- Department of Biological Sciences, University of Cincinnati, Cincinnati, OH, United States
Animals inhabiting subterranean environments tend to evolve a constellation of ‘regressive’ and ‘constructive’ features. Regressive traits like vision and pigmentation are reduced or lost in derived organisms. In contrast, constructive traits like non-visual sensation, are commonly augmented and evolving under strong selection. Numerous studies have examined the genetic, developmental and molecular bases for regressive traits, while constructive traits have received less attention. A key constructive sensory feature in cave animals is the gustatory system which is likely useful for animals living in complete darkness, given the need to secure food for survival. Interestingly, despite having been studied for decades in the Mexican tetra, Astyanax mexicanus, much remains unknown regarding the biological basis, and adaptive relevance, of taste system evolution in cave morphs. Here, we present a brief review of taste system research in this system, conducted over the past ~90 years. We underscore key differences in gustation between cave and surface fish that reside at the levels of anatomy, perception and behavior. From this review, we sought to identify key knowledge gaps in our understanding of constructive taste system evolution. Future studies will provide further insights to the nature of constructive trait evolution by determining if constructive and regressive traits evolve through similar or different genetic and developmental mechanisms, and provide an essential case study for examining convergence of constructive traits across geographically distinct populations.
Introduction
Fish live in an immersive aquatic environment where their sensory systems are continuously in contact with a variety of chemical agents (Hara, 2012). Generally, chemoreception facilitates diverse behaviors in fish including obtaining food, defense from predators, sex identification, sensing carbon dioxide, and discriminating conspecifics, among others (Hara, 1971; Jonz et al., 2015). In fish, chemoreception is governed by broad cell and organ types including taste buds, olfactory epithelia, neuroepithelial cells, and solitary chemosensory cells (Reutter, 1986; Jonz et al., 2015). Two major chemosensory systems commonly examined in cave animals are taste (gustation) and smell (olfaction) – which are frequently augmented in the absence of visual input. Olfaction in fish is detected by neurons directly exposed to the environment, and are responsive to four chemical classes: amino acids, bile salts and acids, prostaglandins and sex steroids (Hara, 1994). Gustation is detected by taste receptor cells housed within taste buds which are responsive to nucleotides, salts, sugars, organic acids and amino acids. While the modalities sensed by these two systems are similar with respect to their detection of chemicals (e.g., amino acids), signals are transmitted to different regions of the brain and mediate different behavioral outputs (Hara, 1994).
As a consequence of life in perpetual darkness, olfaction and gustation have undergone diverse changes in a variety of cavefish systems. For instance, species of the Chinese cavefish genus, Sinocyclocheilus, frequently harbor an expanded number and wider distribution of taste buds, as well as gene duplications of taste receptor genes (Ma et al., 2019). These features imply a ‘highly developed sense of taste’ in this lineage (Yang et al., 2016). Additionally, Sinocyclocheilus are regarded as having more sensitive olfaction as a function of more anteriorly-directed, and larger, nostrils (Ma et al., 2019). Interestingly, in Amblyopsid cavefish such as the Spring Cavefish (Chologaster agassizi), feeding behavior appears to rely heavily on taste, but less so on olfaction (Hill, 1969). In other hypogean species, including Phreatichthys andruzzi (Dezfuli et al., 2009), Astrolebus pholeter (Haspel et al., 2012), and Poecilia mexicana (Parzefall, 2001) a large number of taste buds are found distributed, sometimes among mechanosensitive neuromasts, on the head and jaw.
The taste system in particular, appears to be especially labile – capable of adapting to different life history modes and feeding ecologies (Kasumyan, 2019). Here, we focus our attention to the gustatory system of a well-characterized cave dwelling fish, the Mexican tetra (Astyanax mexicanus). This species has long served as a natural model for examining adaptive change in response to extreme environmental pressures. With the rise of this remarkable animal system has come substantial interest in the developmental and genetic regulation of regressive traits. While the underpinnings of regressed traits have been advanced through integrative studies (Moran et al., 2023), the biological bases for constructive traits remain less well understood. Here we focus on an example of constructive evolution – the augmented taste system in Astyanax.
Studies of the gustatory system in blind Mexican cavefish stretch nearly as far back as their discovery ~90 years ago (Breder and Rasquin, 1943). We begin with a review of the genetic basis for peripheral expansion of taste buds in cavefish, both in number and anatomical distribution (Figure 1). We then summarize recognized differences in tastant perception, as well as current knowledge of the developmental basis for taste system expansion. We finish with a summary of recent studies taking a genome-level approach, examining bitter taste receptor family members and their putative expansion within the Astyanax lineage (Shiriagin and Korsching, 2019). Throughout, we revisit hypotheses seeking to explain the adaptive significance and drivers of taste system expansion, e.g., as a “compensatory” mechanism for survival in the absence of vision (Boudriot and Reutter, 2001). At the close of this review, we share our assessment of important yet unresolved aspects of taste biology in Astyanax, and propose future areas of focus.
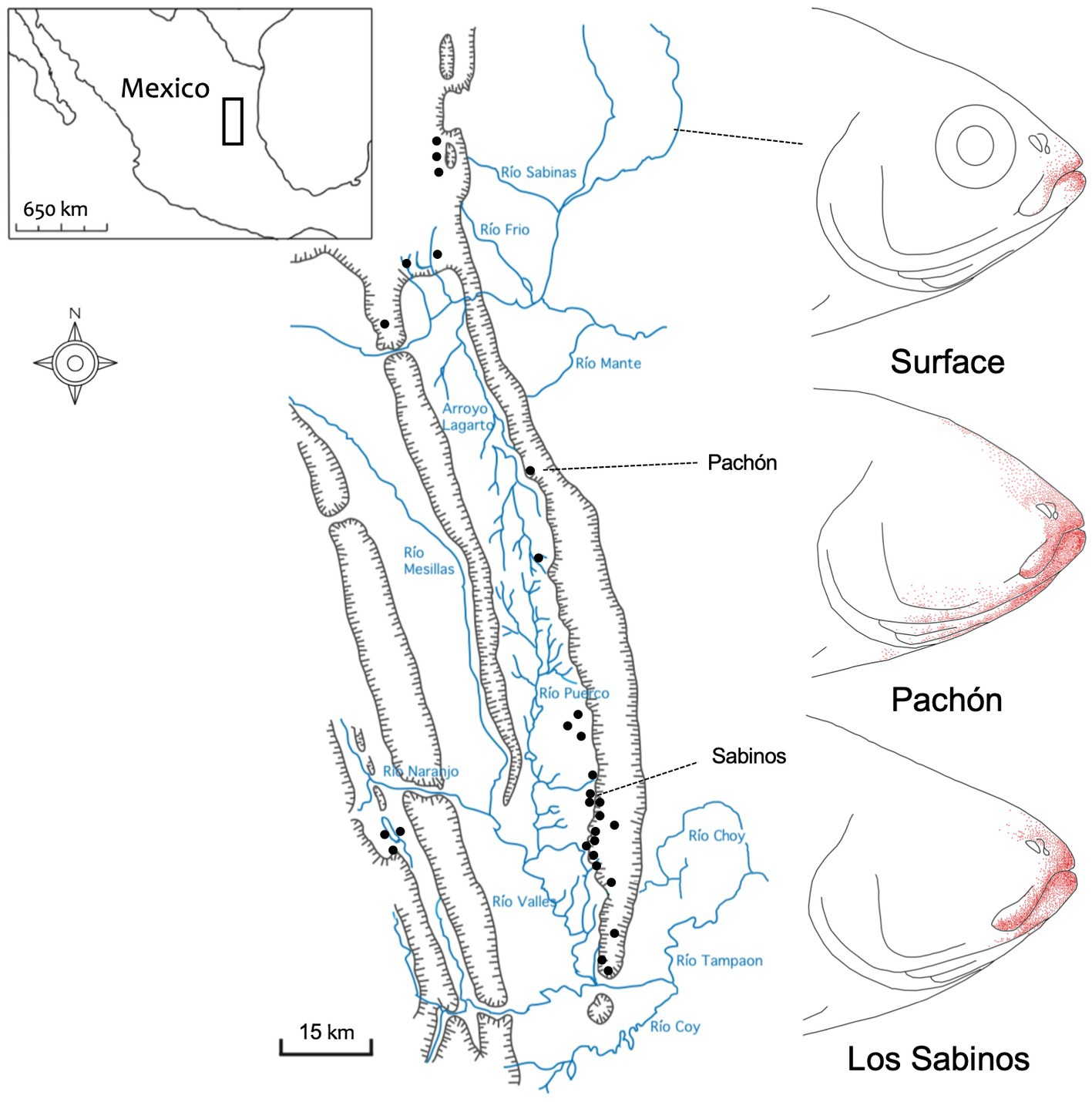
Figure 1. The distribution of extraoral taste buds in three populations of Mexican tetra. Surface-dwelling morphs of Astyanax mexicanus (top) demonstrate a modest distribution of taste buds (red dots) that reside close to the oral opening. Pachón cavefish (middle) have the most significant numbers, and largest distribution, of extraoral taste buds. Cavefish from the Sabinos (bottom) cave locality (see Sierra de El Abra map, left) harbor an intermediate distribution of extraoral taste buds that clearly exceeds those in surface fish. Map scale = 15 km; redrawn from Schemmel (1967).
A key finding from our review is that gustation is enhanced in cavefish compared to surface fish at multiple levels of analysis (i.e., anatomical, morphological, developmental, physiological, behavioral and genetic; Figure 2A). The characterization of these differences in the literature, without an overarching context, has rendered it difficult to understand the adaptive relevance and inter-relationship between features. In the past several decades, research has mostly capitalized on a comparative paradigm wherein cavefish represent the derived morph and surface fish represent the (surrogate) ancestral morph – and the polarity of evolutionary change is clear (Jeffery, 2001). Notably, however, early classical studies did not utilize this paradigm, because cave morphs were regarded as members of their own, separate genus Anoptichthys (Breder and Rasquin, 1943), and contrasts were illustrated by comparison to closely related, or well-characterized, teleost species e.g., (see Humbach, 1960).
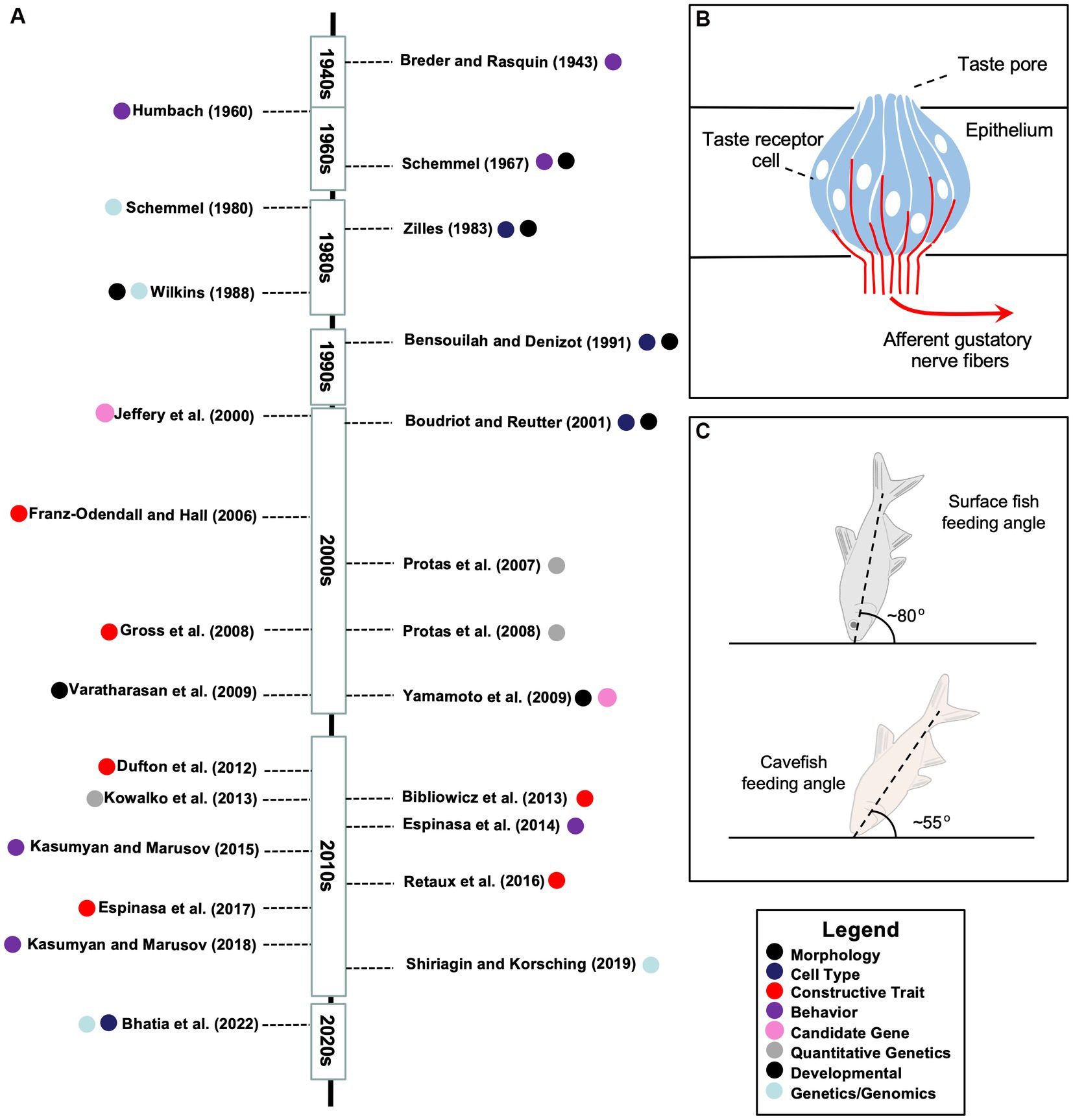
Figure 2. A timeline of research investigations and features of constructive taste evolution in the Mexican tetra. (A) A summary of research articles addressing taste system research across roughly seven decades. The topic of each study is indicated by one of eight principal categories determined by the authors (see Legend, bottom right). (B) A schematic representation of an extraoral taste bud labeled to show the apical taste pore, fusiform taste receptor cells (blue), and afferent gustatory nerve fibers (red). Note that taste buds can harbor between 50 and 150 cells, and include additional cell types (e.g., basal cells) not represented in this schematic. (C) A schematic representation of the different feeding postures of surface (top) and cave (bottom) morphs, which were reported to be ~80° and ~ 55°, respectively (see Schemmel, 1980).
Among the gustatory differences setting cavefish apart from other fish taxa include genetic differences focused on different features of relevance to gustation including threshold sensitivity and feeding behavior, perceptual differences principally based on heightened sensitivity (reduction in threshold sensitivity), anatomical differences which are mainly reflected in the numerical and distributional expansion of extraoral taste buds (see schematic; Figure 2B), and developmental differences relating to the timing of appearance (and number of taste buds) in cave and surface morphs. More recently, genomic differences, has been examined in the context of taste receptor genes between morphs, which may tie together some of the previously-listed categorical differences. The following short review of the historical and contemporary findings examines these categorical differences between Astyanax morphs.
Genetic analyses of the numerical and distributional expansion of taste buds
Schemmel (1967) was the first to report a profound expansion in the distribution of extraoral taste buds in cavefish (Figure 1). Although this work examined fish from two (of the ~30) natural cavefish populations, Pachón cavefish harbored substantially more than Sabinos cavefish (Schemmel, 1967; Rétaux et al., 2016). This work implied a conceptual link between the numerical expansion of peripheral taste buds and a heightened sensitivity to tastants. However, it should be noted that such a causative link between higher numbers of extraoral taste buds and a lower threshold of sensitivity has not been experimentally proven. Moreover, as suggested by Rétaux et al. (2016), these studies also did not discriminate between the probable contribution of olfaction to the enhanced detection sensitivity of cave morphs.
A later study, however, sought to connect the expansion of extraoral taste buds to food-finding behavior. Schemmel (1980) reported an angular difference in feeding between cave and surface morphs. Cavefish approach the bottom substrate at a ~55° angle, while surface fish (in the dark) tended to approach the substrate at a ~ 80° angle (Figure 2C). Using a classical genetics approach, this work concluded that the distributional expansion of taste buds and feeding angle are most likely unlinked, and mediated by complex (polygenic) genetic factors (Schemmel, 1980).
Studies by Wilkens (1988) expanded this classical genetic approach, reporting estimates of the number of genes implicated in taste bud expansion. This work revealed some diversity in the number and (qualitative) pattern of extraoral taste bud expansion among different cavefish populations (Wilkens, 1988). Using the approach of Lande (1981), the estimated number of genes implicated in extraoral expansion of taste buds was ~11–12, with the suspicion being that Pachón cavefish are homozygous for the genes involved in expansion (Schemmel, 1974; Wilkens 1988). This study, however, may have overestimated the number of taste buds by scoring solitary chemosensory cells alongside taste buds, which would likely inflate the number of estimated genes (see Wilkens et al., 2017). By at least one estimate, the number of loci involved in numerical expansion of taste buds may be closer to three, as reported by Protas et al. (2008).
Wilkens (1988) reported that the largest number of taste buds among studied cave populations was Pachón, with the number of taste buds on the ventral jaw exceeding those in the mouth by ~3- to 4-fold. Interestingly, the genetic architecture (i.e., estimate of the number of ‘genetic factors’) for Sabinos cavefish was comparable to Pachón cavefish, despite those fish harboring fewer taste buds (Wilkens, 1988). Cavefish derived from the more western Micos caves have taste bud numbers roughly intermediate between Pachón and Sabinos cavefish. An interesting feature described by Wilkens was that the larger the area of ventral head covered, the higher the density of taste buds. At present, the biological basis for this feature remains unexplained. In sum, Wilkens (1988) concluded that extraoral taste bud expansion is a cave-associated feature demonstrating an additively polygenic basis.
More recently, taste buds were scored as part of a larger genetic analysis examining the role for peripheral taste reception in feeding behavior. This study revisited postural differences in substrate approach by surface and cavefish, examining this feature using a quantitative trait locus (QTL) mapping approach. Kowalko et al. (2013) formally tested whether postural differences in feeding angle were associated with craniofacial morphology, body depth, and numerical expansion of taste buds. The authors examined correlations using experimental F2 individuals derived from both the Tinaja and Pachón caves (each crossed to surface fish), as well as examining co-localization of QTL in association mapping studies (Kowalko et al., 2013).
This work scored taste buds in members of an F2 pedigree, but did not identify a significant association between feeding posture and taste bud numbers. Interestingly, one score – ventral taste bud number – was positively correlated with feeding posture angle, but this association did not reach statistical significance (p = 0.051). Further, this finding did not conform to the prediction that an expanded number of taste buds would yield a more acute feeding angle. Rather, ventral expansion was associated with an increase in feeding angle (reminiscent of surface fish feeding postures). The authors concluded that the derived feeding posture in cavefish is unlikely related, at least directly, to taste bud expansion. Interestingly, this study revealed QTL positions associated with extraoral taste bud numbers were different for Pachón and Tinaja cavefish (Kowalko et al., 2013). Thus, extraoral taste bud expansion has likely evolved independently in these two cave populations, through non-overlapping regions of the genome.
The unclear source of perceptual differences in gustation between surface and cavefish
One of the first studies of the taste system in Astyanax appeared in the early 1940s focusing on heightened tastant detection in cave-dwelling morphs. Breder and Rasquin (1943) examined “chemosensory reactions” by comparing responses of river (i.e., “surface”) morphs and cave morphs to chemical “repellents.” Their anatomical observations revealed larger nasal pits in cave morphs, but they reported no differences in the number or morphology of taste organs (i.e., “taste buds”) between surface- and cave-dwelling morphs (presumably referring strictly to those taste buds in the oral cavity, see above). This study also did not formally discriminate between olfaction and taste, since chemical “repellents” included acetic and citric acid, as well as ammonium carbonate (Breder and Rasquin, 1943). Although these acids are predicted to stimulate sour perception - one of five canonical taste modalities, it is unclear if ammonia would excite bitter taste receptors, olfactory receptors, or both. Interestingly, they noted that acetic acid elicited repulsive behavior but citric acid did not (Breder and Rasquin, 1943). Although the avoidance reaction was comparable between morphotypes, cave morphs were regarded as having a “stronger” reaction, i.e., a reduced threshold of sensitivity for tastant detection. Individuals rendered anosmic lost their reaction to these chemicals, indicating (at least a partial) reliance on olfaction for chemical detection in their assay. In sum, this work provided early evidence for enhanced sensitivity to tastants in cavefish. Over a decade later, however, a similar study by Humbach (1960) reinforced the notion of enhanced taste sensitivity, showing that the bitter modality was ~300x more acute, and salty/acid/sweet were ~2000–4000× more acute, in cavefish compared to the common minnow (Phoxinus; Humbach, 1960).
Studies exploring taste sensitivity differences in Mexican tetra were first scored based on behavioral responses to different chemicals (Breder and Rasquin, 1943). A contemporary QTL mapping study similarly scored threshold sensitivity to “chemical sense.” Accordingly, amino acids dissolved in system water were detected at a much lower concentration in Pachón cavefish compared to surface fish (Protas et al., 2008). A potential mediator of this response could be the savory taste receptor, T1R1, which binds glutamate and mediates the canonical umami taste modality (Oike et al., 2007). However, since several amino acids were examined in this study, the potential participation of olfaction in this enhanced sensitivity cannot be ruled out.
Although the biological basis for this lower threshold of sensitivity has not yet been determined, Schemmel (1967) suggested it may be explained, at least in part, by a richer supply of neurotransmitters and/or neuromodulators in the taste receptors themselves. A study by Bensouilah and Denizot (1991) revealed that Substance P was present in cavefish taste buds, but not surface fish, suggesting this neurotransmitter may be involved in the elevated gustatory sensitivity in cave morphs. A later study by Jeffery (2001) identified expression of another molecule in taste buds using immunohistochemistry, Prox1, however this protein was expressed transiently during development. Interestingly, Prox1 expression persisted in cavefish taste buds until ~14 dpf, while its expression in mechanosensory neuromasts continued much longer. At present, the role of Prox1 in cavefish taste buds is unclear. However, rather than playing a role in signal transmission, this protein may be involved in early taste bud specification, as in mouse taste papillae where it is co-expressed during papillae formation alongside Shh (Nakayama et al., 2008).
Enhanced taste sensitivity may be due to the larger number of peripheral (extraoral) taste buds in cavefish. Humans with more taste papillae (i.e., epithelial specializations housing taste buds) and taste pores have a lower threshold for tastant detection (Miller, 1986; Segovia et al., 2002). Although this mechanism explaining heightened sensitivity is intuitive, it remains to be rigorously tested. Additionally, it is unclear how the numerical expansion of extraoral taste buds integrates with other characterized anatomical, molecular and genetic differences between morphotypes.
Anatomical differences of taste buds and beyond
Using scanning electron microscopy, Zilles et al. (1983) reported no obvious anatomical differences in the surface structure of taste buds between cavefish, surface fish and cave x surface hybrids (Zilles et al., 1983). Similarly, a study by Boudriot and Reutter (2001) using transmission electron microscopy found no obvious ultrastructural differences between taste buds of cave and surface morphs. Notably, however, this group did report that the afferent innervation of types II and III taste receptor cells in cavefish have a higher density of axons in cavefish compared to surface fish (Boudriot and Reutter, 2001). A later qualitative assessment of afferent nerves associated with taste buds during development supports the notion that cavefish taste buds have more axons compared to surface fish (Varatharasan et al., 2009). However, in contrast to findings from ultrastructural analyses, Varatharasan et al. (2009) reported more taste receptor cells in the taste buds of cavefish compared to surface fish. This analysis was restricted to taste buds within the oral cavity, so it is unknown if cavefish extraoral taste buds harbor similar taste receptor cell densities, or cell types, as those in the cavefish oral cavity.
In addition to differences at the periphery, it is known that in cavefish the telencephalon (which processes chemoreceptive modalities of taste and olfaction) is ~40% larger than surface fish (Peters et al., 1993; Franz-Odendaal and Hall, 2006). The extent to which this change impacts taste signaling alone, as opposed to both taste and olfaction, is currently unknown. In sum, although perceptual differences exist, the causative explanation for these differences between morphs remains unknown. A lower threshold for detection of tastants, in principle, could be a function of the increased numbers of taste buds in cavefish, the extraoral distribution of those taste buds, or molecular differences in cavefish taste buds that impact chemoreception. The perceptual differences for tastants between cave and surface morphs are most likely explained by complex interactions traversing these levels of molecular signaling, numerical expansion of taste buds, and higher cortical functions.
The developmental basis for taste system expansion
For years, a persistent belief in the literature was that constructive evolution of the taste system occurred as a “compensatory” mechanism (Schemmel, 1967; Zilles et al., 1983) to counteract loss of vision. Accordingly, in the complete absence of light, there is no visual input. To survive, animals must still obtain nutrition for which the taste system is expected to play a central role. Yamamoto et al. (2009) showed that sonic hedgehog may be the molecule that unites vision loss with gustatory (and mandibular) expansion across a critical period of development. Overexpression of sonic hedgehog at specific times during embryogenesis linked together eye degeneration with increased numbers of oral taste buds (Yamamoto et al., 2009). Consistent with this, F3 hybrids generated from surface x cavefish crosses showed an inverse relationship between taste bud numbers and eye sizes (Yamamoto et al., 2009). Collectively, this work suggested that elevated sonic signaling in cavefish increases taste bud numbers at the expense of eye development.
Other studies examining the timing of taste bud appearance have focused largely on oral taste buds, and their appearance up to about 3 weeks post-fertilization. Interestingly, at 5 days post-fertilization (dpf), there are minimal differences in the numbers of taste buds on the lower jaws of cave and surface morphs. By 22 dpf, however, cavefish had roughly three times the number of oral taste buds as surface fish (Varatharasan et al., 2009). Moreover, this numerical increase in taste buds is not uniform for the upper and lower jaws. Rather, by 22 dpf they observed the numerical expansion of taste buds was greater in the upper compared to the lower jaw (Varatharasan et al., 2009). These authors concluded the expansion is attributable to an acceleration in the rate of taste bud development in cavefish. They further suggested that this timing of expansion is coincident with the timing of eye degeneration – suggesting a potential link between these developmental processes. Finally, although the differences were modest, these authors found that cavefish harbored more cells per taste bud at all three stages of development when compared to surface fish (Varatharasan et al., 2009).
To examine the mechanistic link between eye regression and taste bud expansion, Dufton et al. (2012) performed experimental lens ablations (which lead to eye loss) and examined consequences on different sensory systems. Ablating the lens did not impact the number of taste buds on the jaw (Dufton et al., 2012). Further, Protas et al. (2008) found that eye size “was not significantly correlated” with the number of taste buds (as well as two measures of jaw size). Interestingly, this study showed that the lengths of the dentary and maxillary bones, and taste bud numbers, were significantly correlated (Protas et al., 2008). Thus, although there is evidence of a developmental interaction between vision loss and taste system expansion, the underlying genetic bases for these trait differences remain incompletely understood. Further, because the timing of numerical expansion of extraoral taste buds is unknown, the extent to which eye loss is associated with external taste bud expansion is unclear.
Genome-level analyses of gustation in Astyanax
A recent analysis of the genes encoding taste receptors in cavefish was performed following the release of the first draft cavefish genome (Shiriagin and Korsching, 2019). The Astyanax genome revealed a typical number of T1R gustatory genes (i.e., four) – which matched the orthologues in zebrafish. Interestingly, however, this study found that cavefish harbor a dramatic expansion of T2R genes which encode bitter receptors (Shiriagin and Korsching, 2019). Specifically, this study reported the presence of 24 T2R genes (three are pseudogenes) in the Pachón cavefish genome, which contrasts starkly with the typical numbers of T2R genes found in other teleosts, which is generally between 3 and 5 (Dong et al., 2009). This gene family expansion in cavefish was unexpected, as the TR gene repertoire is believed to be tuned to the nutritional environment. Since cavefish live amidst nutrient-poor conditions, the relevance (or value) of an expanded T2R bitter gene repertoire is unclear. Interestingly, a dN/dS analysis was suggestive of positive selection in two subclades of T2R bitter genes, however three other subclades did not show evidence of positive selection (Shiriagin and Korsching, 2019).
The authors estimated the T2R gene family expanded tens to hundreds of millions of years ago. This timing estimate would imply the bitter gene repertoire expanded prior to cave colonization (i.e., present in surface fish populations as well), thereby representing a ‘pre-adaptive’ trait (Shiriagin and Korsching, 2019). Importantly, at the time of publication a draft surface fish genome had not yet been completed. Thus, the ecological relevance of the T2R gene expansion is unclear, but the authors suggested it may endow cavefish with a broader bitter sense, which may facilitate avoidance of (bitter-tasting) toxic compounds in the cave.
A more recent publication utilizing an updated genome draft identified only 7 T2Rs in the cavefish genome, as opposed to 21 family members (Bhatia et al., 2022). Interestingly, however, a cell-based analysis showed that receptors encoded by four bitter genes (T2R1, T2R3, T2R4 and T2R114) are functional and responsive to compounds in fish food, as well as a bitter compound (quinine; Bhatia et al., 2022). Future studies examining the surface fish genome are anticipated to provide an important comparison and insight to the timing of the T2R gene family expansion, and the extent to which it is associated with the cave habitat.
Gaps in knowledge and areas of future focus
Although the value of an enhanced gustatory system is intuitive, for an organism adapted to total darkness, it must be stressed that the precise function of cavefish extraoral taste buds remains unclear and largely unexplored. Moreover, although expanded taste bud domains at the periphery is tempting to regard as adaptive, it is unknown if this re-positioning is perhaps a consequence of other evolutionary mechanisms (e.g., indirect pleiotropy). To avoid weak inferences regarding the adaptive significance of extraoral taste buds (see Gould and Lewontin, 1979) rigorous analyses of taste system function are necessary. Along these lines, one recent study argued that external taste buds are used for preliminary assessment of food items during random swimming or targeted searches for food (Kasumyan and Marusov, 2015). Extraoral taste buds thus carry an importance for determining whether to pursue (or avoid) a food item. This work evaluated the sensitivity of Astyanax cavefish to two different amino acids, L-glutamine and L-phenylalanine. At present, it is unclear the extent to which olfaction was implicated in their study since amino acids can excite olfactory receptors. It is interesting, however, to note that L-glutamine is preferred by cave morphs and may be specifically linked to extraoral taste sensitivity (Kasumyan and Marusov, 2015).
To gain a more comprehensive understanding of the constructive evolution of taste, we identified several areas of opportunity. First, with respect to taste perception, it will be important to design studies that discriminate between gustatory and olfactory sensation. Well-designed olfaction studies have been performed in cavefish (Bibliowicz et al., 2013), and similar studies are needed for gustation. With respect to extraoral taste buds, a broader survey of taste bud numbers and distributions will be useful for determining if this feature is uniformly associated with cave life, and how these distributions compare across different populations. Further, presence (and large numbers of) extraoral taste buds may predictably lower the threshold for tastant sensitivity – such a relationship has never been experimentally established. Therefore, studies examining how numerical diversity of taste buds on the external body facilitate food-finding in cavefish will be essential. Relatedly, a remaining unsolved mystery is why cavefish harbor a different feeding posture, if this derived posture does not relate to the expanded spatial domain of extraoral taste buds?
One potential solution to this mystery is an explicit analysis of the genetic architecture (and genetic bases) for the numerical expansion of taste buds. Classic studies revealed a genetic component for these features (Schemmel, 1980; Wilkens, 1988), therefore contemporary genetics techniques may hold promise for identifying the gene(s) responsible for divergent extraoral taste bud phenotypes. How do these genetic changes differ between different populations, such as Pachón and Tinaja?
It is unclear how the expansion of extraoral taste buds at the periphery is involved in the lower threshold for sensitivity to tastants. More broadly, it is unclear how molecular differences in cavefish taste buds (e.g., Prox1, Substance P), neuroanatomical differences (e.g., higher axon density at the TBs, larger telencephalon) are potentially implicated in the elevated sensitivity to tastants.
From a developmental standpoint, a major unanswered question is what developmental process mediates the expanded numbers of taste buds in cavefish. Some work suggests a heterochronic shift in the development of oral taste buds (Varatharasan et al., 2009), but what explains the dramatically increased number and distribution of extraoral taste buds? What is the life history timing of this expansion? Is the expansion of taste bud numbers linked to vision loss, as suggested in the literature (Zilles et al., 1983)? If so, how does this process relate to extraoral taste bud expansion, which may occur over a much more protracted period of life history?
Finally, from a genomic perspective, what is the bitter taste receptor gene family (T2R genes) representation in the recently updated surface fish genome? The absence of this information necessarily limits our ability to infer the evolutionary mechanism(s) mediating this genomic feature. Moreover, taste receptor structure and expression are purportedly tuned to the nutritional environment. Thus, are there numerical and/or structural differences are in taste receptors of cave and surface fish, given that they occupy such different (nutritional) environments? Identifying such differences may be key to discerning how the taste system evolves in natural environments differing markedly in their nutrient composition.
Conclusion
Gustatory expansion is a commonly regarded example of constructive evolution in Astyanax cavefish. Owing to a robust comparative paradigm, several differences have been identified between cave and surface morphs over the past ~90 years (Figure 2A). The question of how these differences inter-relate to yield adaptive improvements to taste sensation remain unclear. Certain assumptions, e.g., relating external taste bud expansion to feeding posture differences, are not supported by contemporary results. Future work examining diverse knowledge gaps hold promise for understanding how the differences in taste identified between cave and surface fish ultimately confer an adaptive advantage in the subterranean environment. This work will provide insights to both the evolution of gustation in this fascinating system, but also provide key insights to the broader nature of constructive trait evolution.
Author contributions
DB and JBG conceived the project, wrote and prepared the manuscript, and reviewed and summarized the primary literature. All authors contributed to the article and approved the submitted version.
Funding
DB was supported by a summer research fellowship from the University of Cincinnati URC Fellowship program. JBG is supported by a grant from the NSF (IOS-2205928).
Acknowledgments
The authors wish to thank members of the Gross lab for useful discussions of this manuscript. The authors wish to thank two reviewers, and the editors of the special issue, “Adaptations to Subterranean Environments,” for helpful comments on an earlier draft of this manuscript.
Conflict of interest
The authors declare that the research was conducted in the absence of any commercial or financial relationships that could be construed as a potential conflict of interest.
Publisher’s note
All claims expressed in this article are solely those of the authors and do not necessarily represent those of their affiliated organizations, or those of the publisher, the editors and the reviewers. Any product that may be evaluated in this article, or claim that may be made by its manufacturer, is not guaranteed or endorsed by the publisher.
References
Bensouilah, M., and Denizot, J. P. (1991). Taste buds and neuromasts of Astyanax jordani: distribution and immunochemical demonstration of co‐localized substance P and enkephalins. Eur. J. Neurosci. 3, 407–414. doi: 10.1111/j.1460-9568.1991.tb00828.x
Bhatia, V., de Jesus, V. C., Shaik, F. A., Jaggupilli, A., Singh, N., Chelikani, P., et al. (2022). Extraoral expression and characterization of bitter taste receptors in Astyanax mexicanus (Mexican tetra fish). FASEB BioAdv. 4, 574–584. doi: 10.1096/fba.2022-00032
Bibliowicz, J., Alie, A., Espinasa, L., Yoshizawa, M., Blin, M., Hinaux, H., et al. (2013). Differences in chemosensory response between eyed and eyeless Astyanax mexicanus of the Rio Subterraneo cave. Evodevo 4:25. doi: 10.1186/2041-9139-4-25
Boudriot, F., and Reutter, K. (2001). Ultrastructure of the taste buds in the blind cave fish Astyanax jordani (“Anoptichthys”) and the sighted river fish Astyanax mexicanus (Teleostei, Characidae). J. Comp. Neurol. 434, 428–444. doi: 10.1002/cne.1185
Breder, C. M., and Rasquin, P. (1943). Chemical sensory reactions in the Mexican blind characins. Zoologica 28, 169–200.
Dezfuli, B. S., Magosso, S., Simoni, E., Hills, K., and Berti, R. (2009). Ultrastructure and distribution of superficial neuromasts of blind cavefish, Phreatichthys andruzzii, juveniles. Microsc. Res. Tech. 72, 665–671. doi: 10.1002/jemt.20714
Dong, D., Jones, G., and Zhang, S. (2009). Dynamic evolution of bitter taste receptor genes in vertebrates. BMC Evol. Biol. 9, 1–9. doi: 10.1186/1471-2148-9-12
Dufton, M., Hall, B. K., and Franz-Odendaal, T. A. (2012). Early lens ablation causes dramatic long-term effects on the shape of bones in the craniofacial skeleton of Astyanax mexicanus. PLoS One 7:e50308. doi: 10.1371/journal.pone.0050308
Franz-Odendaal, T., and Hall, B. K. (2006). Modularity and sense organs in the blind cavefish, Astyanax mexicanus. Evol. Dev. 8, 94–100. doi: 10.1111/j.1525-142X.2006.05078.x
Gould, S. J., and Lewontin, R. C. (1979). The spandrels of San Marco and the Panglossian paradigm: a critique of the adaptationist programme. Proc. R. Soc. Lond. B Biol. Sci. 205, 581–598. doi: 10.1098/rspb.1979.0086
Hara, T. J. (1994). Olfaction and gustation in fish: an overview. Acta Physiol. Scand. 152, 207–217. doi: 10.1111/j.1748-1716.1994.tb09800.x
Haspel, G., Schwartz, A., Streets, A., Camacho, D. E., and Soares, D. (2012). By the teeth of their skin, cavefish find their way. Curr. Biol. 22, R629–R630. doi: 10.1016/j.cub.2012.06.035
Hill, L. G. (1969). Feeding and food habits of the spring cavefish, Chologaster agassizi. Am. Midl. Nat. 82, 110–116. doi: 10.2307/2423821
Humbach, I. (1960). Geruch und Geschmack bei den augenlosen Höhlenfischen Anoptichthys jordani, Hubbs und Innes und Anoptichthys hubbsi, Alvarez. Naturwissenschaften 47:551.
Jeffery, W. R. (2001). Cavefish as a model system in evolutionary developmental biology. Dev. Biol. 231, 1–12. doi: 10.1006/dbio.2000.0121
Jonz, M. G., Zachar, P. C., Da Fonte, D. F., and Mierzwa, A. S. (2015). Peripheral chemoreceptors in fish: a brief history and a look ahead. Comp. Biochem. Physiol. A Mol. Integr. Physiol. 186, 27–38. doi: 10.1016/j.cbpa.2014.09.002
Kasumyan, A. O. (2019). The taste system in fishes and the effects of environmental variables. J. Fish Biol. 95, 155–178. doi: 10.1111/jfb.13940
Kasumyan, A. O., and Marusov, E. A. (2015). Chemoorientation in the feeding behavior of the blind Mexican cavefish Astyanax fasciatus (Characidae, Teleostei). Russ. J. Ecol. 46, 559–563. doi: 10.1134/S1067413615060053
Kowalko, J. E., Rohner, N., Linden, T. A., Rompani, S. B., Warren, W. C., Borowsky, R., et al. (2013). Convergence in feeding posture occurs through different genetic loci in independently evolved cave populations of Astyanax mexicanus. Proc. Natl. Acad. Sci. 110, 16933–16938. doi: 10.1073/pnas.1317192110
Lande, R. (1981). The minimum number of genes contributing to quantitative variation between and within populations. Genetics 99, 541–553. doi: 10.1093/genetics/99.3-4.541
Ma, L., Zhao, Y., and Yang, J.X. (2019). Cavefish of China. In Encyclopedia of caves White, BW, and Culver, DC (pp. 237–254). Cambridge, MA Academic Press.
Miller, I. J. Jr. (1986). Variation in human fungiform taste bud densities among regions and subjects. Anat. Rec. 216, 474–482. doi: 10.1002/ar.1092160404
Moran, R. L., Richards, E. J., Ornelas-Garcia, C. P., Gross, J. B., and Donny, A. (2023). Selection-driven trait loss in independently evolved cavefish populations. Nat. Commun. 14:2557. doi: 10.1101/2022.11.28.518185
Nakayama, A., Miura, H., Shindo, Y., Kusakabe, Y., Tomonari, H., and Harada, S. (2008). Expression of the basal cell markers of taste buds in the anterior tongue and soft palate of the mouse embryo. J. Comp. Neurol. 509, 211–224. doi: 10.1002/cne.21738
Oike, H., Nagai, T., Furuyama, A., Okada, S., Aihara, Y., Ishimaru, Y., et al. (2007). Characterization of ligands for fish taste receptors. J. Neurosci. 27, 5584–5592. doi: 10.1523/JNEUROSCI.0651-07.2007
Parzefall, J. (2001). A review of morphological and behavioural changes in the cave molly, Poecilia mexicana, from Tabasco. Mex. Biol. Hypogean Fish. 62, 263–275. doi: 10.1007/978-94-015-9795-1_23
Peters, V. N., Schacht, V., Schmidt, W., and Wilkens, H. (1993). Gehirnproportionen und Ausprägungsgrad der Sinnesorgane von Astyanax mexicanus (Pisces Characinidae): ein Vergleich zwischen dem Flussfisch und seinen Höhlenderivaten “Anoptichthys”. Z. Zool. Syst. Evol. 31, 144–159.
Protas, M., Tabansky, I., Conrad, M., Gross, J. B., Vidal, O., Tabin, C. J., et al. (2008). Multi-trait evolution in a cave fish, Astyanax mexicanus. Evol. Dev. 10, 196–209. doi: 10.1111/j.1525-142X.2008.00227.x
Rétaux, S., Alié, A., Blin, M., Devos, L., Elipot, Y., and Hinaux, H. (2016). Neural development and evolution in Astyanax mexicanus: comparing cavefish and surface fish brains. New York: Elsevier, Academic Press.
Reutter, K. (1986). Chemoreceptors. Biology of the Integument: 2 Vertebrates. (Bereiter-Hahn): Heidelberg Springer-Verlag. 586–604.
Schemmel, C. (1967). Comparative studies of the cutaneous sense organs in epigean and hypogean forms of Astyanax with regard to the evolution of cavernicoles. [Vergleichende Untersuchungen an den Hautsinnesorganen ober-und unterirdisch lebender Astyanax-Formen-Ein Beitrag zur evolution der Cavernicolen]. Z. Morph. Tiere 61, 255–316.
Schemmel, C. (1974). Genetische Untersuchungen zur Evolution des Geschmacksapparates bei cavernicolen Fischen. Z. Zool. Syst. Evolutionforsch. 12, 196–215. doi: 10.1111/j.1439-0469.1974.tb00165.x
Schemmel, C. (1980). Studies on the genetics of feeding behaviour in the cave fish Astyanax mexicanus f. Anoptichthys. An example of apparent monofactorial inheritance by polygenes. Z. Tierpsychol. 53, 9–22. doi: 10.1111/j.1439-0310.1980.tb00730.x
Segovia, C., Hutchinson, I., Laing, D. G., and Jinks, A. L. (2002). A quantitative study of fungiform papillae and taste pore density in adults and children. Dev. Brain Res. 138, 135–146. doi: 10.1016/s0165-3806(02)00463-7
Shiriagin, V., and Korsching, S. I. (2019). Massive expansion of bitter taste receptors in blind cavefish, Astyanax mexicanus. Chem. Senses 44, 23–32. doi: 10.1093/chemse/bjy062
Varatharasan, N., Croll, R. P., and Franz-Odendaal, T. (2009). Taste bud development and patterning in sighted and blind morphs of Astyanax mexicanus. Dev. Dyn. 238, 3056–3064. doi: 10.1002/dvdy.22144
Wilkens, H. (1988). “Evolution and genetics of epigean and cave Astyanax fasciatus (Characidae, Pisces): support for the neutral mutation theory” in Evolutionary Biology. eds. M. K. Hecht and B. Wallace (New York: Plenum Publishing), 271–367.
Wilkens, H., and Strecker, U. (2017). Regressive and constructive traits in Astyanax surface and cave fish. In Evolution in the Dark: Darwin’s Loss Without Selection, Springer: Wilkens and Strecker Heidelberg. 79–189. doi: 10.1007/978-3-662-54512-6_6
Yamamoto, Y., Byerly, M. S., Jackman, W. R., and Jeffery, W. R. (2009). Pleiotropic functions of embryonic sonic hedgehog expression link jaw and taste bud amplification with eye loss during cavefish evolution. Dev. Biol. 330, 200–211. doi: 10.1016/j.ydbio.2009.03.003
Yang, J., Chen, X., Bai, J., Fang, D., Qiu, Y., Jiang, W., et al. (2016). The Sinocyclocheilus cavefish genome provides insights into cave adaptation. BMC Biol. 14, 1–13. doi: 10.1186/s12915-015-0223-4
Zilles, K., Tillmann, B., and Bennemann, R. (1983). The development of the eye in Astyanax mexicanus (Characidae, Pisces), its blind cave derivative, Anoptichthys jordani (Characidae, Pisces), and their crossbreds: a scanning and transmission-electron microscopic study. Cell Tissue Res. 229, 423–432. doi: 10.1007/BF00214983
Keywords: gustatory, taste buds, taste receptor cells, chemical sensation, subterranean
Citation: Berning D and Gross JB (2023) The constructive evolution of taste in Astyanax cavefish: A review. Front. Ecol. Evol. 11:1177532. doi: 10.3389/fevo.2023.1177532
Edited by:
Helena Bilandzija, Rudjer Boskovic Institute, CroatiaReviewed by:
Michael Tobler, Kansas State University, United StatesYoshiyuki Yamamoto, University College London, United Kingdom
Copyright © 2023 Berning and Gross. This is an open-access article distributed under the terms of the Creative Commons Attribution License (CC BY). The use, distribution or reproduction in other forums is permitted, provided the original author(s) and the copyright owner(s) are credited and that the original publication in this journal is cited, in accordance with accepted academic practice. No use, distribution or reproduction is permitted which does not comply with these terms.
*Correspondence: Joshua B. Gross, Z3Jvc3NqYUB1Y21haWwudWMuZWR1