- 1Department of Crop Production Ecology, Swedish University of Agricultural Sciences, Uppsala, Sweden
- 2Institute of Biology, University of Graz, Graz, Austria
- 3Department of Ecological Dynamics, Leibniz Institute for Zoo and Wildlife Research (LG), Berlin, Germany
- 4Alfred Wegener Institute Helmholtz Centre for Polar and Marine Research (AWI), Bremerhaven, Germany
- 5Doñana Biological Station, Spanish National Research Council (CSIC), Seville, Spain
Editorial on the Research Topic
Stability across spatial and temporal scales
Motivation
Explicitly considering spatial and temporal scale is crucial when studying stability of ecological systems (Levin, 1992; Chase et al., 2018). Because both scale and stability can be defined in different ways (Pimm, 1984; Grimm and Wissel, 1997; Donohue et al., 2016), it is important to explicitly consider the scales at which major ecosystem processes, perturbations, and observations take place—and how each of these scales interact with the stability metric used. For example, the relevant scales for studying the stability of forests are very different from those for grasslands—and different scales must be considered for short-term pulse perturbations (e.g., fires, storms) vs. long-term press perturbations (e.g., nutrient loading, desertification).
Understanding how stability is affected by scale is basic ecological research, but also has important applied implications. For instance, in a successional grassland study (Clark et al., 2019), both invasion-based metrics and asymptotic stability estimates varied so strongly across spatial and temporal scales that no single set of scales existed that fully described community dynamics. In light of global change, and the multiple challenges it poses to ecosystems, understanding the scaling of ecological stability is instrumental for ecology. Some relevant questions to address regarding stability and scale are:
(a) At what scales are particular systems especially vulnerable to specific kinds of perturbations?
(b) At what scales are management interventions likely to be most effective?
(c) What information about a system is necessary in order to scale up small spatial and temporal scale findings to larger scales that are relevant for conservation management?
(d) Where is more research needed in order to measure stability at relevant scales, understand its ramification on ecosystems, and derive cross-system generalizations (e.g., Clark et al., 2021)?
State of stability
Given the numerous recent studies on Ecological stability, it would be useful to review this body of work via the lens of scale. One approach is to map the spatial and temporal scales at which different kinds of ecosystems are typically observed, and compare it with the scales used to study stability. In Figure 1, we showcase such an approach for a limited number of ecosystems and studies, to demonstrate the idea and its utility.
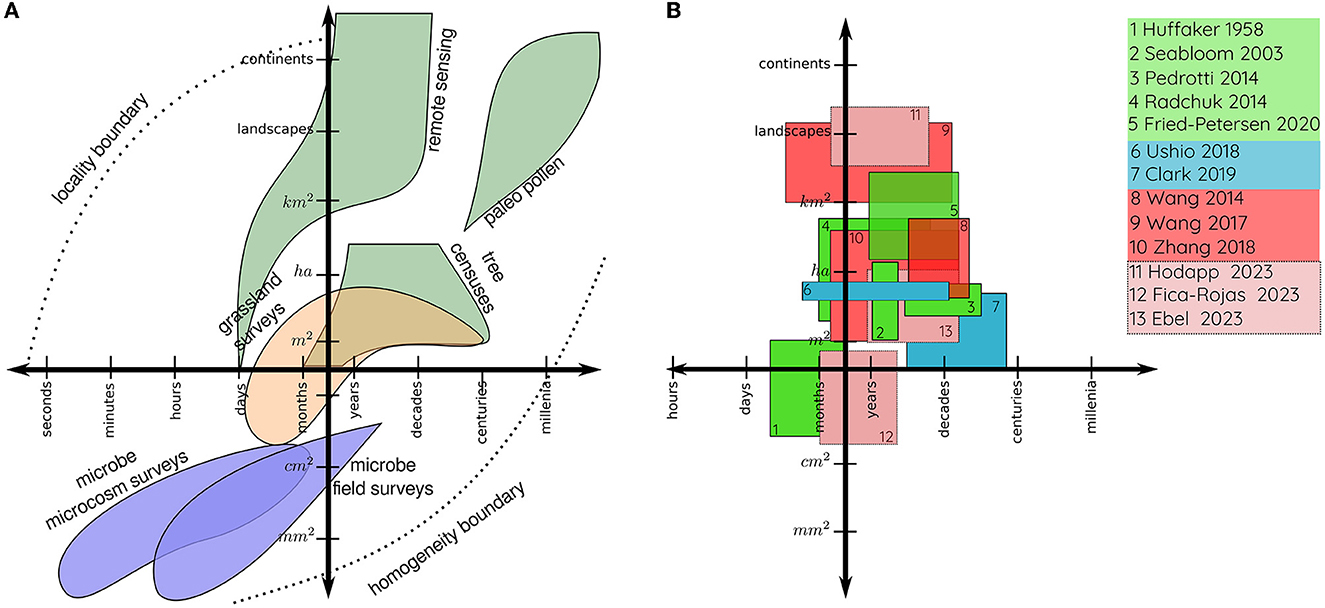
Figure 1. A spatial-temporal map of ecological stability. (A) Scales of observation of different ecosystem types: forests in green, grasslands in orange, and microbes in purple. (B) Scales at which different metrics of ecological stability have been measured: persistence in green, resilience in blue and variability in red. The different studies included in the map are indicated in the legend. The three papers included in this Research Topic where scale is considered in an explicit fashion (11, 12, and 13) are highlighted in light red. Besides the studies referred to in the main text, the figure also refers to the studies of Fried-Petersen et al. (2020), Huffaker (1958), Pedrotti et al. (2014), Radchuk et al. (2014), Seabloom et al. (2003), Ushio et al. (2018), Wang and Michel (2014), and Zhang et al. (2018).
Observations of different ecosystem types, such as forests and grasslands, take place over different regions of our spatial-temporal map, and some regions of this map are not covered at all. Further, many studies focus on small and specific subsets of this map, such as shorter spatial and temporal scales, likely due to logistic constraints associated with observing ecological systems across spatial scales, and the often short-term nature of scientific projects. We also note two demarcation lines, i.e., limits to the scales at which ecological stability can reasonably be studied. The “locality boundary” shows combinations of very large spatial/very short temporal scales, across which the flow of information and materials is too slow to be of much influence (e.g., second-by-second variation at continental scales). The “homogeneity boundary” shows combinations of very small spatial/very long temporal scales, across which processes homogenize completely and thus have no spatial or temporal structure (e.g., century-by-century variation within 1 cm2).
Stability and scales in this Research Topic
Empirical studies differ widely in their temporal and spatial extent and results with respect to stability scaling. For instance, in this Topic spatial scales vary from meters (Fica-Rojas et al.) to hundreds of kilometers (Hodapp et al.) and temporal scales reach from months (Fica-Rojas et al.) to years (Ebel et al.; Hodapp et al.). While some studies did not detect any impact of scale on ecological stability (e.g., Ebel et al.) others demonstrate that scale matters. For example, Hodapp et al. showed that temporal biomass invariability increased with spatial scale. Theoretical studies on stability across scale give insight on which empirical work is most relevant. Studies such as Jarillo et al. clarify when partial sampling is sufficient to infer large-scale stability, and when new methods of extrapolation are necessary. Quévreux and Loreau study how perturbations propagate across space given the community structure, necessary for understanding how stability at small scales determines the behavior of the system at larger scales.
Ways forward
The spatial-temporal maps in Figure 1 show one way to identify gaps in our current scale-wise understanding of ecological stability. We propose that there are at least three categories of knowledge gaps:
1) straight-forward methodology: important gaps in spatiotemporal scales at which studies on stability are conducted, which can be filled by either: (i) synthesis; data are collected at these spatiotemporal scales, but initiative is needed to analyze those data, e.g., the Wang et al. (2017) methodology could probably be applied to global grassland data; or by (ii) data acquisition; appropriate data has not been collected yet, e.g., studies of invariability of soil microbe biomass at the global scale.
2) requiring new theory: important gaps that can be filled by theory (i.e., appropriate data already exist, but the theory needed to analyze them is not yet developed—e.g., studies of persistence or resilience for systems with non-stationary dynamics; Clark et al., 2022). This is most evident when current observations are not possible at certain scales, and theory is needed for extrapolation.
3) limited importance: gaps in observed spatiotemporal scales that are of limited biological importance (e.g., very small spatiotemporal scales for tree communities).
We hope that our proposed approach to mapping spatiotemporal scales of the studies conducted until now will be picked up and used to obtain a general understanding of the gaps pertinent to the field investigating stability across scales. We invite further research instigated by unraveling the gaps: data collection, application of available methods to already collected data, and further development of theory and tools, to advance toward a more coherent understanding of ecological stability.
Author contributions
All authors contributed to the conception and writing of the paper.
Acknowledgments
We would like to thank the participants of the Filling in gaps in global understanding of ecological stability and coexistence (FIGS) workshop for helpful discussions.
Conflict of interest
The authors declare that the research was conducted in the absence of any commercial or financial relationships that could be construed as a potential conflict of interest.
Publisher's note
All claims expressed in this article are solely those of the authors and do not necessarily represent those of their affiliated organizations, or those of the publisher, the editors and the reviewers. Any product that may be evaluated in this article, or claim that may be made by its manufacturer, is not guaranteed or endorsed by the publisher.
References
Chase, J. M., McGill, B. J., McGlinn, D. J., May, F., Blowes, S. A., Xiao, X., et al. (2018). Embracing scale-dependence to achieve a deeper understanding of biodiversity and its change across communities. Ecol. Lett. 21, 1737–1751. doi: 10.1111/ele.13151
Clark, A., Hillebrand, H., and Harpole, W. S. (2019). Scale both confounds and informs characterization of species coexistence in empirical systems. Am. Nat. 194, 794–806. doi: 10.1086/705826
Clark, A. T., Arnoldi, J. F., Zelnik, Y. R., Barabas, G., Hodapp, D., Karakoç, C., et al. (2021). General statistical scaling laws for stability in ecological systems. Ecol. Lett. 24, 1474–1486. doi: 10.1111/ele.13760
Clark, A. T., Mühlbauer, L. K., Hillebrand, H., and Karakoç, C. (2022). Measuring stability in ecological systems without static equilibria. Ecosphere 13, e4328. doi: 10.1002/ecs2.4328
Donohue, I., Hillebrand, H., Montoya, J. M., Petchey, O. L., Pimm, S. L., Fowler, M. S., et al. (2016). Navigating the complexity of ecological stability. Ecol. Lett. 19, 1172–1185. doi: 10.1111/ele.12648
Fried-Petersen, H. B., Araya-Ajoy, Y. G., Futter, M. N., and Angeler, D. G. (2020). Drivers of long-term invertebrate community stability in changing Swedish lakes. Glob. Chang. Biol. 26, 1259–1270. doi: 10.1111/gcb.14952
Grimm, V., and Wissel, C. (1997). Babel, or the ecological stability discussions: an inventory and analysis of terminology and a guide for avoiding confusion. Oecologia 109, 323–334. doi: 10.1007/s004420050090
Huffaker, C. (1958). Experimental studies on predation: dispersion factors and predator-prey oscillations. Hilgardia 27, 343–383. doi: 10.3733/hilg.v27n14p343
Levin, S. A. (1992). The problem of pattern and scale in ecology: the Robert H. MacArthur Award lecture. Ecology 73, 1943–1967. doi: 10.2307/1941447
Pedrotti, E., Rydin, H., Ingmar, T., Hytteborn, H., Turunen, P., and Granath, G. (2014). Fine-scale dynamics and community stability in boreal peatlands: revisiting a fen and a bog in Sweden after 50 years. Ecosphere 5, 1–24. doi: 10.1890/ES14-00202.1
Pimm, S. L. (1984). The complexity and stability of ecosystems. Nature 307, 321–326. doi: 10.1038/307321a0
Radchuk, V., Johst, K., Groeneveld, J., Turlure, C., Grimm, V., and Schtickzelle, N. (2014). Appropriate resolution in time and model structure for population viability analysis: insights from a butterfly metapopulation. Biol. Conserv. 169, 345–354. doi: 10.1016/j.biocon.2013.12.004
Seabloom, E. W., Harpole, W. S., Reichman, O. J., and Tilman, D. (2003). Invasion, competitive dominance, and resource use by exotic and native California grassland species. Proc. Nat. Acad. Sci. 100, 13384–13389. doi: 10.1073/pnas.1835728100
Ushio, M., Hsieh, C. H., Masuda, R., Deyle, E. R., Ye, H., Chang, C. W., et al. (2018). Fluctuating interaction network and time-varying stability of a natural fish community. Nature 554, 360–363. doi: 10.1038/nature25504
Wang, S., Loreau, M., Arnoldi, J. F., Fang, J., Rahman, K. A., Tao, S., et al. (2017). An invariability-area relationship sheds new light on the spatial scaling of ecological stability. Nat. Commun. 8, 15211. doi: 10.1038/ncomms15211
Wang, S., and Michel, L. (2014). Ecosystem stability in space: α, β and γ variability. Ecol. Lett. 17, 891–901. doi: 10.1111/ele.12292
Keywords: stability, perturbation, scale, spatial, temporal
Citation: Zelnik YR, Clark AT, Radchuk V, Hodapp D and Dominguez-Garcia V (2023) Editorial: Stability across spatial and temporal scales. Front. Ecol. Evol. 11:1201269. doi: 10.3389/fevo.2023.1201269
Received: 06 April 2023; Accepted: 02 May 2023;
Published: 16 May 2023.
Edited and reviewed by: Pedro Daleo, Institute of Marine and Coastal Research (IIMyC), Argentina
Copyright © 2023 Zelnik, Clark, Radchuk, Hodapp and Dominguez-Garcia. This is an open-access article distributed under the terms of the Creative Commons Attribution License (CC BY). The use, distribution or reproduction in other forums is permitted, provided the original author(s) and the copyright owner(s) are credited and that the original publication in this journal is cited, in accordance with accepted academic practice. No use, distribution or reproduction is permitted which does not comply with these terms.
*Correspondence: Yuval R. Zelnik, emVsbmlrQHBvc3QuYmd1LmFjLmls