- 1School of Environmental and Life Sciences, The University of Newcastle, Ourimbah, NSW, Australia
- 2School of Biological, Earth and Environmental Science, The University of New South Wales, Randwick, NSW, Australia
Coral disease prevalence has significantly increased under a changing climate, impacting coral community structure and functionality. The impacts and ecology of coral diseases are unclear in most high-latitude reefs (coral reefs above 28° north and below 28° south). High-latitude locations are vulnerable to climate change; therefore, identifying diseases and developing region-specific baselines are important for local management. We report the first coral disease findings at the UNESCO World Heritage listed Lord Howe Island Marine Park (31.5°S, 159°E), the southernmost coral reef system. This study assessed coral disease prevalence during November 2018, March 2019 and October 2019. Surveys from three lagoonal reefs identified four coral diseases: white syndrome, skeletal eroding band, growth anomalies and endolithic hypermycosis impacting six coral taxa (Acropora, Isopora, Montipora, Pocillopora, Porites and Seriatopora). Overall, disease prevalence was 5 ± 1% and significantly differed between time and site. Disease prevalence was highest in November 2018 (10 ± 1%) and significantly lower during March 2019 (5 ± 1%), coinciding with a bleaching event. White syndrome was the most prevalent disease (4 ± 1%) with 83 colonies of six taxa affected, predominately Isopora. Acroporids recorded the highest disease susceptibility, with three of the four diseases observed. Documenting baseline coral disease prevalence and monitoring throughout a bleaching event assists our understanding of disease ecology dynamics under current climate change impacts at high-latitude reefs.
1 Introduction
The Anthropocene is characterised by a global degradation of coral reefs (Pratchett et al., 2014; Hughes et al., 2018). The widespread degradation of coral reefs is due to local-scale stressors such as water pollution, habitat degradation, invasive species domination and overfishing (Wilkinson, 2000; Carpenter et al., 2008) and global scale stressors resulting from climate change (Hoegh-Guldberg et al., 2007). Rising ocean temperatures have led to a notable surge in both coral bleaching incidents (Sheppard, 2003; Hughes et al., 2017a; Hughes et al., 2017b; Frölicher and Laufkötter, 2018; Lough et al., 2018; Sully et al., 2019), and coral disease prevalence on coral reefs (Bruno et al., 2007; Harvell et al., 2007; Ruiz-Moreno et al., 2012; Maynard et al., 2015).
Coral disease outbreaks are projected to escalate on a global scale due to mounting local and global pressures. These pressures are expected to enhance pathogen virulence, expand the pathogen’s range, and prolong its survival (Maynard et al., 2015). Moreover, these stressors contribute to heightened coral susceptibility to diseases, reduced immune function, and the emergence of previously unseen and opportunistic pathogens within the coral reef environment. Coral diseases are becoming increasingly widespread due to various non-biological stressors, such as elevated water temperatures (Aeby et al., 2020), sunlight light intensity (Muller and van Woesik, 2009), pollution (Redding et al., 2013; Page et al., 2023), sedimentation (Gintert et al., 2019), degraded water quality (Thompson et al., 2014; Yoshioka et al., 2016), plastic pollution (Lamb et al., 2018), in-water recreational activities (Lamb et al., 2014; Hein et al., 2015), proliferation of algae and their spatial distribution (Nugues et al., 2004; Smith et al., 2006; Sweet et al., 2013; Casey et al., 2014), and eutrophication (Bruno et al., 2003; Vega Thurber et al., 2014). These disease outbreaks and occurrences pose a significant threat to coral reefs, as they lead to a decline in coral fitness recorded as: reduced growth (Meesters et al., 1994; Nugues, 2002; Stimson, 2011), decreased fecundity (Weil et al., 2009; Stimson, 2011; Palmer and Baird, 2018), and increased mortality (Miller et al., 2009; Precht et al., 2016), consequently disrupting ecosystem function (Nugues, 2002; Haapkylä et al., 2013). Coral diseases have now gained recognition as one of the most critical environmental issues affecting the Indo-Pacific region, demanding urgent conservation attention (Sutherland et al., 2015). The preservation of coral reefs is of paramount importance to safeguard marine biodiversity and ecosystem health.
Coral disease was first recorded in the Caribbean in the 1970s (Antonius, 1976). New coral diseases have since been identified (Richardson, 1998; Rosenberg and Loya, 2013) with coral diseases recorded beyond the Caribbean and across all major coral reef ecosystems, including reefs of the Indo-Pacific (Willis et al., 2004; Myers and Raymundo, 2009; Page and Stoddart, 2010; Montano et al., 2012; Schleyer et al., 2018; Subhan et al., 2020). On the Great Barrier Reef (GBR), coral disease prevalence has been routinely monitored through the Long-Term Monitoring Program (LTMP) (Willis et al., 2004), with at least nine coral diseases identified, including the first description of “white syndrome” a term used to describe unidentified coral diseases showing signs of rapid tissue loss resulting in white regions of exposed coral skeleton. Other diseases include atramentous necrosis, black band disease, brown band disease, growth anomalies, pink spot, skeletal eroding band, ulcerative white spot syndrome, white syndrome, and white blotch disease (Jones et al., 2004; Willis et al., 2004; Bourne, 2005; Haapkylä et al., 2010). As such, studies on coral disease have expanded 50 years, but overall, coral diseases have remained poorly understood, with ambiguity in both disease identification and diagnostics (Richardson, 1998; Work and Aeby, 2006; Work et al., 2008c; Moriarty et al., 2020). Similarly, while coral disease records have become more common throughout tropical reef ecosystems, and recordings of coral diseases from subtropical high-latitude reefs (coral reefs located below 28° south and above 28° north.) (Dalton and Smith, 2006; Dalton et al., 2010; Schleyer et al., 2018), coral disease monitoring efforts have been patchy through time and space across Australia’s high-latitude coral reefs.
The absence of comprehensive historical long-term data on disease identification, ecology and prevalence in high-latitude areas has made it difficult for researchers to interpret baseline surveys accurately representing disease prevalence in the area or potential outbreak numbers across high-latitude coral reefs. The reefs of Florida, USA are commonly referred to as high-latitude coral reefs and are where coral disease monitoring efforts have continued for over four decades (Richardson, 1998; Rosenberg and Loya, 2013). In 2014 a new disease, Stony Coral Tissue Loss Disease (SCTLD), was reported on the reefs of Florida (Precht et al., 2016), with the prevalence of the disease as high as 61%, causing significant mortalities to boulder coral morphologies reducing coral population densities to < 3% (Precht et al., 2016). STLD has spread across reefs in the region and is now prolific in the wider Caribbean (Alvarez-Filip et al., 2019; Weil et al., 2019). In comparison, other high-latitude reefs have had fewer extensive coral disease studies and report substantially less disease prevalence, for example, South Africa’s Sodwana Bay 1.9–5.7% (Schleyer et al., 2018), the Northwestern Hawaiian Islands 0–7.09% (Aeby, 2006) and Eastern Australia’s Solitary Islands 6.21–13.58% (Dalton and Smith, 2006). In 2003, the subtropical Solitary Islands experienced a concerning surge in the prevalence of a unique white syndrome, which had a significant impact on six common taxa of coral: Pocillopora, Stylophora, Turbinaria, Acropora, Goniastrea and Porites (Dalton and Smith, 2006; Dalton et al., 2010). The disease led to coral mortality, affecting approximately 27% of the colonies that were afflicted (Dalton and Smith, 2006). LHI’s neighbouring high-latitude island, Norfolk Island is about 570 km north east of LHI, with similar coral taxa (Moriarty pers. obs.). In 2020, a serious outbreak of a white syndrome coral disease was triggered by heat stress and pollution events affecting the reef-building taxa Montipora spp. in the Norfolk Island lagoon (Page et al., 2023). During the outbreak, around 60% of the Montipora community had been impacted, and the disease severity increased by 54% during this period (Page et al., 2023). The spatial distribution of the disease suggested a strong association with exposure to poor water quality and the size class of coral colonies (Page et al., 2023). This highlights the vulnerability of coral reef ecosystem to the combined impacts of warming and pollution.
High-latitude reefs experience both lower water temperatures and light in comparison to their tropical coral reef counterparts. High-latitude coral reef ecosystems are widely reported as high-value ecosystems, and potentially protected from the most extreme impacts of climate change due to the reef’s geographic location at the fringes of coral reef growth. These high-latitude coral reefs have been hypothesised as sites of refuge (Riegl, 2003), potentially providing resilience from warming ocean temperatures (Pandolfi et al., 2011). High-latitude reefs in Australia and Japan have also already experienced the expansion of tropical corals moving poleward, suggesting these marginal reef locations are at risk of both loss of endemics and habitat expansion of tropical species (Yamano et al., 2011; Baird et al., 2012). In essence, high-latitude coral reefs are now recognised as at risk from local and global stressors, species invasions, and associated impacts to the coral populations, such as the emergence of coral disease. Here we surveyed the World Heritage Listed coral reef lagoon of Lord Howe Island (LHI) Marine Park from November 2018 to October 2019 to identify the occurrence and prevalence of coral disease at the world’s southernmost coral reef system. We also report a disease outbreak and disease occurrence during a coral bleaching event, and in doing so, aim to provide a comprehensive description of coral disease ecology as a baseline for ongoing coral disease monitoring within the LHI Marine Park.
2 Methods
2.1 Study site
This study was undertaken under the Department of Primary Industries LHI Marine Park Research permit number: LHIMP/R/18015/12112018. The lagoonal coral reef of Lord Howe Island (31.5°S, 159°E, Figures 1A, B) was surveyed for benthic composition and disease prevalence in November 2018, March 2019, and October 2019, at three sites Coral Gardens, Erscotts and North Bay (Figure 1B). Lord Howe Island experiences tropical cyclones and thermal anomaly events (Steinberg et al., 2022; Moriarty et al., 2023). There have been four recorded mass bleaching events recorded since 1998, resulting in mass mortality and coral genera compositional changes (Harrison et al., 2011; Dalton et al., 2020; Steinberg et al., 2022). Environmental conditions vary across the reef sites, with Erscotts experiencing high wave action and tidal influence, which is also evident at Coral Gardens, whilst North Bay is a protected and sheltered bay (Figure 1B). The three sites are also situated in three different protection zones within the marine protected area (MPA), North Bay inside the fully protected sanctuary zone (SZ), Erscotts is located in the LHI lagoon SZ and Coral Gardens is situated inside of the habitat protection zone HPZ.
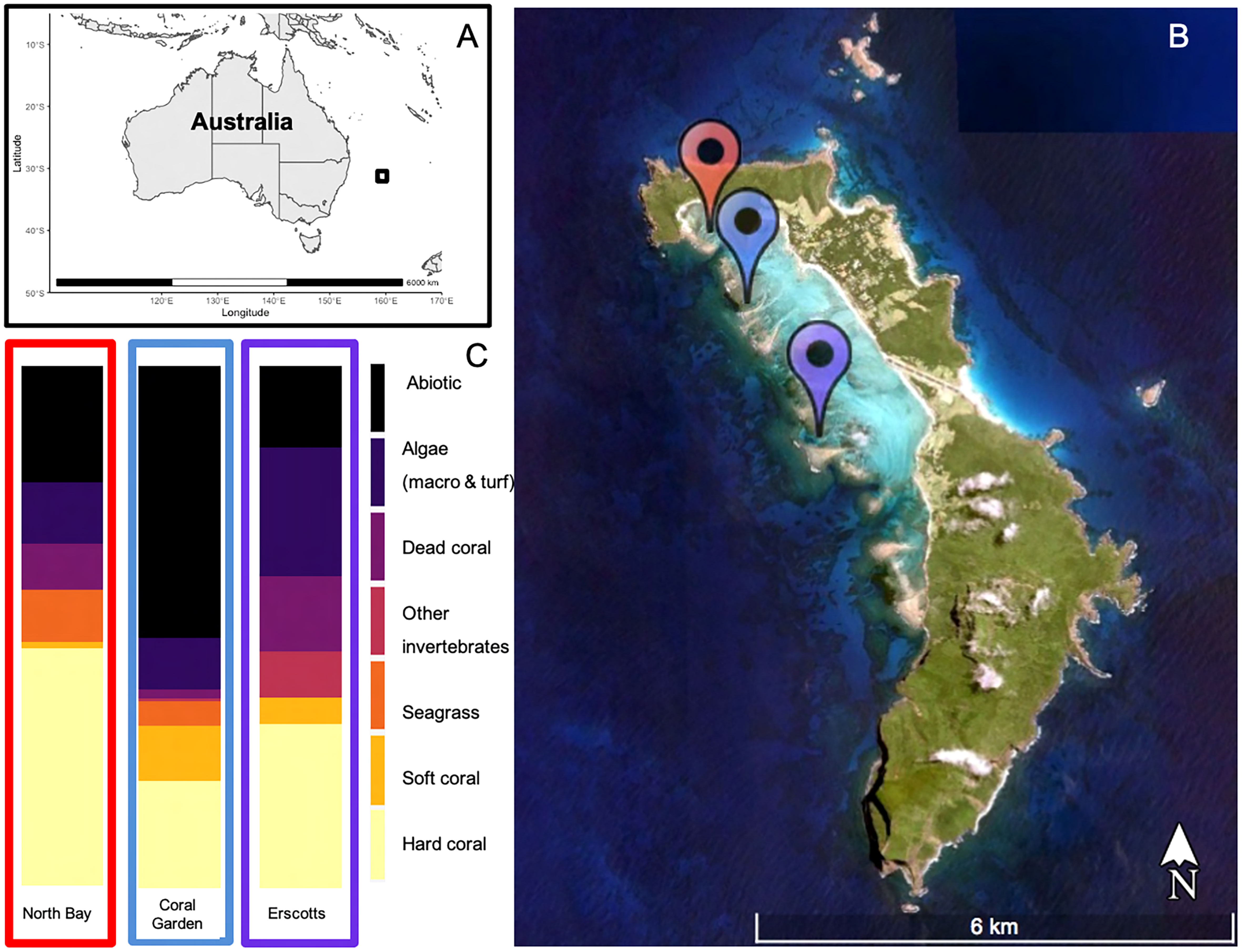
Figure 1 Lord Howe Island (black square) located ~600 km off the east coast of mainland Australia (A). The three study sites were located in the LHI lagoon, North Bay (Red), Coral Gardens (Blue) and Erscotts Reef (Purple) (B) and their respective benthic compositions during November 2018 (C).
2.2 Belt transects
Transect tape was laid haphazardly parallel to shore at one depth interval of 0–5 m. Three 1 m × 20 m belt transects were conducted at each of the three sites, North Bay, Erscotts and Coral Gardens. Every hard coral colony within the three 1 m × 20 m belt transects were classified as categorical conditions as healthy or unhealthy based on typical disease signs. Unhealthy colonies were further assessed for the seven varying compromised health categories: diseased, bleached, injured, predation, dead, partially dead, and competition (Figure 2). The diseased coral colonies were further classified by disease and the characteristics of the disease recorded, including the percentage of the coral colony affected, the location of the unhealthy section on the colony, shape of lesion, structure affected, lesion description (for example, discolouration, tissue loss, growth) (Figure 2). The coral colonies were identified to species level where possible along with their respective growth form (branching, sub-massive, encrusting, massive, tabular, staghorn and digitate). Coral diseases were identified using visual classification and samples taken for further disease examination later. Visual disease identification was made using distinguishing features as seen in Moriarty et al. (2020).
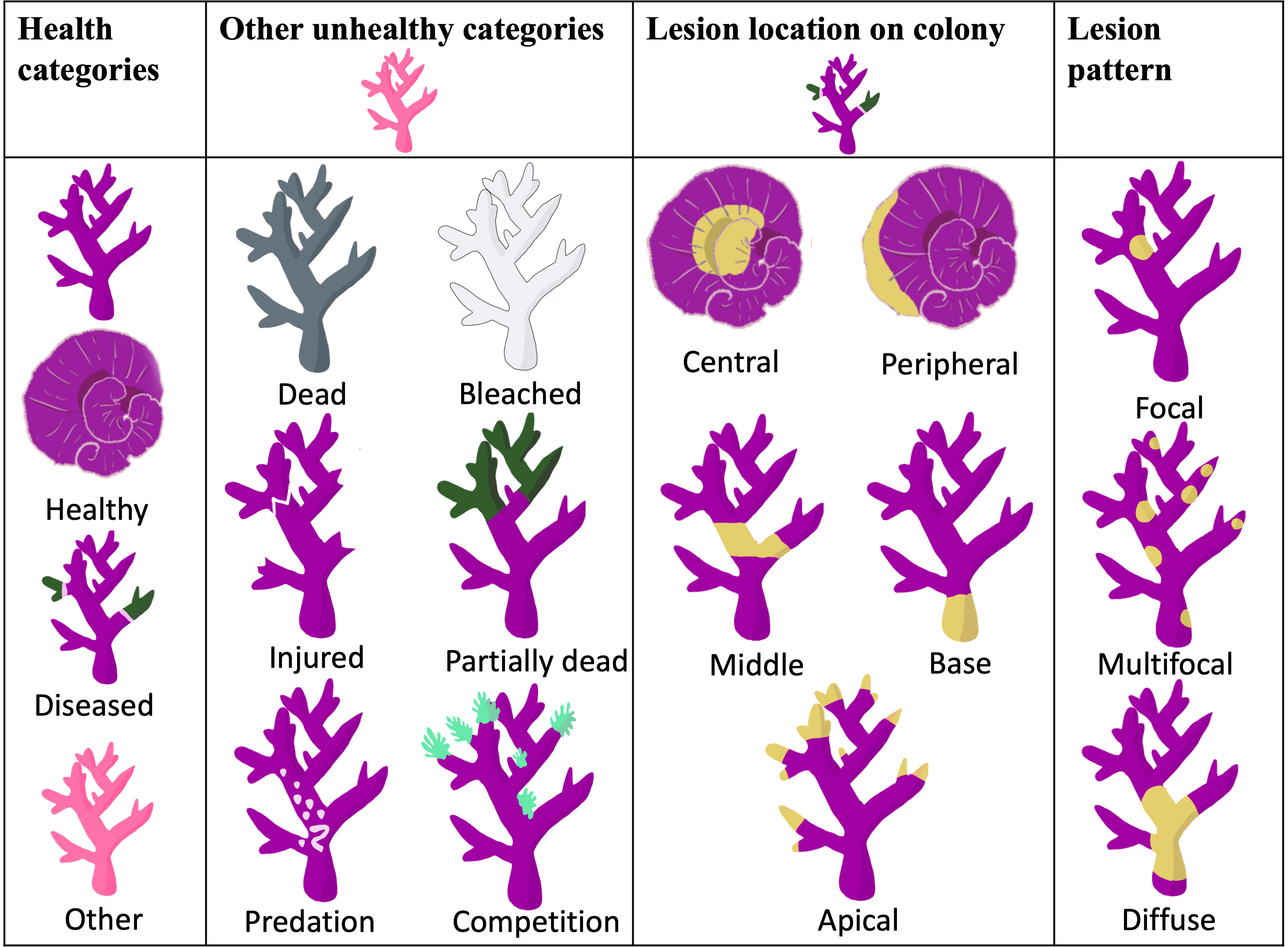
Figure 2 Health categories were assigned to the coral colonies surveyed within the belt transects at three distinct sites (see Figure 1). The classification of lesions on diseased corals was based on both the lesion's location on the colony and the specific pattern it exhibited. These criteria for lesion location and pattern have been adapted from the research conducted by Work and Aeby in 2006.
2.3 Benthic composition
Images were collected from the tape measure laid out for the belt transects using a Nikon COOLPIX W300 underwater camera. Three 1 m2 photo quadrats were taken along each of the three transects per site at 4–5 m, 12–13 m and 16–17 m. The images were cropped to exhibit only the benthos within the quadrat and then uploaded to the web-based annotation program CoralNet (coralnet.ucsd.edu). Photographs were overlaid with 64 fixed points, which were analysed to species and functional groups (Table 1). Total live coral cover was recorded to genera and when possible species level.
2.4 Statistical analysis
Disease prevalence and health categories were determined by calculating the proportion of damaged/visually unhealthy colonies compared to the total number of coral colonies:
Prevalence was calculated for each: monitored month, site, taxa, and coral disease. All statistical analysis was carried out in R version 3.6.2. Benthic composition was calculated by counting the number of each of the benthic categories observed and dividing it by the number of total counts per transect and then averaging the 3 transects to get the site benthic composition. The benthic composition was compared between sites (Sylph’s Hole, North Bay and Coral Garden) using a one-way ANOVA and normality tests performed. To investigate significant effects a Tukey’s post hoc analysis was conducted within each site and each benthic composition group. Disease prevalence was assessed using generalized linear mixed effects models (GLMM) in R using the package “glmmTMB” v1.0.1 for 1) health categories within sites, between months, and between sites within months and 2) within health categories for coral taxa within sites between months and between sites within months. Site and month were fixed variables, and transect was used as a random effect. A Poisson and binomial distribution were used, and best fit applied. Residual plots were visually inspected for appropriate fit with regards to dependency structure and deviations from homoscedasticity or normality. p-Values were calculated by likelihood-ratio tests and were considered significant using a confidence interval of 95% (alpha level = p ≤ 0.05).
3 Results
3.1 Benthic composition
A total of 27 photo quadrats were collected from each site of the three monitoring months. The overall average hard coral cover at LHI was 33 ± 6%, average abiotic 30 ± 6%, average algae 16 ± 4%, average dead hard coral 8 ± 4%, average soft coral 6 ± 3%, seagrass 5 ± 4%. North Bay, Erscotts and Coral Gardens were dominated by hard coral (46 ± 13%, 31 ± 8% and 52 ± 11% respectively, Figure 1C), abiotic benthos (22 ± 9%, 16 ± 8% and 52 ± 11% respectively, Figure 1C) and algae (12 ± 8%, 25 ± 8% and 10 ± 5% respectively, Figure 1C). Soft coral contributed 11 ± 10% at Coral Gardens and only 1 ± 1% and 5 ± 3% at North Bay and Erscotts, respectively. The benthic composition was not significantly different between the sites (p > 0.05, Figure 1C).
3.2 Coral assemblage
Porites spp., Pocillopora damicornis, Stylophora pistillata, Seriatopora hystrix and Isopora cuneata were the most abundant corals between all sites and monitored months (Figure 3). Coral assemblages within site and taxa did not change significantly over time (p > 0.05; Figure 3A). Overall, the least abundant coral taxa at all three sites were Acropora spp. In comparison, P. damicornis had the highest individual number of coral colonies relative abundance of all coral taxa at Coral Gardens and Erscotts. Porites spp. was the highest relative abundant taxa at North Bay (Figure 3A). Acropora had the least amount of individual coral colonies between sites and months ranging from 1 ± 0.2% SE to 7 ± 3% relative abundance in comparison to P. damicornis, which had the highest relative abundance (17 ± 5% to 48 ± 7%; Figure 3A). Acropora spp. colonies recorded were mostly arborescent, staghorn and tabular and the largest in size of any other coral taxa (Sups 1). Absolute abundance of coral taxa did not differ statistically between sites and monitored months and within sites between months (p > 0.05; Figure 3B). Erscotts observed the most live coral colonies between the three monitored months (225 to 313 colonies; Figure 3B). Acropora spp. contributed to 20 ± 7% of the hard coral cover at LHI and was the highest overall taxa between all three sites (Table 2). P. damicornis contributed the second overall highest coral cover with 5 ± 2%, contributing to the second-highest coral taxa cover at North Bay (8 ± 5%, Table 2). The coral I. cuneata contributed to the second-highest percent cover at Coral Gardens and Erscotts (6 ± 5% and 5 ± 2% respectively, Table 2).
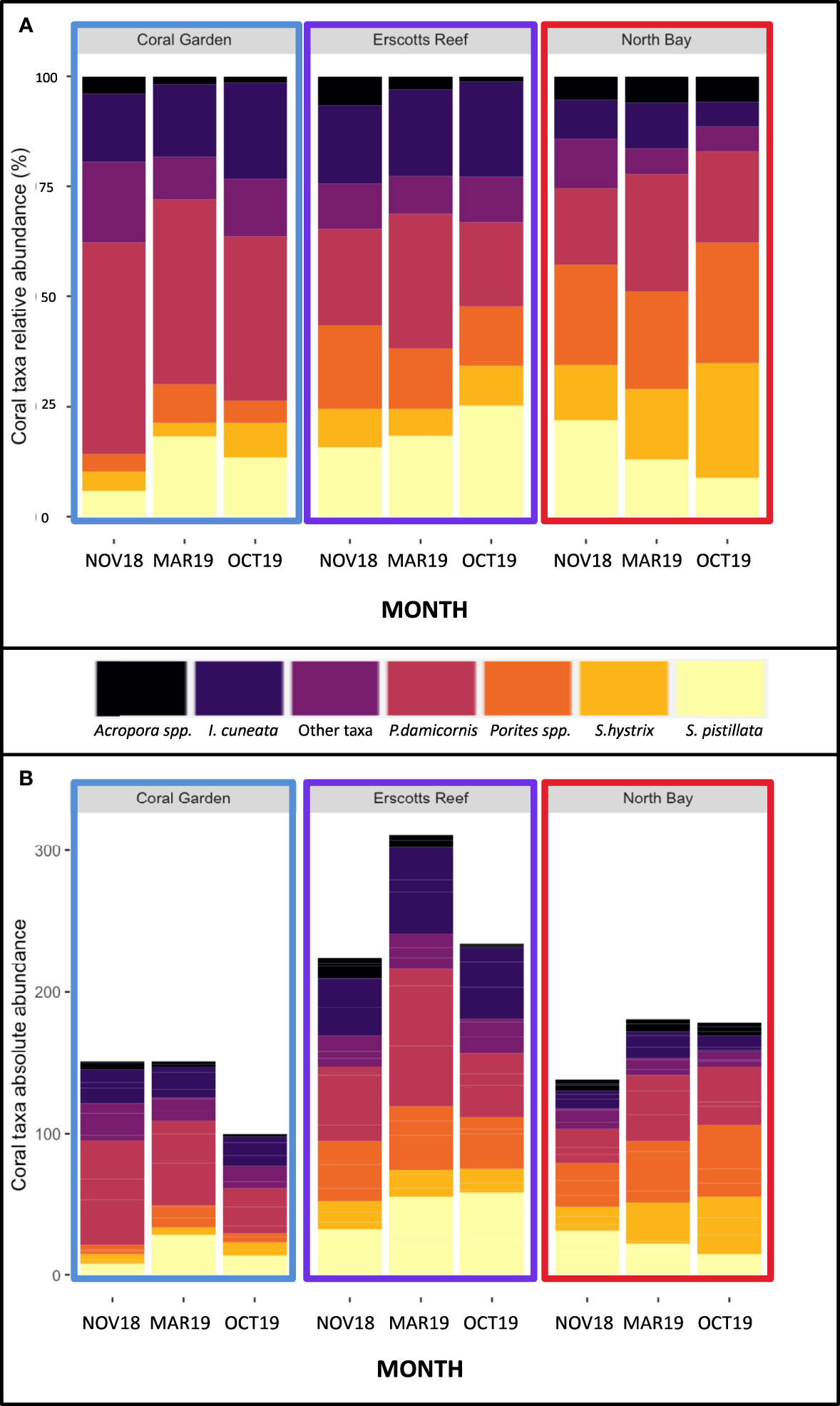
Figure 3 Coral taxa relative abundance (A) and absolute abundance (B) at Coral Garden (blue), Erscotts Reef (purple) and North Bay (Red) during November 2018 (NOV18), March 2019 (MAR19) and October 2019 (OCT19).
3.3 Coral disease surveys
Over the three monitoring months (November 2018, March 2019 and October 2019), a total of 1,751 coral colonies were observed from 17 genera (Table 3). Of these, 442 (26%) of the coral colonies showed signs of compromised health consistent with disease or bleaching (endosymbiosis breakdown) (Table 4, Figure 4). Coral disease accounted for 21% of the colonies observed with compromised health, and “other” signs accounted for 6% observed unhealthy colonies (Table 4, Figures 4, 5). During March 2019, a mass coral bleaching event was recorded at LHI (Steinberg et al., 2022; Moriarty et al., 2023) and was still evident in October 2019 (Figure 4), during which time bleached corals were the most frequently observed (73%) (including mortality, partial mortality and 100% bleached colonies; Table 4; Figure 4). Coral disease was highest in November 2018 (10 ± 1%) and not as evident in March 2019 (5 ± 1%, GLM; p = 0.0007, Figure 4A, due to bleaching). Coral bleaching was not observed during the November 2018 surveys (GLM; p = 0.0078, Figure 4). Where disease was distinguishable from bleaching in March, Erscotts had the highest disease prevalence (9 ± 2%) compared to North Bay (3 ± 2%) and Coral Gardens (2 ± 1%), with Erscotts significantly higher than Coral Gardens (GLM; p = 0.0084; Figure 4B). No coral diseases were identified at Coral Gardens during October 2019 (Figure 4B). Erscotts highest coral disease prevalence was recorded during November (9 ± 2%) and March (9 ± 3%) before significant declines were recorded in disease prevalence in October (1 ± 1%; GLM; p = 0.0067 and GLM; p = 0.0086 respectively; Figure 4B). North Bay had significantly higher disease prevalence during November 2018 (10 ± 3%) than that recorded in March (3 ± 2%) and October (1 ± 1%; GLM; p = 0.0023; GLM; p = 0.0049 respectively; Figure 4B). At Coral Gardens, disease prevalence in November 2018 was 11 ± 3%, whereas coral disease was difficult to distinguish from bleached corals in March 2019 when prevalence was 2 ± 1% (GLM; p = 0.0032) and no disease was recorded in October 2019 (Figure 4B).
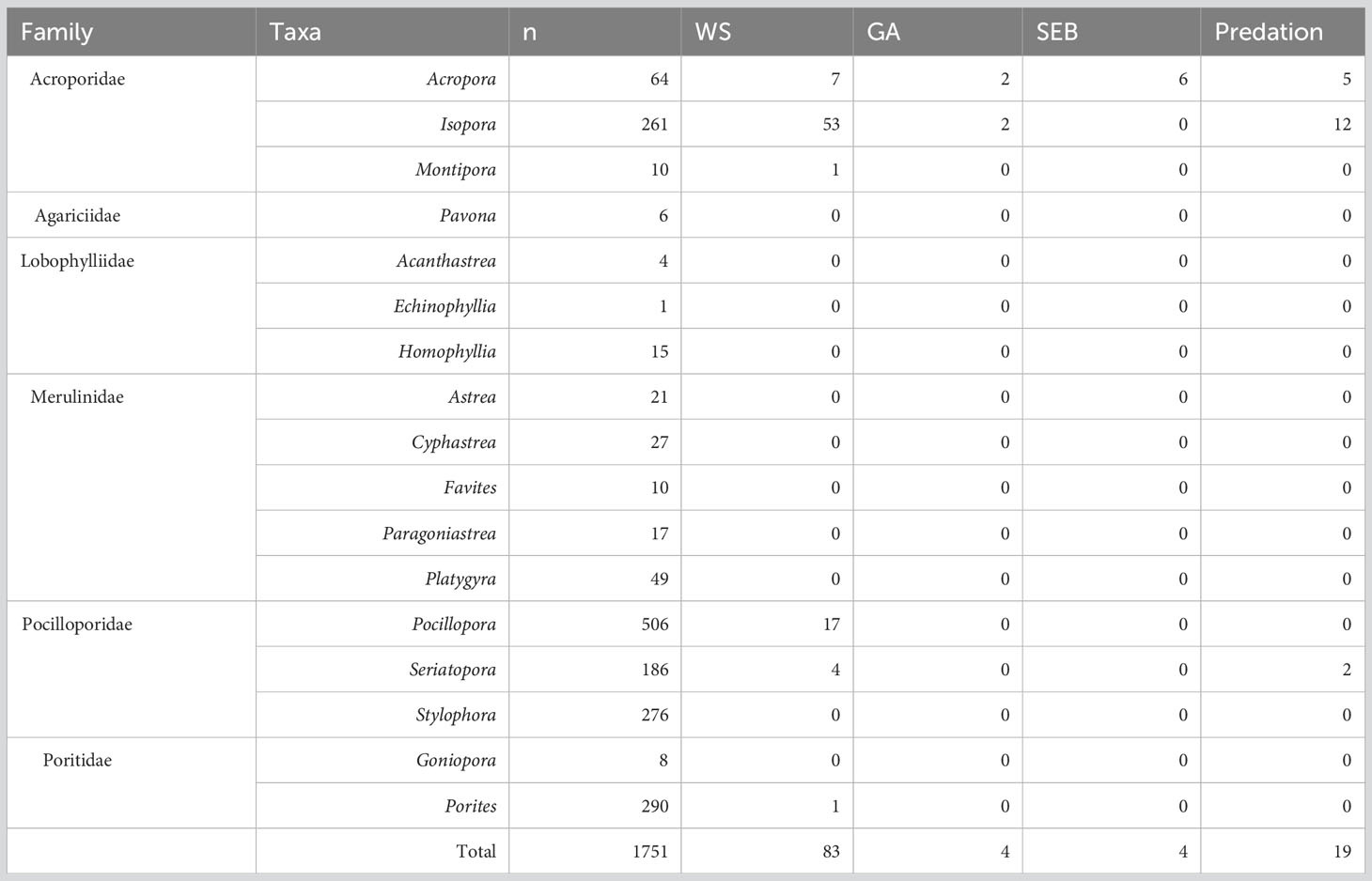
Table 3 Abundance of white syndrome (WS), growth anomalies (GA) and skeletal eroding band (SEB) coral disease for each taxa.
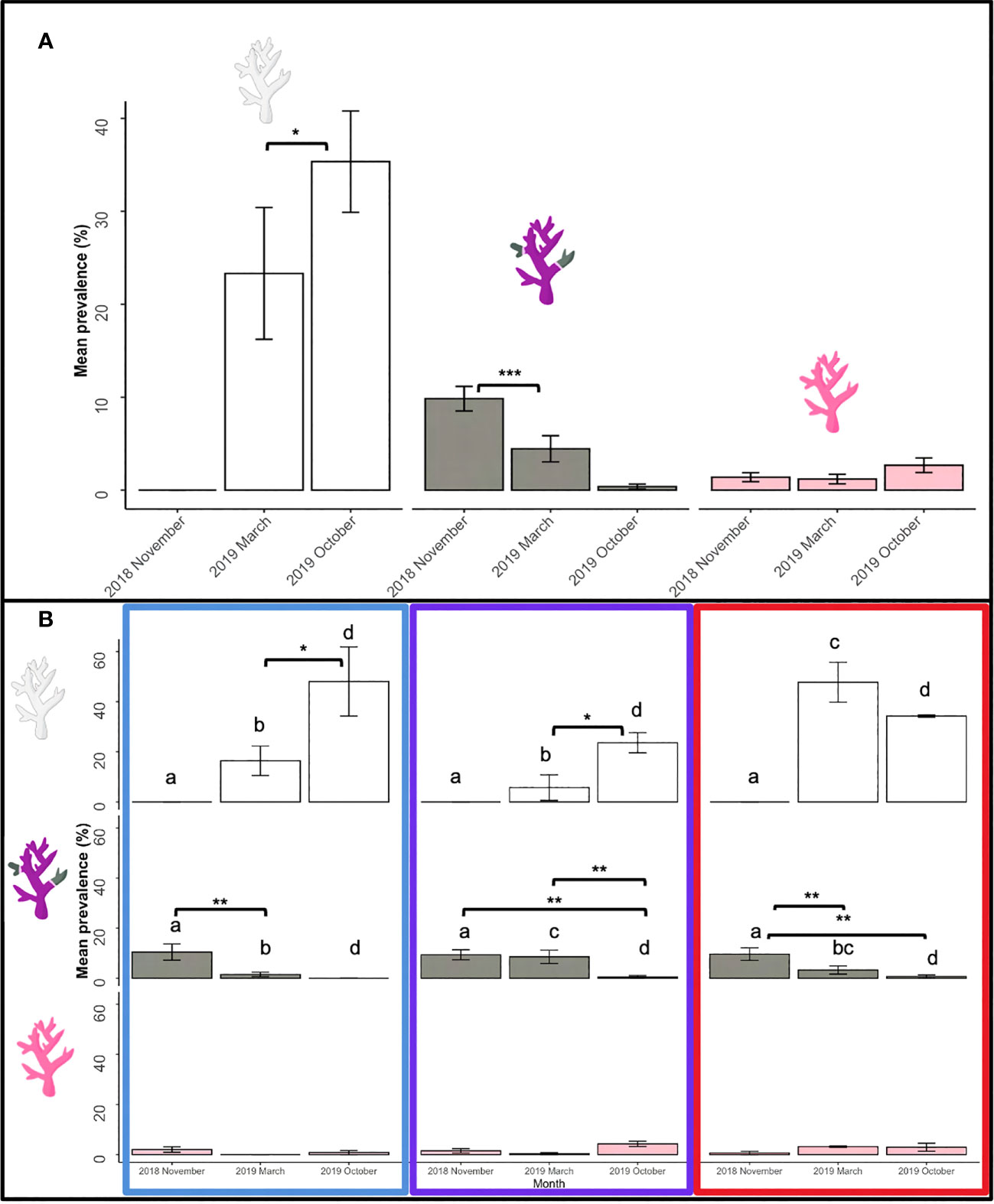
Figure 4 Unhealthy coral prevalence at LHI of bleached (white), diseased (grey) and other (pink) during November 2018, March 2019 and October 2019 (A). Prevalence of coral bleaching (white), disease (grey) and other abnormalities (pink) between Coral Gardens (blue), Erscotts Reef (purple) and North Bay (red) (B). Significant differences were determined using GLM analysis (p <0.05). Asterisks indicate significant levels; * 0.05, ** 0.01, and *** 0.001.
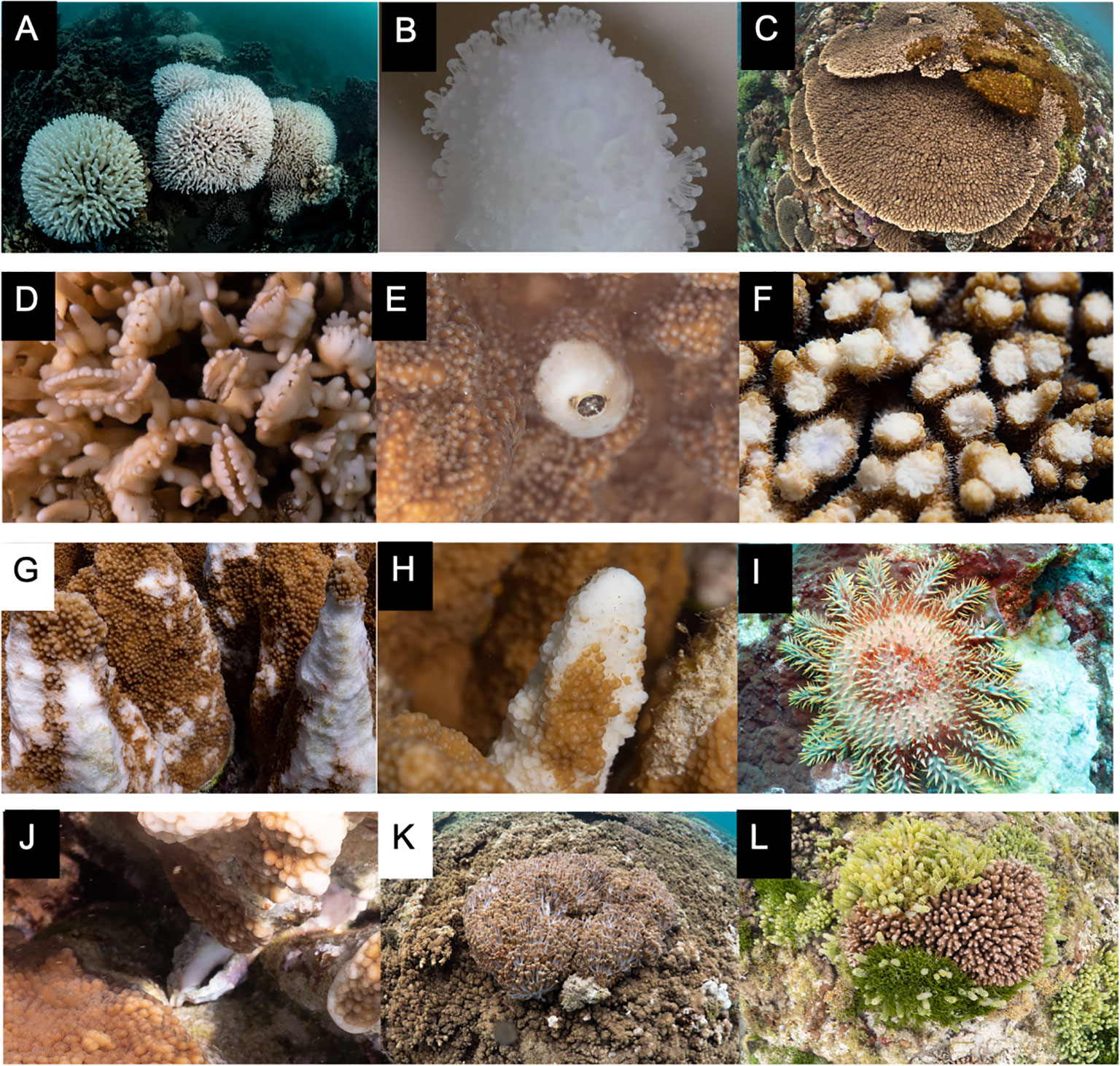
Figure 5 Other coral ailments found at LHI. (A, B) Coral bleaching of P. damicornis and S. pistillata, (C) algae cover in a tabular Acropora colony, (D) galls in S. hystrix from gall crabs, (E) invertebrate burrow in I cuneata, (F) predation of a tabular Acropora colony, (G) fish predation on I cuneata, (H) invertebrate predation on I cuneata, (I) COTS predation on Turbinaria colony, (J) Drupella predation, (K) soft coral competion on tabular Acropora colony, and (L) macro algae competition on a P. damicornis colony.
3.4 Coral health conditions
Three distinct coral health conditions were identified in the LHI lagoon at Coral Gardens, North Bay and Erscotts and one off transect:
(1) white syndrome (WS) (Figures 6A–F),
(2) skeletal eroding band (SEB) (Figure 6G),
(3) growth anomalies (GA) (Table 3, Figures 6I–L) and
(4) endolithic hypermycosis (EH) (Figure 6H, off transect).
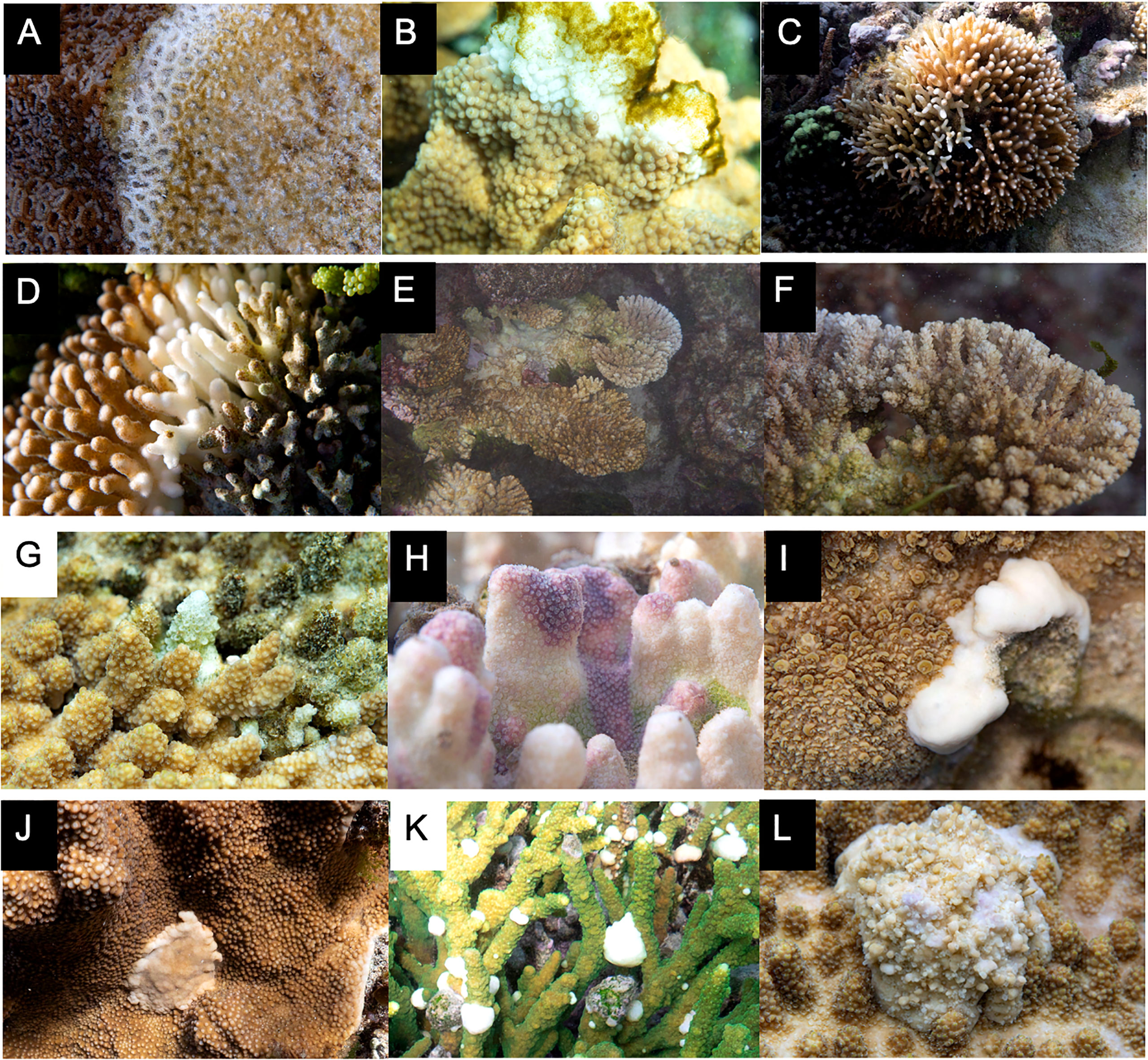
Figure 6 Coral diseases recorded within the LHI lagoon between November 2018 and October 2019. (A–F) White syndrome found in (A) Homophyllia, (B) I cuneata, and S. pistillata, (C) S. hystrix, (D) P. damicornis, (E, F) tabular Acropora, (G) skeletal eroding band in a tabular Acropora, (H) endolithic hypermycosis in Porites. (I–L) growth anomalies: (I) bosselated Isopora growth anomaly, (J) umbonate Isopora growth anomaly, (K) nodular Acropora growth anomaly and (L) exophytic Acropora growth anomaly.
The overall coral disease prevalence for LHI lagoon was 5 ± 1%, and a total of 93 colonies were found with disease conditions (WS, SEB or GA) on all six coral genera, Acropora, Isopora, Montipora, Pocillopora, Porites and Seriatopora (Table 3, Figure 6) (three hard coral families, Acroporidae, Pocilloporidae, and Poritidae). The Acroporidae family accounted for 76% of the diseased colonies compared to Pocilloporidae and Poritidae, which only accounted for 23% and 1%, respectively (Table 3). Six coral species were found with coral disease, Acropora spp., Isopora cuneata, Montipora spp., Pocillopora damicornis, Porites spp. and Seriatopora hystrix (Table 3, Figure 7). I. cuneata was found to have the highest abundance of disease, 55 colonies impacted by white syndrome. In contrast, 21% of affected colonies were Acropora spp. (Table 3, Figure 7). In October 2019, only one I. cuneata colony was observed with a growth anomaly, found at Erscotts, one Acropora spp. colony with SEB and one S. hystrix colony with white syndrome (Table 3, Figure 6). White syndrome was recorded in all of the six taxa impacted by disease and was most prevalent in Acropora spp. (12 ± 6%) and I. cuneata (17 ± 5%; Table 3, Figure 7A). Growth anomalies were present only in Acropora spp. and I. cuneata colonies, and skeletal eroding band was only observed in Acropora spp. (Table 3, Figure 7A). White syndromes had the highest overall prevalence of 4 ± 1%, while growth anomalies and skeletal eroding band had similar prevalence’s overall (0.3 ± 0.1% and 0.4 ± 0.3% respectively) (Figure 8). White syndrome prevalence was highest at Erscotts (6 ± 2%) in comparison to Coral Gardens (4 ± 2%) and North Bay (3 ± 1%) however, no significant differences were found between sites and month for any of the three observed diseases (p > 0.05). White syndrome was recorded at all three monitored months compared to Coral Gardens and Erscotts, where no white syndrome was recorded within the belt transects during October 2019 (Figure 8). Growth anomalies were also found at all three sites with a similar overall prevalence between Coral Gardens, Erscotts and North Bay (0.3 ± 0.3%, 0.3 ± 0.2% and 0.3 ± 0.3% respectively; Figure 8). Skeletal eroding band was only observed in transects at North Bay (1 ± 1%) and was found at all three monitoring months (3 ± 2%, 0.5 ± 0.5% and 0.5 ± 0.5%; Figure 8). Endolithic hypermycosis was recorded off transect in the LHI lagoon. Endolithic hypermycosis was reported in a recently bleached Porites spp. colony (Figure 5H).
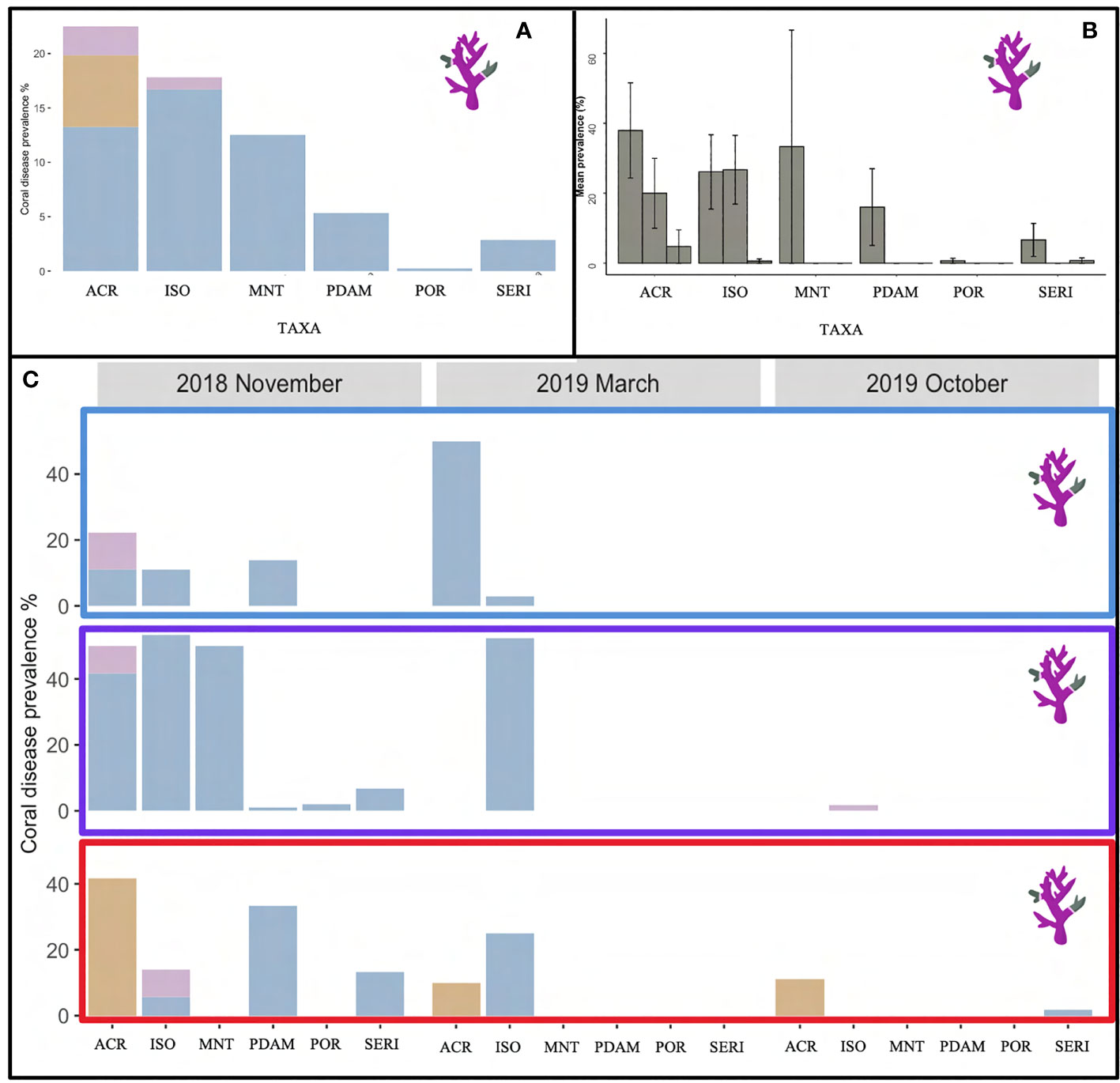
Figure 7 (A) Overall coral disease prevalence (%) found in the six impacted taxa, Acropora (ACR), I cuneata (ISO), Montipora (MNT), P. damicornis (PDAM), Porites (POR) and S. hystrix (Seri) within the LHI Marine Park lagoon for the three diseases found within the transects, growth anomalies (purple), skeletal eroding band (brown) and white syndrome (blue). (B) Coral disease prevalence in each of the six taxa during November 2018, March 2019 and October 2019 (respectively) and (C) between the three sites, Coral Gardens (blue rectangle), Erscotts Reef (purple rectangle) and North Bay (red rectangle) for growth anomalies (purple), skeletal eroding band (brown) and white syndrome (blue).
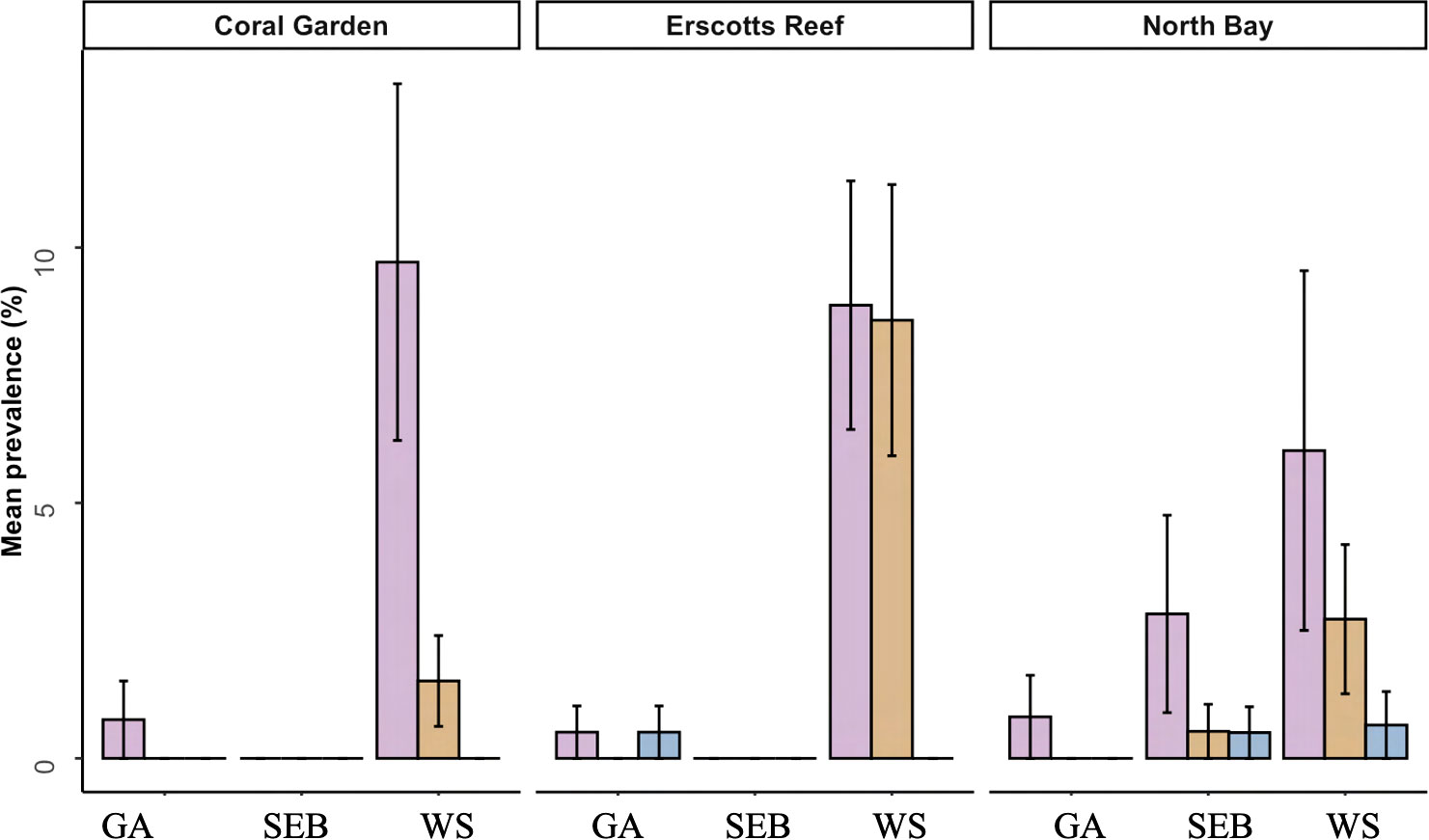
Figure 8 Mean prevalence of the three coral diseases recorded within the transects at the LHI lagoon, growth anomalies (GA), skeletal eroding band (SEB) and white syndrome (WS) at Coral Gardens, Erscotts Reef and North Bay in November 2018 (orange), March 2019 (maroon) and October 2019 (purple).
3.5 Disease descriptions
Three of the four coral diseases (GA, SEB and WS) was recorded in Acropora spp. colonies. I. cuneata and Porites spp. recorded two of the four diseases (GA and WS and WS and EH, respectively). The remaining taxa only recorded one disease (Table 3). White syndrome was the most prevalent disease at LHI, impacting 83 colonies (Table 3, Figures 7, 8). White syndrome had the highest taxa range (n = 6; Table 3, Figure 7). Lesion sizes for white syndrome ranged from 1–90% of the colony (Table 5).
• WS was characterised as a disease displaying white symptoms with a progression of tissue loss exposing the underlying coral skeleton. Tissue loss impacted both the polyps and coenosarc of colonies impacted by white syndrome, and lesion location on the colony was observed over the entire colony with a diffuse pattern (Table 5, Figures 9A, B).
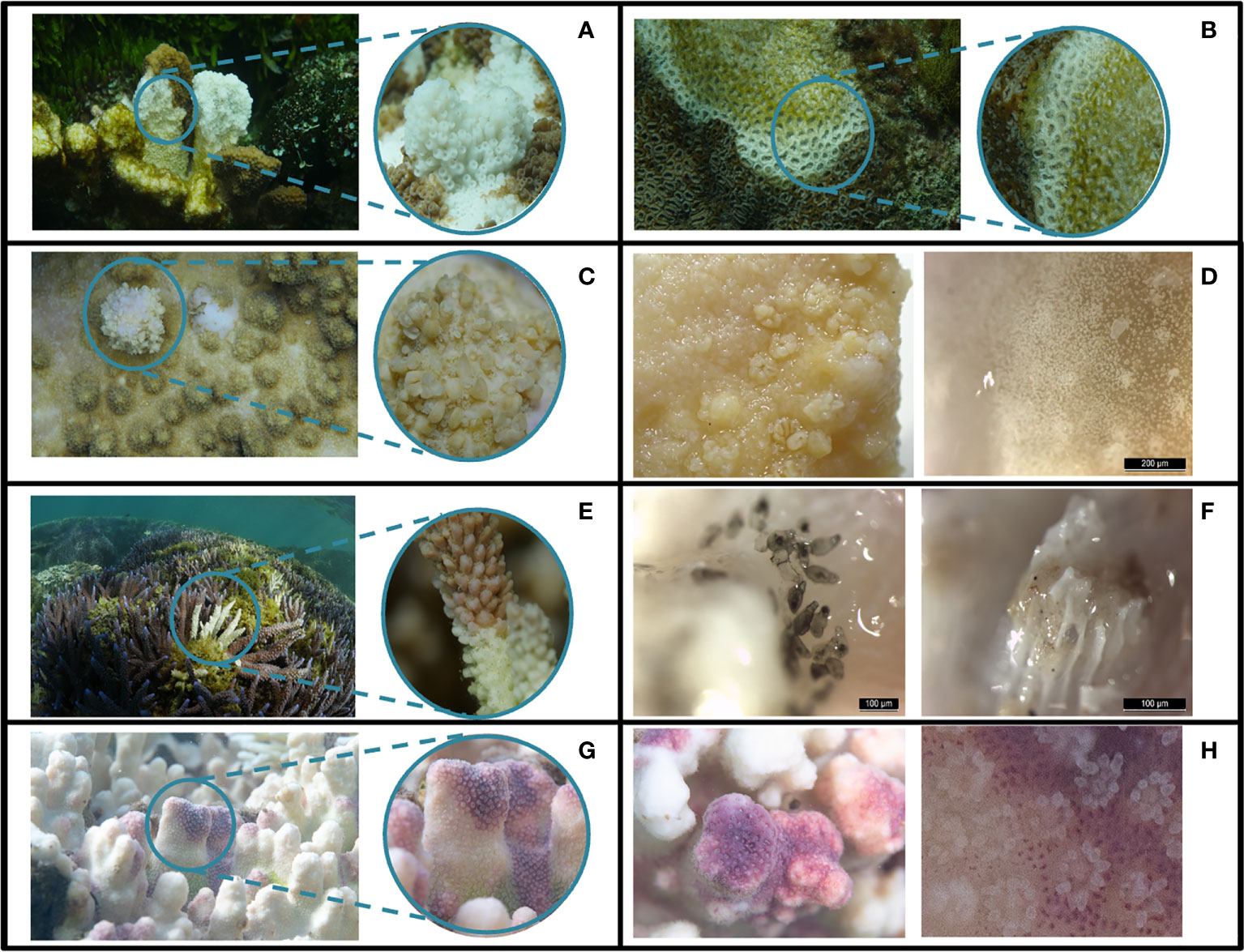
Figure 9 Coral diseases identified at LHI in 2018/2019. White syndrome (A, B), growth anomaly (C, D), skeletal eroding band (E, F), and endolithic hypermycosis (G, H).
• GAs were all located in the central regions of the coral colony, and colonies had focal and multifocal growth anomalies (Table 5). No damage or partial mortality or tissue loss was observed for colonies impacted by growth anomalies (Figures 9C, D).
• SEB impacted the apical regions of medium to large Acropora spp. colonies and was multifocal (Table 5) and was distinguished from white syndrome because small Halofolliculina corallasia (Figure 5F) were observed on the exposed skeleton, a characteristic of SEB (Willis et al., 2004) (Figures 9E, F).
• EH impacted the apical regions on the Porites spp. colony and was distinguished from normal pigmentation because pigmentation was under the bleached tissue (Figures 9G, H).
Both WS and SEB affected colonies were closely examined for corallivore snails, and crown-of-thorns starfish (COTS) as the predation lesions can appear similar to the WS and SEB disease lesions; however, none were found on or around surveyed colonies. There was a total of three Drupella found throughout the three monitored months in the LHI lagoon. COTS were observed at a close-by reef at depths greater than 16 m.
4 Discussion
Coral diseases contribute to global coral reef declines impacting the functionality and composition of reef systems (Bruno et al., 2007; Harvell et al., 2007; Thompson et al., 2014; Heres et al., 2021). Although coral disease studies and identification has been well established in the Caribbean reefs and the closer tropical reefs of the GBR, baseline studies at sites such as the UNESCO World Heritage-listed LHI Marine Park are lacking. Here we identify and describe four coral diseases, their prevalence and susceptible coral taxa within the lagoon of the LHI Marine Park. This study provides baseline coral disease data which is particularly important because of ongoing impacts resulting from climate change which has already caused detrimental impacts to the coral reef communities of LHI. Considering this reef holds high ecological importance, a resource such as this for managers would assist in identifying potential threats to the reef in the face of climate change. LHI Marine Park is the southernmost coral reef in the world, located approximately 600 km east of mainland Australia and over 1,000 km south of the GBR. The LHI Marine Park is regularly utilised for recreational fishing, SCUBA diving, snorkelling, surfing and commercial tourism (e.g., glass bottom boat tours, fishing and sightseeing), and the lagoon is subject to groundwater influxes, freshwater and sediment runoff. Two successive bleaching events were reported in 2010 and 2011 (Harrison et al., 2011; Dalton et al., 2020), with four individual coral bleaching events as a result of thermal stress between 1998 (Harrison et al., 2011; Dalton et al., 2020) and 2019 (Steinberg et al., 2022; Moriarty et al., 2023). Predation by the corallivore crown-of-thorns starfish has not been reported in the LHI lagoon, but these species have been commonly observed at depth around LHI (De Vantier and Deacon, 1990; Moriarty pers. obs.). In 2008 an outbreak of the sea urchin Tripneustes gratilla was recorded at offshore locations around the island, impacting the densities of brown and red algae cover (Valentine and Edgar, 2010).
We surveyed three reefs within the LHI lagoon and found hard coral cover within the LHI lagoon contributes 33 ± 6% of the benthic composition (Coral Gardens, Erscotts and North Bay, Figure 1). The coral cover in the LHI lagoon is greater than other high-latitude reefs such as the Bahamas (28 ± 11%) (Weiler et al., 2019), South Africa (27%) (Schleyer et al., 2018), and lower than Japan’s Tatsukushi reef on Shikoku Island (60%) (Denis et al., 2013) and Western Australia’s Houtman Abrolhos Islands (> 70%) (Bridge et al., 2014). Algae cover is similar to the islands of Hawaii (Vroom and Braun, 2010) and the subtropical Solitary Islands (Dalton and Roff, 2013). Similar benthic compositions were recorded at LHI for hard coral, algae and soft coral in 1992–1993 (Harriott, 1999). In 1999 North Bay experienced high abundances of cyanobacteria and dead coral following the 1998 bleaching event (Harriott, 1999). However, our study found that hard coral cover was highest amongst the three sites at North Bay (46 ± 13%; Figure 1C), suggesting an increase in coral cover since 1999. The LHI reefs within the lagoon are dominated by colonies of Porites spp., P. damicornis, S. pistillata, S. hystrix and I. cuneata at all sites and between all monitored months (Figure 3A). P. damicornis being the most common species at Coral Gardens and Erscotts (17 ± 5%–48 ± 7%) with Porites spp. dominating the coral colony composition at North Bay (24 ± 3%) and apart from the individual taxa from the grouped “other taxa”, Acropora spp. is the least common taxa in terms of colony abundance (1 ± 0.2%–7 ± 3%; Figure 3A). However, the large Acropora spp. colonies contribute a significant amount to the coral percent cover within the LHI lagoon (Table 2), contributing few but large colonies contributing to the reef composition. Acropora spp. is known to be susceptible to perturbations and, in particular, disease globally (Patterson et al., 2002; Williams and Miller, 2005; Ainsworth et al., 2007; Work et al., 2008a; Aeby et al., 2011; Irikawa et al., 2011; Roff et al., 2011; Wilson et al., 2012; Brodnicke et al., 2019).
Disease prevalence at LHI was found to be low during the survey period (5 ± 1%; Figure 4) and similar to disease prevalence previously reported on the southern GBR at Heron Island (August 2008; 4.22 ± 1.72%) (Haapkylä et al., 2010) and the subtropical Solitary Islands (Dalton and Smith, 2006). LHI’s overall disease prevalence was also similar to the remote high-latitude Northwestern Hawaiian Islands, where overall prevalence ranged from 0.5% to 7.09% (Aeby, 2006). Three of the four diseases identified in LHI lagoon, white syndrome (WS), growth anomalies (GA) and skeletal eroding band (SEB), have previously been identified and described from Australia’s Great Barrier Reef (Willis et al., 2004) with the exception of endolithic hypermycosis (EH). We found coral disease in six scleractinian coral genera over the 12-month period, with all three diseases present in Acropora spp.
4.1 Coral diseases at LHI
The four coral diseases recorded at LHI over the 12-month period is fewer in comparison to other Indo-Pacific reef systems. In comparison, six diseases have been recorded at Heron Island, GBR (Haapkylä et al., 2010), five at Mansuar Island, Raja Ampat Indonesia (Subhan et al., 2020), five at Wakatobi, southeast Sulawesi, Indonesia (Haapkylä et al., 2007), four at Koh Tao, Thailand (Lamb et al., 2014), five (possibly six) in the Maldives (Montano et al., 2012), six in the Philippines (Raymundo et al., 2018) and ten in the Northwestern Hawaiian Islands (Aeby, 2006).
All four diseases reported at LHI have been recorded in the Indo-Pacific. Growth anomalies have a global distribution (Raymundo et al., 2005; Aeby, 2006; Work et al., 2008a; Irikawa et al., 2011; Subhan et al., 2020; Aeby et al., 2021; Preston and Richards, 2021) and have been recorded in at least 14 coral taxa globally (Moriarty et al., 2020), here we recorded GAs in two taxa, Acropora a spp. and I. cuneata (Table 3). GAs have been recorded more commonly in Acropora species in Australia (Willis et al., 2004; Haapkylä et al., 2010), Japan (Irikawa et al., 2011), French Frigate Shoals, Johnston Atoll and American Samoa (Work et al., 2008a) and India (Rajasabapathy et al., 2020). Isopora growth anomalies were less commonly documented, with recordings only in Cocos Keeling Islands (Preston and Richards, 2021). The umbrella term for an unidentified disease that is experiencing white symptoms is called white syndrome. At LHI, we recorded white syndrome in five coral taxa: Acropora spp., I. cuneata, Montipora spp., P. damicornis, Porites spp., and S. hystrix. White syndrome has been recorded simultaneously in one or more of these species and taxa in the GBR (Willis et al., 2004; Haapkylä et al., 2010), Indonesia (Haapkylä et al., 2007), Maldives (Montano et al., 2012), Philippines (Raymundo et al., 2005), American Samoa (Wilson et al., 2012) and Norfolk Island (Page et al., 2023). Although white syndrome is found on coral reefs worldwide, it should be noted that individual species and regions could be experiencing varying region-specific diseases and have varying aetiologies. Skeletal eroding band was only recorded in Acropora spp. at LHI, and this disease has been recorded in Acropora spp., in the Maldives (Montano et al., 2012), the GBR (Haapkylä et al., 2010), and the Red Sea (Winkler et al., 2004). The one recorded off-transect endolithic hypermycosis was found in a Porites spp. colony which was currently 100% bleached. This was the only disease not reported in Acropora spp. and has been reported to impact massive boulder coral morphologies in the Pacific (Work et al., 2008b) and the Red Sea (Aeby et al., 2021).
Coral disease prevalence at LHI during the study period was potentially declining over time, although surveys in March 2109 were confounded by the occurrence of coral bleaching, making disease identification and distinguishing prior disease from bleaching more complex (Figure 4). In November 2018, all three sites were found to have similar disease prevalence. However, we found that North Bay and Erscotts disease prevalence had significantly declined in October 2019 compared to November 2018 (Figure 7). White syndrome was recorded at all three sites but not at all survey months. White syndrome was recorded in November 2018 and March 2019 at Coral Gardens and all three months at Erscotts and North Bay. North Bay was the only site at which all three diseases were recorded from the transects. Given the slow recovery from bleaching recorded (Moriarty et al., 2023), it is also possible that post-stress disease emergence, if occurring, was not distinguishable from bleaching recovery in October 2019. A temporal offset between bleaching and post-bleaching disease emergence has been found in many studies. For example, a coral disease outbreak was reported in the US Virgin Islands nine months following a coral bleaching event in 2005 (Miller et al., 2009). White syndrome outbreaks have been reported in the winter of the year after bleaching, indicating a link between cold ocean temperatures up to two years after a bleaching event (Heron et al., 2010).
Interestingly, the Acropora spp. corals had the highest prevalence of disease, and all three disease states found within the transects were reported in Acropora spp. This pattern in disease occurrence in a species with low coral cover was also found in the GBR where pocilloporid species had the highest disease occurrence but the least cover on the reef (Willis et al., 2004). Skeletal eroding band was the first disease identified on the GBR and one of the most prevalent, with reports of the disease found on 90–100% of 18 reefs surveyed in 2004–2006, impacting about 82 hard coral species (Page and Willis, 2008). This study, however, reported SEB as the second most prevalent coral disease at LHI all of which were recorded on Acropora species (Figures 7, 8). Globally Acropora spp. have the highest number of disease reports in the literature (Ainsworth et al., 2007; Work et al., 2008a; Aeby et al., 2011; Irikawa et al., 2011; Roff et al., 2011; Muller et al., 2018; Rajasabapathy et al., 2020). Acropora spp. is thus considered to be susceptible to heat stress and disease (Brown, 1997; Madin et al., 2008; Aeby et al., 2011; Gilmour et al., 2013; Hobbs et al., 2015; Muller et al., 2018; Singh et al., 2019). Acropora spp. diseases have been recorded on most coral reefs, including on the Caribbean (Patterson et al., 2002; Sutherland and Ritchie, 2004; van Woesik and Randall, 2017), GBR (Willis et al., 2004; Haapkylä et al., 2010), Red Sea (Winkler et al., 2004; Mohamed and Sweet, 2019; Aeby et al., 2020; Aeby et al., 2021), and the Indo-Pacific Ocean (Aeby, 2006; Haapkylä et al., 2007; Montano et al., 2012). Acropora spp. dominated the reefs in the Maldives before the 1998 bleaching event comprising approximately 46% of the taxonomic composition reefs but now only contributes 1% of the coral cover (Edwards et al., 2001).
The most severely impacted coral taxa on LHI during the 2019 bleaching event were: P.damicornis, S. pistillata, S. hystrix and Porites spp (Moriarty et al., 2023). Furthermore, Acropora spp. and I. cuneata, two of the least susceptible to bleaching species at LHI in 2019 (Moriarty et al., 2023) where the only two species in which disease was observed during the bleaching event (Figure 7). Interestingly, in contrast to observations documented in the Great Barrier Reef where four diseases were identified (white syndrome, skeletal eroding band, black band and an unidentified disease) on S. pistillata (Willis et al., 2004), we found that although S. pistillata is one of the most prevalent coral taxa at LHI (n = 276), we recorded no signs of coral disease at any of the survey timepoints or at any of the reef sites (Table 3, Figure 7).
5 Conclusions
The occurrence and prevalence of coral diseases at the World Heritage Listed LHI could be the result of numerous factors such as, localised environmental parameters, host susceptibility and mode of transmission, and the normal disease occurrence expected within a population. To the best of our knowledge, this is the first coral disease publication from LHI, documenting disease identification and prevalence within the LHI lagoon. Importantly, coral disease was recorded in Acropora spp., a taxa which dominates the LHI lagoon benthos (Table 2) and had reported a higher thermal tolerance than some of the other reef building important taxa during the 2019 bleaching (Moriarty et al., 2023). Our observations suggest Acropora spp. colonies are also experiencing physiological perturbations, highlighting the need to identify disease causation within the LHI Marine Park. Coral and algae interactions have been linked with increased disease prevalence, and algae have been identified as a possible reservoir for coral disease pathogens (Nugues et al., 2004; Smith et al., 2006; Sweet et al., 2013; Casey et al., 2014) as well as increased water temperatures (Bruno et al., 2007; Ward et al., 2007; Heron et al., 2010; Ruiz-Moreno et al., 2012; van de Water et al., 2018; Aeby et al., 2020). Interestingly, algae composition is highest at Erscotts where coral disease was most prevalent and could be a contributing factor to disease prevalence (Figure 7C). In light of the bleaching event documented on the reef in 2019, it is imperative to conduct comprehensive and extended monitoring of the reef, including the tracking of localised disease occurrence and prevalence. Such efforts would provide management authorities with essential information in regards to the health of the reef post the 2019 bleaching event.
Data availability statement
The datasets presented in this study can be found in online repositories. A link to the data can be found below: https://github.com/tess-moriarty/LHI-coral-disease.
Ethics statement
The manuscript presents research on animals that do not require ethical approval for their study.
Author contributions
TM: Conceptualisation, Methodology, Validation, Formal Analysis, Investigation, Data curation, Writing – Original draft, Writing – Review and editing, Visualisation, Project administration, Funding acquisition. WL: Conceptualisation, Validation, Resources, Writing – Review and editing, Visualisation, Supervision, Project administration, Funding acquisition. TA: Conceptualisation, Validation, Resources, Writing – Review and editing, Visualisation, Supervision, Project administration, Funding acquisition. All authors contributed to the article and approved the submitted version.
Funding
This project was partially funded by a research grant from UNSW Scientia program awarded to TA 2018-2028, as well as funding from the Australian Research Council Discovery Project grant (DP180103199) awarded to TA and WL and the Women’s Diving Hall of Fame – Marine Conservation Scholarship awarded to TM. The Lord Howe Island Marine Park, Environmental Tours Lord Howe and Pro Dive provided in-kind contributions providing boat support and access allowing the collection of data.
Acknowledgments
I would like to acknowledge the Darkinjung people the traditional owners on the land in which the write up of this research took place and pay my respects to the traditional owners of the land, past, present and emerging. The authors would like to thank New South Wales Department of Primary Industries (NSW DPI) – in particular Justin Gilligan, Emma Henry, Caitlin Woods, and Britt Anderson, for their knowledge and support during data collection. As well as NSW DPI’s, Sallyann Gudge for going above and beyond with ongoing support and assistance throughout this project, we sincerely thank you. We thank all the LHI marine operators and in particular Dean Hiscox, Kayla Hiscox and family as well as Lisa and Aaron Ralph providing advice and access to sites. Rosie Steinberg (UNSW), Jesse Bergman (UNSW), Charlotte Page (UNSW) and Melissa Pappas (UNSW) for their assistance in the field with data collection. We would sincerely like to thank Blue Lagoon and Somerset Apartment Lord Howe Island for their generosity and support during our stays at LHI. And lastly, we thank the LHI Board for their support. The authors declare that they have no known conflicts of interest.
Conflict of interest
The authors declare that the research was conducted in the absence of any commercial or financial relationships that could be construed as a potential conflict of interest.
Publisher’s note
All claims expressed in this article are solely those of the authors and do not necessarily represent those of their affiliated organizations, or those of the publisher, the editors and the reviewers. Any product that may be evaluated in this article, or claim that may be made by its manufacturer, is not guaranteed or endorsed by the publisher.
References
Aeby G. S. (2006). Baseline levels of coral disease in the Northwestern Hawaiian Islands. Atoll Res Bull. 543, 471–488.
Aeby G. S., Howells E., Work T., Abrego D., Williams G. J., Wedding L. M., et al. (2020). Localized outbreaks of coral disease on Arabian reefs are linked to extreme temperatures and environmental stressors. Coral Reefs 39 (3), 829–846. doi: 10.1007/s00338-020-01928-4
Aeby G. S., Shore A., Jensen T., Ziegler M., Work T., Voolstra C. R. (2021). A comparative baseline of coral disease in three regions along the Saudi Arabian coast of the central Red Sea. PloS One 16 (7), e0246854. doi: 10.1371/journal.pone.0246854
Aeby G. S., Williams G. J., Franklin E. C., Haapkyla J., Harvell C. D., Neale S., et al. (2011). Growth anomalies on the coral genera acropora and porites are strongly associated with host density and human population size across the indo-pacific. PloS One 6 (2), e16887. doi: 10.1371/journal.pone.0016887
Ainsworth T. D., Kvennefors E. C., Blackall L. L., Fine M., Hoegh-Guldberg O. (2007). Disease and cell death in white syndrome of Acroporid corals on the Great Barrier Reef. Mar. Biol. 151 (1), 19–29. doi: 10.1007/s00227-006-0449-3
Alvarez-Filip L., Estrada-Saldívar N., Pérez-Cervantes E., Molina-Hernández A., González-Barrios F. J. (2019). A rapid spread of the stony coral tissue loss disease outbreak in the Mexican Caribbean. PeerJ 7, e8069. doi: 10.7717/peerj.8069
Antonius A. (1976). New observations on coral destruction in reefs. Mtg. Assoc. Isl. Mar. Lab. Carib., Mayaguez, Puerto Rico, 10, 3.
Baird A. H., Sommer B., Madin J. S. (2012). Pole-ward range expansion of Acropora spp. along the east coast of Australia. Coral Reefs 31 (4), 1063. doi: 10.1007/s00338-012-0928-6
Bourne D. (2005). Microbiological assessment of a disease outbreak on corals from Magnetic Island (Great Barrier Reef, Australia). Coral Reefs 24 (2), 304–312. doi: 10.1007/s00338-005-0479-1
Bridge T. C., Ferrari R., Bryson M., Hovey R., Figueira W. F., Williams S. B., et al. (2014). Variable responses of benthic communities to anomalously warm sea temperatures on a high-latitude coral reef. PloS One 9 (11). doi: 10.1371/journal.pone.0113079
Brodnicke O. B., Bourne D. G., Heron S. F., Pears R. J., Stella J. S., Smith H. A., et al. (2019). Unravelling the links between heat stress, bleaching and disease: fate of tabular corals following a combined disease and bleaching event. Coral Reefs 38 (4), 591–603. doi: 10.1007/s00338-019-01813-9
Brown B. E. (1997). Disturbances to reefs in recent times. In: Birkeland C. (ed) Life Death coral reefs (New York: Chapman & Hall), 354–379. doi: 10.1007/978-1-4615-5995-5_15
Bruno J. F., Petes L. E., Drew Harvell C., Hettinger A. (2003). Nutrient enrichment can increase the severity of coral diseases. Ecol. letters 6 (12), 1056–1061. doi: 10.1046/j.1461-0248.2003.00544.x
Bruno J. F., Selig E. R., Casey K. S., Page C. A., Willis B. L., Harvell C. D., et al. (2007). Thermal stress and coral cover as drivers of coral disease outbreaks. PloS Biol. 5 (6), e124. doi: 10.1371/journal.pbio.0050124
Carpenter K. E., Abrar M., Aeby G., Aronson R. B., Banks S., Bruckner A., et al. (2008). One-third of reef-building corals face elevated extinction risk from climate change and local impacts. Science 321 (5888), 560–563. doi: 10.1126/science.1159196
Casey J. M., Ainsworth T. D., Choat J. H., Connolly S. R. (2014). Farming behaviour of reef fishes increases the prevalence of coral disease associated microbes and black band disease. Proc. R. Soc. B: Biol. Sci. 281 (1788), 20141032. doi: 10.1098/rspb.2014.1032
Dalton S. J., Carroll A. G., Sampayo E., Roff G., Harrison P. L., Entwistle K., et al. (2020). Successive marine heatwaves cause disproportionate coral bleaching during a fast phase transition from El Niño to La Niña. Sci. Total Environment 715, 136951. doi: 10.1016/j.scitotenv.2020.136951
Dalton S. J., Godwin S., Smith S., Pereg L. (2010). Australian subtropical white syndrome: a transmissible, temperature-dependent coral disease. Mar. Freshw. Res. 61 (3), 342–350. doi: 10.1071/MF09060
Dalton S. J., Roff G. (2013). Spatial and temporal patterns of eastern Australia subtropical coral communities. PloS One 8 (9), e75873. doi: 10.1371/journal.pone.0075873
Dalton S. J., Smith S. D. (2006). Coral disease dynamics at a subtropical location, Solitary Islands Marine Park, eastern Australia. Coral Reefs 25 (1), 37–45. doi: 10.1007/s00338-005-0039-8
Denis V., Mezaki T., Tanaka K., Kuo C.-Y., De Palmas S., Keshavmurthy S., et al. (2013). Coverage, diversity, and functionality of a high-latitude coral community (Tatsukushi, shikoku island, Japan). PloS One 8 (1), e54330. doi: 10.1371/journal.pone.0054330
De Vantier L., Deacon G. (1990). Distribution of Acanthaster planci at Lord Howe Island, the southern-most Indo-Pacific reef. Coral Reefs 9 (3), 145–148. doi: 10.1007/BF00258226
Edwards A. J., Clark S., Zahir H., Rajasuriya A., Naseer A., Rubens J. (2001). Coral bleaching and mortality on artificial and natural reefs in Maldives in 1998, sea surface temperature anomalies and initial recovery. Mar. pollut. Bulletin 42 (1), 7–15. doi: 10.1016/S0025-326X(00)00200-9
Frölicher T. L., Laufkötter C. (2018). Emerging risks from marine heat waves. Nat. Commun. 9 (1), 650. doi: 10.1038/s41467-018-03163-6
Gilmour J. P., Smith L. D., Heyward A. J., Baird A. H., Pratchett M. S. (2013). Recovery of an isolated coral reef system following severe disturbance. Science 340 (6128), 69–71. doi: 10.1126/science.1232310
Gintert B. E., Precht W. F., Fura R., Rogers K., Rice M., Precht L. L., et al. (2019). Regional coral disease outbreak overwhelms impacts from a local dredge project. Environ. Monit. Assessment 191 (10), 630. doi: 10.1007/s10661-019-7767-7
Haapkylä J., Melbourne-Thomas J., Flavell M., Willis B. (2010). Spatiotemporal patterns of coral disease prevalence on Heron Island, Great Barrier Reef, Australia. Coral Reefs 29 (4), 1035–1045. doi: 10.1007/s00338-010-0660-z
Haapkylä J., Melbourne-Thomas J., Flavell M., Willis B. (2013). Disease outbreaks, bleaching and a cyclone drive changes in coral assemblages on an inshore reef of the Great Barrier Reef. J. Int. Soc. Reef Stud. 32 (3), 815–824. doi: 10.1007/s00338-013-1029-x
Haapkylä J., Seymour A., Trebilco J., Smith D. (2007). Coral disease prevalence and coral health in the Wakatobi Marine Park, south-east Sulawesi, Indonesia. J. Mar. Biol. Assoc. united Kingdom 87 (2), 403–414. doi: 10.1017/S0025315407055828
Harriott V. (1999). Coral growth in subtropical eastern Australia. Coral Reefs 18 (3), 281–291. doi: 10.1007/s003380050195
Harrison P. L., Dalton S. J., Carroll A. G. (2011). Extensive coral bleaching on the world’s southernmost coral reef at Lord Howe Island, Australia. Coral Reefs 30 (3), 775. doi: 10.1007/s00338-011-0778-7
Harvell D., Jordán-Dahlgren E., Merkel S., Rosenberg E., Raymundo L., Smith G., et al. (2007). Coral disease, environmental drivers, and the balance between coral and microbial associates. Oceanography 20, 172–195. doi: 10.5670/oceanog.2007.91
Hein M. Y., Lamb J. B., Scott C., Willis B. L. (2015). Assessing baseline levels of coral health in a newly established marine protected area in a global scuba diving hotspot. Mar. Environ. Res. 103, 56–65. doi: 10.1016/j.marenvres.2014.11.008
Heres M. M., Farmer B. H., Elmer F., Hertler H. (2021). Ecological consequences of stony coral tissue loss disease in the Turks and Caicos Islands. Coral Reefs 40 (2), 609–624. doi: 10.1007/s00338-021-02071-4
Heron S. F., Willis B. L., Skirving W. J., Eakin C. M., Page C. A., Miller I. R. (2010). Summer hot snaps and winter conditions: modelling white syndrome outbreaks on great barrier reef corals. PloS One 5 (8), e12210. doi: 10.1371/journal.pone.0012210
Hobbs J.-P. A., Frisch A. J., Newman S. J., Wakefield C. B. (2015). Selective impact of disease on coral communities: outbreak of white syndrome causes significant total mortality of acropora plate corals. PloS One 10 (7), e0132528. doi: 10.1371/journal.pone.0132528
Hoegh-Guldberg O., Mumby P. J., Hooten A. J., Steneck R. S., Greenfield P., Gomez E., et al. (2007). Coral reefs under rapid climate change and ocean acidification. Science 318 (5857), 1737–1742. doi: 10.1126/science.1152509
Hughes T. P., Anderson K. D., Connolly S. R., Heron S. F., Kerry J. T., Lough J. M., et al. (2018). Spatial and temporal patterns of mass bleaching of corals in the Anthropocene. Science 359 (6371), 80–83. doi: 10.1126/science.aan8048
Hughes T. P., Barnes M. L., Bellwood D. R., Cinner J. E., Cumming G. S., Jackson J. B. C., et al. (2017a). Coral reefs in the anthropocene. Nature 546 (7656), 82–90. doi: 10.1038/nature22901
Hughes T. P., Kerry J. T., Álvarez-Noriega M., Álvarez-Romero J. G., Anderson K. D., Baird A. H., et al. (2017b). Global warming and recurrent mass bleaching of corals. Nature 543 (7645), 373–377. doi: 10.1038/nature21707
Irikawa A., Casareto B. E., Suzuki Y., Agostini S., Hidaka M., van Woesik R. (2011). Growth anomalies on Acropora cytherea corals. Mar. pollut. Bulletin 62 (8), 1702–1707. doi: 10.1016/j.marpolbul.2011.05.033
Jones R. J., Bowyer J., Hoegh-Guldberg O., Blackall L. L. (2004). Dynamics of a temperature-related coral disease outbreak. Mar. Ecol. Prog. Series 281, 63–77. doi: 10.3354/meps281063
Lamb J. B., True J. D., Piromvaragorn S., Willis B. L. (2014). Scuba diving damage and intensity of tourist activities increases coral disease prevalence. Biol. Conserv. 178, 88–96. doi: 10.1016/j.biocon.2014.06.027
Lamb J. B., Willis B. L., Fiorenza E. A., Couch C. S., Howard R., Rader D. N., et al. (2018). Plastic waste associated with disease on coral reefs. Science 359 (6374), 460–462. doi: 10.1126/science.aar3320
Lough J. M., Anderson K. D., Hughes T. P. (2018). Increasing thermal stress for tropical coral reefs: 1871–2017. Sci. Rep. 8 (1), 6079. doi: 10.1038/s41598-018-24530-9
Madin J. S., O'Donnell M. J., Connolly S. R. (2008). Climate-mediated mechanical changes to post-disturbance coral assemblages. Biol. Letters 4 (5), 490–493. doi: 10.1098/rsbl.2008.0249
Maynard J., van Hooidonk R., Eakin C. M., Puotinen M., Garren M., Williams G., et al. (2015). Projections of climate conditions that increase coral disease susceptibility and pathogen abundance and virulence. Nat. Climate Change 5 (7), 688–694. doi: 10.1038/nclimate2625
Meesters E. H., Noordeloos M., Bak R. P. M. (1994). Damage and regeneration: links to growth in the reef-building coral Montastrea annularis. Mar. Ecol. Prog. Series 112 (1/2), 119–128. Available at: https://www.jstor.org/stable/24847643
Miller J., Muller E., Rogers C., Waara R., Atkinson A., Whelan K., et al. (2009). Coral disease following massive bleaching in 2005 causes 60% decline in coral cover on reefs in the US Virgin Islands. Coral Reefs 28 (4), 925. doi: 10.1007/s00338-009-0531-7
Mohamed A. R., Sweet M. (2019). “Current Knowledge of Coral Diseases Present Within the Red Sea,” in Oceanographic and Biological Aspects of the Red Sea. Eds. Rasul N. M. A., Stewart I. C. F. (Cham: Springer International Publishing), 387–400.
Montano S., Strona G., Seveso D., Galli P. (2012). First report of coral diseases in the Republic of Maldives. Dis. Aquat. Organisms 101 (2), 159–165. doi: 10.3354/dao02515
Moriarty T., Leggat W., Heron S. F., Steinberg R., Ainsworth T. D. (2023). Bleaching, mortality and lengthy recovery on the coral reefs of Lord Howe Island. The 2019 marine heatwave suggests an uncertain future for high-latitude ecosystems. PloS Climate 2 (4), e0000080. doi: 10.1371/journal.pclm.0000080
Moriarty T., Leggat W., Huggett M. J., Ainsworth T. D. (2020). Coral disease causes, consequences, and risk within coral restoration. Trends Microbiol. 28 (10), 793–807. doi: 10.1016/j.tim.2020.06.002
Muller E. M., Bartels E., Baums I. B. (2018). Bleaching causes loss of disease resistance within the threatened coral species Acropora cervicornis. Elife 7, e35066. doi: 10.7554/eLife.35066.028
Muller E. M., van Woesik R. (2009). Shading reduces coral-disease progression. Coral Reefs 28 (3), 757–760. doi: 10.1007/s00338-009-0504-x
Myers R. L., Raymundo L. J. (2009). Coral disease in Micronesian reefs: a link between disease prevalence and host abundance. Dis. Aquat. Organisms 87 (1-2), 97–104. doi: 10.3354/dao02139
Nugues M. M. (2002). Impact of a coral disease outbreak on coral communities in St. Lucia: What and how much has been lost? Mar. Ecol. Prog. Series 229, 61–71. doi: 10.3354/meps229061
Nugues M. M., Smith G. W., van Hooidonk R. J., Seabra M. I., Bak R. P. M. (2004). Algal contact as a trigger for coral disease. Ecol. Letters 7 (10), 919–923. doi: 10.1111/j.1461-0248.2004.00651.x
Page C. E., Leggat W., Egan S., Ainsworth T. D. (2023). A coral disease outbreak highlights vulnerability of remote high-latitude lagoons to global and local stressors. iScience 26 (3), 106205. doi: 10.1016/j.isci.2023.106205
Page C. A., Stoddart J. A. (2010). New records of five coral diseases from the Pilbara Region of Western Australia. Coral Reefs 29 (4), 987. doi: 10.1007/s00338-010-0659-5
Page C. A., Willis B. L. (2008). Epidemiology of skeletal eroding band on the Great Barrier Reef and the role of injury in the initiation of this widespread coral disease. Coral Reefs 27 (2), 257–272. doi: 10.1007/s00338-007-0317-8
Palmer C. V., Baird A. H. (2018). Coral tumor-like growth anomalies induce an immune response and reduce fecundity. Dis. Aquat. Organisms 130 (1), 77–81. doi: 10.3354/dao03258
Pandolfi J. M., Connolly S. R., Marshall D. J., Cohen A. L. (2011). Projecting coral reef futures under global warming and ocean acidification. Science 333 (6041), 418–422.
Patterson K. L., Porter J. W., Ritchie K. B., Polson S. W., Mueller E., Peters E. C., et al. (2002). The etiology of white pox, a lethal disease of the Caribbean elkhorn coral, Acropora palmata. Proc. Natl. Acad. Sci. 99 (13), 8725–8730. doi: 10.1073/pnas.092260099
Pratchett M. S., Hoey A. S., Wilson S. K. (2014). Reef degradation and the loss of critical ecosystem goods and services provided by coral reef fishes. Curr. Opin. Environ. Sustainability 7, 37–43. doi: 10.1016/j.cosust.2013.11.022
Precht W. F., Gintert B. E., Robbart M. L., Fura R., Van Woesik R. (2016). Unprecedented disease-related coral mortality in Southeastern Florida. Sci. Rep. 6 (1), 1–11. doi: 10.1038/srep31374
Preston S., Richards Z. (2021). Biological consequences of an outbreak of growth anomalies on Isopora palifera at the Cocos (Keeling) Islands. Coral Reefs 40 (1), 97–109. doi: 10.1007/s00338-020-02019-0
Rajasabapathy R., Ramasamy K. P., Manikandan B., Mohandass C., Arthur James R. (2020). Bacterial communities associated with healthy and diseased (Skeletal growth anomaly) reef coral acropora cytherea from palk bay, India. Front. Mar. Sci. 7 (92). doi: 10.3389/fmars.2020.00092
Raymundo L. J., Licuanan W. L., Kerr A. M. (2018). Adding insult to injury: Ship groundings are associated with coral disease in a pristine reef. PloS One 13 (9), e0202939. doi: 10.1371/journal.pone.0202939
Raymundo L. J., Rosell K. B., Reboton C. T., Kaczmarsky L. (2005). Coral diseases on Philippine reefs: genus Porites is a dominant host. Dis. Aquat. organisms 64 (3), 181–191. doi: 10.3354/dao064181
Redding J. E., Myers-Miller R. L., Baker D. M., Fogel M., Raymundo L. J., Kim K. (2013). Link between sewage-derived nitrogen pollution and coral disease severity in Guam. Mar. pollut. Bulletin 73 (1), 57–63. doi: 10.1016/j.marpolbul.2013.06.002
Richardson L. L. (1998). Coral diseases: what is really known? Trends Ecol. Evol. 13 (11), 438–443. doi: 10.1016/S0169-5347(98)01460-8
Riegl B. (2003). Climate change and coral reefs: different effects in two high-latitude areas (Arabian Gulf, South Africa). Coral reefs 22 (4), 433–446. doi: 10.1007/s00338-003-0335-0
Roff G., Kvennefors E. C. E., Fine M., Ortiz J., Davy J. E., Hoegh-Guldberg O. (2011). The ecology of ‘Acroporid white syndrome', a coral disease from the southern great barrier reef. PloS One 6 (12), e26829. doi: 10.1371/journal.pone.0026829
Ruiz-Moreno D., Willis B. L., Page A. C., Weil E., Cróquer A., Vargas-Angel B., et al. (2012). Global coral disease prevalence associated with sea temperature anomalies and local factors. Dis. Aquat. Organisms 100 (3), 249–261. doi: 10.3354/dao02488
Schleyer M. H., Floros C., Laing S. C. S., Macdonald A. H. H., Montoya-Maya P. H., Morris T., et al. (2018). What can South African reefs tell us about the future of high-latitude coral systems? Mar. pollut. Bull. 136, 491–507. doi: 10.1016/j.marpolbul.2018.09.014
Sheppard C. R. C. (2003). Predicted recurrences of mass coral mortality in the Indian Ocean. Nature 425 (6955), 294–297. doi: 10.1038/nature01987
Singh T., Iijima M., Yasumoto K., Sakai K. (2019). Effects of moderate thermal anomalies on Acropora corals around Sesoko Island, Okinawa. PloS One 14 (1), e0210795. doi: 10.1371/journal.pone.0210795
Smith J. E., Shaw M., Edwards R. A., Obura D., Pantos O., Sala E., et al. (2006). Indirect effects of algae on coral: algae-mediated, microbe-induced coral mortality. Ecol. Letters 9 (7), 835–845. doi: 10.1111/j.1461-0248.2006.00937.x
Steinberg R. K., Ainsworth T. D., Moriarty T., Bednarek T., Dafforn K. A., Johnston E. L. (2022). Bleaching susceptibility and resistance of octocorals and anemones at the world’s southern-most coral reef. Front. Physiol. 13. doi: 10.3389/fphys.2022.804193
Stimson J. (2011). Ecological characterization of coral growth anomalies on Porites compressa in Hawai ‘i. Coral Reefs 30 (1), 133–142. doi: 10.1007/s00338-010-0672-8
Subhan B., Arafat D., Rahmawati F., Dasmasela Y., Royhan Q., Madduppa H., et al. (2020). Coral disease at Mansuar Island, Raja Ampat, Indonesia. Earth Environ. Sci. 429 (1), 12027.
Sully S., Burkepile D. E., Donovan M. K., Hodgson G., van Woesik R. (2019). A global analysis of coral bleaching over the past two decades. Nat. Commun. 10 (1), 1264. doi: 10.1038/s41467-019-09238-2
Sutherland W. J., Clout M., Depledge M., Dicks L. V., Dinsdale J., Entwistle A. C., et al. (2015). A horizon scan of global conservation issues for 2015. Trends Ecol. Evolution 30 (1), 17–24. doi: 10.1016/j.tree.2014.11.002
Sutherland K. P., Ritchie K. B. (2004). “White Pox Disease of the Caribbean Elkhorn Coral, Acropora palmata,” in Coral Health and Disease. Eds. Rosenberg E., Loya Y. (Berlin, Heidelberg: Springer Berlin Heidelberg), 289–300.
Sweet M. J., Bythell J. C., Nugues M. M. (2013). Algae as reservoirs for coral pathogens. PloS One 8 (7), e69717. doi: 10.1371/journal.pone.0069717
Thompson A., Schroeder T., Brando V. E., Schaffelke B. (2014). Coral community responses to declining water quality: Whitsunday Islands, Great Barrier Reef, Australia. Coral Reefs 33 (4), 923–938. doi: 10.1007/s00338-014-1201-y
Valentine J., Edgar G. (2010). Impacts of a population outbreak of the urchin Tripneustes gratilla amongst Lord Howe Island coral communities. Coral Reefs 29 (2), 399–410. doi: 10.1007/s00338-010-0610-9
van de Water J. A. J. M., Chaib De Mares M., Dixon G. B., Raina J.-B., Willis B. L., Bourne D. G., et al. (2018). Antimicrobial and stress responses to increased temperature and bacterial pathogen challenge in the holobiont of a reef-building coral. Mol. Ecol. 27 (4), 1065–1080. doi: 10.1111/mec.14489
van Woesik R., Randall C. J. (2017). Coral disease hotspots in the Caribbean. Ecosphere 8 (5), e01814. doi: 10.1002/ecs2.1814
Vega Thurber R. L., Burkepile D. E., Fuchs C., Shantz A. A., McMinds R., Zaneveld J. R. (2014). Chronic nutrient enrichment increases prevalence and severity of coral disease and bleaching. Global Change Biol. 20 (2), 544–554. doi: 10.1111/gcb.12450
Vroom P. S., Braun C. L. (2010). Benthic composition of a healthy subtropical reef: baseline species-level cover, with an emphasis on algae, in the northwestern Hawaiian islands. PloS One 5 (3), e9733. doi: 10.1371/journal.pone.0009733
Ward J. R., Kim K., Harvell C. D. (2007). Temperature affects coral disease resistance and pathogen growth. Mar. Ecol. Prog. Ser. 329, 115–121. doi: 10.3354/meps329115
Weil E., Cróquer A., Urreiztieta I. (2009). Yellow band disease compromises the reproductive output of the Caribbean reef-building coral Montastraea faveolata (Anthozoa, Scleractinia). Dis. Aquat. Organisms 87 (1-2), 45–55. doi: 10.3354/dao02103
Weil E., Hernández-Delgado E., Gonzalez M., Williams S., Suleimán-Ramos S., Figuerola M., et al. (2019). Spread of the new coral disease “SCTLD” into the Caribbean: implications for Puerto Rico. Reef Encounter 34, 38–43.
Weiler B. A., Van Leeuwen T. E., Stump K. L. (2019). The extent of coral bleaching, disease and mortality for data-deficient reefs in Eleuthera, The Bahamas after the 2014–2017 global bleaching event. Coral Reefs 38 (4), 831–836. doi: 10.1007/s00338-019-01798-5
Wilkinson C. ed. (2000). Status of coral reefs of the world: 2000. (Townsville/Dampier, Australia: Australia Institute Marine Science)
Williams D. E., Miller M. W. (2005). Coral disease outbreak: pattern, prevalence and transmission in Acropora cervicornis. Mar. Ecol. Prog. Series 301, 119–128. doi: 10.3354/meps301119
Willis B. L., Page C. A., Dinsdale E. A. (2004). Coral disease on the Great Barrier Reef. In: Rosenberg E., Loya Y., editors. Coral Health and Disease. (Berlin: Springer-Verlag), pp. 69–104.
Wilson B., Aeby G. S., Work T. M., Bourne D. G. (2012). Bacterial communities associated with healthy and Acropora white syndrome-affected corals from American Samoa. FEMS Microbiol. Ecol. 80 (2), 509–520. doi: 10.1111/j.1574-6941.2012.01319.x
Winkler R., Antonius A., Abigail Renegar D. (2004). The skeleton eroding band disease on coral reefs of Aqaba, Red Sea. Mar. Ecol. 25 (2), 129–144. doi: 10.1111/j.1439-0485.2004.00020.x
Work T. M., Aeby G. S. (2006). Systematically describing gross lesions in corals. Dis. Aquat. Organisms 70 (1-2), 155–160. doi: 10.3354/dao070155
Work T. M., Aeby G. S., Coles S. L. (2008a). Distribution and morphology of growth anomalies in Acropora from the Indo-Pacific. Dis. Aquat. Organisms 78 (3), 255–264. doi: 10.3354/dao01881
Work T. M., Aeby G., Stanton F., Fenner D. (2008b). Overgrowth of fungi (endolithic hypermycosis) associated with multifocal to diffuse distinct amorphous dark discoloration of corals in the Indo-Pacific. Coral Reefs 27 (3), 663. doi: 10.1007/s00338-008-0374-7
Work T. M., Richardson L. L., Reynolds T. L., Willis B. L. (2008c). Biomedical and veterinary science can increase our understanding of coral disease. J. Exp. Mar. Biol. Ecol. 362 (2), 63–70. doi: 10.1016/j.jembe.2008.05.011
Yamano H., Sugihara K., Nomura K. (2011). Rapid poleward range expansion of tropical reef corals in response to rising sea surface temperatures. Geophys. Res. Letters 38 (4). doi: 10.1029/2010GL046474
Keywords: coral disease, world heritage listed, high-latitude reef, coral bleaching, subtropical, Lord Howe Island
Citation: Moriarty T, Ainsworth TD and Leggat W (2023) Identification of coral disease within the high-latitude reef, Lord Howe Island Marine Park. Front. Ecol. Evol. 11:1194485. doi: 10.3389/fevo.2023.1194485
Received: 27 March 2023; Accepted: 11 September 2023;
Published: 20 October 2023.
Edited by:
Michael P. Doane, Flinders University, AustraliaReviewed by:
Thamasak Yeemin, Ramkhamhaeng University, ThailandJesse Zaneveld, University of Washington Bothell, United States
Copyright © 2023 Moriarty, Ainsworth and Leggat. This is an open-access article distributed under the terms of the Creative Commons Attribution License (CC BY). The use, distribution or reproduction in other forums is permitted, provided the original author(s) and the copyright owner(s) are credited and that the original publication in this journal is cited, in accordance with accepted academic practice. No use, distribution or reproduction is permitted which does not comply with these terms.
*Correspondence: Tess Moriarty, dGVzcy5tb3JpYXJ0eUB1b24uZWR1LmF1