- 1Department of Biology, Université du Québec à Rimouski, Rimouski, QC, Canada
- 2Department of Biology, Cégep de Rimouski, Rimouski, QC, Canada
Decreased productivity in long-lived bird species is linked to prey depletion in marine ecosystems. Seabirds, however, exhibit behavioral flexibility at individual level to prevent this outcome. One such strategy to alleviate any impact on fitness would be to divorce from their partners. Although changing mates and increasing foraging effort have been shown to increase or maintain reproductive success, how the behavioral flexibility affects fundamental physiological parameters remains to be elucidated. Here, we compared physiological components (nutritional status, muscle damage and oxidative stress) of northern gannets (Morus bassanus) in relation to their partnership status and foraging effort. Specifically, we used a cross-sectional data set (at the population level) of three contrasted years to compare retained and changed mates. We predicted that mate change is a stressful event with impacts on health condition and those effects are higher during unfavorable years with food depletion. Our study showed that gannets changing mate increase parental effort only during years of low food abundance, with consequences on health condition (increased body mass loss, higher protein catabolism and higher oxidative damage during chick rearing period). Ultimately, our study suggests that partnership decision is not likely to reduce the long-term quality and the fitness of parents. Reproduction during harsh conditions would however likely be one of the primary causes of individual quality loss and fitness decline in this long-lived bird species.
Introduction
Seabirds exhibit considerable ecological, demographic, and behavioral adjustment capacity (e.g., Garthe et al., 1996; Weimerskirch, 2002; Hamer et al., 2007; Guillemette et al., 2018) to respond to both short—and long-term changes in ocean conditions (e.g., Aebischer et al., 1990; Sydeman et al., 2009), via different strategies as behavioral flexibility (Williams et al., 2008). For example, parents may modify their attendance and foraging behavior under conditions of low prey availability (Piatt et al., 2007). In optimal conditions, seabirds mates share parental effort to protect and feed their chicks (Bried and Jouventin, 2002) and foraging effort vary according to their breeding stage (incubation, chick rearing) (Shaffer et al., 2003). During food resources reduction, both parents must forage simultaneously (Regehr and Montevecchi, 1997) and they can increase foraging distance (Guillemette et al., 2018). This behavioral adjustment may compromise breeding success as they must leave their offspring unattended. Thus, the usual benefit of breeding at high density as an anti-predator strategy (Danchin and Wagner, 1997) may be reduced. Moreover, poor breeding performance may negatively influence the occurrences of a pair reunion the next year (but see Choudhury, 1995; Ens et al., 1996; Taborsky and Taborsky, 1999; Bried and Jouventin, 2002; Dubois and Cézilly, 2002).
Divorce has been recorded in 92% of socially monogamous birds (Jeschke and Kokko, 2008), with divorce rates being highly variable both among species and within species (Black, 1996). Many hypotheses have been suggested concerning the costs and benefits associated with mate retention versus divorce (Choudhury, 1995). Because biparental care is essential in the majority of monogamous bird species (Bennett and Owens, 2002), divorce has frequently been suggested to represent a way to correct for suboptimal partnerships, which may result from different types of constraints on initial mate choice (Johnston and Ryder, 1987; Moller, 1992; Sullivan, 1994; Choudhury, 1995; Botero and Rubenstein, 2012) or to secure genetically more diverse offspring [see references in Arnqvist and Kirkpatrick (2005)]. Divorce has been proposed to be an adaptive strategy where at least one member of a pair increases its breeding success (see review by Choudhury, 1995). According to this hypothesis, partner change represents a form of adaptive choice triggered either by low breeding success as a result of poor conditions (Dubois and Cézilly, 2002) or by the potential to improve breeding success with a new partner (Choudhury, 1995; Black, 1996), or both. In northern gannets (Morus bassanus, hereafter gannets), divorce rate is negatively correlated with breeding success and prey biomass stocks (Pelletier and Guillemette, 2022). Gannets that change mates increase the probability of breeding the following year. It has been suggested that divorce could be an example of behavioral flexibility to counteract food depletion and breeding failure (Pelletier and Guillemette, 2022). However, this individual’s decision-mating process with short-term benefits could also have disadvantages when re-mating with a new partner.
Beginning a partnership with a new mate is associated with different kinds of costs. As described by Ens et al. (1996), at least four different kinds of costs may be envisaged: costs for searching for a new mate, for fighting rivals, for ending up with a poorer mate, and costs related to an initial inefficiency in reproduction with a new mate. At the individual level, these consequences may increase physiological stress, particularly if an individual of an obligate biparental care species must compensate for decreased parental effort by their partner (Houston et al., 2005). In an experimental study, the nest visitation rate as proxy of parental effort of starlings (Sturnus vulgaris) has been decreased (by attaching small weights to the base of a bird’s tail feathers), followed by an increase in the level of care by its partner (Wright and Cuthill, 1989). Such parental compensation observed in many species shows that parents respond to each other’s behavior (Harrison et al., 2009), and, because parental care involves a high level of energy expenditure, any augmentation in parental (or foraging) effort might reduce the health condition of the parent at the end of the breeding season.
Although reproduction is one of the most studied phases of the avian life cycle, knowledge on the physiological underpinnings of parental care and its key component (foraging behavior) brings varied results (Williams, 2012; Williams and Fowler, 2015; Williams, 2018). Some studies found evidence that adults with higher level of parental investment result in higher level of oxidative stress (Norte et al., 2010; Guindre-Parker and Rubenstein, 2018), while others found no such relationship (Beaulieu et al., 2011; Wegmann et al., 2015). According to Williams (2018), reproductive trade-offs should only be expected under food stress (Stearns, 1992), but it is often unclear in studies if parents are “food stressed” or not.
During the last decades, physiological condition attributed to stress and related to parental effort has been measured in terms of body mass (BM, decrease, Hillstrom, 1995; Merila and Wiggins, 1997; Gebhardt-Henrich et al., 1998), immune function (decrease, Deerenberg et al., 1997; Nordling et al., 1998; Cichoń et al., 2001; Hasselquist et al., 2001), leukocyte counts (modification and decline, Gustafsson et al., 1994; Dubiec et al., 2005), hematocrit (decrease, Moreno et al., 2002), heterophil/lymphocyte ratio (increase, Ots and Horak, 1996; Davis et al., 2008), plasma triglycerides (TRIG, decrease, Masello and Quillfeldt, 2004), oxidative stress (increase, Norte et al., 2010; Romero-Haro et al., 2016), antioxidant protection (decrease, Alonso-Alvarez et al., 2004), and telomere dynamics (telomere attrition, Monaghan, 2014). Nevertheless, few studies combined biomarkers to evaluate the physiological condition attributed to parental effort (but see González-Medina et al., 2015; Fowler et al., 2018), and to our knowledge, no study evaluated the physiological condition associated with partnership status and mate change.
To ascertain the relationship between partnership status, parental effort, nutritional status, and oxidative stress in a free-living and long-lived bird species, we measured and compared 15 behavioral and physiological variables among individuals that retained their mates and others that changed their mates (including lost mates and divorced). We used cross-sectional tests (for interindividual comparison) with data recorded in northern gannets during three subsequent and highly contrasted years in terms of population breeding success and food conditions. We evaluated partnership status with a long-term monitoring program (breeding success, body mass and body mass variation of ringed individuals). We used GPS tracking to evaluate parental effort (as foraging behavior at sea). We sampled blood to measure physiological biomarkers of health condition: nutritional status (plasma glucose, triglycerides, beta-hydroxybutyrate, total protein, albumin [A] and globulin [G], uric acid concentration, A/G ratio), muscle damage (creatine kinase activity), and oxidative stress (thiobarbituric acid reactive substances and total antioxidant capacity).
We tested two main hypotheses. First, behavioral flexibility expressed as mate change increases parental investment and affects the physiological status of adults compared to individuals that retain their partner (H1). Second, the negative impact of mate change occurs only during unfavorable years associated with low breeding success and food stress (H2). According to life-history theory (Stearns, 1992) and the literature cited above, we generated the following predictions: changed mates compared to retained mates (P1) increase foraging behavior at sea due to parental compensation and mate adjustment, (P2) increase their nutritional stress (expressed as decrease in glucose, triglycerides, total protein, albumin, and increase in beta-hydroxybutyrate and uric acid) mostly during unfavorable years, (P3) increase their muscle damage (expressed as increase in creatine kinase activity), (P4) increase their oxidative damage, and (P5) decrease their plasma total antioxidant capacity.
Methods
Study site
The fieldwork was conducted on Bonaventure Island (48°30′ N, 64°09′ W) located in the Parc national de l’Île-Bonaventure-et-du-Rocher-Percé in the Gulf of St. Lawrence (Québec, Canada) where a long-term study started in 2008. This colony holds approximately 50,000 pairs of breeding gannets as well as several thousand immatures. This is the largest gannet colony in Canada. Annually, this colony is monitored, for partnership status and breeding success from incubation to the chick rearing period (from May to August or September) within 180 to 184 nests in four plots in the peripheral section of the colony. Gannets in this population are known to have a highly variable divorce rate between 2009 and 2019 (22 ± 3%, range: 13–46%) that is influenced by the previous year’s breeding success. During this same period, breeding success was low and variable (32 ± 5%, range: 3–60%) (Pelletier and Guillemette, 2022). Breeding success of the colony is estimated as the number of fledged chicks divided by the number of nests with eggs. Gannets were caught using a noose-pole and all methods were approved by the Animal Care Committee (ACC) of the Université du Québec à Rimouski and complied with the guidelines of the Canadian Council on Animal Care (CCAC). These birds were marked with a U.S. Fish and Wildlife Service steel ring with an alphanumeric coded and colored plastic band (permit numbers SC25, RE-27, 10,704) with approval by Société des établissements de plein air du Québec (permit numbers PNIBRP-2008-001 to PNIBRP-2019-001). Presence/absence of individually banded gannets were established twice daily from the end of May (before hatching) to the end of August (or September), from 2008 to 2019. We identified established partnerships in the set of monitored nests and an intensive coverage of the study area and surrounding sectors were done to ensure that we resighted all live breeding birds. Because gannets have a strong breeding philopatry (Nelson, 2002), absence of a partner was considered as a death or a skip of the current reproduction year.
Fieldwork, GPS loggers and sex determination
We captured individuals once or twice during the breeding season (incubation and chick rearing periods) and body mass (BM) was measured. When two BM measurements were made, the difference between the two was calculated (body mass variation [BMvar], averaging 27 ± 7 days).
We collected breast feathers, and birds were fitted with a GPS logger. Feathers were taken for sex determination analysis and blood samples, for biomarkers analysis. A small amount of blood (less than 5 ml, approx. 0.16% volume/weight) was drawn from the medial metatarsal vein of each bird using butterfly needles and BD Vacutainer™ tubes with lithium heparin. In the field, blood samples were centrifuged (5 min; 3,900 rpm). Plasma and blood cells phases were separated in cryotubes and were stored immediately in liquid nitrogen, and subsequently in a − 80°C freezer until biomarkers analyses.
Birds were tagged with a GPS tracker iGotU GT-120 (Mobile Action Technology, 34 g) or CatLog Gen 2 (Perthold Engineering, 34 g) set to record at 10 min intervals. Tags were attached to three to four central tail feathers using two strips of a 50 mm wide waterproof Tesa tape (no 4651). Total deployment weight was 1.0–1.2% of adult body mass (mean ± SEM: 2993 ± 23 g). The birds were immediately released near their nesting site after blood sampling. Handling time was kept to a maximum of 15 min. All birds showed no signs of immediate adverse effects.
For sex determination, four thoracic covert feathers were plucked and stored in plastic bags at −20°C until subsequent DNA analysis as described in Pelletier and Guillemette (2022).
Blood analysis
Nutritional status and muscle damage
We evaluated nutritional status (fasting/starvation levels) by measuring glucose (GLU), triglycerides (TRIG), uric acid (URIC), total protein (TP), albumin (ALB), globulins (GLOB) concentrations in plasma and albumin/globulin ratio (A/G ratio) with an IDEXX Catalyst Dx™. We evaluated muscle damage by measuring creatine kinase (CK) activity in plasma with the same device. We followed the manufacturer’s instructions (IDEXX, 2015) with a dilution of 2:3 (100 µL of plasma into 50 µL of phosphate buffered saline, pH 7,4). Birds maintain higher plasma GLU concentrations than other vertebrates of similar body mass and, contrary to mammals, these elevated concentrations do not promote oxidative stress and tissue damage, due to higher antioxidant capacity (Cohen and McGraw, 2009). GLU levels are normally maintained high and seem to be independent of breeding or molting stages (e.g., in mallards Anas platyrynchos, Fairbrother et al., 1990) and of fasting duration during breeding (e.g., in penguins species, Groscolas, 1986; Vleck and Vleck, 2002). TRIG is a marker of fatty acid anabolism and fat deposition. URIC is a product of protein catabolism and fasting or rapid body mass loss can elevate URIC levels. TP concentration comprises all the proteins found in the plasma phase where ALB is the single major component. The remaining proteins are the alpha, beta, and gamma GLOB that increase in chronic infections or inflammatory diseases (Lumeij, 1987). A TP increase may indicate dehydration or inflammatory disease. ALB plays important roles in the transport of endogenous and exogenous compounds by binding with those compounds, in osmoregulation and in antioxidant capacity (Harr, 2006; Harrison et al., 2006; Hochleithner, 2013). Beta-hydroxybutyrate (BHB), a ketone body, is a marker of fatty acid catabolism during fasting (Vleck and Vleck, 2002) and it was measured (in triplicate) with a commercial colorimetric assay kit (Beta-Hydroxybutyrate [Ketone Body] Colorimetric Assay Kit, Cayman Chemical Assay, USA), with a dilution of 1:10 (30 µL plasma and 270 µL assay buffer) and with an EnVision Multimode Microplate Reader (reading at 450 nm). CK is found at high activity only in the cytoplasm of cardiac and skeletal muscle. An increase in plasma CK identifies a muscle injury due to muscle protein mobilization caused by trauma or vigorous exercise (Knuth and Chaplin, 1994; Guglielmo et al., 2001).
Oxidative stress
We measured one biomarker commonly used of oxidative damage (TBARS) and one biomarker of antioxidant protection (TAC). Oxidative lipid damage, or lipid peroxidation, produces different metabolites found in plasma. Malondialdehyde (MDA) is one of them and is produced rapidly after a cellular burst of reactive oxygen species (ROS). MDA is detected and monitored by the measurement of TBARS as a general quantification of oxidative damage molecules that occur in the plasma. This assay is based on the formation of a MDA-TBA adducted by the reaction of MDA and TBA under high temperature and acidic conditions, which generates a color directly proportional to the concentration of oxidative damage molecules (Uchiyama and Mihara, 1978). First, 17 µL of each plasma sample (dilution factor: 2.94) or 50 µl of standard was added to 50 µL of sodium dodecyl sulfate (8.1%), 375 µL of thiobarbituric acid (the color reagent, 0.8%), 375 µL of acetic acid (20%), and distilled water (183 μL for plasma or 150 µL for standard) into 2 mL tubes, and mixed. Tubes were capped and kept in a hotplate at 95–100°C for 1 h. After 1 h, the tubes were removed from the hotplate and immediately put onto ice for 10 min to stop the reaction. Tubes were centrifuged 10 min at 1600×g at 4°C. Finally, 250 μL of each solution (in triplicate) was randomly pipetted in well plates and readings were taken at 530 nm with an EnVision Multimode Microplate Reader. Standard curves were obtained from serial dilutions of a standard of MDA (from 0 to 12.5 μM). Considering that TBARS can be altered by the lipid content of the sample, TBARS were corrected for TRIG concentration in plasma with TBARS/TRIG = TBARSt. The mean ± standard deviation (SD) intraplate coefficient of variation was 3.4 ± 2.7% and the interplates coefficient of variation was 3.4%.
We estimated non-enzymatic TAC by measuring the capacity of a plasma sample to quench a standardized free radical challenge. Plasma TAC includes macromolecules such as albumin, ceruloplasmin, and ferritin, and small molecules including ascorbic acid, α-tocopherol, β-carotene, uric acid and bilirubin. TAC was determined using a colorimetric assay kit (Cayman Chemicals, USA) and EnVision Multimode Microplate Reader (reading at 750 nm). We used kit instructions for measurement of TAC (in triplicate) with a dilution of 1:20 (10 μL of plasma into 190 μL of buffer). Plasma TAC values are expressed as Trolox-equivalent antioxidant concentrations. Trolox is a water-soluble tocopherol analog. In some avian species, as TAC is influenced by the antioxidant effects of URIC (Cohen and McGraw, 2009) and by ALB (Cohen et al., 2007; Roche et al., 2008), we verified predictive capacity of plasma URIC and ALB concentrations for plasma TAC with linear mixed model with URIC and ALB as covariates. The mean ± SD intraplate coefficient of variation was 2.0 ± 2.2%, and the interplates coefficient of variation was 2.4%.
Data analysis
Partnership status
Three categories were determined for partnership status: retained mates, divorced, and lost mates. Mate retention occurred when partners are together at year and . A divorce is said to have occurred when two birds that bred together in year t-l are alive and present in the colony in year , but not breeding together. Mate loss occurred when one partner is absent in our study plot (Coulson, 1966; Harris et al., 1987; Ens et al., 1993; Choudhury, 1995). We used the same dataset of partnership status as Pelletier and Guillemette (2022) in which they monitored a total of 809 birds from 2008 to 2019. In the present study, we used only two categories: “retained mates” and “changed mates” in which we grouped lost mates and divorced due to the low number of lost mates and because we wanted to investigate first the effect of parental effort after mating with a new partner.
GPS
We used GPS locations to describe foraging behavior and estimate foraging trip range and distance. A complete foraging trip begins when an individual goes outside Bonaventure Island, a polygon delimited by these coordinates: 48.483 N, 48.507 N, 64.144 W, 64.178 W, during at least 30 min and ends when it comes back inside the polygon. We excluded incomplete trips. Foraging trip range was calculated with the maximal Euclidean distance on a spheroid between the last GPS location at the colony before the foraging trip as reference (GPS location with latitude 1 and longitude 1) and each GPS location at sea with this formula: arccos(sin[latitude1]×sin[latitude2]+cos[latitude1]×cos[latitude2]×cos[latitude2]-[latitude1]×6371. Foraging trips were recorded as time series of the successive continuous positions (with latitude and longitude) of the gannet at time t. Distance between each position, the step length, was calculated with the same Euclidean distance formula above. Foraging trip distance was the total cumulative distance of each step length recorded in a foraging trip (Grémillet et al., 2004; Pichegru et al., 2007).
Statistical analysis
All statistical analyses were conducted using the statistical software R 4.0.3 (R Core Team, 2020). Plots were made with ‘ggplot2’ package (Wickham, 2016) and maps with ‘ggmap’ (Kahle and Wickham, 2013) and ‘sf’ (Pebesma, 2018) packages.
Because we could not follow the same individuals over 3 years for each behavioral and physiological biomarker, we conducted cross-sectional rather than longitudinal tests. Although longitudinal tests and intraindividual comparison are more robust (Pelletier et al., in prep.), cross-sectional tests and interindividual comparison have the advantage of producing larger sample size.
Linear mixed models were used to analyze the influence of partnership status (changed vs. retained mates), annual environmental condition for breeding (represented by the explanatory variable “year”), breeding stage (incubation, chick rearing or empty nest) and sex on each different independent variable (biomarkers). The function “lmer” (linear mixed models) in the package ‘lme4’ was used to run mixed-effect models (Bates et al., 2015). Independent variables were log-transformed and standardized to respect statistical test assumptions and to improve comparability with other studies (Verhulst, 2020). We systematically build a null model and different models including partnership status, year, breeding stage, sex and interactions (partnership status:year and/or partnership status:breeding stage) and bird ID as a random factor to avoid pseudoreplication.
We investigated the potential relationship between BM, BMvar., julian date and each biomarker to extract and use residuals eventually, when relationships were detected. Thus, we removed the effect of BM on TRIG, and the effect of julian date on BM, BMvar., A/G ratio and CK activity. We added URIC as covariate in models for TAC, knowing that they are perhaps correlated (Cohen et al., 2007; Costantini, 2011).
We derived all possible submodels from this set of predictors with the function ‘dredge’ in the package ‘MuMIn’ (Barton, 2009) and we calculated an Akaike information criterion value corrected for small sample (AICc) (Burnham and Anderson, 2002). Based on selection by the AICc, the most parsimonious model including partnership status (first objective of the study) with the lowest AICc among those falling within AICc <2 was retained for interpretation of each independent variable. We also used a likelihood ratio test (using the Chi square distribution) of the best model compared to a null model to determine if a set of predictors retained in the best model was significant factors in explaining variation of each independent variable. With the ‘summ’ function (‘jtools’ package, Long, 2020), models were fitted using the restricted maximum likelihood (REML) approach, p values were derived using the Satterthwaite approximations and variance explained by the linear mixed model was evaluated using a pseudo r-squared (Nakagawa et al., 2013). We also computed p values with ‘joint tests’ for comparison, based on conditional F-tests with Kenward-Roger approximation for the degrees of freedom. Normality of residuals and homogeneity of variances was confirmed visually for all models.
When required, and because sample sizes differed between predictor categories, we conducted post hoc tests with the Tukey method for comparing groups of 3 estimates with estimated marginal means (aka ‘least-squares means’) using the function ‘emmeans’ (‘emmeans’ package, Lenth, 2020). We used the Kenward-Roger and Satterhwaite approximations because they have been suggested as the best methods for deriving p-values when mixed models are fitted with REML because type 1 error rates are most optimal for different sample sizes (Luke, 2017). We also presented effect size (Supplementary Table S1) by calculating Cohen’d (Cohen, 1988) and using these categories: d = 0.1 for very small effect size, d = 0.2 for small effect size, d = 0.5 for medium effect size, d = 0.8 for large effect size, d = 1.2 for very large effect size, and d = 2.0 for huge effect size (Sawilowsky, 2009).
Considering that gannets’ breeding success is highly correlated with the abundance of their preferential prey (Guillemette et al., 2018), we used annual breeding success monitored in the colony as proxy of environmental conditions. From this, we qualified 2018 as a ‘good or favorable year’ (breeding success: 60%), 2019 as ‘bad or unfavorable year’ (breeding success: 13%), and 2017 as a ‘medium or intermediate year’ (breeding success: 41%).
All values are reported as mean ± standard error of the mean (SEM) in the text and mean ± 95% confidence interval (CI) in the figures.
Results
We tested the influence of partner change on parental effort (foraging effort) and health condition (body mass, nutritional status, muscle damage, oxidative stress) on a total of 68 northern gannets of which 35 in 2017, 38 in 2018, and 46 in 2019 were replicated. Partnership status recorded for these individuals varied between years: 7 birds changed their partner in 2017 (20%), 7 in 2018 (18%) and 16 in 2019 (35%). The breeding success of these individuals was similar to that of the colony: 36% in 2017, 49% in 2018 and 0% in 2019. Means (± SEM) of behavioral variables and physiological biomarkers are shown in Table 1, and the summary of the most parsimonious linear mixed models including partnership status and calculated for each behavioral and physiological variable are presented in Table 2 (see Supplementary Table S1 for details).
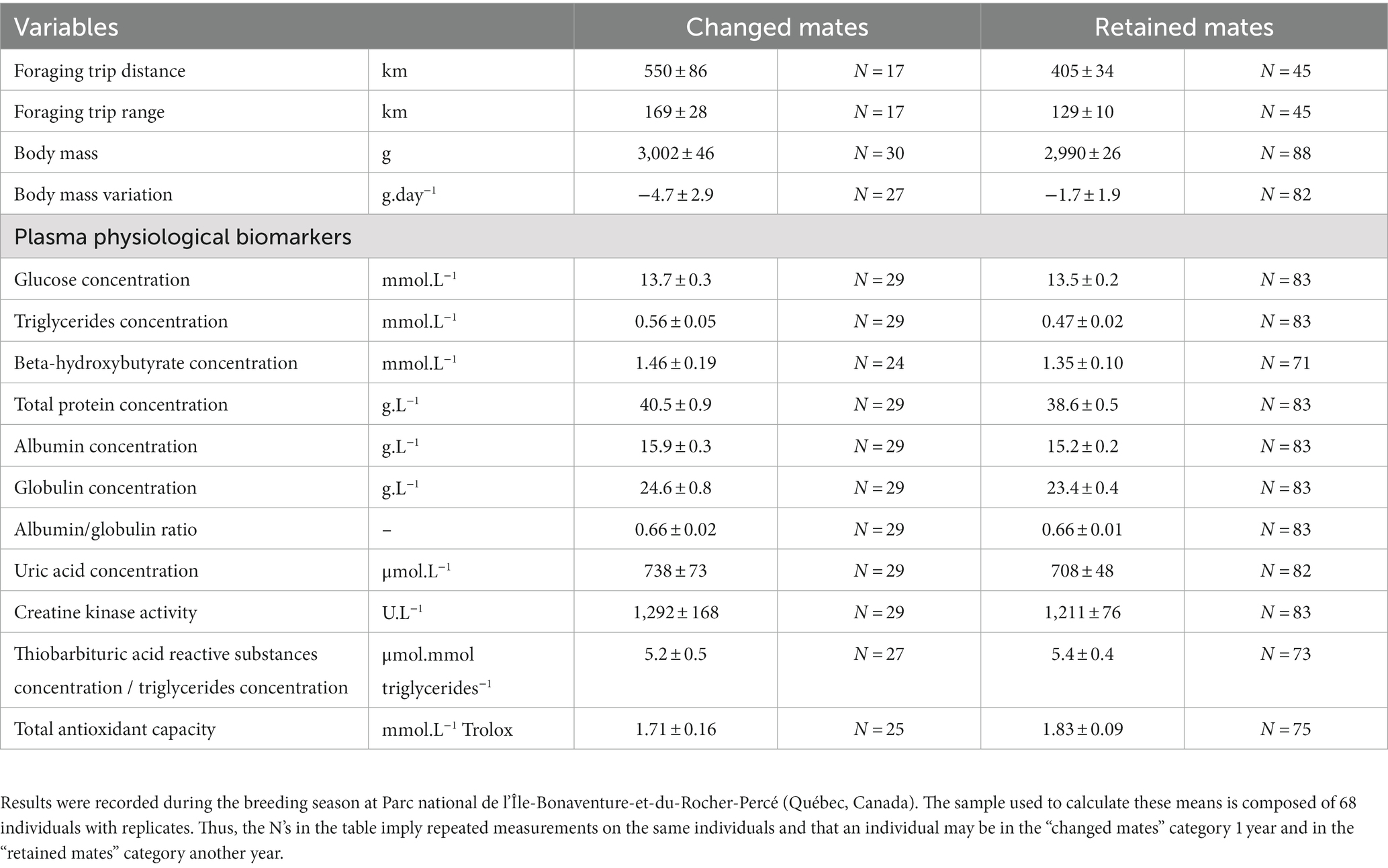
Table 1. Mean ± SEM of 15 behavioral and physiological biomarkers compared between northern gannets that changed or retained their mates in 2017, 2018 and 2019.
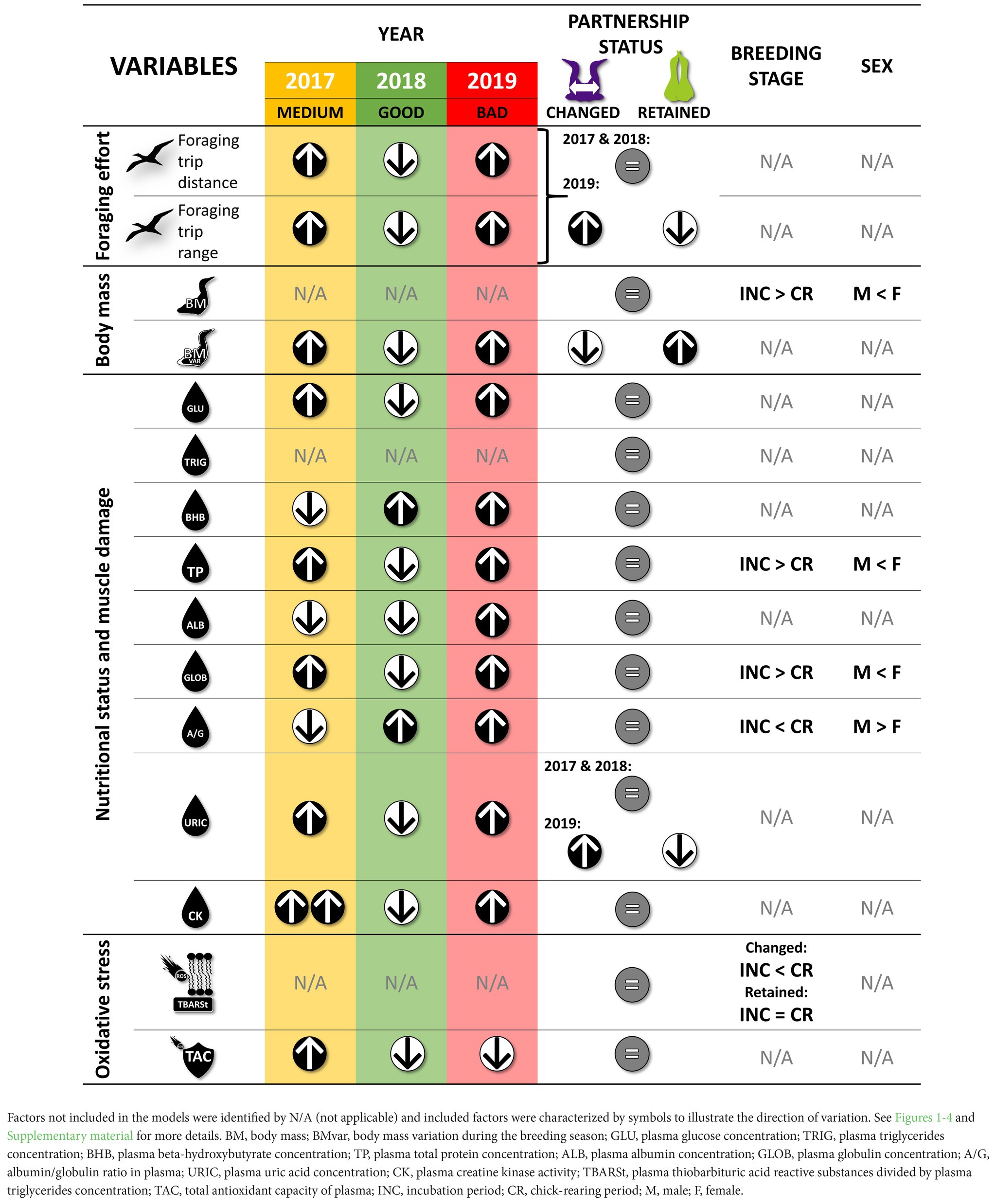
Table 2. Summary of the most parsimonious linear mixed models including partnership status calculated for behavioral and physiological variables (parental foraging effort, body mass, nutritional status, muscle damage, oxidative stress) with year, partnership status, breeding stage, sex, and interactions as fixed factors, and bird ID as random parameters.
Foraging effort
We recorded 288,718 GPS locations during 1,008 complete foraging trips across three breeding seasons. Mean foraging trip range ± SEM was 139 ± 11 km with a mean distance traveled per trip of 445 ± 35 km. Trip distance and trip range were highly contrasted according to years. Mean ± SEM in 2017, 2018 and 2019 was 576 ± 61 km, 186 ± 17 km and 530 ± 51 km for trip distance, and 180 ± 18 km, 66 ± 6 km and 163 ± 17 km for trip range, respectively. Foraging effort is best explained by partnership status and year for trip distance (Figure 1, = 66.93, df = 4, p 0.0001) and for trip range ( = 40.20, df = 3, p 0.0001) (Supplementary Table S1). Foraging distance varied with year (F2,54 = 40.55, p 0.0001) and with a significant partnership status:year interaction (F2,55 = 3.39, p 0.04). We obtained similar results for foraging trip range with year (F2,54 = 32.30, p 0.0001) and partnership status:year interaction (F2,55 = 4.48, p 0.02). During seasons of medium and high breeding success, there was no difference in parental foraging effort between partnership status. During the favorable year (2018), parents foraged 84% nearer from the colony than the three-year average. However, during the most unfavorable year (2019), parents that retained their mate dispersed 83% less than parents that changed their mate (Figure 1). Between the best and the worst year, birds that changed their mate increased their foraging trip distance by 790% and those that retained their mates increased by 248%. Similar results were obtained for foraging trip range.
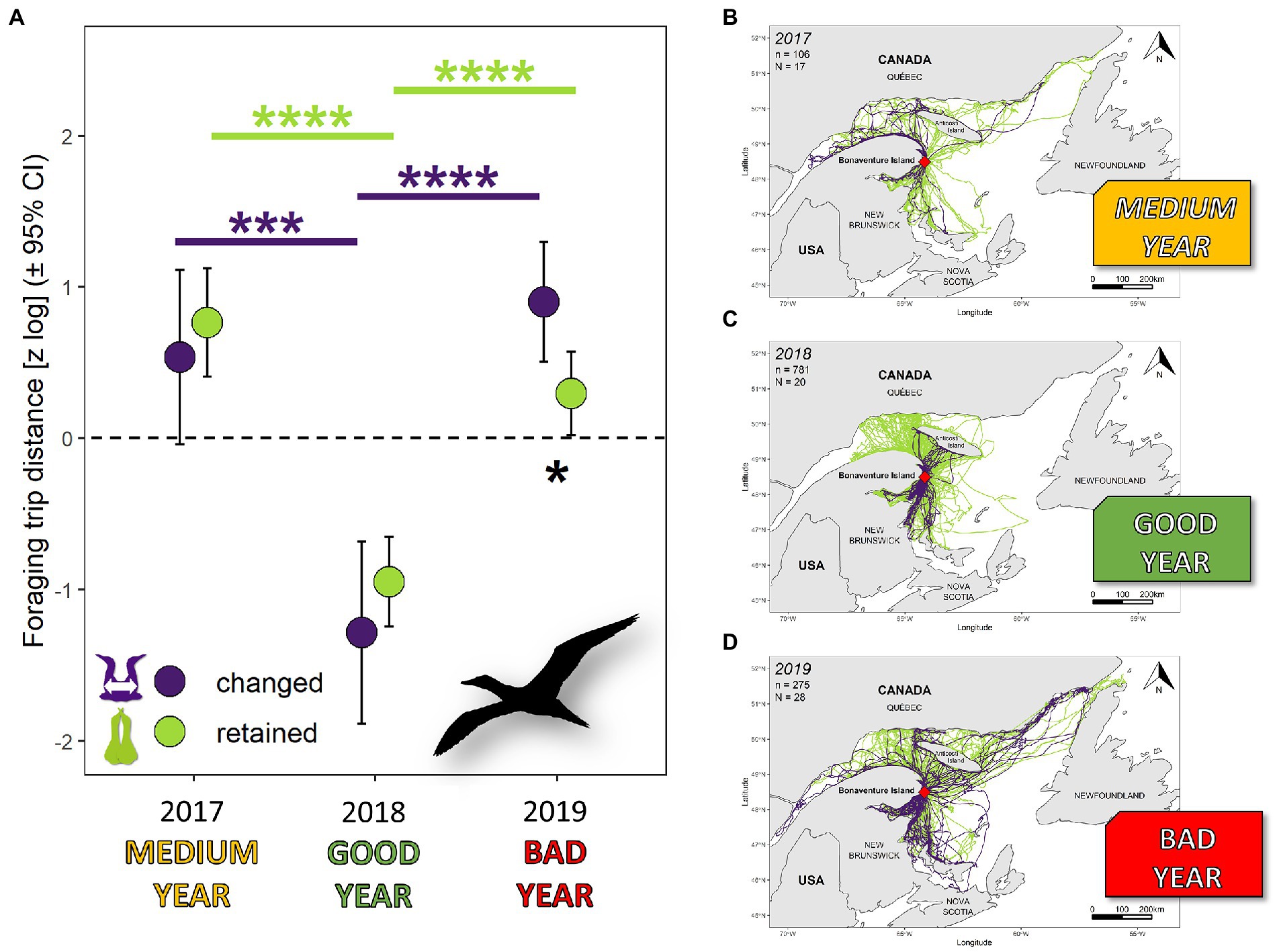
Figure 1. Variation in foraging trip distance during breeding season by northern gannets that changed or retained their mates in 2017 (N = 16), 2018 (N = 20), and 2019 (N = 28). Results at left (A) are estimated marginal means (±95% CI) by year obtained from a mixed linear model with year and partnership status:year interaction as fixed parameters and bird ID as random parameter. Data were log-transformed and standardized (*p < 0.05; ***p < 0.001; ****p < 0.0001). Maps on the right side show the total foraging trips recorded each year with GPS devices: (B) 2017 = medium year (breeding success in the colony: 43%), (C) 2018 = good year (breeding success: 60%), and (D) 2019 = bad year (breeding success: 13%).
Body mass
Mean body mass (BM) ± SEM of gannets was 2,971 ± 246 g. BM was best explained by a model with partnership status, breeding stage and sex (Figure 2A, χ2 = 12.20, df = 1, p = 0.0005, pseudo-R2 total = 0.12; details in Supplementary Table S1). BM did not vary with partnership status (F1,115 = 0.171, p = 0.68), but varied with breeding stage (F1,122 = 11.97, p = 0.0007) and sex (F1,62 = 5.31, p = 0.02). BM was 43% higher during the incubation period, and 21% lower for males. Body mass variation (BMvar) was best explained by partnership status, breeding stage and year (Figure 2B, χ2 = 18.84, df = 2, p < 0.0001, details in Supplementary Table S1). BMvar varied with partnership status (F1,97 = 4.95, p = 0.03) and year (F2,85 = 9.68, p = 0.0002). Gannets that have changed their partner had 25% higher daily mass loss than individuals that have retained their partner. Daily mass loss recorded during good year (2018) was 35% lower than the medium year (2017) and 29% the bad year (2019).
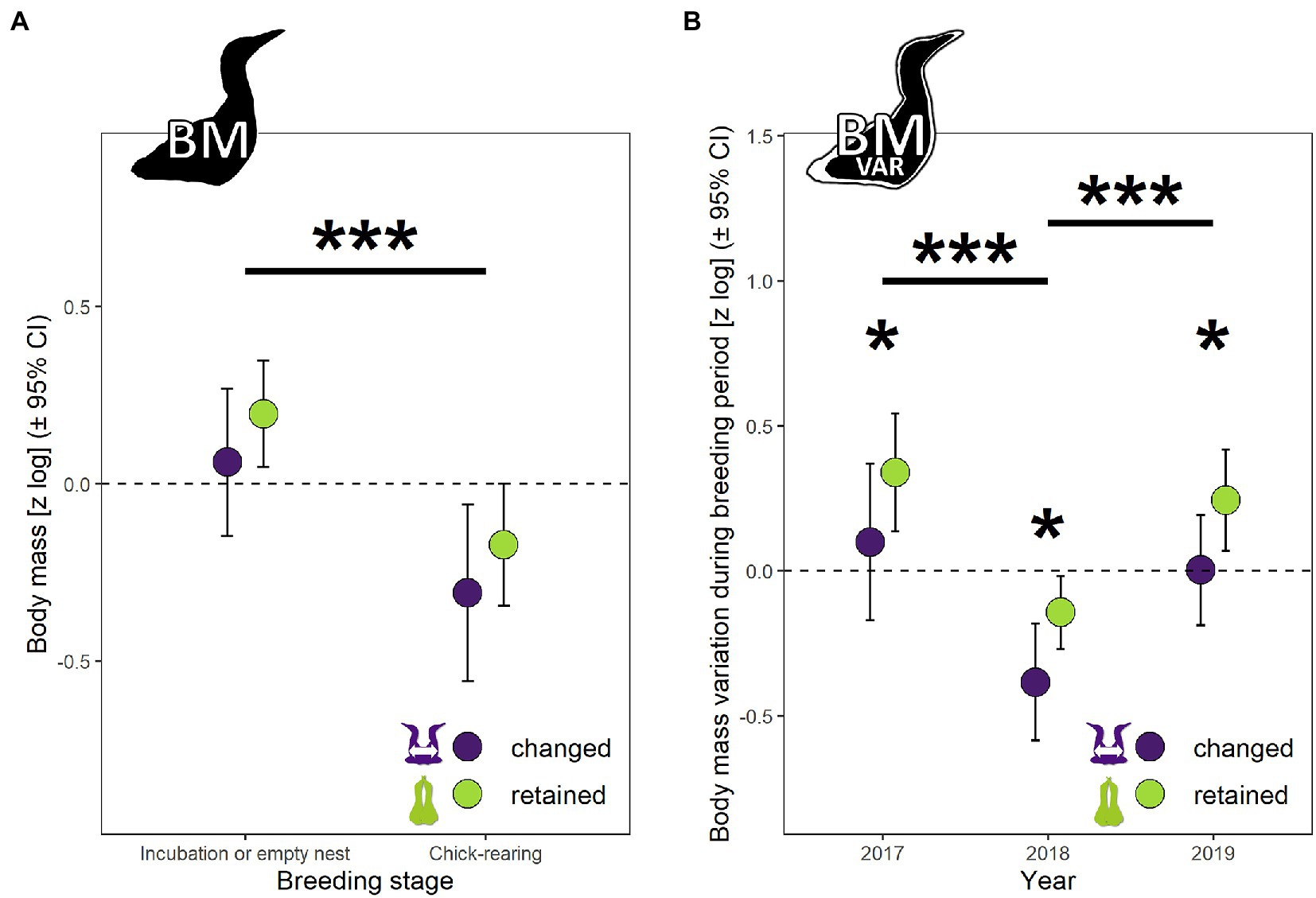
Figure 2. (A) Body mass (BM) and (B) body mass variation (BMvar) measured in breeding northern gannets that changed and retained their mates in 2017, 2018 and 2019. Results exposed are estimated marginal means (± 95% CI) by year obtained from a mixed linear model controlling for julian date (for body mass and body mass variation) with bird ID as random parameter and these fixed parameters: partnership status, breeding stage and sex for (A), and partnership status and year for (B). Data were log-transformed and standardized (*p < 0.05, **p < 0.01, ***p < 0.001, ****p < 0.0001).
Nutritional status and muscle damage
Of these nine biomarkers of nutritional status and muscle damage (Figure 3), only plasma uric acid (URIC) concentration varied with an interaction in partnership status and year (Figure 3H, F2,104 = 4.24, p = 0.02, Supplementary Table S1), varied with year (F2,99 = 7.81, p = 0.0007), but did not vary with partnership status alone (F1,100 = 0.13, p = 0.72). In retained mates, URIC was higher in 2017 (+115% and + 84% higher than 2018 [p = 0.007] and 2019 [p = 0.046], respectively). At the opposite, in changed mates, URIC was the lowest in 2018, −70% lower than 2017 (p = 0.04) and − 79% lower than 2019 (p = 0.001). During 2019, changed mates exhibited a 101% higher URIC concentration than retained mates.
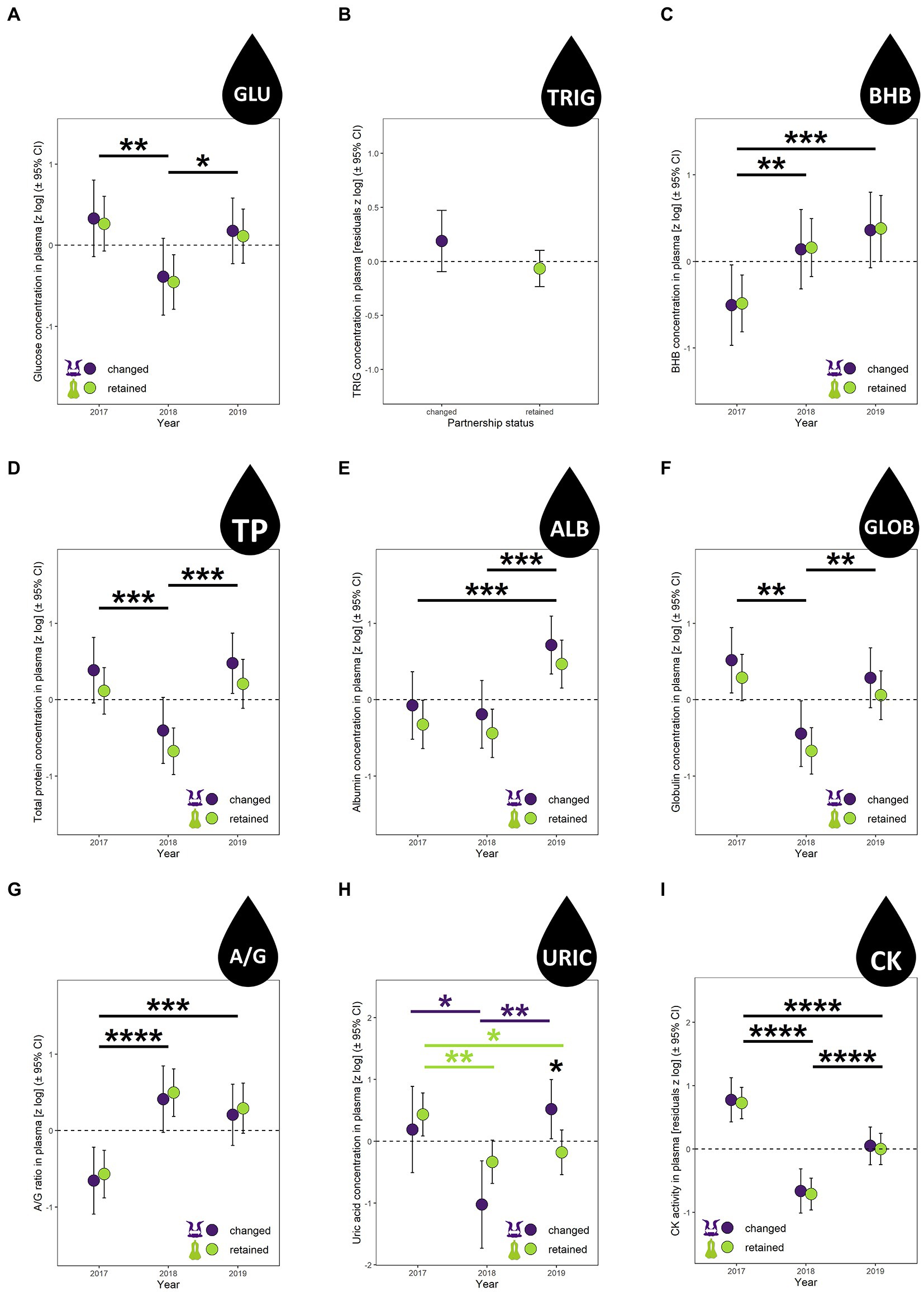
Figure 3. Plasma biomarkers concentration of nutritional status [(A) glucose (GLU), (B) triglycerides (TRIG), (C) beta-hydroxybutyrate (BHB), (D) total protein content (TP), (E) albumin (ALB), (F) globulins (GLOB), (G) albumin/globulins ratio (A/G), (H) uric acid (URIC)] and muscle damage [(I) creatine kinase activity (CK)] in northern gannets that changed and retained their mates in 2017, 2018, and 2019. Results exposed are estimated marginal means (±95% CI) by year obtained from mixed linear models build with bird ID as random parameter and these fixed parameters: partnership status for (B), partnership status and year for (A,C,E), partnership status, year and partnership status:year interaction for (H), partnership status, year, sex and breeding stage for (D,F,G), and partnership status, year and sex for (I). Data were log-transformed and standardized (*p < 0.05, **p < 0.01, ***p < 0.001, ****p < 0.0001; green symbols represent significant differences for individuals that retained their mate and purple symbols represent significant differences for individuals that changed mates).
The plasma concentration in glucose (GLU), triglycerides (TRIG), beta-hydroxybutyrate (BHB), total protein (TP), albumin (ALB), globulin (GLOB), the albumin/globulin (A/G) ratio, and the creatine kinase (CK) activity did not vary with partnership status (Figures 3A–G,J, p > 0.05) but GLU, BHB, TP, ALB, GLOB, A/G, and CK varied with year (p < 0.01) with medium to very large effect size (Cohen’s d > 0.5, Supplementary Table S1). All of these biomarkers were lower in the good year (2018) than in the bad year (2019) (except for BHB and A/G ratio), ranging from −43 to −147%.
TP, GLOB and A/G ratio varied also with breeding stage and sex (p < 0.05), where TP and GLOB were higher during incubation period than chick-rearing period and higher for females than males (with the opposite result for A/G ratio).
Oxidative stress
Thiobarbituric acid reactive substances concentration (TBARS) divided by triglycerides concentration (TBARSt) was best explained by a model with partnership status, breeding stage and partnership status:breeding stage interaction (Figure 4A, χ2 = 11.05, df = 2, p = 0.004, Supplementary Table S1). TBARSt did not vary with partnership status alone (F1,103 = 0.04, p = 0.85), but varied with breeding stage (F1,107 = 10.80, p = 0.001). TBARSt between incubation and chick rearing periods increased by 217% (p = 0.008) for changed mates (only a trend [p = 0.06] for retained mates, it was 52% greater during chick rearing period).
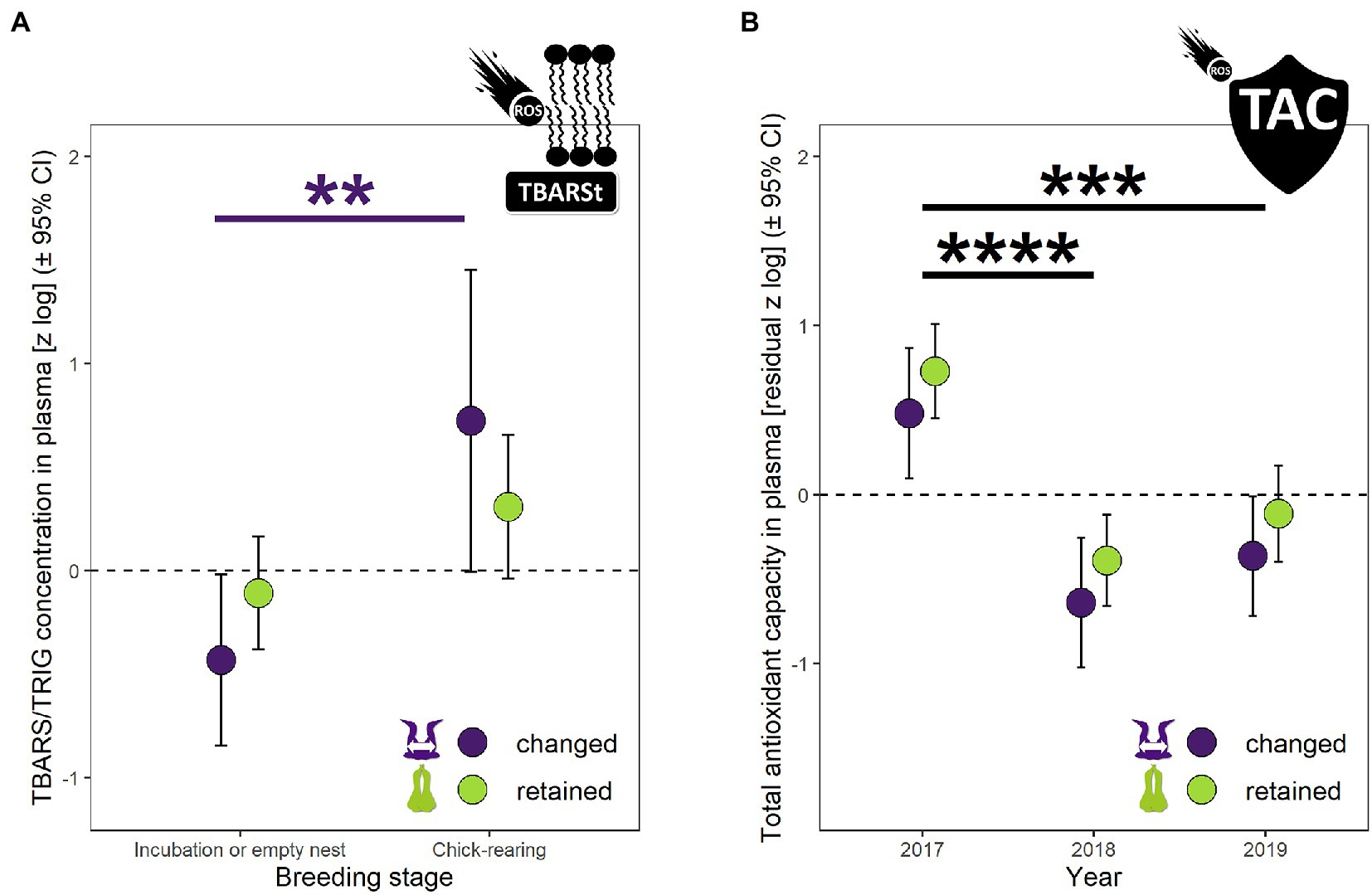
Figure 4. Biomarkers of (A) oxidative damage in plasma (thiobarbituric acid reactive substance concentration corrected for triglycerides – TBARSt) and (B) total antioxidant capacity of plasma (TAC) in northern gannets that changed and retained their mates in 2017, 2018 and 2019. Results exposed are estimated marginal means (±95% CI) by year obtained from a mixed linear model with bird ID as random parameter and these fixed parameters: partnership status, breeding stage and partnership status:breeding stage interaction for (A), and partnership status and year for (B). Data were log-transformed and standardized. Because TAC varied positively with uric acid (URIC, Pearson’s r = 0.46, F1,98 = 24.53, p < 0.0001), TAC was controlled for URIC by using residuals of lmer (zlTAC ~ (1|ID) + zlURIC) (**p < 0.01, ***p < 0.001, ****p < 0.0001).
Total antioxidant capacity (TAC) in plasma varied positively with uric acid (URIC, Pearson’s r = 0.46, F1,98 = 24.53, p < 0.0001). TAC was best explained by a model with partnership status and year (Figure 4B, χ2 = 34.02, df = 2, p < 0.0001, Supplementary Table S1). TAC did not vary with partnership status (F1,89 = 1.96, p = 0.17), but varied with year (F12,80 = 19.19, p < 0.0001). The highest TAC concentration measured in 2017 was 245% higher than 2018 (p < 0.0001) and 144% higher than 2019 (p < 0.0001).
Discussion
Our study helps fill a knowledge gap on how behavioral flexibility (expressed as mate change and foraging behavior) affects key physiological traits of seabirds during the breeding season. Our study shows that behavioral flexibility influences short-term health status of northern gannets through a variety of biomarkers including nutritional status, oxidative stress, and muscle damage (summary of significant results in Table 2). This study shows that individuals breeding with a new mate face a short-term constraint during the chick rearing period, during years with poor reproduction output. This is associated with increased foraging effort following mate changes. Knowing that the gannets are plunge-divers searching for food during flight (Nelson, 2002), an increase in total flight cost may cause a short-term increase in oxidative stress and explain the concomitant decrease in body mass during the breeding season and higher level of protein catabolism. Most importantly variation in physiological health status is influenced more by behavioral adjustment related to environmental foraging conditions during reproduction, rather than by behavioral adjustment resulting from re-pairing with a new partner. When gannets breed in a less favorable year, all individuals must increase foraging effort, which is associated with higher levels of glucose, ketone bodies, protein (albumin and globulins), uric acid, and muscle damage.
Behavioral flexibility, partnership status and parental effort
We previously shown that divorce can be considered as an adaptive strategy to counteract the impact of food depletion and low breeding success (Pelletier and Guillemette, 2022). The act of changing mates in gannets after breeding failure in a context of food stochasticity, and even food insecurity, may be interpreted as an ability for individuals to change their partnership status for future reproductive outcomes. In a review, Beever et al. (2017) showed that behavioral flexibility allows animals to rapidly cope with changing environmental conditions because behavior is an important component of species’ adaptive capacity in the face of global changes. Thus, behavioral flexibility can prevent, correct, or minimize the negative consequences of adverse environmental changes (Williams, 2018) or of sudden reversals in the relative quality of mates (Botero and Rubenstein, 2012). However, it is likely affected by the variability and predictability of local to global environmental attributes (Botero and Rubenstein, 2012), and by intrinsic life history traits as age and health status.
In addition, with mate adjustment or mate compensation, mate changes are associated with different costs (see Ens et al., 1996) that may contribute to an increase in physiological stress. In our study, gannets increased parental investment during the breeding season by enhancing foraging trip distance and range, particularly during unfavorable years. In a new pair, this increase in parental effort may occur in one or both partners, but our sample is not sufficient to explore this variation in couples. In any case, even though biparental care is widespread in birds (80% of species, see Cockburn, 2006), the division of labor within the pair can be highly variable. In a new pair, re-pairing mates may cooperate and share equally and rapidly their parental investment (Hinde, 2006; Hinde and Kilner, 2007), or one individual may contribute less to the protection of young and provisioning at the cost of its new partner (‘sexual conflict’, Houston et al., 2005). In both cases, parental care can be costly to a parent, in that it reduces their reproductive success in other breeding attempts (Clutton-Brock, 1991; Royle et al., 2012) and their survival probability (Stearns, 1992; but see Cruz-Flores et al., 2021).
Any enhancement in parental effort implies an increment in energy expenditure, especially when this investment is related to foraging and flight behavior. In our study, foraging efforts increase during detrimental years and particularly for changed mates (consistent with our first prediction P1). Flight is the dominant behavior of gannets during foraging, searching for prey and plunge-diving (Lewis et al., 2002). As many other nesting birds, gannets adopt a central-place foraging strategy during reproduction (Ashmole, 1963), i.e., they need to collect and return with food at the nest regardless of the prey density and environmental conditions. Thus, when the distribution of prey is changing or when prey biomass decreases, seabirds must have the capacity to broaden their foraging range. As observed between 2003 and 2012–2014, gannets nesting at Bonaventure Island expanded substantially their time searching for food (+ 57%) due to biomass decline of their preferred prey (Atlantic mackerel, Guillemette et al., 2018). Trip distance and trip range measured in our study are very contrasted according to the years: lower distances during the favorable year (2018) than those found in literature for the same species, but during less favorable years (2017 and 2019) distances are much higher or similar (e.g., mean trip duration = 232 km in Hamer et al. (2000), data from 18 to 595 km in Pettex et al. (2012), 266 km for males or 519 km for females in Stauss et al. (2012), means from 480 to 563 km in Guillemette et al. (2018), means from 369 to 601 km, Malvat et al. (2020)). Given the high rate of energy expenditure associated with flight, an estimated cost of flight during foraging (84 W, Pelletier et al., 2020) was in average 2,848 kJ for a flight of 550 km and 2097 kJ for a flight of 405 km (Table 1). This high level of energy expenditure, viewed as an augmentation in parental effort, might potentially reduce the health condition of the parent at the end of the breeding season, limiting survival probability and the opportunity of breeding the following year (Stearns, 1992).
Physiological condition
Nutritional status and muscle damage
Results presented in our study did not highlight a clear increase in nutritional stress related with partnership status (according to carbohydrates, lipids, and protein metabolism). For instance, we found no evidence to support our initial prediction (P2), where changed mates, compared to retained mates, increase their nutritional stress (except for uric acid).
Plasma GLU levels measured in our study (13.6 mM) are similar to those obtained by Braun and Sweazea (2008) predicted by the relationship between plasma GLU and log BM (15.3–0.44*logBM = 13.8 mM where BM = 3,000 g) most likely to ensure the bird’s central nervous system homeostasis (Totzke et al., 1999; Braun and Sweazea, 2008).
Combination of results for TRIG, BHB and URIC plasma content were suspected to reflect nutritional and physiological state of birds (Jenni-Eiermann and Jenni, 1998), but the results did not reveal any trend related to partnership status. Because levels of plasma TRIG were found to reflect reproductive effort in breeding birds (Masello and Quillfeldt, 2004) and flying effort during migration (Jenni-Eiermann and Jenni, 1992), this biomarker could have been useful for tracking foraging effort, but this is not the case. TRIG are involved in lipid transport and are the main energy source during prolonged flight (Rothe et al., 1987). From our results the total quantity of lipids delivered to active muscles during flight, and fat deposition, do not appear to be associated to partnership status, breeding stage, or annual environmental conditions. In addition, this absence of association with TRIG is reflected by plasma levels of BHB, a by-product of the breakdown of fatty acids. In other words, fasting did not appear to be more severe in birds that changed mates. Nevertheless, the inter-annual comparison suggests that fat catabolism was intensely mobilized during the detrimental year, suggesting fasting in the whole population when feeding conditions are critical.
We found no evidence of influence of partnership status on protein metabolism via TP, ALB and GLOB, but we recorded highest rates of these metabolites during unfavorable years. TP, ALB and GLOB ranges were similar to those found in literature for similar species (Balasch et al., 1974; Lumeij, 1987; Newman et al., 1997). Increase in TP and ALB may also result from dehydration, and increase in TP, but not ALB, may be due to acute or chronic inflammatory conditions implying an elevation in α, β or γ globulin concentrations and frequently, a decrease in ALB. The combined effect of these changes is a decrease in A/G ratio. Chronic infectious diseases such as aspergillosis and tuberculosis are some possible examples (Lumeij, 1987).
We found high variability in protein catabolism via plasma URIC concentration between partnership status and year, but the highest URIC values were measured in changed mates during 2019. URIC results measured in our study (767 μmol.L-1 in average) are higher than reported values for other avian species: 178–595 μmol.L−1 (Campbell and Grant, 2010). Therefore, our study suggests that gannets breeding during unfavorable years increased protein catabolism. Studies of starvation in large birds (see Jenni-Eiermann and Jenni, 1998) have shown that URIC increases dramatically with, conversely, a rapid drop in TRIG and/or BHB levels, indicating that mobilization of protein concord with diminution of the reserves of stored fat. The extrinsic conditions affect breeding gannets and during unfavorable years, gannets of any partnership status are probably at the threshold of starvation. Moreover, during unfavorable years, birds that changed their mates are possibly in a more critical health condition with higher levels of nutritional stress and dehydration.
The plasma CK activity changes significantly between years and not between partnership status, which did not support our initial prediction (P3) where changed mates increase their muscle damage (expressed as increase in CK activity). This result again demonstrates that increased foraging effort related to unfavorable environmental conditions for reproduction is a determinant of physiological condition, more than increased effort associated with mate change. Indeed, the CK activity increased the most during years when the foraging distances traveled by gannets are greater. These results are consistent with the highest foraging effort associated with flight (Guglielmo et al., 2001; Swanson and Thomas, 2007) and the highest protein catabolism in changed mates during unfavorable years.
Oxidative stress
Oxidative stress regulation has been proposed as a key physiological mechanism underlying life-history trade-offs in vertebrates (Costantini, 2017). However, long-lived bird species are known to produce less blood cell mitochondrial ROS than short-lived birds (Delhaye et al., 2016) and to have normally low levels of reactive oxygen species in circulation (Xia and Møller, 2018). Long-lived birds should suffer less oxidative damage than short-lived birds and therefore, inter-individual differences are probably difficult to distinguish without considering intra-individual variability.
In a meta-analysis (with 82 species), Costantini (2017) found no differences between males and females in terms of oxidative damage when both sexes contribute to parental care, suggesting that equivalent workload (e.g., foraging flights, protection behavior, etc.) drives equivalent oxidative balance. Sex has not been included in any model calculated for TBARSt, but we found higher short-term plasma oxidative damage (higher TBARSt) in changed mates between incubation and chick-rearing periods. Thus, this increase is only greater during the year when environmental conditions were most unfavorable for gannet breeding (prediction P4 where changed mates increase their oxidative damage more than retained mates). The short-term increase in oxidative damage related to parental adjustment or parental compensation could however be partly offset by mechanisms of protection. Biomarkers that assess stress and oxidative stress in mid- or long-term perspective (such as biomarkers of DNA damage or telomere dynamics) should be used to investigate the long-term influence of increased parental effort promoted by changes in partnership status.
Plasma TAC levels were not influenced by partnership status (prediction P5 where changed mates decrease their antioxidant capacity). However, as shown above, gannets that changed their mate, increased their foraging effort during the worst unfavorable breeding conditions and then, the increased workload due to this energetic demand should have negatively affected the non-enzymatic antioxidant capacity of plasma. As demonstrated in an experimental study by Alonso-Alvarez et al. (2004), an augmentation in parental effort in zebra finches (from no breeding to six chicks rearing) contribute to decreasing plasma antioxidant capacity and thus, to increase potential oxidative stress. However, long-lived bird species, as gannets, have higher levels of antioxidants to cope and live longer with many oxidative attacks caused through the life by aerobic metabolism (Falnes et al., 2007; Galván et al., 2012) (but see Cohen et al., 2008). Antioxidants protect them against alteration of gene expression that ultimately leads to cellular senescence and cell death (Finkel and Holbrook, 2000). It has been shown that king penguins are naturally equipped to resist stress with an up-regulation in endogenous antioxidant defenses (glutathione antioxidant system) and a decrease in mitochondrial efficiency (Stier et al., 2019).
Conclusion
The goal of this paper was to identify the potential physiological consequences of behavioral flexibility (as divorce and foraging effort) in relation to annual variation in breeding conditions (as food stochasticity). Our study enabled us to provide insights into the impacts of an individual’s decision-making process as change mates on nutritional status and oxidative stress. At the breeding season temporal scale, we found that birds that changed mate had to increase parental effort (e.g., foraging effort). Individuals with a new partner were affected by greater increases of lipid oxidative damage and higher mass loss during chick rearing period, and higher protein catabolism (supporting our first hypothesis). In addition, the negative impact of mate change on physiological condition occurs mostly during unfavorable years when environmental conditions lead to food insecurity. The interannual variability in physiological biomarker suggests that regulation of nutritional status and antioxidant capacity may have a primary role to play in explaining the impact of parental effort on physiological condition. Most importantly, using annual breeding success as a proxy, our results show that the very high interannual environmental variability seems to be a major factor in explaining the long-term physiological condition of gannets.
Data availability statement
The raw data supporting the conclusions of this article will be made available by the authors, without undue reservation.
Ethics statement
The animal study was reviewed and approved by Animal Care Committee (ACC) of the Université du Québec à Rimouski and complied with the guidelines of the Canadian Council on Animal Care (CCAC).
Author contributions
DP: conceptualization (equal), data curation (lead), investigation (lead), formal analysis (lead), and writing-original draft (equal). PB: writing-review and editing (equal). FV: writing-review and editing (equal). MG: conceptualization (equal) and writing-original draft (equal). All authors contributed to the article and approved the submitted version.
Funding
Natural Sciences and Engineering Research Council of Canada (NSERC) Discovery Grant (to MG) and Fonds de recherche du Québec Nature et Technologies (FRQNT) – Programme de recherche pour les chercheurs de collège (to DP), grant number: 193215. Alexander Graham Bell Canada Graduate Scholarship – Doctoral award (to DP). Grants from Environment and Climate Change Canada and from BOREAS-UQAR (to DP).
Acknowledgments
Thanks for the extensive commitment of sampling, laboratory and analytical personnel involved in the Laboratoire d’ornithologie marine de Rimouski (LOMR) at Université du Québec à Rimouski (Yannick Seyer, Laurie Maynard, Sarah Wing, Isabeau Pratte, Mélanie Laflèche, Catherine Ayotte, Dévrig Bouillet, Félix Larochelle, Olivier Buteau, Gabrielle Théroux, Liette Régimbald, Sandrine Gingras, Jolanie Roy, Safouane Khamassi, Jeanne Bouchard, Selma Elfassi-Fihri, Marie-Anne Robitaille, Richard Gravel, Roxanne Turgeon, Camille Novales, Laurence Gagnon, Marie-Eve Labonté Dupras, Catherine Destrempes, Fanny May Couture-Charron, Andra Florea), Cégep de Rimouski (Catherine Bouchard, Laury-Ann Dumoulin, Angéline Robichaud, Gabrielle Bouchard, Anne-Charlotte Lebel, Daisy Turcotte, Laura Turcotte, Catherine Gloutnez, Andréa Lévesque, Isabelle Demalsy, Alexia Tremblay, Emma Côté, Lydiane Parent, Sandrine St-Pierre-Lepage), CWS and DFO during all those years. We sincerely thank France Dufresne (UQAR) for having welcomed us in its laboratory for the sex determination analyzes and to Geneviève Côté and Jean-Michel Martin for helping us with sex determination. Thanks to Parc national de l’Île-Bonaventure-et-du-Rocher-Percé and its staff for transportation and supportive collaboration. Final thanks to Frédérique Paquin, France Dufresne and Felix Christen for helpful comments. The title is inspired by “Good Times Bad Times,” the opening song of the first Led Zeppelin album released in 1969 describing the challenges and experiences that can arise in any relationship, whether it ultimately ends or continues.
Conflict of interest
The authors declare that the research was conducted in the absence of any commercial or financial relationships that could be construed as a potential conflict of interest.
Publisher’s note
All claims expressed in this article are solely those of the authors and do not necessarily represent those of their affiliated organizations, or those of the publisher, the editors and the reviewers. Any product that may be evaluated in this article, or claim that may be made by its manufacturer, is not guaranteed or endorsed by the publisher.
Supplementary material
The Supplementary material for this article can be found online at: https://www.frontiersin.org/articles/10.3389/fevo.2023.1108293/full#supplementary-material
References
Aebischer, N., Coulson, J., and Colebrookl, J. (1990). Parallel long-term trends across four marine trophic levels and weather. Nature 347, 753–755. doi: 10.1038/347753a0
Alonso-Alvarez, C., Bertrand, S., Devevey, G., Prost, J., Faivre, B., and Sorci, G. (2004). Increased susceptibility to oxidative stress as a proximate cost of reproduction. Ecol. Lett. 7, 363–368. doi: 10.1111/j.1461-0248.2004.00594.x
Arnqvist, G., and Kirkpatrick, M. (2005). The evolution of infidelity in socially monogamous passerines: the strength of direct and indirect selection on extrapair copulation behavior in females. Am. Nat. 165, S26–S37. doi: 10.1086/429350
Ashmole, N. P. (1963). The regulation of numbers of tropical oceanic birds. Ibis 103b, 458–473. doi: 10.1111/j.1474-919X.1963.tb06766.x
Balasch, J., Palomeque, J., Palacios, L., Musquera, S., and Jimenez, M. (1974). Hematological values of some great flying and aquatic-diving birds. Comp. Biochem. Physiol. A Comp. Physiol. 49, 137–145. doi: 10.1016/0300-9629(74)90549-0
Barton, K. (2009). MuMIn: multi-model inference. R package version 0.12.0. Available at: http://r-forge.r-project.org/projects/mumin/
Bates, D., Mächler, M., Bolker, B., and Walker, S. (2015). Fitting linear mixed-effects models using lme4. J. Stat. Softw. 67, 1–48. doi: 10.18637/jss.v067.i01
Beaulieu, M., Reichert, S., Le Maho, Y., Ancel, A., and Criscuolo, F. (2011). Oxidative status and telomere length in a long-lived bird facing a costly reproductive event. Funct. Ecol. 25, 577–585. doi: 10.1111/j.1365-2435.2010.01825.x
Beever, E. A., Hall, L. E., Varner, J., Loosen, A. E., Dunham, J. B., Gahl, M. K., et al. (2017). Behavioral flexibility as a mechanism for coping with climate change. Front. Ecol. Environ. 15, 299–308. doi: 10.1002/fee.1502
Bennett, P.M., and Owens, I.P. (2002). Evolutionary Ecology of Birds: Life Histories, Mating Systems and Extinction. Oxford: Oxford University Press.
Black, J.M. (1996). Partnerships in Birds: The Study of Monogamy. New York: Oxford University Press.
Botero, C. A., and Rubenstein, D. R. (2012). Fluctuating environments, sexual selection and the evolution of flexible mate choice in birds. PLoS One 7:e32311. doi: 10.1371/journal.pone.0032311
Braun, E. J., and Sweazea, K. L. (2008). Glucose regulation in birds. Comp. Biochem. Physiol. B Biochem. Mol. Biol. 151, 1–9. doi: 10.1016/j.cbpb.2008.05.007
Bried, J., and Jouventin, P. (2002). "Site and mate choice in seabirds: an evolutionary approach," in Biology of Marine Birds, ed. E. A. A. B. J. Schreiber (Eds.). (Boca Raton, FL: CRC Press), 263–305.
Burnham, K.P., and Anderson, D.R. (2002). Model Selection and Multimodel Inference – A Practical Information-Theoretic Approach. Colorado: Springer.
Campbell, T.W., and Grant, K.R. (2010). Clinical Cases in Avian and Exotic Animal Hematology and Cytology. Ames, Iowa: Wiley-Blackwell.
Choudhury, S. (1995). Divorce in birds: a review of the hypotheses. Anim. Behav. 50, 413–429. doi: 10.1006/anbe.1995.0256
Cichoń, M., Dubiec, A., and Chadzińska, M. (2001). The effect of elevated reproductive effort onhumoral immune function in collared flycatcher females. Acta Oecol. 22, 71–76. doi: 10.1016/S1146-609X(00)01094-8
Clutton-Brock, T.H. (1991). The Evolution of Parental Care. Princeton, NJ Princeton University Press.
Cockburn, A. (2006). Prevalence of different modes of parental care in birds. Proc. R. Soc. B Biol. Sci. 273, 1375–1383. doi: 10.1098/rspb.2005.3458
Cohen, J. (1988). Statistical Power Analysis for The Behavioral Sciences. Hillsdale, NJ Academic Press.
Cohen, A., Klasing, K., and Ricklefs, R. (2007). Measuring circulating antioxidants in wild birds. Comp. Biochem. Physiol. B: Biochem. Mol. Biol. 147, 110–121. doi: 10.1016/j.cbpb.2006.12.015
Cohen, A. A., and McGraw, K. J. (2009). No simple measures for antioxidant status in birds: complexity in inter-and intraspecific correlations among circulating antioxidant types. Funct. Ecol. 23, 310–320. doi: 10.1111/j.1365-2435.2009.01540.x
Cohen, A. A., McGraw, K. J., Wiersma, P., Williams, J. B., Robinson, W. D., Robinson, T. R., et al. (2008). Interspecific associations between circulating antioxidant levels and life-history variation in birds. Am. Nat. 172, 178–193. doi: 10.1086/589456
Costantini, D. (2011). On the measurement of circulating antioxidant capacity and the nightmare of uric acid. Methods Ecol. Evol. 2, 321–325. doi: 10.1111/j.2041-210X.2010.00080.x
Costantini, D. (2017). Meta-analysis reveals that reproductive strategies are associated with sexual differences in oxidative balance across vertebrates. Curr. Zool. 64:11. doi: 10.1093/cz/zox0002
Coulson, J. C. (1966). The influence of the pair-bond and age on the breeding biology of the kittiwake Gull Rissa tridactyla. J. Anim. Ecol. 35, 269–279. doi: 10.2307/2394
Cruz-Flores, M., Pradel, R., Bried, J., González-Solís, J., and Ramos, R. (2021). Sex-specific costs of reproduction on survival in a long-lived seabird. Biol. Lett. 17:20200804. doi: 10.1098/rsbl.2020.0804
Danchin, E., and Wagner, R. H. (1997). The evolution of coloniality: the emergence of new perspectives. Trends Ecol. Evolut. 12, 342–347. doi: 10.1016/S0169-5347(97)01124-5
Davis, A. K., Maney, D. L., and Maerz, J. C. (2008). The use of leukocyte profiles to measure stress in vertebrates: a review for ecologists. Funct. Ecol. 22, 760–772. doi: 10.1111/j.1365-2435.2008.01467.x
Deerenberg, C., Arpanius, V., Daan, S., and Bos, N. (1997). Reproductive effort decreases antibody responsiveness. Proceedings of the Royal Society of London. Series B: Biological Sciences 264, 1021–1029.
Delhaye, J., Salamin, N., Roulin, A., Criscuolo, F., Bize, P., and Christe, P. (2016). Interspecific correlation between red blood cell mitochondrial ROS production, cardiolipin content and longevity in birds. Age 38, 433–443. doi: 10.1007/s11357-016-9940-z
Dubiec, A., Witek, M., and Cichoń, M. (2005). Seasonal decline in leukocyte concentrations and reproductive output in female Great Tits (Parus major). Auk 122, 829–834. doi: 10.1093/auk/122.3.829
Dubois, F., and Cézilly, F. (2002). Breeding success and mate retention in birds: a meta-analysis. Behav. Ecol. Sociobiol. 52, 357–364. doi: 10.1007/s00265-002-0521-z
Ens, B. J., Choudhury, S., and Black, J. M. (1996). “Mate fidelity and divorce in monogamous birds” in Partnerships in Birds: The Study of Monogamy. ed. J. M. Black (New York: Oxford University Press), 344–401.
Ens, B. J., Safriel, U. N., and Harris, M. P. (1993). Divorce in the long-lived and monogamous oystercatcher, Haematopus ostralegus: incompatibility or choosing the better option? Anim. Behav. 45, 1199–1217. doi: 10.1006/anbe.1993.1142
Fairbrother, A., Craig, M. A., Walker, K., and O'Loughlin, D. (1990). Changes in mallard (Anas platyrhynchos) serum chemistry due to age, sex, and reproductive condition. J. Wildl. Dis. 26, 67–77. doi: 10.7589/0090-3558-26.1.67
Falnes, P. O., Klungland, A., and Alseth, I. (2007). Repair of methyl lesions in DNA and RNA by oxidative demethylation. Neuroscience 145, 1222–1232. doi: 10.1016/j.neuroscience.2006.11.018
Finkel, T., and Holbrook, N. J. (2000). Oxidants, oxidative stress and the biology of ageing. Nature 408, 239–247. doi: 10.1038/35041687
Fowler, M. A., Paquet, M., Legault, V., Cohen, A. A., and Williams, T. D. (2018). Physiological predictors of reproductive performance in the European Starling (Sturnus vulgaris). Front. Zool. 15:45. doi: 10.1186/s12983-018-0288-3
Galván, I., Erritzøe, J., Karadaş, F., and Møller, A. P. (2012). High levels of liver antioxidants are associated with life-history strategies characteristic of slow growth and high survival rates in birds. J. Comp. Physiol. B. 182, 947–959. doi: 10.1007/s00360-012-0671-x
Garthe, S., Camphuysen, K., and Furness, R. W. (1996). Amounts of discards by commercial fisheries and their significance as food for seabirds in the North Sea. Mar. Ecol. Prog. Ser. 136, 1–11. doi: 10.3354/meps136001
Gebhardt-Henrich, S. G., Heeb, P., Richner, H., and Tripet, F. (1998). Does loss of mass during breeding correlate with reproductive success? A study on Blue Tits Parus caeruleus. Ibis 140, 210–213. doi: 10.1111/j.1474-919X.1998.tb04381.x
González-Medina, E., Castillo-Guerrero, J. A., Santiago-Quesada, F., Villegas, A., Masero, J. A., Sánchez-Guzmán, J. M., et al. (2015). Regulation of breeding expenditure in the blue-footed booby, Sula nebouxii: an experimental approach. Anim. Behav. 108, 9–16. doi: 10.1016/j.anbehav.2015.06.025
Grémillet, D., Dell’Omo, G., Ryan, P. G., Peters, G., Ropert-Coudert, Y., and Weeks, S. J. (2004). Offshore diplomacy, or how seabirds mitigate intra-specific competition: a case study based on GPS tracking of Cape gannets from neighbouring colonies. Mar. Ecol. Prog. Ser. 268, 265–279. doi: 10.3354/meps268265
Groscolas, R. (1986). Changes in body mass, body temperature and plasma fuel levels during the natural breeding fast in male and female emperor penguins Aptenodytes forsteri. J. Comp. Physiol. B. 156, 521–527. doi: 10.1007/BF00691038
Guglielmo, C. G., Piersma, T., and Williams, T. D. (2001). A sport-physiological perspective on bird migration: evidence for flight-induced muscle damage. J. Exp. Biol. 204, 2683–2690. doi: 10.1242/jeb.204.15.2683
Guillemette, M., and Butler, P. J. (2012). Seasonal variation in energy expenditure is not related to activity level or water temperature in a large diving bird. J. Exp. Biol. 215, 3161–3168. doi: 10.1242/jeb.061119
Guillemette, M., Grégoire, F., Bouillet, D., Rail, J. F., Bolduc, F., Caron, A., et al. (2018). Breeding failure of seabirds in relation to fish depletion: is there a single threshold of food abundance? Mar. Ecol. Prog. Ser. 587, 235–245. doi: 10.3354/meps12442
Guindre-Parker, S., and Rubenstein, D. R. (2018). The oxidative costs of parental care in cooperative and pair-breeding African starlings. Oecologia 188, 53–63. doi: 10.1007/s00442-018-4178-3
Gustafsson, L., Nordling, D., Andersson, M., Sheldon, B., and Qvarnström, A. (1994). Infectious diseases, reproductive effort and the cost of reproduction in birds. Philos. Trans. R. Soc. Lond. B Biol. Sci. 346, 323–331.
Hamer, K. C., Humphreys, E. M., Garthe, S., Hennicke, J., Peters, G., Grémillet, D., et al. (2007). Annual variation in diets, feeding locations and foraging behaviour of gannets in the North Sea: flexibility, consistency and constraint. Mar. Ecol. Prog. Ser. 338, 295–305. doi: 10.3354/meps338295
Hamer, K., Phillips, R., Wanless, S., Harris, M., and Wood, A. (2000). Foraging ranges, diets and feeding locations of gannets Morus bassanus in the North Sea: evidence from satellite telemetry. Mar. Ecol. Prog. Ser. 200, 257–264. doi: 10.3354/meps200257
Harr, K. E. (2006). “Diagnostic value of biochemistry” in Clinical Avian Medicine. eds. G. J. Harrison and T. L. Lightfoot (Palm Beach, FL, USA: Spix Publishing Inc.), 611–630.
Harris, M. P., Safriel, U. N., Brooke, M. D. L., and Britton, C. K. (1987). The pair bond and divorce among Oystercatchers Haematopus ostralegus on Skokholm Island, Wales. Ibis 129, 45–57. doi: 10.1111/j.1474-919X.1987.tb03158.x
Harrison, F., Barta, Z., Cuthill, I., and Szekely, T. (2009). How is sexual conflict over parental care resolved? A meta-analysis. J. Evolut. Biol. 22, 1800–1812. doi: 10.1111/j.1420-9101.2009.01792.x
Harrison, G.J., Lightfoot, T.L., and Harrison, L.R. (2006). Clinical Avian Medicine. Palm Beach FL Spix Publishing.
Hasselquist, D., Wasson, M. F., and Winkler, D. W. (2001). Humoral immunocompetence correlates with date of egg-laying and reflects work load in female tree swallows. Behav. Ecol. 12, 93–97. doi: 10.1093/oxfordjournals.beheco.a000384
Hillstrom, L. (1995). Body mass reduction during reproduction in the pied flycatcher Ficedula hypoleuca: physiological stress or adaptation for lowered costs of locomotor? Funct. Ecol. 9:807. doi: 10.2307/2389978
Hinde, C. (2006). Negotiation over offspring care? A positive response to partner-provisioning rate in great tits. Behav. Ecol. 17, 6–12. doi: 10.1093/beheco/ari092
Hinde, C. A., and Kilner, R. M. (2007). Negotiations within the family over the supply of parental care. Proc. R. Soc. B Biol. Sci. 274, 53–60. doi: 10.1098/rspb.2006.3692
Hochleithner, M. (2013). “Biochemistries” in Avian Medicine, Principles and Application. eds. G. H. B. Ritchie and L. Lake Worth, Florida: Harrison (Wingers Publishing), 223–245.
Houston, A. I., Székely, T., and McNamara, J. M. (2005). Conflict between parents over care. Trends Ecol. Evolut. 20, 33–38. doi: 10.1016/j.tree.2004.10.008
IDEXX (2015). Manuel d’utilisation de l’analyseur biochimie IDEXX Catalyst Dx Burlington, ON: IDEXX Laboratories Inc.
Jenni-Eiermann, S., and Jenni, L. (1992). High plasma triglyceride levels in small birds during migratory flight: a new pathway for fuel supply during endurance locomotion at very high mass-specific metabolic rates? Physiol. Zool. 65, 112–123. doi: 10.1086/physzool.65.1.30158242
Jenni-Eiermann, S., and Jenni, L. (1998). What can plasma metabolites tell us about the metabolism, physiological state and condition of individual birds? An overview. Biol. Cons. Fauna 102, 312–319.
Jeschke, J. M., and Kokko, H. (2008). Mortality and other determinants of bird divorce rate. Behav. Ecol. Sociobiol. 63, 1–9. doi: 10.1007/s00265-008-0646-9
Johnston, V. H., and Ryder, J. P. (1987). Divorce in Larids: a review. Colon. Waterbirds 10, 16–26. doi: 10.2307/1521226
Kahle, D., and Wickham, H. (2013). ggmap: spatial visualization with ggplot2. R Journal 5, 144–161. doi: 10.32614/RJ-2013-014
Knuth, S. T., and Chaplin, S. B. (1994). The effect of exercice on activities of lactate dehydrogenase an creatine kinase in red-tailed hawks. J. Raptor. Res. 28, 27–33.
Lenth, Russell V. (2020). emmeans: Estimated Marginal Means, aka Least-Squares Means. R package version 1.7.0. https://CRAN.R-project.org/package=emmeans
Lewis, S., Benvenuti, S., Dall'Antonia, L., Griffiths, R., Money, L., Sherratt, T. N., et al. (2002). Sex-specific foraging behaviour in a monomorphic seabird. Proc. Biol. Sci. 269, 1687–1693. doi: 10.1098/rspb.2002.2083
Long, J.A. (2020). jtools: Analysis and Presentation of Social Scientific Data_. R package version 2.2.0, URL: https://cran.r-project.org/package=jtools
Luke, S. G. (2017). Evaluating significance in linear mixed-effects models in R. Behav. Res. Methods 49, 1494–1502. doi: 10.3758/s13428-016-0809-y
Lumeij, J. T. (1987). The diagnostic value of plasma proteins and non-protein nitrogen substances in birds. Vet. Q. 9, 262–268. doi: 10.1080/01652176.1987.9694111
Malvat, Z., Lynch, S. A., Bennison, A., and Jessopp, M. (2020). Evidence of links between haematological condition and foraging behaviour in northern gannets (Morus bassanus). R. Soc. Open Sci. 7:192164. doi: 10.1098/rsos.192164
Masello, J. F., and Quillfeldt, P. (2004). Are haematological parameters related to body condition, ornamentation and breeding success in wild burrowing parrots Cyanoliseus patagonus? J. Avian Biol. 35, 445–454. doi: 10.1111/j.0908-8857.2004.03278.x
Merila, J., and Wiggins, D. A. (1997). Mass loss in breeding blue tits: the role of energetic stress. J. Anim. Ecol. 66, 452–460. doi: 10.2307/5940
Moller, A. P. (1992). Frequency of female copulations with multiple males and sexual selection. Am. Nat. 139, 1089–1101. doi: 10.1086/285374
Monaghan, P. (2014). Organismal stress, telomeres and life histories. J. Exp. Biol. 217, 57–66. doi: 10.1242/jeb.090043
Moreno, J., Yorio, P., Garcia-Borboroglu, P., Potti, J., and Villar, S. (2002). Health state and reproductive output in Magellanic penguins (Spheniscus magellanicus). Ethol. Ecol. Evol. 14, 19–28. doi: 10.1080/08927014.2002.9522758
Nakagawa, S., Schielzeth, H., and O'Hara, R. B. (2013). A general and simple method for obtaining R2 from generalized linear mixed-effects models. Methods Ecol. Evol. 4, 133–142. doi: 10.1111/j.2041-210x.2012.00261.x
Newman, S. H., Piatt, J. F., and White, J. (1997). Hematological and plasma biochemical reference ranges of Alaskan seabirds: their ecological significance and clinical importance. Colon. Waterbirds 20, 492–504. doi: 10.2307/1521600
Nordling, D., Andersson, M., Zohari, S., and Lars, G. (1998). Reproductive effort reduces specific immune response and parasite resistance. Proceedings of the Royal Society of London. Series B: Biological Sciences 265, 1291–1298.
Norte, A. C., Ramos, J. A., Sampaio, H. L., Sousa, J. P., and Sheldon, B. C. (2010). Physiological condition and breeding performance of the great tit. Condor 112, 79–86. doi: 10.1525/cond.2010.080071
Ots, I., and Horak, P. (1996). Great tits Parus major trade health for reproduction. Proceed. R. Soc. London B Biol. Sci. 263, 1443–1447.
Pebesma, E. J. (2018). Simple features for R: standardized support for spatial vector data. R J. 10:439. doi: 10.32614/RJ-2018-009
Pelletier, D., and Guillemette, M. (2022). Times and partners are a-changin': relationships between declining food abundance, breeding success, and divorce in a monogamous seabird species. PeerJ 10:e13073. doi: 10.7717/peerj.13073
Pelletier, D., Seyer, Y., Garthe, S., Bonnefoi, S., Phillips, R. A., and Guillemette, M. (2020). So far, so good.. Similar fitness consequences and overall energetic costs for short and long-distance migrants in a seabird. PLoS One 15:e0230262. doi: 10.1371/journal.pone.0230262
Pettex, E., Lorentsen, S.-H., Grémillet, D., Gimenez, O., Barrett, R. T., Pons, J.-B., et al. (2012). Multi-scale foraging variability in Northern gannet (Morus bassanus) fuels potential foraging plasticity. Mar. Biol. 159, 2743–2756. doi: 10.1007/s00227-012-2035-1
Piatt, J. F., Harding, A. M. A., Shultz, M., Speckman, S. G., van Pelt, T. I., Drew, G. S., et al. (2007). Seabirds as indicators of marine food supplies: Cairns revisited. Mar. Ecol. Prog. Ser. 352, 221–234. doi: 10.3354/meps07078
Pichegru, L., Ryan, P. G., van der Lingen, C. D., Coetzee, J., Ropert-Coudert, Y., and Grémillet, D. (2007). Foraging behaviour and energetics of cape gannets Morus capensis feeding on live prey and fishery discards in the Benguela upwelling system. Mar. Ecol. Prog. Ser. 350, 127–136. doi: 10.3354/meps07128
R Core Team (2020). R: A Language and Environment for Statistical Computing – Reference Index. Vienna, Austria: R Foundation for Statistical Computing.
Regehr, H. M., and Montevecchi, W. A. (1997). Interactive effects of food shortage and predation on breeding failure of black-legged kittiwakes: indirect effects of fisheries activities and implications for indicator species. Mar. Ecol. Prog. Ser. 155, 249–260. doi: 10.3354/meps155249
Roche, M., Rondeau, P., Singh, N. R., Tarnus, E., and Bourdon, E. (2008). The antioxidant properties of serum albumin. FEBS Lett. 582, 1783–1787. doi: 10.1016/j.febslet.2008.04.057
Romero-Haro, A. A., Sorci, G., and Alonso-Alvarez, C. (2016). The oxidative cost of reproduction depends on early development oxidative stress and sex in a bird species. Proc. Biol. Sci. 283, 1–9. doi: 10.1098/rspb.2016.0842
Rothe, H. J., Biesel, W., and Nachtigall, W. (1987). Pigeon flight in a wind tunnel. J. Comp. Physiol. B. 157, 99–109. doi: 10.1007/BF00702734
Royle, N.J., Smiseth, P.T., and Kölliker, M. (2012). The Evolution of Parental Care. Oxford, UK: Oxford University Press.
Sawilowsky, S. S. (2009). New effect size rules of thumb. J. Mod. Appl. Stat. Methods 8, 597–599. doi: 10.22237/jmasm/1257035100
Shaffer, S. A., Costa, D. P., and Weimerskirch, H. (2003). Foraging effort in relation to the constraints of reproduction in free-ranging albatrosses. Funct. Ecol. 17, 66–74. doi: 10.1046/j.1365-2435.2003.00705.x
Stauss, C., Bearhop, S., Bodey, T. W., Garthe, S., Gunn, C., Grecian, W. J., et al. (2012). Sex-specific foraging behaviour in northern gannets Morus bassanus: incidence and implications. Mar. Ecol. Prog. Ser. 457, 151–162. doi: 10.3354/meps09734
Stier, A., Schull, Q., Bize, P., Lefol, E., Haussmann, M., Roussel, D., et al. (2019). Oxidative stress and mitochondrial responses to stress exposure suggest that king penguins are naturally equipped to resist stress. Sci. Rep. 9:8545. doi: 10.1038/s41598-019-44990-x
Sullivan, M. S. (1994). Mate choice as an information gathering process under time constraint: implications for behaviour and signal design. Anim. Behav. 47, 141–151. doi: 10.1006/anbe.1994.1016
Swanson, D. L., and Thomas, N. E. (2007). The relationship of plasma indicators of lipid metabolism and muscle damage to overnight temperature in winter-acclimatized small birds. Comp. Biochem. Physiol. A Mol. Integr. Physiol. 146, 87–94. doi: 10.1016/j.cbpa.2006.09.004
Sydeman, W. J., Mills, K. L., Santora, J. A., Thompson, S. A., Bertram, D. F., Morgan, K. H., et al. (2009). Seabirds and climate in the California current – A synthesis of change. CalCOFI Rep. 50, 82–104.
Taborsky, B., and Taborsky, M. (1999). The mating system and stability of pairs in kiwi Apteryx spp. J. Avian Biol. 30, 143–151. doi: 10.2307/3677123
Totzke, U., Fenske, M., Huppop, O., Raabe, H., and Schach, N. (1999). The influence of fasting on blood and plasma composition of herring gulls (Larus argentatus). Physiol. Biochem. Zool. 72, 426–437. doi: 10.1086/316675
Uchiyama, M., and Mihara, M. (1978). Determination of malonaldehyde precursor in tissues by thiobarbituric acid test. Anal. Biochem. 86, 271–278. doi: 10.1016/0003-2697(78)90342-1
Verhulst, S. (2020). Improving comparability between qPCR-based telomere studies. Mol. Ecol. Resour. 20, 11–13. doi: 10.1111/1755-0998.13114
Vleck, C. M., and Vleck, D. (2002). Physiological condition and reproductive consequences in Adelie penguins. Integr. Comp. Biol. 42, 76–83. doi: 10.1093/icb/42.1.76
Wegmann, M., Voegeli, B., and Richner, H. (2015). Oxidative status and reproductive effort of great tits in a handicapping experiment. Behav. Ecol. 26, 747–754. doi: 10.1093/beheco/arv006
Weimerskirch, H. (2002). “Seabird demography and its relationship with the marine environment” in Biology of Marine Birds. eds. E. A. Schreiber and J. E. Burger (Boca Raton, FL: CRC Press), 115–136.
Williams, T. D. (2012). Physiological Adaptations for Breeding in Birds, Princeton, NJ: Princeton University Press.
Williams, T. D. (2018). Physiology, activity and costs of parental care in birds. J. Exp. Biol. 221, 1–8. doi: 10.1242/jeb.169433
Williams, T. D., and Fowler, M. A. (2015). Individual variation in workload during parental care: can we detect a physiological signature of quality or cost of reproduction? J. Ornithol. 156, 441–451. doi: 10.1007/s10336-015-1213-6
Williams, S. E., Shoo, L. P., Isaac, J. L., Hoffmann, A. A., and Langham, G. (2008). Towards an integrated framework for assessing the vulnerability of species to climate change. PLoS Biol. 6:e325, 2621–2626. doi: 10.1371/journal.pbio.0060325
Wright, J., and Cuthill, I. (1989). Manipulation of sex differences in parental care. Behav. Ecol. Sociobiol. 25, 171–181. doi: 10.1007/BF00302916
Keywords: parental effort, behavioral flexibility, oxidative stress, nutritional status, seabirds, physical condition
Citation: Pelletier D, Blier P, Vézina F and Guillemette M (2023) Good times bad times — Unfavorable breeding conditions, more than divorce, lead to increased parental effort and reduced physiological condition of northern gannets. Front. Ecol. Evol. 11:1108293. doi: 10.3389/fevo.2023.1108293
Edited by:
Andrea Grunst, Université de la Rochelle, FranceReviewed by:
Manrico Sebastiano, UMR7372 Centre d'études biologiques de Chizé (CEBC), FranceJose A. Masero, University of Extremadura, Spain
Copyright © 2023 Pelletier, Blier, Vézina and Guillemette. This is an open-access article distributed under the terms of the Creative Commons Attribution License (CC BY). The use, distribution or reproduction in other forums is permitted, provided the original author(s) and the copyright owner(s) are credited and that the original publication in this journal is cited, in accordance with accepted academic practice. No use, distribution or reproduction is permitted which does not comply with these terms.
*Correspondence: David Pelletier, ZGF2aWQucGVsbGV0aWVyQGNlZ2VwLXJpbW91c2tpLnFjLmNh