- 1University of Belgrade - Faculty of Biology, Belgrade, Serbia
- 2Institute for Biological Research “Siniša Stanković” - National Institute of the Republic of Serbia, University of Belgrade, Belgrade, Serbia
- 3University of Belgrade - Faculty of Chemistry, Belgrade, Serbia
- 4University of Niš - Faculty of Sciences and Mathematics, Niš, Serbia
- 5Geographical Institute “Jovan Cvijić”, Serbian Academy of Sciences and Arts, Belgrade, Serbia
- 6University of Belgrade - Faculty of Geography, Belgrade, Serbia
In the present study, we investigated in vitro the antimicrobial activity of the pygidial gland secretion of the guanophilic ground beetle Laemostenus (Pristonychus) punctatus (Dejean, 1828) and some of its chemicals against resistant and non-resistant bacteria and Candida species, the synergistic and additive potential of combinations of selected chemicals and antimicrobial drugs against resistant bacterial and fungal strains, anti-adhesive and anti-invasive potential of the secretion and formic acid alone and in selected combinations with antimicrobial drugs against methicillin-resistant Staphylococcus aureus (MRSA) toward spontaneously immortalized human keratinocyte cell line (HaCaT cells). In addition, we examined the antiproliferative activity of the secretion and formic acid in vitro. The tested secretion and the standards of formic and oleic acids possessed a significant level of antimicrobial potential against all tested strains (P < 0.05). The isolate from guano Pseudomonas monteilii showed the highest resistance to the secretion and formic acid, while MRSA achieved a significantly high level of susceptibility to all agents tested, particularly to the combinations of formic acid and antibiotics, but at the same time showed a certain level of resistance to the antibiotics tested individually. Candida albicans and C. tropicalis were found to be the most sensitive fungal strains to the secretion. Formic acid (MIC 0.0005 mg/mL) and gentamicin (MIC 0.0010 mg/mL) in the mixture achieved synergistic antibacterial activity against MRSA (FICI = 0.5, P < 0.05). The combination of formic acid, gentamicin and ampicillin accomplished an additive effect against this resistant bacterial strain (FICI = 1.5, P < 0.05). The secretion achieved a better inhibitory effect on the adhesion ability of MRSA toward HaCaT cells compared to formic acid alone, while formic acid showed better results regarding the invasion (P < 0.001). The combinations of gentamicin and ampicillin, as well as of formic acid and gentamicin and ampicillin achieved similar anti-adhesive and anti-invasive effects, with a slight advantage of formic acid and antibiotics in combination (P < 0.001). The secretion and formic acid were found to be non-toxic to HaCaT cells in vitro (IC50 ≥ 401 μg/mL).
1. Introduction
Natural products and their synthetic analogs contributed significantly to pharmacotherapy in the past, particularly in the treatment of infectious diseases (Atanasov et al., 2021; Zhao et al., 2022). Insects and insect-derived products have been used in traditional medicine for centuries in various regions of the world (Costa-Neto, 2005). Studies published in recent years emphasize the importance of insects and their potential application in biomedicine (Guo et al., 2017; Seabrooks and Hu, 2017; Bairagi, 2019; Nenadić, 2019). Beetles (Coleoptera) nowadays represent the source of biologically active chemicals (Adamski et al., 2019). Many of them, including quinones, glycosides, alkaloids, sugars, polyols, polypeptides, and terpenoids (Costa-Neto, 2005), have been recently identified and shown to have various properties, such as antimicrobial, anti-inflammatory, antiseptic, antioxidant, antitumor, etc. (Cherniack, 2010; Mandal and Mandal, 2011; Seabrooks and Hu, 2017). Combining these compounds with conventional drugs could undoubtedly offer significant advantages for future treatments (Cherniack, 2010). The search for more effective therapies is currently a top priority, particularly because of drug resistance (Murray et al., 2022). Bacterial infections caused by Staphylococcus aureus, Streptococcus pneumoniae, Escherichia coli, and Pseudomonas aeruginosa are a major cause of morbidity and mortality in the world (O’Neill, 2014; Asadi et al., 2019). The annual number of deaths from antibiotic resistance may reach approximately 10 million by 2050 (Cars et al., 2021; Laurence et al., 2021; Walsh et al., 2021). Methicillin-resistant Staphylococcus aureus (MRSA) strains are thought to be responsible for more than 100,000 fatal cases related to antimicrobial resistance in 2019 (Murray et al., 2022). MRSA strains are specific due to their multi-drug resistance and as such can be the main cause of a number of serious diseases of the skin, soft tissues, bones, and bloodstream, but also could be the cause of serious treatment problems among hospitalized patients (Gordon and Lowy, 2008; Jiang et al., 2021). Although antibiotics are available to treat nosocomial infections, the increasing emergence of antibiotic resistance has rendered many treatments ineffective (Walsh et al., 2021). It has been established that staphylococcal strains are resistant to glycopeptide antibiotics, which are used in the ultimate fight against MRSA strains. In this sense, a new therapeutic approach in recent years refers to the synergistic action of existing antibiotics and natural products (Álvarez-Martínez et al., 2020). In addition, anti-adhesion-based therapies are important to reduce the interaction between pathogens and host tissues by preventing or precluding bacterial adherence and entry into cells, which may result in invasion, directly leading to the development of infection and disease (Madle et al., 2018; Roberts et al., 2018). A number of fungal infections have also continued to increase over the past 20 years (Wiederhold, 2017; Denning, 2022). Some strains of Candida glabrata, C. krusei, and the recently emerged species C. auris show decreased susceptibility to commonly used antifungal agents (Gow et al., 2022). Increased azole resistance among Candida spp. isolates (excluding C. albicans) and echinocandin resistance in C. glabrata are some of the recent trends in the development of fungal resistance (Wiederhold, 2017). These problems have led scientists to focus on the study of oral, anal, and exocrine gland secretions in insects, as well as related secondary metabolites with potential antimicrobial properties (Giglio et al., 2011). It is generally known that ground beetles (Carabidae), which represent the largest family within the suborder Adephaga (order Coleoptera), possess a pair of abdominal glands known as pygidial (Forsyth, 1972; Eisner et al., 2000; Giglio et al., 2011, 2021; Nenadić et al., 2016a,2017b; Nenadić, 2019). Recently published papers indicate the importance of the pygidial gland secretions of some Calosoma and Carabus species, which may contain various organic compounds that possess antimicrobial properties, such as short-chain fatty acids (butyric, isobutyric, formic, acetic, etc.) and aromatic aldehydes (salicylaldehyde) (Giglio et al., 2011; Nenadić et al., 2016a; Vesović et al., 2020). These secretions have demonstrated antimicrobial activity against a wide range of human pathogens, as well as an interesting negligible level of toxicity in several species (Giglio et al., 2011; Nenadić et al., 2016a,2017a,2017b,2018; Nenadić, 2019; Vesović et al., 2020, 2022, 2023). The function of these glands is defense against predators (Giglio et al., 2011), but an additional role is observed in freshwater-dwelling Adephaga (Hydradephaga) (Francke and Dettner, 2005), whose pygidial gland secretions exhibit antimicrobial properties that are very important for their aquatic respiration, allowing inhibition of the growth of microorganisms and preventing contamination and wetting of the cuticular respiratory structures (Kovac and Maschwitz, 1990). The other example is the pupa of ground beetle Carabus (Chaetocarabus) intricatus lefebvrei Dejean, 1826, which produces monoterpenes in its pygidial gland secretion that may presumably have a prophylactic function against environmental pathogens (Giglio et al., 2009).
Laemostenus (Pristonychus) punctatus (Dejean, 1828) (Coleoptera: Carabidae) represents a predominantly troglophilic and guanophilic ground beetle species, which is widely distributed in the Balkan Peninsula (Casale, 1988). Individuals of this species could be found in burrows of mammals and caves (Casale, 1988), where they usually come into contact with bat guano, which is a significant source of various microorganisms (Nenadić et al., 2017a; Dimkić et al., 2020). Different organic compounds have been detected in the pygidial gland secretion mixture of L. (P.) punctatus (Vesović et al., 2015; Nenadić et al., 2016a). Undecane (with a relative percentage of 40.4%) and dodecyl acetate (with a relative percentage of 34.2%) (Table 1) are the most abundant components of this secretion (Vesović et al., 2015), but there is no clear evidence of their antimicrobial properties. On the contrary, the correlation between some organic constituents, such as various short- and long-chain fatty acids, and their biological activity has been established in the past (Glassman, 1948; Raftari et al., 2009; Desbois and Smith, 2010).
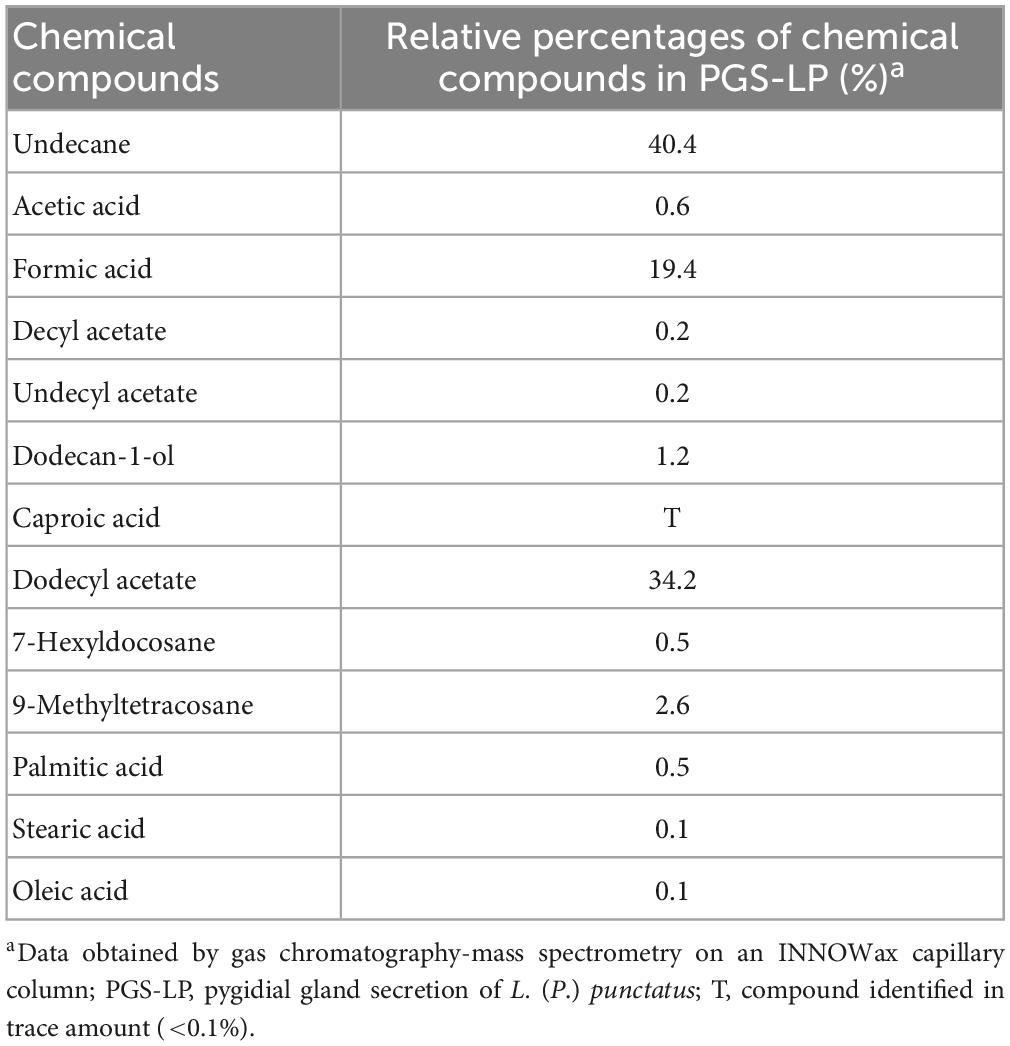
Table 1. Chemical composition of the pygidial gland secretion of the ground beetle L. (P.) punctatus (after Vesović et al., 2015).
In this study, we assume that, apart from the dominant components, only minor chemical constituents that have at least a hint of antimicrobial properties can contribute to the antimicrobial potential of the tested secretion mixture. The effects of minor constituents, such as dodecan-1-ol, decyl acetate, undecyl acetate, caproic acid, 7-hexylcocosane, and 9-methyltetracosane (Table 1), were not investigated as there is no evidence in the literature of their possible antimicrobial properties. However, this is not the case with acetic acid (Table 1), which antimicrobial potential has been demonstrated in the past (Ryssel et al., 2009; Fraise et al., 2013), or oleic acid, which antimicrobial properties have been proved several times in recent decades, particularly when it comes to inhibiting the growth of gram-positive bacteria, such as S. aureus (Speert et al., 1979; Dilika et al., 2000; Stenz et al., 2008; Desbois and Smith, 2010). Palmitic and stearic acids also exhibit antibacterial activity against both gram-positive and gram-negative bacteria (Casillas-Vargas et al., 2021). The production of formic acid as a defensive chemical is widespread in different ground beetles (Francke and Dettner, 2005; Lečić et al., 2014; Nenadić et al., 2016b). It is well-known that formic acid has been added to poultry diets to limit Salmonella spp. and other foodborne pathogens in the feed and potentially in the gastrointestinal tract (Gómez-García et al., 2019; Ricke et al., 2020).
Nenadić et al. (2016b) gave the very first data on the antimicrobial potential of the pygidial gland secretion of L. (P.) punctatus against various human pathogens (clinical and laboratory gram-negative and gram-positive bacterial strains and fungal species), while Dimkić et al. (2020) analyzed the antimicrobial activity of this secretion against guano-dwelling bacterial and fungal strains. Prior to these studies, taxa within the ground beetle subfamily Platyninae were generally poorly known in this regard.
In the current study, we presented the first data on the antimicrobial activity of the pygidial gland secretion of L. (P.) punctatus against resistant bacterial strains and Candida species, as well as on its cytotoxicity and inhibitory effect on the adhesion and invasion ability of MRSA toward spontaneously immortalized human keratinocyte cell line (HaCaT cells). Therefore, the objectives of this study were to investigate: (i) the antimicrobial activity of the pygidial gland secretion of L. (P.) punctatus and selected secretion’s chemicals against resistant and non-resistant strains of various bacterial (laboratory, clinical, and guano-dwelling) and Candida species; (ii) the type of interaction between selected secretion’s chemicals and antimicrobial drugs in combinations; (iii) the antimicrobial potential of these combinations against all resistant strains tested using a two-dimensional (2D) and a three-dimensional (3D) checkerboard method; (iv) in vitro inhibitory effect of the secretion and formic acid alone and in combination with antimicrobial drugs on the adhesion and invasion ability of MRSA toward HaCaT cells; and (v) the antiproliferative activity of the secretion and formic acid toward HaCaT cells to test the cytotoxicity in vitro.
2. Materials and methods
2.1. Collection and handling of specimens of L. (P.) punctatus under laboratory conditions
A total of 79 adult specimens of L. (P.) punctatus were manually collected by S. Ćurčić, N. Vesović, V. Žikić, and S. Stanković on 27th September 2020 in the Ogorelička Pećina Cave, village of Sićevo, Svrljiške Planine Mts., southeastern Serbia (average environmental conditions recorded: temperature 13°C, aerial humidity 70.5%). After collection, the live beetles were transported and preserved in cold and portable plastic chamber for a few days before sampling the contents of their pygidial glands according to a previously established protocol (Nenadić et al., 2016a). Preservation of beetles was performed with the aim of reducing the stressful state of insects to the minimum under controlled laboratory conditions (the moist soil obtained from the collection sites, complete darkness, a constant temperature of 10°C, and feeding on earthworms and springtails collected from the Ogorelička Pećina Cave). After isolation and quantitative analysis of the pygidial gland secretion, the dried beetle specimens were deposited in the collection of the Institute of Zoology, University of Belgrade - Faculty of Biology, Belgrade, Serbia.
2.2. In vitro antimicrobial assay
2.2.1. Isolation procedure and quantitative assessment of biological material
Pygidial gland secretion was isolated and quantitatively analyzed under sterile conditions at room temperature according to a previously established protocol (Nenadić et al., 2016a). Analysis was performed immediately after sampling to reduce the impact of composition-altering oxidation, possible evaporation and degradation of the chemical constituents of the secretion. The total amount of the pygidial gland secretion from 79 specimens of L. (P.) punctatus was obtained by stimulating their legs and pressing the tip of their abdomen to discharge pygidial gland secretion into a 2 mL glass vial. The secretion was measured on a Sartorius Model 2405 analytical balance (Sartorius Group, Göttingen, Germany) for weighing up to 30 g maximum, with 0.001 mg resolution in the laboratory of the Department of Organic Chemistry, University of Belgrade - Faculty of Chemistry, Belgrade, Serbia. The secretion mass was measured as described in Nenadić et al. (2016a).
To check the validity of the results, all measurements were performed three times.
2.2.2. Preparation of biological material for in vitro antimicrobial susceptibility testing
The extracted biological sample was prepared by dissolving it in 0.01 M phosphate buffer solution (PBS) (Sigma-Aldrich, Munich, Germany) under sterile conditions at room temperature and then stored in a freezer at −20°C in the laboratory of the Institute of Zoology, University of Belgrade - Faculty of Biology, Belgrade, Serbia until the antimicrobial effect is tested.
2.2.3. Antimicrobial drugs
The following synthetic commercial antibiotics were used as positive controls in this study: streptomycin (1 mg of active substance in 1 mL of physiological solution) (Sigma-Aldrich, Munich, Germany), ampicillin (1 mg of active substance in 1 mL of physiological solution) (Panfarma, Belgrade, Serbia), gentamicin (500 mg of amoxicillin trihydrate in 500 mL of saline) (Panfarma, Belgrade, Serbia), and cephalexin (1 mg of active substance in 1 mL of physiological solution) (Panfarma, Belgrade, Serbia).
The synthetic commercial antifungal drugs used as positive controls in this study were amphotericin B (1 mg of active substance in 1 mL of physiological solution) (Sigma-Aldrich, Munich, Germany) and nystatin (1 mg of active substance in 1 mL of physiological solution) (Sigma-Aldrich, Munich, Germany).
2.2.4. Commercial standards tested
The following commercial standards were tested in this study: acetic acid (ethanoic acid, IUPAC) 99.8% (Sigma-Aldrich, St. Louis, MO, USA) (1 mg of active substance in 1 mL of 0.01 M PBS), dodecyl acetate (lauryl acetate, IUPAC) 97% (Sigma-Aldrich, St. Louis, MO, USA) (1 mg of active substance in 1 mL of 0.01 M PBS), formic acid (methanoic acid, IUPAC) 95% (Tokyo Chemical Industry Co., Ltd., Tokyo, Japan) (1 mg of active substance in 1 mL of 0.01 M PBS), oleic acid [(9Z)-octadec-9-enoic acid, IUPAC] 99% (Tokyo Chemical Industry Co., Ltd., Tokyo, Japan) (1 mg of active substance in 1 mL of 0.01 M PBS), palmitic acid (hexadecanoic acid, IUPAC) 95% (Tokyo Chemical Industry Co., Ltd., Tokyo, Japan) (1 mg of active substance in 1 mL of 0.01 M PBS), stearic acid (octadecanoic acid, IUPAC) 98% (Tokyo Chemical Industry Co., Ltd., Tokyo, Japan) (1 mg of active substance in 1 mL of 0.01 M PBS), and undecane (hendecane, IUPAC) 99.5% (Tokyo Chemical Industry Co., Ltd., Tokyo, Japan) (1 mg of active substance in 1 mL of 0.01 M PBS).
2.2.5. Bacterial and fungal strains tested
In total 16 microbial pathogens (12 bacterial and four fungal strains), including resistant and non-resistant strains, have been tested in this study. The human pathogens used in this research were: Escherichia coli ATCC 25922, E. coli resistant strain IBRS E003, Pseudomonas aeruginosa ATCC 27853, P. aeruginosa resistant strain IBRS P001 (gram-negative bacteria), Staphylococcus aureus ATCC 11632, methicillin-resistant S. aureus (MRSA) IBRS 011 (clinical isolate) (gram-positive bacteria), Candida albicans ATCC 10231, C. krusei H1/16 (clinical isolate), C. tropicalis ATCC 750, and C. auris CDC B11903 (fungal strains). They are deposited in the Mycological Laboratory, Department of Plant Physiology, Institute for Biological Research “Siniša Stanković” - National Institute of the Republic of Serbia, University of Belgrade, Belgrade, Serbia. In addition, we tested the following bacterial strains obtained from bat guano: Bacillus subtilis GT1, B. clausii GT2, B. toyonensis GT3, Peribacillus simplex GT4, Pseudomonas monteilii GT5, and Staphylococcus arlettae GT6. These were previously identified and deposited in the laboratory of the Department of Biochemistry and Molecular Biology, University of Belgrade - Faculty of Biology, Belgrade, Serbia.
2.2.6. Preparation of the overnight cultures of bacterial and Candida species
All tested strains of bacterial and Candida species, previously stocked in 80% glycerol at −80°C, were seeded on 2 mL Tryptic Soy Broth (TSB) growth medium (15 g of casein, 5 g of peptone, 5 g of NaCl, 15 g of agar, 1 L of K2HPO4, and 1,000 mL of distilled water) (Biolife Italiana, Milan, Italy). Overnight cultures with 1.0 × 109 colony-forming units (CFU)/mL were established after incubation for 24 h at 37°C in a thermostat. The final solution of culture and medium (1.0 × 108 CFU/mL) was prepared by dissolving 100 mL of primary culture in 900 mL of TSB and stored in a freezer at −20°C until use in the antimicrobial assay. The final concentration tested per well of a 96-well microtiter plate was 1.0 × 106 CFU/mL.
2.2.7. In vitro broth microdilution
The in vitro antimicrobial assay was performed using the broth microdilution method (Hänel and Raether, 1988; Daouk et al., 1995; CLSI, 2009; Soković et al., 2010; Nenadić et al., 2016a,2017b) to determine the minimum inhibitory concentrations (MICs), minimum bactericidal concentrations (MBCs), and minimum fungicidal concentrations (MFCs) of the tested agents. The method was carried out using a 96-well system, i.e., 96-well sterile microtiter plates with a flat bottom (F-microtiter plate with a cover) (Spektar, Čačak, Serbia), and through 2-fold serial dilutions of the tested samples dissolved in 0.01 M PBS (pygidial gland secretion and the commercial standards of selected chemicals) or physiological solution (antimicrobial drugs). Each well of the first row of microtiter plates had a total volume of 200 μL (180 μL of added tested agents and 20 μL of TSB), whereas the sterility control was inoculated only with 100 μL of TSB. Finally, each well was inoculated with 10 μL of bacterial/fungal culture (1.0 × 108 CFU/mL). After performing 2-fold serial dilutions, the suspensions were adjusted to a final volume of 100 μL. The microtiter plates were incubated at 37°C for 24 h.
The antibiotics streptomycin, ampicillin, cephalexin, and gentamicin, as well as the antimycotics nystatin and amphotericin B were used as positive controls in final tested concentration ranges of 0.001–0.900 mg/mL (antibiotics) and 0.028–0.450 mg/mL (antimycotics), respectively, while 0.01 M PBS was used as a negative control. The final tested concentration of the secretion of L. (P.) punctatus was in a range of 0.004–0.112 mg/mL for 12 bacterial strains, and 0.024–0.096 mg/mL for four strains of Candida species, respectively. The final tested concentration range of the selected chemicals tested was 0.001–0.600 mg/mL for 12 bacterial strains, and 0.008–0.600 mg/mL for four strains of Candida species, respectively.
2.2.8. Determination of MICs, MBCs, and MFCs
Minimum inhibitory concentrations (MICs) are defined as the lowest concentrations that cause inhibition of bacterial or fungal growth, which can be observed under a binocular microscope (Rodríguez-Tudela et al., 2003), while MBCs and MFCs represent the lowest concentrations with no bacterial or fungal growth observed, indicating that 99.5% of the original inoculum has been killed (Soković et al., 2010).
Minimum inhibitory concentration values were determined by using a colorimetric microbial viability assay based on the reduction in color of p-iodonitrotetrazolium violet, 2-(4-iodophenyl)-3-(4-nitrophenyl)-5-phenyl-2H-tetrazolium chloride (INT) (Sigma-Aldrich, Munich, Germany) (0.2 mg of the substance in 1 mL of double distilled water). Colonies were stained by adding 40 μL of INT and then incubated at 37°C for 2 h.
Pale yellow color pointed to inhibition and unstained microtiter plate chambers indicated that observed tested agent achieved bactericidal effect (CLSI, 2009). A microplate reader (Tecan Austria GmbH, Grödig, Austria; Eppendorf AG, Hamburg, Germany) was used to assess the intensity of yellow color and MICs were determined by counting cell numbers using spectrophotometric methods (Nenadić et al., 2016a,2017b). The optical density of each well was measured at a wavelength of 655 nm and compared to a blank (broth medium and diluted agent) and a positive control. In addition, MBCs and MFCs were confirmed by reinoculating of 2 μL of the tested dilutions with bacterial or fungal inoculum from each well without color change into 100 μL of TSB and incubating at 37°C for 24 h, after which no growth was detected. MIC, MBC, and MFC values were obtained as mg/100 μL and finally expressed in mg/mL.
2.2.9. In vitro 2D and 3D checkerboard assay
To determine the interactions (synergism, additive effect, indifference or antagonism) between selected agents (formic and oleic acids, the antibiotics streptomycin, ampicillin, and gentamicin, and the antifungal agents amphotericin B and nystatin) in combinations and the antimicrobial potential of the tested combinations in vitro, selected resistant bacterial strains (E. coli, P. aeruginosa, and MRSA) and the resistant C. auris strain were studied using a 2D and a 3D checkerboard method. For this assay, we used 96-well microtiter plates containing TSB growth medium with the tested acids and antimicrobial agents combined in a checkerboard style at concentrations ranging from 1/8 MIC to 2 × MIC (0.0005–1 mg/mL). The microtiter plates were incubated at 37°C for 24 h (Jacqueline et al., 2005). To evaluate the effect of three selected components (formic acid, ampicillin, and gentamicin), the 3D checkerboard method according to Stein et al. (2015) was performed using 96-well microtiter plates containing TSB medium with the tested compounds. The bacterial and fungal inoculum per well was 1.0 × 106 CFU/mL.
Fractional Inhibitory Concentration Index (FICI) was determined according to the following formula:
where FIC10 and FIC20 are the MIC values of the tested compounds and antibiotics in combination, while MIC10 and MIC20 represent the MIC values of the individual activity of selected agents. For the interpretation of the obtained results, the following threshold values according to Lorian (2005) were used: FICI ≤ 0.5 = synergistic effect, 2 ≥ FICI > 0.5 = additive effect, 4 ≥ FICI > 2 = indifferent effect, and FICI > 4 = antagonistic effect.
2.2.10. In vitro assay for evaluation of the adhesion and invasion ability of MRSA toward HaCaT cells
Percentages of adhesion and invasion were determined as described by Ahmed et al. (2014), with some modifications. HaCaT cells were prepared and grown in 24-well plates with an adhesive bottom. Afterward, the medium was removed and fresh Dulbecco’s Modified Eagle Medium (DMEM) without fetal bovine serum (FBS) (Invitrogen, Waltham, MA, USA) containing the tested agents in concentrations of the determined MIC and MBC values was added to the cells. The first incubation was then carried out for 15 min at 37°C, after which 100 μL of MRSA culture (1.0 × 108 CFU/mL) was added to the cells and incubated again with the cells for 1 h at 37°C for testing adhesion capacity, and 2 h at 37°C for testing invasion capacity, respectively. To kill the adhering and free-floating bacterial cells, gentamicin at a concentration of 300 μg/mL was added to the cells for 1 h. Finally, the cells were washed three times with DMEM without FBS and lyzed for 30 min at 37°C with 1 mL of 1% (v/v) Tween 20 (Sigma-Aldrich, Munich, Germany).
For the adhesion assay, the previously prepared sample was diluted 100 times. For the invasion test, the prepared sample was not diluted. A total of 10 μL of both samples were inoculated and spread on tryptone soy agar (TSA) growth medium (Torlak, Belgrade, Serbia) using a sterile stick. After 18 h of incubation at 37°C, the number of CFU was determined. The final results of this assay were expressed as percentages (%). Gentamicin and ampicillin in combination were used as positive controls, while untreated cultures were used as negative controls (100% of adhesion/invasion).
This assay was performed in triplicate and three independent experiments were repeated.
2.2.11. In vitro crystal violet cytotoxicity assay
In vitro analysis of cytotoxicity of the tested secretion of L. (P.) punctatus, commercial standards and their combinations with antibiotics was performed on HaCaT cells using a modified crystal violet assay as described by Stojković et al. (2020).
HaCaT cells were grown in high-glucose DMEM supplemented with 10% FBS, 2 mM L-glutamine and 1% antibiotic/antimycotic (Invitrogen, Waltham, MA, USA) in 5% CO2 at 37°C. Cells were prepared and incubated in each well of 96-well microtiter plates with an adhesive bottom 48 h prior to treatment. After the initial incubation, the clear growth medium was removed, and a new medium containing dissolved tested agents at various concentrations (up to a final concentration of 8 mg/mL) was added to the cells, which were treated for 24 h. Afterward, the medium was removed and the cells were washed twice with PBS. In addition, the cells were stained with 0.4% crystal violet staining solution at room temperature for 20 min. The crystal violet staining solution was then removed, the cells were washed in a stream of tap water and air-dried at room temperature. The absorbance of dye dissolved in methanol was measured at 570 nm (OD 570) using a microplate reader (Tecan Austria GmbH, Grödig, Austria; Eppendorf AG, Hamburg, Germany).
The results were expressed as IC50 values, indicating 50% inhibition of proliferation of HaCaT cells compared to the untreated positive control. The criterion for determining and classifying the antiproliferative activity of the tested agents and combinations toward HaCaT cells was as follows: IC50 ≤ 20 μg/mL = highly cytotoxic effect, IC50 21–200 μg/mL = moderately cytotoxic effect, IC50 201–400 μg/mL = weakly cytotoxic effect, and IC50 ≥ 401 μg/mL = no cytotoxicity observed (Stojković et al., 2020).
For each sample, concentration procedures were carried out in triplicate and three independent experiments were performed.
2.2.12. Statistical analysis of the results
One-way analysis of variance (ANOVA) was used to interpret the results, which were presented as mean values (MEAN) and standard errors (SE), followed by Tukey’s HSD test with a significance level of 0.05. Statgraphics Centurion software XVI, version 16.2 was used, and graphs were generated using GraphPad Prism, version 8.0.
3. Results
3.1. Total amount of discharged pygidial gland secretion
The total amount of the discharged pygidial gland secretion obtained from 79 adult individuals of L. (P.) punctatus was 0.395 ± 0.001 mg, which was used for further investigations in this study. The average amount of the secretion per one adult specimen of the analyzed species was 0.005 ± 0.001 mg.
3.2. In vitro screening of antibacterial activity
The initial analysis indicated that all tested bacterial strains showed a high susceptibility to undecane, dodecyl acetate, and acetic acid (MIC values were not obtained due to the bactericidal effect of undecane, dodecyl acetate, and acetic acid even at the lowest concentration tested) and simultaneously a high resistance to palmitic and stearic acids (MIC values were not detected due to the observed growth of bacterial cultures even at the highest concentration tested), so these chemicals were excluded from further examinations.
The pygidial gland secretion of L. (P.) punctatus and the standards of two secretion’s compounds (formic and oleic acids), tested in comparison with commercial antibiotics, were found to have a certain antibacterial potential that differed between all resistant and non-resistant strains (P < 0.05). Resistant bacterial strains generally showed lower susceptibility to all tested agents compared to non-resistant bacteria. The beta-lactam antibiotics tested (ampicillin and cephalexin), the aminoglycoside streptomycin, and the standard of oleic acid showed a lower antibacterial potential compared to the secretion of L. (P.) punctatus and formic acid (Table 2).
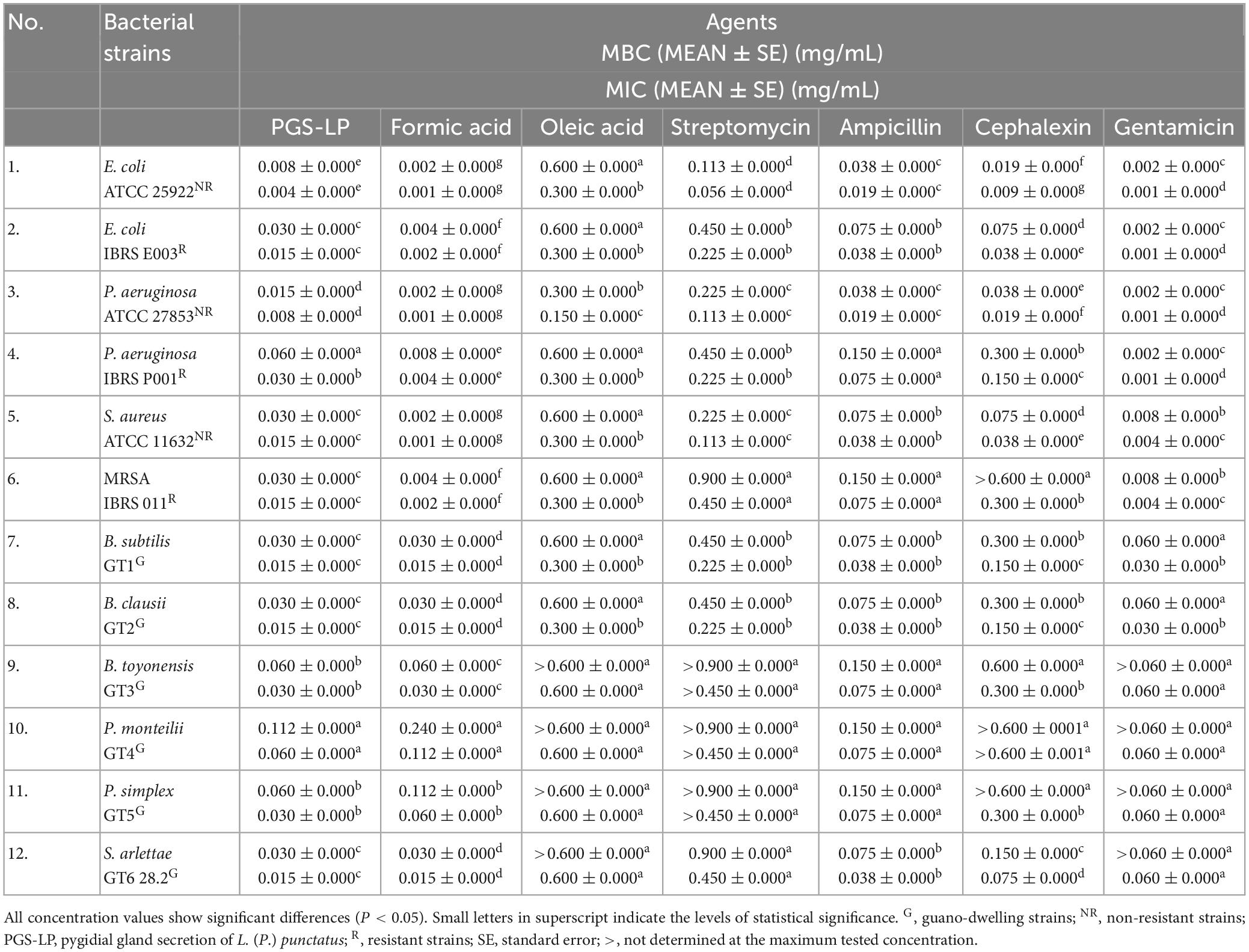
Table 2. In vitro screening of antibacterial activity displayed as mean values (MEAN) of the minimum bactericidal concentrations (MBCs) and minimum inhibitory concentrations (MICs) of the pygidial gland secretion of L. (P.) punctatus and the standards of formic and oleic acids alone compared to positive controls (antibiotics streptomycin, ampicillin, cephalexin, and gentamicin).
With respect to the tested standards of organic constituents contained in the secretion mixture of L. (P.) punctatus, formic acid achieved much higher antibacterial potential against all tested bacteria (MIC 0.001–0.112 mg/mL) compared to oleic acid (MIC 0.150–0.600 mg/mL) (Table 2).
Formic acid was found to have antimicrobial activity equivalent to the aminoglycoside gentamicin, with MIC values 2–15 times lower than the MICs of the secretion of L. (P.) punctatus, except for the strain from guano P. monteilii, which showed the highest resistance to formic acid (MIC 0.112 ± 0.000 mg/mL, MBC 0.240 ± 0.000 mg/mL) and the secretion of L. (P.) punctatus (MIC 0.060 ± 0.000 mg/mL, MBC 0.112 ± 0.000 mg/mL).
Regarding resistance to gentamicin and formic acid, S. aureus and MRSA were found to be more resistant to gentamicin, whereas the resistant E. coli and P. aeruginosa showed a higher resistance to formic acid in relation to the non-resistant E. coli and P. aeruginosa that displayed equal susceptibility. Gentamicin and formic acid accomplished the highest bactericidal potential against resistant strains, thus both were tested in combination in further steps of this study.
Escherichia coli and MRSA were found to be the most sensitive to the secretion of L. (P.) punctatus among the resistant strains (MIC 0.015 ± 0.000 mg/mL, MBC 0.030 ± 0.000 mg/mL), while the non-resistant E. coli showed the highest susceptibility to the secretion (MIC 0.004 ± 0.000 mg/mL, MBC 0.008 ± 0.000 mg/mL) compared to all tested bacteria (Table 2).
The resistant P. aeruginosa strain was found to be less sensitive to the secretion of L. (P.) punctatus (MIC 0.030 ± 0.000 mg/mL, MBC 0.060 ± 0.000 mg/mL) compared to the other strains tested.
All guano-dwelling bacterial strains showed a similar susceptibility to the secretion of L. (P.) punctatus, with a MIC range of 0.015–0.060 mg/mL (Table 2). With respect to the tested secretion, B. subtilis, B. clausii, and S. arlettae proved to be the most sensitive strains (with MIC 0.015 ± 0.000 mg/mL and MBC 0.030 ± 0.000 mg/mL, respectively), while the remaining three guano-dwelling strains tested (B. toyonensis, P. monteilii, and P. simplex) showed a higher level of resistance.
The resistant E. coli and MRSA showed a similar susceptibility to the secretion of L. (P.) punctatus, approximately the same as the guano-dwelling B. subtilis, B. clausii, and S. arlettae strains (with MIC 0.015 ± 0.000 mg/mL and MBC 0.030 ± 0.000 mg/mL, respectively) that were found to be less resistant to the secretion compared to other isolates from guano tested.
Formic acid was found to achieve a stronger antibacterial activity against most clinical and laboratory strains compared to the secretion of L. (P.) punctatus, but the secretion was more effective against two guano-dwelling strains — P. monteilii (MIC 0.060 ± 0.000 mg/mL) and P. simplex (MIC 0.030 ± 0.000 mg/mL). The other guano-dwelling strains tested showed the same susceptibility to formic acid.
In contrast to all resistant strains, MRSA showed a higher susceptibility to the secretion of L. (P.) punctatus (MIC 0.015 ± 0.000 mg/mL) and formic acid (MIC 0.002 ± 0.000 mg/mL), but exhibited a higher resistance to all positive controls. Among all positive controls tested, gentamicin and ampicillin achieved the highest antimicrobial potential against MRSA. Based on these facts, this bacterial strain was tested in further steps of this research.
3.3. In vitro screening of antifungal activity
The initial analysis indicated that all tested strains of Candida species showed a high susceptibility to undecane, dodecyl acetate, and acetic acid (MIC values were not obtained due to the fungicidal effect of undecane, dodecyl acetate, and acetic acid even at the lowest concentration tested) and simultaneously a high resistance to palmitic and stearic acids (MIC values were not detected due to the observed growth of fungal cultures even at the highest concentration tested), thus these chemicals were excluded from further examinations.
The tested pygidial gland secretion of L. (P.) punctatus and the standards of formic and oleic acids achieved a certain antifungal potential against all tested Candida species, including the resistant C. auris and non-resistant strains, compared to the commercial antimycotics nystatin and amphotericin B (P < 0.05) (Table 3).
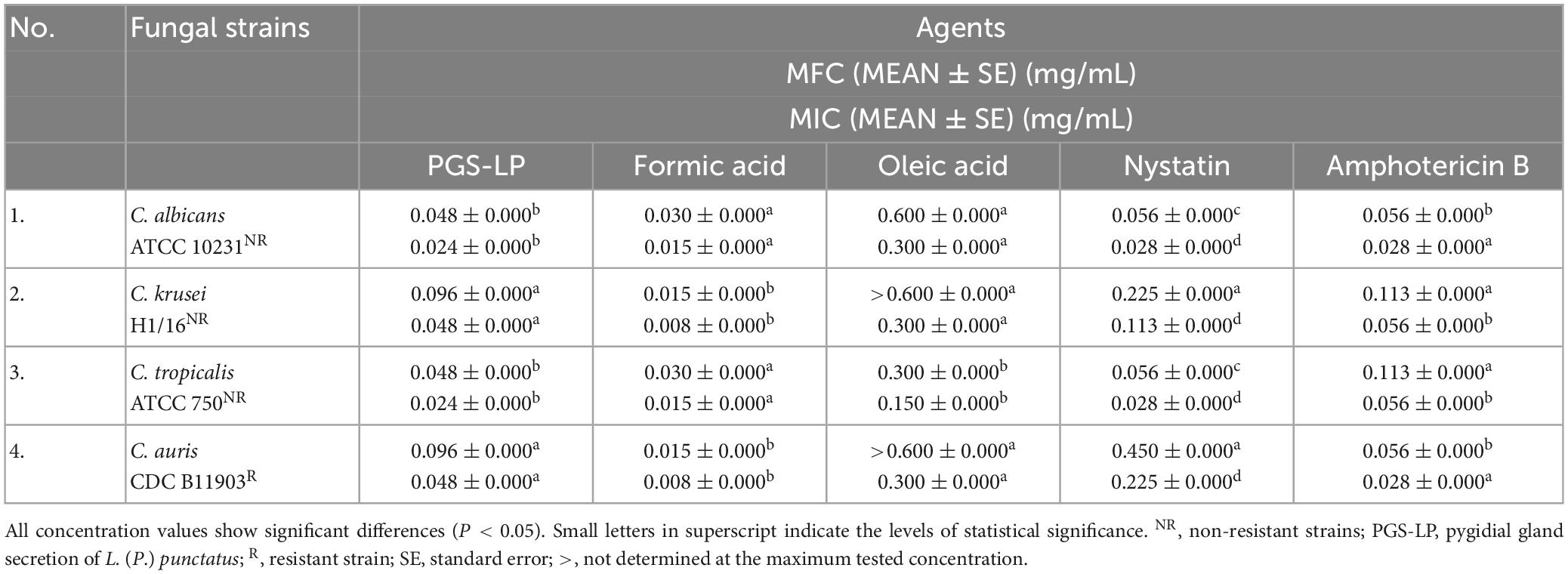
Table 3. In vitro screening of antifungal activity displayed as mean values (MEAN) of the minimum fungicidal concentrations (MFCs) and minimum inhibitory concentrations (MICs) of the pygidial gland secretion of L. (P.) punctatus and the standards of formic and oleic acids alone compared to positive controls (antimycotics nystatin and amphotericin B).
Candida albicans and C. tropicalis were found to be more sensitive to the secretion of L. (P.) punctatus (with MIC 0.024 ± 0.000 mg/mL and MFC 0.048 ± 0.000 mg/mL, respectively) compared to the clinical isolate C. krusei and the resistant C. auris strain (with MIC 0.048 ± 0.000 mg/mL and MFC 0.096 ± 0.000 mg/mL, respectively). Candida krusei and the resistant C. auris showed a higher susceptibility to formic acid (with MIC 0.008 ± 0.000 mg/mL and MFC 0.015 ± 0.000 mg/mL, respectively) (Table 3).
Oleic acid achieved a significantly weaker antifungal activity compared to all tested agents, with MIC value in a range of 0.150–0.300 mg/mL.
When comparing the positive controls, amphotericin B proved to have a better antifungal activity (MIC 0.028–0.056 mg/mL) than nystatin (MIC 0.028–0.225 mg/mL).
With respect to all Candida strains tested, the secretion of L. (P.) punctatus and formic acid showed higher antifungal potential compared to positive controls.
3.4. In vitro screening of antimicrobial activity of selected agents tested in combinations using a 2D and a 3D checkerboard assay
An in vitro checkerboard method showed that among the selected combinations against resistant laboratory and clinical strains tested only formic acid (MIC 0.0005 ± 0.0000 mg/mL) in combination with gentamicin (MIC 0.0010 ± 0.0000 mg/mL) achieved a synergistic antibacterial effect against MRSA (FICI = 0.5, P < 0.05) (Figure 1 and Table 4), whereas the other combinations tested achieved an additive effect (P < 0.05), with the exception of the combination of formic and oleic acids that showed indifference to all tested bacteria and C. auris, as well as the combination of formic acid and gentamicin that demonstrated indifference to the resistant P. aeruginosa strain (Figure 1). Based on this fact, only formic acid in combination with selected antimicrobial drugs was further tested using a 2D and a 3D checkerboard method.
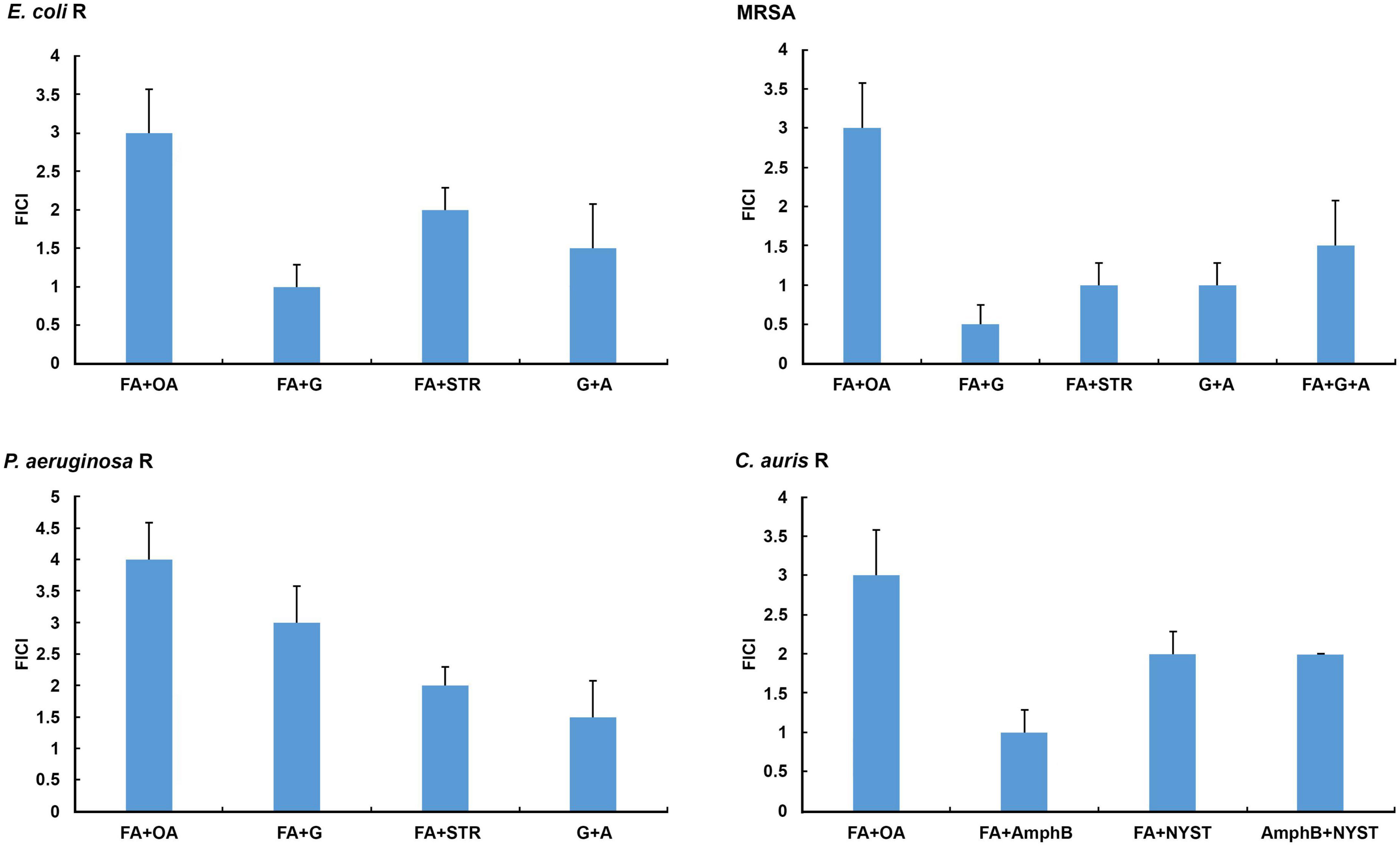
Figure 1. In vitro determination of interactions of selected secretion’s compounds and antibiotics/antimycotics tested in combinations against the resistant E. coli, methicillin-resistant Staphylococcus aureus (MRSA), P. aeruginosa, and C. auris. A, ampicillin; AmphB, amphotericin B; FA, formic acid; G, gentamicin; NYST, nystatin; OA, oleic acid; R, resistant strains; STR, streptomycin. FICI ≤ 0.5 = synergistic effect, 2 ≥ FICI > 0.5 = additive effect, 4 ≥ FICI > 2 = indifferent effect, FICI > 4 = antagonistic effect. All Fractional Inhibitory Concentration Index (FICI) values show significant differences (P < 0.05).
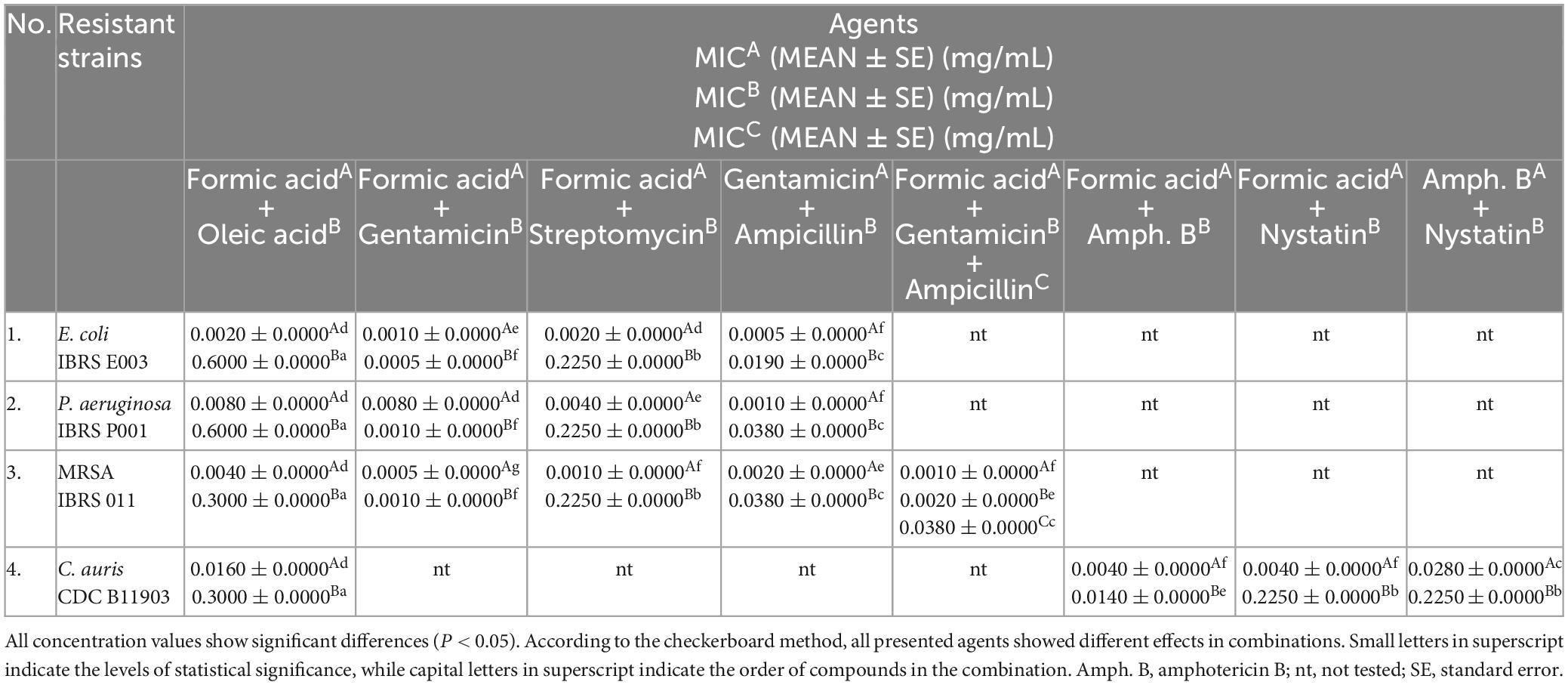
Table 4. In vitro screening of the antimicrobial activity of the tested combinations against resistant strains displayed as mean values (MEAN) of the minimum inhibitory concentrations (MICs) of the mixtures.
The results of the in vitro 3D checkerboard method showed that the combination of formic acid, gentamicin, and ampicillin expresses an additive effect (FICI = 1.5, P < 0.05) (Figure 1) against the MRSA strain, with the following MIC values in the tested combination, respectively: 0.0010 ± 0.0000 mg/mL (formic acid), 0.0020 ± 0.0000 mg/mL (gentamicin), and 0.0380 ± 0.0000 mg/mL (ampicillin) (Table 4).
All tested bacteria showed higher resistance to oleic acid in combinations, while formic acid and gentamicin in combination showed stronger antimicrobial activity against the tested strains (Table 4).
Candida auris showed the highest susceptibility to the combination of formic acid and amphotericin B (Figure 1 and Table 4).
3.5. In vitro screening of anti-adhesive, anti-invasive, and cytotoxic effect toward HaCaT cells
In vitro antimicrobial screening indicated that MRSA showed a significantly high level of susceptibility to all tested agents compared to other resistant strains, especially to the secretion of L. (P.) punctatus (MIC 0.015 ± 0.000 mg/mL, MBC 0.030 ± 0.000 mg/mL, P < 0.05), formic acid (MIC 0.002 ± 0.000 mg/mL, MBC 0.004 ± 0.000 mg/mL, P < 0.05) and, in particular, the combinations of formic acid and antibiotics acting additively and synergistically, but at the same time showed a certain level of resistance to the antibiotics tested individually. In accordance with the preceding facts, this strain was used to analyze the effects of the selected agents tested (secretion, formic acid, formic acid in combination with gentamicin, and ampicillin) on the adhesion and invasion ability of MRSA toward HaCaT cells. Likewise, the relative proliferation rate of HaCaT cells after treatment with different concentrations of the secretion of L. (P.) punctatus and formic acid (MIC 0.025-0.400 mg/mL) was observed to exhibit the level of cytotoxicity. All tested agents alone and in combination achieved a significant level of anti-adhesive and anti-invasive effect compared to the negative control (100% of adhesion and invasion) (P < 0.001) (Figures 2A, B). The secretion of L. (P.) punctatus with a MIC of 0.015 ± 0.001 mg/mL caused a significant decrease in the adhesion rate of MRSA toward HaCaT cells to 44.075 ± 6.585%, which was higher than the one of formic acid (adhesion rate 59.471 ± 0.290%) (P < 0.001) (Figure 2A). The combination of formic acid, gentamicin, and ampicillin proved to have the highest level of anti-adhesive effect compared to the negative control and the other tested agents by reducing the adhesion rate of MRSA toward HaCaT cells to 1.623 ± 0.491% (P < 0.001), including gentamicin and ampicillin in combination (adhesion rate 2.366 ± 0.409%), but with no significant difference (Figure 2A). In contrast, the secretion of L. (P.) punctatus showed a significantly lower inhibitory effect against MRSA, with the invasion rate of 56.189 ± 6.676%, compared to the rate of formic acid of 42.105 ± 10.255% (P < 0.001) (Figure 2B). Likewise, the combination of formic acid, gentamicin, and ampicillin achieved a similar effect in an invasion assay (invasion rate 11.011 ± 1.266%) as did gentamicin and ampicillin in combination (11.444 ± 0.987%), with no significant difference. The combination of formic acid, gentamicin, and ampicillin significantly reduced adhesion and invasion compared to the secretion of L. (P.) punctatus and formic acid alone (P < 0.001). An in vitro crystal violet assay showed that the secretion and formic acid had no antiproliferative activity against HaCaT cells (IC50 values were ≥ 0.401 mg/mL for all samples tested) (Figures 2C, D).
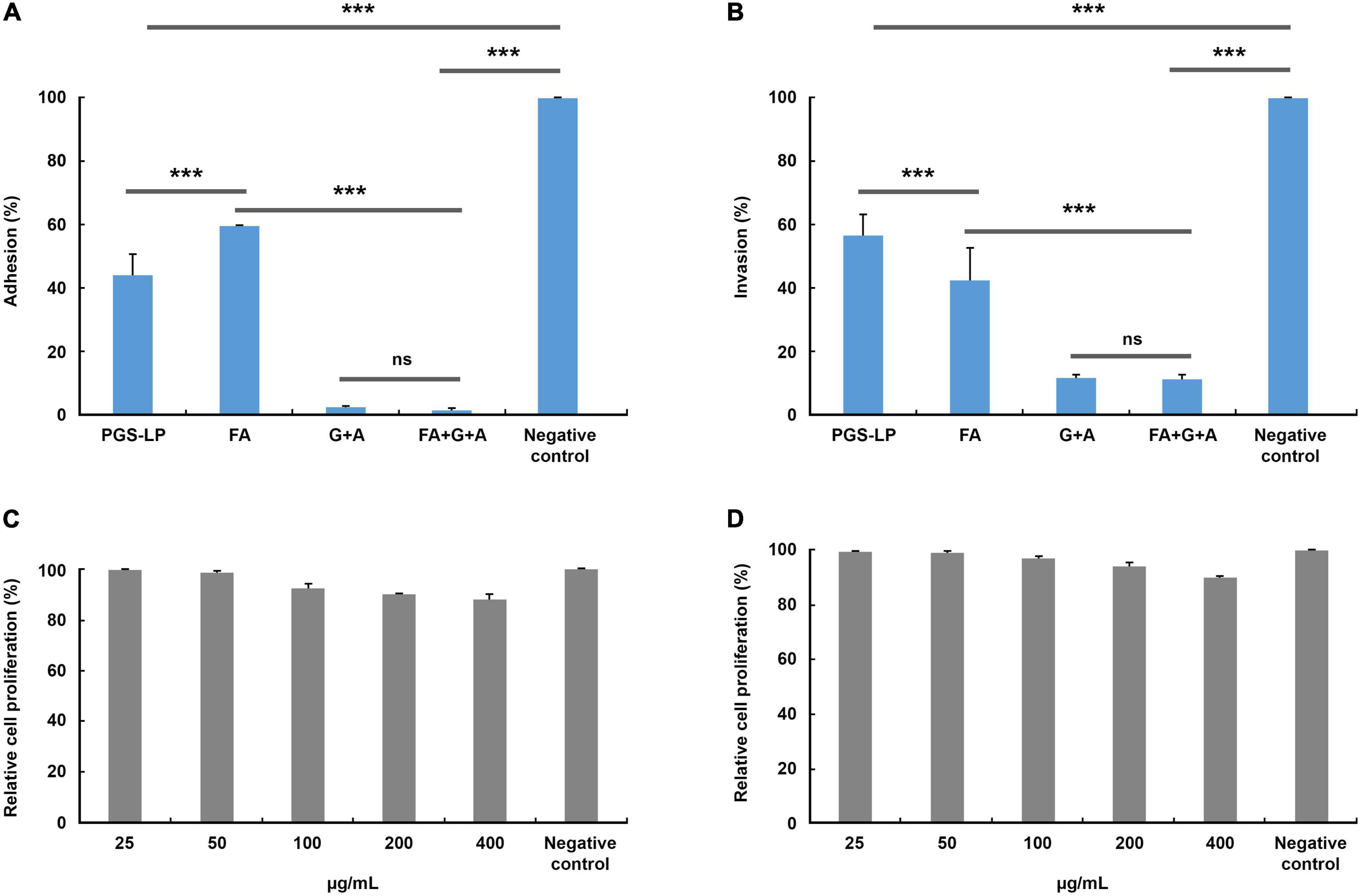
Figure 2. Adhesion (A) and invasion (B) of methicillin-resistant Staphylococcus aureus (MRSA) toward spontaneously immortalized human keratinocyte cell line (HaCaT cells) (%) after treatment with the minimum inhibitory concentrations (MICs) of selected tested agents compared to the negative control (no treatment, 100% of adhesion/invasion) and relative rate of in vitro proliferation of HaCaT cells (%) after treatment with formic acid (C) and the pygidial gland secretion of L. (P.) punctatus (D), where all concentration values show no significant differences compared to the negative control (P > 0.05). A, ampicillin (0.038 mg/mL in combinations G + A and FA + G + A); FA, formic acid (0.002 mg/mL; 0.0005 mg/mL in combination FA + G; 0.001 mg/mL in combination FA + G + A); G, gentamicin (0.001 mg/mL in combination FA + G; 0.002 mg/mL in combinations G + A and FA + G + A); ns, not significant; PGS-LP, pygidial gland secretion of L. (P.) punctatus (0.015 mg/mL); ***P < 0.001.
4. Discussion
In recent years, the number of publications focused on the importance of insect products in biomedicine is increasing (Ratcliffe et al., 2014; Seabrooks and Hu, 2017; Nenadić, 2019). These studies have revealed that among organic compounds, salicylaldehyde, short-chain fatty acids, such as formic, butyric, and isobutyric, and long-chain fatty acids could be associated with the antimicrobial activity of insect products (Glassman, 1948; Raftari et al., 2009; Desbois and Smith, 2010; Nenadić et al., 2016a,2017b; Nenadić, 2019). One of the latest in vitro studies indicates that the defensive gland secretion of Luprops tristis Fabricius, 1801 (Coleoptera: Tenebrionidae) could be a very effective antimicrobial agent against S. aureus and E. coli as it contains oleic acid and specific compounds, such as 2,3-dimethyl-1,4-benzoquinone, 1,3-dihydroxy-2-methylbenzene, 2,5-dimethylhydroquinone, tetracosane, hexacosane, pentacosane, 7-hexadecenal, and tert-hexadecanethiol (Sabira et al., 2022).
The antimicrobial activity of the secretions of representatives of the ground beetle subfamily Platyninae was initially investigated by Nenadić et al. (2016b), who presented the first in vitro antimicrobial screening of the pygidial gland secretion of L. (P.) punctatus against various human microbial pathogens, including clinical and laboratory strains. Although undecane and dodecyl acetate were found in its secretion mixture in the highest percentage (Vesović et al., 2015), their possible antimicrobial properties have not been reported in the literature. Likewise, all tested bacterial and fungal strains showed high susceptibility to undecane and dodecyl acetate in the current study. Hydrocarbons, such as undecane, are thought to act as penetration facilitators of active compounds through the lipophilic cuticle of predators (Blum, 1981). Despite the fact that formic acid was present in a smaller percentage (19.4%) in this secretion than undecane and dodecyl acetate (Vesović et al., 2015), there is evidence of the antimicrobial properties of this compound (Gómez-García et al., 2019; Luise et al., 2020; Peh et al., 2020). Oleic acid, which was represented in this secretion mixture with 0.1% (Vesović et al., 2015), proved to have certain antimicrobial properties in this study, so it could probably give an additional contribution to the antimicrobial activity of this secretion (Vesović et al., 2015; Nenadić, 2019).
In previous studies, it was assumed that the antimicrobial activity of the secretion of L. (P.) punctatus is probably caused by the interaction of several chemical components acting synergistically (Nenadić et al., 2016b; Nenadić, 2019), but in this study we found that the selected fatty acids from the secretion were indifferent in combination as formic acid achieved much stronger antimicrobial activity compared to oleic acid.
In this study, the beta-lactam antibiotics ampicillin and cephalexin and the aminoglycoside streptomycin were found to have weaker antibacterial activity than the tested secretion and formic acid. In contrast, Nenadić et al. (2016b) noted that the commercial antibiotics streptomycin and ampicillin and the antifungal agents ketoconazole and bifonazole generally exhibited higher antimicrobial potential against all tested bacterial and fungal strains, with the exception of Penicillium ochrochloron, which was found to be more resistant to ketoconazole and more sensitive to the pygidial gland secretion of L. (P.) punctatus (MIC 1.1 mg/mL, MBC 2.2 mg/mL). Interestingly, the secretion displayed a higher antibacterial potential against the non-resistant E. coli, P. aeruginosa, and S. aureus strains in the current study, with MIC values about 100 times lower.
Nenadić et al. (2016b) showed that the non-resistant E. coli ATCC 35210 proved to be the most sensitive bacterium to the secretion of L. (P.) punctatus, while in the current study the non-resistant E. coli ATCC 25922 strain showed the highest sensitivity to this secretion compared to all tested bacteria. On the other hand, in this study, the resistant E. coli strain and MRSA showed higher sensitivity compared to all resistant strains tested. Regarding antifungal activity, the secretion and formic acid showed higher antifungal potential in the present study compared to the positive controls amphotericin B and nystatin.
Nenadić et al. (2017a) reported the first evidence of the antimicrobial properties of the same secretion against the cave-dwelling micromycetes Talaromyces duclauxii, Aspergillus brunneouniseriatus, Penicillium sp., Rhizopus stolonifer, and Trichoderma viride. The most sensitive fungal isolate proved to be Penicillium sp. (MIC 0.500 ± 0.029 mg/mL), while the other isolates displayed a high level of resistance to the secretion. According to Dimkić et al. (2020), the most sensitive guano-dwelling fungal strains to this secretion were Meyerozyma guilliermondii, Penicillium expansum, and Trichoderma harzianum (each with MIC 0.150–0.300 mg/mL). These studies indicate that the defensive strategy of L. (P.) punctatus, primarily aiming to protect the beetle from predators, can also be associated with defense against microorganisms from the same habitat (Nenadić et al., 2017a; Nenadić, 2019; Dimkić et al., 2020).
According to Dimkić et al. (2020), the pygidial gland secretion of L. (P.) punctatus proved to have certain antibacterial properties against 12 tested guano-dwelling bacterial isolates. Likewise, Dimkić et al. (2020) revealed that gram-positive bacterial isolates generally showed a higher level of sensitivity to this secretion compared to gram-negative strains. This is confirmed in the current study as the gram-negative bacterium P. monteilii proved to be the most resistant guano-dwelling strain to the secretion compared to other tested gram-positive guano-dwelling isolates. Escherichia fergusonii showed a pronounced sensitivity to the secretion, almost twice as high compared to the tested antibiotic streptomycin. The most sensitive isolate proved to be Enterococcus eurekensis, with the lowest recorded MIC value of 0.007 mg/mL (Dimkić et al., 2020), while the guano-dwelling strains with the highest susceptibility in the current study were B. subtilis, B. clausii, and S. arlettae, each with a MIC value of 0.015 mg/mL. Interestingly, in this study, B. subtilis, B. clausii, B. toyonensis, and S. arlettae showed the same level of susceptibility to the secretion and the standard of formic acid, with simultaneous resistance to the antibiotics streptomycin, cephalexin, ampicillin, and gentamicin, while oleic acid achieved inhibitory effect at the highest concentration tested, with 20 times higher MIC compared to the secretion and formic acid alone. The resistant P. aeruginosa strain was the least susceptible bacterium to the secretion, together with P. monteilii. As guano-dwelling bacteria showed a significant level of sensitivity to the secretion of L. (P.) punctatus and formic acid as an important antibacterial constituent of this mixture (Nenadić, 2019) (P < 0.05), the cause of proved antimicrobial, anti-adhesive, and anti-invasive potential of this secretion and formic acid against clinical and laboratory strains probably lies in a possible defensive strategy against potential microbial pathogens from the same habitat, with which these insects frequently come into contact (Nenadić, 2019; Dimkić et al., 2020). It is worth mentioning that the bacterial community of guano may contain pathogens responsible for various diseases important in human medicine (representatives of the genera Bacillus, Pseudomonas, Staphylococcus, Escherichia, Enterococcus, etc.), which further confirms the significance of the relevant studies (Banskar et al., 2016; Dimkić et al., 2020). This means that the antimicrobial properties of the tested secretion, apart from its ecological importance, could also have medical significance in the future.
Methicillin-resistant Staphylococcus aureus undeniably represents a significant strain due to its resistance to many antibiotics, such as methicillin, penicillin, oxacillin, cloxacillin, cefazolin, cefoxitin, etc. (Larsen et al., 2022; Nandhini et al., 2022). For this reason, but also due to its demonstrated increased susceptibility to the natural product (secretion) and formic acid compared to other resistant bacteria, this strain was the focus of this study. Regarding the antimicrobial properties of insect products against MRSA, literature data mainly refer to the effect of antimicrobial proteins. Li et al. (2017) showed that insect defensins DLP2 and DLP4 have higher antimicrobial potential, better efficiency, faster action, and longer post-antibiotic effect compared to the antibiotic vancomycin. A novel strategy against resistant microorganisms is a synergistic or combination therapy, particularly when it involves the combinations of existing commercial medications and natural products (Pancu et al., 2021). Regarding the antimicrobial activity of commercial antimicrobials in combination with natural products against MRSA, there is evidence of higher efficacy of gentamicin in combination with plant extracts. According to Blesson et al. (2015), the synergistic antibacterial activity of gentamicin and an aqueous extract of Colocasia esculenta (L.) Schott, a widely used traditional medicinal plant, may be a promising future therapy in the fight against MRSA infections. Similarly, the combination of formic acid and monolaurin may reduce intestinal inflammation caused by enterotoxigenic E. coli in piglets by inhibiting their NF-κB/MAPK pathways (Ren et al., 2020). In this study, we found that the combination of formic acid, gentamicin, and ampicillin showed an additive effect against MRSA strain. Simultaneously, the same combination significantly reduced the adhesion rate of MRSA toward HaCaT cells. Nenadić et al. (2018) investigated the antitumor and antiproliferative potential of the pygidial gland secretion of L. (P.) punctatus. Although this secretion showed certain level of inhibition of porcine liver primary cell proliferation (14.72 ± 0.86%) (Nenadić et al., 2018), it wasn’t found to be antiproliferative toward HaCaT cells in this study, which was also the case with formic acid (IC50 ≥ 401 μg/mL). Bioactive natural products in combination with commercial antibiotics and antifungal drugs could represent an alternative source of antimicrobial agents. In recent years, a new therapeutic approach based on the synergistic effect of existing antibiotics and natural products has been mainly explored. On the other hand, anti-adhesion-based therapies are promising in reducing the interaction between pathogens and host tissues, which could lead to the prevention of any possibility of infection and disease development (Ofek et al., 2003; Jiang et al., 2012; Asadi et al., 2019). The current study suggests that the secretions of ground beetles and other insects, presumably with the aim of protecting themselves against pathogens from the same habitat (Giglio et al., 2011; Nenadić, 2019; Dimkić et al., 2020), have unpredictably become a source of potential therapeutics, which is very important for the fight against the current global antimicrobial resistance. Likewise, this study indicates that the future of treating bacterial and fungal infections lies in the use of anti-adhesive therapeutics. Since current scientific research on the potential therapeutic functions of insects suggests that these organisms may become an important source of novel drugs (Feng et al., 2009; Nenadić, 2019), the present study may inspire the development of new synthetic analogs with potential medical application in combined therapies in the future. Further research will aim to better understand the biological activities of antimicrobial agents from the pygidial gland secretions of ground beetles in vitro and in vivo.
Data availability statement
The original contributions presented in this study are included in the article/supplementary material, further inquiries can be directed to the corresponding author.
Author contributions
MN: study design, conceptualization, software - statistical analysis, processing of figures, writing—original draft preparation, and visualization. MN, MS, AĆ, DS, ID, and SĆ: methodology - adaptation. SĆ, NV, and SSS: fieldwork investigation and collection of insect samples. LV and MT: quantitative analysis. MN and AĆ: methodology - in vitro screening of antimicrobial activity. MN and DS: methodology - in vitro assay for evaluation of anti-adhesive and anti-invasive potential of tested agents and in vitro cytotoxicity assay. ID and TJ: preparation of cultures of guano-dwelling bacterial strains. NV: software - figures editing. SĆ, AĆ, DS, MS, ID, SSS, and MN: writing—review and editing. SĆ: supervision. NBĆ, UM, DP, MM, and SĆ: project administration. MM: funding acquisition. All authors contributed to the article and approved the submitted version.
Funding
This study was supported by the Serbian Ministry of Science, Technological Development and Innovation (contracts nos. 451-03-47/2023-01/200178, 451-03-47/2023-01/200168, 451-03-47/2023-01/200007, 451-03-47/2023-01/200124, and 451-03-47/2023-01/200172).
Acknowledgments
We thank Vladimir Žikić (University of Niš - Faculty of Sciences and Mathematics, Niš, Serbia) for collecting some insect samples, as well as Marija Ivanov (Institute for Biological Research “Siniša Stanković” - National Institute of the Republic of Serbia, University of Belgrade, Belgrade, Serbia) and Ana Janjić (Belgrade, Serbia) for partial contributing to the in vitro assay aiming to evaluate the anti-adhesive and anti-invasive effects of the tested agents.
Conflict of interest
The authors declare that the research was conducted in the absence of any commercial or financial relationships that could be construed as a potential conflict of interest.
Publisher’s note
All claims expressed in this article are solely those of the authors and do not necessarily represent those of their affiliated organizations, or those of the publisher, the editors and the reviewers. Any product that may be evaluated in this article, or claim that may be made by its manufacturer, is not guaranteed or endorsed by the publisher.
References
Adamski, Z., Bufo, S. A., Chowański, S., Falabella, P., Lubawy, J., Marciniak, P., et al. (2019). Beetles as model organisms in physiological, biomedical and environmental studies – A review. Front. Physiol. 10:319. doi: 10.3389/fphys.2019.00319
Ahmed, G. F., Elkhatib, W. F., and Noreddin, A. M. (2014). Inhibition of Pseudomonas aeruginosa PAO1 adhesion to and invasion of A549 lung epithelial cells by natural extracts. J. Infect. Public Health 7, 436–444. doi: 10.1016/j.jiph.2014.01.009
Álvarez-Martínez, F. J., Barrajón-Catalán, E., and Micol, V. (2020). Tackling antibiotic resistance with compounds of natural origin: A comprehensive review. Biomedicines 8:405. doi: 10.3390/biomedicines8100405
Asadi, A., Razavi, S., Talebi, M., and Gholami, M. (2019). A review on anti-adhesion therapies of bacterial diseases. Infection 47, 13–23. doi: 10.1007/s15010-018-12225
Atanasov, A. G., Zotchev, S. B., and Dirsch, V. M. (2021). Natural products in drug discovery: Advances and opportunities. Nat. Rev. Drug Discov. 20, 200–216. doi: 10.1038/s41573-020-00114-z
Bairagi, S. H. (2019). Insects with potential medicinal significance: A review. Biomed. J. Sci. Technol. Res. 16, 12024–12027. doi: 10.26717/BJSTR.2019.16.002849
Banskar, S., Bhute, S. S., Suryavanshi, M. V., Punekar, S., and Shouche, Y. S. (2016). Microbiome analysis reveals the abundance of bacterial pathogens in Rousettus leschenaultii guano. Sci. Rep. 6:36948. doi: 10.1038/srep36948
Blesson, J., Saji, C. V., Nivya, R. M., and Kumar, R. (2015). Synergistic antibacterial activity of natural plant extracts and antibiotics against methicillin resistant Staphylococcus aureus (MRSA). J. Sci. Technol. Res. 4, 741–763.
Blum, M. S. (1981). Chemical defenses of arthropods. New York, NY: Academic Press. doi: 10.1016/B978-0-12-108380-9.X5001-7
Cars, O., Chandy, S. J., Mpundu, M., Peralta, A. Q., Zorzet, A., and So, A. D. (2021). Resetting the agenda for antibiotic resistance through a health systems perspective. Lancet Glob. Health 9, 1022–1027. doi: 10.1016/S2214-109X(21)00163-7
Casale, A. (1988). Revisione degli Sphodrina (Coleoptera, Carabidae, Sphodrini). Turin: Regional Museum of Natural Sciences.
Casillas-Vargas, G., Ocasio-Malavé, C., Medina, S., Morales Guzmán, C., Del Valle, R. G., Carballeira, N. M., et al. (2021). Antibacterial fatty acids: An update of possible mechanisms of action and implications in the development of the next-generation of antibacterial agents. Prog. Lipid Res. 82:101093. doi: 10.1016/j.plipres.2021.101093
Cherniack, E. P. (2010). Bugs as drugs, part 1: Insects: The “new” alternative medicine for the 21st century? Altern. Med. Rev. 15, 124–135.
CLSI (2009). Clinical and Laboratory Standards Institute methods for dilution antimicrobial susceptibility tests for bacteria that grow aerobically. Approved standard, 8th Edn. Wayne, IL: Clinical and Laboratory Standards Institute.
Daouk, K. D., Dagher, M. S., and Sattout, J. E. (1995). Antifungal activity of the essential oil of Origanum syriacum L. J. Food Prot. 58, 1147–1149. doi: 10.4315/0362-028X-58.10.1147
Denning, D. W. (2022). Antifungal drug resistance: An update. Eur. J. Hosp. Pharm. 29, 109–112. doi: 10.1136/ejhpharm-2020-002604
Desbois, A. P., and Smith, V. J. (2010). Antibacterial free fatty acids: Activities, mechanisms of action and biotechnological potential. Appl. Microbiol. Biotechnol. 85, 1629–1642. doi: 10.1007/s00253-009-2355-3
Dilika, F., Bremner, P. D., and Meyer, J. J. (2000). Antibacterial activity of linoleic and oleic acids isolated from Helichrysum pedunculatum: A plant used during circumcision rites. Fitoterapia 71, 450–452. doi: 10.1016/S0367-326X(00)00150-7
Dimkić, I., Stanković, S., Kabić, J., Stupar, M., Nenadić, M., Ljaljević-Grbić, M. et al. (2020). Bat guano-dwelling microbes and antimicrobial properties of the pygidial gland secretion of a troglophilic ground beetle against them. Appl. Microbiol. Biotechnol. 104, 4109–4126. doi: 10.1007/s00253-020-10498-y
Eisner, T., Aneshansley, D. J., Eisner, M., Attygalle, A. B., Alsop, D. W., and Meinwald, J. (2000). Spray mechanism of the most primitive bombardier beetle (Metrius contractus). J. Exp. Biol. 203, 1265–1275. doi: 10.1242/jeb.203.8.1265
Feng, Y., Zhao, M., He, Z., Chen, Z., and Sun, L. (2009). Research and utilization of medicinal insects in China. Entomol. Res. 39, 313–316. doi: 10.1111/j.17485967.2009.00236.x
Forsyth, D. J. (1972). The structure of the pygidial defence glands of Carabidae (Coleoptera). Trans. Zool. Soc. London 32, 249–309. doi: 10.1111/j.1096-3642.1972.tb00029.x
Fraise, A. P., Wilkinson, M. A., Bradley, C. R., Oppenheim, B., and Moiemen, N. (2013). The antibacterial activity and stability of acetic acid. J. Hosp. Infect. 84, 329–331. doi: 10.1016/j.jhin.2013.05.001
Francke, W., and Dettner, K. (2005). “Chemical signalling in beetles”, in The chemistry of pheromones and other semiochemicals II. Topics in current chemistry, Vol. 240, ed. S. Schulz (Berlin: Springer), 85–166. doi: 10.1007/b98316
Giglio, A., Brandmayr, P., Dalpozzo, R., Sindona, G., Tagarelli, A., Talarico, F., et al. (2009). The defensive secretion of Carabus lefebvrei Dejean, 1826 pupa (Coleoptera, Carabidae): Gland ultrastructure and chemical identification. Microsc. Res. Tech. 72, 351–361. doi: 10.1002/jemt.20660
Giglio, A., Brandmayr, P., Talarico, F., and Zetto, T. (2011). Current knowledge on exocrine glands in carabid beetles: Structure, function and chemical compounds. ZooKeys 100, 193–201. doi: 10.3897/zookeys.100.1527
Giglio, A., Vommaro, M. L., Brandmayr, P., and Talarico, F. (2021). Pygidial glands in Carabidae, an overview of morphology and chemical secretion. Life 11:562. doi: 10.3390/life11060562
Glassman, H. N. (1948). Surface active agents and their application in bacteriology. Bacteriol. Rev. 12, 105–148. doi: 10.1128/br.12.2.105-148.1948
Gómez-García, M., Sol, C., de Nova, P. J. G., Puyalto, M., Mesas, L., Puente, H., et al. (2019). Antimicrobial activity of a selection of organic acids, their salts and essential oils against swine enteropathogenic bacteria. Porc. Health Manag. 5:32. doi: 10.1186/s40813-019-0139-4
Gordon, R. J., and Lowy, F. D. (2008). Pathogenesis of methicillin-resistant Staphylococcus aureus infection. Clin. Infect. Dis. 46(Suppl. 5) 350–359. doi: 10.1086/533591
Gow, N. A. R., Johnson, C., Berman, J., Coste, A. T., Cuomo, C. A., Perlin, D. S., et al. (2022). The importance of antimicrobial resistance in medical mycology. Nat. Commun. 13:5352. doi: 10.1038/s41467-022-32249-5
Guo, J., Li, J., Chen, H., Bond, P. L., and Yuan, Z. (2017). Metagenomic analysis reveals wastewater treatment plants as hotspots of antibiotic resistance genes and mobile genetic elements. Water Res. 123, 468–478. doi: 10.1016/j.watres.2017.07.002
Hänel, H., and Raether, W. (1988). A more sophisticated method of determining the fungicidal effect of water-insoluble preparations with a cell harvester, using miconazole as an example. Mycoses 31, 148–154. doi: 10.1111/j.1439-0507.1988.tb03718.x
Jacqueline, C., Navas, D., Batard, E., Miegeville, A. F., Le Mabecque, V., Kergueris, M. F., et al. (2005). In vitro and in vivo synergistic activities of linezolid combined with subinhibitory concentrations of imipenem against methicillin-resistant Staphylococcus aureus. Antimicrob. Agents Chemother. 49, 45–51. doi: 10.1128/AAC.49.1.45-51.2005
Jiang, H., Wang, K., Yan, M., Ye, Q., Lin, X., Chen, L., et al. (2021). Pathogenic and virulence factor detection on viable but non-culturable methicillin-resistant Staphylococcus aureus. Front. Microbiol. 12:630053. doi: 10.3389/fmicb.2021.630053
Jiang, X., Abgottspon, D., Kleeb, S., Rabbani, S., Scharenberg, M., Wittwer, M., et al. (2012). Antiadhesion therapy for urinary tract infections – A balanced PK/PD profile proved to be key for success. J. Med. Chem. 55, 4700–4713. doi: 10.1021/jm300192x
Kovac, D., and Maschwitz, U. (1990). Secretion-grooming in aquatic beetles (Hydradephaga): A chemical protection against contamination of the hydrofuge respiratory region. Chemoecology 1, 131–138. doi: 10.1007/BF01241654
Larsen, J., Raisen, C. L., Ba, X., Sadgrove, N. J., Padilla-González, G. F., Simmonds, M. S. J., et al. (2022). Emergence of methicillin resistance predates the clinical use of antibiotics. Nature 602, 135–141. doi: 10.1038/s41586-021-04265-w
Laurence, V. M., Jeroen, D. S., Paul, C., and Leen, V. C. (2021). Microbial symbionts of insects as a source of new antimicrobials: A review. Crit. Rev. Microbiol. 47, 562–579. doi: 10.1080/1040841X.2021.1907302
Lečić, S., Ćurčić, S., Vujisić, L., Ćurčić, B., Ćurčić, N., Nikolić, Z., et al. (2014). Defensive secretion in three ground-beetle species (Insecta: Coleoptera: Carabidae). Ann. Zool. Fenn. 51, 285–300. doi: 10.5735/086.051.0301
Li, Z., Mao, R., Teng, D., Hao, Y., Chen, H., Wang, X., et al. (2017). Antibacterial and immunomodulatory activities of insect defensins-DLP2 and DLP4 against multidrug-resistant Staphylococcus aureus. Sci. Rep. 7:12124. doi: 10.1038/s41598-017-10839-4
Lorian, V. (2005). Antibiotics in laboratory medicine, 5th Edn. Philadelphia, PA: Lippincott Williams & Wilkins.
Luise, D., Correa, F., Bosi, P., and Trevisi, P. (2020). A review of the effect of formic acid and its salts on the gastrointestinal microbiota and performance of pigs. Animals 10:887. doi: 10.3390/ani10050887
Madle, W. K., Ajeel, M. A., Alkataan, M. A., Ajeel, A. A., and Abd-alwahab, W. I. A. (2018). Anti-adhesion therapy, a promising alternative in the infections treatment. Iraq. J. Pharm. 15, 46–60. doi: 10.33899/iphr.2018.164196
Mandal, M. D., and Mandal, S. (2011). Honey: Its medicinal property and antibacterial activity. Asian Pac. J. Trop. Biomed. 1, 154–160. doi: 10.1016/S2221-1691(11)60016-6
Murray, C. J. L., Ikuta, K. S., Sharara, F., Swetschinski, L., Aguilar, G. R., Gray, A., et al. (2022). Global burden of bacterial antimicrobial resistance in 2019: A systematic analysis. Lancet 399, 629–655. doi: 10.1016/S0140-6736(21)02724-0
Nandhini, P., Kumar, P., Mickymaray, S., Alothaim, A. S., Somasundaram, J., and Rajan, M. (2022). Recent developments in methicillin-resistant Staphylococcus aureus (MRSA) treatment: A review. Antibiotics 11:606. doi: 10.3390/antibiotics11050606
Nenadić, M. (2019). Antimicrobial and antitumor potential of the secretions and morphological characterization of the glandular structures of the pygidial glands of selected species of ground beetles (Coleoptera: Carabidae). Belgrade: University of Belgrade - Faculty of Biology.
Nenadić, M., Ljaljević-Grbić, M., Stupar, M., Vukojević, J., Ćirić, A., Tešević, V., et al. (2017a). Antifungal activity of the pygidial gland secretion of Laemostenus punctatus (Coleoptera: Carabidae) against cave-dwelling micromycetes. Sci. Nat. 104:52. doi: 10.1007/s00114-017-1474-4
Nenadić, M., Soković, M., Calhelha, R. C., Ferreira, I. C. F. R., Ćirić, A., Vesović, N., et al. (2018). Inhibition of tumour and non-tumour cell proliferation by pygidial gland secretions of four ground beetle species (Coleoptera: Carabidae). Biologia 73, 787–792. doi: 10.2478/s11756-018-0082-x
Nenadić, M., Soković, M., Glamočlija, J., Ćirić, A., Perić-Mataruga, V., Ilijin, L., et al. (2016a). Antimicrobial activity of the pygidial gland secretion of three ground beetle species (Insecta: Coleoptera: Carabidae). Sci. Nat. 103:34. doi: 10.1007/s00114-016-1358-z
Nenadić, M., Soković, M., Glamočlija, J., Ćirić, A., Perić-Mataruga, V., Ilijin, L., et al. (2017b). The pygidial gland secretion of the forest caterpillar hunter, Calosoma (Calosoma) sycophanta: The antimicrobial properties against human pathogens. Appl. Microbiol. Biotechnol. 101, 977–985. doi: 10.1007/s00253-016-8082-7
Nenadić, M., Soković, M., Glamočlija, J., Ćirić, A., Perić-Mataruga, V., Tešević, V., et al. (2016b). Antimicrobial activity of the pygidial gland secretion of the troglophilic ground beetle Laemostenus (Pristonychus) punctatus (Dejean, 1828) (Insecta: Coleoptera: Carabidae). Bull. Entomol. Res. 106, 474–480. doi: 10.1017/S0007485316000109
O’Neill, J. (2014). Antimicrobial resistance: Tackling a crisis for the health and wealth of nations. Review on antimicrobial resistance. London: UK Government.
Ofek, I., Hasty, D. L., and Sharon, N. (2003). Anti-adhesion therapy of bacterial diseases: Prospects and problems. FEMS Immunol. Med. Microbiol. 38, 181–191. doi: 10.1016/S0928-8244(03)00228-1
Pancu, D. F., Scurtu, A., Macasoi, I. G., Marti, D., Mioc, M., Soica, C., et al. (2021). Antibiotics: Conventional therapy and natural compounds with antibacterial activity – A pharmaco-toxicological screening. Antibiotics 10:401. doi: 10.3390/antibiotics10040401
Peh, E., Kittler, S., Reich, F., and Kehrenberg, C. (2020). Antimicrobial activity of organic acids against Campylobacter spp. and development of combinations – A synergistic effect? PLoS One 15:e0239312. doi: 10.1371/journal.pone.0239312
Raftari, M., Jalilian, F. A., Abdulamir, A. S., Son, R., Sekawi, Z., and Fatimah, A. B. (2009). Effect of organic acids on Escherichia coli O157:H7 and Staphylococcus aureus contaminated meat. Open Microbiol. J. 3, 121–127. doi: 10.2174/1874285800903010121
Ratcliffe, N., Azambuja, P., and Mello, C. B. (2014). Recent advances in developing insect natural products as potential modern day medicines. Evid. Based Complement. Altern. Med. 2014, 04958. doi: 10.1155/2014/904958
Ren, C., Wang, Y., Lin, X., Song, H., Zhou, Q., Xu, W., et al. (2020). Combination of formic acid and monolaurin attenuates enterotoxigenic Escherichia coli induced intestinal inflammation in piglets by inhibiting the NF-κB/MAPK pathways with modulation of gut microbiota. J. Agric. Food Chem. 68, 4155–4165. doi: 10.1021/acs.jafc.0c01414
Ricke, S. C., Dittoe, D. K., and Richardson, K. E. (2020). Formic acid as an antimicrobial for poultry production: A review. Front. Vet. Sci. 7:563. doi: 10.3389/fvets.2020.00563
Roberts, P. A., Huebinger, R. M., Keen, E., Krachler, A.-M., and Jabbarim, S. (2018). Predictive modelling of a novel anti-adhesion therapy to combat bacterial colonisation of burn wounds. PLoS Comput. Biol. 14:e1006071. doi: 10.1371/journal.pcbi.1006071
Rodríguez-Tudela, J. L., Barchiesi, F., Bille, J., Chryssanthou, E., Cuenca-Estrella, M., Denning, D., et al. (2003). Method for the determination of minimum inhibitory concentration (MIC) by broth dilution of fermentative yeasts. Clin. Microbiol. Infect. 9, 1–8. doi: 10.1046/j.1469-0691.2003.00789.x
Ryssel, H., Kloeters, O., Germann, G., Schäfer, T., Wiedemann, G., and Oehlbauer, M. (2009). The antimicrobial effect of acetic acid – An alternative to common local antiseptics? Burns 35, 695–700. doi: 10.1016/j.burns.2008.11.009
Sabira, O., Vignesh, A. R., Ajaykumar, A. P., Varma, S. R., Jayaraj, K. N., Sebastin, M., et al. (2022). The chemical composition and antimitotic, antioxidant, antibacterial and cytotoxic properties of the defensive gland extract of the beetle, Luprops tristis Fabricius. Molecules 27:7476. doi: 10.3390/molecules27217476
Seabrooks, L., and Hu, L. (2017). Insects: An underrepresented resource for the discovery of biologically active natural products. Acta Pharm. Sin. B 7, 409–426. doi: 10.1016/j.apsb.2017.05.001
Soković, M., Glamočlija, J., Marin, P. D., Brkić, D., and Van Griensven, L. J. L. D. (2010). Antibacterial effects of the essential oils of commonly consumed medicinal herbs using an in vitro model. Molecules 15, 7532–7546. doi: 10.3390/molecules15117532
Speert, D. P., Wannamaker, L. W., Gray, E. D., and Clawson, C. C. (1979). Bactericidal effect of oleic acid on group A streptococci: Mechanism of action. Infect. Immun. 26, 1202–1210. doi: 10.1128/iai.26.3.1202-1210.1979
Stein, C., Makarewicz, O., Bohnert, J. A., Pfeifer, Y., Kesselmeier, M., Hagel, S., et al. (2015). Three dimensional checkerboard synergy analysis of colistin, meropenem, tigecycline against multidrug-resistant clinical Klebsiella pneumonia isolates. PLoS One 10:e0126479. doi: 10.1371/journal.pone.0126479
Stenz, L., François, P., Fischer, A., Huyghe, A., Tangomo, M., Hernandez, D., et al. (2008). Impact of oleic acid (cis-9-octadecenoic acid) on bacterial viability and biofilm production in Staphylococcus aureus. FEMS Microbiol. Lett. 287, 149–155. doi: 10.1111/j.1574-6968.2008.01316.x
Stojković, D., Drakulić, D., Gašić, U., Zengin, G., Stevanović, M., Rajčević, N., et al. (2020). Ononis spinosa L. an edible and medicinal plant: UHPLC-LTQ-Orbitrap/MS chemical profiling and biological activities of the herbal extract. Food Funct. 11, 7138–7151. doi: 10.1039/D0FO01595D
Vesović, N., Ćurčić, S., Todosijević, M., Nenadić, M., Zhang, W., and Vujisić, L. (2020). Pygidial gland secretions of Carabus Linnaeus, 1758 (Coleoptera: Carabidae): Chemicals released by three species. Chemoecology 30, 59–68. doi: 10.1007/s00049-019-00298-w
Vesović, N., Ćurčić, S., Vujisić, L., Nenadić, M., Krstić, G., Perić-Mataruga, V., et al. (2015). Molecular diversity of compounds from pygidial gland secretions of cave-dwelling ground beetles (Insecta: Coleoptera: Carabidae): The first evidence. J. Chem. Ecol. 41, 533–539. doi: 10.1007/s10886-015-0593-7
Vesović, N., Nenadić, M., Soković, M., Ćirić, A., Vujisić, L., Todosijević, M., et al. (2022). Pygidial glands of the blue ground beetle Carabus intricatus: Chemical composition of the secretion and its antimicrobial activity. Sci. Nat. 109:19. doi: 10.1007/s00114-022-01790-0
Vesović, N., Nenadić, M., Vranić, S., Vujisić, L., Milinčić, K. M., Todosijević, M., et al. (2023). The chemical composition of the secretions, their antibacterial activity, and the pygidial gland morphology of selected European Carabini ground beetles (Coleoptera: Carabidae). Front. Ecol. Evol. 11:1120006. doi: 10.3389/fevo.2023.1120006
Walsh, T. L., Bremmer, D. N., Moffa, M. A., Trienski, T. L., Buchanan, C., Stefano, K., et al. (2021). Impact of an antimicrobial stewardship program-bundled initiative utilizing accelerate Pheno™ system in the management of patients with aerobic Gram-negative bacilli bacteremia. Infection 49, 511–519. doi: 10.1007/s15010-021-01581-1
Wiederhold, N. P. (2017). Antifungal resistance: Current trends and future strategies to combat. Infect. Drug Resist. 10, 249–259. doi: 10.2147/IDR.S124918
Keywords: beetle, antimicrobial, resistant bacteria, Candida species, methicillin-resistant Staphylococcus aureus (MRSA), formic acid, human keratinocyte cell line, IC50
Citation: Nenadić M, Stojković D, Soković M, Ćirić A, Dimkić I, Janakiev T, Vesović N, Vujisić L, Todosijević M, Stanković SS, Ćurčić NB, Milinčić U, Petrović D, Milinčić M and Ćurčić S (2023) The pygidial gland secretion of Laemostenus punctatus (Coleoptera, Carabidae): a source of natural agents with antimicrobial, anti-adhesive, and anti-invasive activities. Front. Ecol. Evol. 11:1148309. doi: 10.3389/fevo.2023.1148309
Received: 19 January 2023; Accepted: 11 April 2023;
Published: 02 June 2023.
Edited by:
Aijun Zhang, United States Department of Agriculture (USDA), United StatesReviewed by:
Mehdi Fatahi-Bafghi, Shahid Sadoughi University of Medical Sciences and Health Services, IranAzucena Gonzalez Coloma, Spanish National Research Council (CSIC), Spain
Copyright © 2023 Nenadić, Stojković, Soković, Ćirić, Dimkić, Janakiev, Vesović, Vujisić, Todosijević, Stanković, Ćurčić, Milinčić, Petrović, Milinčić and Ćurčić. This is an open-access article distributed under the terms of the Creative Commons Attribution License (CC BY). The use, distribution or reproduction in other forums is permitted, provided the original author(s) and the copyright owner(s) are credited and that the original publication in this journal is cited, in accordance with accepted academic practice. No use, distribution or reproduction is permitted which does not comply with these terms.
*Correspondence: Marija Nenadić, bWFyaWphLm5lbmFkaWNAYmlvLmJnLmFjLnJz