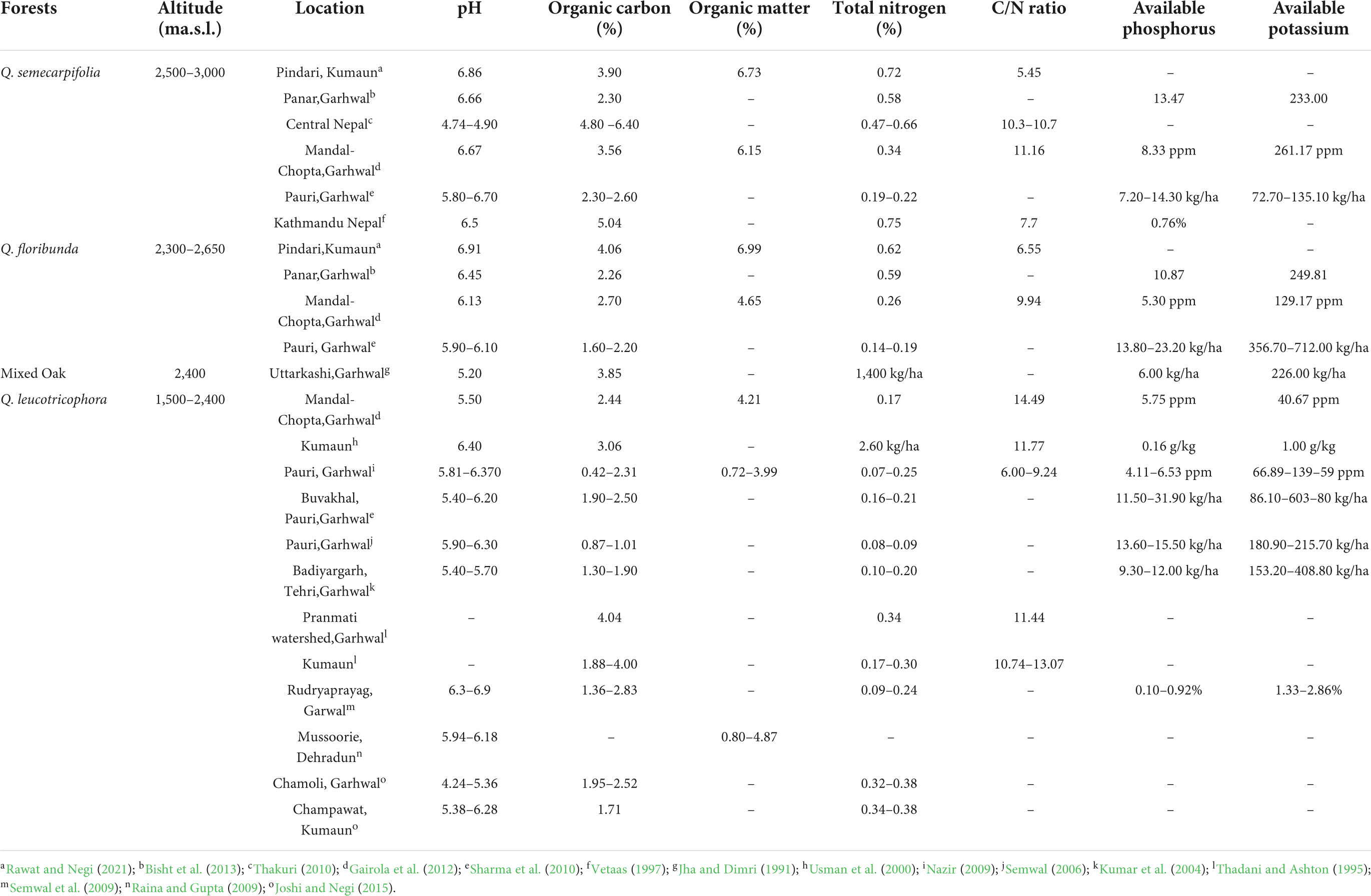
Table 2. Comparative account of chemical properties of soil in different types of Oak forests in the western Himalayas.
Distribution
Quercus semecarpifolia is a key element of central and Western Himalayan vegetation. In this region, the Q. semecarpifolia tree has existed for millions of years. This species invaded the Steppe, which was formed after the final uplift of the Himalayas and later became the dominant species of the subalpine and alpine forest (Singh and Singh, 1992). It is widely distributed in moist temperate and subalpine regions characterized by heavy snowfall and moderate rainfall, whereas it is lacking in the dry regions of the inner Himalayas (Negi and Naithani, 1995). In the Himalayas, this species is distributed in Afghanistan, China, India, Nepal, Bhutan, and Pakistan (Orwa et al., 2009). Q. semecarpifolia is a dominant species in the Himalayas, at elevations of 2,100–3,800 m asl (from southwest China to Afghanistan), occasionally descending to approximately 2,000 m (Polunin and Stainton, 1984; Shrestha, 2003; Shrestha et al., 2013). It is well distributed throughout the Himalayas from Bhutan westwards into Afghanistan, on the Myanmar–Manipur frontier, Thailand, and into China (Negi and Rawal, 2018). It is a characteristic tree of Western Himalayan upper oak/fir forest types, generally predominating on the exposed ridges. In the Western Himalayas, it is well distributed across various elevational ranges, that is, 2,000–3,500 m asl in the Western Himalayas (Vetaas, 1997, 2000; Vetaas and Chaudhary, 1998; Rawat et al., 2021a,b); 2,400–3,540 m asl in the Garhwal Himalayas (Singh et al., 1997; Sharma et al., 2016); 2,400–3,500 m asl in the Kumaun Himalayas (Saran et al., 2010) and ascending to approximately 4,000 m elevation (Tashi, 2004) on northern and southern aspects, respectively. According to Orwa et al. (2009), the suitable native range for plantation of this species is Afghanistan, China, India, and Pakistan and the exotic range includes France (Figure 1).
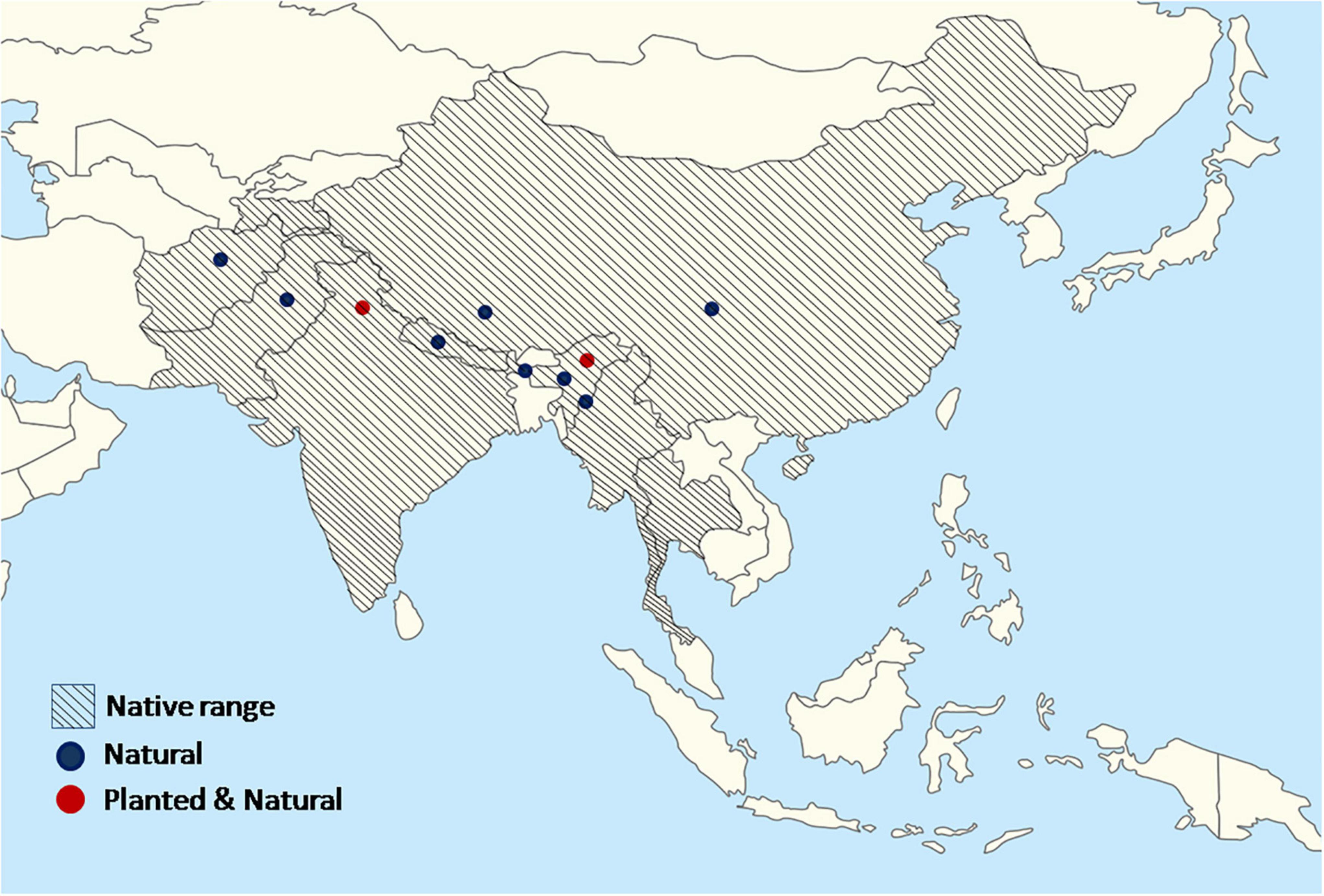
Figure 1. A map showing the countries where the species occurs naturally and has been planted. The native range does neither suggest that the species can be available in every ecological zone within that country nor that the species cannot be planted in other countries than those depicted.
Compositional patterns
Quercus semecarpifolia occurs gregariously within its marked zone, forming pure stands along the upper slopes and ridges. In the Indian Himalayan region (IHR), Q. semecarpifolia is frequently mixed with spruce (Picea smithiana), silver fir (Abies pindrow), and yew (Taxus baccata; Taxus wallichiana). In some localities, it is mixed with blue pine (Pinus wallichiana) also. Some other common broad-leaved trees associated with this are Rhododendron arboreum, Rhododendron barbatum, Q. leucotrichophora, Q. floribunda, Prunus cornuta, Cotoneaster acuminatus, Viburnum mullaha, Acer caesium, Juglans regia, Q. glauca, Betula utilis, etc. (Shrestha, 2003; Orwa et al., 2009; Thakuri, 2010; Singh et al., 2021). Shrubs are also found to be associated in the Q. semecarpifolia forest such as Rosa, Rubus, Viburnum, Lonicera, Rhododendron, Myrsine, Berberis, Daphne, and dwarf bamboo (Troup, 1921; Viswanath et al., 2002).
Recently, Fartyal et al. (2022) have reported the impact of altitudinal variation on community composition, structure, dispersion, and regeneration status of Q. semecarpifolia. They found that increasing altitude is inversely proportional to the number of tree species. Q. semecarpifolia was the dominant tree species at all the studied sites; however, the absence of seedlings indicated no regeneration or poor regeneration of the seeds (Fartyal et al., 2022). In the Q. semecarpifolia-dominated forests, tree species richness ranges between 5 and 23 species, sapling richness from 4 to 14, species and seedling richness from 6 to 16 species (Rawat, 2014; Rawat et al., 2015). Species density (ind ha–1) varies between 250–2,070 individuals ha–1 (tree), 200–1,242 individuals ha–1 (sapling), and 348–4,663 individuals ha–1 (seedling). In the case of a pure forest, the species density of Q. semecarpifolia species may reach up to 1,202 individuals ha–1 (tree), 864 individuals ha–1 (sapling), and 2,200 individuals ha–1 (seedling) (Thakuri, 2010). The details of quantitative characteristics of Q. semecarpifolia forests are presented in Table 3.
Regeneration pattern
The demographic profile, including recruitment patterns, acts as an indicator to understand the past, present, and future patterns of any forest community. The diversity and abundance of tree species at the recruitment stage provide a broader idea about the future composition of forests. Furthermore, this life stage of tree species is highly sensitive to biotic and abiotic factors, and understanding recruitment dynamics becomes an essential component of forest studies. Such studies, in particular, assume greater importance in mountain forests where lack of adequate regeneration is frequently reported as a major problem (Krauchi et al., 2000). Korner (1998) reported that the most sensitive state in a plant’s life cycle at high altitudes is the emergence and establishment of seedlings. This depends on various factors impacting directly or indirectly. For example, light and thickness of litter present on the forest floor affect the seed germination variably (Shrestha, 2003). Generally, thin litter is good for seed germination and the establishment of seedlings, whereas thick litter reduces the rate of germination. However, several workers have also reported that herbaceous cover instead of litter on the forest floor has a more adverse effect on the emergence of seedlings and their survival (Tripathi and Khan, 1990; Dzwonko and Gawronski, 2002). Recently, Tewari et al. (2019) highlighted acorn maturation and regeneration problem in Q. semecarpifolia. They found that anthropogenic pressure was very high in the form of grazing animals on the selected sites and badly affects the seedling density. Saplings were completely absent at the study sites. They reported that a reduction in moisture content is a good indicator of acorn maturity in Q. semecarpifolia. They also found that global warming would not severely affect the acorn maturation, fall, and germination of this species (Tewari et al., 2019). Aspects and habitats are responsible for the proper establishment of the species. Recently, the correlation between species abundance and aspect has been analyzed (Singh et al., 2021). In their study, the density of species in shady moist habitats in the southern aspect of subalpine zones reveals that the shady moist habitat is suitable for the germination of seeds and overall growth of seedlings and saplings in Great Himalayan National Park, India (Singh et al., 2021). Information on the above aspects from high-altitude areas in the Himalayas is relatively meager (Rawal and Pangtey, 1994a,b; Dhar et al., 1997; Joshi and Anderson, 2020). Dense growth of weeds such as Pteracanthus alatus (Wallich ex Nees) Bremek and Pteracanthus urticifolius (Kuntze) Bremek inhibit the survival of seedlings and saplings; their removal has resulted in the establishment of seedlings at previously unproductive sites (Negi and Naithani, 1995). Singh et al. (1997), based on the size and age relationship, have suggested that failure of regeneration in Q. semecarpifolia is persisting for the last 6–10 decades. They further added that proper canopy gap and exposed soil are good conditions for the regeneration of brown oak. Among the major reasons that have been suggested to explain the poor regeneration of oak forests across the globe are erratic seed production, defoliation, acorn herbivory, browsing damage to seedlings, forest fire, extensive lopping, accumulation of thick litter with slow decomposition rate, infestation by stem parasites such as mistletoe, and leaf damage by insect pests (Andersson, 1991; Lorimer et al., 1994; Bisht and Kuniyal, 2013). Poor regeneration of oak across the Himalayan region can be attributed to seed wastage due to frugivory, inadequate regeneration, and low establishment of seedlings and trampling-driven damages to seedlings caused by browsing animals (Thadani and Ashton, 1995; Tashi, 2004; Bisht et al., 2011; Singh et al., 2014; Tewari et al., 2019). Failure of regeneration of Q. semecarpifolia under its own canopy has already been studied and climate change as a possible cause has been predicted (Upreti et al., 1985). Evidence of increasing annual temperature and declining precipitation has already been reported (Yadav et al., 2014); thus, the vulnerability of Q. semecarpifolia to climate change has also been predicted (Saran et al., 2010; Bisht et al., 2013). Shrestha et al. (2012) revealed severe pressure on the survival of Q. semecarpifolia under a relatively higher rate of temperature rise in some regions of the Himalayas.
Human threat
The Himalayas is not only known for its unique plant biodiversity but also known as the home to many unique and diverse human groups. These human beings live in the river valleys and mountain slopes that differ from each other in terms of their language, culture, tradition, religion, and also resource use patterns. Large-scale human interference over the last decade and human settlements in the middle to high elevations in the Himalayas is evident for thousands of years (Shrestha et al., 2013). Due to overexploitation of these areas by tourists and local people, the forests are degrading rapidly. The increase in human population, widespread logging, overgrazing, and removal of leaf and wood litter from the forests floor are the major factors that affect the forest adversely and are responsible for forest degradation in the region (Gorrie, 1937; Chaturvedi, 1985; Singh and Singh, 1987; Tewari et al., 2019). In the Himalayan region, five distinct pastoral practices—(i) nomadic, (ii) semi-nomadic, (iii) nuclear transhumance, (iv) trans-migratory, and (v) sedentary—have been reported (Rawat, 2007). The livestock grazing and biomass collection alter the understory growth of shrubby species and regeneration of oak (Saxena et al., 1984; Upreti et al., 1985; Thadani and Ashton, 1995; Maren and Vetaas, 2007). It is estimated that 3 months of grazing by cattle can cause 75% of seedling mortality in oak forests. In many parts of the Western Himalayas, man has influenced mountain forests and tree lines as agro-pastorals uses, timberlines, and nearby alpine meadows for grazing of thousands of cattle and sheep/goats; most of the tourist routes and temples come across the tree line and people excessively use forests for burning as fuelwood and tree lines as grazing lands that severely affects the regeneration and establishment of oak seedlings (Tewari et al., 2019). Singh et al. (1997) underlined that the proper canopy gap created by forest biomass collection favors regeneration of Q. semecarpifolia but the cutting of the whole tree followed by human settlement in search of fuel and fodder collection harms regeneration. In Nepal, excessive use of fodder for livestock farming and uncontrolled grazing are among common land-use practices in middle hills (Moench and Bandyopadhyay, 1986; Springate-Baginski et al., 2003).
In the Himalayas, the main component of household economies is fuelwood. In the subalpine and alpine regions, there are limited villages; however, several pilgrimage/tourist areas are present in the region where plenty of Dhaba owners (temporary shops), shepherds, and migratory communities (viz., Gujjar) use fuelwood from the nearby forests. It has been assessed that each Dhaba owner in this region uses an average of 30 kg of fuelwood per day and gets a major portion of fuelwood from Rhododendron campanulatum, Q. semecarpifolia, and R. arboreum trees (Singh et al., 2010). Lopping for fodder, charcoal production, and fuelwood consumption have been reported as severe threats to Q. semecarpifolia forests (Shrestha et al., 2013). High disturbance leads to the invasion of alien species, which, due to high reproductive vigor and faster colonizing capacity, causes a serious threat to native vegetation (Akter and Zuberi, 2009). Aggressive invasion of Eupatorium odoratum in Q. semecarpifolia forests has been recorded from highly disturbed sites in central Himalaya, Nepal (Shrestha et al., 2013). The variety of threats to Q. semecarpifolia is well depicted in Figure 2.
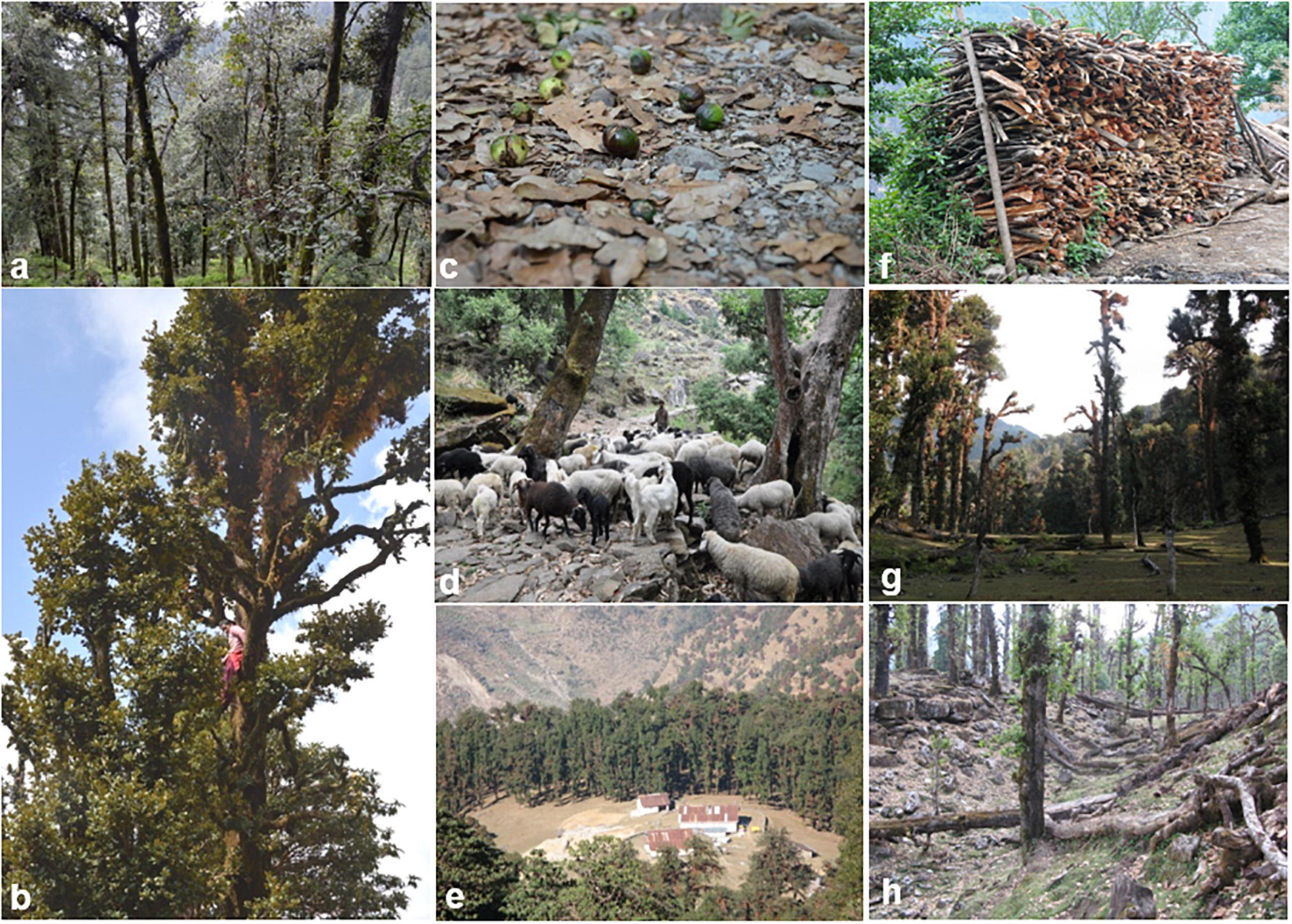
Figure 2. Status of Quercus semecarpifolia forests under different use values and disturbance regimes; (a) dense forest; (b) anthropogenic pressure (cutting and lopping); (c) seed fall before maturity; (d) grazing by animals; (e) human encroachment (Dhakuri, Uttarakhand); (f) fuel wood collection; (g) forest degradation by human interference; and (h) naturally degraded forest.
Biotechnological interventions in oak
Seed germination
Production of the plants through seed regeneration is a well-known and highly acceptable method of propagation in many plant species (Hartmann and Kester, 1990). Seeds are the best way to preserve genetic diversity. They are also a reliable source of plant production and an easy way of plant transportation (Vazquez and Rojas, 1996). Like all other plants, oaks are also naturally regenerated through seeds, although their regeneration is very poor in natural conditions (Troup, 1921). Just like other oak species, Q. semecarpifolia seeds also behave as recalcitrant seeds (Bisht, 2001). Being a recalcitrant seed, the seeds of Q. semecarpifolia are big in size, short-viable, shed at very high moisture content, and also chilling-sensitive (Bisht, 2001). Moreover, the seed germination process in these seeds is quite different from other oaks and associated species. Seeds take too much time and progress very slowly during germination. It is well known that seed germination studies are a very important and acceptable approach to understanding the seed behavior and its requirement during germination (Chauhan et al., 2006). Although several studies have been published on various aspects such as phytosociology and ecology (Bisht et al., 2013) of Q. semecarpifolia, only a few reports are available on seed germination and factors affecting it (Bisht et al., 2011). Therefore, the species needs extra attention to study the regeneration potential and factors affecting the seedling establishment in Q. semecarpifolia.
Several workers have reported very low seed regeneration rate in Q. semecarpifolia under natural conditions (Vetaas, 2000; Bisht, 2001; Shrestha, 2003; Tashi, 2004; Thakuri, 2010; Singh and Rawat, 2012; Pandey and Tamta, 2013; Amare and Bharadwaj, 2017). There are several steps in the seed germination process of Q. semecarpifolia, viz., the emergence of radicle, thickening of radical, swelling of approximately 2 cm part of radical, and then roots and shoot appear. Bisht (2001) observed that chilling treatment is required for the swollen portion of radical to initiate the emergence of seedling, but at/below 0°C seeds lose their viability due to the formation of ice crystal in the cells. Pandey and Tamta (2013) observed that pretreated seeds germinated better in comparison with control, and target species responded differently to the treatments. In another study, the canopy conditions along with litter (under the canopy and outside the canopy) have been proven responsible factors for the germination of Q. semecarpifolia seeds (Bisht et al., 2011). The freshly fallen seeds have shown better germination when grown outside canopy conditions with or without litter than those shown beneath the canopy conditions where partial light, along with very high moisture and microbial activities, influences regeneration. Bisht et al. (2013) reported that light is one of the most important factors which affects seed germination in Q. semecarpifolia. Naturally occurring subalpine zones of the Western Himalayas are typically anthropogenic zones, where natural regeneration is very low or negligible. In these conditions (in disturbed or undisturbed natural habitats), species fail to regenerate adequately due to the long history of pastoral migratory communities and recent unmanaged tourism in the Himalayan regions.
In vitro propagation
In vitro propagation is a widely used technique for large-scale production of many economically important plant species (Bhojwani et al., 1996; Ravishankar and Venkatraman, 1997). It is not only used for rapid production of the plants but also for conservation of some threatened plant species. It is also useful for the propagation of those plants whose seeds are very poor in germination in natural conditions. It has already been mentioned that the natural regeneration through seeds is poor in Q. semecarpifolia (Troup, 1921) and vegetative propagation using stem cuttings has not shown encouraging results in oaks (Chalupa, 1982; Tamta et al., 2008; Brennan et al., 2017); therefore, in vitro propagation can be a powerful means for the rapid production of this important multipurpose tree.
Although in vitro propagation has been reported for several oak species, namely, Q. robur, Q. suber, Quercus shumardii, Quercus acutissima, Quercus serrata, Quercus rubra, Q. leucotrichophora, Q. glauca, and Q. floribunda (Pardos, 1981; Vieitez et al., 1985; Bennet and Davies, 1986; Favre and Juncker, 1987; Sato et al., 1987; Chalupa, 1988; Bellarosa, 1989; Manzanera and Pardos, 1990; San-Jose et al., 1990; Schwarz and Schlarbaum, 1993; Purohit et al., 2002a,b,c; Brennan et al., 2017), a few studies are available on somatic embryogenesis and in vitro propagation of Q. semecarpifolia (Tamta et al., 2008; Tamta et al., 2009). Tamta et al. (2008) used the petiolar tube as an explant to develop an in vitro propagation protocol of Q. semecarpifolia. They used woody plant medium supplemented with 20 μM BAP for the multiplication. Tamta et al. (2009) also reported plant regeneration through somatic embryogenesis in Q. semecarpifolia.
Molecular analysis
Oak species diversity is greatly affected by dissimilar topology, unique climate, habitat, and very importantly radiation in those areas where it grows (Nixon, 1993). Several authors (Elena-Rossello et al., 1993; Kremer and Petit, 1993; Zanetto and Kremer, 1995; Bacilieri et al., 1996; Bruschi et al., 2000; Johnson et al., 2001; Dodd and Kashani, 2003) have reported the diverse morphology in oaks that also indicates the variation at the genetic level in Quercus populations (within and among). Due to this, the oak species have attracted serious attention from researchers to evaluate the genetic diversity and study the population genetic structure for the management of forest ecosystems. It has been reported that the genetic diversity in oaks (within and among populations) is directly related to life, migration history, and geoclimatic forces (Kremer et al., 2002; Petit et al., 2002; Pizzurro et al., 2010; Valero et al., 2011a,b). Paleobotanical evidence also suggests that the evolution and migration of Quercus are encouraged by variable climates (cooler and drier) that developed after the Eocene–Oligocene transition in North America. The study also suggests that intermediacy in morphology and genetic structure represents the ancestral state and apparent parent, which is the result of divergence from a common gene pool.
Identification has traditionally been based on morphological parameters like plant height, leaf length, leaf shape, young leaf type, floral characteristics, and fruit shape. Physiological markers were also used for the characterization purposes. Biochemical markers like isozymes are also used for the characterization of several plant species. Several researchers have used isozymes as a useful marker to evaluate genetic diversity, phylogenetic relations, and taxonomic and evolutionary studies in many plant populations (Hokanson et al., 1993; Finkeldey, 2001; Valero et al., 2011a). Although isozyme markers are easy to use because of their simplicity and rapidity, however, only a limited number of polymorphic loci are available for the analysis of genetic variation. The major limitation of most morphological, physiological, and biochemical markers is that these markers are influenced by the environment and affect the result. Keeping this problem in mind, scientists have developed many molecular markers like random amplified polymorphic DNAs, restriction fragment length polymorphism, inter simple sequence repeats, and simple sequence repeats. These markers are easy to use and are more reliable as they are DNA-based markers.
Molecular markers are very useful to analyze genetic variations as they can provide a large number of neutral and independent characteristics. In the field of forest genetics and tree breeding research, including genetic diversity, population structure, phylogeny, mating systems, and tree classification, use of a genetic marker is very reliable and has potential application. Random Amplified Polymorphic DNA (RAPD), Restriction Fragment Length Polymorphism (RFLP), Inter Simple Sequence Repeats (ISSR), Simple Sequence Repeats (SSR), Start Codon Targeted (SCoT) and other Polymerase Chain Reaction (PCR) restriction-based markers are being invented for this purpose. Several studies have been reported where molecular markers were used to assess the genetic diversity analysis of oak species (Schiller et al., 2003; Schiller et al., 2004a,b), but, in the case of Q. semecarpifolia, only a few studies have been reported till date (Kumar and Kumar, 2014). Many workers used RAPD and ISSR markers to evaluate genetic diversity (Williams et al., 1990). Although it is one of the most preferred DNA markers due to its greater speed, easy-to-perform, and non-requirement of radioactive materials, however, ISSR, RFLP, and SCOT are more reliable genetic markers for the study of genetic diversity. Recently, Jawarneh et al. (2013) and Kumar and Kumar (2014) have reported the genetic variation in oak.
Conservation and management
Quercus semecarpifolia forests in the Himalayas are very useful in protecting soil fertility and are intimately linked with subsistence hill agriculture, watershed, and local biodiversity. They are a good source of fodder, leaf litter, firewood, and timber (Springate-Baginski et al., 2003; Maren and Vetaas, 2007). They are also known as the lifeline of the region and are considered one of the oldest plants in the area. It is also very clear that oaks are the most overexploited species in the subalpine zones due to their multiple uses in daily life (Subedi, 2006). Other than the anthropogenic activities, bears, langurs, monkeys, Himalayan tahr, barking deer, goral, and birds devour the acorns. To date, as such, there is no such policy or plan for the conservation and long-term monitoring of Q. semecarpifolia in the IHR. However, there are four national parks (Valley of Flower, Nanda Devi, Gangotri, and Govind), three wildlife sanctuaries (Askote, Kedarnath, and Govind), and one biosphere reserve (Nanda Devi) representing 10.9% geographical area of the state, covering entirely or partially the habitat of Q. semecarpifolia forests in Uttarakhand, Western Himalayas. Except for the Valley of Flowers national park, most of them have focused attention on the conservation of faunal diversity.
In the Indian Himalayas, as much as 90–100% of the acorn crop can be destroyed by mammal herbivory (Anonymous, 1928). The acorns are readily eaten by bears, monkeys, flying squirrels, rats, and birds, especially jays and nutcrackers (Hawkins, 1986). Monkeys and birds are reported to be the most destructive to acorns (Thadani, 1999), while bears often “smash the oak trees badly” and cause extensive damage to branches and leaves (Champion, 1922). As plantation has not been successful for Q. semecarpifolia, natural regeneration plays an important role in maintaining the forests in the subalpine zone. Collective efforts are needed from researchers, management authorities, and local and migratory pastoral communities to capitalize on the mass seed production by Q. semecarpifolia to ensure the successful establishment of the regenerating individuals (Singh et al., 2011). The decline of Quercus biodiversity in nature is now a matter of global concern. Increased land colonization, changes in land use, overexploitation (deforestation and overgrazing), and climatic factors led to the destruction and extinction threat of many species of oak. Thus, efforts on restoration and rehabilitation programs to protect oak biodiversity need the attention of ecologists and policymakers. In order to achieve this, investigations on genetic variations within species and among populations of oak need to be carried out. Furthermore, a community forestry program can be implemented where local inhabitants may be essential to reduce the frequency of lopping, grazing, and cutting (Shrestha et al., 2013). Regular thinning, harvesting, and selective removal of oak to enhance seed dispersal, survival, and growth through the implementation of community forest management policy may be a major step toward the promotion of a healthy ecosystem (Singh et al., 1997; Bajracharya et al., 2005; Maren and Vetaas, 2007; Shrestha et al., 2013). Annapurna Conservation Area (ACAP) in Nepal is one of its kinds where regulated land-use practices have been proven effective in the maintenance and growth of oak forests (Maren and Vetaas, 2007). In ACAP, local communities take part in conservation planning and management by following certain cutting and lopping regulations apart from their traditional land-use practices.
Gap areas and future prospects
The extent of Quercus semecarpifolia forests
Many studies on Q. semecarpifolia so far focused on studying the structure and composition of forests, regeneration pattern, anthropogenic interventions, regeneration status, and a few biotechnological studies on seeds. However, there are limited landscape-level studies on the extent of Q. semecarpifolia. Quantitative information on the microhabitat preferences and extent of distribution is virtually lacking in the Himalayan region. Studies on this aspect of economically important species would be very useful in understanding the distribution ecology and pattern of its change across time and space.
Impact of climate change and habitat alteration
Studies on species response to climatic change demand long-term data on key ecological processes (tree growth, establishment, competition, succession), in-depth knowledge on the ecology of species, as well as of the historical factors of the studied area to show the potential impacts of climate change on the species using dynamic vegetation models that could only be possible for well-studied species and habitats. With changing socioeconomic scenarios and developmental initiatives in the subalpine and alpine regions, researchers have a great opportunity to analyze the effects of climate change on phenology, species range shifts, regeneration pattern, and habitat alternation. The vulnerability of Q. semecarpifolia to climate change has already been predicted (Saran et al., 2010). They stated that the area under Q. semecarpifolia distribution may get reduced by 40 and 76% with an expected + 1oC and + 2oC increase in temperature, respectively. Seed maturation time in some species, including Q. semecarpifolia, is synchronized with the rainy season, which, due to global warming, might get affected (early maturation of seed) and thus result in failure of regeneration (Tewari et al., 2019). However, based on results, Tewari et al. (2019) underline that the production and regeneration of Q. semecarpifolia seeds are unaffected by climate change. It is also a better time to assess the effect of climatic conditions on secondary metabolite production of Q. semecarpifolia and its associated tree species. Recently, an altitudinal shift of Q. semecarpifolia in the northwestern Himalayas has been analyzed using a spatiotemporal distribution model. This study predicts an upslope movement of Q. semecarpifolia in response to climate change where topography and climatic factors could play a significant role (Shekhar et al., 2022).
C:N ratio
Carbon (C) and nitrogen (N) are both very essential for the growth and development of plants. C:N ratio is one of the most important parameters that affect soil behavior, crop yield, and the mineralization process. The ratio of these two elements in the soil influences plant growth. Furthermore, the soil C:N ratio determines the decomposability of soil organic matter, thereby having an important impact on plant N availability. Stoichiometry of plant carbon (C) and nitrogen (N) has a fundamental role in the maintenance of ecosystem structure and function (Yuan and Chen, 2015). It can be used to predict plant growth and development by understanding the relative strength of C and N metabolism in plants (Elser et al., 2010; Luo et al., 2015). Therefore, understanding variation in plant and soil C and N relationships has been a key focus for both plant physiologists and ecologists. In the case of Q. semecarpifolia, all these parameters are still untouched or poorly understood. Studies on soil aspects in Q. semecarpifolia forests are very limited to a few localities (Singh and Negi, 2018). They observed an increasing trend of C:N ratio in Q. semecarpifolia forests across the elevation in the Tunganth region of Western Himalayas.
Factors affecting seedling establishment
The growth and sustainability of a forest community are based on its structure and composition. Regeneration is a fundamental characteristic of forest composition and structure of species. The success of regeneration is determined by the presence of adequate populations of seedlings, saplings, and mature plants (Austin, 1977). Regeneration allows the determination of the distribution of seedlings, saplings, and adults, along with analysis of size class distributions, which further helps understand changes in soil parameters (Han et al., 2011). Thus, a change in soil nutrients may lead to a change in forest composition. Regeneration, poor or good, under a canopy of mature vegetation indicates the future structure of a community (Austin, 1977). Q. semecarpifolia is a very slow-growing tree species. In several parts of the Western Himalayas, the understory of these forests is deprived of saplings and seedlings, causing a decline in population structure (Maren and Vetaas, 2007). It requires extra attention to study and determine the factors, including both external (climate, human disturbance, habitat, competition) and internal (seed viability, seed vigor, seed germination, seed mortality, genetic variability), which affect the seed germination and establishment. Singh et al. (1997) underlined that activities like “ban on cutting” contributed to the failure of regeneration in brown oak and reported very few individuals in the sapling stage under dense canopy. There is also an urgent need to study the regeneration potential of the species which will be helpful in establishing in vitro propagation protocols for rapid and large-scale multiplication of this important multipurpose tree.
Efficient regeneration approach
The unsatisfactory emergence and establishment of seedlings in the oaks under their habitat conditions are reported (Vetaas, 2000). Among major responsible factors for the failure of establishment of regeneration, massive lopping for fodder, habitat degradation, and trampling damages caused by grazers and browsers are considerable ones (Bisht and Kuniyal, 2013). Germination of seed is also affected variably by light, thickness of litter, mountain aspect, impact of weed, acorn herbivory by rodents, frugivory, damage by insects and pests, and vulnerability to climate change (Tripathi and Khan, 1990; Andersson, 1991; Lorimer et al., 1994; Negi and Naithani, 1995; Singh et al., 1997, 2021; Dzwonko and Gawronski, 2002; Shrestha, 2003; Saran et al., 2010; Bisht et al., 2013). The effect of masting of seed on the enhancement of regeneration has already been investigated (Singh et al., 2011). However, studies on regeneration establishment on Q. semecarpifolia are still lacking from the Himalayan region. Planting healthy and well-grown seedlings in the field is essential for the success of a forestry program. Such seedlings can be grown in the nurseries by the stakeholders themselves who want to establish an extension forestry system. Successful seed germination (50–80%) of Q. semecarpifolia in the nursery located in or around mother forests has already been achieved in Himachal Pradesh (Lalhal et al., 1996) and Uttarakhand (Bisht et al., 2011) when grown in different environmental conditions. In other locations, direct seeding has been a primary method used to regenerate oak on thousands of hectares in the LMAV (Schoenholtz et al., 2005). The inefficiency of pre-sowing treatments and laboratory-based mass propagation, nursery-raised seedling, and direct sowing can be the best approach for the extension of the oak forests in the Himalayan region and the continuous supply of essential resources to the dependent communities. Proper canopy gap by the collection of fuelwood and fodder in a regular interval may lead to successful regeneration (Singh et al., 1997).
Effective conservation approach
Being substantially useful for Himalayan ecosystems, including local inhabitants, Q. semecarpifolia deserves an effective conservation approach for its future existence. Legally forced conservation approach in natural forests may not result in success stories until local inhabitants are convinced or satisfied; however, it turns into big conflicts. Reaching a mutually agreeable solution by understanding each other’s needs may be one good way of conflict resolution and development of policy for conservation (Lewis, 1996). Among suggested many conservation initiatives, community-based forestry programs have been proven the most effective (Bajracharya et al., 2005; Maren and Vetaas, 2007; Shrestha et al., 2013). Replication of the ACAP model in Himalayan forests seems a better conservation and management approach at present. Besides, natural regeneration plays an important role in maintaining forests especially when plantation has not been proven successful for this species (Singh et al., 2011). To ensure successful establishment of the regenerating individuals, efforts are required from different groups of society such as researchers, villagers, management authorities, policymakers, and migratory pastoral communities to capitalize on the mass seed production by Q. semecarpifolia. Furthermore, the identification of suitable habitat and aspects followed by a proper canopy gap may enhance generation establishment (Singh et al., 1997, 2021). Proper monitoring and conservation of such sites may lead to a sustainable future for these forest communities in the IHR. Restoration of exploited forest areas by allowing them gradually to return to a near-natural state through non-intervention has already been proven (Hanski, 2003; Bernes, 2011).
Data availability statement
The original contributions presented in this study are included in the article/supplementary material, further inquiries can be directed to the corresponding authors.
Author contributions
BR, JR, and SP: conception, data collection, and redaction. GS, PS, AC, JS, DV, MJ, and KQ: critical revision from draft to version to be published. All authors reviewed and approved the final version of the manuscript.
Funding
The article processing charges were funded by the King Abdullah University of Science and Technology (KAUST), Thuwal, Saudi Arabia. Uarakhand Biotechnology Council (UCB/R&D-Project/2018/319), Government of Uttarakhand is acknowledged for the project support for this study.
Conflict of interest
The authors declare that the research was conducted in the absence of any commercial or financial relationships that could be construed as a potential conflict of interest.
Publisher’s note
All claims expressed in this article are solely those of the authors and do not necessarily represent those of their affiliated organizations, or those of the publisher, the editors and the reviewers. Any product that may be evaluated in this article, or claim that may be made by its manufacturer, is not guaranteed or endorsed by the publisher.
Footnotes
- ^ https://webofknowledge.com
- ^ www.sciencedirect.com
- ^ https://www.scopus.com
- ^ https://pubmed.ncbi.nlm.nih.gov/
- ^ https://www.nlm.nih.gov/
References
Adams, P. W., and Sidle, R. C. (1987). Soil conditions in three recent landslides in southeast Alaska. Forest Ecol. Manage. 18, 93–102. doi: 10.1016/0378-1127(87)90136-8
Adhikari, B. S., Rawat, Y. S., and Singh, S. P. (1995). Structure and function of high altitude forests of Central Himalaya I. Dry matter dynamics. Ann. Bot. 72, 237–248.
Akter, A., and Zuberi, M. I. (2009). Invasive alien species in Northern Bangladesh: Identification, inventory and impacts. Int. J. Biodivers. Conserv. 1, 129–134.
Amare, T., and Bharadwaj, D. R. (2017). Germination behavior and biochemical contents of Querecus glauca Thunb. Acorns in relation to pre-showing treatments and acorn sizes. Indian Forester. 143, 43–49.
Andersson, C. (1991). Distribution of seedlings and saplings of Quercus robur in a grazed deciduous forest. J. Veg. Sci. 2, 279–282. doi: 10.2307/3235960
Austin, M. P. (1977). Use of ordination and other multivariate descriptive methods to study succession. Vegetatio 35, 165–175. doi: 10.1007/BF02097067
Baduni, N. P., and Sharma, C. M. (1996). Effect of aspect on the structure of some natural stands of Quercus semecarpifolia in Himalayan moist temperate forest. Indian J. For. 19, 335–341.
Bacilieri, R., Ducousso, A., Petit, R., and Kremer, A. (1996). Mating system and asymmetric hybridization in a mixed stand of European oaks. Evolution 50, 900–908. doi: 10.1111/j.1558-5646.1996.tb03898.x
Bajracharya, S. B., Furley, P. A., and Newton, A. C. (2005). Effectiveness of community involvement in delivering conservation benefits to the Annapurna Conservation Area. Nepal. Environ. Conserv. 32, 1–9. doi: 10.1017/S0376892905002298
Bankoti, N. S. (1990). Woody vegetation along elevational gradient (2000–3600 m) of upper Pindari catchment (Kumaun Himalaya). Ph.D. Thesis. Nainital: Kumaun Unversity.
Bean, W. (1981). “Trees and Shrubs Hardy in Great British Isles,” in Sir george taylor, Vol. 4, ed. D. L. Clarke (New York: St. Martin’s Press).
Bellarosa, R. (1989). “Oak (Quercus spp),” in Biotechnology in agriculture and forestry, Vol. 5, ed. Y. P. S. Bajaj (Berlin: Springer), 387–401. doi: 10.1007/978-3-642-61535-1_20
Bennet, L. K., and Davies, F. T. (1986). In vitro propagation of Quercus shumardii seedlings. HortScience 21, 1045–1047.
Bernes, C. (2011). Biodiversity in Sweden. Monitor 22. Stockholm: Swedish Environmental Protection Agency.
Bhojwani, S. S., Razdan, M. K., and Davies, F. T. Jr. (eds) (1996). “Clonal propagation,” in Plant tissue culture: Theory and practice, ed. L. K. Bennett (Amsterdam: Elsevier), 483–536. doi: 10.1016/S0928-3420(96)80018-8
Bisht, H. (2001). Physiobiochemical aspects of seed viability in Quercus semecarpifolia Smith.: A possible recalcitrant seed. PhD Thesis. Srinagar: HNB Garhwal University, 45–48.
Bisht, H., Prakash, V., and Nautiyal, A. R. (2011). Conservation strategies for the threatened Quercus semecarpifolia Sm. a Himalayan timberline oak. Int. J. Conserv. Sci. 2, 275–384.
Bisht, V. K., and Kuniyal, C. P. (2013). Climate change matters because the oaks cannot move upward. Curr. Sci. 104, 689–690.
Bisht, V. K., Kuniyal, C. P., Nautiyal, B. P., and Prasad, P. (2013). Spatial distribution and regeneration of Quercus semecarpifolia and Quercus floribunda in a subalpine forest of western Himalaya. India. Physiol. Mol. Biol. Plants 19, 443–448. doi: 10.1007/s12298-013-0189-z
Brennan, A. N., Pence, V. C., Taylor, M. D., Trader, B. W., and Westwood, M. (2017). Tissue culture using mature material for the conservation of oaks. Horrtechnology 27, 644–649. doi: 10.21273/HORTTECH03801-17
Bruschi, P., Vendramin, G., Bussotti, F., and Grossoni, P. (2000). Morphological and molecular differentiation between Quercus petraea (Matt.) Liebl. and Quercus pubescens Wild. (Fagaceae) in Northern and Central Italy. Ann. Bot. 85, 325–333. doi: 10.1006/anbo.1999.1046
Chalupa, V. (1988). Large scale micropropagation of Quercus robur L. using adenine type cytokinins and thiadiazuron to stimulate shoot prolification. Biol. Plant. 306, 414–421. doi: 10.1007/BF02890509
Champion, H. G. (1922). An investigation of certain factors concerning the resin-tapping industry in Pinus longifolia. Ind. For. Bull. 51:20.
Chaturvedi, A. N. (1985). “Fuel and fodder trees for man-made forests in hills,” in Environmental regeneration in Himalaya: Concepts and strategies, ed. J. S. Singh (Nainital: Central Himalayan Environment Association and GyanodayaPrakashan).
Chauhan, B. S., Gill, G., and Preston, C. (2006). African mustard (Brassica tournefortii) germination in southern Australia. Weed Sci. 54, 891–897. doi: 10.1614/WS-06-053R.1
Chittendon, F. J. (1951). The royal horticultural society dictionary of gardening: A practical and scientific encyclopaedia of horticulture, Vol. I - IV, Plus Supplement. Oxford: Oxford University Press.
Dhar, U., Rawal, R. S., and Samant, S. S. (1997). Structural diversity and representativeness of forest vegetation in a protected area of Kumaun Himalaya, India: Implications for conservation. Biodivers. Conserv. 6, 1045–1062. doi: 10.1023/A:1018375932740
Dimri, B. M., Singh, S. B., Baneriee, S. K., and Singh, B. (1987). Relation of age and dominance of tree species with soil chemical attributes in Kalimpong and Kurseong District of West Bengal. Indian For. 113, 307–311.
Dobremez, J. F. (1976). Le nepal: Ecologie et biogeographie. Paris: Centre National de la Rechereche Scientifique.
Dodd, R., and Kashani, N. (2003). Molecular differentiation and diversity among the California red oaks section Lobatae. Theor. Appl. Genet. 107, 884–892. doi: 10.1007/s00122-003-1290-4
Dzwonko, Z., and Gawronski, S. (2002). Influence of litter and weather on seedling recruitment in a mixed oak pine woodland. Ann. Bot. 90, 245–251. doi: 10.1093/aob/mcf178
Elena-Rossello, J., de Rio, J., Garcia Valdecantos, J., and Santamaria, I. (1993). Ecological aspects of the floral phenology of the cork-oak (Q. suber L): Why do annual and biennial biotypes appear? Ann. Forest Sci. 50, 114–121. doi: 10.1051/forest:19930710
Elser, J. J., Fagan, W. F., Kerkhoff, A. J., Swenson, N. G., and Enquist, B. J. (2010). Biological stoichiometry of plant production: Metabolism, scaling and ecological response to global change. New Phytol. 186, 593–608. doi: 10.1111/j.1469-8137.2010.03214.x
Fartyal, A., Khatri, K., Bargali, K., and Bargali, S. S. (2022). Altitudinal variation in plant community, population structure and carbon stock of Quercus semecarpifoila Sm. forest in Kumaun Himalayas. J. Environ. Biol. 43, 133–146. doi: 10.22438/jeb/43/1/MRN-2003
Favre, J. M., and Juncker, B. (1987). In vitro growth of buds taken from seedlings and adult plant material in Quercus robur L. Plant Cell Tissue Organ Cult. 8, 49–60. doi: 10.1007/BF00040732
Finkeldey, R. (2001). Genetic variation of oaks (Quercus Spp.) in Switzerland allelic diversity and differentiation at isozyme gene loci. Forest Genet. 8, 185–195.
Gairola, S., Sharma, C. M., Ghildiyal, S. K., and Suyal, S. (2011). Live tree biomass and carbon variation along an altitudinal gradient in moist temperate valley slopes of the Garhwal Himalaya (India). Curr. Sci. 100, 1862–1870.
Gairola, S., Sharma, C. M., Ghildiyal, S. K., and Suyal, S. (2012). Chemical properties of soils in relation to forest composition in moist temperate valley slopes of Garhwal Himalaya. India. Environ. 32, 512–523. doi: 10.1007/s10669-012-9420-7
Gamble, J. S. (1972). A manual of indian timbers. Dehradun: Bishen Singh Mahendra Pal Singh, 1–168. doi: 10.5962/bhl.title.44593
Giri, A., and Katzensteiner, K. (2013). Anthropogenic disturbances on the regeneration of tree species in the mixed broad leaved forest of Himalayan region, Nepal. J. Nat. Hist. Mus. 27, 35–44. doi: 10.3126/jnhm.v27i0.14151
Han, W. X., Fang, J. Y., Reich, P. B., Ian Woodward, F., and Wang, Z. H. (2011). Biogeography and variability of eleven mineral elements in plant leaves across gradients of climate, soil and plant functional type in China. Ecol. Lett. 14, 788–796. doi: 10.1111/j.1461-0248.2011.01641.x
Hanski, I. (2003). Extinction debt and species credit in boreal forests: Modelling the consequences of different approaches to biodiversity conservation. Ann. Zool. Fennici 37, 271–280.
Hartmann, H. T., and Kester, D. E. (1990). Plant propagation: Principles and Practices. Hoboken, NJ: Prentice-Hall.
Hawkins, R. E. (1986). Encyclopedia of Indian Natural History. Delhi: Bombay Natural History Society- Oxford University Press.
Hokanson, S. C., Isebrands, J. C., Jensen, R. J., and Hancock, J. F. (1993). Isozyme analysis in oaks of the Apostle island in Wisconsin: Genetic structure and levels of inbreeding in Quercus rubra and Q. ellipsoidsalis. Am. J. Bot. 80, 1349–1357. doi: 10.1002/j.1537-2197.1993.tb15374.x
Jawarneh, M. S., Barke, M. H., Muhaidat, R., Migdadi, H. M., Lahham, J. N., and El-Oqlah, A. (2013). Characterization of Quercus species distributed in Jordan using morphological and molecular markers. Afr. J. Biotechnol. 12, 1326–1334.
Jha, M. N., and Dimri, B. M. (1991). Soil nutrients and forest productivity in four natural stands. J. Indian Soc. Soil Sci. 39, 735–738.
Johnson, P., Shifley, S., and Rogers, R. (2001). The ecological and silviculture of oaks. New York, NY: CABI publishing. doi: 10.1079/9780851995700.0000
Joshi, G., and Negi, G. C. S. (2015). Physico-chemical properties along soil profiles of two dominant forest types in Western Himalaya. Curr. Sci. 109, 798–803.
Joshi, H. C., and Samant, S. S. (2014). Change in structural and compositional diversity with altitude: A study from Nanda Devi Biosphere Reserve (NDBR). West Himalaya, India. Indian J. Forestr. 37, 121–136. doi: 10.54207/bsmps1000-2014-KM91X3
Joshi, S. C., and Anderson, O. R. (2000). Poor regeneration of Brown Oak (Quercus semecarpifolia Sm.) in high altitudes: A case study from Tungnath, Western Himalaya. Int. J. Biodivers. Conserv. 12, 137–141. doi: 10.5897/IJBC2020.1409
Joshi, S. C., and Anderson, O. R. (2020). Poor regeneration of Brown Oak (Quercus semecarpifolia Sm.) in high altitudes: A case study from Tungnath, Western Himalaya. Int. J. Biodivers. Conserv. 12, 137–141.
Korner, C. (1998). A re-assessment of high elevation tree line positions and their explanation. Oecologia 115, 445–459. doi: 10.1007/s004420050540
Krauchi, N., Brang, P., and Schönenberger, W. (2000). Forests of mountainous regions: Gaps in knowledge and research needs. For. Ecol. Manage. 132, 73–82. doi: 10.1016/S0378-1127(00)00382-0
Kremer, A., and Petit, R. (1993). Gene diversity in natural populations of oaks species. Ann. For. Sci. 50, 186–202. doi: 10.1051/forest:19930717
Kremer, A., Kleinschmit, J., Cottrell, J., Cundall, E., Deans, J., Ducousso, A., et al. (2002). Is there a correlation between chloroplasticand nuclear divergence, or what are the roles of history and selection on genetic diversity in European oaks. For. Ecol. Manage. 156, 75–87. doi: 10.1016/S0378-1127(01)00635-1
Kumar, M., Rajwar, G. S., and Sharma, C. M. (2004). Physico-Chemical properties of forest soils along altitudinal gradient in the Garhwal Himalayas. J. Hill Res. 17, 60–64.
Kumar, S., and Kumar, S. S. (2014). Studies on molecular marker based genetic diversity in Quercus species of Nainital, Uttarakhand. Int. J. Latest Res. Sci. Technol. 3, 106–110.
Lalhal, J. S., Jishtu, V., and Singh, O. (1996). Seed collection and nursery emergence in Quercus semecarpifolia (Smith). Indian For. 122, 85–86.
Leskiw, L. A. (1998). Land capability classification for forest ecosystem in the oil stands region. Edmonton: Alberia Environmental Protection.
Lorimer, C. G., Chapman, W., and Lambert, W. D. (1994). Tall understory vegetation as a factor in the poor development of oak seedlings beneath mature stands. J. Ecol. 82, 227–237. doi: 10.2307/2261291
Luo, W., Elser, J. J., Lü, X. T., Wang, Z., Bai, E., Yan, C., et al. (2015). Plant nutrients do not covary with soil nutrients under changing climatic conditions. Glob. Biogeochem. Cycle 29, 1298–1308. doi: 10.1002/2015GB005089
Manzanera, J. A., and Pardos, J. A. (1990). Micropropagation of juvenile and adult Quercus suber L. Plant Cell Tissue Organ. Cult. 21, 1–8.
Maren, I. E., and Vetaas, O. R. (2007). Does regulated land use allow regeneration of keystone forest species in the Annapurna Conservation Area, Central Himalaya. Mt. Res. Dev. 27, 345–351. doi: 10.1659/mrd.0893
Moench, M., and Bandyopadhyay, J. (1986). People-forest interactions: A neglected parameter in Himalayan forest management. Mt. Res. Dev. 6, 3–16. doi: 10.2307/3673336
Nazir, T. (2009). Estimation of site quality of important temperate forest cover on the basis of soil nutrient and growing stock in Garhwal Himalaya. Ph.D. Thesis. Srinagar: HNB Garhwal University.
Negi, M., and Rawal, R. S. (2018). “Querecus semecarpifolia,” in Species spot light. International Oak Socity (Petersburg, US).
Negi, S. S., and Naithani, H. B. (1995). Oaks of India, Nepal and Bhutan. Dehra Dun: International Book Distributors.
Negi, V. S., Giri, L., and Sekar, K. C. (2018). Floristic diversity, community composition and structure in Nanda Devi National Park after prohibition of human activities, Western Himalaya, India. Curr. Sci. 115, 1056–1064. doi: 10.18520/cs/v115/i6/1056-1064
Nixon, K. C. (1993). Infrageneric classification of Quercus (Fagaceae) and typification of sectional names. Annales des sciences forestières. INRA/EDP Sci. 50, 25s–34s. doi: 10.1051/forest:19930701
Orwa, C., Mutua, A., Kindt, R., Jamnadass, R., and Anthony, S. (2009). Agroforestree database: A tree reference and selection guide version 4.0. Nairobi: World Agro forestry Centre.
Pandey, A., and S, Tamta. (2013). Effect of pre-showing treatments on seed germination in Querecus serrata Thunb. and Q. semecarpifolia Sm. (2014). Int. J. Biodivers. Sci. Manage. 5, 791–795.
Pardos, J. A. (1981). “In vitro plant formation from stem pieces of Q. suber L,” in Coll Int Sur La Culture in vitro des essences Forestiéres, ed. Afocel (France: IUFRO Fontainebleau), 186–190.
Petit, R., Latouche-Halle, C., Pemonge, M., and Kremer, A. (2002). Chloroplast DNA variation of oaks in France and the influence of forest fragmentation on genetic diversity. For. Ecol. Manage. 156, 115–129. doi: 10.1016/S0378-1127(01)00638-7
Pizzurro, G. M., Maetzke, F., and La Mela Veca, D. S. (2010). Differences of raw cork quality in productive cork oak woods in Sicily in relation to stand density. For. Ecol. Manage. 260, 923–929. doi: 10.1016/j.foreco.2010.06.013
Purohit, V. K., Palni, L. M. S., Nandi, S. K., and Rikhari, H. C. (2002a). In vitro plant regeneration through cotyledonary nodes of Quercus floribunda Lindl. ex A. Camus (Tilonj oak), a high value tree species of central Himalaya. Curr. Sci. 833, 101–104.
Purohit, V. K., Palni, L. M. S., Nandi, S. K., Vyas, P., and Tamta, S. (2002b). “Somatic embryogenesis and regeneration in Quercus floribunda: An important central Himalayan Oak,” in Role of plant tissue culture in biodiversity conservation and economic development, eds S. K. Nandi, L. M. S. Palni, and A. Kumar (Nainital: Gyanodaya Prakashan), 41–52.
Purohit, V. K., Tamata, S., Chandra, S., Vyas, P., Palni, L. M. S., and Nandi, S. K. (2002c). In vitro multiplication of Quercus leucotrichophora and Q. glauca: Important Himalayan Oaks. Plant Cell Tissue Organ Cult. 69, 121–133. doi: 10.1023/A:1015296713243
Rai, I. D., Adhikari, B. S., Rawat, G. S., and Kiran, B. (2012). Community structure along timberline ecotone in relation to micro-topography and disturbances in Western Himalaya. Not. Sci. Biol. 4, 41–52.
Raina, A. K., and Gupta, M. K. (2009). Soil and vegetation studies in relation to parent material of Garhwal Himalaya. Uttarakhand (India). Ann. For. 17, 71–82.
Raju, J., Sahoo, B., Chandrakar, A., and Pandy, A. B. (2015). Effect of feeding oak leaves (Quercus semecarpifolia vs Quercus leucotricophora) on nutrient utilization, growth performance and gastro intestinal nematodes of goats in temperate sub Himalayas. Small Rumin. Res. 125, 1–9. doi: 10.1016/j.smallrumres.2014.12.013
Raju, J., Sahoo, B., Chandrakar, A., Garg, A. K., and Mohanta, R. K. (2018). Effect of Varied sources of tannin on micro-mineral bioavailability in goats fed oak leaves based diets. Anim. Nutr. Feed Technol. 18, 25–35. doi: 10.5958/0974-181X.2018.00003.3
Ralhan, P. K., Saxena, A. K., and Singh, J. S. (1982). Analysis of forest vegetation at and around Nainital in Kumaun Himalaya. Proc. Natl. Sci. Acad. B 48, 122–138.
Ram, J., Kumar, A., and Bhatt, J. (2004). Plant diversity in six forest types of Uttaranchal, Central Himalaya, India. Curr. Sci. 86, 975–978.
Ravishankar, G. A., and Venkatraman, L. V. (1997). Biotechnological applications of plant tissue culture and cell culture. New Delhi: Oxford and IBH Publishing Co, 440.
Rawal, R. S., and Pangtey, Y. P. S. (1994a). Distribution and structural-functional attributes of trees in the high altitude zone of central Himalaya. India. Vegetatio 112, 29–34. doi: 10.1007/BF00045097
Rawal, R. S., and Pangtey, Y. P. S. (1994b). “High altitude forests with special reference to timber line in Kumaun, central Himalaya,” in High altitudes of the himalaya, eds Y. P. S. Pangtey and R. S. Rawal (Nainital: Gyanodaya Prakashan), 353–399.
Rawal, R. S., Gairola, S., and Dhar, U. (2012). Effects of disturbance intensities on vegetation patterns in Oak forests of Kumaun, west Himalaya. J. Mt. Sci. 9, 157–165.
Rawat, B. (2014). Changes in vegetation diversity and plant responses in Nanda Devi Biosphere Reserve over the last two decades. PhD thesis. Nainital: Kumaun University, 182.
Rawat, B. (2021). Assessing species abundance and habitat preferences in a part of nanda devi biosphere reserve, western Himalaya. Vegetos 34, 413–421. doi: 10.1007/s42535-021-00212-2
Rawat, B., and Negi, A. S. (2021). Plant diversity patterns along environmental gradients in nanda devi biosphere reserve. West Himalaya. Trop. Ecol. 62, 61–70. doi: 10.1007/s42965-020-00122-5
Rawat, B., Gaira, K. S., Gairola, S., Tewari, L. M., and Rawal, R. S. (2021a). Spatial prediction of plant species richness and density in high-altitude forests of Indian west Himalaya. Trees For. People 6:100132. doi: 10.1016/j.tfp.2021.100132
Rawat, B., Gairola, S., and Rawal, R. S. (2015). Assessing conservation values of forest communities in nanda devi biosphere reserve: Plant diversity, species distribution and endemicity. J. Mt. Sci. 12, 878–899. doi: 10.1007/s11629-014-3000-x
Rawat, B., Gairola, S., Tewari, L. M., and Rawal, R. S. (2021b). Long-term forest vegetation dynamics in nanda devi biosphere reserve, Indian west Himalaya: Evidence from repeat studies on compositional patterns. Environ. Monit. Assess. 193:459. doi: 10.1007/s10661-021-09227-3
Rawat, G. S. (2007). Pastoral practices, wild mammals and conservation status of alpine meadows in Western Himalaya. J. Bombay Nat. Hist. Soc. 104, 251–257.
Rikhari, H. C., Adhikari, B. S., and Rawat, Y. S. (1997). Woody species composition of temperate forests along an elevational gradient in Indian central Himalaya. J. Trop. For. Sci. 10, 197–211.
Robertson, G. P., and Vitousek, P. M. (1981). Nitrification in primary and secondary succession. Ecology 62, 376–386. doi: 10.2307/1936712
San-Jose, M. C., Vieitez, A. M., and Ballester, A. (1990). Clonal propagation of juvenile and adult trees of sessile oak by tissue culture technique. Silvac. Gen. 39, 50–55.
Saran, S., Joshi, R., Sharma, S., Padalia, H., and Dadhwal, V. K. (2010). Geospatial modeling of Brown Oak (Quercus semecarpifolia) habitats in the Kumaun Himalaya under climate change scenario. J. Indian Soc. Remote Sens. 38, 535–547. doi: 10.1007/s12524-010-0038-2
Sato, T., Mori, N., and Saito, A. (1987). In vitro plantlet propagation from epicotyls segments of young seedlings of kunugi (Quercus acutissima). J. Jpn. For. Soc. 69, 113–117.
Saxena, A. K., Singh, S. P., and Singh, J. S. (1984). Population structure of forest of Kumaun Himalaya: Implication for management. J. Environ. Manage. 19, 307–324.
Schiller, G., Shklar, G., and Koral, L. (2003). Genetic diversity assessment by random amplified polymorphic DNA of oaks in Israel.1. Tabor oak (Quercus aegilops L. ssp. ithaburensis [Decne] Boiss.). Isr. J. Plant Sci. 51, 1–10. doi: 10.1560/4G87-1U7A-9D7V-HDH0
Schiller, G., Shklar, G., and Korol, L. (2004a). Genetic diversity assessment by random amplified polymorphic DNA of oaks in Israel. 2. Quercus boissieri Reut. Isr. J. Plant Sci. 52, 315–322. doi: 10.1560/XMP3-XK58-XY9R-6E2W
Schiller, G., Shklar, G., Ungar, D., Omari, A., Zyadin, F., and Korol, L. (2004b). Genetic diversity assessment by random amplified polymorphic DNA of oaks: 3. Quercus calliprinos Webb. in Israel and Jordan. Isr. J. Plant Sci. 54, 137–148. doi: 10.1560/IJPS_54_2_137
Schoenholtz, S. H., Stanturf, J. A., Allen, J. A., and Schweitzer, C. J. (2005). “Afforestation of agricultural lands in the lower mississippi alluvial valley: The state of our understanding,” in Proceedings of the Ecology and management of bottomland hardwood systems symposium, eds L. H. Fredrickson, S. L. King, and R. M. Kaminski (Puxico, MO: Gaylord Memorial Lab), 413–431.
Schwarz, Q. J., and Schlarbaum, S. E. (1993). Axillary bud proliferation of two American oak species: Quercus alba and Quercus rubra. Ann. For. 150, 340–343. doi: 10.1051/forest:19930737
Semwal, D. P., Uniyal, P. L., Bahuguna, Y. M., and Bhatt, A. B. (2009). Soil nutrient storage under different forest types in a part of Central Himalayas. India. Ann. For. 17, 43–52.
Semwal, S. (2006). Studies on phytosociology, diversity patterns and competition along an altitudinal gradient in a part of lesser Himalaya in Garhwal, Uttaranchal. D.Phil. thesis. Srinagar: HNB Garhwal University.
Sharma, C. M., Baduni, N. P., Gairola, S., Ghildiyal, S. K., and Suyal, S. (2010). Tree diversity and carbon stocks of some major forest types of Garhwal Himalaya. India. For. Ecol. Manage. 60, 2170–2179. doi: 10.1016/j.foreco.2010.09.014
Sharma, C. M., Tiwari, O. P., Rana, Y. S., Krishan, R., and Mishra, A. K. (2016). Plant diversity, tree regeneration, biomass production and carbon storage in different oak forests on ridge tops of Garhwal Himalaya. J. For. Environ. Sci. 32, 329–343. doi: 10.7747/JFES.2016.32.4.329
Shekhar, C., Ginwal, H. S., Bhandari, M. S., and Barthwal, S. (2021). Quantification and diminution of Quercus semecarpifolia forest ecosystem services in Himalayan region-An overview. Int. J. Agric. Environ. Biotechnol. 14, 83–87. doi: 10.30954/0974-1712.01.2021.9
Shekhar, C., Ginwal, H. S., Meena, R. K., Shankhwar, R., Martins-Ferreira, M. A. C., Pandey, S., et al. (2022). Spatio-temporal distribution of broad-leaved Quercus semecarpifolia indicates altitudinal shift in northwestern Himalayas. Plant Ecol. 223, 671–697. doi: 10.1007/s11258-022-01240-x
Shenbin, S., Yunfeng, L., and Thomas, A. (2006). Climatic change on the Tibetan Plateau: Potential evapotranspiration trends from 1961–2000. Clim. Change 76, 291–319. doi: 10.1007/s10584-006-9080-z
Shrestha, B. B. (2003). Quercus semecarpifolia Sm. in the Himalayan region: Ecology, exploitation and threats. Himalayan J. Sci. 1, 126–128. doi: 10.3126/hjs.v1i2.212
Shrestha, B. B., and Lekhak, H. D. (2002). Vanishing oak: Shivapuri national park. Sunday Post 10:5.
Shrestha, B. K., Maren, I. E., Arneberg, E., Sah, J. P., and Vetaas, O. R. (2013). Effect of anthropogenic disturbance on plant species diversity in oak forests in Nepal. Central Himalaya. Int. J. Biodivers. Sci. Ecosyst. Serv. Manage. 9, 21–29. doi: 10.1080/21513732.2012.749303
Shrestha, R. K., and Paudel, K. C. (1996). “Oak forest under threat: An urgent concern for the mountain environment,” in Environment and biodiversity: In the context of South Asia, eds P. K. Jha, G. P. S. Ghimire, S. B. Karmacharya, S. R. Baral, and P. Lacoul (Kathmandu: ECOS), 114–119.
Shrestha, U. B., Gautam, S., and Bawa, K. S. (2012). Widespread climate change in the Himalayas and associated changes in the local ecosystems. PLoS One 7:e36741. doi: 10.1371/journal.pone.0036741
Singh, A. K., Parsad, A., and Singh, B. (1986). Availability of phosphorus and potassium and its relationship with physico-chemical properties of some forest soils of Pali-range (Shahodol, M.P.). Indian For. 112, 1094–1104.
Singh, A., Samant, S. S., and Naithani, S. (2021). Population ecology and habitat suitability modelling of Querecus semecarpifolia Sm. in the sub-alpine ecosystem of Great Himalyan national park. North-western Himalaya, India. S. Afr. J. Bot. 141, 158–170. doi: 10.1016/j.sajb.2021.04.022
Singh, B., and Todaria, N. P. (2012). Nutrients composition changes in leaves of Quercus semecarpifolia at different seasons and altitudes. Ann. For. Res. 55, 189–196.
Singh, G., and Rawat, G. S. (2012). “Depletion of Oak (Quercus spp.) forests in the Western Himalaya: Grazing, fuelwood and fodder collection,” in Global perspectives on sustainable forest management, ed. C. A. Okia (Crotia: Intech publisher), 29–42. doi: 10.5772/33554
Singh, G., Rai, I. D., and Rawat, G. S. (2011). Year 2010 was “mast seed year” for the Kharsu oak (Quercus semecarpifolia) in the Western Himalaya. Curr. Sci. 100:1275.
Singh, G., Rawat, G. S., and Verma, D. (2010). Comparative study of fuelwood consumption by villagers and seasonal “Dhaba owners” in the tourist affected regions of Garhwal Himalaya. India. Energy Policy 38, 1895–1899. doi: 10.1016/j.enpol.2009.11.069
Singh, J. S., and Singh, S. P. (1987). Forest vegetation of the Himalaya. Bot. Rev. 53, 80–192. doi: 10.1007/BF02858183
Singh, J. S., and Singh, S. P. (1992). Forests of Himalaya: Structure, functioning and impact of man. Nainital: Gyanodaya Prakashan.
Singh, P., and Negi, G. C. S. (2018). Treeline species phenology: Shoot growth, leaf characteristics and nutrient dynamics. Trop. Ecol. 59, 297–311.
Singh, P., Verma, A. K., Pathak, N. N., and Biswas, J. (1998). Nutritive value of oak (Quercus semecarpifolia) leaves in Pashmina kids. Anim. Feed Sci. Technol. 72, 183–187. doi: 10.1016/S0377-8401(97)00174-0
Singh, S. K., and Rawat, G. S. (2000). Flora of great Himalayan national park: Himachal Pradesh. Dehradun: Bishen singh and Mahendra Pal Singh.
Singh, S. P. (2014). Attributes of Himalayan forest ecosystems: They are not temperate forests. Proc. Indian Natl. Sci. Acad. U.S.A. 80, 221–223. doi: 10.16943/ptinsa/2014/v80i2/55103
Singh, S. P., Adhikari, B. S., and Zobel, D. B. (1994). Biomass productivity, leaf longevity and forest structure along an altitudinal gradient in central Himalaya. Ecol. Monogr. 64, 401–421. doi: 10.2307/2937143
Singh, S. P., and Singh, J. S. (1986). Structure and function of the Central Himalayan oak forests. Proc. Indian Acad. Sci. Sec. A 96, 159–189. doi: 10.1007/BF03053301
Singh, S. P., Rawat, Y. S., and Garkoti, S. C. (1997). Failure of brown oak (Quercus semecarpifolia) to regenerate in central Himalaya: A case of environmental semisurprise. Curr. Sci. 73, 371–374.
Singh, V., Thadani, R., Tewari, A., and Ram, J. (2014). Human influence on banj Oak (Quercus leucotrichophora, A. Camus) forests of Central Himalaya. J. Sustain. For. 33, 373–386. doi: 10.1080/10549811.2014.899500
Singhal, R. M., Rawat, V. R. S., Kumar, P., Sharma, S. D., and Singh, H. B. (1986). Vegetation analysis of woody species of some forest of Chakarta Himalaya, India. Indian For. 112, 819–823.
Springate-Baginski, O., Dev, O. P., Yadav, N. P., and Soussan, J. (2003). Community Forest management in the middle hills of Nepal: The changing context. J. For. Livelihood 3, 5–20.
Subedi, M. N. (2004). Above ground biomass of Quercus semecarpifoliaSm forest surveyed on natural and seminatural stands in Nepal. Indian For. 8, 858–866.
Subedi, M. N. (2006). Conservation and sustainable utilization of oak (Quercus semecorpofolia Sm.) forest in Nepal. Bull. Dep. Plant Resour. 27, 44–50.
Tamta, S., Palni, L. M. S., Purohit, V. K., and Nandi, S. K. (2008). In vitro propagation of brown oak (Quercus semecarpifolia Sm.) from seedling explants. In Vitro Cellular and Developmental Biology-Plant 44, 136–141. doi: 10.1007/s11627-008-9138-x
Tamta, S., Palni, L. M. S., Vyas, P., and Bisht, M. S. (2009). Conservation through in vitro method: A case of plant regeneration through somatic embryogenesis in Quercus semecarpifolia Sm. J. Am. Sci. 5, 70–76.
Tashi, S. (2004). Regeneration of Quercus semecarpifolia Sm. in an old growth forest under Gidakom FMU-Bhutan. M.Sc. thesis. Wageningen: Wageningen University and Research Centrum.
Tewari, A., Shah, S., Singh, N., Tamta, K. K., and Mittal, A. (2019). Acorn maturation and regeneration problem in Quercus semecarpifolia sm. in Himalayan treeline. Int. J. Sci. Technol. Res. 8, 3781–3787.
Thadani, R. (1999). Disturbance, microclimate and the competitive dynamics of tree seedlings in banj oak (Quercus leucotrichophora) forests of the Central Himalaya, India. Ph.D. thesis. Yale: Yale University.
Thadani, R., and Ashton, P. M. S. (1995). Regeneration of banj oak (Quercus leucotrichophora A. Camus) in the central Himalaya. For. Ecol. Manage. 78, 217–224. doi: 10.1016/0378-1127(95)03561-4
Thakuri, P. S. (2010). Plant community structure and regeneration of Quercus semecarpifolia forests in disturbed and undisturbed areas. M.Sc. thesis. Kathmandu: Tribhuvan University.
Tripathi, R. S., and Khan, M. L. (1990). Effects of seed weight and micro-site characteristics on seed germination and seedling fitness in two species of Quercus in a subtropical wet hill forest. OIKOS 57, 289–296. doi: 10.2307/3565956
Tudge, C. (2006). The secret life of trees: How they live and why they matter. London: Penguin Book Ltd.
Upreti, N., Tewari, J. C., and Singh, S. P. (1985). The oak forests of the Kumaun Himalaya (India): Composition, diversity, and regeneration. Mt. Res. Dev. 05, 163–174. doi: 10.2307/3673255
Usman, S., Singh, S. P., Rawat, Y. S., and Bargali, S. S. (2000). Fine root decomposition and nitrogen mineralisation patterns in Quercus leucotrichophora and Pinus roxburghii forests in Central Himalaya. For. Ecol. Mange. 131, 191–199. doi: 10.1016/S0378-1127(99)00213-3
Valero, G. J., Jorrín-Novo, J., Gómez, C., Ariza, D., García-Olmo, J., and Navarro-Cerrillo, R. M. (2011a). Population variability based on the morphometry and chemical composition of the acorn in Holm oak (Quercus ilex subsp. ballota [Desf.] Samp.). Eur. J. For. Res. 131, 893–904. doi: 10.1007/s10342-011-0563-8
Valero, G. J., Valledor, L., Navarro Cerrillo, R. M., Gil, E., and Jorrin, J. V. (2011b). Studies of variability in Holm oak (Quercus ilex subsp. Ballota [Desf.] Samp.) through acorn protein profile analysis. J. Proteom. 74, 1244–1255. doi: 10.1016/j.jprot.2011.05.003
Vazquez, C., and Rojas, M. (1996). Ex situ conservation of tropical rain forest seed: Problems and perspectives. Interciencia 21, 293–298.
Verma, K. S., and Mishra, V. K. (1999). Foliage nutrient concentration and lopping time of agroforestry tree species in the Western Himalayan region of India. Agroforestry Syst. 42, 283–290. doi: 10.1023/A:1006151614882
Vesterdal, L., Schmidt, I. K., Callesen, I., Nilsson, L. O., and Gundersen, P. (2008). Carbon and nitrogen in forest floor and mineral soil under six common European tree species. For. Ecol. Manage. 255, 35–48. doi: 10.1016/j.foreco.2007.08.015
Vetaas, O. R. (1997). The effect of canopy disturbance on species richness in a central Himalayan oak forest. Plant Ecol. 132, 29–38. doi: 10.1023/A:1009751219823
Vetaas, O. R. (2000). The effect of environmental factors on the regeneration of Quercus semecarpifolia Sm. in central Himalayan Nepal. Plant Ecol. 146, 137–144. doi: 10.1023/A:1009860227886
Vetaas, O. R., and Chaudhary, R. P. (1998). Scale and species environment relationships in a central Himalayan Oak forest. Nepal. Plant Ecol. 134, 67–76.
Vieitez, A. M., San Jose, M. C., and Vietiz, E. (1985). In vitro plantlet regeneration from juvenile and mature Quercus robur L. J. Hortic. Sci. 601, 99–106. doi: 10.1080/14620316.1985.11515607
Viswanath, S., Singh, R. P., and Thapliyal, R. C. (2002). Seed germination patterns in a Himalayan moist temperate forest. Trop. Ecol. 43, 265–273.
Williams, K., Kubelik, A. R., Rafalski, J. A., and Tingey, S. V. (1990). DNA polymorphisms amplified by arbitary primers are useful as genetic markers. Nucleic Acid Res. 18, 1631–1635. doi: 10.1093/nar/18.22.6531
Yadav, R., Tripathi, S. K., Pranuthi, G., and Dubey, S. K. (2014). Trend analysis by Mann-Kendall test for precipitation and temperature for thirteen districts of Uttarakhand. J. Agrometeorology 16, 164–171. doi: 10.54386/jam.v16i2.1507
Yang, Y. H., Fang, J. Y., Guo, D. L., Ji, C. J., and Ma, W. H. (2010). Vertical patterns of soil carbon, nitrogen and carbon nitrogen stoichiometry in Tibetan grassland. Biogeosci. Discuss. 7, 1–24. doi: 10.5194/bgd-7-1-2010
Yuan, Z. Y., and Chen, H. Y. H. (2015). Decoupling of nitrogen and phosphorus in terrestrial plants associated with global changes. Nat. Clim. Change 5, 465–469. doi: 10.1038/nclimate2549
Zanetto, A., and Kremer, A. (1995). Geographical structure of gene diversity in Quercus petraea (Matt.) Liebl. I. Monolocus patterns of variation. Heredity 75, 506–517. doi: 10.1038/hdy.1995.167
Keywords: climax community, brown oak, keystone species, regeneration, disturbance
Citation: Rawat B, Rawat JM, Purohit S, Singh G, Sharma PK, Chandra A, Shabaaz Begum JP, Venugopal D, Jaremko M and Qureshi KA (2022) A comprehensive review of Quercus semecarpifolia Sm.: An ecologically and commercially important Himalayan tree. Front. Ecol. Evol. 10:961345. doi: 10.3389/fevo.2022.961345
Received: 04 June 2022; Accepted: 31 August 2022;
Published: 29 September 2022.
Edited by:
Pavel Kindlmann, Charles University, CzechiaReviewed by:
Bikram Shrestha, Global Change Research Institute, CzechiaYu Fei, Henan Normal University, China
Copyright © 2022 Rawat, Rawat, Purohit, Singh, Sharma, Chandra, Shabaaz Begum, Venugopal, Jaremko and Qureshi. This is an open-access article distributed under the terms of the Creative Commons Attribution License (CC BY). The use, distribution or reproduction in other forums is permitted, provided the original author(s) and the copyright owner(s) are credited and that the original publication in this journal is cited, in accordance with accepted academic practice. No use, distribution or reproduction is permitted which does not comply with these terms.
*Correspondence: Janhvi M. Rawat, janhvi.mishra03@gmail.com; Kamal A. Qureshi, ka.qurishe@qu.edu.sa