The contribution of seedbank to the green roof plant community dynamics analogous to semi-natural grasslands
- 1Biodiversity and Landscape, TERRA Research Center, Gembloux Agro-Bio Tech, University of Liège, Gembloux, Belgium
- 2Institut Méditerranéen de Biodiversité et d’Ecologie (IMBE), UMR Avignon University, CNRS, Aix-Marseille University, IRD, Avignon, France
Extensive green roofs have been shown to support native biodiversity and plant communities that are analogous to natural or semi-natural habitats such as grasslands. However, little is known about the role of soil seedbanks in the dynamic of extensive green roof plant communities. The purpose of this study was to analyze the seedbank that developed after 4 years of an extensive green roof analog to dry grassland plant community, seeded with 29 species. We aimed to understand the contribution of seedbank to the resilience of vegetation to harsh conditions of the roof and to colonization by surrounding spontaneous species. We monitored the plant species cover in 36 plots during 4 years in June (between 2018 and 2021), and sampled the seedbank in February 2021. Our results showed that the soil seedbank was dominated by transient spontaneous ruderals species, while the standing vegetation was still dominated by seeded grassland species. We found that seeded grassland species had poor seedbank stock, similar to their natural environments. The similarity index between the standing vegetation and the seedbank increased over time, and we measured a significant correlation between dominant species cover and their seedbank density. Spontaneous species cover was not correlated to the proportion of soil not colonized by seeded species cover, indicating that gaps in vegetation did not influence the development of spontaneous species. Our findings highlight the importance of seedbank in the dynamic of green roof vegetation and demonstrate that analogous habitat species exhibit similar behavior as in their natural environments.
1. Introduction
Extensive green roofs (EGR) are a type of urban ecosystem that offer a range of ecosystem services (Oberndorfer et al., 2007; Kowarik, 2011). One of the ecosystem services provided by EGRs is the support of urban native biodiversity, through the implementation of diversified native plant communities based on the analogous habitat hypothesis (Lundholm and Richardson, 2010; Rivière et al., 2022). The analogous habitats hypothesis proposes that species which are adapted to similar abiotic conditions in natural habitats can also be successful on green roofs (Lundholm, 2006) and can serve as a starting point for selecting species. Green roofs provide solutions to develop urban grassland and herbaceous ecosystem networks in cities (Sutton et al., 2012; Williams et al., 2014; Thuring and Grant, 2015). However, harsh environmental conditions on extensive green roofs (e.g., high level of solar radiation, shallow substrate, poor water retention capacity) associated with future climate evolution can threaten the success of these native plant communities, and associated ecosystem services, when the functioning and dynamic of EGR ecosystems are not well-understood.
Green roof plant communities are dynamic. When initiated by seeding on bare substrate, the EGR plant community is marked by changes in plant composition over time as this situation relates to primary ecological succession conditions (Brown and Lundholm, 2015). The seeded plant community evolves through time in accordance to seeded species response to environmental conditions at the roof scale: roof height, slope, exposure, substrate depth and composition (Madre et al., 2014; Brown and Lundholm, 2015). EGR are also characterized by local microenvironmental variations impacting the composition and dynamic of the plant community (Heim and Lundholm, 2014; Roulston et al., 2020; Bradbury, 2021; Rivière et al., 2022). In addition to the response of seeded species to environmental conditions, a key driver of EGR plant community evolution is spontaneous plant colonization with non-seeded plant species (Thuring and Dunnett, 2019; Vanstockem et al., 2019). Weedy or unwanted species colonizing spontaneously EGR are identified as a limit to EGR acceptance and maintenance (Vanstockem et al., 2019). Spontaneous colonization by plant species is often linked to presence of bare soil or gaps in the vegetation cover due to the extreme abiotic growing conditions on EGR (Köhler, 2006; Sutton and Lambrinos, 2015).
Soil seedbank is a key component of plant community dynamics (Thompson et al., 1997; Vandvik et al., 2016). It influences the vegetation dynamic in the short and long term (Basto et al., 2018). The seedbank can provide information on species that may have formerly existed in the vegetation, species that represent the pool for the vegetation succession, and, represents the source of regenerative potential for the restoration of altered plant communities. Seedbanks on EGR [being biodiversity reservoirs (Vandvik et al., 2016)] may be crucial as an insurance for maintaining plant communities during periods of extreme conditions (e.g., extreme drought or frost) and can potentially offer a basis for restoration for vegetation gaps. Despite its importance in understanding vegetation response to extreme EGR conditions, the study of seedbank from extensive green roof (Vanstockem et al., 2018) or even urban areas (Albrecht et al., 2011) has received little attention. In an explorative study of seedbanks on 109 EGRs in temperate Europe, Vanstockem et al. (2018) confirm that EGRs do develop seedbanks, dominated by weedy plant species and exhibiting a strong discrepancy with vegetation composition.
To promote native biodiversity, EGR should be considered analogous to natural habitats (Lundholm, 2006). The harsh environmental constraints of EGR are similar to abiotic conditions encountered on species rich dry grasslands and rocky environments in temperate Western Europe (high solar radiation, low soil depth (<20 cm) and high variability in soil water retention capacity). Western Europeans dry grasslands are composed of isolated climax herbaceous habitats on most harsher environmental conditions and, for the largest surfaces, seral semi-natural habitats originating from centuries of extensive grazing and mowing. Rivière et al. (2022), demonstrated experimentally that extensive green roofs can act as analogous habitats for native flora of European dry calcareous grasslands (71% of native seeded grassland species established on an experimental green roof). The most successful species were common dry grassland species such as grass species, succulent species (sedum sp.), Echium vulgare L. and Papaver argemone L. However, success of native grassland plant species, plant community composition and structure were demonstrated to vary through time and to depend on microenvironmental conditions at the roof scale, with large surface of bare soils left after 3 years in the most constrained conditions (low substrate depth and high solar exposition). Decrease in plant cover was also measured in years with severe drought. The contribution of spontaneous ruderal species to the plant communities increased over time. Studies of seedbank in natural situations have shown that calcareous grassland species are mainly perennial stress tolerant species presenting mostly transient seedbank that stay viable in the soil for a very limited period of time and/or are absent from the seedbank (Dutoit and Alard, 1996; Willems and Bik, 1998; Bisteau and Mahy, 2005). A lack of well-developed permanent seedbank of target seeded species can be a threat for long term maintenance of analogous dry grassland vegetation of EGR facing colonization by spontaneous ruderal species.
In this study, we analyzed the seedbank contribution to the plant community of an extensive green roof analog to dry calcareous grassland after 4 years of development in order to understand the potential contribution of seedbank to the resilience of vegetation to harsh EGR conditions and to colonization by spontaneous species. The specific questions asked are: (i) what are the main seedbank characteristics and composition of an analogous urban grassland developed on an extensive green roof, (ii) what is the level of similarity between seedbank and vegetation, (iii) how is the seedbank and abundance of spontaneous species related to vegetation gap and microenvironmental variations on EGR.
2. Materials and methods
2.1. Study site and experimental design
The studied green roof is located on top of the TERRA Research Center of Gembloux Agro-Bio-Tech, Belgium (50°33′48″ N 4°41′52″ E). According to Köppen (1923) climate classification, the climate of the region is oceanic temperate (Cfb) (Service Fédéral Belge, 2019).
In October 2017, the EGR was installed with a commercial substrate for EGR: lightweight substrate Zinco© based on a mix of recycled tiles, bricks and ceramic. The roof included nine parcels ranging in size from 14 to 57 m2 with two substrate depths (6 and 11 cm) and an East-West orientation. The roof is bordered on three sides by walls (North, East, and South), resulting in global disparities in sun exposure across parcels. Skylights installed between parcels create an additional fine-scale shade gradient (Figure 1). Three classes of sun exposure (maximum sun exposure on a sunny summer day) were defined: low (3–6 h), Medium (6–9 h), and High (9–12 h). The two parameters of substrate depth and sun exposure were noted: D1 (6 cm depth), D2 (11 cm depth), S1 (low sun exposure), S2 (medium sun exposure), and S3 (high sun exposure) (Rivière et al., 2022).
A mix of 29 native species from Belgian dry calcareous grasslands were selected based on their presence along the soil depth gradient in Belgian dry grasslands (Piqueray et al., 2007; Table 1). Calcareous grasslands are not present in the ecological landscape surrounding the experimental green roof but 12 seeded species have been recorded in 2 km2 around it (Delescaille and Delaite, 2011). Three native Belgian Sedum species were chosen because they are succulent plants already commonly used on ExGR and adapted to harsh conditions (Butler and Orians, 2009). The species were seeded uniformly across the parcels on 10th November 2017, 830 seeds/species/m2 for grass species and 110 seeds/species/m2 for other species. During the research period (2017–2021), no overseeding, irrigation, fertilizers or mowing were applied. Rivière et al. (2022) studied the vegetation development according to substrate depth, sun exposure and changes over time, and showed a strong effect of time since sowing associated with the effects of these microenvironmental variation on plant community composition, plant cover and specific richness.
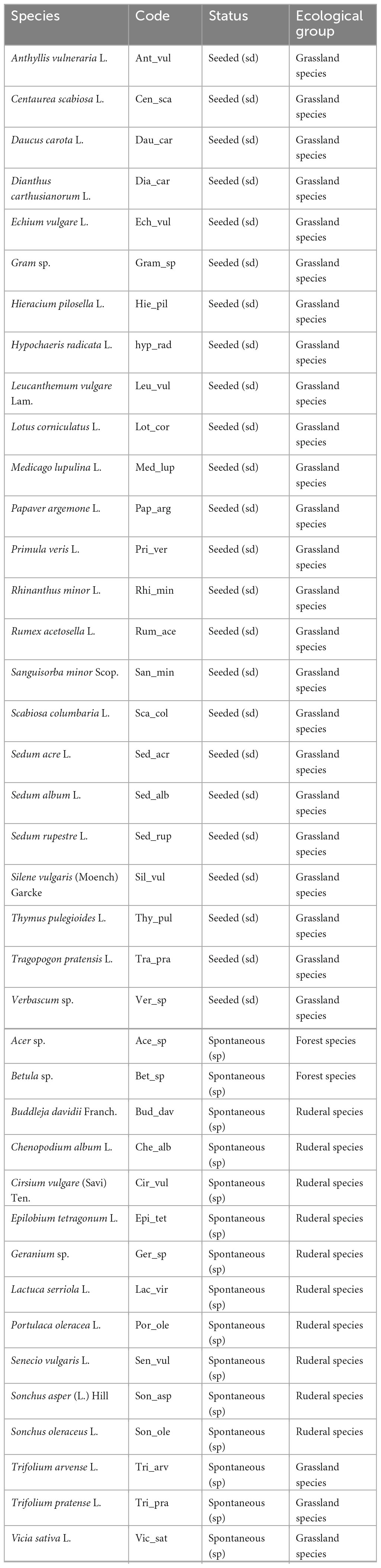
Table 1. Seeded or spontaneous species observed in 2018, 2019, 2020, 2021 or in the seedbank, the code used in figures and their status.
2.2. Data collection
Thirty-six permanent 1 m2 plots were installed on the nine parcels reflecting the variation of substrate depth and sun exposure (Figure 1). Plant species covers were visually measured in these 36 plots in June 2018, 2019, 2020 and 2021, at the peak of vegetative development (Rivière et al., 2022).
The seedbank was sampled in February 2021, before spring germination in order to collect seeds of both transient and persistent seedbanks under Atlantic and Continental climates, following Bekker et al. (1998). In each of the 36 plots, a sample of 10 bulked soil cores (2.5 cm diameter) was collected. Cores were divided in two layers for D1 6 cm deep parcels (layer 1: 0–3 cm and layer 2: 3–6 cm) and three layers for D2 11cm deep parcels (layer 1: 0–3 cm, layer 2: 3–6 cm and layer 3: 6–11 cm). A total of 88 samples were obtained. To extract the seedbank, the dried samples were sieved at 3.66 mm in order to remove roots, vegetative parts and stones. The sieved fractions were sown in 40 cm × 28 cm × 6.5 cm seedling trays with a 3 cm Argex marble and 1 cm organic matter (La plaine Chassart©). The trays were placed in homogeneous greenhouse conditions (22°C, 76% RH) and watered regularly by capillarity. The number of seedlings per species in each tray was counted at weekly intervals and removed after identification; monitoring continued until germination ceased after 15 weeks. The seedlings of the five seeded Poaceae seedlings could not be distinguished and were classified as “grass sp.”
LEDA database (Kleyer et al., 2008) was used to determine seed mass and seed lifetime index (SLI). For seed mass, only the mean “one-seeded generative dispersule” was considered per species. A mean SLI was calculated per species based on their persistence in the seedbank: 0 (all data are transient), 1 (all data are persistent). Seedbank can be transient if the seeds are present in the seedbank less than 1 year; short-term persistent between 1 and 5 years; or long-term persistent more than 5 years. In general, long-term seeds are found in both the deepest and shallowest levels of the substrate, whereas short-term seeds are exclusively found in the shallower layer of the substrate (Christoffoleti and Caetano, 1998).
The status of each species was determined by whether they were seeded (seeded, sd) or not (spontaneous, sp). Spontaneous species represent all unseeded species, annual or perennial, native or alien. The taxonomic reference was the “Nouvelle flore de la Belgique” (Lambinon et al., 2012). Species were classified in three ecological groups according to their natural habitat: grassland species, ruderal species and forest species (Lambinon et al., 2012; Table 1).
2.3. Data analyses
Similarity in species composition (presence/absence) between the initial seeding mix, species composition in vegetation in 2018–2021 and species composition in the 2021 seedbank was assessed with (1) the similarity index of Sokal and Michener (Perrier et al., 2003), and, (2) a Principal Component Analysis (PCA) (“princomp,” stats) performed on all plots. For each species, a Pearson correlation was calculated (“cor,” stats) to identify species that correlated most with the axes of the PCA.
The relationship between the most abundant species in the vegetation and the most abundant species in the seedbank (found in at least half of the plots) was determined by Pearson correlation tests (“cor,” stats) to establish if species cover was correlated to its seedbank density. To test if grassland vegetation development influenced establishment of spontaneous species by pre-empting bare soils surfaces (Vanstockem et al., 2018), we tested the regression between the spontaneous species total cover and the proportion of soil not colonized by grassland species in 2021 (100%–%total seeded species cover in 2021) with a linear regression (“lm,” cor).
To test whether microenvironmental condition (depths: D1, D2 and sun exposure: S1, S2, S3) variations on the experimental EGR influenced similarly plant community and seedbank variations, we performed independent Principal Coordinate Analysis (PCoA) on (1) cover of species in the vegetation, (2) seed density of species in seedbank, in all plots. Ordispider were used to depict the relationships between microenvironmental conditions and position of plots in the factorial space.
Data analyses were performed in R 4.0.2 (R Core Team, 2022).
3. Results
3.1. Seedbank characteristics
In 2021, 4 years after the creation of the green roof, the seedbank included 18 species (nine seeded species and nine spontaneous species, grass species considered as a single taxon due to difficulties identify seedlings) with an average of 2.5 ± 1.1 species and 120 ± 172 seeds per square meter plot. There was no seedbank observed in three plots. Seed density decreased with substrate depth with 42.8% of seed located at 0–3 cm depth, 34.7% at the 3–6 cm depth, and 22.5% at the 6–11 cm (Figure 2).
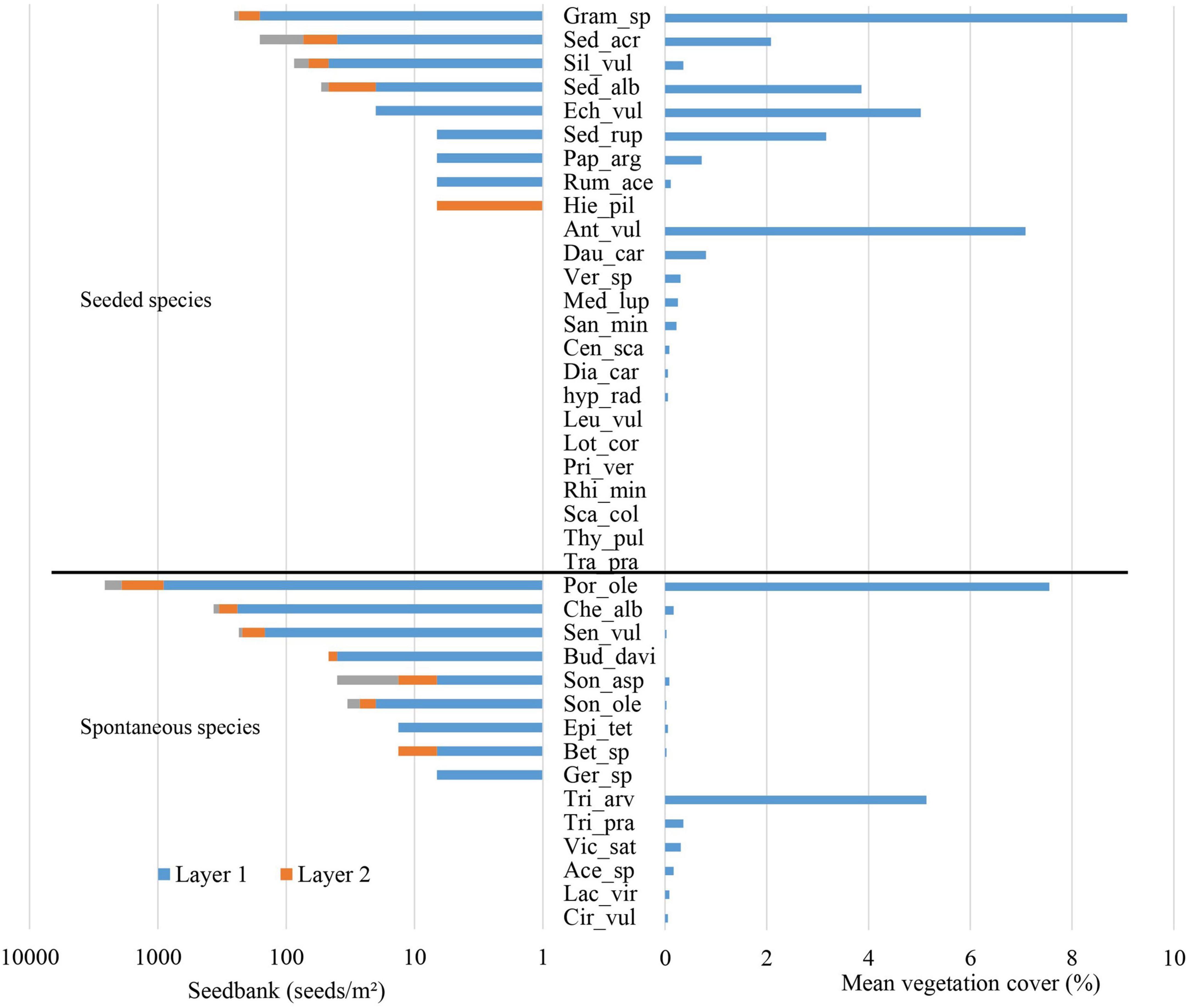
Figure 2. Seedbank density (seeds/m2) and mean vegetation cover of 2021 (%) for seeded species and spontaneous species (see Table 1 for species name).
The standing vegetation included 29 species (16 seeded species and 13 spontaneous species, grass species considered as one taxon) with an average of 5.7 ± 1.8 species and 47.3% ± 39.3% of cover per square meter plot (Figure 2). There was no plot without vegetation cover.
The seedbank (mean SLI: 0.34 ± 0.13) and the vegetation (mean SLI: 0.25 ± 0.16) were both composed of species with a transient seedbank. All species have a SLI of less than 0.5 except Chenopodium album L. (0.59) and Portulaca oleracea L. (0.68) both spontaneous species and present in the vegetation and in the seedbank.
3.2. Seedbank and vegetation similarity
The seedbank was dominated by seeds from spontaneous species (84.8%), while seeds from seeded species represent only 15.23%. In contrast, seeded species represent 70.4% of plant cover in the vegetation, while spontaneous species represented only 29.6%. The seedbank was dominated by the ruderal ecological group (84.4% of all seeds) followed by grassland ecological group (15.2%). In contrast, the plant cover was dominated by species from the grassland ecological group (82.6%), followed by species from ruderal ecological groups species (17.0%). Both the seedbank and the standing vegetation had low occurrence of species from forest ecological group (0.34 and 0.40%, respectively).
The seedbank was dominated at 65.6% of P. oleracea. While spontaneous species dominated the seedbank (P. oleracea, C.album and Senecio vulgaris L. at 65.5, 9.3, and 5.9%, respectively), they were seldom developed in the vegetation with cover less than 1% per species, except for P. oleracea (7.56%/m2) and Trifolium arvense L. (5.14%/m2), a cover similar to dominant seeded species (Figure 2).
Only nine out of 24 seeded species (considering all grasses as a single taxon) were detected in the seedbank with densities ranging from 6.7 to 254.4 seeds/m2, from which eight were also present in the vegetation. Grasses (254.4 seeds/m2, 9.08% cover), Sedum acre L. (160.7 seeds/m2, 2.08% cover), Silene vulgaris (Moench) Garcke (87.03 seeds/m2, 0.36% cover), Sedum album L. (53.6 seeds/m2, 3.9% cover), and Echium vulgare L. (20.08 seeds/m2, 5.03% cover) were the seeded species developing the densest seedbank. Only S. acre has a higher seedbank density than the initial seeding mix (110 seeds/m2). Eight seeded species present in the vegetation were not detected in the seedbank, including Anthyllis vulneraria L., one of the vegetation dominant species. Seven seeded species were not observed in the vegetation, nor in the seedbank (Figure 2).
The lowest similarity (Sokal and Michener index) in species composition was measured between the initial seeding mix and the seedbank after 4 years of vegetation development. Similarity between initial seeding mix composition and standing vegetation decreased over time from 0.75 to 0.50 while similarity between the vegetation and the seedbank increased over time from 0.5 to 0.61 (Figure 3). The vegetation differed each year a little more from the initial seeding and became more similar to the seedbank sampled in 2021.

Figure 3. Sokal and Michener index between 2017 seeding, 2018, 2019, 2020, and 2021 vegetation cover and 2021 seedbank.
The first and second axes of the PCA (Figure 4) based on the presence/absence of species in the initial seeding mix, the plant community in 1 m2 plots in 2018, 2019, 2020, and 2021 and the seedbank in 1 m2 plots in 2021 explained 61.8% of the global species composition variation (Comp1 = 54.1%–eigenvalue 1 = 2.7, Comp2 = 7.7%–eigenvalue 2 = 0.4). Axis 1 strongly differentiated the initial seeding mix from standing vegetation and seedbank. Axis 2 indicated a trend for plant community to become more similar to seedbank over years. The correlation circle indicated that the first axis was correlated with the presence of seeded species. The plant community of 2018, 2019, 2020, and 2021 and the seedbank were differentiated from the initial seeded community with the presence of spontaneous species and with the absence of seeded species that never germinated over time (Hieracium pilosella L., Leucanthemum vulgare Lam., Hypochaeris radicata L., Primula veris L., Thymus pulegioides L., and Tragopogon pratensis L.).
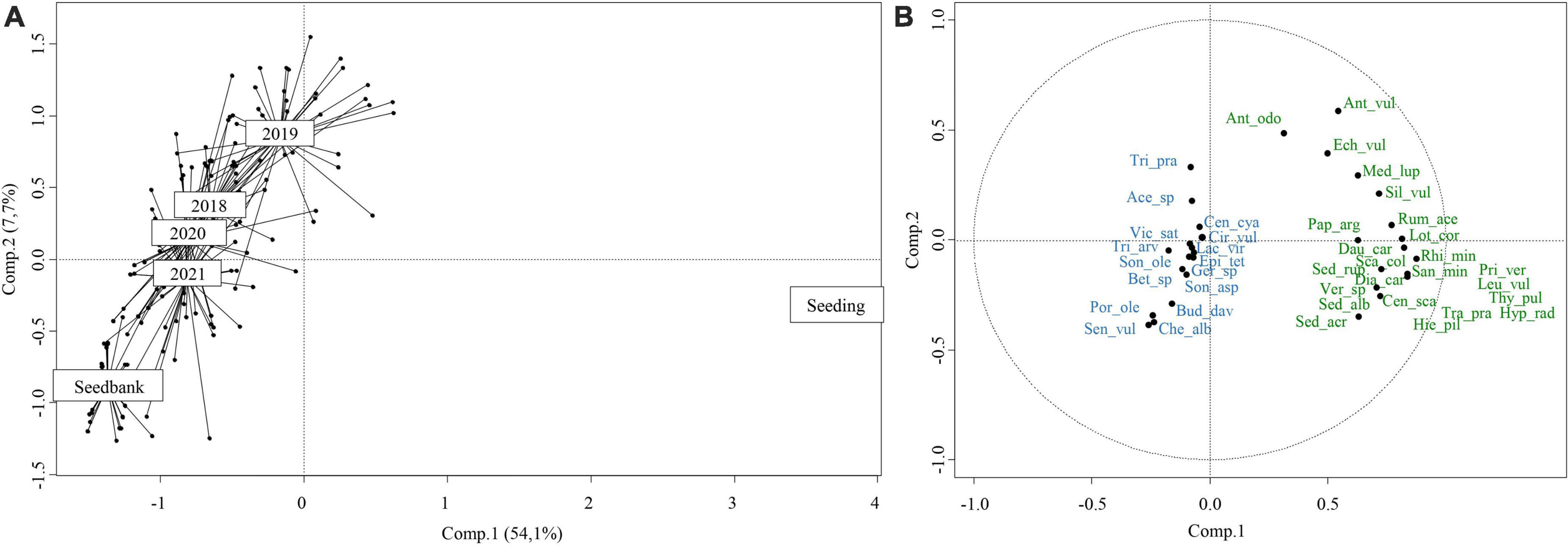
Figure 4. Principal Component Analysis (PCA) based on presence/absence species composition for initial seeding, 2018, 2019, 2020, and 2021 vegetation cover and 2021 seedbank. (A) Ordi spiders pool by year. (B) Correlation circle of species, blue species: spontaneous species, green species: seeded species.
3.3. Influence of plant communities on seedbank
Correlation between plant cover and seed density at 1 m2 plot scale was tested for species found in the seedbank and vegetation cover in at least half of plots. Pearson correlation tests indicated a significant correlation and a high correlation for grass species (P < 0.001–cor = 0.31) and P. oleracea (P < 0.001–cor = 0.57). The Pearson correlation test was high with an almost significant P value for E. vulgare (P = 0.07—cor = 0.32), S. acre (P = 0.07—cor = 0.75), and C. album (P = 0.08—cor = 0.34). The seedbank density and the vegetation cover of S. vulgaris were not significantly correlated (P = 0.50—cor = −0.12).
The proportion of soil not colonized by seeded species (100%–%total seeded species cover in 2021) had no significant effect on the spontaneous species cover (linear regression, P = 0.93, R2 = −0.03).
3.4. Influence of microenvironmental variation on plant communities and seedbank
When examining the effect of microenvironmental variation on plant community composition of standing vegetation in 2021 (taking into account plant species cover), the first and second axes of the PCoA (Figure 5A explained 25.2% of the global variation (Dim1 = 13.7%–eigenvalue = 1.9, Dim2 = 11.5%–eigenvalue = 1.6). Position of ordispider gravity centre on first axis discriminated plant communities in plots with shallow substrate depth (D1: 6 cm) (negative coordinates) from plant communities on deeper substrate (D2: 12 cm) (positive coordinates). Position of ordispider gravity centre on second axis discriminated plant communities in plots with high sun exposure S3 (negative coordinates) from plant communities with medium to low sun exposure S1 and S2 (positive coordinates).
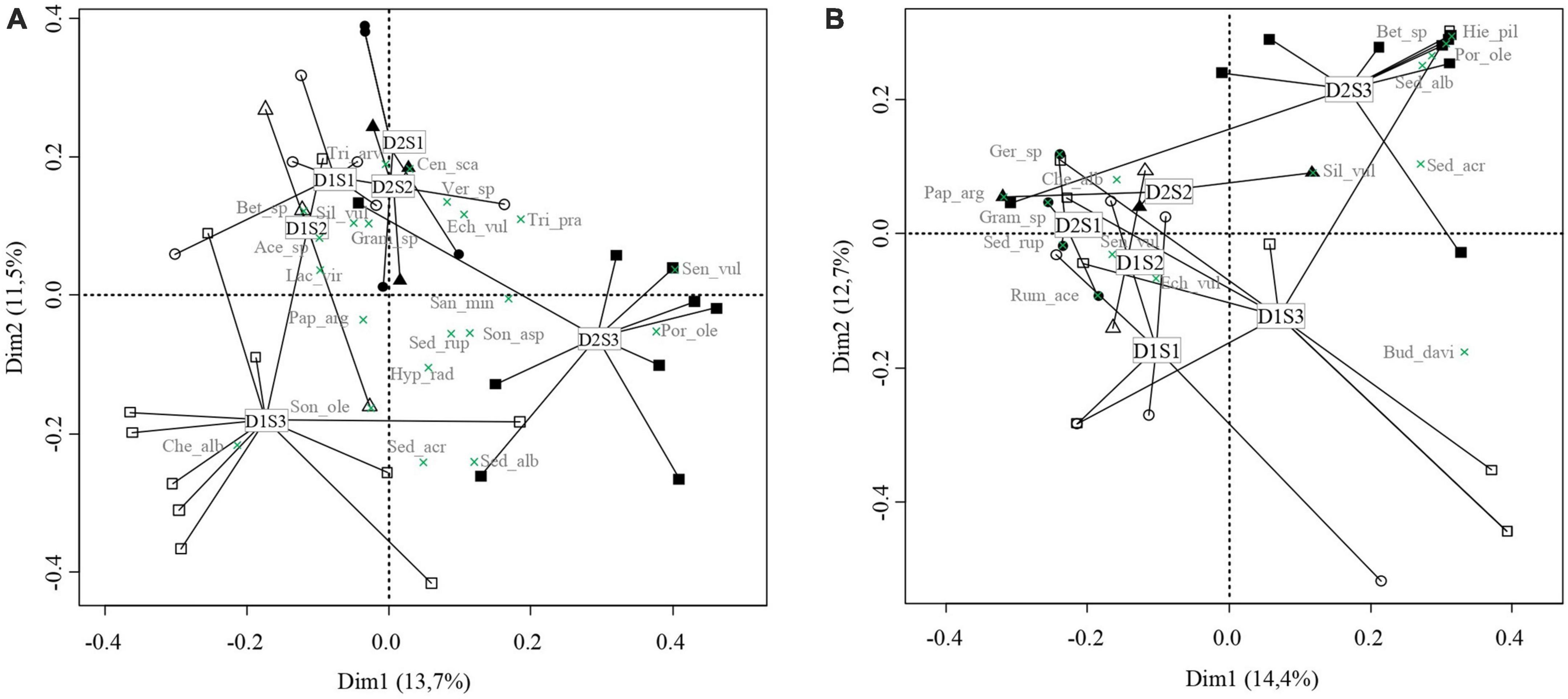
Figure 5. Principal Coordinate Analysis (PCoA) based on (A) plant composition of standing vegetation in 2021 and on (B) seedbank composition. Ordi spiders are pooled according to microenvironmental conditions. Symbols represent sun exposure modalities (circle = S1: low sun exposure, triangle = S2: medium sun exposure, and square = S3: high sun exposure) and substrate depth modalities (white = D1: shallow depth 6 cm, black = D2: deep depth 11 cm). Species are indicated with green crosses.
When examining the effect of microenvironmental variation on seedbank composition (taking into account species seed density), the first and second axes of the PCoA (Figure 5B explained 27.1% of the global variation (Dim1 = 14.4%–eigenvalue = 2.0, Dim2 = 12.7%–eigenvalue = 1.7). Position of ordispider gravity centre on first axis discriminated seedbank composition in plots with high sun exposure S3 (positive coordinates), characterized by the presence of P. oleracea, Buddleja davidii Franch., and Betula sp, from seedbank composition in plots with medium to low sun exposure S1 and S2 (negative coordinates), characterized by the presence of C. album, Geranium sp. and grass sp. Position of ordispider gravity centre on second axis discriminated seedbank composition in plots with shallow substrate depth (D1: 6 cm) (negative coordinates) from seedbank composition on deep substrate depth (D2: 12 cm) (positive coordinates).
4. Discussion
Increasing attention has been paid to plant community dynamics on extensive green roof in ecological literature (Roulston et al., 2020; Bradbury, 2021; Rivière et al., 2022). Change in plant community over time is key to EGR design and their acceptability (Thuring and Dunnett, 2019; Vanstockem et al., 2019). It is also a major determinant of EGR capacity to sustain native biodiversity through development of analog habitat (Lundholm, 2006; Rivière et al., 2022). In this study, we examined the development of seedbank in relation to the installation of an analogous dry grassland on an experimental EGR. In contrast to our recent knowledge increase on above ground vegetation patterns and processes on green roofs (Madre et al., 2014; Brown and Lundholm, 2015), seedbank development and its role for green roof vegetation has been seldom explored directly (Vanstockem et al., 2018). The study of seedbank is important to understand its contribution to the resilience of vegetation to harsh EGR conditions. Specifically, a lack of well-developed permanent seedbank of target seeded species can be a threat for long term maintenance of analogous dry grassland vegetation of EGR facing colonization by spontaneous ruderal species.
Our study of the standing plant community evolution on an experimental EGR indicates that green roofs work as an analogous habitat supporting seeded species from native dry grasslands (Rivière et al., 2022). However, the evolution over time of plant community on the EGR diverges from the initial seeding by the absence of a set of seeded grassland species and the increase in spontaneous species cover over time. The species richness of spontaneous species tends to increase to the same level as species richness of seeded species (12 vs. 16) in 4 years. Development of a high richness of spontaneous species on experimental green roofs over a short time period has been repeatedly reported. For example, Dunnett et al. (2008) in Sheffield, UK, identified 35 colonizing species for 15 planted species, Olly et al. (2011) on experimental plots in Birmingham, UK, identified 29 spontaneous species for 17 seeded species and Bates et al. (2013) identified 31 and 37 colonizing species on two brown roofs in the same city. All the spontaneous species observed on the studied EGR are anemochorous except C. album, Geranium sp, P. oleracea and Vicia sativa L. Kleyer et al. (2008). Those latter species may have been imported to the roof by birds or by people visiting the roof. This suggests that most spontaneous species reach our EGR by wind, a pattern reported by Madre et al. (2014). We cannot exclude that some species might have been introduced via seed-contaminated substrate, however, we think that this is negligible as there was very low spontaneous species development on the first year of the experience.
Using a “space for time” approach, Vanstockem et al. (2018) demonstrated the presence of seedbanks on established extensive green roofs aged of 19 years. In the current study, for the first time, we experimentally demonstrate the accumulation of a seedbank on an experimental green roof over a short period of time (4 years) from its settlement from bare substrate. A total of 18 species were found in the seedbank. Mean seedbank SLI indicated a transient global seedbank, but seed accumulation in the deepest substrate layers suggested ongoing constitution of a permanent seedbank, mainly due to spontaneous species with permanent seedbanks, such as C. album and P. oleracea.
Despite a set of common species between the seedbank and the vegetation, and a trend for plant community to become more similar to the seedbank through time, in depth comparison reveal divergence between both, with spontaneous ruderal species dominating the seedbank (mainly P. oleracea) when considering seedbank density, and seeded grassland species dominating the vegetation when considering the species cover. High dissimilarity between the composition of species of the seedbank and the vegetation has been measured in established EGR and the majority of species in EGR seedbanks are spontaneous colonizers (Vanstockem et al., 2018).
Only a small proportion (9 out of 24) of seeded grassland species are detected in the seedbank with mostly lower seed densities as compared to the original seeding, indicating that a seed reserve has not accumulated over time. Limited development of grassland seedbank is due to the non-development of some seeded species (eight species) and to the absence of seedbank for a set of species (eight) present in the vegetation.
Comparison with the seedbank of (semi-)natural systems with comparable environmental characteristics [habitat template sensu Lundholm (2006)], such as calcareous grasslands on shallow soils, can provide useful insights on EGR seedbanks. Many authors showed that species dominating calcareous grassland vegetation were mostly absent from the seedbank, and when present were not abundant (Davies and Waite, 1998; Bisteau and Mahy, 2005; Bossuyt et al., 2006). Almost all calcareous grassland species produce short-lived (transient) seeds that stay viable in the soil for a limited time period. Grassland species invest more resources in individual survival than in seeds production (Eriksson and Eriksson, 1997).
Spontaneous species observed on the experimental EGR are mainly ruderal and for the majority (60%) have accumulated a seedbank despite low cover in the above ground vegetation, to the exception of P. oleracea, which was one of the dominant species of the vegetation. Vanstockem et al. (2018) also reported a dominance of ruderal species in seedbank of established EGR. Ruderal species allocate more resources to producing a large number of seeds rather than developing their cover (Grime, 1988; Šerá and Šerý, 2004). Ruderal spontaneous species are also present in seedbank composition of natural dry grassland but have a limited development in the vegetation (Bisteau and Mahy, 2005). In contrast to Vanstockem et al. (2018) who observed limited presence in the seedbank species pool in comparison to the vegetation, the two most abundant phanerophyte species in the seedbank of our EGR (Buddleja davidii Franch., Betula sp) were almost absent from the vegetation, indicating that seed rain may be an agent responsible for seed accumulation in the seedbank (Davies and Waite, 1998). The presence of B. davidii in the seed bank may pose a potential problem for future vegetation development on the roof as it has a very high invasive potential in disturbed, open and dry environments (Tallent-Halsell and Watt, 2009; Monty et al., 2019), even though it is currently absent from the established vegetation in permanent plots.
We demonstrate that after 4 years of development, both standing vegetation and seedbank species composition were influenced by the variation of environmental factors: substrate depth and sun exposure. The substrate depth is known to be an important factor structuring green roof vegetation both for seeded/planted species and for colonizing species (Durhman et al., 2007; Köhler and Poll, 2010; Madre et al., 2014). In more stressful environment on our EGR (low substrate depth, high sun exposure) vegetation cover was limited with large proportion of bare substrate (Rivière et al., 2022). Vegetation gaps are an important component of seeded EGR, during the vegetation development phase and along the life-cycle of EGR when extreme environmental conditions and abiotic conditions can locally affect vegetation (Vanstockem et al., 2019). Colonizing plants can develop on green roofs because there is available space due to changes in the planted populations, with a decrease in some of the planted species (Dunnett et al., 2008). Gaps in vegetation can impact seedbank characteristics and the development of vegetation by providing an opportunity for colonization by spontaneous and ruderal species (Vanstockem et al., 2018). However, in our study, we do not find a relationship between space left vacant by grassland vegetation and spontaneous species development. This can result from extreme environmental conditions affecting in the same way the two categories of species and preventing ruderal spontaneous species to germinate and colonize bare substrate. This lack of gap colonization potential may be reinforced by the fact that seedbank density was correlated to dominant species cover in the vegetation, indicating, that seedbank accumulates in the vicinity of mature individuals growing in the most favorable environment of the green roof. Also, the role of the seedbank in gap regeneration depends on the severity of disturbance which should relocated buried seed to the surface. In undisturbed vegetation, seedlings very rarely originate from the seedbank (Milberg and Persson, 1994; Kalamees and Zobel, 2002).
In conclusion, in contrast to Vandvik et al. (2016) and Vanstockem et al. (2019) suggestion that seedbanks on EGR could serve as a diversity insurance during periods of extreme conditions (e.g., extreme drought or frost) and can therefore potentially offer a basis for restoration of vegetation gaps, we experimentally measured that the maintenance of a diversified analogous grassland on an extensive green roof cannot rely mainly on an accumulated seedbank. Grassland seeded species did not contribute significantly to the seedbank. Seeded community is therefore unstable and vulnerable to sudden and profound changes under extreme hydric stress conditions. In this case, standing vegetation will be dominated by spontaneous species and the habitat developed will no longer be analogous to dry grassland. Maintenance of target grassland vegetation, in response to disturbance by extreme events, will depend mainly on the perennial habit of grassland species and their high tolerance to stress. Punctual seeding intervention may be needed in case of extreme climatic events affecting grassland species survival.
Data availability statement
The raw data supporting the conclusions of this article will be made available by the authors, without undue reservation.
Author contributions
GM and LR carried out the conception, design of the study, and wrote the first draft of the manuscript. LR and AS carried out the experiment and performed the statistical analysis. All authors contributed to the conception of the manuscript, manuscript revision, read, and approved the submitted version.
Funding
This work was funded by ARC CityRoof (University of Liège).
Acknowledgments
The authors acknowledge the ARC CityRoof project for funding and UCL for providing access to their greenhouses.
Conflict of interest
The authors declare that the research was conducted in the absence of any commercial or financial relationships that could be construed as a potential conflict of interest.
Publisher’s note
All claims expressed in this article are solely those of the authors and do not necessarily represent those of their affiliated organizations, or those of the publisher, the editors and the reviewers. Any product that may be evaluated in this article, or claim that may be made by its manufacturer, is not guaranteed or endorsed by the publisher.
References
Albrecht, H., Elisabeth, E., Thomas, L., and Clara, T. (2011). The soil seed bank and its relationship to the established vegetation in urban wastelands. Landsc. Urban Plan. 100, 87–97. doi: 10.1016/j.landurbplan.2010.11.011
Basto, S., Thompson, K., Grime, J., Fridley, J., Calhim, S., Askew, A., et al. (2018). Severe effects of long-term drought on calcareous grassland seed banks. NPJ Clim. Atmos. Sci. 1:1. doi: 10.1038/s41612-017-0007-3
Bates, A., Sadler, J. P., and Mackay, R. (2013). Vegetation development over four years on two green roofs in the UK. Urban For. Urban Green. 12, 98–108. doi: 10.1016/j.ufug.2012.12.003
Bekker, R. M., Bakker, J. P., Grandin, U., Kalamees, R., Milberg, P., Poschlod, P., et al. (1998). Seed size, shape and vertical distribution in the soil: Indicators of seed longevity. Funct. Ecol. 12, 834–842. doi: 10.1046/j.1365-2435.1998.00252.x
Bisteau, E., and Mahy, G. (2005). Vegetation and seed bank in a calcareous grassland restored from a Pinus forest. Appl. Veg. Sci. 8, 167–174. doi: 10.1658/1402-2001
Bossuyt, B., Butaye, J., and Honnay, O. (2006). Seed bank composition of open and overgrown calcareous grassland soils—a case study from southern Belgium. J. Environ. Manag. 79, 364–371. doi: 10.1016/j.jenvman.2005.08.005
Bradbury, C. (2021). Functional difference in plant communities as a driver of green roof ecosystem services. Ph.D. thesis. Halifax, NS: Saint Mary University. Available online at: http://library2.smu.ca/xmlui/handle/01/29518
Brown, C., and Lundholm, J. (2015). Microclimate and substrate depth influence green roof plant community dynamics. Landsc. Urban Plann. 143, 134–142. doi: 10.1016/j.landurbplan.2015.07.009
Butler, C., and Orians, C. (2009). Session 3.3: Natives vs. non-natives, the debate on the merits of each continues. Conf. Proc. 1–14.
Christoffoleti, P. J., and Caetano, R. S. X. (1998). Soil seed banks. Sci. Agric. 55, 74–78. doi: 10.1590/S0103-90161998000500013
Davies, A., and Waite, S. (1998). The persistence of calcerous grassland species in the soil seed bank under developing and established scrub. Plant Ecol. 136, 27–39. doi: 10.1023/A:1009759227900
Delescaille, L.-M., and Delaite, S. (2011). Latlas floristique de wallonie: Où en est-on? Adoxa 68, 17–19.
Dunnett, N., Nagase, A., and Hallam, A. (2008). The dynamics of planted and colonising species on a green roof over six growing seasons 2001-2006: Influence of substrate depth. Urban Ecosyst. 11, 373–384. doi: 10.1007/s11252-007-0042-7
Durhman, A., Bradley Rowe, D., and Rugh, C. L. (2007). Effect of substrate depth on initial growth, coverage, and survival of 25 succulent green roof plant taxa. HortScience 42, 588–595. doi: 10.21273/HORTSCI.42.3.588
Dutoit, T., and Alard, D. (1996). “Chapitre second. Mécanisme d’une succession végétale secondaire en pelouse calcicole: Une approche historique,” in Dynamique et gestion des pelouses calcaires de haute-normandie, Hors collection, ed. P. Antilogus (Mont-Saint-Aignan: Presses universitaires de Rouen et du Havre), 91–108.
Eriksson, Å., and Eriksson, O. (1997). Seedling recruitment in semi-natural pastures: The effects of disturbance, seed size, phenology and seed bank. Nord. J. Bot. 17, 469–482. doi: 10.1111/j.1756-1051.1997.tb00344.x
Grime, J. P. (1988). “The C-S-R model of primary plant strategies — origins, implications and tests,” in Plant evolutionary biology, eds L. D. Gottlieb and S. K. Jain (Dordrecht: Springer), 371–393. doi: 10.1007/978-94-009-1207-6_14
Heim, A., and Lundholm, J. (2014). The effects of substrate depth heterogeneity on plant species coexistence on an extensive green roof. Ecol. Eng. 68, 184–188. doi: 10.1016/j.ecoleng.2014.03.023
Kalamees, R., and Zobel, M. (2002). The role of the seed bank in gap regeneration in a calcareous grassland community. Ecology 83, 1017–1025. doi: 10.2307/3071910
Kleyer, M., Bekker, R. M. I, Knevel, C., Bakker, J. P., Thompson, K., Sonnenschein, M., et al. (2008). The LEDA traitbase: A database of life-history traits of the northwest European flora. J. Ecol. 96, 1266–1274. doi: 10.1111/j.1365-2745.2008.01430.x
Köhler, M. (2006). Long-term vegetation research on two extensive green roofs in Berlin. Urban Habitats 4, 3–26.
Köhler, M., and Poll, P. H. (2010). Long-term performance of selected old berlin greenroofs in comparison to younger extensive greenroofs in Berlin. Ecol. Eng. 36, 722–729. doi: 10.1016/j.ecoleng.2009.12.019
Köppen, W. (1923). Die klimate der erde: Grundriss der klimakunde. Die klimate der erde. Berlin: Walter de Gruyter & Co. doi: 10.1515/9783111491530
Kowarik, I. (2011). Novel urban ecosystems, biodiversity, and conservation. Environ. Pollut. 159, 1974–1983. doi: 10.1016/j.envpol.2011.02.022
Lambinon, J., Delvosalle, L., and Duvigneaud, J. (2012). Nouvelle flore de la belgique, du grand-duché de luxembourg, du nord de la France et des régions voisines, 6 Edn. Meise: Editions du Patrimoine du Jardin Botanique National de Belgique.
Lundholm, J., and Richardson, P. J. (2010). MINI-REVIEW: Habitat analogues for reconciliation ecology in urban and industrial environments: Habitat analogues. J. Appl. Ecol. 47, 966–975. doi: 10.1111/j.1365-2664.2010.01857.x
Lundholm, J. T. (2006). Green roofs and facades: A habitat template approach. Urban Habitats 4, 87–101.
Madre, F., Vergnes, A., Machon, N., and Clergeau, P. (2014). Green roofs as habitats for wild plant species in urban landscapes: First insights from a large-scale sampling. Landsc. Urban Plan. 122, 100–107. doi: 10.1016/j.landurbplan.2013.11.012
Milberg, P., and Persson, T. S. (1994). Soil seed bank and species recruitment in road verge grassland vegetation. Ann. Bot. Fenn. 31, 155–162.
Monty, A., Jorion, A., Pitz, C., Géron, C., and Mahy, G. (2019). Alien invasive plants in Belgian limestone quarries. Biotechnol. Agron. Soc. Environ. 3, 160–164. doi: 10.25518/1780-4507.17984
Oberndorfer, E., Lundholm, J., Bass, B., Coffman, R. R., Doshi, H., Dunnett, N., et al. (2007). Green roofs as urban ecosystems: Ecological structures, functions, and services. Bioscience 57, 823–833. doi: 10.1641/B571005
Olly, L., Bates, A. J., Sadler, J. P., and Mackay, R. (2011). An initial experimental assessment of the influence of substrate depth on floral assemblage for extensive green roofs. Urban For. Urban Green. 10, 311–316. doi: 10.1016/j.ufug.2011.07.005
Perrier, X., Flori, A., and Bonnot, F. (2003). Data analysis methods. In genetic diversity of cultivated tropical plants, trans by P. Hamon, M. Seguin, X. Perrier, and J.C. Glaszmann. Enfield: Science Publishers, 43–76.
Piqueray, J., Bisteau, E., Bottin, G., and Mahy, G. (2007). Plant communities and species richness of the calcareous grasslands in southeast Belgium. Belgian J. Bot. 140, 157–173.
R Core Team (2022). Methodology reference. R: A language and environment for statistical computing. Vienna: R Foundation for Statistical Computing.
Rivière, L., Delruelle, A., Reniers, J., Boisson, S., and Mahy, G. (2022). Disentangling dynamics of green roof vegetation analogue to dry grassland over 3 years: Plant and substrate response to microenvironmental variations. J. Living Archit. 9, 1–17. doi: 10.46534/jliv.2022.09.02.001
Roulston, T., Heim, A., and Lundholm, J. (2020). Heterogeneous substrate depth supports greater functional diversity with comparable stormwater retention and substrate temperature services to sedum-dominant green roofs. J. Living Archit. 7, 19–39. doi: 10.46534/jliv.2020.07.02.019
Šerá, B., and Šerý, M. (2004). Number and weight of seeds and reproductive strategies of herbaceous plants. Folia Geobot. 39, 27–40. doi: 10.1007/BF02803262
Service Fédéral Belge (2019). Climat.be: Le climat en Belgique. Available online at: https://www.climat.be/fr-be/changements-climatiques/en-belgique/climat-en-belgique/ (accessed April 17, 2019).
Sutton, R., Harrington, J. A., Skabelund, L., MacDonagh, P., Coffman, R. R., and Koch, G. (2012). Prairie-based green roofs: Literature, templates, and analogs. J. Green Build. 7, 143–172. doi: 10.3992/jgb.7.1.143
Sutton, R., and Lambrinos, J. (2015). “Green roof ecosystems: Summary and synthesis,” in Green roof ecosystems ecological studies, ed. R. K. Sutton (Cham: Springer International Publishing), 423–440. doi: 10.1007/978-3-319-14983-7_17
Tallent-Halsell, N., and Watt, M. S. (2009). The invasive Buddleja davidii (butterfly bush). Bot. Rev. 75, 292–325. doi: 10.1007/s12229-009-9033-0
Thompson, K., Bakker, J. P., and Bekker, R. M. (1997). The soil seed banks of North West Europe: Methodology, density and longevity. Vol. 7. Seed science research 3. Cambridge: Cambridge University Press.
Thuring, C., and Dunnett, N. P. (2019). Persistence, loss and gain: Characterising mature green roof vegetation by functional composition. Landsc. Urban Plan. 185, 228–236. doi: 10.1016/j.landurbplan.2018.10.026
Thuring, C., and Grant, G. (2015). The biodiversity of temperate extensive green roofs: A review of research and practice. Isr. J. Ecol. Evol. 62, 44–57. doi: 10.1080/15659801.2015.1091190
Vandvik, V., Klanderud, K., Meineri, E., Måren, I. E., and Töpper, J. (2016). Seed banks are biodiversity reservoirs: Species–area relationships above versus below ground. Oikos 125, 218–228. doi: 10.1111/oik.02022
Vanstockem, J., Ceusters, C., Van Dyck, K., Somers, B., and Hermy, M. (2018). Is there more than meets the eye? Seed bank analysis of a typical novel ecosystem, the extensive green roof. Appl. Veg. Sci. 21, 419–430. doi: 10.1111/avsc.12383
Vanstockem, J., Somers, B., and Hermy, M. (2019). Weeds and gaps on extensive green roofs: Ecological insights and recommendations for design and maintenance. Urban For. Urban Green. 46:126484. doi: 10.1016/j.ufug.2019.126484
Willems, J. H., and Bik, L. P. M. (1998). Restoration of high species density in calcareous grassland: The role of seed rain and soil seed bank. Appl. Veg. Sci. 1, 91–100. doi: 10.2307/1479088
Keywords: plant dynamic, ruderal, spontaneous species, green infrastructure, seed persistence, urban ecology, substrate depth, sun exposure
Citation: Rivière L, Sellier A, Dutoit T, Vidaller C, Buisson E and Mahy G (2023) The contribution of seedbank to the green roof plant community dynamics analogous to semi-natural grasslands. Front. Ecol. Evol. 11:1152319. doi: 10.3389/fevo.2023.1152319
Received: 27 January 2023; Accepted: 17 May 2023;
Published: 01 June 2023.
Edited by:
Lilia L. Roa-Fuentes, Pontifical Javierian University, ColombiaReviewed by:
Maan Rokaya, Institute of Botany (ASCR), CzechiaJeremy Goodall, ARC Centre of Excellence for Electromaterials Science (ACES), Australia
Copyright © 2023 Rivière, Sellier, Dutoit, Vidaller, Buisson and Mahy. This is an open-access article distributed under the terms of the Creative Commons Attribution License (CC BY). The use, distribution or reproduction in other forums is permitted, provided the original author(s) and the copyright owner(s) are credited and that the original publication in this journal is cited, in accordance with accepted academic practice. No use, distribution or reproduction is permitted which does not comply with these terms.
*Correspondence: Lucie Rivière, lucie.rivere@uliege.be