- 1U.S. Geological Survey, Western Ecological Research Center, San Diego, CA, United States
- 2U.S. Forest Service, Sierra National Forest, Prather, CA, United States
- 3Hobbs Ecology, San Jose, CA, United States
- 4Dokken Engineering, San Diego, CA, United States
- 5Anthony Hardwood Composites and Emtek Matting Solutions, Sheridan, AR, United States
Introduction: Designs for safe and effective road crossing structures for small animals are typically under-road microtunnels and culverts which have varying levels of effectiveness reported in the scientific literature. Many species, particularly migratory amphibians, may have limited ability to find and use passages if they are too far apart, resulting in substantial barrier effects.
Methods: We designed a novel open elevated passage (elevated road segment: ERS), similar to a low terrestrial bridge, that could theoretically be built to any length based upon species needs and movement characteristics. A 30 m length prototype ERS was installed along a forest road with a history of amphibian road mortality in Sierra National Forest, Fresno County, CA, USA. From 2018 to 2021, we monitored small animal activity under the ERS in relation to surrounding roadside and forest habitats using active infrared cameras.
Results: We documented a total of 8,815 unique use events, using species specific independence criteria, across 22 species of amphibians (3), reptiles (4), and small mammals (15). Poisson regression modeling of taxonomic group activity under the ERS, roadside and forest, showed that amphibian activity was highest in the forest habitat, no differences were observed for reptiles, and small mammal activity was highest under the ERS. However, mean activity estimates under the ERS were equal to or greater than the open roadside habitat for all 22 species, suggesting that adding cover objects, such as downed logs and vegetation may further enhance passage use.
Discussion: Overall, results showed that the design of the ERS crossing has potential to provide high connectivity for a wide range of amphibian, reptile, and small mammal species while reducing road mortality. ERS systems can also be used in areas with challenging terrain and other hydrological and environmental constraints. Incorporating current road ecology science, we provide supplemental ERS concept designs for secondary roads, primary roads and highways to help increase the options available for road mitigation planning for small animals.
1. Introduction
Directly and indirectly, roads represent a substantial threat to wildlife at individual, population, and meta-population levels (e.g., Forman et al., 2003; Andrews et al., 2015; van der Ree et al., 2015). Directly, roads affect wildlife via road-associated mortality, which can threaten population persistence by reducing survivorship (Gibbs and Shriver, 2002; Ceia-Hasse et al., 2018; Barrientos et al., 2021). Indirectly, species behavioral avoidance of roads and altered roadside habitats can reduce population connectivity resulting in habitat fragmentation effects (Jackson and Fahrig, 2011; van der Ree et al., 2015; D’Amico et al., 2016). Not all roads are equal in their impacts, but negative impacts have been documented from low-use unpaved roads to high-use multi-lane highways for many wildlife species (Goosem, 2002; Forman et al., 2003; Andrews et al., 2008; Dean et al., 2019).
Species at greatest risk of negative road impacts are those with large movement distances or home ranges that encounter roads as part of daily, seasonal, or annual movements, those that are slow moving, and those that do not exhibit road or vehicle avoidance behaviors (Jaeger and Fahrig, 2004; Glista et al., 2008; Fahrig and Rytwinski, 2009; Rytwinski and Fahrig, 2012, Brehme et al., 2018). For example, many reptiles and amphibian species are particularly susceptible to the negative impacts from roads as they slowly migrate among different habitats to meet their basic life history requirements, such as breeding, development, foraging, and overwintering (e.g., Gibbs and Shriver, 2002; Andrews et al., 2008; Beebee, 2013). Many reptiles may be attracted to the road surface to thermoregulate (Jochimsen et al., 2014). Similarly, amphibians often do not avoid roads or may be attracted to roads during or after rainfall to easily absorb surface water through their permeable ventral skin (Beebee, 2013). Once on roads, they often “freeze” in response to oncoming traffic, further exacerbating the risk of road mortality (Andrews and Gibbons, 2005; Mazerolle et al., 2005). When roads intersect vital habitats for these species, it can result in reduced gene flow, lower population sizes and increased probability of extirpation (e.g., Gibbs and Shriver, 2002; Semlitsch, 2008; Van Buskirk, 2012; Beebee, 2013; Brehme et al., 2018).
Road avoidance behaviors that can fragment populations have also been documented for reptiles and small mammals. For instance, Andrews and Gibbons (2005) found that smaller snakes were much more likely to avoid roads than larger snakes. Similarly, smaller mammals, particularly closed-habitat specialists, are more likely to avoid roads of varying traffic volumes, and there is evidence that multiple species may be repelled by paved road substrates (Goosem, 2002; McGregor et al., 2008; Brehme et al., 2013; Ascensão et al., 2016; Chen and Koprowski, 2019).
Finally, many herpetofauna species migrate among wide swaths of terrestrial and aquatic habitats using straight line trajectories (Matthews and Pope, 1999; Sinsch, 2006; Liang, 2010; Southwood and Avens, 2010), and small mammals move among suitable patches of habitat or along road verges (Gunson et al., 2011). Therefore, road mortality for many small animals is often not concentrated at a particular corridor or “hot spot.” Instead, high roadkill numbers are often spread along many meters or kilometers of roads (Glista et al., 2008; Matos et al., 2012; Garrah et al., 2015).
Current practice to mitigate negative impacts on migrating amphibians and other small animals is to install one or several small tunnels under the roadway with stand-alone barrier fencing to help lead animals to the passages (Schmidt et al., 2008; Andrews et al., 2015; Langton and Clevenger, 2021). However, there is still a need for more studies on their effectiveness (Lesbarreres and Fahrig, 2012; Beebee, 2013; Langton and Clevenger, 2017). Recent studies have also found that migrating amphibians will turn around after a short distance if they do not readily find a passage after encountering road barrier fencing. For instance, migrating common toads (Bufo bufo), California tiger salamanders (Ambystoma californiense), and Yosemite toads (Anaxyrus canorus) have average “give-up” distances of 40–50 m along road fencing although they migrate a kilometer or more (Ottburg and van der Grift, 2019; Brehme et al., 2021, 2022a). This behavior can result in a “filter effect” that could be more detrimental to the population than road mortality by substantially reducing connectivity and breeding opportunities (Allaback and Laabs, 2002-2003; Ceia-Hasse et al., 2018; Ottburg and van der Grift, 2019). “Give-up” distances directly inform passage spacing as distance between built passages can be optimized for differing target levels of permeability. To our knowledge, it is unknown if this phenomenon occurs with reptiles and small mammals, although for non-migratory species, passage spacing greater than the width of a core home range would be expected to filter movements among individuals of populations separated by road fencing.
Installing passages underneath the road surface with raised barrier fencing often requires some grading and recontouring slopes to the passage entrance(s) which can potentially damage natural resources or change hydrological flows. Considering these factors and limitations at our study site, we designed a novel small animal road passage we call an elevated road segment (ERS). The ERS is an elevated road passage that can be built to any length and is permeable to light and rainfall, allowing for a wetted passage for amphibians moving during rainfall events. The ERS prototype was installed directly on top of a forest road with a history of substantial amphibian road mortality, particularly of federally threatened Yosemite toads (Vaughan et al., 2023), in Sierra National Forest, Fresno County, CA, USA. Although Yosemite toads were the impetus of this project, SNF harbors a wide variety of amphibians, reptiles, and small mammals that may be at risk from road related impacts.
The first objective of this study was to evaluate the efficacy of the ERS by monitoring the relative activity of amphibians, reptiles, and small mammals under the ERS, in comparison to adjacent roadside habitat and interior forest habitat. We postulated that any activity under the ERS would represent some level of permeability to movement for small animal species and that equal levels of activity between the ERS and adjacent habitat would represent high levels of permeability to movement, suggesting no avoidance. If the ERS was effective, our second objective was to provide suitability considerations and engineered concept designs for transportation planners and conservation agencies to apply the open ERS passage concept to a wide variety of road types (low-use unpaved roads to high-use highways).
2. Materials and methods
2.1. Study site
Our study took place on US Forest Service (USFS) road 9S09 in Sierra National Forest, Fresno County, CA, USA from 2018 to 2021 (Figure 1). The road was the focus of mitigation as it bisects breeding meadow and upland habitat for the federally threatened Yosemite toad (Brehme et al., 2022a; Vaughan et al., 2023). The conifer forest is primarily dominated by lodgepole pine and wet meadow habitat that include sandy lupine areas, herbaceous plants, rocky outcroppings, rotting tree stumps, fallen logs, and leaf litter.
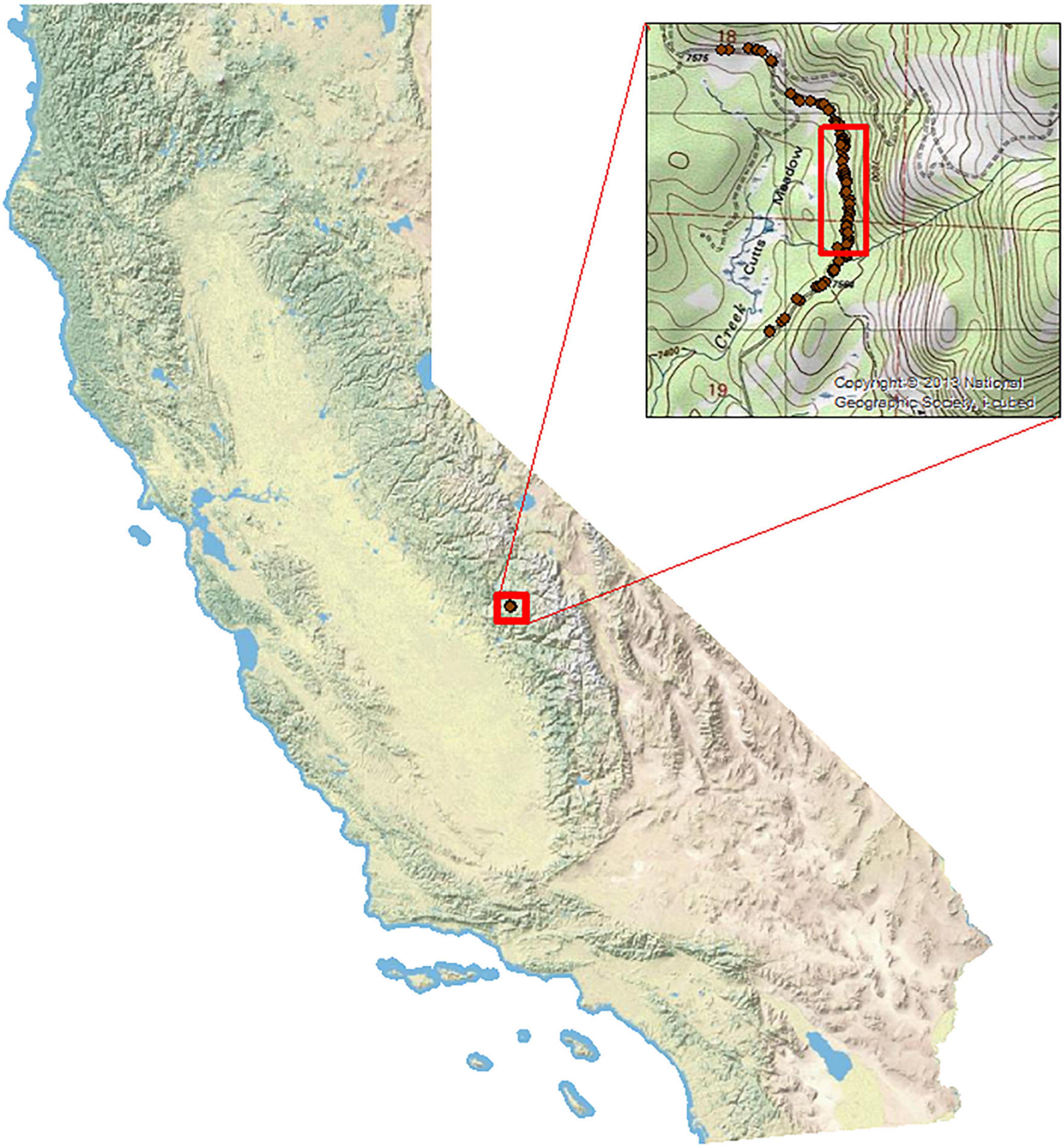
Figure 1. Study area in Sierra National Forest, Fresno County, CA, USA. Dark red points represent Yosemite toad mortalities in 2017 prior to installation of the elevated road segment (ERS) and barrier fencing (footprint designated by red open rectangle).
In June 2018, the ERS was installed on top of a solid impervious aggregate roadbed (USFS Rd. 9S09). The prototype ERS is a 30 m long and 4.9 m wide road crossing structure that was placed on top of the existing road surface and is composed of hardwood laminated billet road mats that are designed for use by heavy equipment at construction sites (Emtek®; Figure 2). The road mats are approximately 15 cm thick, installed on top of 20 cm (8 in.) high laminated billet support bars placed approximately 2 m apart directly on and perpendicular to the road, that allows for passage of small animals underneath. In addition, the ERS is permeable to light and rainfall, allowing a wetted passage for amphibians moving during rainfall events. It was built to meet codes and specifications for USFS, County, and City roads and can theoretically be built to any length, width, or at increased heights depending upon the size of the supports used.
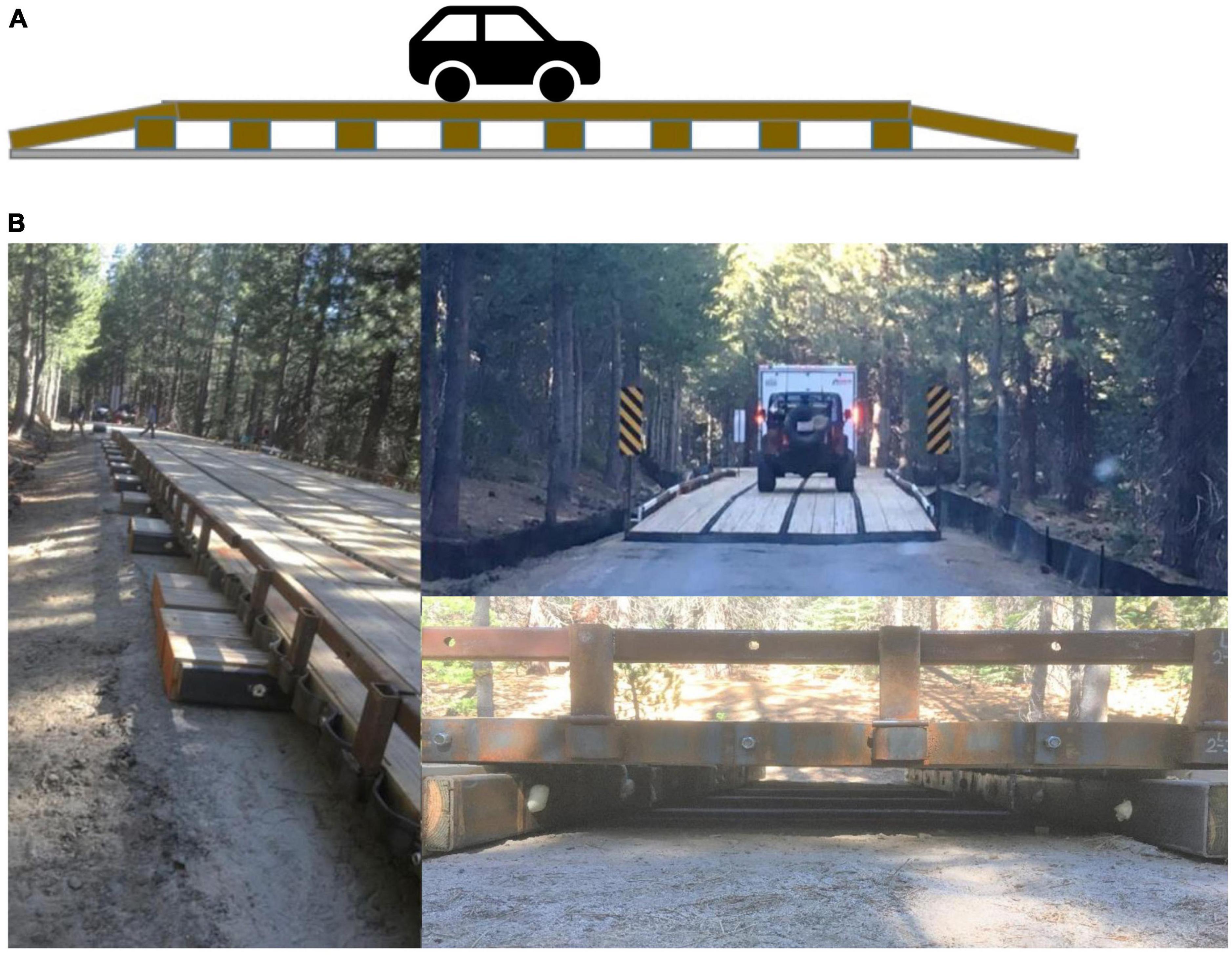
Figure 2. Diagram (A) and photos (B) of elevated road segment in Sierra National Forest, Fresno County, CA, USA.
Approximately 120 m lengths of polymer mesh and solid barrier fencing were installed along both sides of the road leading to the ERS passage [ERTEC® rigid polymer matrix E-Fence™ and Animex® high-density polyethylene (HDPE-2)]. Jump-outs (ERTEC® cones and high berms) were installed a minimum of every 10 m along the fence to provide toads and other small vertebrates opportunities to safely return into the habitat if they incidentally accessed the road within the barrier fencing. The first 15 to 20 m of fencing connected to the ERS passage spanned roadside habitat that was clear of most trees and leaf litter, while the remaining fencing spanned out into forested habitat. At outer fence ends, turnarounds were installed to redirect animals away from the road and back toward the habitat in a U-shaped fashion. The turnarounds were approximately 2 m long and 1 m in width. Fencing was installed with the bottom buried in the ground according to manufacturer’s guidelines. The length of the fencing is longer than would be recommended for high permeability of toad movement on each side of the passage; however, this was done as part of a concurrent study on fence movement behavior for the Yosemite toad (Brehme et al., 2022a).
2.2. Study design
A total of 18 HALT® active-trigger camera systems (Hobbs and Brehme, 2017) were used to monitor small animal activity along the fencing in the habitat leading to the ERS and then immediately adjacent and under the ERS. The system uses an active infrared trigger beam integrated into a standard 37 cm threshold so that when animals pass over the threshold and intercept the beam, the camera is triggered. All cameras were programmed to take a short video or 3 rapid-fire photos with a 5 s interval set between possible trigger events.
Fourteen cameras were placed along fencing on one side of the road (west) every 20 m and at the end of fence end turnarounds (Figure 3). The cameras were set only along the west fence line that contained forest habitat adjacent to a Yosemite toad breeding meadow. Fence camera placements and differences in barrier fence types also supported a concurrent study on Yosemite toad fence movement distances as they migrate from the meadow (Brehme et al., 2022a). The number of cameras available did not support monitoring both sides of the road. In 2019, we added an additional camera between the 2 cameras closest to the ERS on each side to increase sampling of adjacent roadside habitat. The closest cameras to the ERS along the fence were approximately 8 m from each side of the ERS opening to shield the cameras from the view of forest visitors.
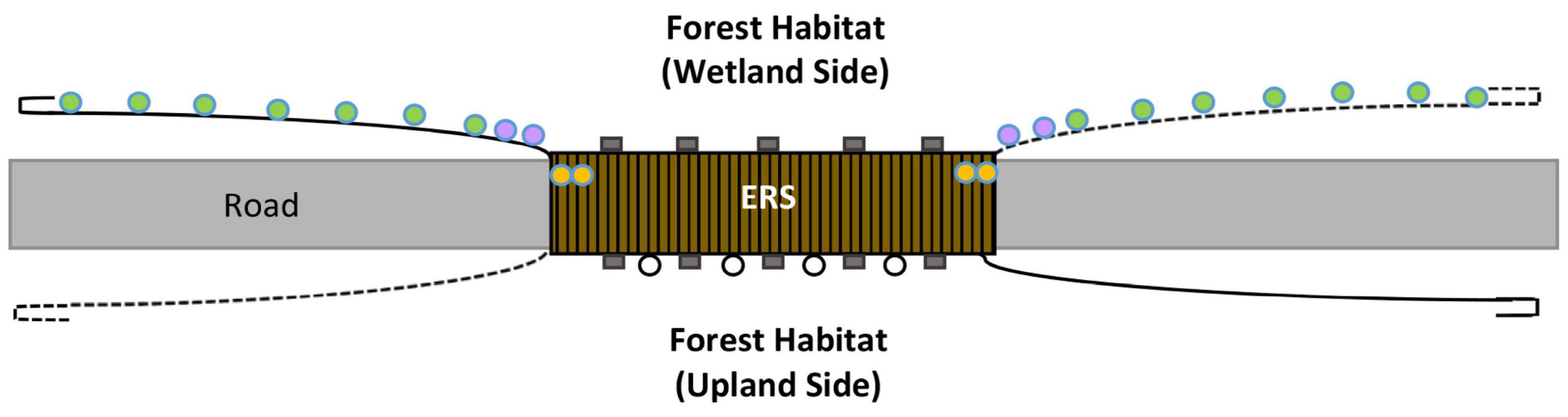
Figure 3. Schematic of elevated road segment (ERS), HALT cameras (circles: green = forest, purple = roadside habitat, and yellow = under ERS), and time-lapse cameras (black open circles; 4 shown of 8); not to scale.
The expansive length of the ERS underpass made it impossible to sample completely. We placed one HALT camera system under both sides of the ERS, where it intersected with the fence line, to best capture photos of animals that entered the ERS after moving along the fence. After the first year, we used triggers with an extended 1 m trigger length to better cover the passage opening and to help capture images of animals that did not take a hard turn into the structure. Therefore, we targeted animals moving along the fence line and directly into the structure. The under ERS camera triggers were placed a minimum of 30 to 60 cm deep into the passage to ensure animals were fully under the ERS. Additional HALT cameras and triggers were added within the same passage segments in 2020 and 2021 for better coverage (Brehme et al., 2022a), but these were not used in the analysis due to very low activity, as most animals crossed close to the fence line. Finally, we also installed eight Reconyx cameras (factory set with 3–5 m focal distances with a 60-degree field of view) under multiple subsections of the east side of the ERS (facing west); they were set to a time lapse of every 5 min to gather more data on Yosemite toad specific movements. Due to the vast number of photos generated by these cameras, they were only analyzed only for Yosemite toads during rain events and during times when toads were detected on habitat cameras along the fence line.
Each year, all cameras were set as soon as the snow melted and the road opened. Cameras were checked and reset on a weekly basis during the late spring and summer (May–October 2018, July–October 2019, June–September 2020, May–October 2021), and ran for a total of 10,546 days. Total summer precipitation in nearby Huntington Lake during the monitoring periods was approximately 23 mm for 2018 (June–October), 33 mm for 2019 (July–October), 10 mm for 2020 (June–September), and 18 mm for 2021 (May–October) after the snow melt (California Nevada River Forecast Center).1 Summer seasons were approximately 76 mm or more below average rainfall during these periods (Western Regional Climate Center 044176-5).
In 2020, cameras were removed in early September due the “Creek Fire,” which burned almost 400,000 acres in Sierra National Forest and right up to the edge of the Yosemite toad breeding meadow adjacent to our study site. After the fire, as part of suppression repair work, the USFS piled downed wood and debris next to the study area and close to the fence line. In addition to below normal rainfall, it is unknown how these factors affected small animal activity or numbers. Road mortality surveys were also conducted along a 2 miles section of 9S09 that encompassed the ERS study site by the USFS in all years (Vaughan et al., 2023).
2.3. Analysis
All photos from the HALT cameras were reviewed and animals identified to species. Due to the extremely large number of time-lapse photos from Reconyx cameras, all photos that were within 1 h of any Yosemite toad HALT camera detection were reviewed. Therefore, time lapse Reconyx camera data was specific for Yosemite toad use only.
To assess relative ERS permeability for all species, results were analyzed for 3 general locations: (1) under the ERS passage, (2) along the roadside habitat immediately outside the ERS passage (within 0–28 m of passage and <5 m from the road), and (3) within the interior forest habitat approximately 28 m from passage and >5 m from road). Roadside habitat designation was based on a general lack of ground cover on the west side of the road immediately adjacent to the ERS, in comparison to abundant trees, shrubs, woody debris, and leaf litter at approximately 5 m and beyond. We identified all animals to species or genus and processed images into animal records using CamtrapR v. 2.2.0.7 (Niedballa et al., 2016). We conservatively considered images for non-migrating species to represent unique, independent use events when a minimum of 30 min had passed between the last unique observation of a species at the same camera (Si et al., 2014; Laughlin et al., 2020; Vilella et al., 2020). For Yosemite toads migrating from wetland to upland habitats, we used a 5-min window to reduce the likelihood of excluding multiple individuals moving in pulses such as during rainfall events. For modeling, species counts represented the sum of unique use events per year at each camera location. Controlling for variation in temporal activity among years, abundance of use (hereby referred to as “activity”) was a relative measure of frequency of space-use by each species (Gilbert et al., 2021) and represented general patterns in species activity across spatial and temporal gradients.
To analyze the use of the ERS by amphibians, reptiles, and small mammals, we modeled the activity at each individual camera using Poisson regression models with Bayesian inference (Kery et al., 2009; Yamaura et al., 2016; Gould et al., 2022). Our observed data are yikt, where y is the count (Poisson regression) of species k, use events at camera i, in year t. In contrast to Brehme et al. (2022a), where an n-mixture model was used to analyze activity data, Poisson regression does not include a model for observation process, as these data may violate population closure assumptions required for N-mixture models (Royle, 2004). Poisson regression remains a robust tool for estimating relative abundance when N-mixture model assumptions are not met (Barker et al., 2018). Active trigger cameras are not subject to differences in detection probability due to variations that are inherent in passive infrared cameras (Hobbs and Brehme, 2017), and differences in camera activity that could lead to increased counts were accounted for by effort (number of days active). Therefore, beyond these, we assumed all cameras have equal detection probabilities, and therefore, patterns in activity observed here are not systematically biased.
For the community Poisson regression, the relative abundance model for counts yikt is defined:
where w indicates if camera i was active during year t, and λikt is the rate parameter of species k at camera i, during year t. We estimated λikt with a log link-function and included the covariates about camera location and days of camera activity (effort):
where each covariate now corresponds to the change in activity on the log scale. In this case, β0k corresponds to the relative activity of animals for cameras under the ERS, while βroadside,k and βforest,k represent the relative effects of roadside and forest habitats on activity, respectively. The parameter βeffort,k accounts for differences in effort among camera location and years. We also included an observation-level random-effect, εikt, which is useful in accounting for over-dispersion present in the data (Harrison, 2014).
All model parameters were specified as either centered or non-centered random effects, with shared precision parameters. Specifically, we specified the prior for β0 as being a non-centered, normally distributed random prior.
All other beta parameters were specified with centered, normally distributed priors, centered on parameter-specific hyper-parameter means and standard deviations.
Finally, we specified the prior for the observation-level random-effect, εikt, to be normally distributed with a mean of zero and a gamma distributed standard deviation, with shape and rate parameters of 0.9.
Models were run in JAGS v 4.3.0 (Plummer, 2003) using R v. 4.1.2 (R Development Core Team, 2013) with package jagsUI (V 1.5.2; Kellner, 2015). Models were run for 100,000 iterations with an adaptation of 1,000 iterations, a burn-in of 1,000 iterations, and a thinning rate of 5, across 6 chains, yielding a total of 59,400 posterior samples. We visually inspected trace plots for model convergence and considered all parameters to have converged when Gelman–Ruben values were <1.10. We examined model explanatory power by comparing Freeman-Tukey residuals for the observed and model generated dataset. We calculated a Bayesian P-value for the model by calculating Freeman-Tukey residuals across sites, years, and species. Values close to 0.5 indicate high explanatory power. We summarized all parameters using 90% Highest Density Intervals (HDI), and effects were considered strong if HDI intervals did not include zero. We also report Poisson rate ratios (relative species activity in forest or roadside in comparison to under ERS) and effects were considered strong if HDI intervals did not include one.
3. Results
Across 4 years of sampling comprising 10,546 camera trap 24-h periods (cameras x trap periods), we observed 8,850 unique use events, given our species specific independence criteria. These included 6,742 observations of mammals, 1,515 observations of amphibians, and 593 observations of reptiles, across 22 species (Table 1). The most common species included Peromyscus mice (Peromyscus spp., n = 5,097) and Pacific tree frogs (Pseudacris regilla, n = 1,160). Lower numbers of animals in general were documented in 2019, likely due to the shorter monitoring period that year, from a late snow melt and other factors.
3.1. Activity patterns
Activity patterns of the species-groups within our study generally fit either nocturnal or diurnal activity (Figure 4). All three species of amphibians, 7/15 species of mammals, and 1/4 reptiles exhibited nocturnal activity patterns. The remaining reptiles and mammal species exhibited more diurnal activity patterns, although Douglas squirrel activity exhibited a peak near sunrise, consistent with crepuscular activity. Spatially explicit species count indices in relation to unique camera locations for each species are shown in Figure 5.
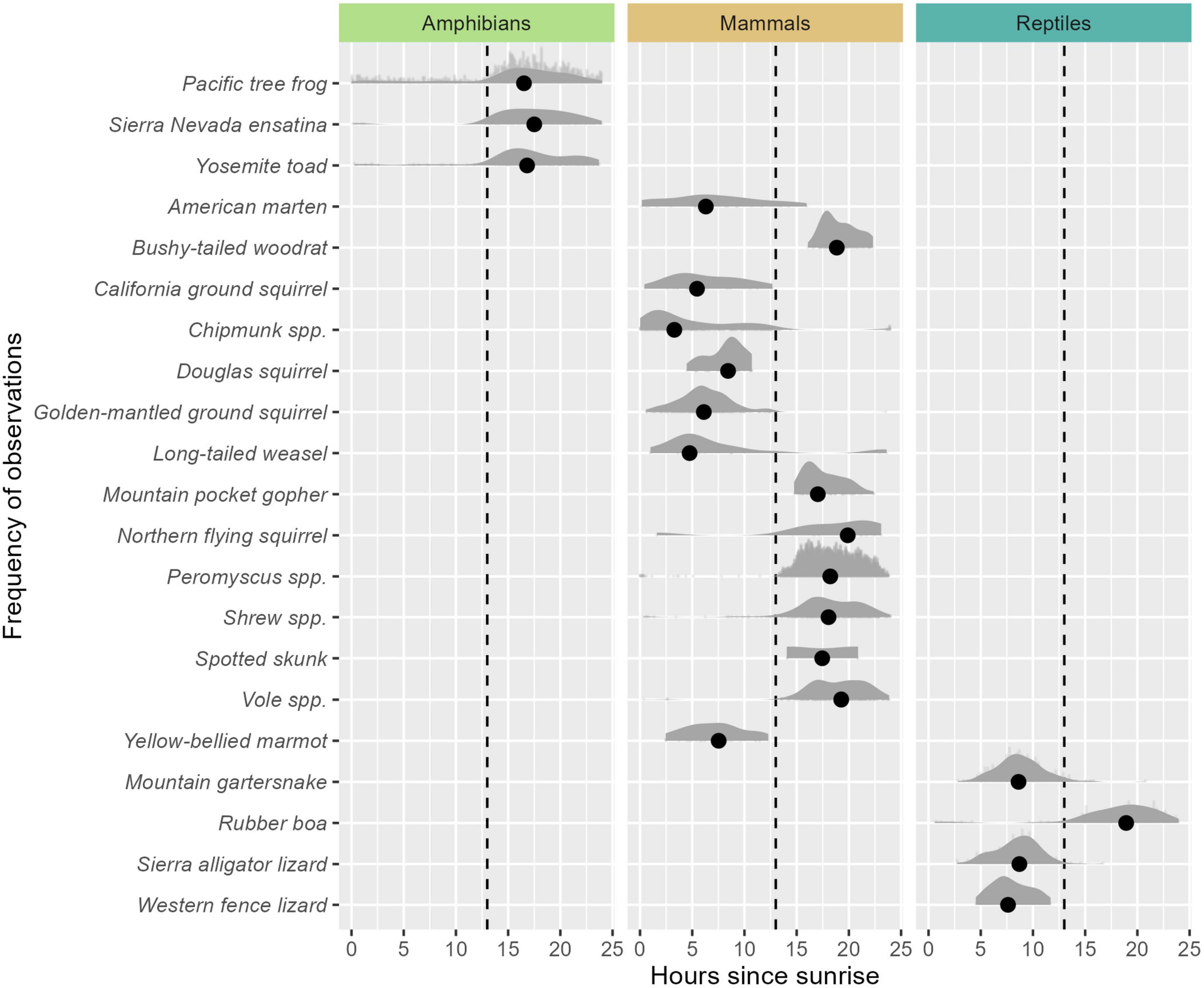
Figure 4. Temporal activity patterns of animals detected represented by scaled density. Sunrise times are based on 6:00 A.M., PST and represent an approximation of when diurnal or crepuscular activity may be expected to be observed. The dashed line indicates 7:00 P.M. PST and represents an approximate average sunset time across the study period. Activity density curves to the left of the dashed line indicate animals with diurnal activity. Activity density curves to the right of the dashed line indicate animals with more nocturnal activity patterns. Dots represent the mean time-since-sunrise of all observed images.
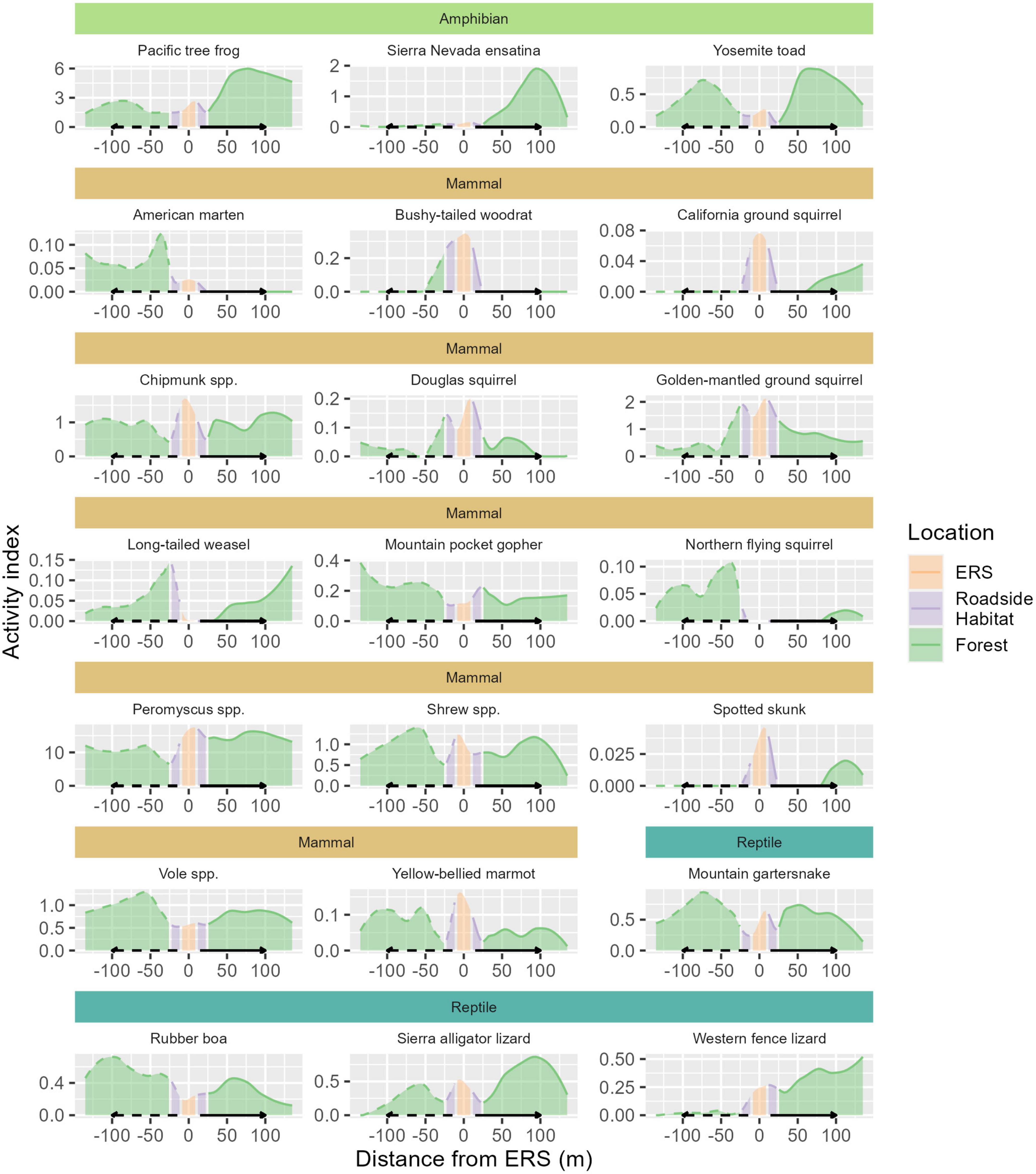
Figure 5. Spatial activity patterns of animals detected among specific camera locations within the elevated road segment (ERS), roadside habitat, and forest, for 21 species groups. ERS cameras are indicated by orange shading, roadside habitat cameras are shaded purple, and forest cameras are shaded green. Each species has a unique y-axis scale, for visual purposes. The x-axis represents the distance from ERS passage (0) in meters.
3.2. Poisson count model
Overall, the Poisson model showed animal activity was strongly associated with location and effort (Table 2). The community-wide response to roadside habitat was significantly negative, indicating roadside habitat had less overall species activity compared to under the ERS (Table 3). There was also evidence that overall activity was lower in the interior forest, compared to under the ERS (0.69×, 0.39–0.98); however, there was considerably more uncertainty (SD = 1.10) compared to roadside habitat (SD = 0.35) due to species specific responses. Additionally, the community-wide response to camera effort was significantly positive, as increased active time allowed for more observations, and the responses were relatively consistent across species (SD = 0.54).
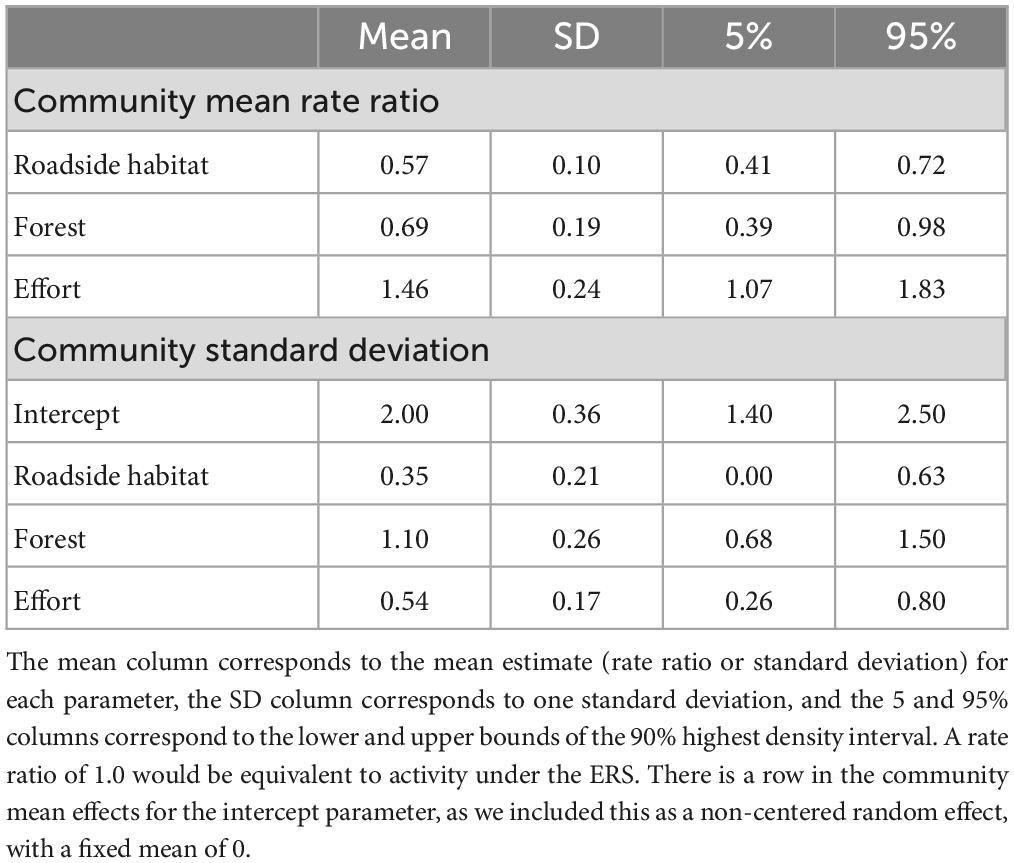
Table 2. Community mean activity rate ratios (compared to ERS) and standard deviation parameters for random effects.
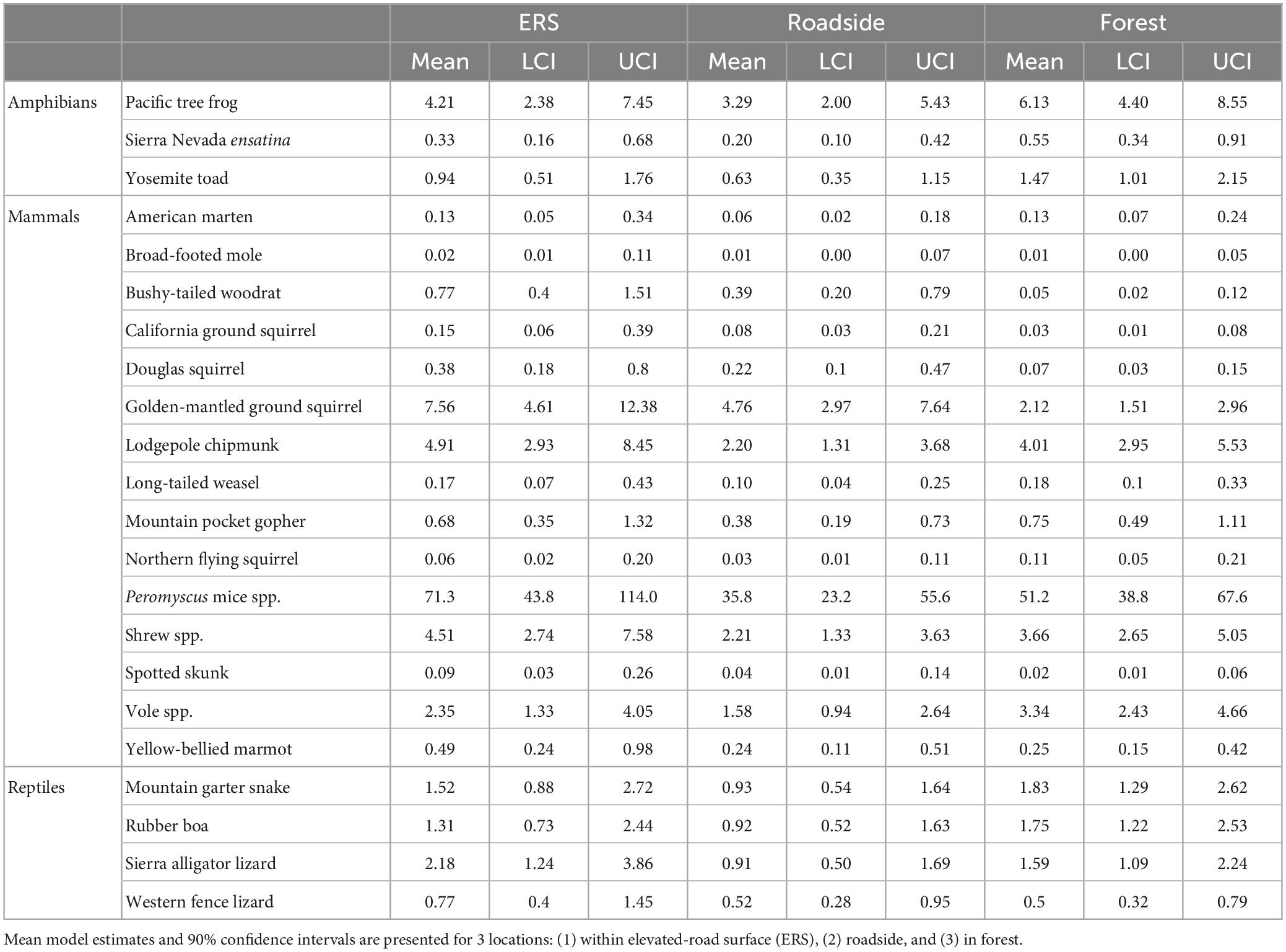
Table 3. Effect of location (under the ERS, roadside habitat, and forest) on the relative activity of amphibians, small mammals, and reptiles.
Within the community, significant taxonomic patterns were present in response to location (Figure 5 and Table 3). Mammal activity was significantly lower in both roadside (mean = 0.53×, 90% HDI: 0.39-0.68) and interior forest (0.52×, 0.37–0.66), in comparison to under the ERS. Amphibians (0.71×, 0.44–0.98) and reptiles (0.60×, 0.42–0.78) were less active in roadside habitat than ERS. Additionally, amphibians were significantly more active in the forest than under the ERS (1.68×, 1.00–2.22).
All mammal species exhibited higher mean activity estimates within the ERS than roadside habitat (Figure 6 and Table 3). Further, 4/14 species (long-tailed weasel, mountain pocket gopher, northern flying squirrel, and voles) had higher mean activity in the forest, although not significantly more, compared to under the ERS. Relative to the taxonomic average effects of location, 5 species (bushy-tailed woodrat, California ground squirrel, chipmunk, Douglas squirrel, and golden-mantled ground squirrel) exhibited less activity in forest than under the ERS (Figure 6 and Table 3).
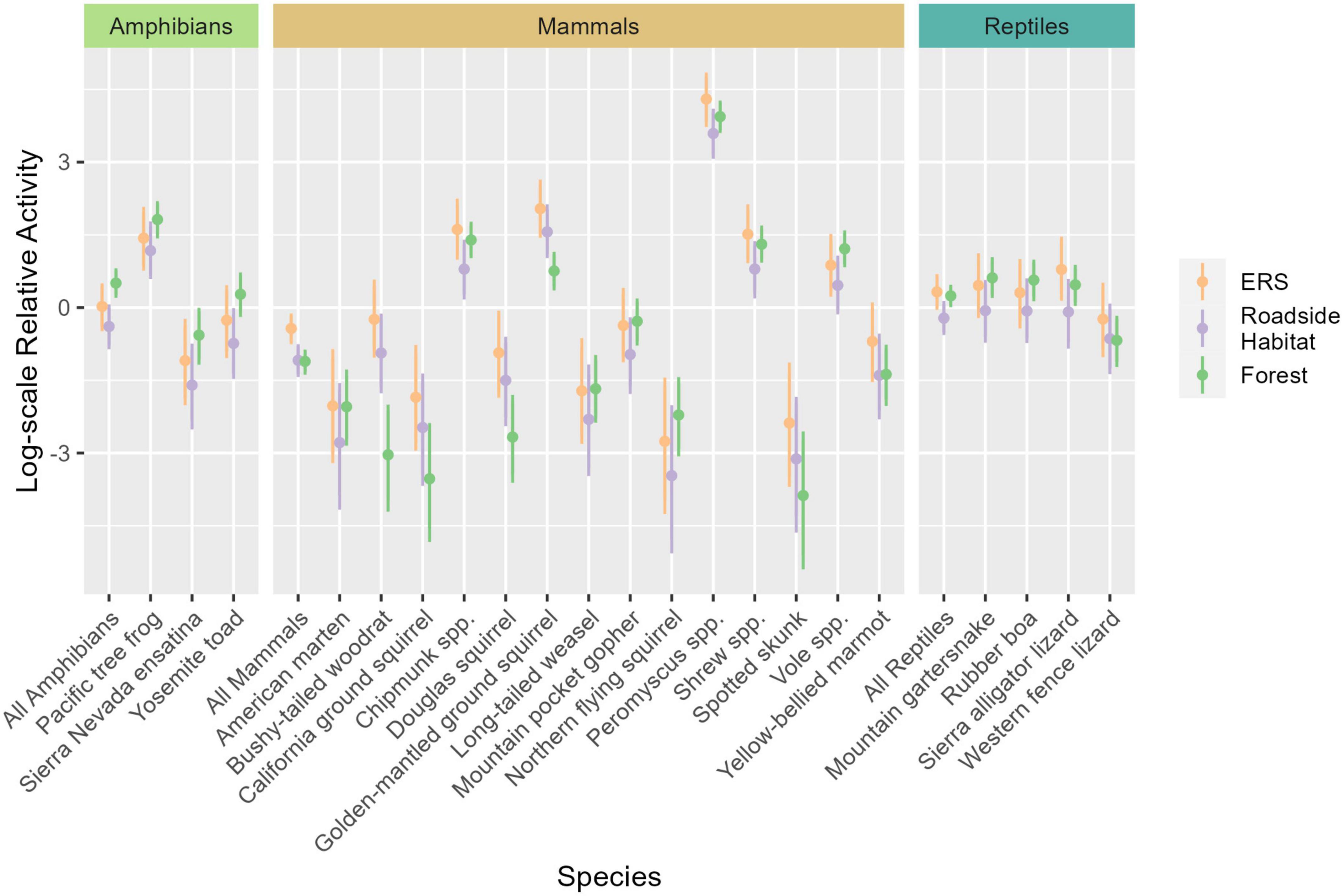
Figure 6. Marginal effect plot showing the difference in relative species activity among the elevated road segment (ERS), roadside habitat, and forest, for 21 species groups. Points represent the mean estimates for each species. Intervals represent the 90% highest density intervals (HDI), with thick lines represent the 75% HDI. Under ERS activity is represented by orange point-intervals, roadside activity is purple, and forest activity is green. The dashed line represents the community-wide average activity. Taxonomic mean effects are derived from the mean of model coefficients for species in that taxon and are represented by the intervals “all amphibians”, “all mammals”, and “all reptiles”.
Amphibian activity patterns were more consistent than patterns observed for mammals. All three species were, on average, more active in the forest than in roadside habitat (Figure 6 and Table 3). Although no amphibian species exhibited significantly greater activity within the forest than under the ERS, the taxonomic average activity for amphibians was significantly greater in the forest.
Reptiles exhibited moderately heterogenous activity patterns relative to location. Sierra alligator lizards exhibited the strongest, and only, significant negative response to roadside habitat (0.41×, 0.22–0.74). Two species, the mountain garter snake and rubber boa, exhibited significantly higher activity in the forest in comparison to roadside habitat, while western fence lizard activity averaged highest under than ERS (Figure 6 and Table 3).
All measures of convergence and explanatory power indicated the model fit the data well. The community Bayesian P-value was 0.53, indicating the model had high explanatory power. Further, all species-level Bayesian P-values were between 0.1 and 0.9, except one (northern flying squirrel, n = 11, BP = 0.93).
4. Discussion
Wildlife crossing designs are known to play a critical role in how effective crossings are for different species. Results showed that the design of the ERS crossing has substantial potential to provide connectivity for a wide range of amphibian, reptile, and small mammal species. Across all species in our study, the results did not suggest species were avoiding the ERS crossing. All small animal species that were detected in the forest habitat were also detected under the ERS, with the exception of the broad-footed mole (n = 1) and northern flying-squirrel (n = 11). Amphibian activity generally trended highest in the forest habitat, while small mammals trended highest underneath the ERS structure. Estimates of activity for all amphibians, reptiles, and small mammals were lower in the more open roadside habitat, in comparison to the forest and under the ERS. These results suggest that adding cover objects, such as downed logs, and/or planting and maintenance of shrubs adjacent to the structure (but not to create access points to the top of the structure) may enhance passage for species that are more likely to avoid open areas (e.g., McDonald and St. Clair, 2004; D’Amico et al., 2015; Jackson et al., 2015). Only 9 small animals (4 frogs, 2 snakes, 2 lizards, and 1 mouse) were documented from weekly road mortality surveys within the project footprint over the 4-year period (S. Barnes, C. Vaughan, USFS, pers. com). Therefore, the evidence suggests the ERS design promotes permeability for small wildlife movement while also minimizing road mortality.
4.1. ERS Permeability: amphibians and reptiles
At the taxonomic group level, amphibian activity was significantly lower in the open roadside habitat compared to the interior forest, while mean activity was higher under the ERS structure than in roadside habitat (but less than forest). Roadside habitat was largely open without trees or shrubs and with little leaf litter in comparison to the interior forest. We postulate that these general trends in amphibian activity are most likely associated with the lack of leaf litter and cover in the roadside habitat. Amphibians other than Yosemite toads (e.g., Pacific tree frogs, Ensatina salamanders) were likely most often actively foraging within their home ranges, rather than migrating. Natural forest habitat with abundant leaf litter from trees and shrubs provides higher soil moisture levels for amphibians, reducing desiccation risk while also providing cover for their invertebrate prey (e.g., Duellman and Trueb, 1994). The ERS structure in the Sierra National Forest was also set on top of a solid impervious aggregate roadbed, and we don’t know if the lack of natural soil under the ERS affected passage use.
Similar to Pacific tree frogs and Ensatina salamanders, the relative mean activity of Yosemite toads under the ERS was higher than in the roadside habitat and lower than in the interior forest. Based on local expert knowledge and Yosemite toad telemetry (Liang, 2010), these toads were likely migrating from breeding meadow to upland habitat through the forest. Individual identification supported this, as individual toads were not documented along the fence on different days within the season, as would be expected if it was primarily used as foraging habitat (Brehme et al., 2022a). Although 2 toads were documented moving from one side of the fence line to the other without interacting with the ERS, 29 individual toads were documented moving under the ERS with no evidence of turning around after entering the passage (20 on HALT trigger, 9 on time-lapse), and we suspect many more toads passed under the crossing but were undetected. The fact that some toads reached but did not turn into the passage suggests that small segments of fencing perpendicular to the fence line and parallel to the passage (e.g., Langton and Clevenger, 2021) may be important to help change their trajectories and more effectively lead animals into the passage. However, a majority of unique Yosemite toads “gave-up” migration after moving back and forth along the extended forest fence lines; they presumably moved back to into the forest habitat (Brehme et al., 2022a). This may also help to explain the higher relative mean activity in the forest in comparison to the roadside for this migratory species and may further support the use of mitigation designs with wide passages and/or frequent crossing opportunities for migrating amphibians.
Reptiles had similar patterns as amphibians, in that 3 out of 4 species (mountain garter snake, rubber boa, Sierra alligator lizard) had lower mean activity estimates in the open roadside habitat; however, mean activity for reptiles under the ERS and in the forest were almost identical. These species also prefer habitats with moisture and shelter opportunities, such as downed wood, logs, and leaf litter (Stebbins and McGinnis, 2012), which is consistent with lower activity in the open roadside habitat. Activity of western fence lizards, a habitat generalist, was similar between roadside and forest habitats and slightly higher under the ERS. These results indicate the ERS was also effective in promoting road permeability to the reptiles in our study site.
4.2. ERS permeability: small mammals
Many small mammal species are known to use road crossings (e.g., Clevenger et al., 2001; Martinig and Bélanger-Smith, 2016; Stewart et al., 2020), and our results support this for the ERS as well. All 14 small mammal species analyzed had higher mean estimates of activity under the elevated road in comparison to the roadside habitat, while 10 species also had equal or greater mean activity estimates under the ERS than in the forest. The ERS offers protection to most small mammals from aerial predators and medium to large carnivores. Therefore, it may be an additional source of refuge or shelter within the surrounding habitat, in addition to ground burrows or trees used for shelter by most small mammal species. No predation of small mammals or herpetofauna was observed under or adjacent to the passage during the study. It is also possible that many of these species may predate on invertebrates or other small vertebrate prey under the ERS. Although evidence of predator-prey interactions within road passages has been mixed (Mata et al., 2015; Caldwell and Klip, 2020; Martinig et al., 2020), in a recent study, mice and woodrats were observed using the safety of ledges and internal structural cover to prey upon invertebrates within large underpasses (Brehme et al., 2022b). Although we did not identify all invertebrates as part of this study, we did positively identify at least 104 occurrences of primarily larger beetles and spiders that set off the camera trigger under the ERS, indicating arthropods were also present under the ERS structure.
Three mammal species had almost no difference in mean activity between the ERS and roadside habitat with significantly higher mean estimates in the interior forest. These were the northern flying squirrel, mountain pocket gopher, and voles (California and/or Montane species). The northern flying squirrel is a forest obligate species that spends much of it time up in trees, while both the mountain pocket gopher and vole species are primarily subterranean, not venturing far from their burrow systems to forage above ground (Jameson and Peeters, 2004). Subterranean species in general may have been less likely to use the ERS, as they have increased sensitivity to degraded and impervious substrate and thus require suitable soils they can burrow under (Russell et al., 2007; D’Amico et al., 2015). We documented 28 detections of voles under the ERS during our study. Previous work in Banff National Park showed two species of specialist voles were absent from road-crossing structures, despite being abundant in connected forest and roadside grasslands (D’Amico et al., 2015). This may indicate the ERS is more permeable to vole movement than under-road culverts.
4.3. Limitations
The passage did present challenges in monitoring due to the wide monitoring area. Because we monitored along barrier fencing outside of the passage, we focused on monitoring the sides of the ERS closest to the barrier fencing that helped to lead animals from the forest to the passage. The width of the camera triggers covered a small proportion of the total width of the passage; therefore, we likely missed a substantial amount of activity from; (1) animals moving along the fence into the ERS that did not pass over a camera trigger and (2) animals that used the ERS passage without interacting with the adjacent barrier fencing. The camera triggers within the passage were up to 2.7 times the length of triggers within the habitat (1 m vs. 37 cm). This was important as many animals moving along the fence may not make a hard turn to enter the passage. The additional length should have helped to accommodate this, and some small bricks were set under the passage to help steer animals over the trigger without obstructing the openness of the passage. The height of the passage was also too low for efficient installation of cameras >1 m deep into the passage. Finally, we attempted to add cameras to the exits to document complete movements; however, with the number of available camera systems, we would have had to narrow the passages under the ERS to make this effective, which would have changed the attributes under the ERS to be closer to a small tunnel. Although we only effectively monitored activity under the west side entrances to the ERS, data from individual Yosemite toads did not show that any individuals that entered the ERS from the west side (over the 1 m trigger) subsequently turned around under the ERS (unpublished data, B. Ewing and C. Brehme, USGS). The HALT cameras with active infrared triggers used in this study have very high probabilities of detecting any animal that moves over the trigger (Hobbs and Brehme, 2017). Future monitoring would benefit from infrared trigger beams that span entire subsections of the passage. Also, advances are still needed in small affordable outdoor cameras to obtain high resolution, wide-angle night photos and videos at short focal lengths to capture the details of slow- and fast-moving small animals in remote settings, and there are some examples of other techniques that have been successful in smaller passages (e.g., Gleeson et al., 2018; Jarvis et al., 2019; Corva et al., 2022).
4.4. ERS designs for secondary roads, primary roads and highways
The prototype ERS in our study can theoretically be made to any length, creating a wide passage without constricting migratory movements to small tunnels (Supplementary Data Sheet 1; 2018 cost was approximately $40K USD). The prototype ERS also allows natural light, moisture and rainfall to permeate the length of the passage so that climate and moisture underneath is similar to that outside. The ERS system installed in the Sierra National Forest was built to meet USFS, City and County road specifications and can be removed and re-installed as desired (e.g., on a seasonal basis). This ERS has been in operation since 2018 with frequent use of off-road vehicles, large recreational vehicles, logging trucks, and fire trucks. Semi-annual to annual checks and maintenance have been required to ensure all bolts and connectors are tight. Replacement of wood mats or portions thereof may be required in the future. However, with ongoing regular maintenance, these may be permanent structures (Jon Fiutak, Anthony Composites, pers. com). Similar structures fabricated with other materials could also be designed.
For high-use primary roads and highways, we worked with engineers (Dokken engineering) to develop concept ERS designs that range from open low bridge type designs (pre-cast longitudinal bridge and pre-cast horizontal bridge) to less costly repeating passage designs along a raised roadbed (repeating elevated pre-cast box culverts or repeating short span pre-cast abutments: Supplementary Data Sheet 2). They include guardrails for traffic safety and can be designed to accommodate shoulders if necessary. Climbing barriers for small animals are also included along abutments and on the roadside walls between passages. Further information about these is provided in Brehme et al. (2022a). These engineered concept designs are meant to provide a starting point for local and DOT engineers to design and build permanent ERS type structure(s) to enhance the movement of migratory amphibians and other small wildlife species over wide stretches of high use roadways. They are planning level documents; therefore, additional engineering and reinforcements may be added to meet specific road requirements. For repeating passage designs, the distance between passages may be informed by fence movement distance studies of target or similar species, such as those previously conducted with common toads, California tiger salamanders, and Yosemite toads (Ottburg and van der Grift, 2019; Brehme et al., 2021, 2022a), or potentially informed by home range lengths for non-migratory species.
4.5. ERS and current passage design considerations
Overall, permeability of passages to small animal movements appear to vary greatly according to species behavior, location, passage spacing, and passage characteristics (e.g., Lesbarrères et al., 2004; Pagnucco et al., 2012; Beebee, 2013; Langton and Clevenger, 2017; Hedrick et al., 2019; Jarvis et al., 2019; Matos et al., 2019; Schmidt et al., 2020; Boyle et al., 2021). Recent evidence and literature reviews are starting to indicate that wider passages are generally more permeable to more small animal species than small tunnels (e.g., Martinig and Bélanger-Smith, 2016; Langton and Clevenger, 2017; Schmidt et al., 2020), although small tunnels have been shown to be effective for species in some locations, particularly where spacing between passages was short (i.e., Helldin and Petrovan, 2019; Jarvis et al., 2019). Finally, passages that provide microhabitat conditions (e.g., temperature, substrate, light, and vegetation) similar to the surrounding habitat have been shown to most effective [see reviews by Jackson et al. (2015), Langton and Clevenger (2017), and Gunson and Huijser (2019)].
In line with the current knowledge and guidance for small animal crossing systems, the ERS was designed so that target species can move along a relatively natural terrain path and cross the roadway with conditions similar to the surrounding habitat (Gunson and Huijser, 2019; IENE, 2020; Langton and Clevenger, 2021). All ERS passage designs (Supplementary Data Sheets 1, 2) have a suggested height of at least 0.3 m, and passage opening widths of 1.5 m wide or greater for repeating passage designs on a 2-lane roadway, to ensure better openness for high permeability to animal movement. Wider passages may be considered for wider multi-lane roadways. Except for ERS systems placed on top of roads, the designs incorporate natural soil bottoms. However, the “top of road” systems, like the prototype ERS in our study, allow for ease in installation and removal (e.g., seasonally) for lower use roadways. Grated openings on top of the ERS serve to decrease temperature and moisture differentials between the passage and outside environment, allowing some natural light within the passage, as well as moisture to reach the passage floor during rainfall events. These passage attributes are particularly important to help to minimize passage rejection for amphibians and reptiles, and potentially open-habitat specialist species (e.g., Jackson et al., 2015; Langton and Clevenger, 2017; Gunson and Huijser, 2019). This does not include having the passages used as road drainage systems, as this may result in inundation or increase pollutants that could potentially be harmful to amphibians (White et al., 2023). Also, noise from vehicles is not alleviated with these concepts and deserves consideration and study.
Finally, road barrier fencing is a common problem in the efficacy of passage systems due to the need for frequent maintenance (e.g., Baxter-Gilbert et al., 2015; IENE, 2020; Langton and Clevenger, 2021). In a recent review, 44 studies reported an issue with barriers within 2.2 years after installation due to gaps, washouts, deterioration and movement around, under, or over the fencing (Gunson and Huijser, 2019). There is some evidence solid fencing may be safer and more effective in leading small animals to passages than fencing they can see through (Milburn-Rodríguez et al., 2016; Brehme and Fisher, 2021; Brehme et al., 2022a,Conan et al., 2022); however, installation of solid fencing must be accompanied by consideration of potential effects on hydrological flows during rain events, particularly when rights of way are limited. The ERS designs eliminate or reduce the need for stand-alone barrier fencing along their length. All plans include smooth solid side walls and overhangs along bridge abutments or sides of raised roadbeds to prevent climbing and to keep small animals off the roadway, while barriers for traffic are included to meet safety standards for vehicles, bicyclists, and pedestrians. These designs do not require “jump-outs” to allow animals to escape back into the habitat if they wander onto the road, as do those that have elevated fencing (Langton and Clevenger, 2021). The ERS repeating passage design options for primary roads and highways are similar to systems in the Netherlands and elsewhere where topography, grading or lowered road verges on both sides of the road essentially create an elevated road surface bed, although here the passages are wide, and the footprint is limited to the road itself.
Although these designs may have a higher initial cost, there are other advantages in building elevated road structures in comparison to below grade crossings in some landscapes, including smaller area of impact, less susceptibility to flooding and inundation, and greater suitability in areas with challenging topography (flat lands, hilly, and extreme terrains).
5. Conclusion
Our analysis of the prototype ERS passage in Sierra National Forest, Fresno County, CA, USA, indicates that this wide and open design is permeable to movements of migratory and non-migratory amphibians, reptiles, and small mammals. Overall, amphibian and reptile activity under the ERS did not significantly differ from the forest, while small mammal activity was greater under the ERS than in the forest. Relative use of the ERS was equal to or greater than roadside habitat for all 22 species. Lower activity of some species in comparison to the interior forest can likely be attributed to decreased use of sparsely vegetated habitat within several meters of the road. These results suggest that adding downed logs and vegetation adjacent to the structure may enhance passage for these species.
Current road mitigation practices for small animals have primarily focused on amphibians and turtles, with designs largely comprised of stand-alone barrier fencing and small passages under the roadway. The breadth of scientific knowledge on the efficacy of these mitigation systems is limited but growing. Recent work has shown the negative filtering effect on amphibians if passages are too far apart or if fencing is too long. In general, the literature suggests that as a group, small animals are more likely to use and benefit from wider, more frequent, and more natural passages that offer cover or protection from larger predators. The ERS was designed to meet these needs and can theoretically be built to any length and width.
The ERS and additional concept designs provided are meant to increase the options available for consideration in road mitigation planning for small animals (Supplementary Data Sheets 1, 2), while incorporating the most recent scientific information. These and other possible ERS designs are particularly relevant for species or communities that require permeability across long lengths of roadway to help meet their connectivity needs. Although these designs were largely based on permeability to migratory amphibians, their use for non-migratory amphibians, reptiles and small mammals has been shown. They also offer alternative solutions for road mitigation systems in areas with challenging terrain and other hydrological or environmental constraints. We encourage the continued development and testing of these and other potential road mitigation solutions for small animals.
Data availability statement
The raw data supporting the conclusions of this article will be made available by the authors, without undue reservation.
Ethics statement
Ethical review and approval was not required for this study as passive camera methods were used for animal detection that did not involve capture, handling or marking of live vertebrates.
Author contributions
CB, SB, and JF designed the ERS passage. CB conceived the study with input from SB, MH, and RF. CV, SB, BE, and MH led the field effort for all camera data. BE analyzed all the photos, identified animals to species, and organized the database. PG performed the statistical analysis and produced results, figures, and tables. CB and PG wrote the manuscript. JF produced Supplementary file 1. CT, SH, and HS produced Supplementary file 2. All authors contributed to manuscript revision, read, and approved the submitted version.
Funding
Funding for the ERS and installation was provided by the U.S. Forest Service. Study funding was provided by the California Department of Transportation, Division of Research, Innovation and System Information (DRISI; Agreement 65A0553), Nevada Department of Transportation, Transportation Pooled Fund Program Project in 2022 (P342-20-803), and U.S. Forest Service, and Ecosystems Mission Area of the U.S. Geological Survey.
Acknowledgments
We thank the U.S. Forest Service (USFS) for funding the ERS and for conducting maintenance and camera monitoring in all years of the study. Sterling Walker and Jim Weber from Anthony Hardwood Composites/EMTEK worked with the authors to design the ERS and turn our vision into reality. ERTEC generously donated water-permeable polymer matrix fencing and materials. We thank USFS for assisting in the installation and maintenance of the fencing. It was a group effort including several of the authors and Devin Adsit-Morris, Denise Clark, Tristan Edgarian, Jennifer Kingston, and Chase Stafford (USGS); Wesley Burton, Tony Borelli, Alonso Ruiz, Mindy Mcclurg, and Phil Mazon (USFS); Ed Schiedel (Volunteer USFS). We greatly appreciate the work of Dokken Engineering for the ERS design concepts and guidance to include John Klemunes; and associated input and comments from Tom Langton (Herpetofauna Consultants International Ltd.), Carl Savage, Lindsay Vivian, Luz Quinnel, Robert Young, Robert Blizzard, Sophie Golding, Arnica McCarthy, and Blake Hirara (Caltrans). Finally, we thank the California Department of Transportation, Division of Research, Innovation and System Information (DRISI; Agreement 65A0553) for helping to fund the initial stages of the project in 2018–2019, USFWS, and Hobbs Ecology for funding and support in 2020, and the Nevada Department of Transportation, Transportation Pooled Fund Program Project for funding in 2022 (P342-20-803). Partial funding was provided by Ecosystems Mission Area of the U.S. Geological Survey. This is Contribution Number 874 of the U.S. Geological Survey Amphibian Research and Monitoring Initiative (ARMI). Any use of trade, firm or product names is for descriptive purposes only and does not imply endorsement by the U.S. Government.
Conflict of interest
CT, SH, and HS were paid to engineer concept designs for elevated road segments (see Supplementary material). JF designed (with USGS and USFS input) the elevated road segment installed in the Sierras and was paid for installation and materials. MH helped with the study design and was paid for camera rental.
The remaining authors declare that the research was conducted in the absence of any commercial or financial relationships that could be construed as a potential conflict of interest.
The reviewer VP is currently organizing a Research Topic with CB and RF.
Publisher’s note
All claims expressed in this article are solely those of the authors and do not necessarily represent those of their affiliated organizations, or those of the publisher, the editors and the reviewers. Any product that may be evaluated in this article, or claim that may be made by its manufacturer, is not guaranteed or endorsed by the publisher.
Supplementary material
The Supplementary Material for this article can be found online at: https://www.frontiersin.org/articles/10.3389/fevo.2023.1145322/full#supplementary-material
Footnotes
References
Allaback, M. L., and Laabs, D. M. (2002-2003). Effectiveness of road tunnels for the Santa Cruz long-toed salamander. Trans. West. Sect. Wildl. Soc. 38/39, 5–8. Available online at: https://www.wildlifeprofessional.org/western/transactions/tr2002_2.html
Andrews, K. M., and Gibbons, J. W. (2005). How do highways influence snake movement? Behavioral responses to roads and vehicles. Copeia 2005, 772–782. doi: 10.1643/0045-8511(2005)005[0772:HDHISM]2.0.CO;2
Andrews, K. M., Gibbons, J. W., Jochimsen, D. M., and Mitchell, J. (2008). Ecological effects of roads on amphibians and reptiles: a literature review. Herpetol. Conserv. 3, 121–143.
Andrews, K. M., Nanjappa, P., and Riley, S. P. (2015). Roads and ecological infrastructure: concepts and applications for small animals. Baltimore, MD: John Hopkins University Press. doi: 10.1353/book.39710
Ascensão, F., Mata, C., Malo, J. E., Ruiz-Capillas, P., Silva, C., Silva, A. P., et al. (2016). Disentangle the causes of the road barrier effect in small mammals through genetic patterns. PLoS One 11:e0151500. doi: 10.1371/journal.pone.0151500
Barker, R. J., Schofield, M. R., Link, W. A., and Sauer, J. R. (2018). On the reliability of N-mixture models for count data. Biometrics 74, 369–377. doi: 10.1111/biom.12734
Barrientos, R., Ascensão, F., D’Amico, M., Grilo, C., and Pereira, H. M. (2021). The lost road: do transportation networks imperil wildlife population persistence? Perspect. Ecol. Conserv. 19, 411–416. doi: 10.1016/j.pecon.2021.07.004
Baxter-Gilbert, J. H., Riley, J. L., Lesbarréres, D., and Litzgus, J. D. (2015). Mitigating reptile road mortality: Fence failures compromise ecopassage effectiveness. PLoS One 10:e0120537. doi: 10.1371/journal.pone.0120537
Beebee, T. J. (2013). Effects of road mortality and mitigation measures on amphibian populations. Conserv. Biol. 27, 657–668. doi: 10.1111/cobi.12063
Boyle, S. P., Keevil, M. G., Litzgus, J. D., Tyerman, D., and Lesbarrères, D. (2021). Road-effect mitigation promotes connectivity and reduces mortality at the population-level. Biol. Conserv. 261:109230. doi: 10.1016/j.biocon.2021.109230
Brehme, C. S., and Fisher, R. N. (2021). Research to Inform Caltrans Best Management Practices for Reptile and Amphibian Road Crossings. USGS Cooperator Report 65A0553. San Diego, CA: California Department of Transportation, Division of Research and Innovation. Available online at: https://dot.ca.gov/-/media/dot-media/programs/environmental-analysis/documents/final-caltrans-usgs-report-herproadresearch-rev.pdf
Brehme, C. S., Barnes, S., Ewing, B., Vaughan, C., Hobbs, M., Tornaci, C., et al. (2022a). Research to inform passage spacing for migratory amphibians and to evaluate efficacy and designs for open elevated road passages. USGS cooperator report to Nevada department of transportation, transportation pooled fund program project P342-20-803. Available online at: https://www.dot.nv.gov/home/showpublisheddocument/21592/638161089758230000 (accessed May 02, 2023).
Brehme, C. S., Tracey, J. A., Gould, P. R., Rochester, C. J., and Fisher, R. N. (2022b). Internal structures and ledges facilitate the use of large underpasses by multiple wildlife species and groups. USGS cooperator report to Nevada department of transportation, transportation pooled fund program project P200-20-803. Available online at: https://www.dot.nv.gov/home/showpublisheddocument/21590/638161089738400000 (accessed May 02, 2023).
Brehme, C. S., Hathaway, S. A., and Fisher, R. N. (2018). An objective road risk assessment method for multiple species: ranking 166 reptiles and amphibians in California. Landsc. Ecol. 33, 911–935. doi: 10.1007/s10980-018-0640-1
Brehme, C. S., Tracey, J. A., Ewing, B. A., Hobbs, M. T., Launer, A. E., Matsuda, T. A., et al. (2021). Responses of migratory amphibians to barrier fencing inform the spacing of road underpasses: a case study with California tiger salamanders (Ambystoma californiense) in Stanford, CA, USA. Glob. Ecol. Conserv. 31:e01857. doi: 10.1016/j.gecco.2021.e01857
Brehme, C. S., Tracey, J. A., McClenaghan, L. R., and Fisher, R. N. (2013). Permeability of roads to movement of scrubland lizards and small mammals. Conserv. Biol. 27, 710–720. doi: 10.1111/cobi.12081
Caldwell, M. R., and Klip, J. M. K. (2020). Wildlife interactions within highway underpasses. J. Wildl. Manag. 84, 227–236. doi: 10.1002/jwmg.21801
Ceia-Hasse, A., Navarro, L. M., Borda-de-Água, L., and Pereira, H. M. (2018). Population persistence in landscapes fragmented by roads: disentangling isolation, mortality, and the effect of dispersal. Ecol. Model. 375, 45–53. doi: 10.1016/j.ecolmodel.2018.01.021
Chen, H. L., and Koprowski, J. L. (2019). Can we use body size and road characteristics to anticipate barrier effects of roads in mammals? A meta-analysis. Hystrix 30:1.
Clevenger, A. P., Chruszcz, B., and Gunson, K. (2001). Drainage culverts as habitat linkages and factors affecting passage by mammals. J. Appl. Ecol. 38, 1340–1349. doi: 10.1046/j.0021-8901.2001.00678.x
Conan, A., Fleitz, J., Garnier, L., Le Brishoual, M., Handrich, Y., and Jumeau, J. (2022). Effectiveness of wire netting fences to prevent animal access to road infrastructures: an experimental study on small mammals and amphibians. Nat. Conserv. 47, 271–281. doi: 10.3897/natureconservation.47.71472
Corva, D. M., Semianiw, N. I., Eichholtzer, A. C., Adams, S. D., Mahmud, M. P., Gaur, K., et al. (2022). A smart camera trap for detection of endotherms and ectotherms. Sensors 22:4094. doi: 10.3390/s22114094
D’Amico, M., Périquet, S., Román, J., and Revilla, E. (2016). Road avoidance responses determine the impact of heterogeneous road networks at a regional scale. J. Appl. Ecol. 53, 181–190. doi: 10.1111/1365-2664.12572
D’Amico, M., Román, J., De los Reyes, L., and Revilla, E. (2015). Vertebrate road-kill patterns in Mediterranean habitats: Who, when and where. Biol. Conserv. 191, 234–242. doi: 10.1016/j.biocon.2015.06.010
Dean, W. R. J., Seymour, C. L., Joseph, G. S., and Foord, S. H. (2019). A review of the impacts of roads on wildlife in semi-arid regions. Diversity 11:81. doi: 10.3390/d11050081
Fahrig, L., and Rytwinski, T. (2009). Effects of roads on animal abundance: an empirical review and synthesis. Ecol. Soc. 14:21. doi: 10.5751/ES-02815-140121
Forman, R., Sperling, D., Bissonette, J., Clevenger, A., and Cutshall, C. (2003). Road ecology: science and solutions. Washington, DC: Island Press.
Garrah, E., Danby, R. K., Eberhardt, E., Cunnington, G. M., and Mitchell, S. (2015). Hot spots and hot times: Wildlife road mortality in a regional conservation corridor. Environ. Manage. 56, 874–889. doi: 10.1007/s00267-015-0566-1
Gibbs, J. P., and Shriver, W. G. (2002). Estimating the effects of road mortality on turtle populations. Conserv. Biol. 16, 1647–1652. doi: 10.1046/j.1523-1739.2002.01215.x
Gilbert, N. A., Clare, J. D., Stenglein, J. L., and Zuckerberg, B. (2021). Abundance estimation of unmarked animals based on camera-trap data. Conserv. Biol. 35, 88–100. doi: 10.1111/cobi.13517
Gleeson, T., Petrovan, S., and Muir, A. (2018). The effect of rainfall upon the behaviour and use of under-road culverts in four amphibian species. Biosci. Horiz. Int. J. Student Res. 11:hzz001. doi: 10.1093/biohorizons/hzz001
Glista, D. J., DeVault, T. L., and DeWoody, J. A. (2008). Vertebrate road mortality predominantly impacts amphibians. Herpetol. Conserv. Biol. 3, 77–87.
Goosem, M. (2002). Effects of tropical rainforest roads on small mammals: fragmentation, edge effects and traffic disturbance. Wildl. Res. 29, 277–289. doi: 10.1071/WR01058
Gould, P. R., Gade, M. R., Wilk, A. J., and Peterman, W. E. (2022). Short-term responses of riparian salamander populations to wildfire in the Southern Appalachians. J. Wildl. Manag. 86:e22282. doi: 10.1002/jwmg.22282
Gunson, K. E., and Huijser, M. P. (2019). Road passages and barriers for small terrestrial wildlife: summary and repository of design examples. Project summary report to AASHTO committee on environment and sustainability. National cooperative highway research program (NCHRP project 25-25, task 113). Available online at: http://apps.trb.org/cmsfeed/TRBNetProjectDisplay.asp?ProjectID=4337 (accessed May 02, 2023).
Gunson, K. E., Mountrakis, G., and Quackenbush, L. J. (2011). Spatial wildlife-vehicle collision models: a review of current work and its application to transportation mitigation projects. J. Environ. Manag. 92, 1074–1082. doi: 10.1016/j.jenvman.2010.11.027
Harrison, X. A. (2014). Using observation-level random effects to model overdispersion in count data in ecology and evolution. PeerJ 2:e616. doi: 10.7717/peerj.616
Hedrick, B. P., Vander Linden, A., Cordero, S. A., Watt, E., O’Roark, P. M., Cox, S. L., et al. (2019). Keeping salamanders off the streets: evaluating and modifying one of the first US amphibian road tunnels 30 years after installation. J. Urban Ecol. 5:juz023. doi: 10.1093/jue/juz023
Helldin, J. O., and Petrovan, S. O. (2019). Effectiveness of small road tunnels and fences in reducing amphibian roadkill and barrier effects at retrofitted roads in Sweden. PeerJ 7:e7518. doi: 10.7717/peerj.7518
Hobbs, M. T., and Brehme, C. S. (2017). An improved camera trap for amphibians, reptiles, small mammals, and large invertebrates. PLoS One 12:e0185026. doi: 10.1371/journal.pone.0185026
IENE (2020). Wildlife and traffic: a European handbook for identifying conflicts and designing solutions. In the XXIInd PIARC World Road Congressworld Road Association (PIARC). Available online at: https://handbookwildlifetraffic.info/about-iene/ (accessed January 15, 2023).
Jackson, N. D., and Fahrig, L. (2011). Relative effects of road mortality and decreased connectivity on population genetic diversity. Biol. Conserv. 144, 3143–3148. doi: 10.1016/j.biocon.2011.09.010
Jackson, S. D., Langen, T. A., Marsh, D. M., and Andrews, K. M. (2015). Natural history and physiological characteristics of small animals in relation to roads. Roads and ecological infrastructure. Concepts and applications for small animals. Baltimore, MD: Johns Hopkins University Press, 21–41.
Jaeger, J. A., and Fahrig, L. (2004). Effects of road fencing on population persistence. Conserv. Biol. 18, 1651–1657. doi: 10.1111/j.1523-1739.2004.00304.x
Jameson, E. W., and Peeters, H. J. (2004). Mammals of California (No. 66). Berkeley, CA, University of California Press.
Jarvis, L. E., Hartup, M., and Petrovan, S. O. (2019). Road mitigation using tunnels and fences promotes site connectivity and population expansion for a protected amphibian. Eur. J. Wildl. Res. 65, 1–11. doi: 10.1007/s10344-019-1263-9
Jochimsen, D. M., Peterson, C. R., and Harmon, L. J. (2014). Influence of ecology and landscape on snake road mortality in a sagebrush-steppe ecosystem. Anim. Conserv. 17, 583–592. doi: 10.1111/acv.12125
Kellner, K. (2015). jagsUI: A wrapper around rjags to streamline JAGS analyses. Available online at: https://github.com/kenkellner/jagsUI (accessed March 27, 2023).
Kery, M., Dorazio, R. M., Soldaat, L., Van Strien, A., Zuiderwijk, A., and Royle, J. A. (2009). Trend estimation in populations with imperfect detection. J. Appl. Ecol. 46, 1163–1172. doi: 10.1111/j.1365-2664.2009.01724.x
Langton, T. E. S., and Clevenger, A. P. (2017). Amphibian and reptile highway crossings: state of the practice, gap analysis and decision support tool. Report prepared for the state of California, department of transportation, division of research and innovation, office of materials and infrastructure research, June 2017. Available online at: https://westerntransportationinstitute.org/wp-content/uploads/2021/05/4W5589_Lit_Review_amphib-reptile-crossings_3July2017.pdf (accessed May 02, 2023).
Langton, T. E. S., and Clevenger, A. P. (2021). Measures to reduce road impacts on amphibians and reptiles in California. Best management practices and technical guidance. Prepared by Western Transportation Institute for California Department of Transportation, Division of Research and Innovation and System Information. Available online at: https://dot.ca.gov/-/media/dot-media/programs/research-innovation-system-information/documents/final-reports/ca20-2700-finalreport-a11y.pdf (accessed May 02, 2023).
Laughlin, M. M., Martin, J. G., and Olson, E. R. (2020). Arboreal camera trapping reveals seasonal behaviors of Peromyscus spp. in Pinus strobus canopies. Am. Midl. Nat. 183, 210–222.
Lesbarreres, D., and Fahrig, L. (2012). Measures to reduce population fragmentation by roads: what has worked and how do we know? Trends Ecol. Evol. 27, 374–380. doi: 10.1016/j.tree.2012.01.015
Lesbarrères, D., Lodé, T., and Merilä, J. (2004). What type of amphibian tunnel could reduce road kills? Oryx 38, 220–223. doi: 10.1017/S0030605304000389
Liang, C. T. P. (2010). Habitat modeling and movements of the Yosemite toad (Anaxyrus (= Bufo) canorus) in the Sierra Nevada, California. Davis, CA: University of California.
Martinig, A. R., and Bélanger-Smith, K. (2016). Factors influencing the discovery and use of wildlife passages for small fauna. J. Appl. Ecol. 53, 825–836. doi: 10.1111/1365-2664.12616
Martinig, A. R., Riaz, M., and St Clair, C. C. (2020). Temporal clustering of prey in wildlife passages provides no evidence of a prey-trap. Sci. Rep. 10, 1–9. doi: 10.1038/s41598-020-67340-8
Mata, C., Bencini, R., Chambers, B. K., and Malo, J. E. (2015). “Predator-prey interactions at wildlife crossing structures: between myth and reality,” in Handbook of road ecology, 1st Edn, eds R. van der Ree, D. J. Smith, and C. Grilo (Oxford: Wiley), 190–197. doi: 10.1002/9781118568170.ch23
Matos, C., Petrovan, S. O., Wheeler, P. M., and Ward, A. I. (2019). Short-term movements and behaviour govern the use of road mitigation measures by a protected amphibian. Anim. Conserv. 22, 285–296. doi: 10.1111/acv.12467
Matos, C., Sillero, N., and Argaña, E. (2012). Spatial analysis of amphibian road mortality levels in northern Portugal country roads. Amphibia Reptilia 33, 469–483. doi: 10.1163/15685381-00002850
Matthews, K. R., and Pope, K. L. (1999). A telemetric study of the movement patterns and habitat use of Rana muscosa, the mountain yellow-legged frog, in a high-elevation basin in Kings Canyon National Park, California. J. Herpetol. 33, 615–624. doi: 10.2307/1565578
Mazerolle, M. J., Huot, M., and Gravel, M. (2005). Behavior of amphibians on the road in response to car traffic. Herpetologica 61, 380–388. doi: 10.1655/04-79.1
McDonald, W., and St. Clair, C. C. (2004). Elements that promote highway crossing structure use by small mammals in Banff National Park. J. Appl. Ecol. 41, 82–93. doi: 10.1111/j.1365-2664.2004.00877.x
McGregor, R. L., Bender, D. J., and Fahrig, L. (2008). Do small mammals avoid roads because of the traffic? J. Appl. Ecol. 45, 117–123. doi: 10.1111/j.1365-2664.2007.01403.x
Milburn-Rodríguez, J. C., Hathaway, J., Gunson, K., Moffat, D., Béga, S., and Swensson, D. (2016). Road mortality mitigation: the effectiveness of Animex fencing versus mesh fencing. Available online at: https://www.researchgate.net/publication/323385190_Road_Mortality_Mitigation_The_Effectiveness_of_Animex_Fencing_vs_Mesh_Fencing (accessed May 02, 2023).
Niedballa, J., Sollmann, R., Courtiol, A., and Wilting, A. (2016). camtrapR: An R package for efficient camera trap data management. Methods Ecol. Evol. 7, 1457–1462. doi: 10.1111/2041-210X.12600
Ottburg, F. G., and van der Grift, E. A. (2019). Effectiveness of road mitigation for common toads (Bufo bufo) in the Netherlands. Front. Ecol. Evol. 7:23. doi: 10.3389/fevo.2019.00023
Pagnucco, K. S., Paszkowski, C. A., and Scrimgeour, G. J. (2012). Characterizing movement patterns and spatio-temporal use of under-road tunnels by long-toed salamanders in Waterton Lakes National Park, Canada. Copeia 2012, 331–340. doi: 10.1643/CE-10-128
Plummer, M. (2003). “JAGS: A program for analysis of Bayesian graphical models using Gibbs sampling,” in Proceedings of the third international workshop on distributed statistical computing (DSC 2003) (DSC). V 4.3.0. Available online at: www.r-project.org/conferences/DSC-2003/Proceedings/index.html (accessed March 27, 2023).
R Development Core Team (2013). R: A language and environment for statistical computing. Vienna: R Foundation for Statistical Computing.
Royle, J. A. (2004). N-mixture models for estimating population size from spatially replicated counts. Biometrics 60, 108–115. doi: 10.1111/j.0006-341X.2004.00142.x
Russell, R. E., Swihart, R. K., and Craig, B. A. (2007). The effects of matrix structure on movement decisions of meadow voles (Microtus pennsylvanicus). J. Mammal. 88, 573–579. doi: 10.1644/06-MAMM-A-080R1.1
Rytwinski, T., and Fahrig, L. (2012). Do species life history traits explain population responses to roads? A meta-analysis. Biol. Conserv. 147, 87–98. doi: 10.1016/j.biocon.2011.11.023
Schmidt, B. R., Brenneisen, S., and Zumbach, S. (2020). Evidence-based amphibian conservation: a case study on toad tunnels. Herpetologica 76, 228–239. doi: 10.1655/0018-0831-76.2.228
Schmidt, B. R., Zumbach, S., Mitchell, J. C., Jung Brown, R. E., and Bartolomew, B. (2008). Amphibian road mortality and how to prevent it: a review. Herpetol. Conserv. 3, 157–167.
Semlitsch, R. D. (2008). Differentiating migration and dispersal processes for pond-breeding amphibians. J. Wildl. Manag. 72, 260–267. doi: 10.2193/2007-082
Si, X., Kays, R., and Ding, P. (2014). How long is enough to detect terrestrial animals? Estimating the minimum trapping effort on camera traps. PeerJ 2:e374. doi: 10.7717/peerj.374
Sinsch, U. (2006). Orientation and navigation in Amphibia. Mar. Freshw. Behav. Physiol. 39, 65–71. doi: 10.1080/10236240600562794
Southwood, A., and Avens, L. (2010). Physiological, behavioral, and ecological aspects of migration in reptiles. J. Comp. Physiol. B 180, 1–23. doi: 10.1007/s00360-009-0415-8
Stebbins, R. C., and McGinnis, S. M. (2012). Field guide to amphibians and reptiles of California: revised edition, Vol. 103. Berkeley, CA: University of California Press.
Stewart, L., Russell, B., Zelig, E., Patel, G., and Whitney, K. S. (2020). Wildlife crossing design influences effectiveness for small and large mammals in Banff National Park. Case Stud. Environ. 4:1231752. doi: 10.1525/cse.2020.1231752
Van Buskirk, J. (2012). Permeability of the landscape matrix between amphibian breeding sites. Ecol. Evol. 2, 3160–3167. doi: 10.1002/ece3.424
van der Ree, R., Smith, D. J., and Grilo, C. (2015). Handbook of road ecology. West Sussex: John Wiley and Sons. doi: 10.1002/9781118568170
Vaughan, C., Barnes, S., Brehme, C. S., Liang, C. T., and Brown, C. (2023). Spatial analysis of Yosemite toad road mortality in Sierra National Forest, California.
Vilella, M., Ferrandiz-Rovira, M., and Sayol, F. (2020). Coexistence of predators in time: effects of season and prey availability on species activity within a Mediterranean carnivore guild. Ecol. Evol. 10, 11408–11422. doi: 10.1002/ece3.6778
White, K. J., Petrovan, S. O., and Mayes, W. M. (2023). Pollutant accumulation in road mitigation tunnels for amphibians: a multisite comparison on an ignored but important issue. Front. Ecol. Evol. 11:1133253. doi: 10.3389/fevo.2023.1133253
Keywords: road ecology, road crossing, passage, herpetofauna, design, tunnel, toad, salamanders
Citation: Brehme CS, Barnes S, Ewing B, Gould P, Vaughan C, Hobbs M, Tornaci C, Holm S, Sheldon H, Fiutak J and Fisher RN (2023) Elevated road segment (ERS) passage design may provide enhanced connectivity for amphibians, reptiles, and small mammals. Front. Ecol. Evol. 11:1145322. doi: 10.3389/fevo.2023.1145322
Received: 16 January 2023; Accepted: 17 April 2023;
Published: 24 May 2023.
Edited by:
Arnaldo Marín, University of Murcia, SpainReviewed by:
Viorel Dan Popescu, Ohio University, United StatesCristina Mata, Autonomous University of Madrid, Spain
Claudia Garcia-Gonzalez, Instituto de Investigación Sanitaria del Principado de Asturias (ISPA), Spain
Copyright © 2023 Brehme, Barnes, Ewing, Gould, Vaughan, Hobbs, Tornaci, Holm, Sheldon, Fiutak and Fisher. This is an open-access article distributed under the terms of the Creative Commons Attribution License (CC BY). The use, distribution or reproduction in other forums is permitted, provided the original author(s) and the copyright owner(s) are credited and that the original publication in this journal is cited, in accordance with accepted academic practice. No use, distribution or reproduction is permitted which does not comply with these terms.
*Correspondence: Cheryl S. Brehme, cbrehme@usgs.gov
†These authors have contributed equally to this work