- 1Programa de Pós-Graduação em Ecologia e Conservação da Biodiversidade, Universidade Estadual de Santa Cruz, Ilhéus, Bahia, Brazil
- 2Tropical Herpetology Lab, Universidade Estadual de Santa Cruz, Ilhéus, Bahia, Brazil
- 3Laboratório de Ecologia Aplicada à Conservação, Universidade Estadual de Santa Cruz, Ilhéus, Bahia, Brazil
- 4Zoologisches Forschungsmuseum Alexander Koenig, Herpetology Section, Bonn, North Rhine-Westphalia, Germany
Climate change can affect species distribution patterns in three different ways: pushing them to disperse to new suitable areas, forcing them to adapt to novel climatic conditions, or driving them to extinction. However, the biological and geographical traits that lead to these different responses remain poorly explored. Here, we evaluated how ecological and biogeographic traits influence amphibians’ response to climate change. We performed a systematic review searching for studies that evaluated the effects of future climate change on amphibian’s distribution. Our research returned 31 articles that projected the distribution of 331 amphibians. Our results demonstrate that species inhabiting an elevation above 515 m will lose a significant portion of their climatically suitable area. We also found that as isothermality increases, the amount of area suitable in response to climate change also increases. Another important discovery was that as the size of the baseline area increases, the greater must be the loss of climatically suitable areas. On the other hand, species with very small areas tend to keep their current climatically suitable area in the future. Furthermore, our results indicate that species that inhabit dry habitats tend to expand their suitable area in response to climate change. This result can be explained by the environmental characteristics of these habitats, which tend to present extreme seasonal climates with well-defined periods of drought and rain. We also found that anurans that inhabit exclusively forests are projected to lose a greater portion of their suitable areas, when compared to species that inhabit both forest and open areas, wetlands, and dry and rupestrian environments. The biogeographical realm also influenced anuran’s range shifts, with Afrotropic and Nearctic species projected to expand their geographical ranges. The assessment of climate change effects on amphibian distribution has been the focus of a growing number of studies. Despite this, some regions and species remain underrepresented. Current literature evaluates about 4% of the 7,477 species of Anura and 8% of the 773 species of Caudata and some regions rich in amphibian species remain severely underrepresented, such as Madagascar. Thus, future studies should focus on regions and taxas that remain underrepresented.
Introduction
Climate change determines large scale patterns of species distribution in three principal ways: pushing them to disperse to new suitable areas, forcing them to adapt to novel climatic conditions, or driving them to extinction (Holt, 1990; Parmesan, 2006; Diniz-Filho and Bini, 2007; Araújo et al., 2008). While some species are losing part of their current geographic range due to climate change (Zank et al., 2014; Struecker and Milanovich, 2017; Zhang et al., 2020), other species can even expand their suitable areas (Mokhatla et al., 2015; Toranza et al., 2016). Therefore, although we expect that most species are likely to be equally affected by global warming, the response patterns can be quite contrasting (Winter et al., 2016; Vasconcelos et al., 2018; Menéndez-Guerrero et al., 2020).
Among vertebrates, amphibians represent one of the most vulnerable groups to global warming (Pounds, 2001; Blaustein et al., 2010), because almost all species are highly dependent of specific climatic conditions (Zeisset and Beebee, 2008) and have narrow ecological niches (Blaustein et al., 2010). Climate change may increase vulnerability of amphibians by acting synergistically with other impacts like habitat loss, emerging diseases, and chemical contaminants (Stuart et al., 2004; Collins, 2010). According to the Global Amphibian Assessment (GAA), these threats have already placed 32% of amphibian species under some of IUCN Red List threat categories (i.e., Vulnerable, Endangered, or Critically Endangered) (Stuart et al., 2004). Thus, anticipating the effects of climate change on the amphibians’ distribution and identifying the traits that make the species more vulnerable to climate change has become a priority for conservation. In this sense, the use of Ecological Niche Models (ENM, Araújo and Peterson, 2012) has been an essential tool to generate conservation strategies based on the climate effect on distribution (Sillero et al., 2021). The ENMs use occurrence records and bioclimatic variables to make mathematical approximations on species climatic niche and allow the prediction of climatically suitable areas under various climate scenarios (Taylor et al., 2020), allowing the anticipation of species responses to climate change (Urbina-Cardona and Loyola, 2008).
Life history traits can be important candidates to explain the variation in species vulnerability to global warming (Foden et al., 2013; Beissinger and Riddell, 2021). For example, anurans with specialized reproductive modes are expected to be more strongly dependent on the integrity of very specific habitats (Loyola et al., 2008), and therefore should be more negatively affected by climate change than anurans with generalist reproductive modes. Likewise, species that rely on environmental triggers to initiate activities such as migration or reproduction must also experience heightened sensitivity to climate change (Foden et al., 2013). On the other hand, large ectotherms are expected to be less affected by climate change because the convection limit increases with body size. In this way, heat loss by convection will be lower and make them more resistant to higher temperatures than smaller ectotherms (Seebacher et al., 1999, 2003; Rubalcaba and Olalla-Tárraga, 2020).
The amphibians vulnerability to climate change is also expected to be limited by the amplitude of their thermal tolerance range (Freitas et al., 2010), result of the process of natural selection and adaptation to the extreme temperatures that lineages have experienced throughout their evolution (Denny et al., 2009; Bozinovic et al., 2011; Buckley and Huey, 2016). Therefore, the lineages that tend to experience relatively higher average temperatures and less seasonal variation should have less potential for adaptive rescue (Ghalambor, 2006; Huey et al., 2009), as they already have their maximum thermal tolerance close to or above optimal. In this way, small increases in temperature can have disproportionately large effects on their thermal performance (Pörtner and Knust, 2007; Tewksbury et al., 2008).
Current range size, range of elevation, and biogeographic domain can also be predictors of a “shared destination” in response to climate change, as species found in the same region share certain niche attributes and tend to respond similarly to global warming. For example, species with very narrow distributions tend to occupy a more restricted number of microhabitats and can be more negatively affected by climate change (Foden et al., 2013; Büchi and Vuilleumier, 2014). The same may be true for species coupled with specific environmental conditions (e.g., forest habitats or mountain tops), as suitable climatic conditions can be displaced to regions beyond the species’ ability to disperse, which can lead to local extinction. On the other hand, species that are widely distributed and adapted to a variety of habitats may have more available areas with climatic conditions filling the requirements of their niche, even if the suitable conditions have been locally lost (Clavel et al., 2011).
Although it is already known that biological and biogeographic characteristics can influence the intensity and direction of species response to climate change (Foden et al., 2013; Borges et al., 2019; Alves-Ferreira et al., 2022), a few studies have assessed the existence of global patterns on climate change response using these traits. In this study, we assessed the global patterns of climate change effects on amphibian (Anura and Caudata) distributions through a trait- and biogeographical-based analysis of published data. Specifically, we assessed whether the response of amphibians to climate change is more strongly influenced by life history traits (body size, habit, reproductive mode, and habitat specialty) or by biogeographic characteristics (elevation, biogeographical realm, baseline area, isothermality, precipitation, and temperature seasonality).
Materials and methods
Data compilation
We performed a systematic review of published studies in two databases (Scopus and ISI Web of Science) for manuscripts that evaluated the effects of climate change on the potential distribution of amphibians. We gathered studies published until February, 2022 (Figure 1) using the following keywords: [(“climatic chang*” OR “climate chang*” OR “global warm*” OR “climate warm*” OR “changing climate”) AND (“Amphibian*” OR “Anura*” OR “Caudata” OR “Salamander” OR “Frog” OR “Toad”) AND (”Geographic range” OR “distribut*” OR “suitab*” OR “niche model*” OR “scenario*” OR “range shift*”)]. We used the following eligibility criteria to include studies in our database: they must have (1) assessed the effect of future climate changes on the distribution of Anura or Caudata; (2) presented the size of the potential future distribution; (3) used correlative models. After the first filtering by title and abstract, we excluded studies that evaluated another taxonomic group, worked with invasive species, did not evaluate the effect of climate change on distribution, assessed species at population level only and experimental studies with Critical thermal maximum (CTmax). Our initial search resulted in 1,161 possibly eligible studies. After filtering by eligibility criteria, we obtained 41 studies that modeled the potential distribution of 520 species (Figure 1). For articles that presented the data in graphs, we used the software GetData GraphDigitizer version 2.26 (Fedorov, 2013) to access the information.
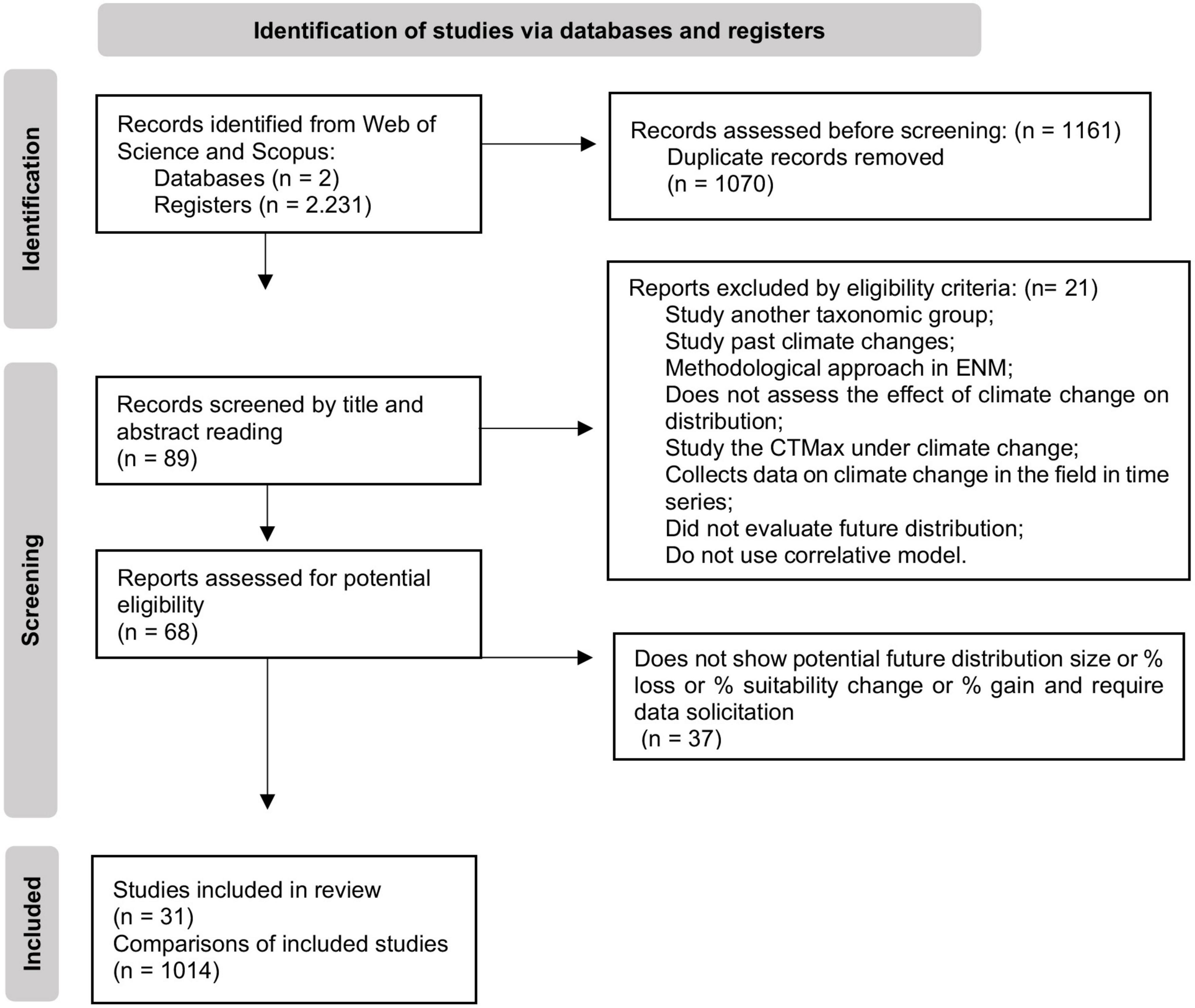
Figure 1. PRISMA diagram representing the selection process of the studies included in our analysis.
Data extraction: Life-history and biogeographic data
We extracted the following information for each study: species, family, order, climatically suitable area size in present and future, and change percentage in climatically suitable areas. Studies that presented data for more than one species had such information recorded as independent data. To associate the climatic suitability with biological and biogeographical attributes, we obtained the biogeographical realm sensu Olson et al. (2001). We achieved the habitat type from the IUCN database (IUCN, 2022) and habit, reproductive mode, and body size (mm) from the AmphiBIO database (Oliveira et al., 2017).
The elevation and bioclimatic variables: Isothermality (BIO3), Temperature Seasonality (BIO4), and Precipitation Seasonality (BIO15) were achieved for baseline scenarios (1970–2000) using resolution of 2.5 arc min from the WorldClim database (Fick and Hijmans, 2017). These variables could be expected to influence amphibian distribution (Whitton et al., 2012; Gouveia et al., 2013; Zank et al., 2014). For example, Sodhi et al. (2008) found that the risk of extinction increases for amphibians that live in regions with very pronounced seasonality of precipitation and temperature.
We used the Global Biodiversity Information Facility (GBIF.org, 2022) database to obtain occurrence records for the species. We considered species with more than three independent occurrence records. Using these records, we extracted the elevation and bioclimatic variables for each occurrence and averaged these values per species. To test whether there is a correlation between continuous variables (Supplementary Figure 1), we conducted a Pearson Correlation test with a threshold of 75%. To measure the association between pairs of categorical variables (Supplementary Figure 2) we used the Goodman Kruskal measure (Goodman and Kruskal, 1972). We used Frost (2022) to update the taxonomic nomenclature.
Data analysis
Statistical analysis
To assess whether the amphibian’s response to climate change is influenced by life history traits and by biogeographic features, we built linear mixed models using the “lme4” R package (Bates et al., 2015). Species and studies were treated as random factors to control for species that appear in multiple studies and to control for repetitions within the same study. As isothermality (BIO3) was highly correlated with seasonality of temperature (BIO4) and seasonality of precipitation (BIO15), we kept only isothermality (BIO3) in further analyses. The predictor variables were: body size, habit, reproductive mode, habitat specialty, elevation, biogeographical realm, baseline area, and isothermality (See Supplementary Table 1 for a more detailed description of each variable). Both types of variables are mixed in the models. The response variable was the square root of the relative area, calculated by dividing the area (km2) in the future by the area (km2) in the reference scenario. We generated 130 eligible models from combinations between predictor variables with a minimum limit of zero (null model) until the maximum of three terms in a single model (excluding the intercept). Finally, we built an average model based on the Akaike Information Criterion corrected for small samples (AICc; Burnham and Anderson, 2002). We calculated the relative importance of each predictor variable using the sum of model weights over all models. All analyses and figures were performed in R (R Core Team, 2022).
Spatial patterns of species area change
We produced a species richness map to identify the regions in the world with the highest number of evaluated species. This map was constructed based on the sum of the binary distributions (absence = 0 or presence = 1) of the species evaluated in the studies included in our review. To demonstrate the spatial pattern of species area changes we cross-referenced the relative proportion of change with the map of binary distributions. Therefore, this map represents the local variability of the climate change effects on species distributions. As species can overlap spatially and this can make it difficult to visualize the spatial trend of loss and gain, we calculated the area change for 5 quantiles (zero, 25, 50, 75, and 100). At one extreme, the lower quantile (0%) represents the lowest values of change (i.e., higher losses to smaller gains) among locally occurring species. The third quantile (50%) represents the central tendency among species. At the other extreme, the upper quantile (100%) represents the highest values of change (i.e., smaller losses to higher gains of area) among species. Since we do not have distribution rasters available for the species of each study, we used the geographic distributions provided by the IUCN (2022) to produce the maps of species richness and area change. All spatial calculations were performed with the “terra” package (Hijmans, 2022) in program R (R Core Team, 2022).
Results
General characteristics of selected papers
Our initial database resulted in 41 manuscripts with data for 520 species. However, not all studies reported suitable baseline and future areas for the species, and not all species have characteristics and occurrence data available to perform our analysis. Therefore, the final dataset included 31 papers that projected the distribution of 331 amphibian species belonging to 35 families from around the globe (Figure 2). Among these species, 268 belong to the order Anura and 64 belong to the order Caudata (see Supplementary Table 2). Among the 331 species evaluated, 112 should gain climatically suitable areas in the future, 214 species are predicted to have adequate climatic conditions reduced and four species should maintain the same area in the present and in the future.
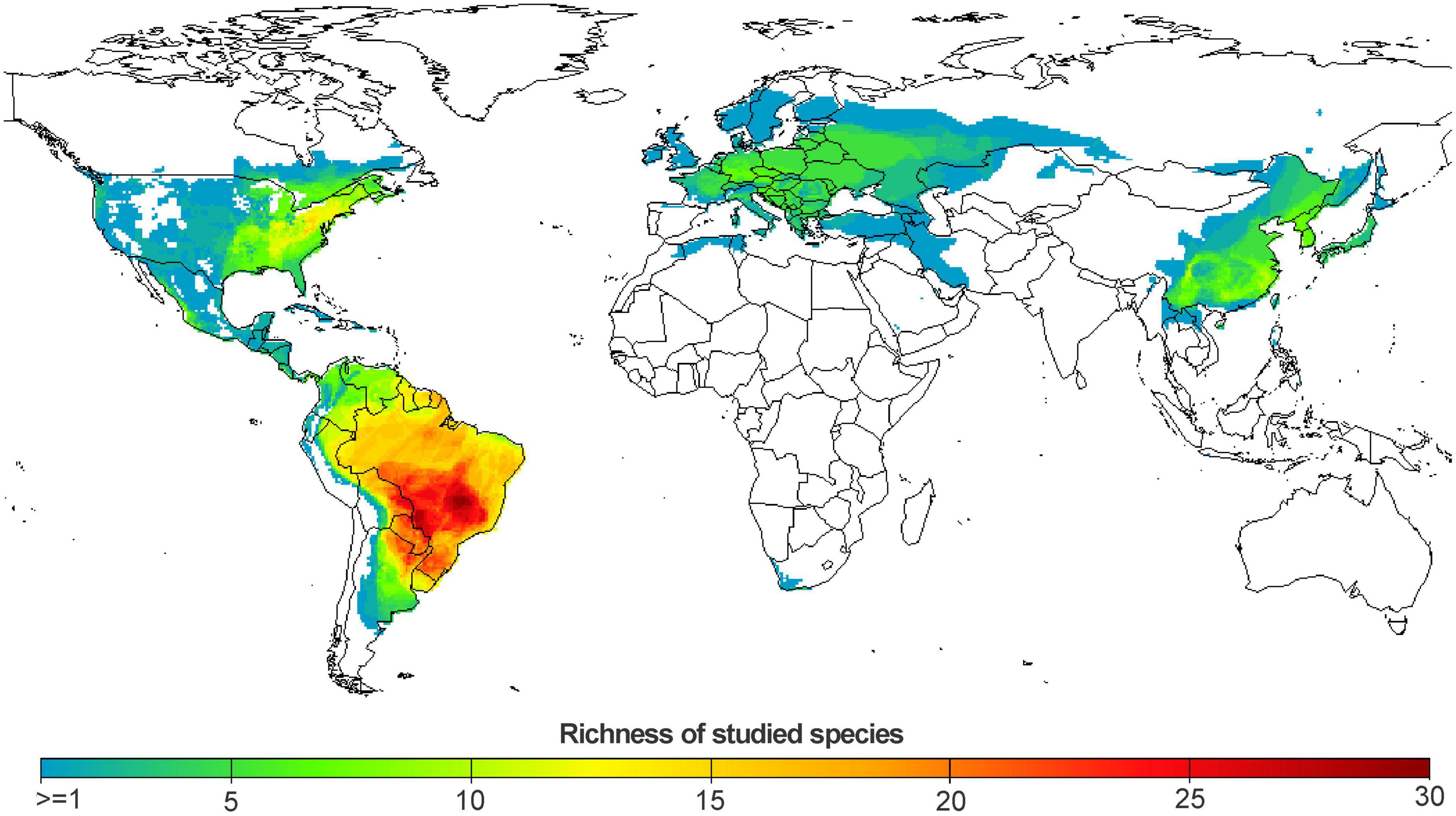
Figure 2. Richness of amphibian species (331 species) studied in the articles included in our review (31 studies). The blue and green colors represent places where a smaller number of species were studied, while the yellow and red colors represent the places with the largest number of species studied.
Biological and biogeographical traits
The most important variables related to amphibian’s range shifts (Table 1) were baseline area (W = 0.99), realm (W = 0.54), isothermality (W = 0.47), elevation (W = 0.41), and habitat type (W = 0.30). The average model coefficient shows that all these variables can significantly affect the amount of amphibian’s suitable areas in response to climate change (Table 2). On the other hand, habit, population trend, body size, and reproductive mode were of minor importance (W = 0.06) and their effect was not significant (Table 2).
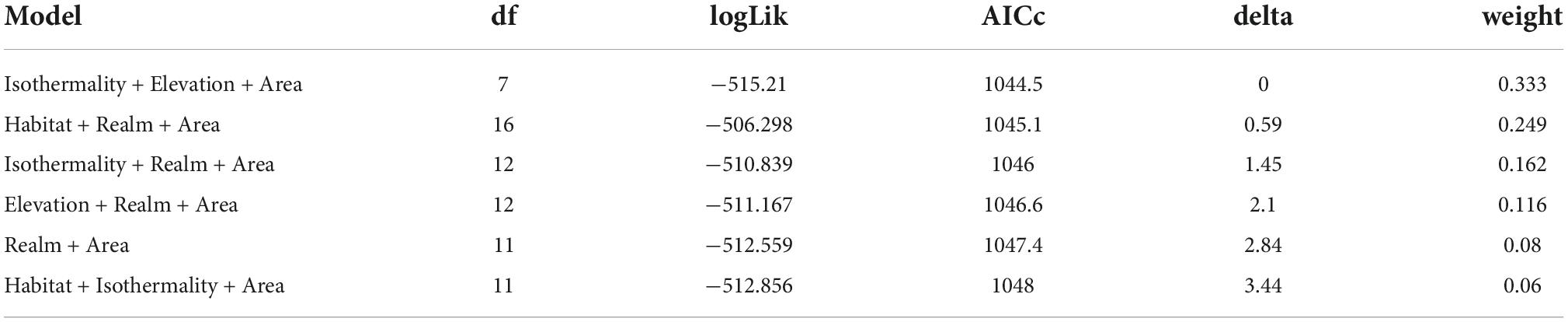
Table 1. The six models relating amphibians range shift and biological and biogeographical traits with the highest rankings among the 130 candidate models and their second-order Akaike information criterion values (AICc), AICc weights (weight), AICc differences (delta), Log-likelihood (loglik), and degrees of freedom (df).
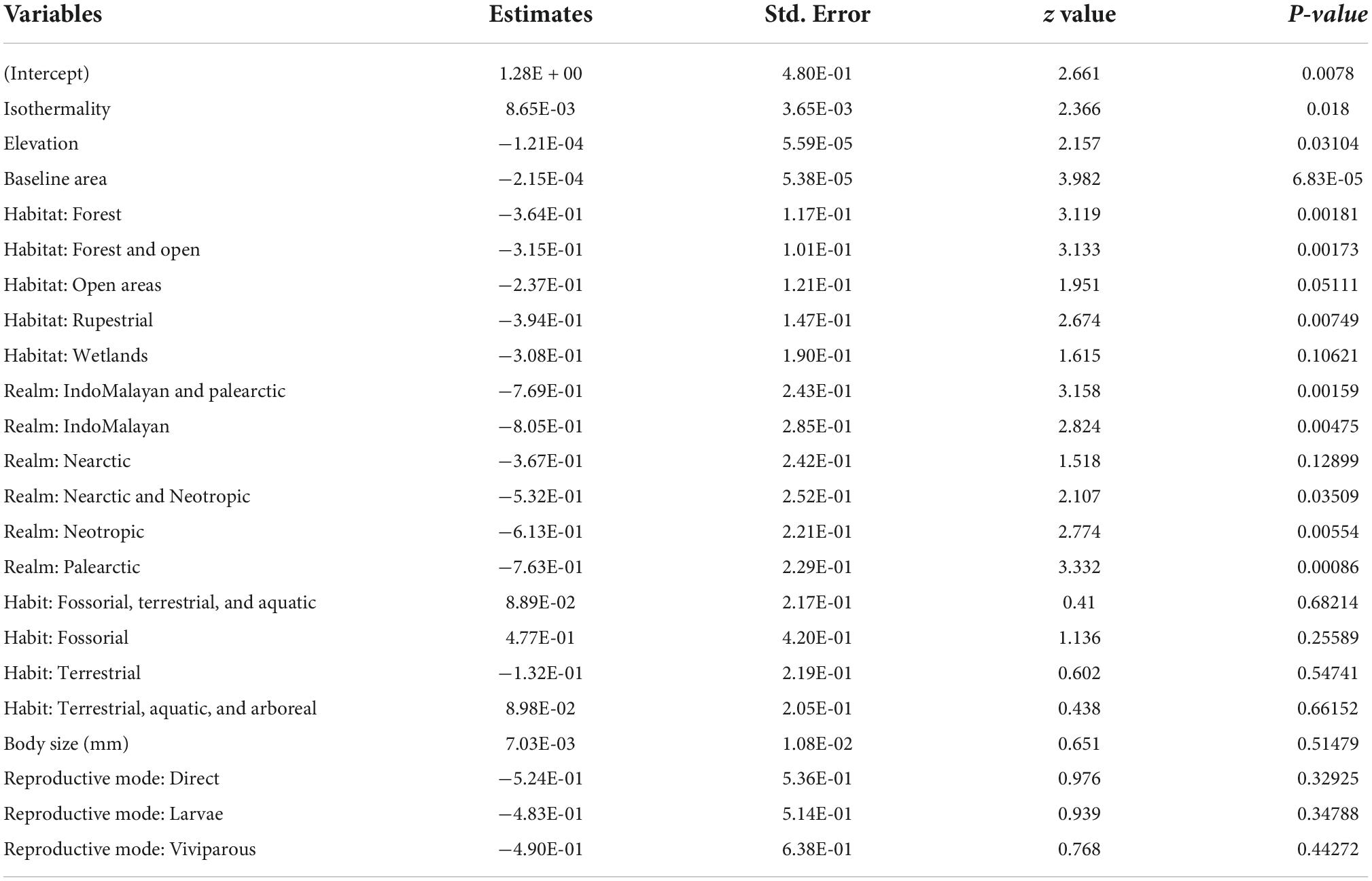
Table 2. Model-averaged coefficients based on conditional average including estimates, p-value, z value, standard error.
The elevation where the species inhabit may explain part of the effect of climate change on suitable areas for amphibians (Beta = −0.002, SE = 0.001, z value = 2.148, p value = 0.031). We found that species inhabiting average elevation above 515 m will lose a significant portion of their climatically suitable area (Figure 3A). Isothermality was another important variable (Beta = 0.008, SE = 0.004, z value = 2.285, p value = 0.022). As isothermality increases, the amount of suitable area gain in response to climate change also increases (Figure 3B). More specifically, species that occur in regions with higher “temperature uniformity” over a year (i.e., isothermality below ∼30%) tend to gain climatically suitable areas, while species that occur in less isothermic regions tend to lose suitable areas with the advancing climate change. Finally, the size of baseline area explained most amphibian distribution changes (Beta = −0.002, SE = 0.001, z value = 3.976, p value = 0.001). We found that as the size of the distribution increases, the greater are projected to be the relative losses in climatically suitable areas. On the other hand, species with very small areas tend to retain most of their current distribution areas in the future (Figure 3C).
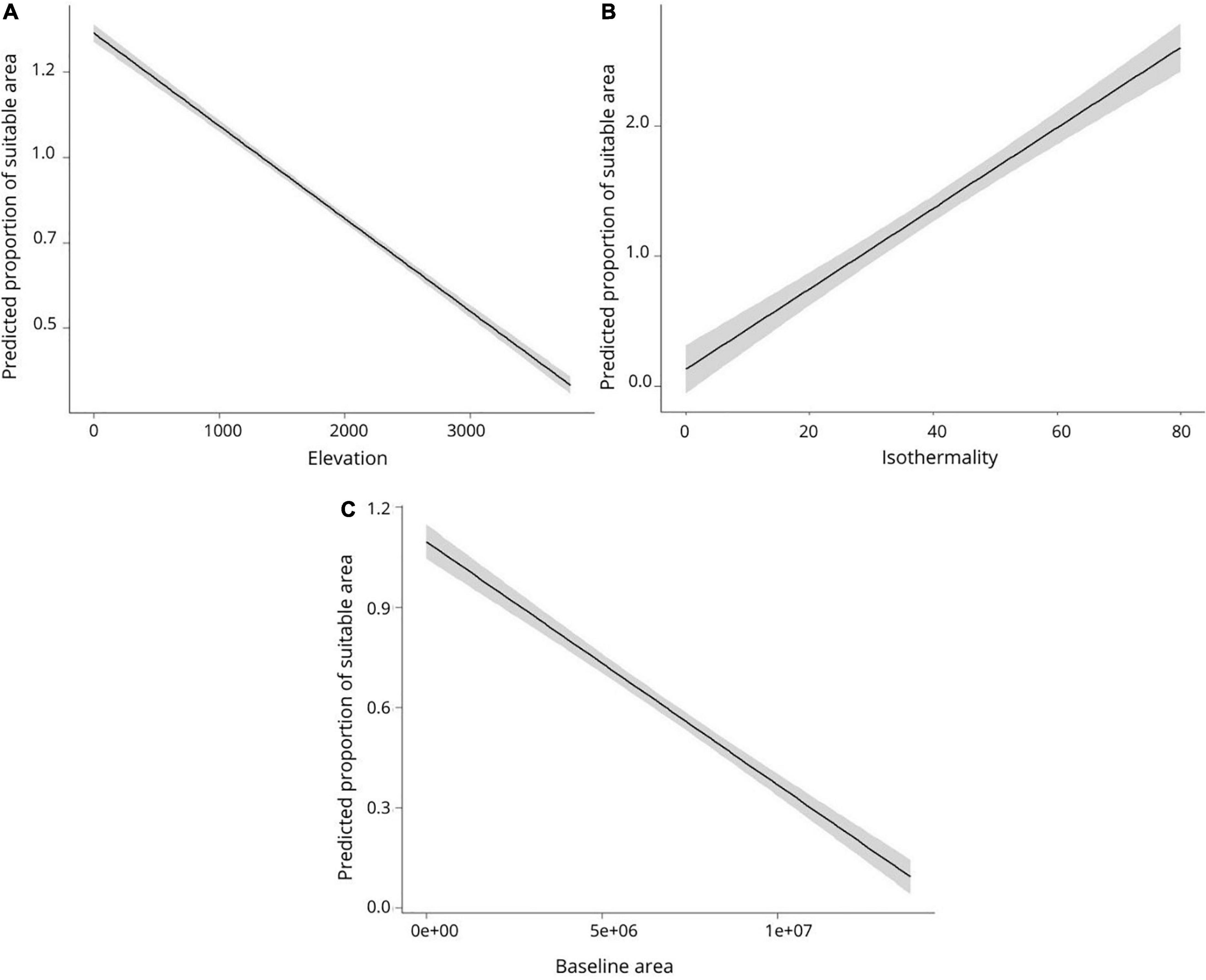
Figure 3. Relationship between the proportion of climatically suitable areas and elevation (A), isothermality (B), and baseline area (C). Values above one indicate gain of suitable area. The gray area represents confidence intervals (95%).
We found that habitat type and biogeographical realm are also good predictors of amphibian’s range shifts. Particularly, the results indicate that species that inhabit dry habitats (such as savannas, arid, and semi-arid habitats) tend to expand their suitable area in response to climate change. We also found that anurans that inhabit exclusively forests (Beta = −0.366, SE = 0.116, z value = 3.149, p value = 0.002) or rupestrial habitats (Beta = −3.957, SE = 1.464, z value = 2.700, p value = 0.007) are projected to lose a greater portion of their suitable area, when compared to species that inhabit both forest and open areas, wetlands, and dry habitats (Figure 4A). The biogeographical realm also influenced anuran’s range shifts, with Afrotropic and Nearctic amphibians amphibians projected to expand their suitable areas in response to global warming when compared to species from the other realms (Figure 4B).
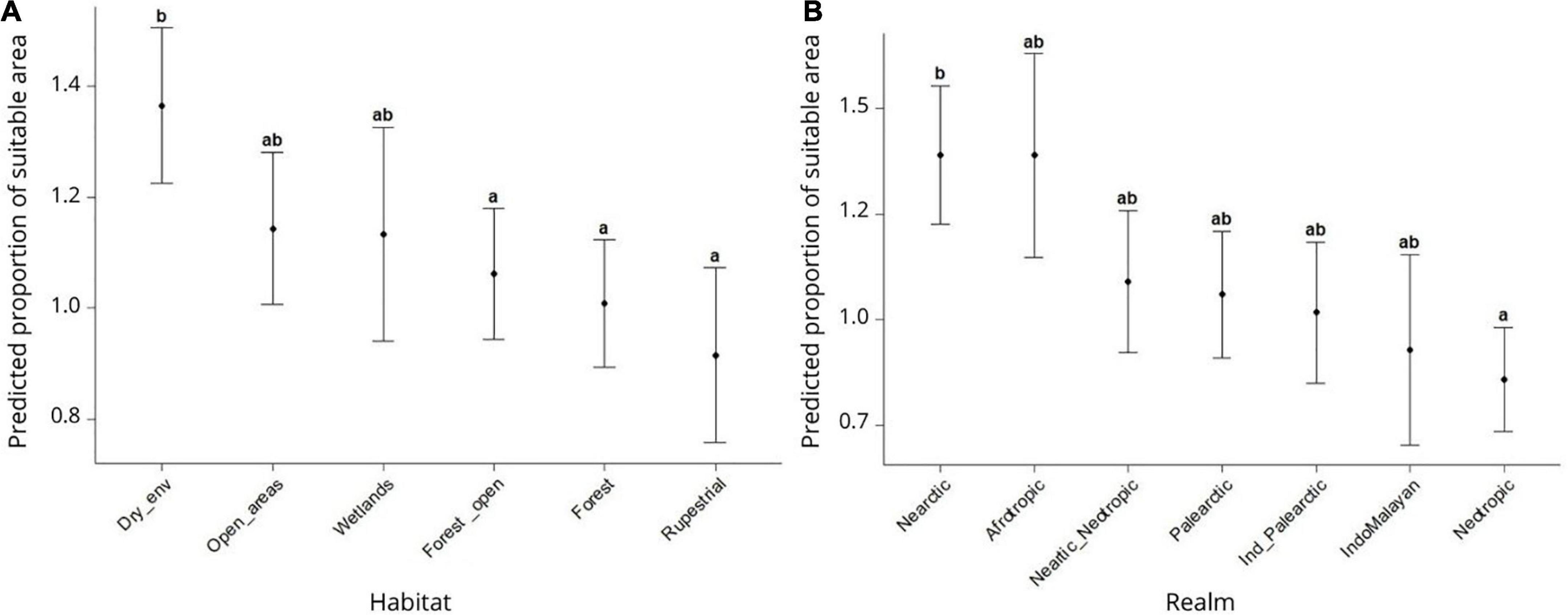
Figure 4. Estimated marginal means of change in climatically suitable area per habitat type (A) and biogeographical realm (B). The standard error of the mean is represented by vertical bars. Letters indicate categories with statistically significant differences.
Spatial patterns of amphibian’s area change
The quartile maps show the local variation in species responses to climate change. The 0% quartile map (i.e., minimum area change values across species for species on each pixel) reveals that at least one species will lose part of its current range in most of the studied regions. Only in a few places (e.g., part of the Andean region of Colombia, extreme North Italy, central south part of Bolivia-Austral Yungas region, north-eastern part of Argentina-La Plata Basin region, Midwestern and South region of United States) the species are projected to gain new climatically suitable areas. The 50% quartile map (central tendency) shows that most species are projected to lose suitable areas across most of the studied regions. The 100% quartile map (i.e., maximum values across species on each pixel) shows that at least one species gains suitable area in most regions, but in several regions there will be only loss of suitable area across all species. Another important information to highlight when comparing the 0 and 100% quartiles maps (minimum and maximum values, respectively) is that while a combination of area loss and gain is projected for the species in most regions, in some areas all the species are projected only to lose (i.e., southwestern Russia, north-central Mexico, the northern United States, and the north-central Andean mountain range) or gain (i.e., southern Canada, the southeastern and northeastern United States, the Brazilian Cerrado and the far south of the Atlantic Forest in Brazil) suitable areas in the future (Figure 5).
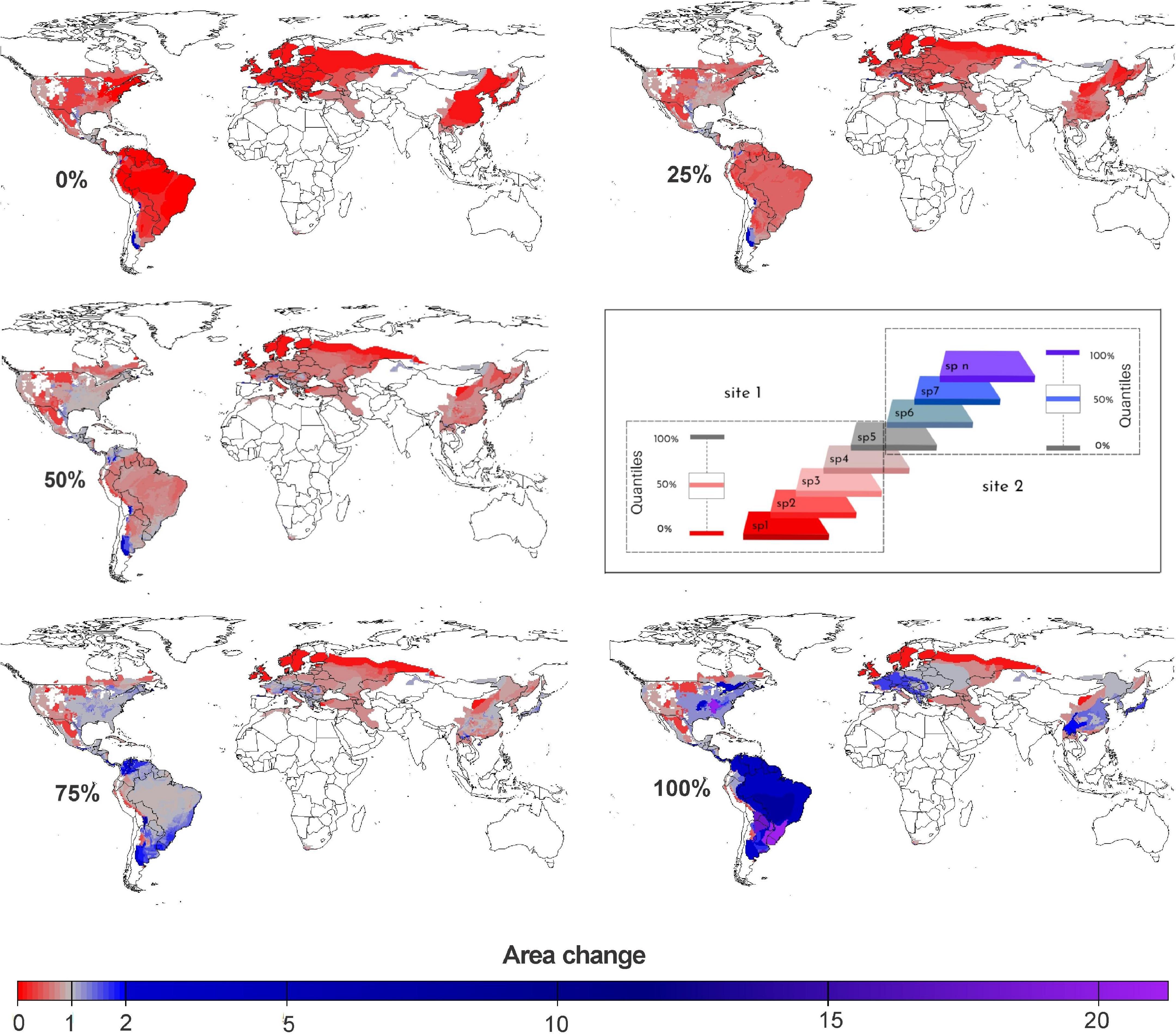
Figure 5. Quartile maps showing the local variation in species responses to climate change. Red indicates losses in suitable areas, gray indicates no change, and blue to purple indicate gains in suitable areas. Quantiles were calculated for the responses of species occurring at each pixel (see graphical scheme of calculations in the center). At one extreme, the lower quantile (0%, minimum values) represents the lowest values of change (i.e., higher losses to smaller gains) among species occurring at each map pixel. The third quantile (50%, median) represents the central tendency among species. At the other extreme, the upper quantile (100%, maximum values) represents the highest values of change (i.e., smaller losses to higher gains of area) among species.
Discussion
In this review, we accessed the global patterns of climate change effects on amphibian distributions through a biological and biogeographical analysis of published data. Our analysis provides evidence that baseline area, isothermality, habitat type, elevation, and realm are consistently important drivers to predict amphibian’s range shifts. This finding reinforces the idea that biogeographic variables may be more important than life history traits in predicting the climate change effects on amphibians’ distribution (Alves-Ferreira et al., 2022). To our knowledge, this is the first systematic review of published data that assesses the global effects of climate change on amphibian distribution using biological and biogeographic traits as predictors.
We found that species inhabiting an elevation above 515 m are projected to lose climatically suitable areas. This is because as elevation increases, the available area for the species decreases, and consequently leads to the loss of suitable area. Therefore, it is expected that species that inhabit higher altitudes will be more negatively affected by climate change, since the available climatic area tends to reduce toward the top of the mountains (Nori et al., 2016). Our results also provide evidence that as isothermality increases, the amount of suitable area gain in response to climate change also increases. Therefore, species from less isothermal regions (i.e., regions with lower “temperature uniformity” and more variation over a year, below 30%) tend to lose climatically suitable areas, while species that occur in more isothermal regions (above 30%) tend to gain suitable areas with advancing climate change. The vast majority of the more isothermal areas in our study are located in tropical and subtropical regions (Neotropic, Afrotropic, and IndoMalayan realms). However, the data are mostly from Neotropical regions, with low representation of studied species from the Afrotropic and Australian realms.
We also found that the size of the baseline area is positively correlated with the projected loss of climatically suitable areas. The result indicates that species with a very restricted distribution should suffer a low proportion of losses in their current distribution areas, while widespread species should suffer the highest proportion of losses. This seems contradictory at first glance, as species with restricted ranges are expected to lose more area than widely distributed ones, due to the expectation that restricted ranges result from narrower niches (Slatyer et al., 2013; Saupe et al., 2015; Evans and Jacquemyn, 2022). A possible explanation for the lower proportion of loss for species with very restricted ranges may be related to the extreme specialization that can lead some species to “escape” the full impacts of climate change (Foden et al., 2013). However, this hypothesis requires that these small ranged species are adapted to unique climatic conditions that will remain stable in the future. Thus, it is more likely that range retention is mostly due to climatic stability than specialization to unique climatic conditions (Fjeldså, 1994; Cardoso da Silva et al., 2004; Harrison and Noss, 2017; Wilson et al., 2019).
Furthermore, our results indicate that species that inhabit dry habitats (such as savannas, arid and semi-arid habits) tend to expand their suitable areas in response to climate change. This result can be explained by the environmental characteristics of these habitats, which tend to present extreme seasonal climates with well-defined periods of drought and rain (Murphy and Lugo, 1986; Gentry, 1995). The Intergovernmental Panel of Climate Change projects future warming scenarios that exacerbate the frequency and intensity of drought and water stress (IPCC, 2022). Therefore, global warming is likely to favor drought-resistant anuran species. We also found that anurans that exclusively inhabit forests and rupestrial environments are projected to lose a greater portion of their suitable areas, when compared to species that inhabit both forest and open areas, and dry environments. This may happen because species strongly associated with specific conditions and requirements (i.e., warm and moist climate found in forests) tend to have a narrow range of available habitats and microhabitats (Foden et al., 2013). In addition, the expansion of dry conditions will unfavour forest habitats. Therefore, it is likely that these species will not have suitable climatic conditions available in the future, which can increase the risk of local extinction.
The biogeographical realm also influenced amphibian’s range shifts, with the most Nearctic and Afrotropic species projected to expand their geographical ranges in response to global warming. It is possible that the environmental conditions that the Afrotropic species are adapted to will expand in future climate scenarios. However, this is one of the realms with the lowest number of evaluated species. In total, 21 species were studied, representing only 2.1% of the region’s amphibian richness (Vallan et al., 2004). On the other hand, species from the Neotropics are projected to have the highest proportion of area loss among all realms. Neotropics is the realm with the largest number of species studied (170). However, harboring nearly 2,916 species (Bolaños et al., 2008), only a small fraction of the richness of the region (5.6%) was represented by the studies. Therefore, there is a huge amount of species that need to be assessed to allow one to indicate the realm with most species vulnerable to climate change.
Besides identifying spatial patterns of range shift at the realm level, we also detected them at the “regional” level. For example, in some regions such as southwestern Russia, north-central Mexico, the northern United States, and the north-central Andean Mountain range, all of the species studied are only decreasing in area. On the other hand, in southern Canada, the southeastern and northeastern United States, the Brazilian Cerrado, and the far south of the Atlantic Forest in Brazil we observed a great increase in the geographic range size of some amphibian species. The projected expansion of the distribution of these species could lead to biotic homogenization at the community level, as specialists can be extirpated from the environment due to competition with the “winning” species (McKinney and Lockwood, 1999; Clavel et al., 2011).
Our study identifies regions with greatest potential for large-scale conservation due to the presence of several vulnerable species, such as those that inhabit mountain and forest regions. An effective conservation strategy for these species can be the creation of ecological corridors or small reserves. This strategy can reduce the risk of local extinction, as it allows species affected by climate change to move through the landscape and colonize new suitable areas (Ovaskainen, 2002). For species projected to maintain their suitable ranges in the future, a more effective strategy may be to create larger and more widely spaced conservation areas that allow large populations to persist under climate change. Therefore, climate change requires different conservation strategies aimed at the different responses that it can generate (Hannah, 2010).
We conclude that biogeographic variables may be good predictors of the climate change effects on amphibians’ distribution. Our results suggest that the baseline area isothermality, habitat type, elevation, and realm explains much of the variation in the intensity and direction of the climate change effect. However, our study shows that, globally, only a small fraction of Anura (3.9% of the 7,477) and Caudata (8% of the 773 species; Frost, 2022) species’ response to climate change have been assessed so far. Yet, we were not able to find a single study considering any of the 214 Gymnophiona species. Regions with high amphibian richness also remain underexplored, such as Madagascar, various parts of the African continent, Australia, Chile, Indonesia, and India. Therefore, we recommend that future studies focus on species and regions that are still underrepresented. Given that over 40% of amphibian species are already experiencing population declines due to other threats (IUCN, 2022), it is important to assess the impact of future climate scenarios on species distribution to optimize resources for conservation.
Data availability statement
The datasets and scripts generated for this study are deposited at Harvard Dataverse https://dataverse.harvard.edu/dataverse/climate_change_amphibians.
Author contributions
GA-F: conceptualization, data curation, formal analysis, investigation, methodology, software, validation, visualization, and writing—review and editing. DT and NH: conceptualization, formal analysis, investigation, methodology, project administration, supervision, validation, visualization, and writing—review and editing. MS: conceptualization, project administration, supervision, validation, visualization, writing—review and editing. MC-L: conceptualization, validation, visualization, and writing—review and editing. All authors contributed to the article and approved the submitted version.
Funding
This work was supported by the Coordenação de Aperfeiçoamento de Pessoal de Nível Superior–Brasil (CAPES) (Finance code 001) through a Doctoral Scholarship to GA-F and MC-L. NH acknowledges his post-doc fellowship received from CAPES. MS acknowledges funding through a research grant (309365/2019-8) from Conselho Nacional de Desenvolvimento Científico e Tecnológico (CNPq).
Acknowledgments
GA-F thanks the group Macroecologia e Conservação (MACON) at the Universidade Estadual de Santa Cruz (UESC) for their helpful comments that originated and improved the initial idea of this manuscript. GA-F also thanks Idea Wild for donating equipment used to develop this article. We are grateful for the comments made by two reviewers, which improved the quality of the manuscript. We also thank all authors who contributed data to our meta-analysis.
Conflict of interest
The authors declare that the research was conducted in the absence of any commercial or financial relationships that could be construed as a potential conflict of interest.
Publisher’s note
All claims expressed in this article are solely those of the authors and do not necessarily represent those of their affiliated organizations, or those of the publisher, the editors and the reviewers. Any product that may be evaluated in this article, or claim that may be made by its manufacturer, is not guaranteed or endorsed by the publisher.
Supplementary material
The Supplementary Material for this article can be found online at: https://www.frontiersin.org/articles/10.3389/fevo.2022.987237/full#supplementary-material
References
Alves-Ferreira, G., Giné, G. A. F., Fortunato, D., de, S., Solé, M., and Heming, N. M. (2022). Projected responses of Cerrado anurans to climate change are mediated by biogeographic character. Perspect. Ecol. Conserv. 20, 126–131. doi: 10.1016/j.pecon.2021.12.001
Araújo, M. B., Nogués-Bravo, D., Diniz-Filho, J. A. F., Haywood, A. M., Valdes, P. J., and Rahbek, C. (2008). Quaternary climate changes explain diversity among reptiles and amphibians. Ecography 31, 8–15. doi: 10.1111/j.2007.0906-7590.05318.x
Araújo, M. B., and Peterson, A. T. (2012). Uses and misuses of bioclimatic envelope modeling. Ecology 93, 1527–1539. doi: 10.1890/11-1930.1
Bates, D., Maechler, M., Bolker, B., and Steve Walker, S. (2015). Fitting linear mixed-effects models using lme4. J. Stat. Softw. 67, 1–48. doi: 10.18637/jss.v067.i01
Beissinger, S. R., and Riddell, E. A. (2021). Why are species traits weak predictors of range shifts? Annu. Rev. Ecol. Evol. Syst. 52, 47–66. doi: 10.1146/annurev-ecolsys-012021-092849
Blaustein, A. R., Walls, S. C., Bancroft, B. A., Lawler, J. J., Searle, C. L., and Gervasi, S. S. (2010). Direct and indirect effects of climate change on amphibian populations. Diversity 2, 281–313. doi: 10.3390/d2020281
Bolaños, F., Castro, F., Cortez, C., De la Riva, I., Hedges, B., Heyer, R., et al. (2008). “Amphibians of the neotropical realm,” in Threatened amphibians of the world, eds S. N. Stuart, M. Hoffmann, J. S. Chanson, N. A. Cox, R. J. Berridge, P. Ramani, et al. (Barcelona: Lynx Edicions), 92–105.
Borges, F. J. A., Ribeiro, B. R., Lopes, L. E., and Loyola, R. (2019). Bird vulnerability to climate and land use changes in the Brazilian Cerrado. Biol. Conserv. 236, 347–355. doi: 10.1016/j.biocon.2019.05.055
Bozinovic, F., Calosi, P., and Spicer, J. I. (2011). Physiological correlates of geographic range in animals. Annu. Rev. Ecol. Evol. Syst. 42, 155–179. doi: 10.1146/annurev-ecolsys-102710-145055
Büchi, L., and Vuilleumier, S. (2014). Coexistence of specialist and generalist species is shaped by dispersal and environmental factors. Am. Nat. 183, 612–624. doi: 10.1086/675756
Buckley, L. B., and Huey, R. B. (2016). How extreme temperatures impact organisms and the evolution of their thermal tolerance. Integr. Comp. Biol. 56, 98–109. doi: 10.1093/icb/icw004
Burnham, K. P., and Anderson, D. R. (2002). Model selection and multi-model inference: A practical information-theoretic approach. New York, NY: Springer.
Cardoso da Silva, J. M., Cardoso de Sousa, M., and Castelletti, C. H. M. (2004). Areas of endemism for passerine birds in the Atlantic forest, South America. Glob. Ecol. Biogeogr. 13, 85–92. doi: 10.1111/j.1466-882X.2004.00077.x
Clavel, J., Julliard, R., and Devictor, V. (2011). Worldwide decline of specialist species: Toward a global functional homogenization? Front. Ecol. Environ. 9, 222–228. doi: 10.1890/080216
Collins, J. P. (2010). Amphibian decline and extinction: What we know and what we need to learn. Dis. Aquat. Organ. 92, 93–99. doi: 10.3354/dao02307
Denny, M. W., Hunt, L. J. H., Miller, L. P., and Harley, C. D. G. (2009). On the prediction of extreme ecological events. Ecol. Monogr. 79, 397–421. doi: 10.1890/08-0579.1
Diniz-Filho, J. A. F., and Bini, L. M. (2007). Macroecology, global change and the shadow of forgotten ancestors. Glob. Ecol. Biogeogr. 17, 11–17. doi: 10.1111/j.1466-8238.2007.00339.x
Evans, A., and Jacquemyn, H. (2022). Range size and niche breadth as predictors of climate-induced habitat change in Epipactis (Orchidaceae). Front. Ecol. Evol. 10:894616. doi: 10.3389/fevo.2022.894616
Fedorov, S. (2013). Getdata graph digitizer. Available online at: http://getdata-graph-digitizer.com (accessed June 1, 2022).
Fick, S. E., and Hijmans, R. J. (2017). Worldclim 2: New 1-km spatial resolution climate surfaces for global land areas. Int. J. Climatol. 37, 4302–4315. doi: 10.1002/joc.5086
Fjeldså, J. (1994). Geographical patterns for relict and young species of birds in Africa and South America and implications for conservation priorities. Biodivers. Conserv. 3, 207–226. doi: 10.1007/BF00055939
Foden, W. B., Butchart, S. H. M., Stuart, S. N., Vié, J.-C., Akçakaya, H. R., Angulo, A., et al. (2013). Identifying the world’s most climate change vulnerable species: A systematic trait-based assessment of all birds, amphibians and corals. PLoS One 8:e65427. doi: 10.1371/journal.pone.0065427
Freitas, V., Cardoso, J. F. M. F., Lika, K., Peck, M. A., Campos, J., Kooijman, S. A. L. M., et al. (2010). Temperature tolerance and energetics: A dynamic energy budget-based comparison of North Atlantic marine species. Philos. Trans. R. Soc. B Biol. Sci. 365, 3553–3565. doi: 10.1098/rstb.2010.0049
Frost, D. (2022). Amphibian species of the world: An online reference. Version 6.1. Available online at: https://amphibiansoftheworld.amnh.org/ (accessed May 15, 2022).
GBIF.org. (2022). GBIF occurrence download. Available online at: https://www.gbif.org (accessed May 25, 2022).
Gentry, A. H. (1995). “Diversity and floristic composition of neotropical dry forests,” in Seasonally dry tropical forests, eds S. H. Bullock, H. A. Mooney, and E. Medina (New York, NY: Cambridge University Press), 146–194. doi: 10.1017/CBO9780511753398.007
Ghalambor, C. K. (2006). Are mountain passes higher in the tropics? Janzen’s hypothesis revisited. Integr. Comp. Biol. 46, 5–17. doi: 10.1093/icb/icj003
Goodman, L. A., and Kruskal, W. H. (1972). Measures of association for cross classifications, IV: Simplification of asymptotic variances. J. Am. Stat. Assoc. 67, 415–421. doi: 10.1080/01621459.1972.10482401
Gouveia, S. F., Hortal, J., Cassemiro, F. A. S., Rangel, T. F., and Diniz-Filho, J. A. F. (2013). Nonstationary effects of productivity, seasonality, and historical climate changes on global amphibian diversity. Ecography 36, 104–113. doi: 10.1111/j.1600-0587.2012.07553.x
Hannah, L. (2010). A global conservation system for climate-change adaptation. Conserv. Biol. 24, 70–77. doi: 10.1111/j.1523-1739.2009.01405.x
Harrison, S., and Noss, R. (2017). Endemism hotspots are linked to stable climatic refugia. Ann. Bot. 119, 207–214. doi: 10.1093/aob/mcw248
Hijmans, J. R. (2022). terra: Spatial data analysis. R package version 1.6-7. Available online at: https://CRAN.R-project.org/package=terra (accessed May 10, 2022).
Holt, R. D. (1990). The microevolutionary consequences of climate change. Trends Ecol. Evol. 5, 311–315. doi: 10.1016/0169-5347(90)90088-U
Huey, R. B., Deutsch, C. A., Tewksbury, J. J., Vitt, L. J., Hertz, P. E., Álvarez Pérez, H. J., et al. (2009). Why tropical forest lizards are vulnerable to climate warming. Proc. R. Soc. B Biol. Sci. 276, 1939–1948. doi: 10.1098/rspb.2008.1957
IPCC (2022). “Climate change 2022: Impacts, adaptation and vulnerability,” in Contribution of Working Group II to the Sixth Assessment Report of the Intergovernmental Panel on Climate Change, eds H.-O. Pörtner, D. C. Roberts, M. Tignor, E. S. Poloczanska, K. Mintenbeck, A. Alegría, et al. (Cambridge: Cambridge University Press), 3056. doi: 10.1017/9781009325844
IUCN (2022). The IUCN red list of threatened species. Version 2022-1. Available online at: https://www.iucnredlist.org (accessed June 20, 2022).
Loyola, R. D., Becker, C. G., Kubota, U., Haddad, C. F. B., Fonseca, C. R., and Lewinsohn, T. M. (2008). Hung out to dry: Choice of priority ecoregions for conserving threatened neotropical anurans depends on life-history traits. PLoS One 3:e2120. doi: 10.1371/journal.pone.0002120
McKinney, M. L., and Lockwood, J. L. (1999). Biotic homogenization: A few winners replacing many losers in the next mass extinction. Trends Ecol. Evol. 14, 450–453. doi: 10.1016/s0169-5347(99)01679-1
Menéndez-Guerrero, P. A., Green, D. M., and Davies, T. J. (2020). Climate change and the future restructuring of neotropical anuran biodiversity. Ecography 43, 222–235. doi: 10.1111/ecog.04510
Mokhatla, M. M., Kemp, G., Rödder, D., and Measey, G. J. (2015). Assessing the effects of climate change on distributions of cape floristic region amphibians. S. Afr. J. Sci. 111, 1–7. doi: 10.17159/sajs.2015/20140389
Murphy, P. G., and Lugo, A. E. (1986). Ecology of tropical dry forest. Annu. Rev. Ecol. Syst. 17, 67–88. doi: 10.1146/annurev.es.17.110186.000435
Nori, J., Moreno Azócar, D. L., Cruz, F. B., Bonino, M. F., and Leynaud, G. C. (2016). Translating niche features: Modelling differential exposure of Argentine reptiles to global climate change. Austral Ecol. 41, 367–375.
Oliveira, B. F., São-Pedro, V. A., Santos-Barrera, G., Penone, C., and Costa, G. C. (2017). AmphiBIO, a global database for amphibian ecological traits. Sci. Data 4:170123. doi: 10.1038/sdata.2017.123
Olson, D. M., Dinerstein, E., Wikramanayake, E. D., Burgess, N. D., Powell, G. V. N., Underwood, E. C., et al. (2001). Terrestrial ecoregions of the world: A new map of life on earth. Bioscience 51, 933–938.
Ovaskainen, O. (2002). Long-term persistence of species and the SLOSS problem. J. Theor. Biol. 218, 419–433. doi: 10.1006/jtbi.2002.3089
Parmesan, C. (2006). Ecological and evolutionary responses to recent climate change. Annu. Rev. Ecol. Evol. Syst. 37, 637–669. doi: 10.1146/annurev.ecolsys.37.091305.110100
Pörtner, H. O., and Knust, R. (2007). Climate change affects marine fishes through the oxygen limitation of thermal tolerance. Science 315, 95–97. doi: 10.1126/science.1135471
R Core Team. (2022). R: A language and environment for statistical computing. Vienna: R Foundation for Statistical Computing. Available online at: https://www.R-project.org
Rubalcaba, J. G., and Olalla-Tárraga, M. Á (2020). The biogeography of thermal risk for terrestrial ectotherms: Scaling of thermal tolerance with body size and latitude. J. Anim. Ecol. 89, 1277–1285. doi: 10.1111/1365-2656.13181
Saupe, E. E., Qiao, H., Hendricks, J. R., Portell, R. W., Hunter, S. J., Soberón, J., et al. (2015). Niche breadth and geographic range size as determinants of species survival on geological time scales. Glob. Ecol. Biogeogr. 24, 1159–1169. doi: 10.1111/geb.12333
Seebacher, F., Elsey, R. M., and Trosclair, P. L. III (2003). Body temperature null distributions in reptiles with nonzero heat capacity: Seasonal thermoregulation in the American Alligator (Alligator mississippiensis). Physiol. Biochem. Zool. 76, 348–359. doi: 10.1086/375426
Seebacher, F., Grigg, G. C., and Beard, L. A. (1999). Crocodiles as dinosaurs: Behavioral thermoregulation in very large ectotherms leads to high and stable body temperatures. J. Exp. Biol. 202, 77–86. doi: 10.1242/jeb.202.1.77
Sillero, N., Arenas-Castro, S., Enriquez-Urzelai, U., Vale, C. G., Sousa-Guedes, D., Martínez-Freiría, F., et al. (2021). Want to model a species niche? A step-by-step guideline on correlative ecological niche modelling. Ecol. Model. 456:109671. doi: 10.1016/j.ecolmodel.2021.109671
Slatyer, R. A., Hirst, M., and Sexton, J. P. (2013). Niche breadth predicts geographical range size: A general ecological pattern. Ecol. Lett. 16, 1104–1114. doi: 10.1111/ele.12140
Sodhi, N. S., Bickford, D., Diesmos, A. C., Lee, T. M., Koh, L. P., Brook, B. W., et al. (2008). Measuring the meltdown: Drivers of global amphibian extinction and decline. PLoS One 3:e1636. doi: 10.1371/journal.pone.0001636
Struecker, B., and Milanovich, J. (2017). Predicted suitable habitat declines for Midwestern United States amphibians under future climate change and land-use change scenarios. Herpetol. Conserv. Biol. 12, 635–654.
Stuart, S. N., Chanson, J. S., Cox, N. A., Young, B. E., Rodrigues, A. S. L., Fischman, D. L., et al. (2004). Status and trends of amphibian declines and extinctions worldwide. Science 306, 1783–1786. doi: 10.1126/science.1103538
Taylor, E. N., Diele-Viegas, L. M., Gangloff, E. J., Hall, J. M., Halpern, B., Massey, M. D., et al. (2020). The thermal ecology and physiology of reptiles and amphibians: A user’s guide. J. Exp. Zool. A Ecol. Integr. Physiol. 335, 13–44. doi: 10.1002/jez.2396
Tewksbury, J. J., Huey, R. B., and Deutsch, C. A. (2008). Putting the heat on tropical animals. Science 320, 1296–1297. doi: 10.1126/science.1159328
Toranza, C., Brazeiro, A., and Maneyro, R. (2016). Anfibios amenazados de Uruguay: Efectividad de las áreas protegidas ante el cambio climático. Ecol. Austral 26, 138–149. doi: 10.25260/EA.16.26.2.0.136
Urbina-Cardona, J. N., and Loyola, R. D. (2008). Applying niche-based models to predict endangered-hylid potential distributions: Are neotropical protected areas effective enough? Trop. Conserv. Sci. 1, 417–445.
Vallan, D., Andreone, F., Raherisoa, V. H., and Dolch, R. (2004). Does selective wood exploitation affect amphibian diversity? The case of An’Ala, a tropical rainforest in eastern Madagascar. Oryx 38, 410–417. doi: 10.1017/S003060530400078X
Vasconcelos, T. S., Nascimento, B. T. M., and Prado, V. H. M. (2018). Expected impacts of climate change threaten the anuran diversity in the Brazilian hotspots. Ecol. Evol. 8, 7894–7906. doi: 10.1002/ece3.4357
Whitton, F. J. S., Purvis, A., Orme, C. D. L., and Olalla-Tárraga, M. Á (2012). Understanding global patterns in amphibian geographic range size: Does Rapoport rule? Glob. Ecol. Biogeogr. 21, 179–190. doi: 10.1111/j.1466-8238.2011.00660.x
Wilson, O. J., Walters, R. J., Mayle, F. E., Lingner, D. V., and Vibrans, A. C. (2019). Cold spot microrefugia hold the key to survival for Brazil’s critically endangered Araucaria tree. Glob. Chang. Biol. 25, 4339–4351. doi: 10.1111/gcb.14755
Winter, M., Fiedler, W., Hochachka, W. M., Koehncke, A., Meiri, S., and De la Riva, I. (2016). Patterns and biases in climate change research on amphibians and reptiles: A systematic review. R. Soc. Open Sci. 3:160158. doi: 10.1098/rsos.160158
Zank, C., Becker, F. G., Abadie, M., Baldo, D., Maneyro, R., and Borges-Martins, M. (2014). Climate change and the distribution of neotropical red-bellied toads (Melanophryniscus, anura, amphibia): How to prioritize species and populations? PLoS One 9:e94625. doi: 10.1371/journal.pone.0094625
Zeisset, I., and Beebee, T. J. C. (2008). Amphibian phylogeography: A model for understanding historical aspects of species distributions. Heredity 101, 109–119. doi: 10.1038/hdy.2008.30
Keywords: systematic review, Anura, Caudata, global warming, suitable area, Ecological Niche Model (ENM), species distribution
Citation: Alves-Ferreira G, Talora DC, Solé M, Cervantes-López MJ and Heming NM (2022) Unraveling global impacts of climate change on amphibians distributions: A life-history and biogeographic-based approach. Front. Ecol. Evol. 10:987237. doi: 10.3389/fevo.2022.987237
Received: 05 July 2022; Accepted: 28 October 2022;
Published: 17 November 2022.
Edited by:
Rafael Arruda, Federal University of Mato Grosso, BrazilReviewed by:
Brunno Oliveira, University of California, Davis, United StatesLuisa Maria Diele Viegas, Federal University of Bahia, Brazil
Copyright © 2022 Alves-Ferreira, Talora, Solé, Cervantes-López and Heming. This is an open-access article distributed under the terms of the Creative Commons Attribution License (CC BY). The use, distribution or reproduction in other forums is permitted, provided the original author(s) and the copyright owner(s) are credited and that the original publication in this journal is cited, in accordance with accepted academic practice. No use, distribution or reproduction is permitted which does not comply with these terms.
*Correspondence: Gabriela Alves-Ferreira, Z2FicmllbGEtYWx2ZXM3N0Bob3RtYWlsLmNvbQ==