- 1Julius Kühn Institute Federal Research Centre for Cultivated Plants, Institute for Ecological Chemistry, Plant Analysis and Stored Product Protection, Berlin, Germany
- 2Dahlem Centre of Plant Science, Institute of Biology, Applied Zoology/Animal Ecology, Freie Universität Berlin, Berlin, Germany
Interactions between stored product pest insects and their parasitoids are well known to be mediated by infochemicals. However, little knowledge is available about the extent to which parasitoid responses to host kairomones improve host search and parasitization success. Here, we tested whether the release of host-specific kairomones of the confused flour beetle, Tribolium confusum, improves host finding of the larval ectoparasitoid Holepyris sylvanidis. Previous studies revealed that volatiles from host larval feces [i.e., (E)-2-nonenal and 1-pentadecene] attract the parasitoid. To determine the most attractive concentration of these two key components, we conduced Y-tube olfactometer bioassays. Most female parasitoids were attracted to a mixture of (E)-2-nonenal and 1-pentadecene at the lowest concentration, while the highest concentration was repellent. Volatiles from host adults (methyl-p-benzoquinone and 4,8-dimethyldecanal) did not attract the parasitoid females at any of the concentrations tested. In flight cage experiments, we analyzed the host finding success of H. sylvanidis by offering host larvae in a Petri dish for 3 days (i) with additionally applied host larval kairomones in the most attractive concentration (test) or (ii) without additional kairomones (control). In test cages, the parasitoids removed a significantly higher number of paralyzed host larvae from the initial population within 48 h than in control cages. After 72 h, significantly more host larvae were parasitized in test cages than in the control. The offspring of parasitoids in test cages differed from the one in control cages by total numbers and especially the number of males. Significantly more parasitoids emerged from the parasitized host larvae in test cages, with enhanced male offspring. Our study demonstrates that the parasitoid’s response to host-associated volatiles can improve parasitization success but also affects the number of males in the parasitoids’ progeny.
1. Introduction
Biological control measures against insect pests have become increasingly important in agriculture in recent decades as knowledge grows about the side effects of conventional chemical pesticides on human health and environment (Cox, 2004; van Lenteren, 2012; van Lenteren et al., 2018). Biologically based approaches such as the use of entomophagous insects (e.g., parasitic wasps) and the application of semiochemicals (e.g., sex pheromones of pest species for monitoring, mass trapping or mating disruption) are already implemented to some extent in integrated management of stored product pests. However, their potential is far from being fully exploited (Schöller et al., 1997; Schöller, 1998; Trematerra, 2012; Fürstenau and Kroos, 2020). For instance, nearly 500 parasitoid species are known to be natural enemies of various insects that attack stored plant products (Hagstrum and Subramanyam, 2009). But so far, only parasitoids belonging to the families Bethylidae and Pteromalidae are commercially available for biological control of stored product pest beetles (Flinn and Schöller, 2012; Amante et al., 2017b; van Lenteren et al., 2018). There are several reasons for the discrepancy between the wide variety of known parasitoids and the low number of commercially available ones. One reason are the costs and efforts that need to be invested for maintaining a rearing of parasitoids (van Lenteren and Tommasini, 1999; Parra and Coelho, 2022). Another one may be our limited knowledge about the biology and odor-mediated host-searching behavior of many species. Such knowledge is necessary for both efficient use of parasitoids in controlling a pest population and for a deeper understanding of the interaction of parasitoids and host insects via infochemicals (Fürstenau and Kroos, 2020).
Foraging parasitoids can eavesdrop on the intraspecific chemical communication of their host species and respond to the sex or aggregation pheromones for host location and recognition. In addition, other volatiles directly associated with the host (e.g., fecal volatiles or compounds on the host’s cuticle) as well as indirectly associated ones (e.g., host feeding substrate or compounds belonging to different developmental stages of the host) also mediate host finding behavior in parasitoids (Vet and Dicke, 1992; Steidle and van Loon, 2003). The identification of host-specific kairomones is crucial for successful application of parasitoids in biological control of storage beetles. One of the major weaknesses of parasitoid use in stored product protection is the poor knowledge about these kairomones that could be used as lures in traps for monitoring parasitoid population densities in storage facilities (Schöller, 1998). Mass release of captured parasitoids could occur with pinpoint accuracy where needed (Trematerra, 2012). Furthermore, application of host-specific kairomones might modify the host-search of parasitoids (Cox, 2004).
Stored product parasitoids have the potential to locate their hosts up to 10 m away in empty storages and up to 4 m horizontally and vertically in grain commodities (Steidle and Schöller, 2002; Niedermayer et al., 2016). However, if local pest populations are widely dispersed, parasitoids might first examine a pest-free area and require more foraging time (Schöller, 1998). Release of synthetic host odors are known to attract parasitoids, guide them directly to an infestation site and increase the host searching activity (Cox, 2004; Phillips and Throne, 2010). The success of biological control of stored product insects by release of parasitoids might be improved by additional application of host kairomones if these host odors improve the parasitoid’s host finding and parasitization success (Gross et al., 1984; Cox, 2004). Despite the potential of host-associated kairomones to enhance biological control of stored product pests, little is known about whether or how the additional release of host-specific kairomones influences host search and host-finding success of parasitoids.
We addressed this gap in knowledge by studying the behavioral responses of the larval ectoparasitoid Holepyris sylvanidis (Bréthes) (Hymenoptera: Bethylidae) to host kairomones. This parasitoid species feeds destructively and oviposits on larvae of several stored product pest beetles, in particular of the genus Tribolium (Evans, 1969; Amante et al., 2017a,b; Awater-Salendo et al., 2020). For oviposition, the female paralyzes a host larva and drags it to a sheltered site where the actual parasitization takes place and the parasitoid progeny can develop on its host (Ahmed et al., 1997). Two kairomonal key components, (E)-2-nonenal and 1-pentadecene released from larval feces of the confused flour beetle, Tribolium confusum (Du Val) (Coleoptera: Tenebrionidae) are known to attract H. sylvanidis over a short range (Fürstenau et al., 2016). While non-infested host feeding substrate (i.e., wheat grist) did not attract the parasitoid, infested wheat grist that naturally releases the kairomonal components, is highly attractive (Fürstenau et al., 2016). Other compounds involved in host location and host recognition of H. sylvanidis are long-chain cuticular hydrocarbons, in particular the methylated ones (Fürstenau and Hilker, 2017; Awater-Salendo et al., 2020, 2021).
A field study by Adler et al. (2012) on the biological control of T. confusum showed that a local pest population in a flour mill was successfully controlled by a biweekly mass release of H. sylvanidis over a period of 6 months. However, because of the lack of suitable traps, it was not possible to monitor released parasitoids during the experiment and to determine their whereabouts at the end. Based on the results from our previous laboratory tests, (E)-2-nonenal and 1-pentadecene represent potential candidate components for such monitoring traps, but this has not been tested so far. Moreover, it is still unclear whether H. sylvanidis also uses other odor cues associated with different developmental stages of T. confusum than the larval stage. Cues from other developmental stages of the host species than the larvae might provide reliable information about the future presence of host larvae. Therefore, in the present study we tested whether two compounds identified from volatiles of adults of different Tribolium species (Suzuki and Sugawara, 1979; Suzuki, 1980; Faustini and Burkholder, 1987) can attract H. sylvanidis females. These two compounds are methyl-p-benzoquinone (MBQ) and 4,8-dimethyldecanal (DMD). MBQ is released with the defensive secretion of adult Tribolium, whereas DMD has been described as part of the aggregation pheromone and is already used for monitoring Tribolium species (Arnaud et al., 2002; Verheggen et al., 2007; Duehl et al., 2011; McKay et al., 2019).
In detail, we studied the following questions: (i) Which concentration of a mix of the two key larval kairomonal compounds, i.e., (E)-2-nonenal and 1-pentadecene (2CM) needs to be added to non-infested host feeding substrate (wheat grist) to render it as attractive as infested feeding substrate? (ii) Is H. sylvanidis also attracted to volatile compounds associated with other developmental stages than beetle larvae (MBQ and DMD) for host search? (iii) Does the release of the specifically host-associated volatiles (2CM) enhance the host search activity and host-finding success of H. sylvanidis?
Therefore, we first performed Y-tube-olfactometer bioassays with different concentrations of 2CM, MBQ and DMD to select the concentration of those compounds which were the most attractive for parasitoid females. Then, we conducted flight cage experiments to analyze how these host larval kairomones affect the host finding behavior of H. sylvanidis. In these cages, we offered 20 host larvae for 3 days with additionally applied host larval kairomones (test) or without (control) and determined host search activity and efficacy, parasitization rate as well as the sex ratio of parasitoid progeny.
2. Methods and material
2.1. Insects
Holepyris sylvanidis and T. confusum specimens were reared at the Julius Kühn Institute (Institute for Ecological Chemistry, Plant Analysis and Stored Product Protection Berlin, Germany) as previously described by Fürstenau et al. (2016). For all bioassays, one- to five-day old H. sylvanidis females without prior oviposition experience and 4th instar T. confusum larvae were used.
2.2. Preparation of kairomonal stimuli
The two-component mix (2CM) of host larval volatiles comprised 1-pentadecene (95%, TCI Europe, Eschborn, Germany) and (E)-2-nonenal (97%, Sigma Aldrich, Taufkirchen, Germany) in a 1:1 ratio. The following concentrations were prepared: 0.02, 0.2, and 2 μg μL−1 in n-hexane (>98%, VWR, Radnor, United States).
To test the parasitoid’s behavioral response to volatiles releases from Tribolium adults, MBQ (98% Sigma Aldrich, Taufkirchen, Germany) was diluted in methanol (99.8%, Merck, Darmstadt, Germany) at the following concentrations: 0.1, 1, 10, and 100 ng μL−1. All solutions were stored at −20°C until usage. Since DMD was not commercially available, we purchased pheromone dispensers with DMD from Trécé Incorporated (Adair, United States). These pheromones dispensers were stored at 5 ± 1°C until usage. Before the DMD-dispensers were used in olfactometer bioassays, they had been placed in a fuming hood for 24 h.
2.3. Y-tube olfactometer bioassays
Behavioral responses of H. sylvanidis females to directly and indirectly host-associated volatiles were analyzed in a Y-shaped glass olfactometer. The olfactometer consisted of a main tube (21 cm long × 15 mm ID) with two 15 cm long arms at an angle of approximately 135° to each other. Each arm was connected via polytetrafluoroethylene (PTFE) tubing to a glass flask (50 mL). The end of each lateral arm was covered with fine-meshed gauze to prevent parasitoid females from escaping. A lid with two entrances sealed each glass flask which contained a test odor sample at the test side and a control sample at the other side. Charcoal-filtered air passed the glass flasks with the samples, entered the Y-tube arms at 100 mL min−1 and flowed to the entrance of the main tube where a parasitoid female was released to the olfactometer. The system was horizontally placed onto a table and homogeneously illuminated by a dimmed neon office lamp (30 lx) placed 50 cm above the olfactometer. All bioassays were conducted under similar conditions from 9:00–17:00 h at 25 ± 1°C and 38 ± 0.2% RH.
Prior to the start of bioassays, Y-tube olfactometers, flasks, and connection tubes were cleaned with a detergent, followed by rinsing with distilled water and 70% ethanol solution (>96%, Berkel AHK, Ludwigshafen, Germany) before being dried in an oven for 3.5 h at 175°C. Unmated parasitoid females were removed from the rearing and kept in plastic Petri dishes (5.0 cm diam., five individuals in each Petri dish) in the experimental room to acclimatize for 1 h before they were placed at the base of the main arm of the olfactometer.
We determined which concentration of kairomones needs to be added to non-infested wheat grist to reach the attractiveness of wheat grist infested with T. confusum larvae. Therefore, we first run bioassays with non-infested wheat grist (NIG) versus air and then infested wheat grist (IG) versus air (Table 1, #1, 2, 7, 12) to check whether our previous results (Fürstenau et al., 2016), which were obtained by using a 4-field olfactometer, can be confirmed with the set-up applied here. We used 5 g finely ground wheat grist (Triticum aestivum Linnaeus 1753). For the IG, we took 5 g infested wheat grist from rearing jars (450 mL) in which about 1,000 T. confusum larvae developed from hatching on for 5 weeks and were removed from the grist prior to the bioassay (compare Fürstenau et al., 2016).
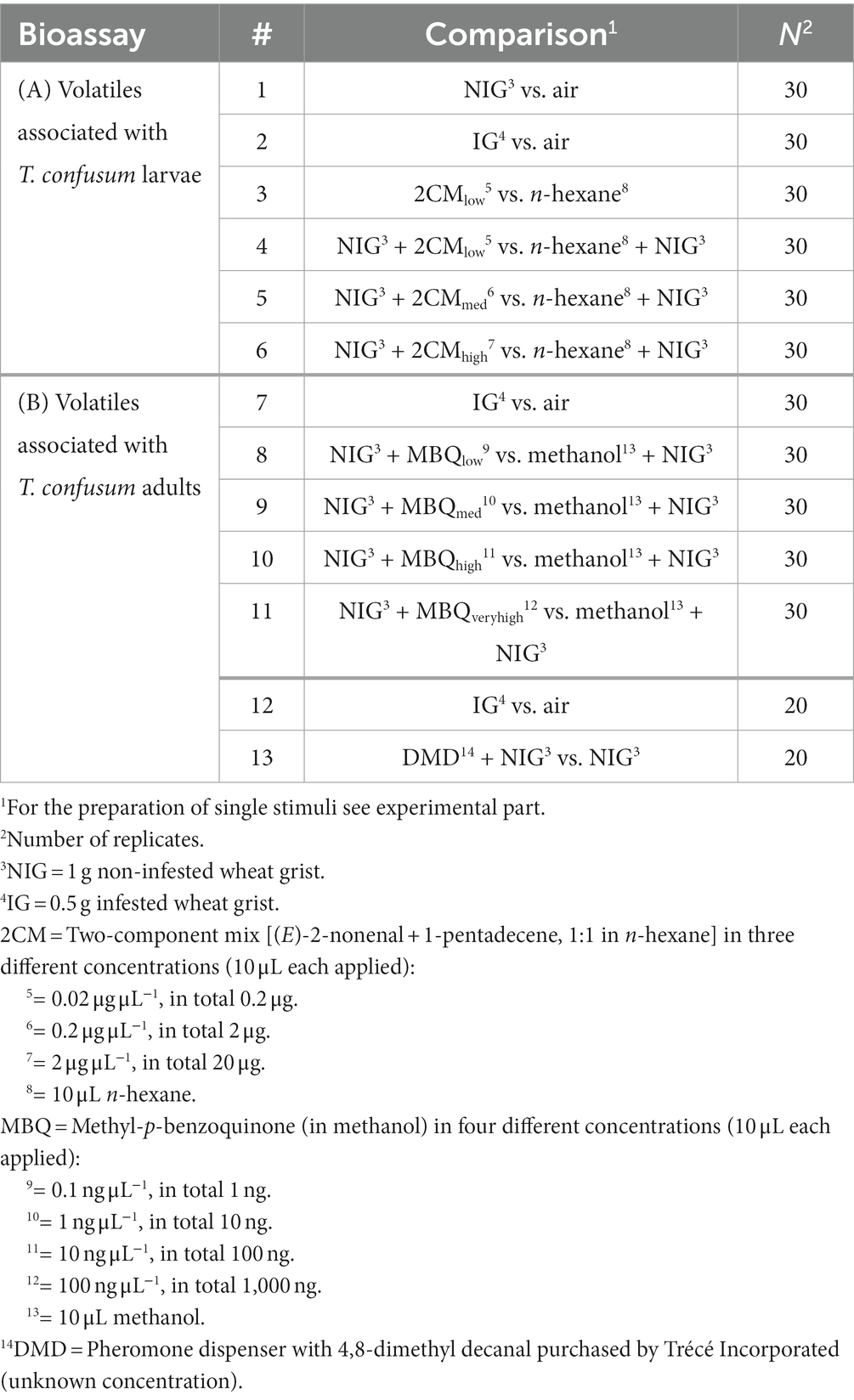
Table 1. Overview of Y-tube olfactometer bioassays to analyze the attraction of Holepyris sylvanidis females to directly and indirectly host-associated volatiles.
We tested the parasitoid’s behavioral response to (i) directly host-associated odors from T. confusum larval feces (2CM) and (ii) indirectly host-associated odors from Tribolium adult volatiles (MBQ and DMD). To test different concentrations of 2CM (Table 1, #3–6), 10 μL of the respective kairomone (test) and 10 μL of the solvent n-hexane (control) were applied on Whatman filter papers (55 mm diam.) and could evaporate for 5 min. The loaded filter papers were then placed into the glass flasks. Likewise, different concentrations of MBQ were tested, but methanol was used for control (Table 1, #8–11). To compare the attractiveness of infested grist with the one of non-infested grist augmented with DMD, we placed a DMD dispenser (compare 2.2) together with 5 g NIG into the test glass flask and 5 g NIG into the control flask (Table 1, #13).
In the beginning of each bioassay, one H. sylvanidis female was released at the bottom of the main arm that was immediately closed with a fine-meshed gauze. The behavioral response of each test individual was observed for max. 300 s. When an upwind-walking individual reached the middle of one arm (crossing a line drawn 30 mm beyond the intersection) and did not return to the intersection within 10 s, this was recorded as positive response to the odor offered in the respective olfactometer arm. Thereafter, the experiment was stopped, and the test individual was removed. After five tests, the Y-olfactometer and glass flask with samples were replaced by new ones. In addition, positions of test and control arms were changed to avoid biased results due to possible side preference of test individuals. A preliminary test in the Y-tube olfactometer with no odor (air vs. air) showed that the parasitoid females showed no side preferences, indicating no positional effect of the experimental setup. Parasitoid females, which had rested for more than 50% of observation time and did not show any preference, were replaced by new ones and were not included in the statistical analysis. This was the case in less than 1% (two observations) of all bioassays listed in Table 1.
Thirty parasitoid females were tested in bioassays offering different concentrations of 2CM or MBQ (N = 30 per compound and concentration). When the effect of DMD on the parasitoid’s olfactory response was studied, twenty replicates were conducted (N = 20).
2.4. Behavioral bioassays in flight cages
To study possible effects of host-associated volatiles (= 2CM in the lowest concentration = 2CMlow) on the host search and host-finding of H. sylvanidis females, we performed behavioral tests using flight cages (32.5 × 32.5 × 77.0 cm, 150 × 150 mesh, cage with sleeve opening, BugDorm, Taiwan). The experiment was conducted in two identical rooms (3 × 4 × 2.5 m), a test and a control room to avoid any biased effects (e.g., by diffusing volatiles from 2CM replicates over control replicates). Both rooms were not air-conditioned or heated. In each room, six flight cages were placed. In the test room, parasitoids were offered host larvae that fed upon wheat grist in the presence of 2CM additionally applied to dispensers (test treatment). In the control room, no 2CM but only hexane was applied in addition to host larvae and wheat grist (control treatment). In both the test and control room, flight cages were placed in two rows. In each row, three cages were located right next to each other, while the distance between the two rows was approx. 63 cm. In front of each row an oscillating table fan (14.4 × 8.0 × 18.8 cm, 5,000 mAh, Comlife, China) was placed behind the side of the cage where the host odor source was. The fan provided a smooth airflow from the site, where host larvae (and kairomonal host odors) were located, to the release points of the parasitoids (Supplementary Figure S1A). All replicates (= flight cages) in one room were treated equally. Two data loggers recorded room temperature and relative humidity in each room (on average 22 ± 1°C and 33 ± 7% RH). The experimental setup is outlined in Supplementary Figure S1A.
Twenty-four hours before starting the experiment, one- to five-day old H. sylvanidis males and females were taken from the permanent rearing and could mate in groups (three females and one male) for approximately 18 h.
On the day of the experiment, H. sylvanidis females were separated from males in the morning and brought into the experimental rooms for 2 h to adapt to local conditions. On one side of the flight cage 20 T. confusum larvae and ca. 10 g of finely grounded wheat grist were evenly distributed in an open Petri dish (55 mm diam) close to an attached rubber septum. Since H. sylvanidis females drag paralyzed host larvae to a shelter for parasitization (Ahmed et al., 1997), pipette tips (0.1–20 μL, Carl Roth, Karlsruhe, Germany) were evenly distributed in the cage as shelter sites (Supplementary Figure S1B). In the test treatment, we applied 10 μL 2CMlow (concentration 0.02 μg μL−1, in total 0.2 μg) to the rubber septum and let the solvent evaporate for 5 min. In the control treatment, 10 μL n-hexane was used.
To start the experiment, three mated parasitoid females (N = 3 per cage) were released 70 cm away from the odor source on the other side of the flight cage (Supplementary Figure S1B).
The experiment took 72 h. Thereafter, we removed the parasitoid females, non-parasitized T. confusum larvae, pipette tips, the dispensers attached to lower parts of Petri dishes, and wheat grist from the cages. All cages were cleaned with 70% ethanol. In total, we repeated the experiments 10 times (N = 6 cages × 10 replicates each per control and test).
To analyze how host-associated volatiles (2CM) affect the parasitoid’s host search and parasitization success, we determined the following parameters: host search activity efficacy in host search, parasitization rate of host larvae, and production of a new parasitoid generation (adult parasitoid offspring).
2.4.1. Host search activity
The number of host larvae dislocated by the parasitoids from the open Petri dish to the pipette tips or somewhere else in the flight cage, was considered a parameter of host search activity. We counted the number of dislocated host larvae at different time point (1, 24, 48, and 72 h after parasitoid release) without removing them from the cage. At the end of the experiment (after 72 h), however, the number of the counted larva did not always match the number of host larvae missing in the open Petri dish (=23 times during the flight cage experiment). This was probably due to the escape of some parasitoid females from the cage through tiny openings; indeed, sometimes, a parasitoid female was seen outside the cage. Therefore, in case of a mismatch between the number of found, dislocated host larvae and number of host larvae missing in the open Petri dish, we considered the number of missing larvae as dislocated ones because host larvae could not leave the Petri dish on their own. We determined the mean number of these dislocated larvae at each time point in test and control cages. The overall dislocation rate was calculated as percentage of dislocated larvae of all offered larvae at the experiment’s end (72 h). In some cases, we could recover all offered T. confusum in the Petri dish 72 h after bioassay start, indicating that no dislocation took place probably due to escaped parasitoids. Those replicates were excluded from further statistical analysis. This was the case for 13 test cages and 20 control cages.
2.4.2. Host search efficacy
For evaluation of the parasitoid’s efficacy in host search, the number of host larvae with feeding marks inflicted by the parasitoids (e.g., brownish bite marks at the forelegs) and that of host larvae that were found dislocated, but without feeding marks were used. The efficacy in search for host larvae was calculated by determining the sum of these larvae as percent of the number of offered host larvae at the beginning of the experiment (N = 20 host larvae per cage). Host larvae with feeding marks are not used for parasitization (Abdella et al., 1985).
2.4.3. Parasitization rate of T. confusum larvae
We determined the number of parasitized host larvae at the end of the experiment (72 h). Parasitized host larvae were paralyzed, and one parasitoid egg was placed externally between the first hind pair of legs (approximately between the first and second abdominal sternite; Ahmed et al., 1997; Amante et al., 2017a). The parasitization rate was calculated as percentage parasitized larvae of the number of initially offered host larvae (N = 20 per cage).
2.4.4. Parasitoid offspring
At the end of the experiment, parasitized host larvae were individually placed in Petri dishes (55 mm diam.) and stored at 25 ± 1°C, 65 ± 5% RH and permanent darkness for further development. Four to 6 weeks after egg deposition, H. sylvanidis adult offspring emerged from the host larvae. For 6 weeks, we determined the number of adult female and male parasitoid individuals emerging from parasitized host larvae. For the mean number of all emerged H. sylvanidis offspring individuals and the mean overall emergence rate, we considered all emerged offspring including those of not identifiable sex. The emergence of the latter was indicated by empty parasitoid cocoons, but the sex could not be identified due to the disappearance of adults after emergence. The mean overall emergence rate was calculated by determining the total number of emerged adult parasitoid offspring as percent of the number of parasitized host larvae. In the case that a parasitoid larva died during its development and no parasitoid adult emerged during the observed period from a host larva, these host larvae were not included in further statistical analysis.
2.5. Statistics
All statistical analysis were performed in “R,” version 4.1.3 (R Core Team, 2021).
For the evaluation of data obtained by the Y-tube olfactometer assays, we used a one-sided binomial test to analyze differences in the parasitoid’s preference for simultaneously offered control and test stimuli. The null hypothesis was that percentages of test individuals choosing the test or the control stimulus were equal. Furthermore, we compared the parasitoid preference for IG and NIG plus kairomones by performing the test for equality of proportions (Newcombe, 1998a,b).
The behavioral parameters recorded in the flight cage experiment were first analyzed for normal distribution by the Shapiro–Wilk test. In the case of normal distribution, the behavioral parameters of parasitoids in test and control were compared by a Student’s t-test. If data were not normally distributed, we applied the Wilcoxon rank-sum test for independent samples instead. To analyze if there were differences in the sex ratios of emerged parasitoid offspring that emerged in the test and control cages, we applied χ2 goodness of fit test.
3. Results
3.1. Response of Holepyris sylvanidis to host-associated volatiles from Tribolium confusum larvae and adults
Adult parasitoid females strongly preferred infested wheat grist (IG, Figure 1). Almost 90% of the parasitoids chose the test arm provided with IG odor over the control arm, whereas they did not prefer non-infested wheat grist (NIG, 57%).
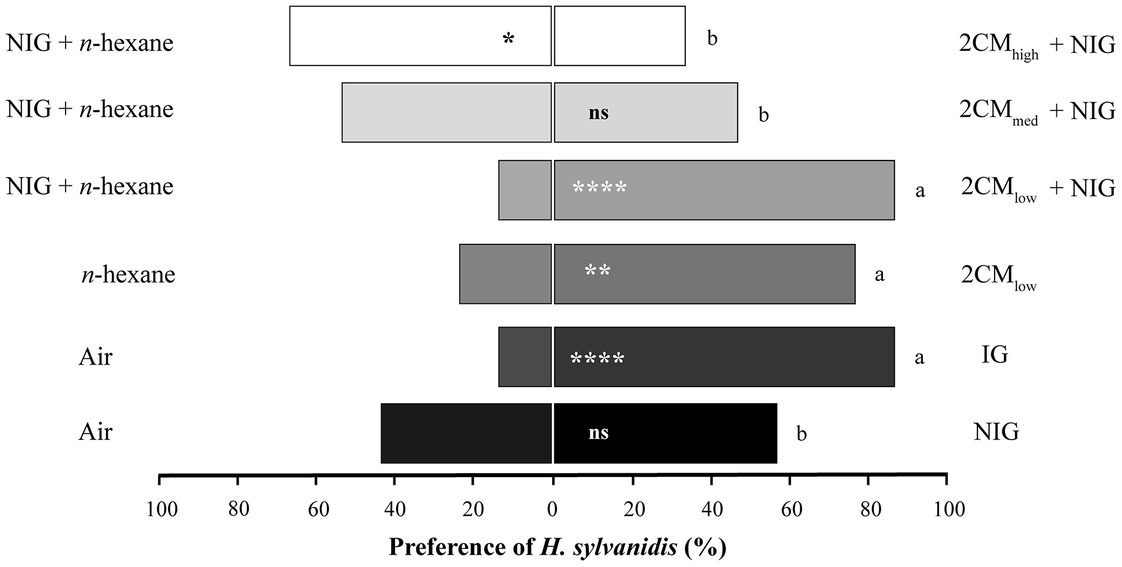
Figure 1. Behavioral response of Holepyris sylvanidis females to larval host-associated odors in pairwise Y-tube olfactometer tests (N = 30 per comparison). NIG = non-infested wheat grist, IG = infested wheat grist, 2CM = Two-Component-Mix in three different concentrations [(E)-2-nonenal and 1-pentadecene 1:1. The compounds were applied onto filter papier (10 μL of each concentration), 2CMlow = 0.02 μg μL−1, in total 0.2 μg, 2CMmed = 0.2 μg μL−1, in total 2 μg, 2CMhigh = 2 μg μL−1, in total 20 μg]. Solvent = 10 μL n-hexane. For the pairwise comparison of two simultaneously offered stimuli, we used a one-sided binomial test. Asterisks indicate significant differences in the parasitoid’s preference (ns = not significant, *p < 0.05, **p < 0.01, ****p < 0.001). To compare the parasitoids’ response to 2CM with one to IG, we applied the test for equality of proportions. Different letters indicate significant differences at p < 0.05.
The response of parasitoids to directly host-associated kairomonal odor (2CM) offered on filter paper in the olfactometer assay were as follows (Figure 1): A low concentration (2CMlow) was significantly preferred by 77% of H. sylvanidis females when tested against the control odor. In comparison to the parasitoid’s response to IG, fewer test individuals preferred 2CMlow but there was no significant difference. When offering a combination of 2CMlow and NIG, the number of test individuals preferred this odor increased (87%); this number was as about high as that preferred IG odor. However, the higher the concentration of (E)-2-nonenal and 1-pentadecene was in the 2CM odor, the fewer individuals preferred the test arm. Only 47% of test individuals preferred the odor of the intermediate 2CM concentration (2CMmed) over the control. In bioassays with the highest concentration of 2CM (2CMhigh) 66% of all test individuals were repelled. The number of parasitoids, which preferred the odor of 2CMmed and 2CMhigh, was significantly lower than the number of parasitoids, which preferred IG odor. Thus, regardless of the presence or absence of non-infested wheat grist, only a low concentration of 2CM turned out to be as attractive to female H. sylvanidis as odor of infested grist.
The indirectly host-associated volatiles from T. confusum adults elicited the following responses of the parasitoids (Figure 2): Odor of all four tested concentrations of MBQ (10 μL each applied onto filter paper) was not significantly attractive when tested against NIG combined with the solvent methanol (Figure 2A). The highest MBQ concentration (MBWvery high, 100 ng μL−1, in total 1,000 ng) even had a significant repellent effect on the parasitoids. The percentage of parasitoids that chose the olfactometer arm provided with MBQ odor decreased from about 40% when testing the lowest MBQ concentration (MBWlow, 0.1 ng μL−1, in total 1 ng) or high MBQ concentration (MBQhigh, 10 ng μL−1, in total 100 ng) to about 17% when testing the highest (repellent) MBQ concentration (MBWvery high). This trend of a decreasing number of parasitoids choosing the MBQ-provided olfactometer arm when the MBQ concentration increased was interrupted by the response of parasitoids to the intermediate MBQ concentration (MBWmed, 1.0 ng μL−1, in total 10 ng). About 60% of the tested individuals chose the olfactometer arm with MBQmed. While this proportion of MBQ-responsive females did not significantly differ from the one responding to NIG only, it was neither statistically different from the percentage of females (~ 80%) responding to the attractive odor of IG. The percentage of parasitoids responding positively to the other tested MBQ concentrations was always significantly lower than the one responding positively to IG odor (Figure 2A). Thus, the behavioral response of the parasitoids to MBQ was dependent on the MBQ concentration.
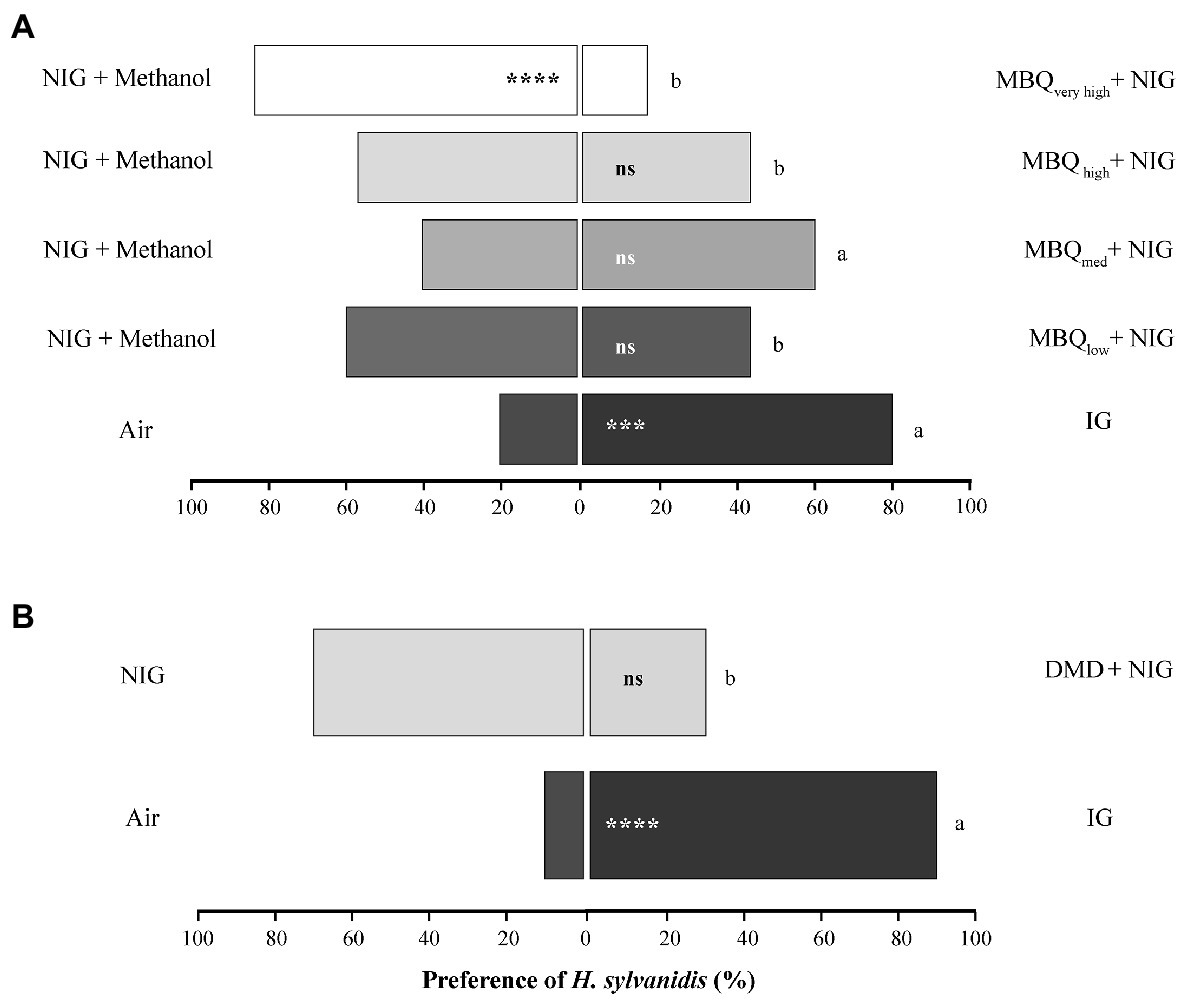
Figure 2. Behavioral response of Holepyris sylvanidis females to adult host-associated odors (A) different concentrations of methyl-p-benzoquinone (MBQ) on filter paper (10 μL of each concentration) and (B) pheromone dispensers loaded with 4,8 dimethyl decanal (DMD) in pairwise Y-tube olfactometer tests (N = 30 per comparison for MBQ, N = 20 for DMD). NIG = non-infested wheat grist, IG = infested wheat grist, MBWlow = 0.1 ng μL−1, in total 1 ng, MBWmed = 1 ng μL−1, in total 10 ng, MBWhigh = 10 ng μL−1, in total 100 ng, MBQveryhigh = 100 ng μL−1, in total 1,000 ng. Solvent = 10 μL methanol. No information is available on the actual concentration of DMD on the commercially purchased dispensers. For the pairwise comparison of two simultaneously offered stimuli, we used a one-sided binomial test. Asterisks indicate significant differences in the parasitoid’s preference (ns = not significant, *p < 0.05, ***p < 0.005, ****p < 0.001). To compare the parasitoids’ response to MBQ (and DMD) with the one to IG, we applied the test for equality of proportions. Different letters indicate significant differences at p < 0.05 for the MBQ tests and p < 0.001 for the DMD test.
When odor of DMD released from a rubber septum was combined with NIG, it did not elicit a significant behavioral response of the parasitoids. Most test individuals (70%) chose the control arm (with NIG only), while 30% went into the test arm supplied with DMD plus NIG, this difference was not statistically significant. The percentage of test individuals choosing the test arm was significantly lower than the one attracted by IG odor (90%, Figure 2B). All details about the statistical evaluation on the above-mentioned results are given in Supplementary Table S1.
3.2. The influence of 2CM on host search of H. sylvanidis
The directly host-associated kairomones (2CM, 10 μL applied onto a rubber septum) exerted significant effects on various parameters of the host location behavior of H. sylvanidis in a flight cage.
3.2.1. Host search activity
When taking the number of dislocated host larvae as indicator for host search activity, the offered low concentration of 2CM (2CMlow, 0.02 μg μL−1, in total 0.2 μg) induced the search activity during the first 2 days after experiment start (Figure 3). Already 1 h after parasitoid release, parasitoid females had dislocated T. confusum larvae in test cages where 2CM was additionally applied. The number of dislocated T. confusum larvae was significantly higher in the test cages than in control cages where no host larvae were dislocated at this time point. Twenty-four hours after parasitoid release dislocated host larvae were found in both test and control cages but their number was significantly higher in the test cages than in the control ones. After 48 h the number of dislocated host larvae duplicated both in test and control flight cages. Nevertheless, parasitoid females dislocated significantly more host larvae in the presence of 2CM than in the control. While the number of dislocated host larvae was still higher in the test cages than in the controls at the end of the experiment (72 h), this difference was not significant anymore when compared to the control cages (Figure 3). Overall, about one third of the offered T. confusum larvae were dislocated in cages provided with 2CM while it was only about 25% in the control cages (Table 2). Thus, the 2CM treatment enhanced the search activity of the parasitoids for about 2 days after application of the kairomones, but not for longer.
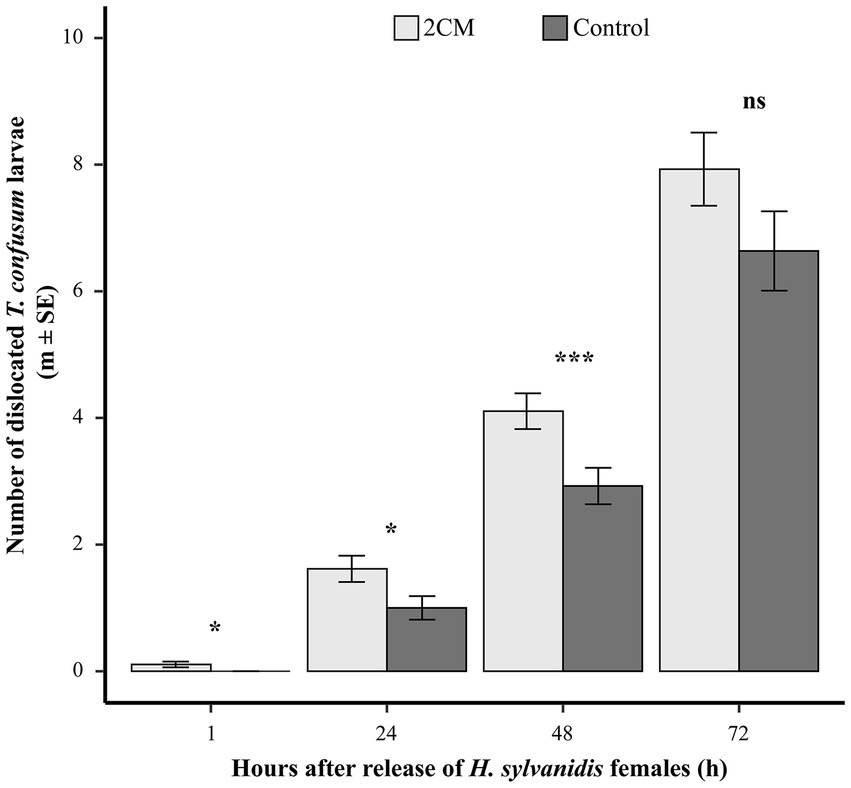
Figure 3. Mean number of dislocated Tribolium confusum larvae in a flight cage trial in the presence or absence of additionally applied larval host kairomones [2CM = (E)-2-nonenal and 1-pentadecene, ratio 1:1 in n-hexane; 10 μL applied onto a rubber septum, concentration = 0.02 μg μL−1, in total 0.2 μg]. Dislocated larvae were counted after 1, 24, 48, and 72 h (N = 40–47 per treatment). For each time interval, differences between the treatments were analyzed by applying a student t-test or Wilcoxon rank-sum test for independent data (ns = not significant, *p > 0.05, ***p > 0.005).
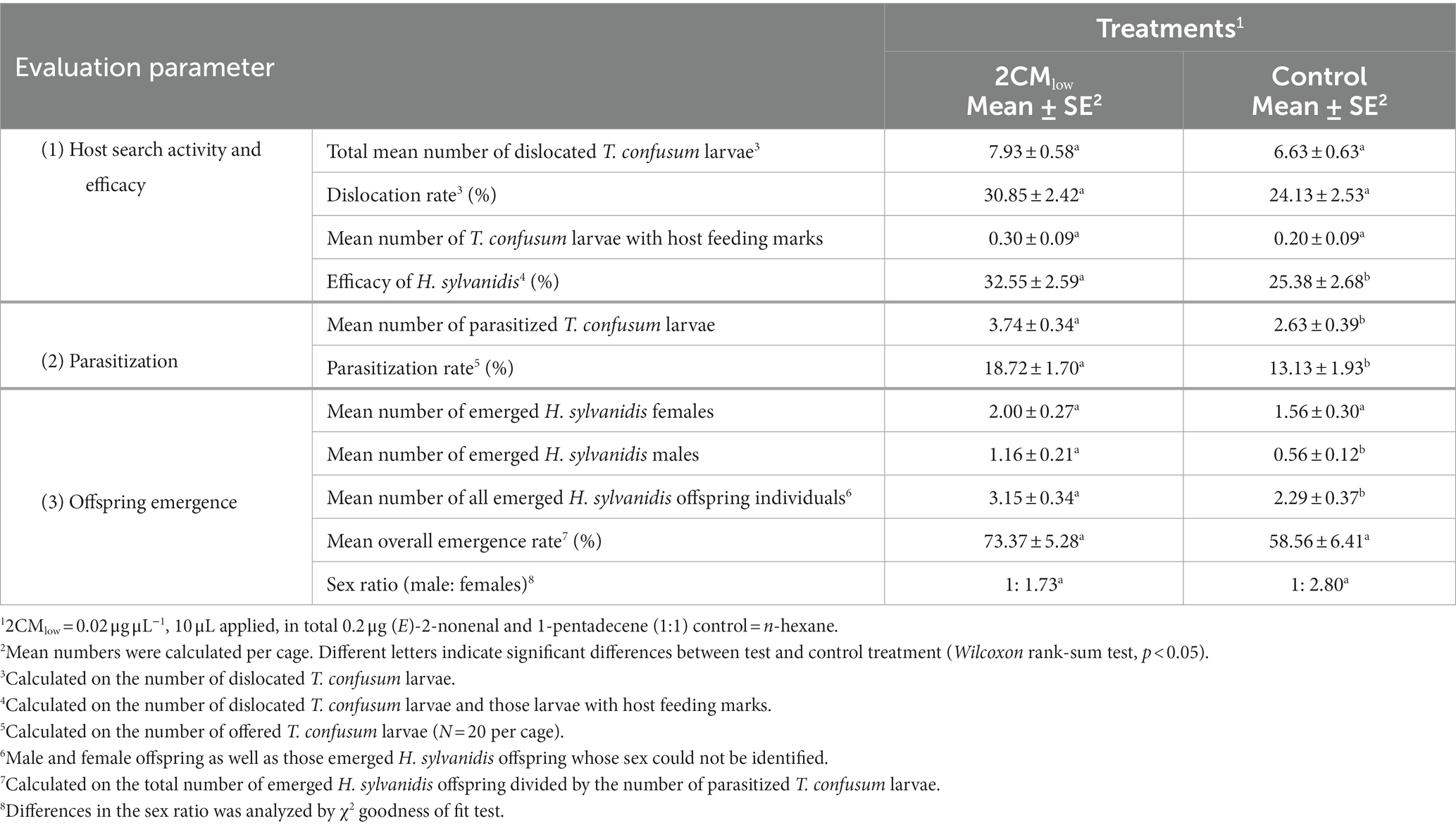
Table 2. Effect of a mixture of synthetic host larval volatiles (2CM) on the host finding success of Holepyris sylvanidis females determined by host search activity and efficacy, parasitization and offspring emergence.
3.2.2. Host search efficacy
At the end of experiments, we detected beetle larvae with host feeding marks in all treatments, but their number did not significantly differ between test and control treatment (Table 2). Nevertheless, we used the number of larvae with host feeding marks and added them to the total number of dislocated T. confusum larvae found without feeding marks within 72 h for calculating the efficacy of H. sylvanidis females as biological control agent. In the presence of 2CM the parasitoid’s search efficacy was reflected by 32.55% of all host larvae offered that were dislocated and/or fed upon. This percentage is significantly higher than that in the absence of host-associated volatiles (~25%), indicating that the host search efficacy is inducible by 2CM.
3.2.3. Parasitization rate
When counting the number of visibly parasitized host larvae, H. sylvanidis parasitized in average significantly more T. confusum larvae per cage in the presence of 2CM when compared to the control (Table 2). When calculating the parasitization rate per 20 host larvae offered in the beginning of the experiment (N = 20) per cage, the parasitization rate was significantly higher in the test treatment than in the control.
3.2.4. Parasitoid offspring
Four-to-six weeks after the bioassays, we recorded the number of adult parasitoids that successfully developed in visibly parasitized larvae and emerged from them. When taking all visibly parasitized larvae as 100%, about three quarters of them in the 2CM-treated cages and nearly 60% of them in the control cages delivered adult parasitoids (Table 2). While the difference in this mean overall emergence rate between test and control cages was not significant, the mean number of all emerged H. sylvanidis offspring individuals was significantly enhanced in the test cages treated with 2CM.
Regarding the sex of the parasitoid offspring, significantly more male offspring emerged from parasitized host larvae that were in the 2CM-treated test cages compared to that from parasitized host larvae in the control cages (Table 2). In contrast, there was no significant difference in the number of female offspring emerging from parasitized larvae in test and control cages. The ratio of male to female offspring was about 1:2 in the test cages and about 1:3 in the control cages but this shift in ratio was not significant. Thus, the additional application of 2CM changed the total parasitoid offspring production, particularly due to the increase of male progeny.
An overview of detailed statistics on the above-mentioned parameters is given in Supplementary Table S2.
4. Discussion
Our study showed that larval host kairomones can significantly enhance the host search activity, efficacy and parasitization rate of the larval ectoparasitoid H. sylvanidis. In contrast, compounds released by adult hosts for aggregation and defense did not elicit positive responses by the parasitoids. The parasitoid’s responses to the larval host kairomonal compounds (2CM) as well as to the compounds released by adult hosts (MBQ, DMD) were concentration dependent. The high concentrations repelled the parasitoids. Interestingly, parasitoid offspring produced in the presence of applied larval host kairomones was increased, more male offspring emerged, and the sex ratio tended to shift toward males (p = 0.13).
High concentrations of larval host kairomones indicate a high host density and thus, a rich resource for the parasitoids. So why do high concentrations of these compounds repel the parasitoids? The attractive concentration of 2CM tested here (2CMlow, 0.02 μg μL−1; 10 μL applied) is equivalent to the quantities of larval feces released by more than 100,000 T. confusum host larvae in the 4th instar (calculated based on data by Fürstenau et al., 2016). Thus, even the lowest concentration of 2CM tested here already indicates a very high host population density. Higher concentrations of 2CM indicate enormously high host densities. Such extraordinarily high host densities might be linked with reduced host quality due to high intraspecific competition among the hosts and a strong risk of entomopathogen infection; it will be beneficial for H. sylvanidis to avoid risky, low-quality hosts in which the chance to successfully produce healthy offspring would be low.
Like for the larval host kairomones, one of the tested indirectly host-associated volatiles, i.e., MBQ, also elicited a repellent effect at a very high concentration. A very high concentration of this compound (100 ng μL−1; 10 μL applied), which is released with the defensive secretion of adults (von Endt and Wheeler, 1971; Wirtz et al., 1978), might indicate a high risk of predation by entomophagous enemies. The parasitoids will benefit from avoiding such habitats. In contrast, the lower concentrations of MBQ tested here (0.1, 1, 10 ng μL−1; 10 μL applied) did not elicit any significant response of the parasitoids, showing quite a “relaxed” behavior in the presence of this aggressive odor. The tested DMD odor was released from purchased dispensers with this aggregation pheromone compound, but the exact concentration is unknown. Females of H. sylvanidis showed no significant preference for or avoidance of this odor. So far, DMD was only detected in odor released by adult host males (Arnaud et al., 2002). If DMD would also be released by adult host females, the odor might indicate a likelihood of host eggs to be laid, from which host larvae will hatch. Thus, the lack of a significant response of the parasitoids to DMD might be explained by the fact that DMD is released only by host males, and thus does probably not provide reliable indication for the presence of host larvae or of host eggs to be laid by host females.
Our results suggest at a first glance that the kairomone function of host odor is specific for the host’s developmental stage in the interaction between T. confusum and H. sylvanidis, since attraction was only found for 1-pentadecene and (E)-2-nonenal, introduced here and known as typical host larval kairomones. However, 1-pentadecene has also been detected in odor released from adult male and female Tribolium species (von Endt and Wheeler, 1971; Keville and Kannowski, 1975; Arnaud et al., 2002; Verheggen et al., 2007). Thus, attraction of parasitoids is obviously possible by odor components that larval and adult hosts have in common.
The above-discussed results were obtained by olfactometer assays. The flight cage bioassays revealed that the host larval kairomones (2CM) can also significantly affect the parasitoid’s behavioral response under conditions mimicking the natural ones a bit more than the olfactometer bioassays. The tiny parasitoids were shown to successfully and quickly locate hosts (and host odor) that is 70 cm away from them.
The loss of the positive effect of 2CM on host search activity 72 h after the start of the flight cage bioassays is probably due to the evaporation of these kairomones. An analysis of the release rate of 2CM from a rubber septum (as applied in the flight cage bioassays) revealed a very rapid release of the highly volatile (E)-2-nonenal within 2 days, while 1-pentadecene was released continuously only in slow quantities (Supplementary material: Release rate; Supplementary Figure S2). The co-incidence of the loss of effect of 2CM on the parasitoids’ search activity after 2 days (Figure 3) and the high evaporation of (E)-2-nonenal within 2 days suggests that the significant behavioral effects of 2CM on host search activity is especially induced by (E)-2-nonenal. On the other hand, our previous bioassays (in a 4-field olfactometer) showed no difference in the attractiveness of (E)-2-nonenal and 1-pentadecene.
At the beginning of the experiment, we observed that H. sylvanidis females in the test cages immediately walked toward the odor source (2CM dispensers) after release, while parasitoid females in the control cages remained at the release site. But during the experiment, these females were observed dispersing throughout the control cages. One further explanation for the loss of the effect of 2CM on the search activity of H. sylvanidis 72 h after experiment start could be also that the less readily and actively searching parasitoids in the control cages could finally catch up with those in the test cages in terms of the number of dislocated larvae because they had that much time for searching (72 h). It is very likely that parasitoids in the control also have completely searched the small cages within 3 days. As a result, the number of dislocated host larvae between test and control treatment converged at the end of the experiment.
The enhancer effect of 2CM on the parasitization rate of H. sylvanidis (calculated based on the number of offered T. confusum larvae) might be a consequence of the increased search activity of the parasitoids during the first 2 days of the experiment. Our results are consistent with those of Gross et al. (1984), who showed that the additional application of kairomones (here: hexane extracts of corn earworm scales, Heliothis zea) can enhance the parasitization activity of the egg parasitoid Trichogramma pretiosum. We also suppose that the parasitization rates in both the test and control treatments were likely higher than our results indicate. In some cases, at the end of experiment we could not recover all of the 20 offered T. confusum larvae. This was probably because some parasitoid females found a way out the cage and were able to drag paralyzed host larvae outside the cage. We do not know exactly whether H. sylvanidis used these dislocated host larvae for oviposition. However, when calculating the parasitization rate based on the number of parasitized, dislocated larvae found (all found dislocated larvae = 100%), the parasitization rate was about 65% in the test cages and about 50% in the controls. This suggests a higher parasitization rate in both treatments. Further studies on a larger scale (e.g., as reported in Niedermayer et al., 2016) are necessary to evaluate the potential of host-associated odors (2CM) on long-range host finding and parasitization rate of H. sylvanidis. However, it should be considered that the application of synthetic kairomones in stored product environments might disturb the host search of parasitoids if these compounds interfere with the kairomones naturally emitted by the host, thus preventing the parasitoids from detecting the exact location of their hosts. Therefore, the concentration of synthetic kairomones should be chosen very carefully.
The application of 2CM resulted in an increased number of male offspring individuals, which contributed to an overall increase in the total number of emerged offspring individuals in test cages. Holepyris sylvanidis is an arrhenotocous species. Male offspring hatches from unfertilized eggs and female offspring from fertilized eggs (Abdella et al., 1985). A higher proportion of male offspring in arrhenotocous parasitoid species may be caused by numerous factors, among them, e.g., mating success, age of mates, maternal crowding, mate competition. Furthermore, the host quality and density, superparasitism, microbial infection and abiotic factors may contribute to an increase in the number of males in a parasitoid population (e.g., Godfray, 1994; Fuester et al., 2003; West et al., 2005; Boulton et al., 2015; Nurkomar et al., 2021). Since parasitoids in the test cages with 2CM treatment and in control cages were exposed to the same mating conditions prior to the experiment, same densities (three parasitoid females of same age per cage), same host qualities and densities, and same abiotic factors, none of the above-mentioned factors is likely to have contributed to the observed changes in the composition of parasitoid progeny. In addition, the proportion of males in parasitoid progeny in the control cages was similar to our permanent rearing of H. sylvanidis (1 male to 3 females).
We suggest that the 2CM-induced search activity of the females negatively affected successful egg fertilization. The increased search activity might have reduced egg fertilization (i) by affecting the sperm release from the female’s spermatheca storing the male’s ejaculate after mating and/or (ii) by changing the quality of the sperm in the female’s spermatheca. It is well known for insects that they can control the release of sperm from their spermatheca (e.g., Klowden, 2009). The 2CM-induced search activity might be linked with reduced release of sperm from the spermatheca. Interestingly, the biogenic amines octopamine and tyramine are known to affect both an insect’s locomotory activity as well as sperm release (Avila et al., 2012; Selcho et al., 2012). Whether indeed 2CM can affect signaling by these biogenic amines remains to be analyzed by future studies. Alternatively or additionally, the 2CM-enhanced search activity might result in reduced sperm quality in the spermatheca because probably energy-taking, enhanced search activity might lead to changes in factors important for sperm maintenance in the spermatheca (Wolfner, 2011; Pascini and Martins, 2017). Another trade-off of intensive searching activity induced by synthetic kairomones might negatively affect the energy budget of parasitoid females by, e.g., reducing their fecundity and longevity.
If a parasitoid population produces more male offspring in the presence of synthetic kairomones, this shift in the population composition may negatively affected success of biological control by parasitoids because parasitoid females are needed to parasitize the host and reduce the host (pest) population (Heimpel and Lundgren, 2000). Thus, the attempt to improve biological control by application of 2CM needs to cope with the trade-off between 2CM-induced parasitization rate and an increasing parasitoid density as well as a 2CM-induced change in the sex distribution of the parasitoid population. It needs to be considered that males are necessary to maintain a parasitoid population stable as the production of female parasitoid offspring requires fertilization of eggs in arrhenotecous species (Mayhew and Heitmans, 2000; Amante et al., 2017a). In some parasitoid species, male parasitoids can influence the offspring sex ratio to some extent, for example, by producing more female offspring to transfer the father’s genetic contribution to the next generation (Henter, 2004; Shuker et al., 2006). Thus, the pendulum could swing back from a male-biased sex ratio in the other direction, thereby stabilizing the population. More research is needed to answer how application of 2CM would affect the population composition and stability of a H. sylvanidis population over time.
In summary, the release of attractive kairomones can improve the host search and thus host-finding success of H. sylvanidis. This approach might be very interesting for future biological control strategies of stored product pests, in particular in bakeries and mills where the use of pesticides is not tolerated. However, further studies are needed to test the potential of host-specific volatiles in larger experimental set-ups and over a longer period, especially to investigate possible effects on the sex allocation of the progeny. These results can contribute in various ways to advance the use of natural enemies in stored product protection and biologically based control of storage pest insects.
Data availability statement
The raw data supporting the conclusions of this article will be made available by the authors, without undue reservation.
Author contributions
SA-S, MH, and BF contributed to the study conception and design. SA-S performed material preparation, data collection, analysis, and wrote the first draft of the manuscript which was revised by BF and MH. All authors commented on previous versions of the manuscript, read, and approved the final manuscript.
Acknowledgments
We thank Tobias Hoffmann (Julius Kühn Institute, Berlin, Germany) for his help in the insect rearing and Raphael Büchner and Max Freihoff for their technical assistance in determining the release rate of 2CM. We also thank Nils Hildebrandt and Peggy Jones (Freie Universität Berlin) for their support in performance of the bioassays. We also thank three reviewers for their helpful comments on an earlier version of this manuscript.
Conflict of interest
The authors declare that the research was conducted in the absence of any commercial or financial relationships that could be construed as a potential conflict of interest.
Publisher’s note
All claims expressed in this article are solely those of the authors and do not necessarily represent those of their affiliated organizations, or those of the publisher, the editors and the reviewers. Any product that may be evaluated in this article, or claim that may be made by its manufacturer, is not guaranteed or endorsed by the publisher.
Supplementary material
The Supplementary material for this article can be found online at: https://www.frontiersin.org/articles/10.3389/fevo.2023.1158081/full#supplementary-material
Abbreviations
2CM, Two-Component-Mix containing (E)-2-nonenal and 1-pentadecene; DMD, 4,8-dimethyldecanal; IG, infested wheat grist; MBQ, methyl-p-benzoquinone; NIG, non-infested wheat grist.
References
Abdella, M. M. H., Tawfik, M. F. S., and Awadallah, K. T. (1985). Biological studies on the bethylid parasition Holepyris sylvanidis Berthes. Ann. Agric. Sci. Moshtohor. 23, 1355–1363.
Adler, C., Schöller, M., and Beier, S. (2012). Development of insects in a flour mill treated with Holepyris sylvanidis (Hym., Bethylidae) for biological control of the confused flour beetle Tribolium confusum (Col., Tenebrionidae). IOBC-WPRS Bull. 81, 169–170.
Ahmed, K. N., Khatun, M., Nargis, A., and Dey, N. C. (1997). Mating, egglaying and host feeding behaviour of Rhabdepyris zeae Waterston (Hymenoptera: Bethylidae) parasitizing Tribolium confusum larvae. Bangl. J. Sci. Ind. Res. 32, 633–637.
Amante, M., Schöller, M., Hardy, I. C. W., and Russo, A. (2017a). Reproductive biology of Holepyris sylvanidis (Hymenoptera: Bethylidae). Biol. Control 106, 1–8. doi: 10.1016/j.biocontrol.2016.12.004
Amante, M., Schöller, M., Suma, P., and Russo, A. (2017b). Bethylids attacking stored-product pests: an overview. Entomol. Exp. Appl. 163, 251–264. doi: 10.1111/eea.12587
Arnaud, L., Lognay, G., Verscheure, M., Leenaers, L., Gaspar, C., and Haubruge, E. (2002). Is dimethyldecanal a common aggregation pheromone of Tribolium flour beetles? J. Chem. Ecol. 28, 523–532. doi: 10.1023/A:1014587927784
Avila, F. W., Bloch Qazi, M. C., Rubinstein, C. D., and Wolfner, M. F. (2012). A requirement for the neuromodulators octopamine and tyramine in Drosophila melanogaster female sperm storage. Proc. Natl. Acad. Sci. U. S. A. 109, 4562–4567. doi: 10.1073/pnas.1117689109
Awater-Salendo, S., Schulz, H., Hilker, M., and Fürstenau, B. (2020). The importance of methyl-branched cuticular hydrocarbons for successful host recognition by the larval ectoparasitoid Holepyris sylvanidis. J. Chem. Ecol. 46, 1032–1046. doi: 10.1007/s10886-020-01227-w
Awater-Salendo, S., Voigt, D., Hilker, M., and Fürstenau, B. (2021). Cuticular hydrocarbon trails released by host larvae lose their kairomonal activity for parasitoids by solidification. J. Chem. Ecol. 47, 998–1013. doi: 10.1007/s10886-021-01310-w
Boulton, R. A., Collins, L. A., and Shuker, D. M. (2015). Beyond sex allocation: the role of mating systems in sexual selection in parasitoid wasps. Biol. Rev. Camb. Philos. Soc. 90, 599–627. doi: 10.1111/brv.12126
Cox, P. D. (2004). Potential for using semiochemicals to protect stored products from insect infestation. J. Stored Prod. Res. 40, 1–25. doi: 10.1016/S0022-474X(02)00078-4
Duehl, A. J., Arbogast, R. T., and Teal, P. E. A. (2011). Density-related volatile emissions and responses in the red flour beetle, Tribolium castaneum. J. Chem. Ecol. 37, 525–532. doi: 10.1007/s10886-011-9942-3
Evans, H. E. (1969). A revision of the genus Holepyris in the Americas (Hymenoptera: Bethylidae). Trans. Am. Entomol. Soc. 95, 181–209. doi: 10.5962/BHL.PART.12349
Faustini, D. L., and Burkholder, W. E. (1987). Quinone-aggregation pheromone interaction in the red flour beetle. Anim. Behav. 35, 601–603. doi: 10.1016/S0003-3472(87)80289-0
Flinn, P. W., and Schöller, M. (2012). “Biological control: insect pathogens, parasitoids, and predators,” in Stored product protection. eds. D. W. Hagstrum, T. W. Philipps, and G. Cuperus (Manhattan, KS: Kansas State University), 203–212.
Fuester, R. W., Swan, K. S., Dunning, K., Taylor, P. B., and Ramaseshiah, G. (2003). Male-biased sex ratios in Glyptapanteles flavicoxis (Hymenoptera: Braconidae), a parasitoid of the gypsy moth (Lepidoptera: Lymantriidae). Ann. Entomol. Soc. Am. 96, 553–559. doi: 10.1603/0013-8746(2003)096[0553:MSRIGF]2.0.CO;2
Fürstenau, B., Adler, C., Schulz, H., and Hilker, M. (2016). Host habitat volatiles enhance the olfactory response of the larval parasitoid Holepyris sylvanidis to specifically host-associated cues. Chem. Senses 41, 611–621. doi: 10.1093/chemse/bjw065
Fürstenau, B., and Hilker, M. (2017). Cuticular hydrocarbons of Tribolium confusum larvae mediate trail following and host recognition in the ectoparasitoid Holepyris sylvanidis. J. Chem. Ecol. 43, 858–868. doi: 10.1007/s10886-017-0885-1
Fürstenau, B., and Kroos, G. M. (2020). “Biologically based control strategies for managing stored-product insect pests,” in Advances in postharvest management of cereals and grains. ed. D. E. Maier (Cambridge: Burleigh Dodds Series in Agricultural Science), 267–318.
Godfray, H. C. J. (1994). Parasitoids: behavioral and evolutionary ecology. Princeton, NY: Princeton University Press.
Gross, H. R., Lewis, W. J., Beevers, M., and Nordlund, D. A. (1984). Trichogramma pretiosum (Hymenoptera: Trichogrammatidae): effects of augmented densities and distributions of Heliothis zea (Lepidoptera: Noctuidae) host eggs and kairomones on field performance. Environ. Entomol. 13, 981–985. doi: 10.1093/ee/13.4.981
Hagstrum, D. W., and Subramanyam, B. (2009). Stored-product insect resource. 1st ed. St. Paul Minnesota: AACC International, Inc.
Heimpel, G. E., and Lundgren, J. G. (2000). Sex ratios of commercially reared biological control agents. Biol. Control 19, 77–93. doi: 10.1006/bcon.2000.0849
Henter, H. J. (2004). Constrained sex allocation in a parasitoid due to variation in male quality. J. Evol. Biol. 17, 886–896. doi: 10.1111/j.1420-9101.2004.00746.x
Keville, R., and Kannowski, P. B. (1975). Sexual excitation by pheromones of the confused flour beetle. J. Insect Physiol. 21, 81–84. doi: 10.1016/0022-1910(75)90070-0
Klowden, M. J. (2009). “Spermatheca,” in Encyclopedia of insects. eds. V. H. Resh and R. T. Cardé (Academic Press), 939–940.
Mayhew, P. J., and Heitmans, W. R. B. (2000). Life history correlates and reproductive biology of Laelius pedatus (Hymenoptera: Bethylidae) in the Netherlands. Eur. J. Entomol. 97, 313–322. doi: 10.14411/eje.2000.048
McKay, T., Bowombe-Toko, M. P., Starkus, L. A., Arthur, F. H., and Campbell, J. F. (2019). Monitoring of Tribolium castaneum (Coleoptera: Tenebrionidae) in rice mills using pheromone-baited traps. J. Econ. Entomol. 112, 1454–1462. doi: 10.1093/jee/toy422
Newcombe, R. G. (1998a). Interval estimation for the difference between independent proportions: comparison of eleven methods. Stat. Med. 17, 873–890. doi: 10.1002/(SICI)1097-0258(19980430)17:8<873::AID-SIM779>3.0.CO;2-I
Newcombe, R. G. (1998b). Two-sided confidence intervals for the single proportion: comparison of seven methods. Stat. Med. 17, 857–872. doi: 10.1002/(SICI)1097-0258(19980430)17:8<857::AID-SIM777>3.0.CO;2-E
Niedermayer, S., Krogmann, L., and Steidle, J. L. M. (2016). Lost in space? Host-finding ability of the parasitoids Lariophagus distinguendus and Anisopteromalus calandrae in empty grain storage facilities to control residual pest populations. Biocontrol 61, 379–386. doi: 10.1007/s10526-016-9717-4
Nurkomar, I., Azhar, A., and Buchori, D. (2021). Sex allocation and field population sex ratio of Apanteles taragamae Viereck (Hymenoptera: Braconidae), a larval parasitoid of the cucumber moth Diaphania indica Saunders (Lepidoptera: Crambidae). Open Agric. 6, 673–681. doi: 10.1515/opag-2021-0045
Parra, J. R. P., and Coelho, A. (2022). Insect rearing techniques for biological control programs, a component of sustainable agriculture in Brazil. Insects 13:105. doi: 10.3390/insects13010105
Pascini, T. V., and Martins, G. F. (2017). The insect spermatheca: an overview. Zoology 121, 56–71. doi: 10.1016/j.zool.2016.12.001
Phillips, T. W., and Throne, J. E. (2010). Biorational approaches to managing stored-product insects. Annu. Rev. Entomol. 55, 375–397. doi: 10.1146/annurev.ento.54.110807.090451
R Core Team (2021). R: A language and environment for statistical computing. R Foundation for Statistical Computing, Vienna, Austria. https://www.r-project.org.
Schöller, M. (1998). Integration of biological and non-biological methods for controlling arthropods infesting stored products. Postharvest News Inform. 9, 15N–20N.
Schöller, M., Prozell, S., Al-Kirshi, A.-G., and Reichmuth, C. (1997). Towards biological control as a major component of integrated pest management in stored product protection. J. Stored Prod. Res. 33, 81–97. doi: 10.1016/S0022-474X(96)00048-3
Selcho, M., Pauls, D., el Jundi, B., Stocker, R. F., and Thum, A. S. (2012). The role of octopamine and tyramine in drosophila larval locomotion. J. Comp. Neurol. 520, 3764–3785. doi: 10.1002/cne.23152
Shuker, D. M., Sykes, E. M., Browning, L. E., Beukeboom, L. W., and West, S. A. (2006). Male influence on sex allocation in the parasitoid wasp Nasonia vitripennis. Behav. Ecol. Sociobiol. 59, 829–835. doi: 10.1007/s00265-005-0129-1
Steidle, J. L. M., and Schöller, M. (2002). Fecundity and ability of the parasitoid Lariophagus distinguendus (Hymenoptera: Pteromalidae) to find larvae of the granary weevil Sitophilus granarius (Coleoptera: Curculionidae) in bulk grain. J. Stored Prod. Res. 38, 43–53. doi: 10.1016/S0022-474X(00)00044-8
Steidle, J. L. M., and van Loon, J. J. A. (2003). Dietary specialization and infochemical use in carnivorous arthropods: testing a concept. Entomol. Exp. Appl. 108, 133–148. doi: 10.1046/j.1570-7458.2003.00080.x
Suzuki, T. (1980). 4,8-Dimethyldecanal: the aggregation pheromone of the flour beetles, Tribolium castaneum and T. confusum (Coleoptera: Tenebrionidae). Agric. Biol. Chem. 44, 2519–2520. doi: 10.1080/00021369.1980.10864359
Suzuki, T., and Sugawara, R. (1979). Isolation of an aggregation pheromone from the flour beetles, Tribolium castaneum and T. confusum (Coleoptera: Tenebrionidae). Appl. Entomol. Zool. 14, 228–230. doi: 10.1303/aez.14.228
Trematerra, P. (2012). Advances in the use of pheromones for stored-product protection. J. Pest. Sci. 85, 285–299. doi: 10.1007/s10340-011-0407-9
van Lenteren, J. C. (2012). The state of commercial augmentative biological control: plenty of natural enemies, but a frustrating lack of uptake. Biocontrol 57, 1–20. doi: 10.1007/s10526-011-9395-1
van Lenteren, J. C., Bolckmans, K., Köhl, J., Ravensberg, W. J., and Urbaneja, A. (2018). Biological control using invertebrates and microorganisms: plenty of new opportunities. Biocontrol 63, 39–59. doi: 10.1007/s10526-017-9801-4
van Lenteren, J. C., and Tommasini, M. G. (1999). “Mass production, storage, shipment and quality control of natural enemies” in Integrated pest and disease management in greenhouse crops: developments in plant pathology. eds. R. Albajes, M. Lodovica Gullino, J. C. van Lenteren, and Y. Elad, vol. 14 (Dordrecht: Springer), 276–294.
Verheggen, F., Ryne, C., Olsson, P.-O. C., Arnaud, L., Lognay, G., Högberg, H.-E., et al. (2007). Electrophysiological and behavioral activity of secondary metabolites in the confused flour beetle, Tribolium confusum. J. Chem. Ecol. 33, 525–539. doi: 10.1007/s10886-006-9236-3
Vet, L. E. M., and Dicke, M. (1992). Ecology of infochemical by natural enemies in a tritrophic context use. Annu. Rev. Entomol. 37, 141–172. doi: 10.1146/annurev.en.37.010192.001041
von Endt, D. W., and Wheeler, J. W. (1971). 1-Pentadecene production in Tribolium confusum. Science 172, 60–61. doi: 10.1126/science.172.3978.60
West, S. A., Shuker, D. M., and Sheldon, B. C. (2005). Sex-ratio adjustment when relatives interact: a test of constraints on adaptation. Evolution 59, 1211–1228. doi: 10.1111/j.0014-3820.2005.tb01772.x
Wirtz, R. A., Taylor, S. L., and Semey, H. G. (1978). Concentrations of substitued p-benzoquinones and 1-pentadecene in the flour beetles Tribolium confusum J. Duv val and Tribolium castaneum and p-benzoquinones (Herbst). Comp. Biochem Physiol. B 61, 25–28. doi: 10.1016/0305-0491(78)90207-9
Keywords: Bethylidae, 1-pentadecene, (E)-2-nonenal, host finding, stored product protection, Tribolium confusum, Tenebrionidae
Citation: Awater-Salendo S, Hilker M and Fürstenau B (2023) Kairomone-induced changes in foraging activity of the larval ectoparasitoid Holepyris sylvanidis are linked with an increased number of male parasitoid offspring. Front. Ecol. Evol. 11:1158081. doi: 10.3389/fevo.2023.1158081
Edited by:
Joachim Ruther, University of Regensburg, GermanyReviewed by:
Eric Conti, University of Perugia, ItalyYooichi Kainoh, University of Tsukuba, Japan
Antonino Cusumano, University of Palermo, Italy
Copyright © 2023 Awater-Salendo, Hilker and Fürstenau. This is an open-access article distributed under the terms of the Creative Commons Attribution License (CC BY). The use, distribution or reproduction in other forums is permitted, provided the original author(s) and the copyright owner(s) are credited and that the original publication in this journal is cited, in accordance with accepted academic practice. No use, distribution or reproduction is permitted which does not comply with these terms.
*Correspondence: Benjamin Fürstenau, YmVuamFtaW4uZnVlcnN0ZW5hdUBqdWxpdXMta3VlaG4uZGU=