- 1State Key Laboratory of Herbage Improvement and Grassland Agro-ecosystems/College of Ecology, Lanzhou University, Lanzhou, China
- 2Key Laboratory of Desert and Desertification/Dunhuang Gobi and Desert Ecology and Environment Research Station, Northwest Institute of Eco-Environment and Resources, Chinese Academy of Sciences, Lanzhou, China
- 3State Key Laboratory of Cryospheric Science, Northwest Institute of Eco-Environment and Resources, Chinese Academy of Sciences, Lanzhou, China
The rapid increase in construction of solar photovoltaic power stations (SPPs) has motivated ecologists to understand how these stations affect terrestrial ecosystems. Comparing study sites, effects are often not consistent, and a more systematic assessment of this topic remains lacking. Here, we evaluated the effects of SPP construction on carbon emissions, edaphic variables, microclimatic factors and vegetation characteristics in a meta-analysis. We employed log response ratios (as effect sizes) to assess how control plots differed from those beneath solar photovoltaic panels. We found that SPP construction decreased the local air temperature and photosynthetically active radiation, while increasing air humidity, especially in grasslands. Furthermore, plant aboveground biomass and vegetation cover were also enhanced by SPP construction in grassland ecosystems. In farmland ecosystems, photovoltaic panel installation increased plant aboveground biomass, soil available phosphorus and soil pH, while reducing CO2 flux, plant species richness and vegetation cover in woodlands. Thus, while SPP construction had profound ecological impacts in terrestrial ecosystems, the direction and strength of these effects were largely dependent on ecosystem type. Most studies of SPP construction to date have focused on local microclimatic and plant diversity effects, but few studies have examined effects on ecosystem functions and services. Future assessments are needed of both the benefits and disbenefits of SPP construction across different ecosystems, to improve SPP site selection and adaptive management.
1. Introduction
Replacing fossil fuels with clean energy sources to reduce carbon emissions is an important step toward achieving carbon neutrality (Armstrong et al., 2014). In recent years, great progress has been made in exploiting renewable resources to optimize existing energy infrastructure (Li, 2021). Photovoltaic (PV) power generation using solar energy is one of the most promising technologies for sustainable energy generation (Wilberforce et al., 2019; Bogdanov et al., 2021). In 2018, global solar PV capacity accounted for 55% of all new renewable energy capacity (Dunnett et al., 2020). The installed capacity of solar PV systems is expected to reach 4,600 GW by 2050 and avert up to 4 Gt of CO2 emissions annually (Wang and Cong, 2021). Currently, the world’s PV power capacity accounts for about 1% of total power capacity, by 2030, it is expected to provide 15% of the global electricity supply (Helveston et al., 2022), crucially helping achieve global carbon neutrality (Liu et al., 2012).
The construction of SPPs has profound effects on terrestrial ecosystems, because ground-mounted PV panels are considered a new form of land use change, shading large areas of previously open land (Turney and Fthenakis, 2011; Armstrong et al., 2016; Chang et al., 2016). Previous studies have found that SPP construction can affect the local climate and soil environment (Hong and Kim, 2008; De Marcoa et al., 2014; Choi et al., 2020) by perturbing the surface energy balance (Mercado et al., 2009; Nemet, 2009; Broadbent et al., 2019; Yu et al., 2020; Lambert et al., 2021). Photovoltaic panels can affect air humidity and soil water content by moderating the photosynthetically active radiation (PAR) received (Weinstock and Appelbaum, 2009; Lu, 2013), as well as by significantly reducing wind speed and turbulence (Armstrong et al., 2016; Zhao, 2016; Yin et al., 2017). These effects in turn affect plant communities, altering plant biomass, species richness and vegetation cover (Liu et al., 2019; Zhai et al., 2020; Lambert et al., 2022). Local impacts of SPPs on the microclimate and vegetation may further influence broader ecosystem processes, such as carbon flux balance and soil water retention capacity, leading to changes in ecosystem services across spatial scales (Armstrong et al., 2014; Liu et al., 2020).
However, the ecological impacts of SPPs reported to date vary substantially among studies. In the Mu Us Desert, China (Liu et al., 2019), PAR was reduced by 67.4% beneath PV panels as compared to an unaltered area. However, the installation of PV panels did not affect PAR in the desert ecosystems of Inner Mongolia, China (Zhao, 2016) or in the farmland ecosystems of Italy (Vervloesem et al., 2022). A 83.9% increase in vegetation cover and 68.7% increase in plant biomass were associated with PV panels in the Gonghe Basin, Qinghai Province, China (Li et al., 2016). Similarly, Wang et al. (2016) reported a 128% increase in the fresh weight of plant biomass under PV panels. In contrast, in a woodland ecosystem in the south of France (Lambert et al., 2022), aboveground biomass, soil temperature and vegetation cover were significantly reduced beneath PV panels, with soil temperature a full 10% lower (Lambert et al., 2021). Similarly, in a farmland ecosystem in central Italy (Moscatelli et al., 2022), soil temperature was reduced by PV panels. However, in the Mu Us Desert (Liu et al., 2019), soil temperature was 3°C higher under PV panels as compared to controls. For soil pH, contrasting results have also been reported. Under PV panels, soil pH increased by 14.04% in central Italy (Moscatelli et al., 2022) but decreased by 13.93% in Datong, China (Liu, 2020).
To understand the overall ecological impacts of SPPs on terrestrial ecosystems. We conducted a meta-analysis of SPP effects on carbon processes, edaphic factors, microclimatic factors and vegetation characteristics. We hypothesized that the mixed effects observed to date were caused by variations among study ecosystems; we therefore examined how ecosystem type (i.e., desert, farmland, grassland, or woodland) affected the SPP ecological impacts. Our study is hoped to provide a comprehensive evaluation of SPP impacts on terrestrial ecosystems and to improve the rational management and ecological restoration of SPP localities.
2. Materials and methods
2.1. Data collection
To test how PV panels affected terrestrial ecosystem ecology, we conducted a systematic literature search using the ISI Web of Science and CNKI for the terms [photovoltaic AND (ecology system OR vegetation OR biodiversity OR soil nutrient OR soil moisture OR soil temperature)]. The investigations spanned published records from 1st January 2010 to 31st December 2022. This search led to an initial set of 1,491 publications. These were checked for relevance by examining the title, abstract and main text in succession (Supplementary Figure S1).
We collected data from publications that met the following three criteria: (1) a focus on the ecological and environmental impact of SPPs, including at least one indicator of carbon flux, microclimatic factors, the soil environment and/or vegetation characteristics; (2) the experimental design included both control and experimental groups, and each group contained at least three replicates; (3) the mean and standard deviation (or variance, standard error and 95% confidence interval) of relevant indexes were provided. After screening, a total of 16 papers were selected for data extraction and further analysis (Supplementary Table S1).
Data were extracted from the main text and tables, or indirectly from figures using “GetData.” The indices we extracted included microclimatic factors (air humidity, air temperature and PAR), soil characteristics (available phosphorus, CO2 flux, pH, soil temperature, soil water content, total carbon and total nitrogen) and vegetation characteristics (plant aboveground biomass, Shannon-Wiener diversity index, Simpson’s diversity index, species richness and vegetation cover). For each indicator, we recorded the mean, standard deviation (or variance, standard error and 95% confidence interval) and sample size.
2.2. Statistical analysis
We calculated effect sizes based on the mean (Y), standard deviation (SD) and sample size (n) of each indicator and derived the variance associated with each study. In cases where only a standard error (SE) was provided, it was converted to a SD using (1):
The log response ratio (RR) was used as a measure of the effect size to quantify differences between control and experimental groups (Hedges et al., 1999) and was calculated as follows:
where Ye is the mean value of the experimental group, and Yc is the mean value of the control group. The corresponding variance (Vi) for RR was calculated as follows:
where Ne is the sample size of the experimental group and Nc the control group.
We built a series of random effects models by employing the rma.mv function from the “metafor” package in R (4.2.1) (Wallace et al., 2017). “Study case” was nested in “literature” as a random effect. The cumulative effect value (), weights of individual studies (wi*), population variance (VM), and the total standard error (SEM) and its 95% confidence interval were calculated according to the following formulas:
If the 95% confidence interval encompassed zero, the indicator in question did not differ significantly between the control and experimental groups (p > 0.05). Lastly, we used “Origin 2022” to draw a “forest map” showing the meta-analysis results.
3. Results
3.1. Effects of solar photovoltaic panel construction on microclimate factors
Overall, air temperature (mean ln RR = −0.073, 95% CI = [−0.125, −0.021], p = 0.006) and PAR (ln RR = −1.563, [−2.537, −0.590], p = 0.002) were significantly lower within PV panel plots than within control plots, while air humidity (ln RR = 0.144, [0.013, 0.274], p = 0.031) showed the opposite pattern (Figure 1). Due to shading by PV panels, the PAR received by farmland (ln RR = −2.830, [−3.664, −1.996], p < 0.001) and grassland (ln RR = −2.100, [−2.558, −1.642], p < 0.001) ecosystems was lower, respectively, as compared to control plots. Meanwhile, air humidity (ln RR = 0.223, [0.088, 0.357], p = 0.001) increased and air temperature (ln RR = −0.099, [−0.144, −0.054], p < 0.001) decreased by in grassland ecosystems in experimental versus control plots (Figure 1).
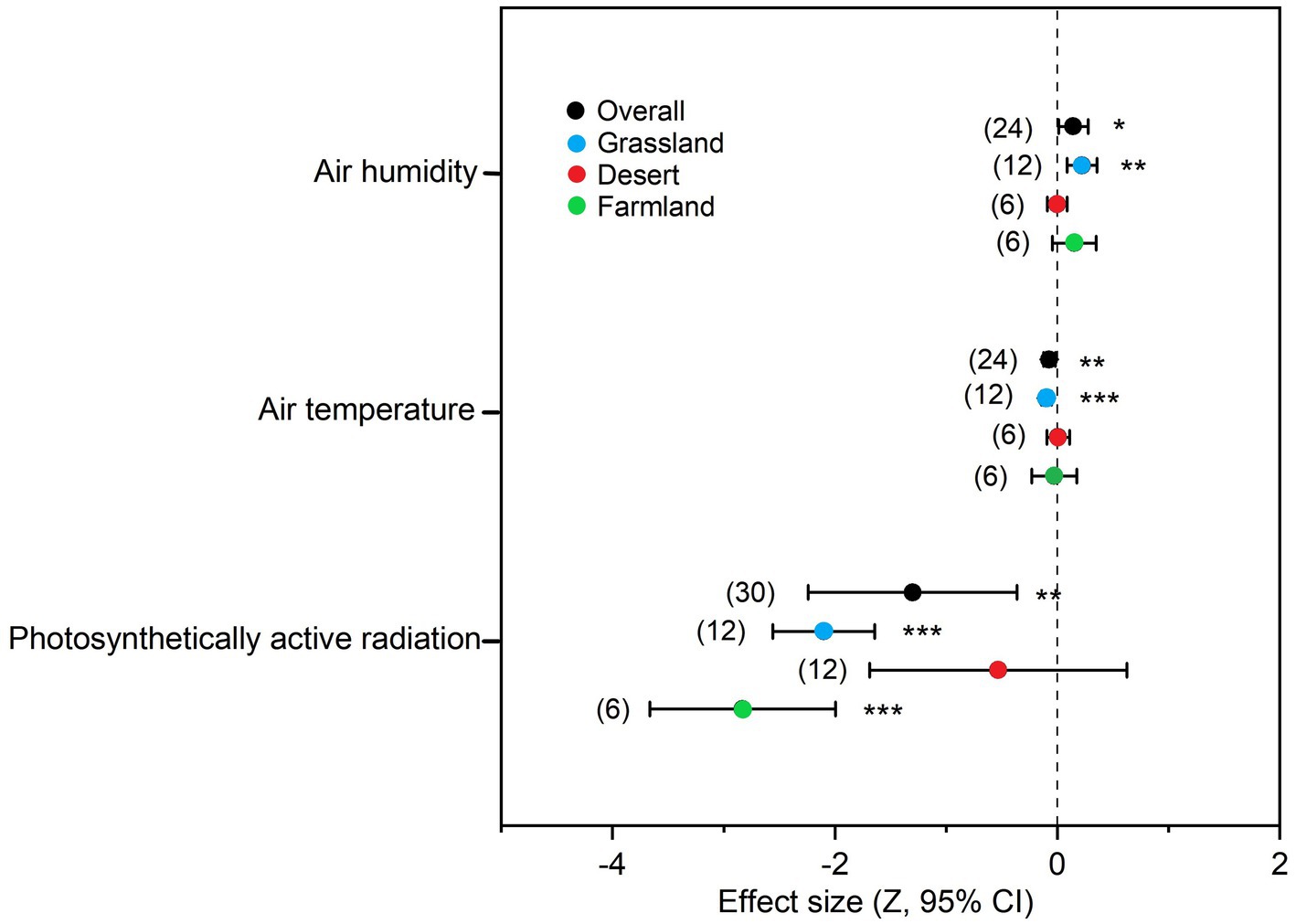
Figure 1. Effects of solar photovoltaic panels on microclimatic conditions in terrestrial ecosystems. Error bars indicate 95% confidence intervals (CIs). If the CI did not overlap zero, the response was considered significant: *p < 0.05, **p < 0.01, and ***p < 0.001. The numbers in parentheses represent the sample size of each observation.
3.2. Effects of solar photovoltaic panel construction on soil conditions
The construction of SPPs had no significant effect on total carbon, soil total nitrogen, soil temperature, or soil water content in terrestrial ecosystems (Figure 2). In farmland ecosystems, the soil available phosphorus (ln RR = 2.363, [0.279, 4.448], p = 0.026) and soil pH (ln RR = 0.154, [0.003, 0.304], p = 0.045) were higher within PV panel plots versus controls, whereas the soil pH (ln RR = −0.108, [−0.136, −0.081], p < 0.001) decreased with PV panel construction in grassland ecosystems. SPP construction did not affect the overall CO2 flux, but reduced CO2 fluxes (ln RR = −1.053, [−1.404, −0.702], p < 0.001) in woodland ecosystems (Figure 2).
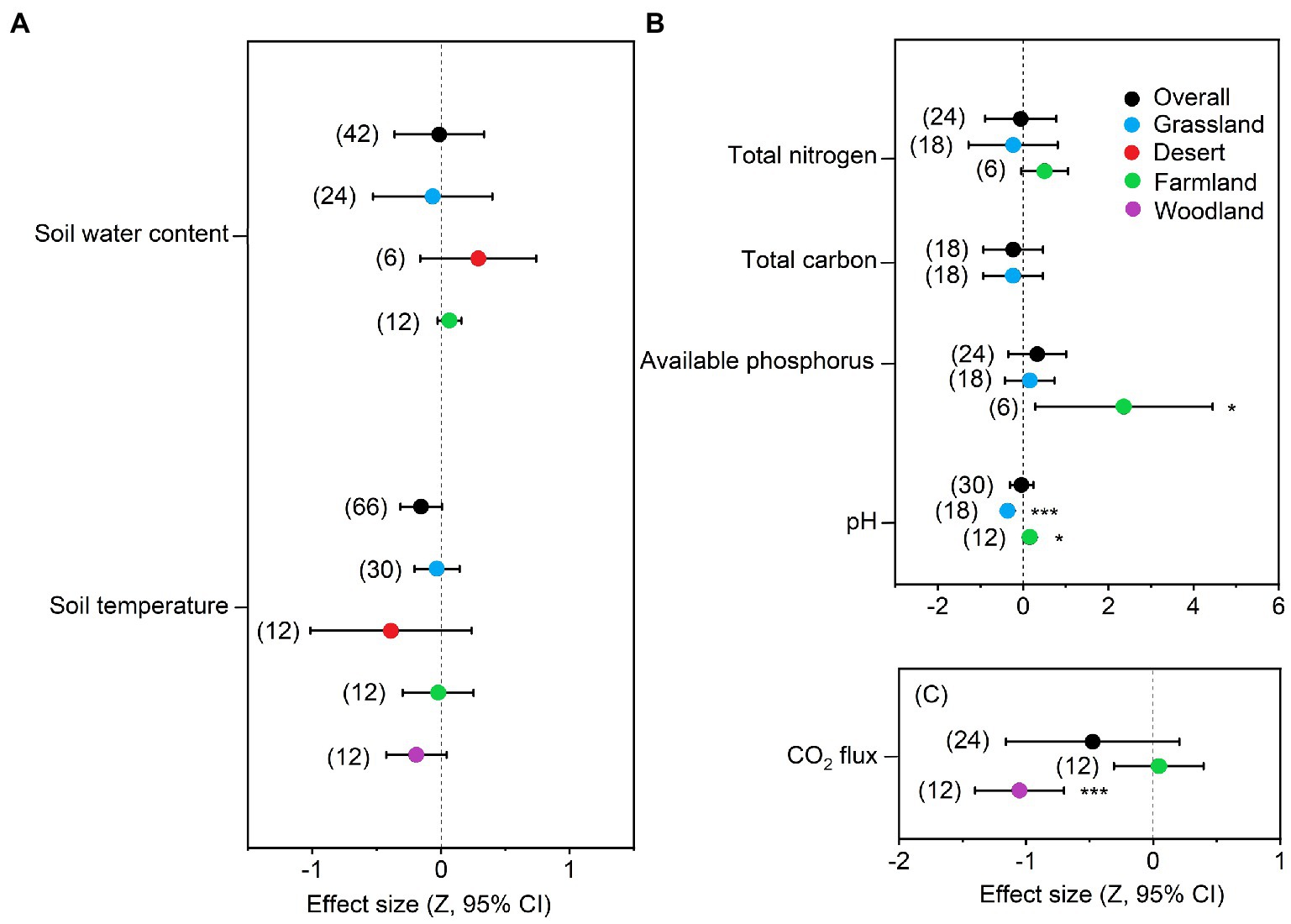
Figure 2. Effects of solar photovoltaic panels on the soil environment in terrestrial ecosystems. (A) Soil physical properties, (B) soil chemical properties, and (C) soil functions. Error bars indicate 95% confidence intervals (CIs). If the CI did not overlap zero, the response was considered significant: *p < 0.05, **p < 0.01, and ***p < 0.001. The numbers in parentheses represent the sample size of each observation.
3.3. Effects of solar photovoltaic panel construction on vegetation characteristics
The use of SPPs enhanced vegetation cover in grassland (ln RR = 1.000, [0.290, 1.709], p = 0.006) and desert (ln RR = 1.960, [1.742, 2.178], p < 0.001) ecosystems while decreasing the vegetation cover in woodland ecosystems (ln RR = −0.806, [−1.230, −0.398], p < 0.001; Figure 3). Compared with control plots, the aboveground biomass was higher in PV panel plots, with the aboveground biomass greater in grasslands (ln RR = 0.751, [0.571, 0.931], p < 0.001) and farmlands (ln RR = 0.823, [0.750, 0.896], p < 0.001), respectively (Figure 3). In general, PV panel construction did not affect plant species diversity indices, with the exception that plant species richness (ln RR = −0.555, [−0.720, −0.390], p < 0.001) was significantly reduced in woodland ecosystems.
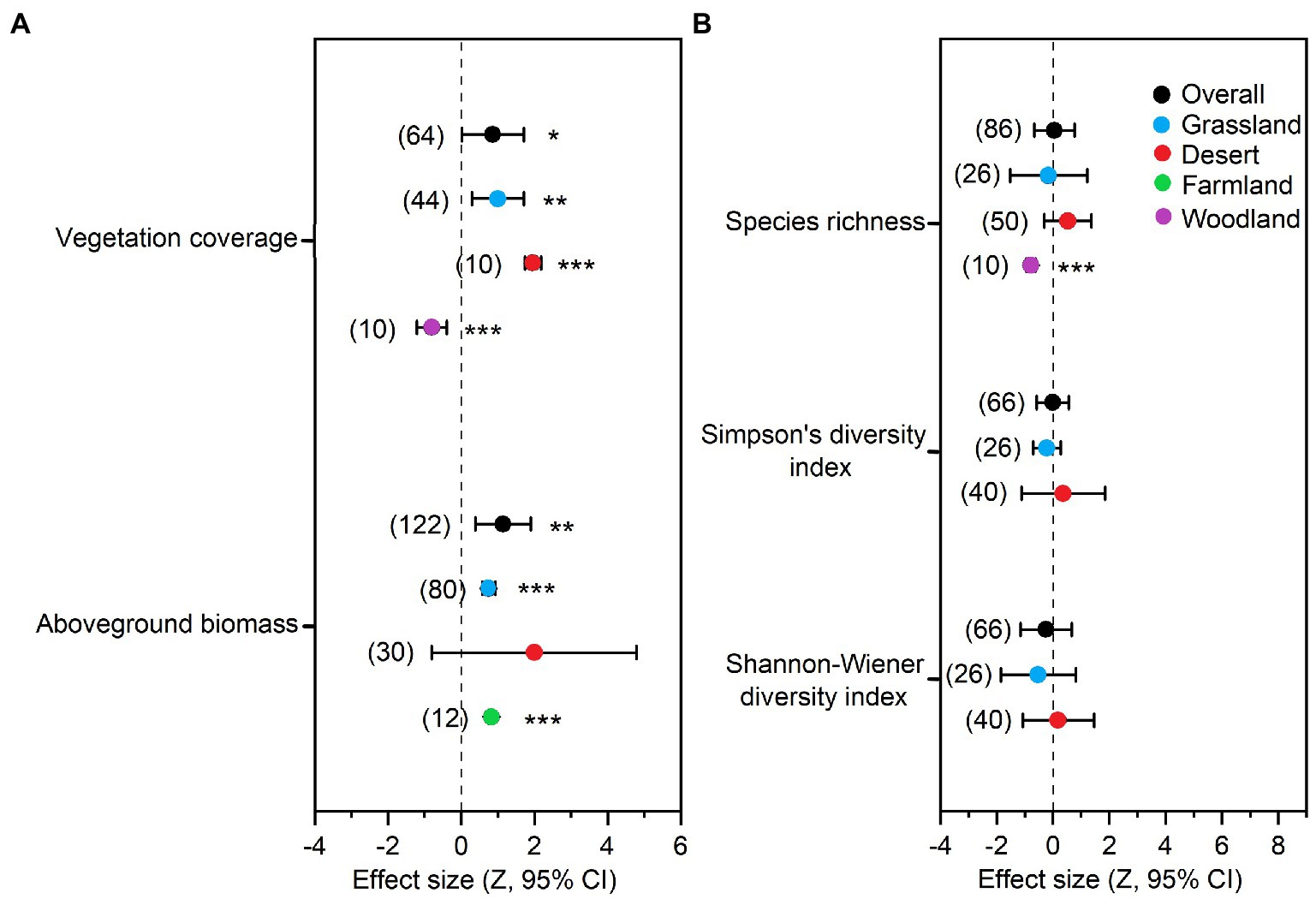
Figure 3. Effects of solar photovoltaic panels on vegetation characteristics in terrestrial ecosystems. (A) Aboveground biomass and cover and (B) plant diversity. Error bars indicate 95% confidence intervals (CIs). If the CI did not overlap zero, the response was considered significant: *p < 0.05, **p < 0.01, and ***p < 0.001. The numbers in parentheses represent the sample size of each observation.
4. Discussion
Overall, SPP construction had a significant effect on microclimatic factors such as air humidity, air temperature and PAR, which are major climate moderators influencing vegetation growth and terrestrial ecosystem functioning. In PV panel plots, PAR was much lower than in control plots, especially in grassland and farmland ecosystems. Photovoltaic panels convert solar radiation into electricity and therefore block sunlight from reaching the ground (Lewis and Nocera, 2006), the land surface beneath PV panels receives less radiation than uncovered land (Zhou et al., 2012). Here, we found that SPP had no significant effect on PAR in deserts, while another study (Liu et al., 2019) found a 67.3% decrease. In the desert system studied by Liu et al. (2019), vegetation cover was enhanced under the PV panels, resulting in less solar radiation reaching the surface. Changes in PAR induced by SPPs may lead to further alterations of other environmental factors. For example, in the Sahara, the PV panels of large-scale solar farms directly reduce surface albedo, creating a positive albedo-precipitation-vegetation feedback loop that triggers increases in temperature and precipitation (Li et al., 2018).
Compared to control plots, PV panel plots had significantly lower air temperature, with an average air temperature decrease in grassland ecosystems. The cooling of the land surface associated with SPP construction is related to the physical shading caused by PV panels (Marrou et al., 2013) and the interception of shortwave radiation by the PV arrays (Weinstock and Appelbaum, 2009). In grassland ecosystems, hot PV panel surfaces can heat the ambient air, driving a PV heat-island effect (Chang et al., 2018).
Air humidity is another important factor affecting vegetation. Overall, SPP construction led to a significant increase in air humidity, especially in grassland ecosystems, where air humidity increased. This increase in air humidity may be caused by a reduction in net radiation and air temperature under the PV panels (Chang et al., 2018), or by changes in wind speed and turbulent mixing at the land surface (Armstrong et al., 2014). How SPPs affect air humidity can also be closely linked to the vegetation status of an area. For example, Zhu et al. (2021) found that SPPs tended to indirectly affect humidity by regulating plant distribution and growth within the area.
Changes in microclimatic factors can produce corresponding changes in soil temperature and soil water content. However, SPP construction did not impact the overall physical properties of the soil. In a recent study (Zhou and Wang, 2019), soil organic matter, pH and total N and P varied little between PV panel rows and undisturbed areas outside the panel rows. We also did not detect an effect of SPP construction on soil total C or N content. However, SPP construction had contrasting effects on soil pH in farmlands versus grasslands. This may be due to variation among ecosystems in a number of important influential factors, including: Na salt leaching from PV panels, precipitation, soil erosion, surface runoff, vegetation characteristics and wind speed (Golubiewski, 2006; Cook, 2011; Barron-Gafford et al., 2019; Moscatelli et al., 2022). In farmland ecosystems, elevation of soil pH (from moderately acidic to slightly acidic) can increase the availability of soil P (Moscatelli et al., 2022). This reduction in soil C and N content is likely caused by the removal of topsoil during PV array construction (Choi et al., 2022). However, soil texture may also be an important determinant of how PV panels affect soil nutrient cycling (Mazaheri and Mahmoodabadi, 2012).
Our study found no net effect of SPP construction on CO2 flux, but a decrease in CO2 flux in woodland ecosystems. This decline in soil respiration may be due to reductions in plant growth and surface clearance under the PV panels (Lambert et al., 2021). To date, there remain few case studies of CO2 fluxes under SPP construction, with only a small amount of data from agricultural and forest ecosystems and no data from other ecosystems. Therefore, our assessment of SPP impacts on CO2 fluxes and other greenhouse gas emissions in terrestrial ecosystems is preliminary, and considerable future research will be required to fully elucidate these effects.
The construction of SPPs leads to changes in local shading and a redistribution of precipitation, as well as the evaporation of soil water (Cui et al., 2017). Together, the combined effect of these changes, as well as shifts in PAR and other soil variables, can greatly affect plant growth and community succession (Yan, 2017; Liu et al., 2022). Overall, in this study, both the vegetation cover and plant productivity (as aboveground biomass) were much increased under PV panels as compared to controls. In farmland and grassland ecosystems, aboveground biomass and vegetation cover increased significantly. For comparison, Wang et al. (2016) found increases in both the fresh (128%) and dry (127%) weight of aboveground plant materials under PV panels, while in the Gonghe Basin of China, PV panels resulted in increases in vegetation cover (83.9%) and aboveground biomass (68.7%) (Li et al., 2016). Despite variation in plant productivity and cover changes, SPP construction appears to be beneficial to plant growth in most cases.
Improved plant growth can lead to the absorption of more carbon from the atmosphere, while also regulating the terrestrial carbon cycle through plant–soil processes and thereby affecting soil carbon storage, greenhouse gas emissions and ecosystem stability (Bardgett et al., 2008). The enhanced plant productivity seen at SPP sites allows more litter to enter the soil, providing a greater influx of nutrients, improving soil structure and increasing soil water holding capacity (Jia et al., 2018). More vegetation cover, especially in arid, sandy areas where vegetation cover is typically low, can provide protection from wind erosion (Wang, 2015). Taken together, the establishment of SPPs can not only provide electricity (via a new energy source), but also precipitate ecological benefits by enhancing vegetation cover.
We found no significant impact of SPP construction on plant species diversity. However, Wang et al. (2016) found that the Shannon-Wiener and Simpson’s diversity indices increased by 60% and 32%, respectively, under PV panels as compared to control areas. The same trend was found by Zhang et al. (2020) in a desert ecosystem, where species richness, the Shannon-Wiener diversity index and Simpson’s diversity indices were higher below PV panels than outside the SPP. In Gonghe Basin, plant species richness increased by 119.2% under PV panels (Li et al., 2016). However, fewer plant species and lower species diversity occurred under PV panels in a typical grassland area (Du and Sun, 2017; Zhai et al., 2018). This indicates that the effects of PV panels on plant diversity are varied and may even be in opposite directions. Furthermore, SPP construction may cause changes in plant community structure, which may not necessarily be reflected in plant diversity shifts (Zhai et al., 2018). Understanding the specifics of how SPP construction affects plant community structure requires further study.
Woodland ecosystems showed different responses to SPP construction than other ecosystem types. In woodlands, we found reductions in plant species richness and vegetation cover beneath PV panels. This is probably because, in woodland ecosystems, woody plants are removed and the land surface cleared prior to PV array construction (Lambert et al., 2022).
5. Conclusion
In our meta-analysis, SPP construction was found to significantly alter the local climate, increasing air temperature and PAR, while decreasing air humidity, these impacts were more prominent in grasslands versus other terrestrial ecosystems. However, SPP effects on soil parameters were generally not significant, perhaps due to the substantial spatial and temporal variation observed in these effects. Nevertheless, changes in vegetation caused by shifting environmental factors were evident. In most studies, SPP construction has been found to increase plant productivity (as aboveground biomass) and vegetation cover, except for in woodlands, where woody plants are removed during SPP construction. SPP projects not only generate clean energy, but also provide additional benefits by greening land surfaces in arid areas and increasing carbon sequestration. However, given the variation in these effects among ecosystem types, caution needs to be taken when considering future SPP development and management across regions.
Data availability statement
The original contributions presented in the study are included in the article/Supplementary material, further inquiries can be directed to the corresponding authors.
Author contributions
JW and SC conceived the work. YZ and ZT collected the data. YZ analyzed the data and wrote the first draft. All authors contributed to the article and approved the submitted version.
Funding
This work was supported by the Gansu Provincial Key Program of Science Fund (22JR5RA396), Fundamental Research Funds for the Central Universities (lzujbky-2021-sp51) and National Key Research and Development Program of China (2022YFB4202102).
Conflict of interest
The authors declare that the research was conducted in the absence of any commercial or financial relationships that could be construed as a potential conflict of interest.
Publisher’s note
All claims expressed in this article are solely those of the authors and do not necessarily represent those of their affiliated organizations, or those of the publisher, the editors and the reviewers. Any product that may be evaluated in this article, or claim that may be made by its manufacturer, is not guaranteed or endorsed by the publisher.
Supplementary material
The Supplementary material for this article can be found online at: https://www.frontiersin.org/articles/10.3389/fevo.2023.1151182/full#supplementary-material
References
Armstrong, A., Ostle, N. J., and Whitaker, J. (2016). Solar park microclimate and vegetation management effects on grassland carbon cycling. Environ. Res. Lett. 11:074016. doi: 10.1088/1748-9326/11/7/074016
Armstrong, A., Waldron, S., Whitaker, J., and Ostle, N. J. (2014). Wind farm and solar park effects on plant-soil carbon cycling: uncertain impacts of changes in ground-level microclimate. Glob. Chang. Biol. 20, 1699–1706. doi: 10.1111/gcb.12437
Bardgett, R. D., Freeman, C., and Ostle, N. J. (2008). Microbial contributions to climate change through carbon cycle feedbacks. ISME J. 2, 805–814. doi: 10.1038/ismej.2008.58
Barron-Gafford, G. A., Pavao-Zuckerman, M. A., Minor, R. L., Sutter, L. F., Barnett-Moreno, I., Blackett, D. T., et al. (2019). Agrivoltaics provide mutual benefits across the food-energy-water nexus in drylands. Nat. Sust. 2, 848–855. doi: 10.1038/s41893-019-0364-5
Bogdanov, D., Gulagi, A., Fasihi, M., and Breyer, C. (2021). Full energy sector transition towards 100% renewable energy supply: integrating power, heat, transport and industry sectors including desalination. Appl. Energy 283:116273. doi: 10.1016/j.apenergy.2020.116273
Broadbent, A. M., Krayenhoff, E. S., Georgescu, M., and Sailor, D. J. (2019). The observed effects of utility-scale photovoltaics on near-surface air temperature and energy balance. J. Appl. Meteorol. Climatol. 58, 989–1006. doi: 10.1175/JAMC-D-18-0271.1
Chang, Z. F., Liu, S. Z., Zhu, S. J., Han, F. G., Zhong, S. N., and Duan, X. F. (2016). Ecological functions of PV power plants in the desert and Gobi. J. Resour. Ecol. 7, 130–136. doi: 10.5814/j.issn.1674-764x.2016.02.008
Chang, R., Shen, Y. B., Luo, Y., Wang, B., Yang, Z. B., and Guo, P. (2018). Observed surface radiation and temperature impacts from the large-scale deployment of photovoltaics in the barren area of Gonghe. Chin. Renew. Energ. 118, 131–137. doi: 10.1016/j.renene.2017.11.007
Choi, C. S., Cagle, A. E., Macknick, J., Macknick, J., Bloom, D. E., and Caplan, J. S. (2020). Effects of revegetation on soil physical and chemical properties in solar photovoltaic infrastructure. Front. Environ. Sci. 8:140. doi: 10.3389/fenvs.2020.00140
Choi, C. S., Cagle, A. E., Macknick, J., Bloom, D. E., Caplan, J. S., and Ravi, S. (2022). Effects of revegetation on soil physical and chemical properties in solar photovoltaic infrastructure. Front. Environ. Sci. 8:140. doi: 10.3389/fenvs.2020.00140
Cook, P. (2011). Infrastructure, rural electrification and development. Energy Sust. Dev. 15, 304–313. doi: 10.1016/j.esd.2011.07.008
Cui, Y. Q., Feng, Q., Sun, J. H., and Xiao, J. H. (2017). A review of revegetation pattern of photovoltaic in Northwest China. B. Soil Water Conserv. 37, 200–203. doi: 10.13961/j.cnki.stbctb.2017.03.033
De Marcoa, A., Petrosillo, I., Semeraro, T., Pasimeni, M. R., Aretano, R., and Zurlini, G. (2014). The contribution of utility-scale solar energy to the global climate regulation and its effects on local ecosystem services. Glob. Ecol. Conserv. 2, 324–337. doi: 10.1016/j.gecco.2014.10.010
Du, H., and Sun, F. (2017). Preliminary study on the impact of photovoltaic power plant operation on vegetation. Environ. Dev. 29, 30–31. doi: 10.16647/j.cnki.cn15–1369/x.2017.08.015
Dunnett, S., Sorichetta, A., Taylor, G., and Eigenbrod, F. (2020). Harmonised global datasets of wind and solar farm locations and power. Sci. Data. 7:130. doi: 10.1038/s41597-020-0469-8
Golubiewski, N. E. (2006). Urbanization increases grassland carbon pools: effects of landscaping in Colorado’s front range. Ecol. Appl. 16, 555–571. doi: 10.1890/1051-0761(2006)016[0555:UIGCPE]2.0.CO;2
Hedges, L. V., Gurevitch, J., and Curtis, P. S. (1999). The meta-analysis of response ratios in experimental ecology. Ecol. 80, 1150–1156. doi: 10.1890/0012-9658(1999)080[1150:TMAORR]2.0.CO;2
Helveston, J. P., He, G., and Davidson, M. R. (2022). Quantifying the cost savings of global solar photovoltaic supply chains. Nature 612, 83–87. doi: 10.1038/s41586-022-05316-6
Hong, J., and Kim, J. (2008). Simulation of surface radiation balance on the Tibetan plateau. Geophys. Res. Lett. 35:L08814. doi: 10.1029/2008GL033613
Jia, C., Huang, Z., Miao, H. T., Lu, R., Li, J., and Liu, Y. (2018). Litter crusts promote herb species formation by improving surface microhabitats in a desert ecosystem. Catena 171, 245–250. doi: 10.1016/j.catena.2018.07.024
Lambert, Q., Bischoff, A., Cueff, S., Cluchier, A., and Gros, R. (2021). Effects of solar park construction and solar panels on soil quality, microclimate, CO2 fluxes, and vegetation under a Mediterranean climate. Land Degrad. Dev. 32, 5190–5202. doi: 10.1002/ldr.4101
Lambert, Q., Gros, R., and Bischoff, A. (2022). Ecological restoration of solar park plant communities and the effect of solar panels. Ecol. Eng. 182:106722. doi: 10.1016/j.ecoleng.2022.106722
Lewis, N. S., and Nocera, D. G. (2006). Powering the planet: chemical challenges in solar energy utilization. Proc. Natl. Acad. Sci. U. S. A. 104, 15729–15735. doi: 10.1073/pnas.0603395103
Li, Q. S. (2021). Discussion on the path of China’s energy transformation under the goal of carbon neutrality. Chin. Coal. 47, 1–7. doi: 10.19880/j.cnki.ccm.2021.08.001
Li, S. H., Gao, Q., Wang, X. Q., Lan, L., and Yang, Z. W. (2016). Characteristics of vegetation and soil property changes by photovoltaic plant interference in alpine desert steppe. J. Soil Water Conserv. 30, 325–329. doi: 10.13870/j.cnki.stbcxb.2016.06.054
Li, Y., Kalnay, E., Motesharrei, S., Rivas, J., Kucharski, F., and Kirk-Davidoff, D. (2018). Climate model shows large-scale wind and solar farms in the Sahara increase rain and vegetation. Science 361, 1019–1022. doi: 10.1126/science.aar5629
Liu, Y. (2020). Effects of photovoltaic power station on two typical degraded ecosystems in northern Shanxi Province. Shanxi Univ. Fin. Econ. 1–80. doi: 10.27283/d.cnki.gsxcc.2020.000066
Liu, J., Cao, L., Ma, J., and Zhang, J. (2012). Economic analysis of user’s grid-connected PV system based on energy storage system. Acta. Energ. Sol. Sin. 33, 1887–1892. doi: 10.3969/j.issn.0254–0096.2012.11.009
Liu, Y., Zhang, R. Q., Huang, Z., Cheng, Z., Lopez-Vicente, M., Ma, X. R., et al. (2019). Solar photovoltaic panels significantly promote vegetation recovery by modifying the soil surface microhabitats in arid sandy ecosystem. Land Degrad. Dev. 30, 2177–2186. doi: 10.1002/ldr.3408
Liu, X., Zhang, P., and Liu, J. Q. (2022). Inorganic fertilizers are limiting factors of vegetation restoration of Qinghai Tala shoal photovoltaic power station. Biodivers. Sci. 30, 22100–22136. doi: 10.17520/biods.2022100
Liu, Y., Zhang, R. Q., Ma, X. R., and Wu, G. L. (2020). Combined ecological and economic benefits of the solar photovoltaic industry in arid sandy ecosystems. J. Clean. Prod. 262:121376. doi: 10.1016/j.jclepro.2020.121376
Lu, X. (2013). The environment effect analysis of PV power plant construction in desert Gobi Lanzhou University Available at: https://kns.cnki.net/KCMS/detail/detail.aspx?dbname=CMFD201302&filename=1013237744.nh.
Marrou, H., Dufour, L., and Wery, J. (2013). How does a shelter of solar panels influence water flows in a soil-crop system? Eur. J. Agron. 50, 38–51. doi: 10.1016/j.eja.2013.05.004
Mazaheri, M. R., and Mahmoodabadi, M. (2012). Study on infiltration rate based on primary particle size distribution data in arid and semiarid region soils. Arab. J. Geosci. 5, 1039–1046. doi: 10.1007/s12517-011-0497-y
Mercado, L. M., Bellouin, N., Sitch, S., Boucher, O., Huntingford, C., Wild, M., et al. (2009). Impact of changes in diffuse radiation on the global land carbon sink. Nature 458, 1014–1017. doi: 10.1038/nature07949
Moscatelli, M. C., Marabottini, R., Massaccesi, L., and Marinari, S. (2022). Soil properties changes after seven years of ground mounted photovoltaic panels in Central Italy coastal area. Geoderma Reg. 29:e00500. doi: 10.1016/j.geodrs.2022.e00500
Nemet, G. F. (2009). Net radiative forcing from widespread deployment of photovoltaics. Environ. Sci. Technol. 43, 2173–2178. doi: 10.1021/es801747c
Turney, D., and Fthenakis, V. (2011). Environmental impacts from the installation and operation of large-scale solar power plants. Renew. Sust. Energ. Rev. 15, 3261–3270. doi: 10.1016/j.rser.2011.04.023
Vervloesem, J., Marcheggiani, E., Choudhury, M. A. M., and Muys, B. (2022). Effects of photovoltaic solar farms on microclimate and vegetation diversity. Sustain. 14:7493. doi: 10.3390/su14127493
Wallace, B. C., Lajeunesse, M. J., Dietz, G., Dahabreh, I. J., Trikalinos, T. A., Schmid, C. H., et al. (2017). OpenMEE: intuitive, open-source software for meta-analysis in ecology and evolutionary biology. Methods Ecol. Evol. 8, 941–947. doi: 10.1111/2041-210X.12708
Wang, T. (2015). The impact of photovoltaic power construction on soil and vegetation in Jingbian County Northwest A&F University Available at: https://kns.cnki.net/kcms/detail/detail.aspx?FileName=1015329905.nh&DbName=CMFD2016.
Wang, W. H., and Cong, W. (2021). Analysis of IEAs net zero by 2050:a roadmap for the global energy sector. Int. Petroleum. Econ. 29, 1–7. Available at: https://kns.cnki.net/kcms/detail/detail.aspx?FileName=GJJJ202106002&DbName=CJFQ2021
Wang, T., Wang, D. X., Guo, T. D., Zhang, G. G., Zhao, S. X., Niu, H. C., et al. (2016). The impact of photovoltaic power construction on soil and vegetation. Res. Soil Water Conserv. 23, 90–94. doi: 10.13869/j.cnki.rswc.2016.03.016
Weinstock, D., and Appelbaum, J. (2009). Optimization of solar photovoltaic fields. J. Sol. Energy. Eng. 131:031003. doi: 10.1115/1.3142705
Wilberforce, T., Baroutaji, A., El Hassan, Z., Thompson, J., Soudan, B., and Olabi, A. G. (2019). Prospects and challenges of concentrated solar photovoltaics and enhanced geothermal energy technologies. Sci. Total Environ. 659, 851–861. doi: 10.1016/j.scitotenv.2018.12.257
Yan, W. M. (2017). Synergic relation between plantgrowth and soil water and evaluation of soil water availability on the loess plateau Northwest A&F University Available at: https://kns.cnki.net/KCMS/detail/detail.aspx?dbname=CDFDLAST2017&filename=1017100365.nh.
Yin, D. Y., Ma, L., Ju, J. J., Zhao, S. P., Yu, Y., Tan, L. H., et al. (2017). Effect of large photovoltaic power station on microclimate of desert region in Gonghe basin. Bull. Soil Water Conserv. 37, 15–21. doi: 10.13961/j.cnki.stbctb.2017.03.003
Yu, G. R., Xu, X. L., and Wang, Q. F. (2020). Progress of the effects of global changes on the resource and environment carrying capacity of the ecological fragile areas. Chin. Basic Sci. 22, 16–20. doi: 10.3969/j.issn.1009–2412.2020.05.003
Zhai, B., Dang, X. H., Chen, X., Liu, X. J., and Yang, S. R. (2020). Difference regularity of precipitation redistribution and soil water evapotranspiration in photovoltaic panels in typical steppe areas of Inner Mongolia. J. Chin. Agric. Univ. 25, 144–155. doi: 10.11841/j.issn.1007-4333.2020.09.15
Zhai, B., Gao, Y., Dang, X. H., Chen, X., Cheng, B., Liu, X. J., et al. (2018). Effects of photovoltaic panels on the characteristics and diversity of Leymus chinensis community. Chin. J. Ecol. 37, 2237–2243. doi: 10.13292/j.1000–4890.201808.029
Zhang, Z. P., Shang, W., Wang, Q., Fu, G. Q., Zhang, W. X., and Wang, X. (2020). Biodiversity of herbaceous species under large photovoltaic power stations in desert region of Hexi corridor. J. Northwest. For. Univ. 35, 190–196. doi: 10.3969/j.issn.1001–7461.2020.02.28
Zhao, P. Y. (2016). Effects of photovoltaic panels on surface soil particles and microclimate Inner Mongolia Agricultural University Available at: https://kns.cnki.net/KCMS/detail/detail.aspx?dbname=CMFD201701&filename=1016249431.nh.
Zhou, L., Tian, Y., Roy, S. B., Thorncroft, C., and Hu, Y. (2012). Impacts of wind farms on land surface temperature. Nat. Clim. Chang. 2, 539–543. doi: 10.1038/nclimate1505
Zhou, M. R., and Wang, X. J. (2019). Influence of photovoltaic power station engineering on soil and vegetation: taking the Gobi Desert area in the Hexi corridor of Gansu as an example. J. Soil Water Conserv. 17, 132–138. doi: 10.16843/j.sswc.2019.02.016
Keywords: comprehensive assessment, ecosystem types, microclimatic factors, vegetation characteristics, soil environment
Citation: Zhang Y, Tian Z, Liu B, Chen S and Wu J (2023) Effects of photovoltaic power station construction on terrestrial ecosystems: A meta-analysis. Front. Ecol. Evol. 11:1151182. doi: 10.3389/fevo.2023.1151182
Edited by:
Hui Zhang, Hainan University, ChinaCopyright © 2023 Zhang, Tian, Liu, Chen and Wu. This is an open-access article distributed under the terms of the Creative Commons Attribution License (CC BY). The use, distribution or reproduction in other forums is permitted, provided the original author(s) and the copyright owner(s) are credited and that the original publication in this journal is cited, in accordance with accepted academic practice. No use, distribution or reproduction is permitted which does not comply with these terms.
*Correspondence: Shengyun Chen, c3ljaGVuQGx6Yi5hYy5jbg==; Jihua Wu, d2poQGx6dS5lZHUuY24=