Early developmental stages of a Lower Ordovician marrellid from Morocco suggest simple ontogenetic niche differentiation in early euarthropods
- 1Czech Academy of Sciences, Institute of Geology, Prague, Czechia
- 2Institute of Geology and Palaeontology, Faculty of Science, Charles University, Prague, Czechia
- 3Institute of Earth Sciences, University of Lausanne, Lausanne, Switzerland
- 4Université Paris-Saclay, CNRS, Ministère de la Culture, UVSQ, MNHN, Institut photonique d’analyse non-destructive européen des matériaux anciens, Saint-Aubin, France
- 5Department of Earth and Environmental Sciences, The University of Iowa, Iowa, IA, United States
- 6Department of Palaeontology, Staatliches Museum für Naturkunde, Stuttgart, Germany
Early developmental stages of euarthropods are exceptionally rare in the fossil record. This hampers our understanding of the biology, phylogeny, and development of this extremely diverse metazoan group. Herein, we use classical paleontological methods in combination with synchrotron X-ray microtomography to explore the morphology in ca. 480 million-year-old early developmental stages of the Lower Ordovician Fezouata Shale marrellid euarthropod. These stages range between 3.8 and 5.3 mm in length and are characterized by three distinct pairs of gently curved spines that projects from the head shield. The first pair of cephalic appendages are represented by uniramous antenullae of a sensory function. The second pair of cephalic appendages is robust, and had an anchoring or stabilizing function. The third cephalic appendage pair is composed of long cylindrical podomeres and was used for walking. The trunk appendages are biramous and consist of an endopod and a lamellate exopod. Two anterior trunk endopods are composed of long slender podomeres and were used for walking, while the more posterior trunk endopods bear robust endites and associated setae and were used for food gathering. The trunk of the earliest developmental stages is composed of thirteen segments, in contrast to more than 22 segments in the adult trunk. The similar appendage morphology and differentiation along the body is evident in adult individuals of the Fezouata marrellid, suggesting these different developmental stages shared similar methods of locomotion and food processing. Given that adults and juveniles are often preserved in the same or nearby sites, the niche differentiation between these life stages would be the result of the absolute smaller appendage size in immature stages compared to larger adults, effectively differentiating the size of food resources consumed by each. In addition, the delicate setae present in the posterior trunk appendages of early developmental stages might have been used to capture smaller food particles. This simple mode of ontogenetic niche differentiation might have been common in the early diverging euarthropod groups.
1 Introduction
The early post-embryonic stages of various metazoans are important constituents of both recent and past marine ecosystems. This is especially the case for euarthropods – a clade that comprises most metazoans on Earth, both in terms of diversity (Zhang, 2011) and biomass (Bar-On et al., 2018). Extant euarthropods show immensely diverse post-embryonic development, and the same was likely the case for extinct groups, although, in the latter case, limited data is available. The only extinct euarthropod group with a well-documented developmental fossil record are trilobites because they biomineralized their dorsal exoskeleton soon after hatching (Chatterton and Speyer, 1997; Hopkins, 2017; Lerosey-Aubril and Laibl, 2021). Trilobites had hemianamorphic development (i.e., they added segments in their early post-embryonic stages, while later stages show segment stability, Minelli and Fusco, 2013). Trilobites also show both direct and indirect development, with or without distinct metamorphosis (Chatterton and Speyer, 1997; Park et al., 2016; Laibl et al., 2018; Laibl et al., 2021; Laibl et al., 2023), and various modes of feeding in their earliest stages (e.g., Chatterton and Speyer, 1989; Laibl et al., 2017).
In contrast to the rich developmental record of trilobites, early developmental stages of other extinct early Paleozoic euarthropods are rare (Lerosey-Aubril and Laibl, 2021). This is striking, considering that adult euarthropods represent a large part of Paleozoic marine ecosystems (Caron and Jackson, 2008; Caron et al., 2014; Zhao et al., 2014; Van Roy et al., 2015; Paterson et al., 2016; Lerosey-Aubril et al., 2018; Fu et al., 2019; Saleh et al., 2020a). Even within the famous Cambrian Burgess Shale-type (BST) deposits, including the iconic Burgess Shale of Canada and Chengjiang Biota of China, where preservation of soft tissues and lightly sclerotized taxa occur, small euarthropod specimens are an exception and fossils are biased towards later developmental stages and adults, except for the recently discovered Haiyan locality (Yang et al., 2021). Correspondingly, larval or juvenile stages have been described for only a handful of species (e.g., García-Bellido and Collins, 2006; Haug et al., 2013; Fu et al., 2014; Liu et al., 2014; Liu et al., 2016; Fu et al., 2018; Liu et al., 2018; Zhai et al., 2019).
Contrary to BST deposits, Orsten-type (OT) deposits preserve secondarily phosphatized body envelopes of tiny stages of various euarthropods but do not contain samples larger than 2 mm (Maas et al., 2006). OT deposits are thus biased toward small species or early developmental stages, whilst adults are generally absent (Maas et al., 2006). Opposing biases in BST and OT deposits mean that two extreme scenarios exist in the fossil record. Some sites provide extensive data on euarthropod adult morphology, with only limited information on their early developmental stages. In other sites, early developmental stages are exquisitely preserved, but no adults are found. Preservational inconsistencies such as this hamper our understanding of the biology, phylogeny, and development of extinct euarthropods.
Bridging these two extremes, rare Konservat-Lagerstätten contain both early and late developmental stages, as is the case for Haiyan (Yang et al., 2021) and the Fezouata Shale Lagerstätte (Van Roy et al., 2010; Saleh et al., 2021b). Recent discoveries from this Lower Ordovician formation in Morocco have revealed numerous early developmental stages of several euarthropods, such as marrellids (herein), xiphosurans (Lustri and Laibl, personal observation 2018–2023) and trilobites (Laibl et al., in press), in addition to other metazoans (Saleh et al., 2018). The Fezouata Shale therefore appears pivotal to partially fill the gap in knowledge of Paleozoic euarthropod development. In this contribution, we describe exquisitely preserved minute early post-embryonic stages of a formally undescribed marrellid euarthropod from the Fezouata Shale.
Marrellids are exclusively Paleozoic euarthropods whose name derives from Marrella splendens, a rather small (up to 25 mm long) but extremely abundant taxon in the Burgess Shale (Whittington, 1971; García-Bellido and Collins, 2006). Marrellids are characterized by a prominent cephalic shield with two or three pairs of primary spines, a pair of uniramous antennulae, and one or two pairs of cephalic appendages (Whittington, 1971; García-Bellido and Collins, 2006; Kühl and Rust, 2010; Rak et al., 2013; Aris et al., 2017; Moysiuk et al., 2022). Their trunk is composed of numerous cylindrical segments, each associated with a pair of biramous appendages (Whittington, 1971; Stürmer and Bergström, 1976; García-Bellido and Collins, 2006; Kühl and Rust, 2010). Trunk appendages are composed of a six-podomere endopod and a multi-annulated exopod with associated lamellae (García-Bellido and Collins, 2006; Haug et al., 2013). The group is known from Cambrian strata of Canada (Whittington, 1971), China (Zhao et al., 2003; Liu, 2013) and Australia (Haug et al., 2013); and from Ordovician strata of Czechia (Chlupáč, 1999), Morocco (Van Roy et al., 2010), Argentina (Aris et al., 2017), Canada (Moysiuk et al., 2022) and the UK (Legg, 2016); as well as from Devonian strata of Germany (Kühl and Rust, 2010).
While rare minute stages of marrellids have been described earlier, they generally do not show any details of the appendages (García-Bellido and Collins, 2006; Kühl and Rust, 2010) or are represented by a single isolated appendage fragment (Haug et al., 2013). The early developmental stages of the Fezouata marrellid described herein show exquisitely preserved appendages, revealed using synchrotron X-ray microtomography. Immature stages of the Fezouata marrellid have a lower number of trunk segments and a slightly different morphology of the cephalic shield when compared to adults of the same species. However, immature and adult stages share many similarities in the appendage differentiation and similar morphology of individual appendage pairs, suggesting that these developmental stages shared the same locomotion and food processing method. We further discuss the development of Marrellida and its ecological and evolutionary significance.
2 Material and methods
2.1 Geologic, stratigraphic, and environmental context
The Lower Ordovician Fezouata Shale crops out in many sites of southeastern Morocco (Martin et al., 2016a; Lefebvre et al., 2018). In the Ternata plain, north of Zagora, this formation reaches a thickness between 900 and 1000 m (Vaucher et al., 2016) and is formed of blue-green to yellow-green mudstones and siltstones (Destombes et al., 1985; Vaucher et al., 2016). It was deposited in a shallow sea dominated by storms and modulated by tides in very high latitudes of the southern hemisphere (Martin et al., 2016a; Vaucher et al., 2017; Cocks and Torsvik, 2021). Its biota encompasses numerous taxa (at least 183 genera; Van Roy et al., 2015; Saleh et al., 2020a; Saleh et al., 2021b), including a considerable diversity of non-mineralized, sclerotized, or cuticularized organisms (Saleh et al., 2020a; Saleh et al., 2021b), and abundant euarthropods (Van Roy et al., 2010; Van Roy et al., 2015; Martin et al., 2016b; Ortega-Hernández et al., 2016; Pérez-Peris et al., 2021a; Potin and Daley, 2023; Potin et al., 2023).
The soft-bodied preservation is known from three stratigraphic intervals within the succession (Lefebvre et al., 2018; Saleh et al., 2022); the lower interval belongs to the Sagenograptus murrayi graptolite biozone (Tremadocian; Lefebvre et al., 2018; Figure 1A), the middle one is in the ?Baltograptus jacksoni biozone (Floian; Lefebvre et al., 2018) and the upper interval approximately belongs to the Baltograptus minutus biozone–‘Azygograptus interval’ (upper Floian, Saleh et al., 2022). Mineralized animals (e.g., trilobites, brachiopods, bivalves) colonized a large part of the proximal–distal axis of the environment (i.e., from around the fair-weather base (FWB) to below the storm-weather base (SWB); Saleh et al., 2018; Saleh et al., 2021a; Saleh et al., 2021c) and are distributed discontinuously throughout the entire succession (Saleh et al., 2021c). On the other hand, soft-bodied preservation occurs mainly in situ in the lower and middle intervals, predominantly in one facies, deposited right under the SWB (Figure 1B), although sclerotized organisms may occur on some occasions in more proximal settings (Saleh et al., 2020d). The depositional setting of the Fezouata Shale is corroborated by normally graded, storm-induced deposits covering organisms (Figure 1C), and most of the exceptional fossil preservation occurs under rather than within these beds (Saleh et al., 2020d). These soft-bodied taxa were often originally preserved in minute details (Van Roy et al., 2015; Saleh et al., 2019) as carbonaceous compressions with authigenic minerals such as pyrite (Saleh et al., 2020c; Saleh et al., 2020d). However, most of the pyrite was oxidized into iron oxides and oxyhydroxides (Saleh et al., 2020b; Saleh et al., 2020d; Potin et al., 2023), and the carbonaceous remains were leached by processes of modern weathering, such as precipitation.
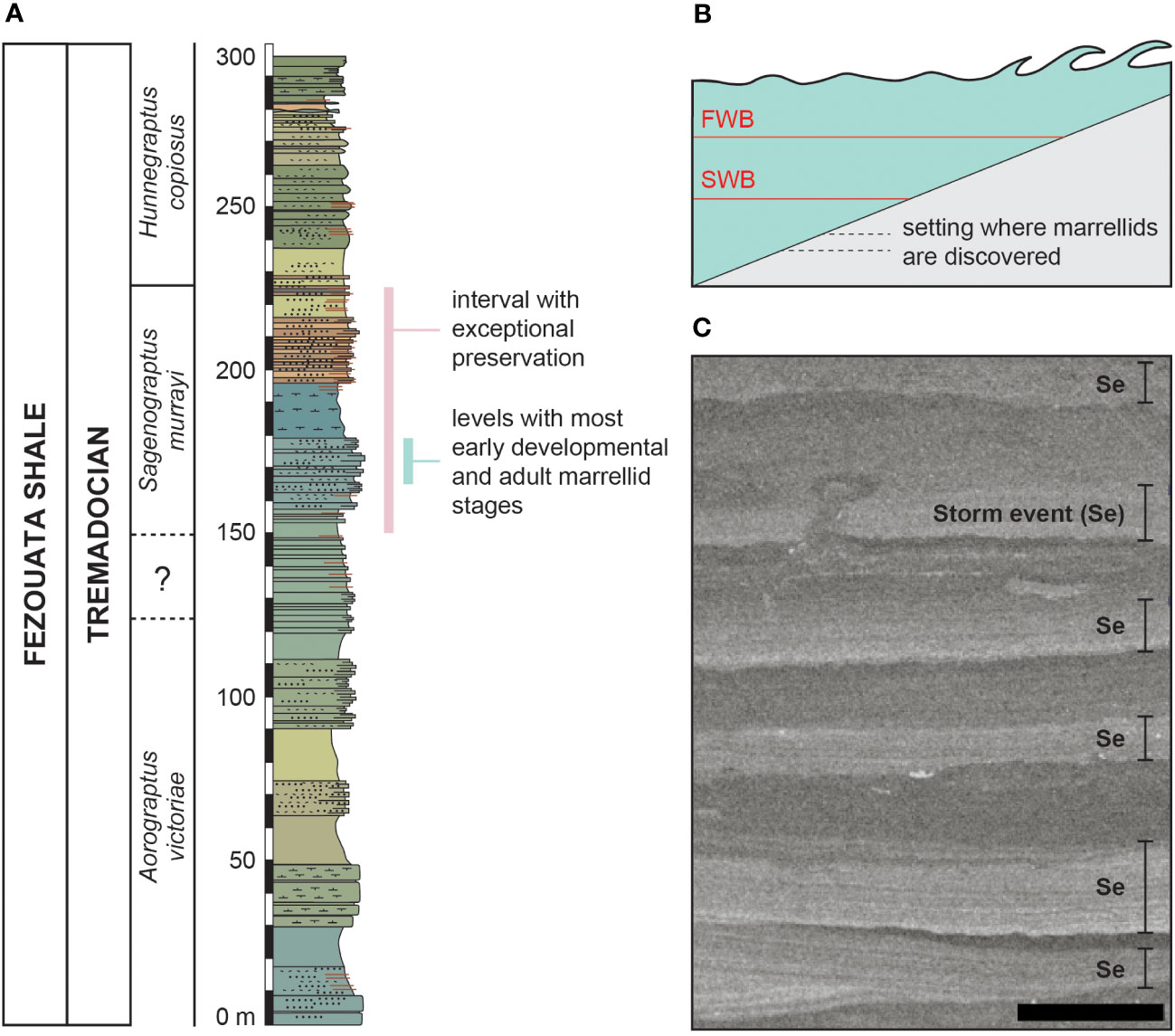
Figure 1 Stratigraphic (A) and environmental (B) settings of the Tremadocian levels of Fezouata Shale with a particular focus on the levels with exceptional preservation. The drill core section (C) shows repeated storm events within the Sagenograptus murrayi Zone. Scale bar in C represents 1 cm. FWB, fair wave base; SWB, storm wave base.
The majority of marrellid specimens studied herein were collected from the facies deposited right below the SWB from the lower interval with exceptional preservation and are Tremadocian in age (Figure 1A). The immature and adult marrellids co-occur in the same excavation sites/collection pits, or come from localities separated by tens of meters on the same hill in nearly equivalent levels with similar lithology and facies.
2.2 Material and institutional repositories
In total, 16 immature specimens of the Fezouata marrellid were studied. Three adult specimens were studied for comparative purposes and are also figured. The studied material is housed and accessioned in public collections, namely in the Muséum cantonal des sciences naturelles, Département de géologie, Lausanne, Switzerland (MGL, 16 specimens) and in the Yale Peabody Museum of Natural History, New Haven, CT, USA (YPM, 3 specimens). All material of Fezouata marrellid used in this study was collected by authorized and academically recognized avocational Moroccan collector Mohamed Ben Moula and his family from 2015 to 2016 (MGL collection), and between 2009 and 2014 (YPM collection); two of the authors (AD and LuL) and Peter Van Roy worked in collaboration with them to produce the metadata associated with the collected fossils.
Mohamed Ben Moula has a long-standing working relationship with several academics, has received the Mary Anning Award from the Palaeontological Association in 2017, and has a radiodont fossil (Aegirocassis benmoulai) named after him in honor of his great contribution to the field of paleontology. The MGL fossil collection was purchased with funds from the University of Lausanne and the Swiss National Science Foundation, following all regulations for purchases. The fossil collection was transported to Casablanca and subjected to export approval by the Ministry of Energy, Mines, and the Environment of the federal government of the Kingdom of Morocco and approved for shipment to Switzerland on 11.05.2017 (export permits curated with the collection). Fossils were shipped by sea and land to the University of Lausanne, where they are curated as part of the collection of the Muséum cantonal des sciences naturelles, Département de géologie. The collections of the Yale Peabody Museum of Natural History were obtained both through direct collection of specimens by Peter Van Roy during fieldwork, and through purchase using dedicated museum funds for the acquisition of scientific collections. Export permits were obtained through the Moroccan Ministry of Energy, Mines, and the Environment, with specimens being transported from Casablanca by sea. For the list of studied immature specimens with details of their preservation and locality data see Supplementary data.
2.3 Taxonomic remarks
The specimens studied herein belong to a marrellid marrellomorph provisionally described by Van Roy (2006) as a new species, Furca mauretanica (nomen nudum). Subsequent papers to figure specimens of the Fezouata marrellid (Van Roy et al., 2010; Van Roy et al., 2015; Lefebvre et al., 2016; Martin et al., 2016a; Vaucher et al., 2016; Saleh et al., 2021a) continued to refer to the species either as Furca sp., or as a marrellomorph arthropod, probably belonging to the genus Furca, yet these fossils have not been formally described. A formal taxonomic description and naming of the Fezouata marrellid falls outside the scope of this paper, which focuses on its ontogeny, and particularly the morphology of its early developmental stages, and will no doubt be addressed independently of this work. As such, we refer to the species throughout this work as the ‘Fezouata marrellid’.
2.4 Preparation and documentation of material
Specimens were mechanically prepared with a Micro-Jack 4 pneumatic air scribe equipped with a pointed stylus. Photographs were taken using a digital camera Canon EOS 800D coupled with a Canon MP-E 65-mm 1:2.8 1-5X macro lens. A polarizing filter was attached to the lens and the light source to reduce reflections and enhance the contrast between the rock and the specimen. To further increase the contrast, all specimens were immersed in diluted ethanol. The images were subsequently processed in Adobe Lightroom 4.3, to enrich brightness, contrast, shadows, highlights, colors, and saturation. Line drawings as well as all reconstructions and diagrams were made in Adobe Illustrator 25.3.1.
The immature specimens were measured using the optical image analyzer TpsDig2 2.31 (Rohlf, 2006). Eight dimensions of the immature specimens were measured, namely the maximal length and width of the cephalic shield (both including and excluding the cephalic spines), the lengths of the anterolateral, mediolateral, and posterolateral cephalic spines, and the observed length of the trunk (see Supplementary data). The measured length and width of the cephalic shield (excl. spines) of 15 specimens were plotted on bivariate plots (Figure 2) using PAST 4.02 (Hammer et al., 2001).
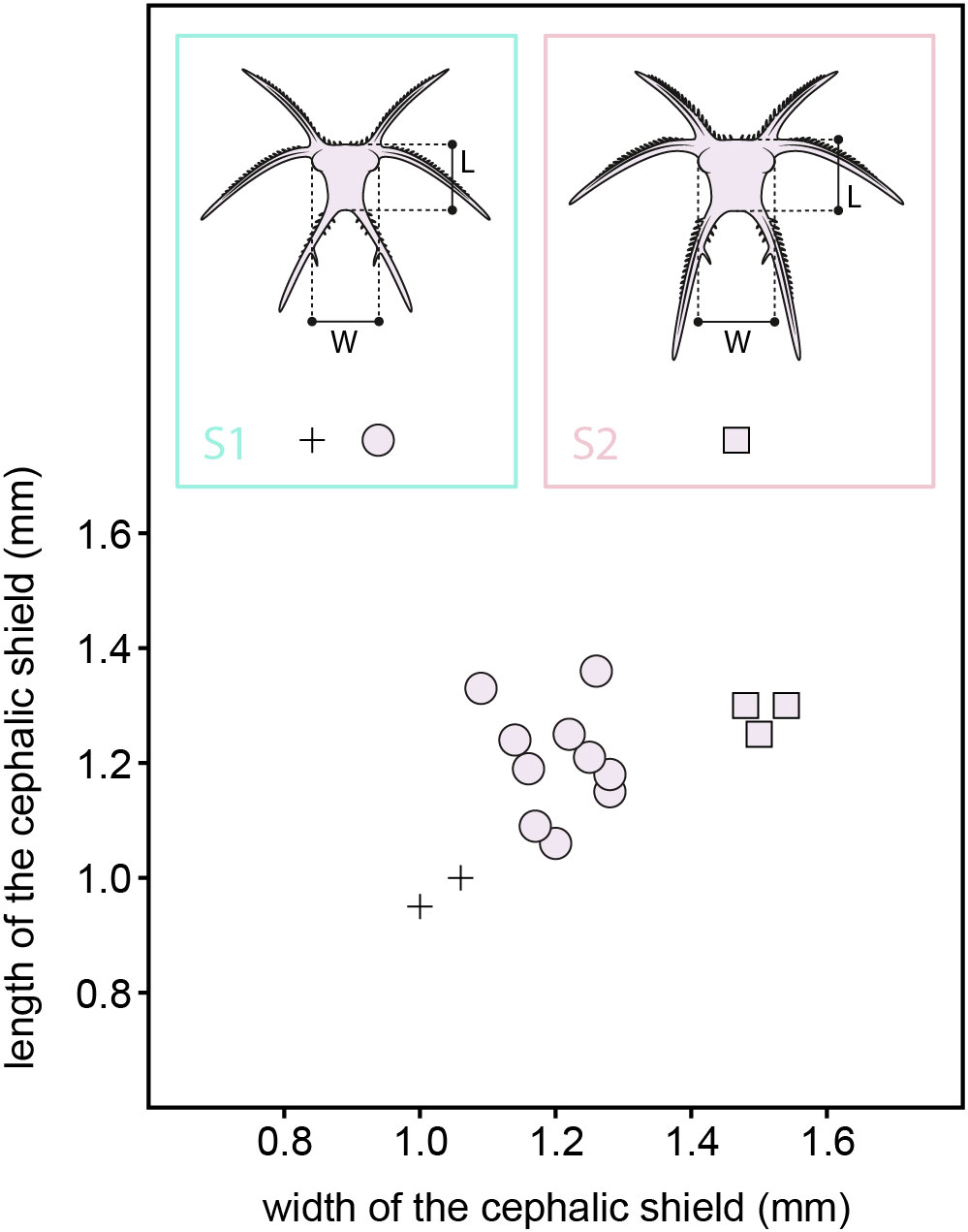
Figure 2 Cephalic shield width vs. length plot of the early developmental stages of Fezouata marrellid. L, cephalic shield length; S1-2 early developmental stages 1 and 2; W, cephalic shield width.
2.5 Synchrotron imaging
The ventrally projected anatomical features such as the hypostome and appendages are rarely exposed on the surface of the fossil samples. For that reason, one specimen (MGL 102399) was imaged using synchrotron X-ray microtomography at the X02DA TOMCAT beamline of the Swiss Light Source, Paul Scherrer Institut, Villigen, Switzerland. Acquisitions were performed using a monochromatic beam of 38 keV, a single propagation distance of 250 mm, a 100 µm LuAg : Ce scintillator, and a 4× objective, yielding reconstructed tomographic data with a voxel size of 1.75 µm. 1501 projections were recorded over 180° with exposure of 1000 ms. Reconstruction was performed on a 60-core Linux PC farm using a Fourier transform routine and a regrinding procedure (Marone et al., 2010). Manual segmentation using level tracing and three-dimensional rendering were performed using 3D Slicer (https://www.slicer.org/). A selection of tomograms, as well as sum intensity projections of several (30 to 110) tomograms processed using ImageJ, are presented in Figure 3.
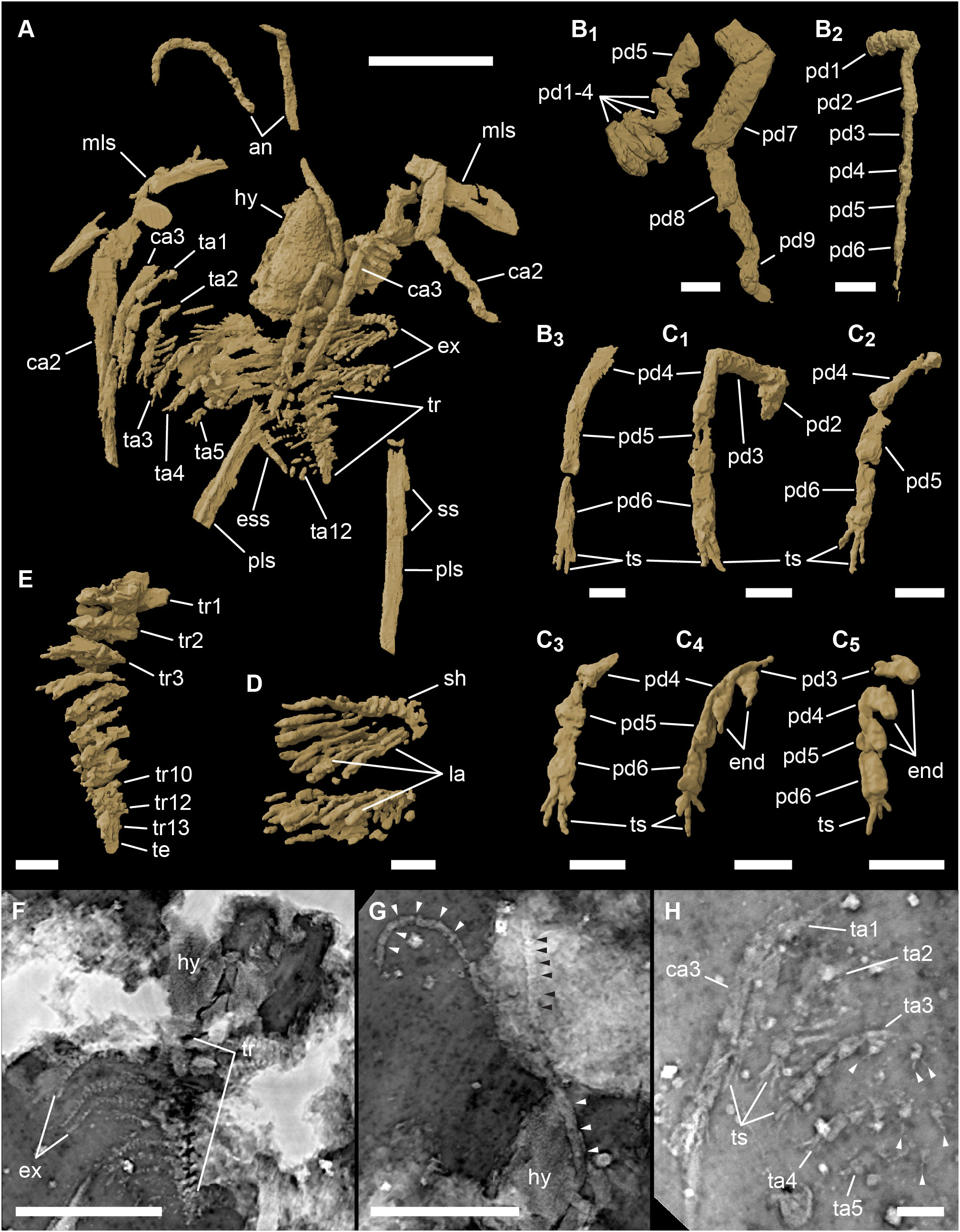
Figure 3 3D rendering of the early developmental stage (S1) of Fezouata marrellid based on synchrotron X-ray tomography, Fezouata Shale, Lower Ordovician, Morocco. (A) oblique ventral 3D rendering of the entire specimen no. MGL 102399. (B) details of cephalic appendages: (B1) second cephalic appendage; (B2) third left cephalic appendage; (B3) third right cephalic appendage. (C) details of trunk appendages: (C1) first left trunk appendage; (C2) first right trunk appendage; (C3) second right trunk appendage; (C4) third right trunk appendage; (C5) fourth right trunk appendage. (D) detail of the trunk exopods. (E) detail of trunk segments. (F–H) sum intensity projections of 30 tomograms through the trunk (F) 75 tomograms through the antennulae (G), and 110 tomograms through right appendages (H). Scale bars represent 500 µm in (A), (F), and (G), and 100 µm in (B–E) and (H). an, antennula; ca2–ca3, cephalic appendage 2 and 3; end, endites; ess, enlarged secondary spine; ex, exopods; hy, hypostome; la, lamellae; mls, mediolateral spine; pd1–pd9, podomere 1 to 9; pls, posterolateral spine; sh, shaft; ss, secondary spines; ta1–ta12, trunk appendage 1 to 12; te, terminal piece; tr, trunk; tr1–tr13, trunk segments 1 to 13; ts, terminal spines. Arrows in G point to article boundaries, in H to setae.
3 Results
3.1 Description of the early developmental stages of the Fezouata marrellid
Plotted dimensions of the cephalic shields (excluding spines) of the 15 smallest measured immature specimens reveal three separate clusters (Figure 2). The cluster with the smallest specimens contains only two samples, 1.00 mm and 1.06 mm wide, and 0.95 mm and 1.00 mm long, respectively. The middle cluster contains ten specimens that range from 1.16 mm to 1.28 mm in width, and from 1.06 mm to 1.36 mm in length. The last cluster is composed of three individuals that range from 1.48 mm to 1.54 mm in width and 1.25 mm to 1.30 mm in length. The smallest and middle cluster individuals share cephalic shields that are morphologically indistinguishable from each other. In contrast, the cephalic shield of the largest cluster individuals differs from that of the smaller individuals in several aspects (see below). For that reason, we interpret the smallest and middle cluster individuals as belonging to early developmental stage 1 (S1), and the largest cluster individuals as belonging to the early developmental stage 2 (S2). This numbering only refers to observed early developmental stages and does not implie that the stages smaller than S1 were not present in the ontogeny of Fezouata marrellid.
The S1 cephalic shields are 3.8–4.6 mm long and 4.8–5.1 mm wide, including the cephalic spines. The shield is sub-trapezoidal in outline, narrowing posteriorly (Figures 4A, B). Three pairs of long cephalic spines project from the cephalic shield (als, mls and pls in Figure 4A). The anterolateral spines (als) are gently curved, 1.28–2.05 mm long, and diverge forward at an angle about 70° near their base. The mediolateral spines (mls) are the longest spine pair in these early stages, with a total length of 1.88–2.35 mm. They are directed laterally and slightly posteriorly, each diverging from the transversal axis about 10–15° in their proximal parts and curving slightly backwards. The anterior margin of the cephalic shield, anterolateral, mediolateral, and posterolateral spines bear numerous small secondary spines (ss in Figures 3A, 4B) developed along their anterior (or outer) margins. In addition, the posterolateral spines bear one elongated secondary spine (ess in Figure 3A), developed on the inner edge approximately at the midpoint of the primary spine. There is a distinct bulge near the posterior base of the mediolateral spines on both sides of the cephalic shield (eb in Figures 4A, B); these bulges are interpreted as a dorsal exoskeletal protrusion that might accommodate ventrally-situated eyes. The posterolateral spines (pls) are straight, 2.07–2.40 mm long, and diverge posteriorly at an angle of 50–60°.
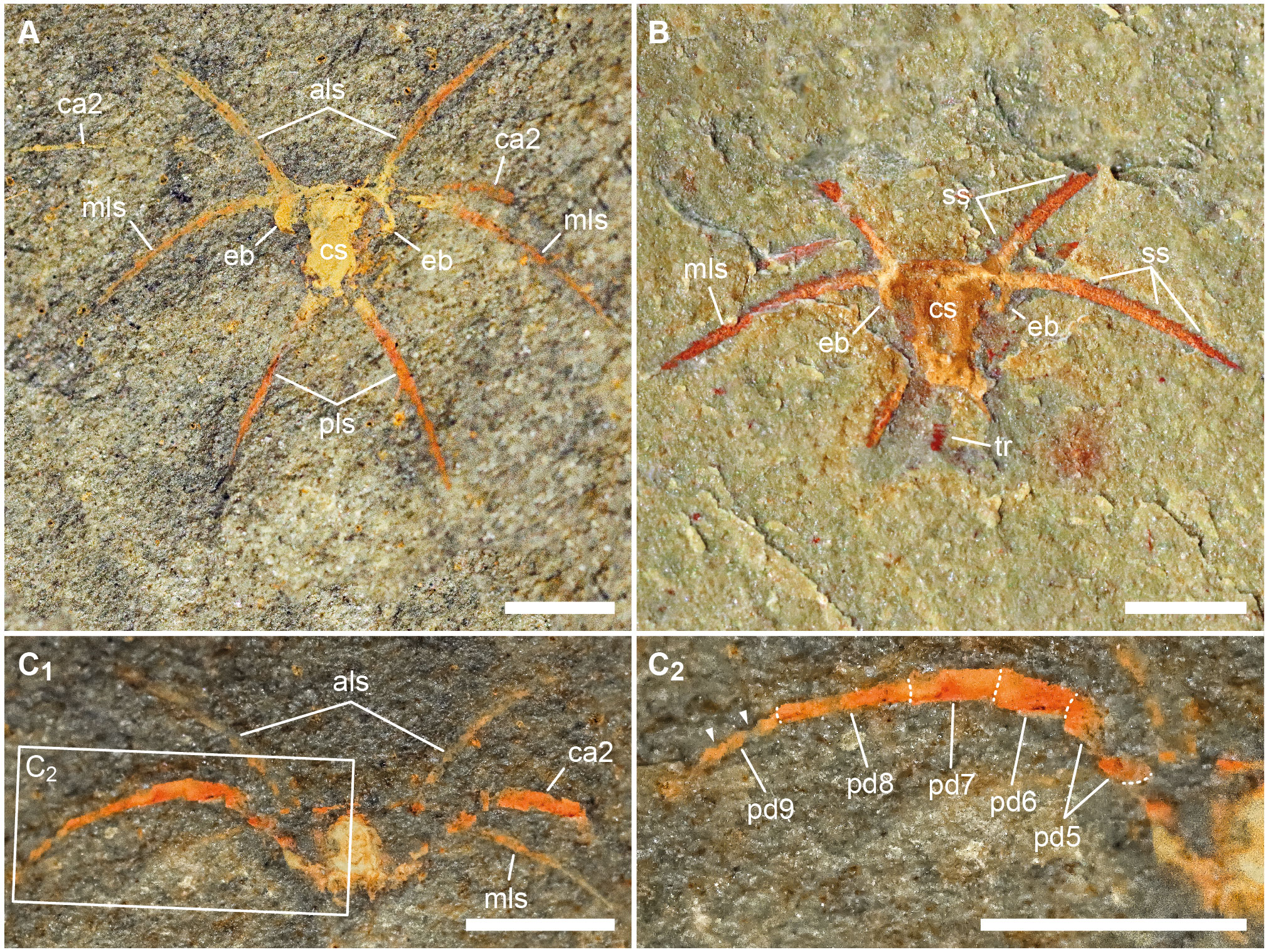
Figure 4 Early developmental stages (S1) of Fezouata marrellid, Fezouata Shale, Lower Ordovician, Morocco. (A) specimen no. MGL 102141; (B) specimen no. MGL 102188; (C1) specimen no. MGL 102143; (C2) detail of second cephalic appendage in the specimen no. MGL 102143. Scale bars represent 1 mm. als, anterolateral spines; ca2, cephalic appendage 2; cs, cephalic shield; eb, eye bulge; mls, mediolateral spine; pd5–pd9, podomere 5 to 9; pls, posterolateral spine; ss, secondary spines; tr, trunk.
The S2 cephalic shields are 4.5–5.3 mm long and 4.6–5.6 mm wide, including spines. Morphologically, the S2 cephalic shields are similar to those of the S1. The major difference between them is the morphology of the posterolateral cephalic spines (cf. Figures 4, 5). These spines are proportionally the longest spine pair in the S2 (Figures 5A, C), and diverge at an angle of about 35–40°. The secondary spines are developed both on the outer and inner edges of the posterior spines. The enlarged secondary spine is located on the inner edge, ca. one-third of the distance from the posterolateral spine base (Figure 5B).
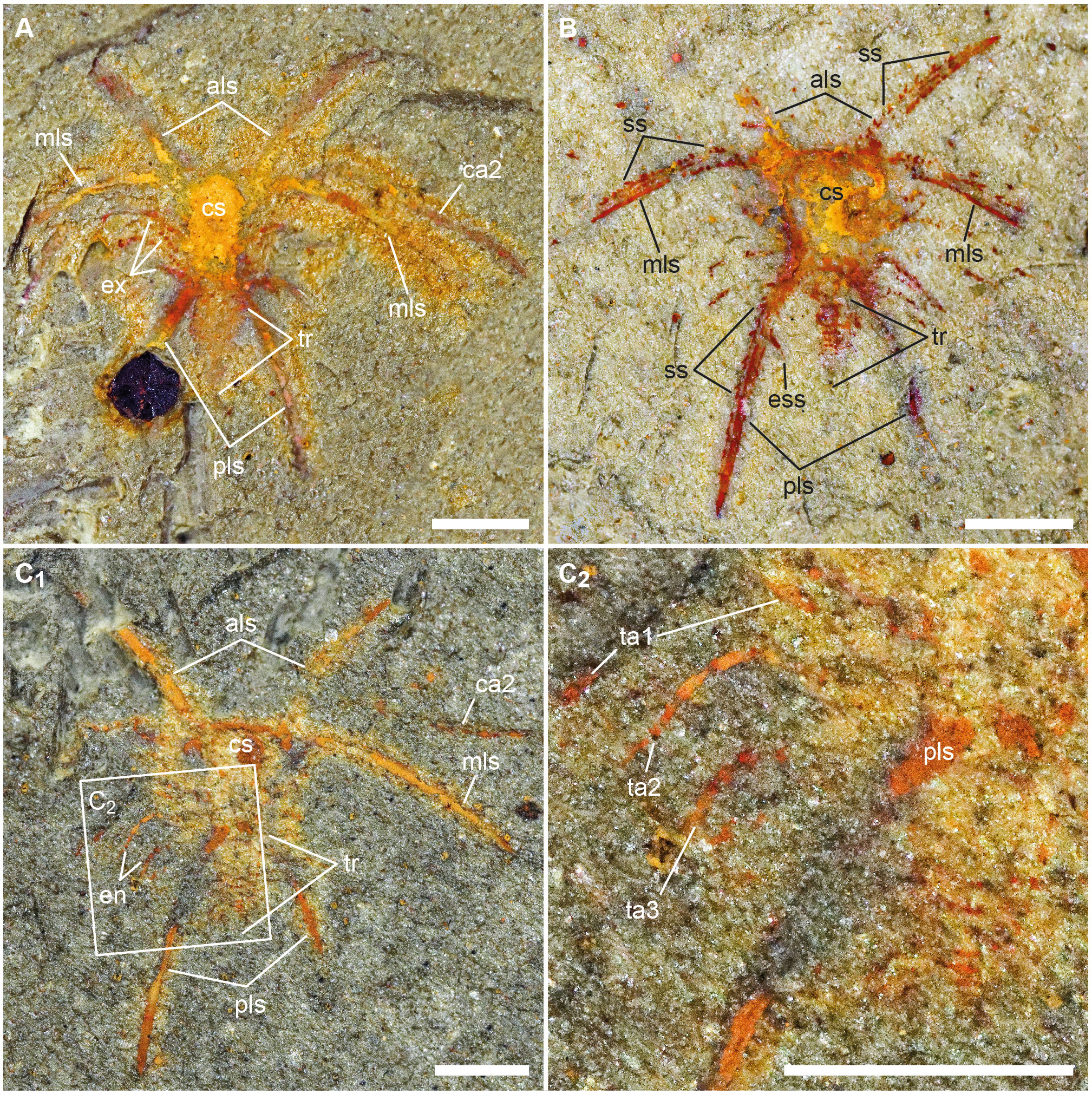
Figure 5 Early developmental stages (S2) of Fezouata marrellid, Fezouata Shale, Lower Ordovician, Morocco. (A) specimen no. MGL 102187; (B) specimen no. MGL 102382; (C1) specimen no. MGL 102140; (C2) detail of the specimen no. MGL 102140. Scale bars represent 1 mm. als, anterolateral spines; ca2, cephalic appendage 2; cs, cephalic shield; en, endopods; ess, enlarged secondary spine; ex, exopods; mls, mediolateral spine; pls, posterolateral spine; ss, secondary spines; ta1–ta3, trunk appendage 1 to 3; tr, trunk.
The hypostome of early developmental stages is developed ventrally from the cephalic shield (hy in Figures 3A, F, G). It is a prominent elongated structure, generally ovoid in outline, covers most of the ventral part of the cephalic shield, and posteriorly reaches to the final cephalic appendages.
The first pair of cephalic appendages are in the form of uniramous antennulae (an in Figure 3A). They are elongated (ca. 1.5 mm long in the smallest recorded specimen) and circular in cross-section. Each antennula is composed of numerous short articles (Figure 3G). Although these are not always easily discernible, it seems that the proximal and distal parts are composed of proportionally longer articles than the middle part of the antennula, which is composed of rather short articles (cf. arrowheads in Figure 3G), though this might also be caused by the orientation of the antennula in sediment. The antennulae insert into the cephalon laterally from the hypostome (Figures 3A, G), posterior to the base of the mediolateral spine, that is, in the area that would be behind the eye bulge, implying their deutocerebral origin.
The second cephalic appendage is robust, uniramous, and seemingly homologous to the second cephalic appendage of Marrella, Mimetaster, and Tomlinsonus based on its similar morphology and topological position (cf., García-Bellido and Collins, 2006; Kühl and Rust, 2010; Moysiuk et al., 2022). This appendage is the longest one (total length 2.3 mm in specimen MGL 102143, Figure 4C) and consists of nine podomeres (pd1–9 in Figure 3B1). The four most proximal podomeres are stout, short, and densely packed, resembling their homologs in Mimetaster and Tomlinsonus (cf., Stürmer and Bergström, 1976; Moysiuk et al., 2022). Podomere five is comparatively long (about 0.4 mm long in specimen MGL 102143), with the width being about 45% of the podomere length. Podomeres six and seven are medium-sized (about 0.3 mm long in specimen MGL 102143), their width/length proportions being 35–45%. Both the sixth and seventh podomeres are narrowest proximally and gently widen distally. Podomere eight is the longest one (0.45 mm long in specimen MGL 102143) and is shaped like a long, slender cylinder (width being only 15% of its length). The nature of the last podomere is somewhat obscured, and it is not clear if it is composed of one segment (as in Marrella and Tomlinsonus, cf., Whittington, 1971; Moysiuk et al., 2022) or tarsus-like annuli (as in Mimetaster, cf., Stürmer and Bergström, 1976; Kühl and Rust, 2010). Both the synchrotron data and the microscopic observation of exposed specimens are ambiguous in this respect, although the contraction in specimen MGL 102143 (arrowheads in Figure 4C2) and the slightly flexed tip in specimen MGL 102399 (Figure 3B1) favor the latter morphology.
The third cephalic appendage is long, slender, and composed of six elongated cylindrical podomeres (Figures 3B2, 3), with their width/length proportions being about 25–30%. The individual podomeres are of similar lengths, with only the most proximal and two distal-most podomeres being slightly shorter than the rest. This appendage terminates with four rather long, tightly-packed terminal spines (ts in Figures 3B3, H). The third cephalic appendage inserts just posteriorly to the second one and bears no exopod.
The trunk projects from under the cephalic shield between the posterolateral spines (Figures 3A, 5A, B). In both S1 and S2, the entire length of the trunk never exceeds the length of the posterolateral spines. The length of the trunk is about 0.6 mm in the S1, while in the S2 the trunk ranges between 1.14–1.37 mm. In the S1, the trunk reaches up to the enlarged secondary spine of the posterolateral spines and is composed of 13 segments and a terminal piece (te in Figure 3E). The trunk segments are cylindrical, wider transversely than sagittally, each bearing one pair of post-cephalic appendages. In the S2, the number of segments in the trunk is unknown.
The trunk appendages (ta in Figure 3A) are biramous and consistent in general morphology. The endopods of trunk appendages 1 and 2 are each composed of six elongated cylindrical podomeres (the proximal ones are poorly preserved) with their width/length proportions being 25–40% (Figures 3C1, 3). The individual podomeres are of similar length, with podomeres five and six being just slightly shorter than podomeres two to four. The distal tips of the endopods each bear four tightly-packed terminal spines (ts in Figures 3C1, 3, H). The exopod is composed of the central shaft (sh in Figure 3D) and comparatively long lamellar setae (la in Figure 3D), similar to those in Austromarrella (Haug et al., 2013).
From the third trunk appendage posteriorly, endopod morphology changes considerably. The podomeres are short (width to length ratio of around 80%) and at least podomeres two to five bear stout sub-triangular endites (end in Figures 3C4, 5). These endites are sharp and project from the endopod medioventrally. The endites are nearly as long as the diameter of the corresponding podomere and some appear to bear delicate setae (arrowheads in Figure 3H). Podomere six lacks the endite and bears four terminal spines on its distal tip (ts in Figures 3C4, 5, H). The endopods of appendage that is posterior to trunk appendage 6 are poorly preserved in specimen MGL 102399, but they diminish in size rapidly, resulting in the minute size of the thirteenth trunk appendage (Figure 3A).
3.2 Description of adults of the Fezouata marrellid
Despite lacking a formal description, the Fezouata marrellid was identified and preliminarily described by Peter Van Roy in his Ph.D. thesis as Furca mauretanica (nomen nudum). The adults of this species have been figured in numerous papers (Van Roy et al., 2010; Van Roy et al., 2015; Lefebvre et al., 2016; Martin et al., 2016a; Vaucher et al., 2016; Saleh et al., 2020a; Saleh et al., 2020d; Saleh et al., 2021b; Drage et al., 2023). Sufficient data exist on the adult specimens to allow for a comparison with the immature material, which is the focus of this study. We therefore describe the adult specimens only briefly, and where appropriate refer to already published figures. In addition, we figure three adult specimens to illustrate the morphological characters discussed and to allow for direct comparison to the immature material.
The adult stages of the Fezouata marrellid have an elongated subtrapezoidal cephalic shield, which bears three pairs of cephalic spines (als, mls, and pls in Figures 6A1, B1). The size of the adult cephalic shield exceeds 5 mm in width and 6 mm in length (excluding spines). The anterolateral cephalic spines project from the anterolateral corners of the cephalic shield and are strongly curved out and backwards. The mediolateral cephalic spines are positioned just posteriorly from the bases of the anterolateral spine pair. They run sub-laterally initially and then curve backwards. The eye bulge is developed near the posterior base of the mediolateral spine, on both sides of the cephalic shield (eb in Figure 6A1). The posterolateral spines project from the posterior margin of the cephalic shield, point posteriorly and can vary from nearly straight to curved towards the medial axis. All pairs of cephalic spines bear comparatively long falcate secondary spines (ss in Figure 6A1) that are developed on both the external and internal edges of the primary spines. There is no evidence of an enlarged secondary spine on the inner edge of the posterolateral spines.
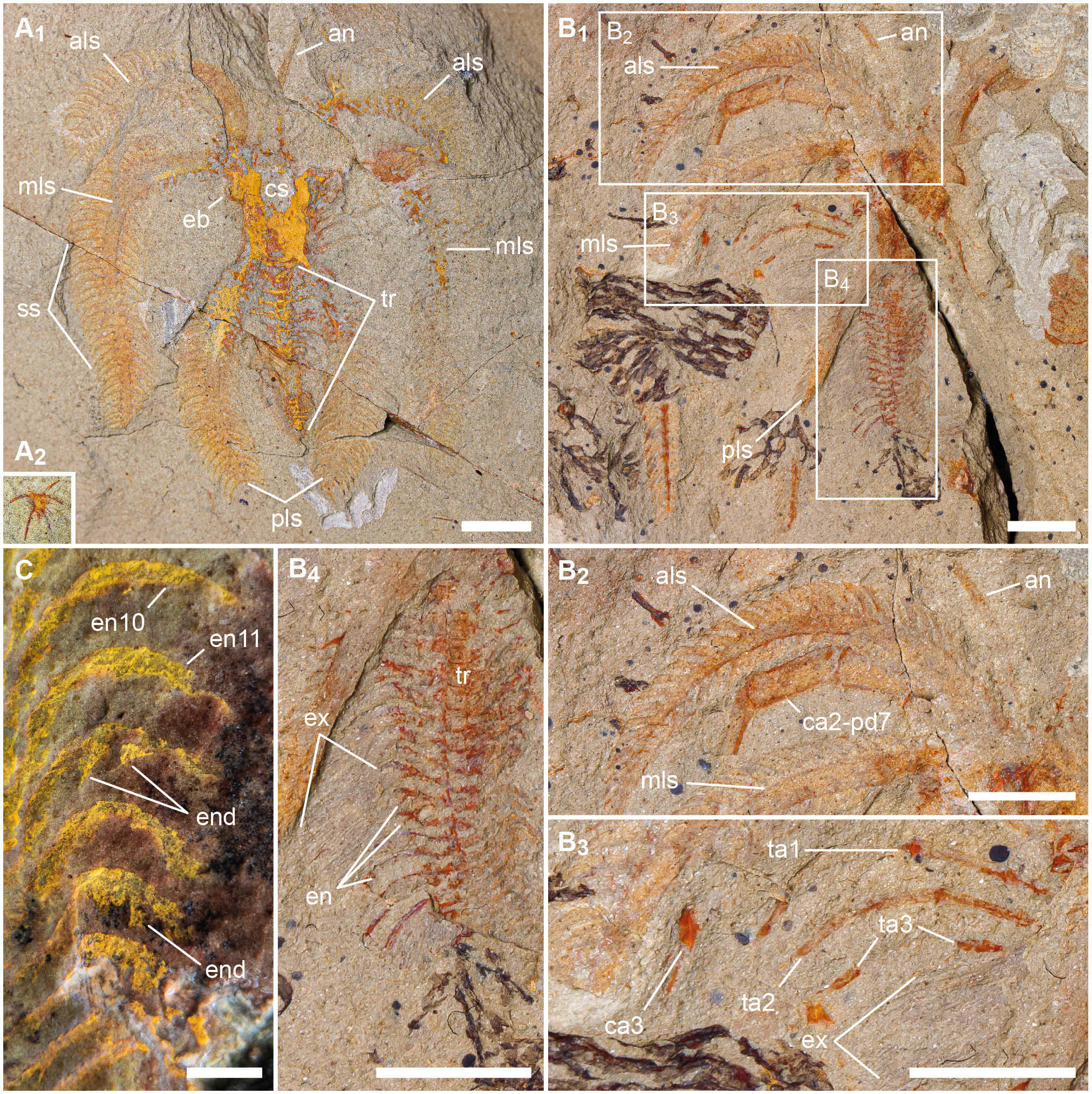
Figure 6 Adult and early developmental stages of Fezouata marrellid, Fezouata Shale, Lower Ordovician, Morocco. (A1) adult specimen no. MGL 102397; (A2) immature specimen (S2) no. MGL 102382 in the same scale as (A1); (B1) adult specimen no. MGL 102390; (B2) detail of the second cephalic appendage of the specimen no. MGL 102390; (B3) detail of trunk appendages of the specimen no. MGL 102390; (B4) detail of the trunk of the specimen no. MGL 102390; (C) detail of the trunk endopods of the adult specimen no. MGL 102392. Scale bars represent 1 cm in A and B, and 1 mm in C. als, anterolateral spines; an, antennula; ca2–ca3, cephalic appendage 2 and 3; cs, cephalic shield; eb, eye bulge; en, endopods; en10–11, endopods 10 and 11; end, endites; ex, exopods; mls, mediolateral spine; pd7, podomere 7; pls, posterolateral spine; ss, secondary spines; ta1–ta3, trunk appendage 1 to 3; tr, trunk.
The first pair of appendages comprise uniramous antennulae (an in Figures 6A1, B1) that are comparatively long and composed of short cylindrical podomeres. The second cephalic appendage is robust, composed of long cylindrical podomeres similar in structure to the second cephalic appendage of early developmental stages (Figure 6B2, see also Martin et al., 2016a, Figure 3C). The third cephalic appendage is composed of very long cylindrical podomeres (Figure 6B3)
The trunk is composed of cylindrical segments that progressively diminish in size posteriorly (Figure 6B4). The total number of trunk segments is over 22 (Figures 6A1, B4). Each trunk segment bears one pair of biramous appendages. The endopods of anterior trunk appendages (at least up to the fourth trunk appendage pair) are composed of long cylindrical podomeres, with a width-to-length ratio of about 20%, and lacking endites (Figure 6B3). The posterior trunk endopods are usually preserved in a curved position. They have comparatively short podomeres (width/length of 50–60%) with pointed endites (Figure 6C). The exopods are composed of a slender shaft and bear long lamellar setae all along the trunk (Figure 6B4).
4 Discussion
4.1 Developmental stage assignment and comparisons
All immature specimens described and figured herein are considered to represent early post-embryonic stages of the undescribed marrellid from the Fezouata Shale. This assignment is based on three lines of evidence. Firstly, the overall morphology of early developmental stage (S1 and S2) and adult cephalic shields is very similar, both bearing three pairs of spines projecting anterolaterally, laterally, and posteriorly fringed with secondary spines, and both with prominent eye bulges on the cephalic shield. Secondly, both early developmental and adult stages have similar appendage differentiation, subdivided from the anterior to the posterior part into antennula, a robust second cephalic appendage, a third cephalic appendage, and a series of biramous trunk appendages of similar composition (see Section 3). Finally, early developmental and adult individuals co-occur in the same stratigraphic levels and facies (Figure 1A), and generally within the same fossiliferous localities in the Fezouata Shale.
The minute size of immature individuals suggests that they represent comparatively early developmental stages; with their whole-body length (excluding spines) ranging between 1.6–2.6 mm (= cephalic shiled + trunk lengths) they are about ten times smaller than the average adult specimens (Figures 6A1, 2). The size of the early developmental stages of Fezouata marrellid is comparable to the size of the larval stage of Leanchoilia illecebrosa (length ca. 2 mm) from the Cambrian Series 2 Chiungchussu Formation of China (Liu et al., 2016), and with larvae of Misszhouia, Leanchoilia, and Isoxys (2.5–3.8 mm) recently reported by Yang et al. (2021) from the same formation. A similar length (2.4 mm) was also reported for the smallest stages of Marrella splendens from the Miaolingian Burgess Shale of Canada (García-Bellido and Collins, 2006; Haug et al., 2013, Figure 3E therein). The size of immature Fezouata marrellids is also comparable to early meraspid stages of various early-diverging Cambrian trilobites (e.g., Dai and Zhang, 2013; Hou et al., 2017; Laibl et al., 2021). Further, the sizes of the early developmental Fezouata marrellid specimens are similar to some Recent marine euarthropods such as the lecithotrophic metanauplii of remipedes (Koenemann et al., 2007), the juvenile stages of cephalocarids (Sanders and Hessler, 1964), the furciliae of euphausiaceans (Mauchline, 1971), and some decapod protozoeae and zoeae (Anger, 2006).
The smallest specimen of the Fezouata marrellid possesses about 13 trunk segments, which would equate to 17 segments plus a terminal piece in total, assuming a four-segmented head. In terms of segmentation, this specimen thereby likely represents an earlier developmental stage than the smallest known specimens of other marrellids, such as Mimetaster hexagonalis (the smallest known specimen has about 19 trunk segments, Kühl and Rust, 2010) and M. splendens (the smallest known specimen has 17 trunk segments, García-Bellido and Collins, 2006; a similar number is plausible for the specimen in Haug et al., 2013, Figure 3E). By the total number of appendages (16 pairs of appendages, derived from 3 cephalic pairs plus 13 trunk pairs), the immature Fezouata marrellid is also comparable to the larva of L. illecebrosa with 14 pairs of appendages. Additionally, the appendages posterior to the fourth appendage pair in the larva of L. illecebrosa have the form of tiny buds (cf., Liu et al., 2016, Figure 4), while in the smallest known specimen of the Fezouata marrellid twelve pairs of anterior appendages are already well developed (Figure 3).
The size and number of segments in the smallest individuals of the Fezouata marrellid suggest they do not represent the earliest post-embryonic developmental stages of the species. However, it is possible that the Fezouata marrellid produced large eggs, like Recent xiphosurans (Shuster and Sekiguchi, 2003) or some anomuran decapods (Lovrich and Vinuesa, 1999), and the immature stages hatched into reasonably advanced forms (i.e., abbreviated development, cf. Rabalais and Gore, 1985). It is equally possible that the Fezouata marrellid hatched into earlier post-embryonic stages than presented here, but that their minute dimensions and fragile exoskeletons might prevent their fossilization. The absence of earlier developmental stages might also result from a collection bias, due to their incredibly small sizes. Similarly, the intermediate developmental stages (i.e., larger than S2 but smaller than adults) with cephalic shield lengths from 1.3 mm to 6 mm are rare in both the MGL (one specimen recorded) and YPM collections (two specimens recorded). Their rarity means that these intermediate stages do not seem to co-occur with adults and early developmental stages (S1 and S2). On the contrary, early post-embryonic stages (S1 and S2) and adults frequently co-occur at the same levels. This latter co-occurrence might be explained by seasonal breeding, and so early developmental stages (S1 and S2) could represent one brood, that lived alongside adults from previous seasons. This might explain why adults and early developmental stages (S1 and S2) are found in association, but without the intermediate stages, though it doesn’t explain why the intermediate size juveniles are generally rarer in the Fezouata Shale.
From a terminological perspective, the immature individuals of the Fezouata marrellid can be characterized as morpho-larva s.l. sensu Haug (2020), as they show morphological differences in the cephalic shield and the number of trunk segments compared to the adults. The immature individuals, however, do not seem to significantly differ in their ecological niches from the adults (see Section 4.4). As such, they do not fulfill the criteria to be characterized as eco-larva (sensu Haug, 2020).
4.2 Morphological comparison of early developmental and adult stages
Despite the numerous similarities between the early developmental and adult stages of Fezouata marrellids, there are several, generally minor, differences (cf., Figures 6–8). The anterolateral and mediolateral cephalic spines are strongly curved in adult specimens, while they are gently curved in juveniles. Such changes in the curvature of primary spines during ontogeny may have been reflected later in marrellid evolution. Mi. hexagonalis, for example, has straight primary cephalic spines (Stürmer and Bergström, 1976; Kühl and Rust, 2010) that resemble those of the immature stages of Fezouata marrellid. The derived marrellids such as Mi. hexagonalis might have acquired their straight cephalic spines via paedomorphic heterochrony.
The secondary spines in adults of Fezouata marrellid are long and strongly developed along both the inner and outer edges of the primary cephalic spines. In immature stages, these secondary spines are minute, developed only along the outer edges of the anterolateral and mediolateral spines, and each posterolateral spine also bears a single elongated secondary spine on the inner margin. These morphological differences between juveniles and adults indicate an allometric growth of both primary and secondary spines in this species.
The differences in the trunk region are related to the lower number of segments and, consequently, the lower number of trunk appendages in immature individuals. The smallest recorded specimen shows 13 trunk segments, while the adults have over 22 trunk segments. It can therefore be reasonably assumed that the Fezouata marrellid grew by anamorphosis, where segments are added during development. Anamorphic development has been suggested in some other marrellids, such as M. splendens (García-Bellido and Collins, 2006) and Mi. hexagonalis (Kühl and Rust, 2010), demonstrating this was a common developmental pattern in Marrellida. Moreover, the anamorphic development was likely a plesiomorphic trait in Marrellida, given this type of development has been suggested to be an ancestral condition for euarthropods (Hughes et al., 2006; Moysiuk and Caron, 2023).
Both early developmental and adult stages share comparatively slender uniramous antennulae, followed by a pair of robust second cephalic appendages and a third pair of cephalic appendages composed of long cylindrical podomeres (Figure 8). The trunk appendages are biramous in both adults and early developmental stages. The exopods have a slender shaft with numerous lamellar setae that differ between the life stages in only their sizes. The immature exopods are also similar to exopods of mature M. splendens (Haug et al., 2013, Figure 3). In the early developmental stages of the Fezouata marrellid, the endopods of the two anterior-most trunk appendages are composed of long cylindrical podomeres, while the more posterior ones have short podomeres with pointed endites (Figure 8). The endites of the posterior endopods also bear delicate setae, while these setae are absent in adults. A similar pattern of appendage differentiation is apparent in adult individuals, although the trunk differentiation is shifted posteriorly (Figure 8). Consequently, in adults the endopods of trunk appendages 1 to 4 are composed of long cylindrical podomeres, while the more posterior trunk appendages have short endite-bearing podomeres. This posterior shift of the trunk appendage differentiation also indicates that some endopod podomeres went through profound allometric changes. For example, the immature endopod of trunk appendage 3 is composed of short endite-bearing podomeres, whereas the homologous endopod in adults is composed of long cylindrical podomeres (Figure 8) meaning the podomeres must have prolonged and lost their endites during development.
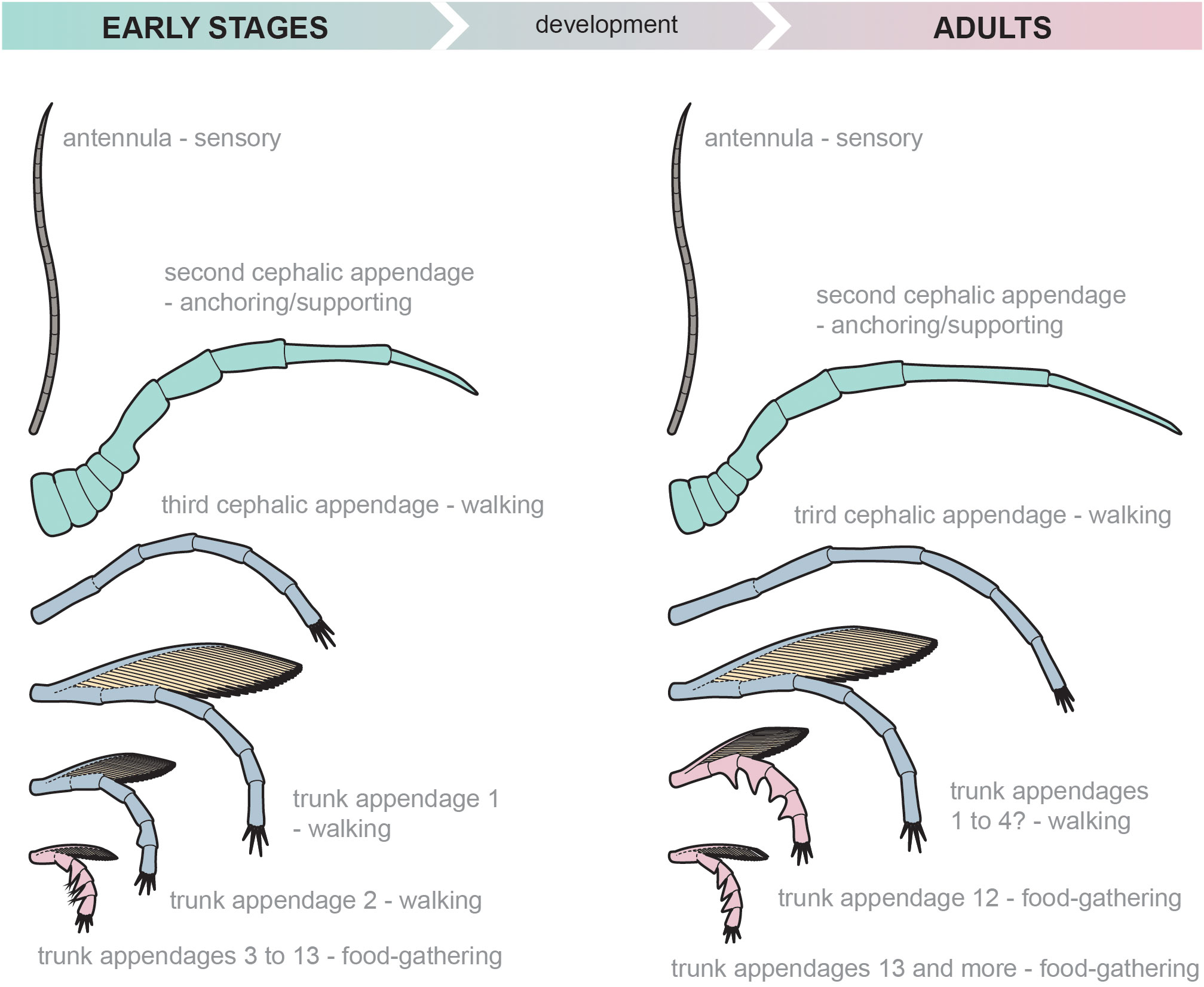
Figure 8 Reconstruction of the appendages of early developmental stages and adults of the Fezouata marrelid. Note the virtually identical appendage differentiation, the posterior shift of the trunk appendage differentiation, and the lack of setae in the posterior trunk appendages of adults. Appendages of the early developmental and adult stages are not to scale.
4.3 Mode of life of early developmental stages of the Fezouata marrellid
Fezouata marrellids were living in high-latitude seasonal seas along the western margin of Gondwana (Saleh et al., 2019; Cocks and Torsvik, 2021; Laibl et al., in press). Considering the morphological similarity in the cephalic shield and appendages of the Fezouata marrellid with those of other marrellids (Whittington, 1971; García-Bellido and Collins, 2006; Kühl and Rust, 2010), it is very likely that the Fezouata species had a benthic or nektobenthic mode of life as proposed for Marrella, Mimetaster, Tomlinsonus, and Furca (Whittington, 1971; Stürmer and Bergström, 1976; Briggs and Whittington, 1985; Conway Morris, 1986; García-Bellido and Collins, 2006; Kühl and Rust, 2010; Rak et al., 2013; Moysiuk et al., 2022). Living close to or on the seafloor, the Fezouata marrellids would have inhabited an offshore environment (Vaucher et al., 2016) with minimal physical disturbance, as evidenced by their deposition below the storm wave base (Saleh et al., 2020a; Saleh et al., 2021b).
The appendages of the immature Fezouata marrellids are morphologically differentiated along the sagittal axis of the body into the antennulae, the second cephalic appendage, the third cephalic appendage, the anterior portion of trunk appendages, and the posterior portion of trunk appendages (Figure 8). The deutocerebral antennulae were likely sensory, as this is their function in many extant mandibulates (Sombke et al., 2012) and as has been suggested for marrellomorphs (Whittington, 1971; Siveter et al., 2007; Kühl and Rust, 2010). However, recent reinvestigation of M. splendens suggests they might have been also used in food gathering (J. Haug, personal communication 2021). The second cephalic appendage might have been used for anchoring, or supporting the animal on the seafloor, given its similarity to the second cephalic appendage of Mi. hexagonalis and Tomlinsonus dimitrii, where it has been interpreted to fulfil this function (cf., Stürmer and Bergström, 1976; Moysiuk et al., 2022). This interpretation is supported by the robust morphology of this appendage, which indicates strong musculature that might have helped to support the cephalic shield and move it above the seafloor. It is unlikely that this appendage was used for swimming, as suggested for M. splendens by García-Bellido and Collins (2006), as, in comparison, M. splendens has a thinner and paddle-like second cephalic appendage with setose fringes.
The last cephalic appendage pair as well as the first two trunk appendage pairs of the early developmental stages of Fezouata marrellid were most likely used for walking on the seafloor. This function is supported by the rather long tightly packed terminal spines in their distal ends. These spines resemble, to some extent, the processes and apoteles of the horseshoe crab pushing legs (Bicknell et al., 2018) or, even more, spines in the prosomal appendages of Weinbergina opitzi (cf., Moore et al., 2005, Figure 6). Such terminal spines in the last cephalic appendage and first trunk appendages of Fezouata marrellid could have pushed the animal over the muddy seafloor in a similar way as in xiphosurans or Weinbergina.
The trunk appendages of the Fezouata marrellid show anteroposterior differentiation into two portions, similar to that observed in adult specimens of M. splendens. The anterior endopods of the latter species are composed of long cylindrical podomeres whereas the posterior endopod podomeres are short and bear prominent endites (cf., Whittington, 1971, p. 13, text – Figure 8; García-Bellido and Collins, 2006, Figure 15C). Whittington (1971) and Briggs and Whittington (1985) argued that this anteroposterior appendage differentiation reflects the mode of life of adult M. splendens. These authors suggested that the long anterior endopods of trunk appendages 1 to 9, with cylindrical podomeres, were used for walking on the seafloor, while the endopods of the posterior appendages were used for food gathering. When Marrella walked on the seafloor, the posterior part of the trunk was curved down, with the posterior endopods forming an arc shape (Whittington, 1971). In this way, the pointed endites on the posterior podomeres formed a network to trap and filter food particles (Whittington, 1971, p. 18; Briggs and Whittington, 1985, p. 156). García-Bellido and Collins (2006) agreed with this interpretation, though they hypothesized that this food capture took place while the animal was swimming. The food gathering function has been also suggested for endites of the trunk endopods of the Silurian marrellomorph Xylokorys chledophilia (Siveter et al., 2007) and for Mi. hexagonalis (Kühl and Rust, 2010), though in these two species, the endites seem to be developed on nearly all trunk appendages. A similar feeding mode might have been also plausible for some trilobites that bear endites in posterior appendages (e.g., Whittington and Almond, 1987; Pérez-Peris et al., 2021b). Consequently, we suggest that appendages posteriorly to the second trunk appendage that shares pointed endites were in the immature stages of Fezouata marrellid employed in food gathering (Figure 8), similar to M. splendens. The network created by pointed endites was likely quite efficient in particle feeding, given the endites were associated with delicate setae. As such, early developmental stages of the Fezouata marrellid were likely able to feed on detritus and/or on the diverse assemblage of unicellular algae found in the Fezouata Shale (Nowak et al., 2016). The function of the trunk exopods in the Fezouata marrellid is less clear, but they likely had a respiratory function as has been suggested for other marrellomorph taxa (Whittington, 1971; Siveter et al., 2007).
4.4 Ecological and evolutionary implications
Both adult and early developmental individuals of the Fezouata marrellid inhabited the same environment, as is evident from their co-occurrence in certain localities and facies. As discussed above (Section 4.2), both adult and immature stages also share the same general appendage differentiation along the body axis (although with different ratios of the walking/food-gathering appendages), showing only minor changes in podomere morphologies and, as expected, differing in overall body size and segment number. It seems reasonable to therefore assume that the minute early developmental stages shared nearly the same modes of locomotion and food processing as the adults (Section 4.3). The smaller size of the immature appendages, the smaller endites on the posterior appendages, as well as the associated setae, suggest the early developmental stages were presumably feeding on smaller particles than the adults. The size differentiation of the food particles would mean that individual developmental phases were not directly competing for food resources, despite similarities in their appendage morphologies and living within the same environments. The posterior shift in trunk appendage differentiation between immature and adult stages (Figure 8, Section 4.2) also suggests that adults had more walking appendages and might have been more mobile than the immature stages. On the contrary, the immature stages likely benefitted from having a higher ratio of the food-gathering limbs, which might have been an adaptation to more efficient nutrient intake.
Ontogenetic niche differentiation is a well-recognized evolutionary strategy that prevents intraspecific competition between juveniles and adults and allows different phases of a life cycle to be subject to different selective forces (Werner and Gilliam, 1984; Ebenman, 1987; Ebenman, 1992; Moran, 1994). It is therefore not surprising that we can observe distinct ontogenetic niche differentiation in the life cycles of many euarthropods. In fossil taxa, this strategy has been reported for the megacheirans Leanchoilia illecebrosa (Liu et al., 2014) and possibly also for Yohoia tenuis (Haug et al., 2012), as well as for the trilobitomorph Naraoia spinosa (Zhai et al., 2019). In all these taxa, the appendages show different morphologies between the immature and adult stages, allowing them to occupy different ecological niches. Similar niche differentiation likely also occurred in trilobites that passed through metamorphoses, such as asaphids, trinucleids, and proetids (cf., Chatterton and Speyer, 1997; Lerosey-Aubril and Feist, 2005; Laibl et al., 2023). In marrellids, this ontogenetic niche differentiation appears to have been driven by the absolute differences in the size between individual developmental stages, by the presence of fine setae on food-gathering appendages, and by the ratio of the walking/food-gathering appendages, rather than by the development of vastly different morphologies, or structures with specific functions. A similar ontogenetic niche differentiation has been demonstrated for the Leanchoilia illecebrosa, which have lost a series of fine setae during the development from larva to adult (cf., Liu et al., 2014).
The phylogenetic position of Marrellida is currently in flux. Marrellids have been resolved within stem-mandibulates (Legg et al., 2013; Legg, 2015; Aria and Caron, 2017a), stem-chelicerates (Aria and Caron, 2017b), close to crustaceans (Chen et al., 2019; Du et al., 2019), or even close to pycnogonids (Vannier et al., 2018; Moysiuk et al., 2022). In any of these scenarios, they tend to occupy an early diverging position within a particular clade. Thus, such a simple mode of ontogenetic niche differentiation might have been common in the early diverging euarthropod groups.
Data availability statement
The datasets presented in this study can be found in online repositories. The names of the repository/repositories and accession number(s) can be found in the article/Supplementary Material.
Author contributions
LuL and PG designed the project under the supervision of AD. LuL photographed the material and measured the specimens. PG collected the synchrotron data and rendered the images. LuL, PG, and FS wrote the original draft. HD, FP-P, LoL, OB, GP, and AD helped to interpret and discuss the results and contributed to the writing of the final version of the manuscript. All authors contributed to the article and approved the submitted version.
Funding
LuL’s research was funded by the Czech Science Foundation (project no. 20-23550Y), and the Center for Geosphere Dynamics (UNCE/SCI/006), and was conducted within institutional support RVO 67985831 of the Institute of Geology of the Czech Academy of Sciences. This research was also funded by the Swiss National Science Foundation, grant number 205321_179084 entitled ‘Arthropod Evolution during the Ordovician Radiation: Insights from the Fezouata Biota’ and awarded to AD. FS work is funded by an SNF Ambizione Grant (no. PZ00P2_209102) and by the Postdoctoral Fellowship of the Faculty of Geosciences and Environment at the University of Lausanne. HD was funded by a Swiss Natural Science Foundation Sinergia Grant (198691).
Acknowledgments
We thank Imran Rahman for beamtime on the X02DA TOMCAT beamline at the Swiss Light Source, and Christian Schlepütz and Federica Marone for assistance at the beamline. Many thanks to Joachim Haug, Carolin Haug, Peter Van Roy, and Javier Ortega-Hernández for inspiring discussions related to Marrellida, Euarthropoda, and their development. The manuscript has benefitted from constructive reviews by James Holmes and Dongjing Fu, as well as from comments by editor Tae-Yoon Park. This work would not be possible without Peter Van Roy’s work on the Fezouata Shale and his detailed field notes. Mark Florence is thanked for access to the comparative material from the Smithsonian collections, and Susan Butts and Jessica Utrup are thanked for providing access to specimens at the Yale Peabody Museum.
Conflict of interest
The authors declare that the research was conducted in the absence of any commercial or financial relationships that could be construed as a potential conflict of interest.
The reviewer JH declared a past co-authorship with the author HD to the handling editor.
Publisher’s note
All claims expressed in this article are solely those of the authors and do not necessarily represent those of their affiliated organizations, or those of the publisher, the editors and the reviewers. Any product that may be evaluated in this article, or claim that may be made by its manufacturer, is not guaranteed or endorsed by the publisher.
Supplementary material
The Supplementary Material for this article can be found online at: https://www.frontiersin.org/articles/10.3389/fevo.2023.1232612/full#supplementary-material
References
Anger K. (2006). Contributions of larval biology to crustacean research: a review. Invertebr. Reproduction. Dev. 49, 175–205. doi: 10.1080/07924259.2006.9652207
Aria C., Caron J.-B. (2017a). Burgess Shale fossils illustrate the origin of the mandibulate body plan. Nature 545, 89–92. doi: 10.1038/nature22080
Aria C., Caron J.-B. (2017b). Mandibulate convergence in an armoured Cambrian stem chelicerate. BMC Evol. Biol. 17, 261. doi: 10.1186/s12862-017-1088-7
Aris M. J., Corronca J. A., Quinteros S., Pardo P. L. (2017). A new marrellomorph euarthropod from the Early Ordovician of Argentina. Acta Palaeontol. Pol. 62, 1–8. doi: 10.4202/app.00240.2016
Bar-On Y. M., Phillips R., Milo R. (2018). The biomass distribution on Earth. PNAS 115, 6506–6511. doi: 10.1073/pnas.1711842115
Bicknell R. D. C., Klinkhamer A. J., Flavel R. J., Wroe S., Paterson J. R. (2018). A 3D anatomical atlas of appendage musculature in the chelicerate arthropod Limulus polyphemus. PloS One 13, e0191400. doi: 10.1371/journal
Briggs D. E. G., Whittington H. B. (1985). Modes of life of arthropods from the Burgess Shale, British Columbia. Trans. R. Soc Edinburgh. Earth Environ. Sci. 76, 149–160. doi: 10.1017/S0263593300010415
Caron J. B., Gaines R. R., Aria C., Mángano M. G., Streng M. (2014). A new phyllopod bed-like assemblage from the Burgess Shale of the Canadian Rockies. Nat. Commun. 5, 3210. doi: 10.1038/ncomms4210
Caron J. B., Jackson D. A. (2008). Paleoecology of the greater phyllopod bed community, Burgess Shale. Palaeogeogr. Palaeoclimatol. Palaeoecol. 258, 222–256. doi: 10.1016/j.palaeo.2007.05.023
Chatterton B. D. E., Speyer S. E. (1989). Larval ecology, life history strategies, and patterns of extinction and survivorship among Ordovician trilobites. Paleobiology 15, 118–132. doi: 10.1017/S0094837300009313
Chatterton B. D. E., Speyer S. E. (1997). ‘Ontogeny,’ in Treatise on invertebrate paleontology, part O, arthropoda 1, trilobita, revised, volume 1: introduction, order agnostida, order redlichiida. Ed. Kaesler R. L. (Boulder, Colorado and Lawrence, Kansas: The Geological Society of America, Inc. and The University of Kansas), 173–248.
Chen X., Ortega-Hernández J., Wolfe J. M., Zhai D., Hou X., Chen A., et al. (2019). The appendicular morphology of Sinoburius lunaris and the evolution of the artiopodan clade Xandarellida (Euarthropoda, early Cambrian) from South China. BMC Evol. Biol. 19, 165. doi: 10.1186/s12862-019-1491-3
Chlupáč I. (1999). Some problematical arthropods from the Upper Ordovician Letná Formation of Bohemia. J. Czech. Geol. Soc 44, 79–87.
Cocks L. R. M., Torsvik T. H. (2021). Ordovician palaeogeography and climate change. Gondwana. Res. 100, 53–72. doi: 10.1016/j.gr.2020.09.008
Conway Morris S. (1986). The community structure of the Middle Cambrian Phyllopod Bed (Burgess Shale). Palaeontology 29, 423–467.
Dai T., Zhang X. (2013). Ontogeny of the redlichiid trilobite Eoredlichia intermediata from the Chengjiang Lagerstätte, lower Cambrian, southwest China. Lethaia 46, 262–273. doi: 10.1111/let.12002
Destombes J., Hollard H., Willefert S. (1985). ‘Lower Palaeozoic rocks of Morocco,’ in Lower Palaeozoic rocks of the world 4. Ed. Holland C. H. (New York: Wiley), 91–336.
Drage H. B., Legg D. A., Daley A. C. (2023). Novel marrellomorph moulting behaviour preserved in the Lower Ordovician Fezouata Shale, Morocco. Front. Ecol. Evol. 11. doi: 10.3389/fevo.2023.1226924
Du K., Ortega-Hernández J., Yang J., Zhang X. (2019). A soft-bodied euarthropod from the early Cambrian Xiaoshiba Lagerstätte of China supports a new clade of basal artiopodans with dorsal ecdysial sutures. Cladistics 35, 269–281. doi: 10.1111/cla.12344
Ebenman B. (1987). Niche differences between age classes and intraspecific competition in age-structured populations. J. Theor. Biol. 124, 25–33. doi: 10.1016/S0022-5193(87)80249-7
Ebenman B. (1992). Evolution in organisms that change their niches during the life cycle. Am. Nat. 139, 990–1021. doi: 10.1086/285370
Fu D., Ortega-Hernández J., Daley A. C., Zhang X., Shu D. (2018). Anamorphic development and extended parental care in a 520 million-year-old stem-group euarthropod from China. BMC Evol. Biol. 18, 147. doi: 10.1186/s12862-018-1262-6
Fu D., Tong G., Dai T., Liu W., Yang Y., Zhang Y., et al. (2019). The Qingjiang biota—A Burgess Shale–type fossil Lagerstätte from the early Cambrian of South China. Sci. (80-. ) 363, 1338–1342. doi: 10.1126/science.aau8800
Fu D., Zhang X., Budd G. E., Liu W., Pan X. (2014). Ontogeny and dimorphism of Isoxys auritus (Arthropoda) from the Early Cambrian Chengjiang biota, South China. Gondwana. Res. 25, 975–982. doi: 10.1016/j.gr.2013.06.007
García-Bellido D. C., Collins D. H. (2006). A new study of Marrella splendens (Arthropoda, Marrellomorpha) from the Middle Cambrian Burgess Shale, British Columbia, Canada. Can. J. Earth Sci. 43, 721–742. doi: 10.1139/e06-012
Hammer Ø., Harper D. A. T., Ryan P. D. (2001). Past: paleontological statistics software package for education and data analysis. Palaeontol. Electron. 4, 1–9.
Haug J. T. (2020). Why the term ‘larva’ is ambiguous, or what makes a larva? Acta Zool. 101, 167–188. doi: 10.1111/azo.12283
Haug J. T., Castellani C., Haug C., Waloszek D., Maas A. (2013). A Marrella-like arthropod from the Cambrian of Australia: A new link between ‘Orsten’-type and Burgess Shale assemblages. Acta Palaeontol. Pol. 58, 629–639. doi: 10.4202/app.2011.0120
Haug J. T., Waloszek D., Maas A., Liu Y., Haug C. (2012). Functional morphology, ontogeny and evolution of mantis shrimp-like predators in the Cambrian. Palaeontology 55, 369–399. doi: 10.1111/j.1475-4983.2011.01124.x
Hopkins M. J. (2017). Development, trait evolution, and the evolution of development in trilobites. Integr. Comp. Biol. 57, 488–498. doi: 10.1093/icb/icx033
Hou J. B., Hughes N. C., Yang J., Lan T., Zhang X. G., Dominguez C. (2017). Ontogeny of the articulated yiliangellinine trilobite Zhangshania typica from the lower Cambrian (Series 2, Stage 3) of southern China. J. Paleontol. 91, 86–99. doi: 10.1017/jpa.2016.118
Hughes N. C., Minelli A., Fusco G. (2006). The ontogeny of trilobite segmentation: a comparative approach. Paleobiology 32, 602–627. doi: 10.1666/06017.1
Koenemann S., Schram F. R., Bloechl A., Iliffe T. M., Hoenemann M., Held C. (2007). Post-embryonic development of remipede crustaceans. Evol. Dev. 9, 117–121. doi: 10.1111/j.1525-142X.2007.00142.x
Kühl G., Rust J. (2010). Re-investigation of Mimetaster hexagonalis: a marrellomorph arthropod from the Lower Devonian Hunsrück Slate (Germany). Paläontologische. Z. 84, 397–411. doi: 10.1007/s12542-009-0049-x
Laibl L., Cederström P., Ahlberg P. (2018). Early post-embryonic development in Ellipsostrenua (Trilobita, Cambrian, Sweden) and the developmental patterns in Ellipsocephaloidea. J. Paleontol. 92, 1018–1027. doi: 10.1017/jpa.2018.25
Laibl L., Drage H. B., Pérez-Peris F., Schöder S., Saleh F., Daley A. C. (in press). Babies from the Fezouata Biota: Early developmental trilobite stages and their adaptation to high latitudes. Geobios. doi: 10.1016/j.geobios.2023.06.005
Laibl L., Esteve J., Fatka O. (2017). Giant postembryonic stages of Hydrocephalus and Eccaparadoxides and the origin of lecithotrophy in Cambrian trilobites. Palaeogeogr. Palaeoclimatol. Palaeoecol. 470, 109–115. doi: 10.1016/j.palaeo.2017.01.023
Laibl L., Maletz J., Olschewski P. (2021). Post-embryonic development of Fritzolenellus suggests the ancestral morphology of the early developmental stages in Trilobita. Pap. Palaeontol. 7, 839–859. doi: 10.1002/spp2.1324
Laibl L., Saleh F., Pérez-Peris F. (2023). Drifting with trilobites: The invasion of early post-embryonic trilobite stages to the pelagic realm. Palaeogeogr. Palaeoclimatol. Palaeoecol. 613, 111403. doi: 10.1016/j.palaeo.2023.111403
Lefebvre B., El Hariri K., Lerosey-Aubril R., Servais T., Van Roy P. (2016). The Fezouata Shale (Lower Ordovician, Anti-Atlas, Morocco): A historical review. Palaeogeogr. Palaeoclimatol. Palaeoecol. 460, 7–23. doi: 10.1016/j.palaeo.2015.10.048
Lefebvre B., Gutiérrez-Marco J. C., Lehnert O., Martin E. L. O., Nowak H., Akodad M., et al. (2018). Age calibration of the lower Ordovician Fezouata Lagerstätte, Morocco. Lethaia 51, 296–311. doi: 10.1111/LET.12240
Legg D. A. (2015). The morphology and affinities of Skania fragilis (Arthropoda) from the middle Cambrian Burgess Shale. Bull. Geosci. 90, 509–518. doi: 10.3140/bull.geosci.1532
Legg D. A. (2016). A new marrellid arthropod from the Ordovician of Wales. Acta Palaeontol. Pol. 61, 617–619. doi: 10.4202/app.00246.2016
Legg D. A., Sutton M. D., Edgecombe G. D. (2013). Arthropod fossil data increase congruence of morphological and molecular phylogenies. Nat. Commun. 4, 2485. doi: 10.1038/ncomms3485
Lerosey-Aubril R., Feist R. (2005). Ontogeny of a new cyrtosymboline trilobite from the Famennian of Morocco. Acta Palaeontol. Pol. 50, 449–464.
Lerosey-Aubril R., Gaines R. R., Hegna T. A., Ortega-Hernández J., Van Roy P., Kier C., et al. (2018). The Weeks Formation Konservat-Lagerstätte and the evolutionary transition of Cambrian marine life. J. Geol. Soc London. 175, 705–715. doi: 10.1144/jgs2018-042
Lerosey-Aubril R., Laibl L. (2021). Protaspid larvae are unique to trilobites. Arthropod. Struct. Dev. 63, 101059. doi: 10.1016/j.asd.2021.101059
Liu Q. (2013). The first discovery of Marrella (Arthropoda, Marrellomorpha) from the Balang Formation (Cambrian Series 2) in Hunan, China. J. Paleontol. 87, 391–394. doi: 10.1666/12-118.1
Liu Y., Haug J. T., Haug C., Briggs D. E. G., Hou X. (2014). A 520 million-year-old chelicerate larva. Nat. Commun. 5, 4440. doi: 10.1038/ncomms5440
Liu J., Lerosey-Aubril R., Steiner M., Dunlop J. A., Shu D., Paterson J. R. (2018). Origin of raptorial feeding in juvenile euarthropods revealed by a Cambrian radiodontan. Natl. Sci. Rev. 5, 863–869. doi: 10.1093/nsr/nwy057
Liu Y., Melzer R. R., Haug J. T., Haug C., Briggs D. E. G., Hörnig M. K., et al. (2016). Three-dimensionally preserved minute larva of a great-appendage arthropod from the early Cambrian Chengjiang biota. PNAS 113, 5542–5546. doi: 10.1073/pnas.1522899113
Lovrich G. A., Vinuesa J. H. (1999). Reproductive potential of the lithodids Lithodes santolla and Paralomis granulosa (Anomura, Decapoda) in the Beagle Channel, Argentina. Sci. Mar. 63, 355–360. doi: 10.3989/scimar.1999.63s1355
Maas A., Braun A., Dong X.-P., Donoghue P. C. J., Müller K. J., Olempska E., et al. (2006). The ‘Orsten’—More than a Cambrian Konservat-Lagerstätte yielding exceptional preservation. Palaeoworld 15, 266–282. doi: 10.1016/j.palwor.2006.10.005
Marone F., Münch B., Stampanoni M. (2010). Fast reconstruction algorithm dealing with tomography artifacts. Proc. Vol. 7804. Dev. X-Ray. Tomogr. VII. 7804, 292–302. doi: 10.1117/12.859703
Martin E. L. O., Pittet B., Gutiérrez-Marco J. C., Vannier J., El Hariri K., Lerosey-Aubril R., et al. (2016a). The Lower Ordovician Fezouata Konservat-Lagerstätte from Morocco: Age, environment and evolutionary perspectives. Gondwana. Res. 34, 274–283. doi: 10.1016/J.GR.2015.03.009
Martin E. L. O., Vidal M., Vizcaïno D., Vaucher R., Sansjofre P., Lefebvre B., et al. (2016b). Biostratigraphic and palaeoenvironmental controls on the trilobite associations from the Lower Ordovician Fezouata Shale of the central Anti-Atlas, Morocco. Palaeogeogr. Palaeoclimatol. Palaeoecol. 460, 142–154. doi: 10.1016/J.PALAEO.2016.06.003
Mauchline J. (1971). Euphausiacea larvae (Copenhagen: Conseil International pour l’Exploration de la Mer).
Minelli A., Fusco G. (2013). ‘Arthropod post-embryonic development,’ in Arthropod biology and evolution. Eds. Minelli A., Boxshall G., Fusco G. (Berlin, Heidelberg: Springer-Verlag), 91–122. doi: 10.1007/978-3-642-36160-9_5
Moore R. A., Briggs D. E. G., Bartels C. (2005). A new specimen of Weinbergina opitzi (Chelicerata: Xiphosura) from the Lower Devonian Hunsrück Slate, Germany. Paläontologische. Z. 79, 399–408. doi: 10.1007/BF02991931
Moran N. A. (1994). Adaptation and constraint in the complex life cycles of animals. Annu. Rev. Ecol. Syst. 25, 573–600. doi: 10.1146/annurev.es.25.110194.003041
Moysiuk J., Caron J. B. (2023). A quantitative assessment of ontogeny and molting in a Cambrian radiodont and the evolution of arthropod development. Paleobiology, 1–16. doi: 10.1017/pab.2023.18
Moysiuk J., Izquierdo-López A., Kampouris G. E., Caron J. B. (2022). A new marrellomorph arthropod from southern Ontario: a rare case of soft-tissue preservation on a Late Ordovician open marine shelf. J. Paleontol. 96, 859–874. doi: 10.1017/jpa.2022.11
Nowak H., Servais T., Pittet B., Vaucher R., Akodad M., Gaines R. R., et al. (2016). Palynomorphs of the Fezouata Shale (Lower Ordovician, Morocco): Age and environmental constraints of the Fezouata Biota. Palaeogeogr. Palaeoclimatol. Palaeoecol. 460, 62–74. doi: 10.1016/j.palaeo.2016.03.007
Ortega-Hernández J., Van Roy P., Lerosey-Aubril R. (2016). A new aglaspidid euarthropod with a six-segmented trunk from the Lower Ordovician Fezouata Konservat-Lagerstätte, Morocco. Geol. Mag. 153, 524–536. doi: 10.1017/S0016756815000710
Park T.-Y. S., Kihm J.-H., Woo J., Kim Y. H. G., Lee J. I. (2016). Ontogeny of the Furongian (late Cambrian) trilobite Proceratopyge cf. P. lata Whitehouse from northern Victoria Land, Antarctica, and the evolution of metamorphosis in trilobites. Palaeontology 59, 657–670. doi: 10.1111/pala.12251
Paterson J. R., García-Bellido D. C., Jago J. B., Gehling J. G., Lee M. S. Y., Edgecombe G. D. (2016). The Emu Bay Shale Konservat-Lagerstätte: a view of Cambrian life from East Gondwana. J. Geol. Soc London. 173, 1–11. doi: 10.1144/jgs2015-083
Pérez-Peris F., Laibl L., Lustri L., Gueriau P., Antcliffe J. B., Bath Enright O. G., et al. (2021a). A new nektaspid euarthropod from the Lower Ordovician strata of Morocco. Geol. Mag. 158, 509–517. doi: 10.1017/S001675682000062X
Pérez-Peris F., Laibl L., Vidal M., Daley A. C. (2021b). Systematics, morphology, and appendages of an Early Ordovician pilekiine trilobite Anacheirurus from Fezouata Shale and the early diversification of Cheiruridae. Acta Palaeontol. Pol. 66, 857–877. doi: 10.4202/app.00902.2021
Potin G. J.-M., Daley A. C. (2023). The significance of Anomalocaris and other Radiodonta for understanding paleoecology and evolution during the Cambrian explosion. Front. Earth Sci. 11. doi: 10.3389/feart.2023.1160285
Potin G. J.-M., Gueriau P., Daley A. C. (2023). Radiodont frontal appendages from the Fezouata Biota (Morocco) reveal high diversity and ecological adaptations to suspension-feeding during the Early Ordovician. Front. Ecol. Evol. 11. doi: 10.3389/fevo.2023.1214109
Rabalais N. N., Gore R. H. (1985). ‘Abbreviated development in decapods,’ in Crustacean issues 2: larval growth (Rotterdam: A.A. Balkema), 67–126.
Rak Š., Ortega-Hernández J., Legg D. A. (2013). A revision of the Late Ordovician marrellomorph arthropod Furca bohemica from Czech Republic. Acta Palaeontol. Pol. 58, 615–628. doi: 10.4202/app.2011.0038
Rohlf F. J. (2006). TpsDig2, digitize landmarks and outlines, version 2.10. Available at: http://life.bio.sunysb.edu/morph/.
Saleh F., Antcliffe J. B., Lefebvre B., Pittet B., Laibl L., Perez Peris F., et al. (2020a). Taphonomic bias in exceptionally preserved biotas. Earth Planet. Sci. Lett. 529, 115873. doi: 10.1016/j.epsl.2019.115873
Saleh F., Bath-Enright O. G., Daley A. C., Lefebvre B., Pittet B., Vite A., et al. (2021a). A novel tool to untangle the ecology and fossil preservation knot in exceptionally preserved biotas. Earth Planet. Sci. Lett. 569, 117061. doi: 10.1016/J.EPSL.2021.117061
Saleh F., Candela Y., Harper D. A. T., Polechová M., Lefebvre B., Pittet B. (2018). Storm-induced community dynamics in the Fezouata Biota (Lower Ordovician, Morocco). Palaios 33, 535–541. doi: 10.2110/PALO.2018.055
Saleh F., Daley A. C., Lefebvre B., Pittet B., Perrillat J. P. (2020b). Biogenic iron preserves structures during fossilization: A hypothesis. BioEssays 42, 1900243. doi: 10.1002/BIES.201900243
Saleh F., Lefebvre B., Hunter A. W., Nohejlová M. (2020c). Fossil weathering and preparation mimic soft tissues in eocrinoid and somasteroid echinoderms from the Lower Ordovician of Morocco. Micros. Today 28, 24–28. doi: 10.1017/S1551929519001238
Saleh F., Pittet B., Perrillat J. P., Lefebvre B. (2019). Orbital control on exceptional fossil preservation. Geology 47, 103–106. doi: 10.1130/G45598.1
Saleh F., Pittet B., Sansjofre P., Gueriau P., Lalonde S., Perrillat J. P., et al. (2020d). Taphonomic pathway of exceptionally preserved fossils in the Lower Ordovician of Morocco. Geobios 60, 99–115. doi: 10.1016/J.GEOBIOS.2020.04.001
Saleh F., Vaucher R., Antcliffe J. B., Daley A. C., El Hariri K., Kouraiss K., et al. (2021b). Insights into soft-part preservation from the Early Ordovician Fezouata Biota. Earth-Sci. Rev. 213, 103464. doi: 10.1016/j.earscirev.2020.103464
Saleh F., Vaucher R., Vidal M., El Hariri K., Laibl L., Daley A. C., et al. (2022). New fossil assemblages from the Early Ordovician Fezouata Biota. Sci. Rep. 12, 20773. doi: 10.1038/s41598-022-25000-z
Saleh F., Vidal M., Laibl L., Sansjofre P., Gueriau P., Pérez-Peris F., et al. (2021c). Large trilobites in a stress-free Early Ordovician environment. Geol. Mag. 158, 261–270. doi: 10.1017/s0016756820000448
Sanders H. L., Hessler R. R. (1964). The larval development of Lightiella incisa Gooding (Cephalocarida). Crustaceana 7, 81–97. doi: 10.1163/156854064X00326
Shuster C. N., Sekiguchi K. (2003). ‘Growing up takes about ten years and eighteen stages,’ in The American horseshoe crab. Eds. Shuster C. N., Barlow R. B., Brockmann H. J. (Cambridge: Harvard University Press), 103–132.
Siveter D. J., Fortey R. A., Sutton M. D., Briggs D. E. G., Siveter D. J. (2007). A silurian ‘marrellomorph’ arthropod. Proc. R. Soc B. Biol. Sci. 274, 2223–2229. doi: 10.1098/rspb.2007.0712
Sombke A., Lipke E., Kenning M., Müller C. H. G., Hansson B. S., Harzsch S. (2012). Comparative analysis of deutocerebral neuropils in Chilopoda (Myriapoda): implications for the evolution of the arthropod olfactory system and support for the Mandibulata concept. BMC Neurosci. 13, 1. doi: 10.1186/1471-2202-13-1
Stürmer W., Bergström J. (1976). The arthropods Mimetaster and Vachonisia from the Devonian HunsrüCk Shale. Paläontologische. Z. 50, 78–111. doi: 10.1007/BF03001974
Vannier J., Aria C., Taylor R. S., Caron J.-B. (2018). Waptia fieldensis Walcott, a mandibulate arthropod from the middle Cambrian Burgess Shale. R. Soc Open Sci. 5, 172206. doi: 10.1098/rsos.172206
Van Roy P. (2006). Non-trilobite arthropods from the ordovician of Morocco. (PhD dissertation. Ghent: Ghent University).
Van Roy P., Briggs D. E. G., Gaines R. R. (2015). The Fezouata fossils of Morocco; an extraordinary record of marine life in the Early Ordovician. J. Geol. Soc London. 172, 541–549. doi: 10.1144/jgs2015-017
Van Roy P., Orr P. J., Botting J. P., Muir L. A., Vinther J., Lefebvre B., et al. (2010). Ordovician faunas of Burgess Shale type. Nature 465, 215–218. doi: 10.1038/nature09038
Vaucher R., Martin E. L. O., Hormière H., Pittet B. (2016). A genetic link between Konzentrat- and Konservat-Lagerstätten in the Fezouata Shale (Lower Ordovician, Morocco). Palaeogeogr. Palaeoclimatol. Palaeoecol. 460, 24–34. doi: 10.1016/J.PALAEO.2016.05.020
Vaucher R., Pittet B., Hormière H., Martin E. L. O., Lefebvre B. (2017). A wave-dominated, tide-modulated model for the Lower Ordovician of the Anti-Atlas, Morocco. Sedimentology 64, 777–807. doi: 10.1111/SED.12327
Werner E. E., Gilliam J. F. (1984). The ontogenetic niche and species interactions in size-structured populations. Annu. Rev. Ecol. Syst. 15, 393–425. doi: 10.1146/annurev.es.15.110184.002141
Whittington H. B. (1971). Redescription of Marrella splendens (Trilobitoidea) from the Burgess Shale, Middle Cambrian, British Columbia. Bull. Geol. Surv. Canada. 209, 1–24.
Whittington H. B., Almond J. E. (1987). Appendages and habits of the Upper Ordovician trilobite Triarthrus eatoni. Philos. Trans. R. Soc London. B. Biol. Sci. 317, 1–46. doi: 10.1098/RSTB.1987.0046
Yang X., Kimmig J., Zhai D., Liu Y., Kimmig S. R., Peng S. (2021). A juvenile-rich palaeocommunity of the lower Cambrian Chengjiang biota sheds light on palaeo-boom or palaeo-bust environments. Nat. Ecol. Evol. 5, 1082–1090. doi: 10.1038/s41559-021-01490-4
Zhai D., Edgecombe G. D., Bond A. D., Mai H., Hou X., Liu Y. (2019). Fine-scale appendage structure of the Cambrian trilobitomorph Naraoia spinosa and its ontogenetic and ecological implications. Proc. R. Soc B. Biol. Sci. 286, 20192371. doi: 10.1098/rspb.2019.2371
Zhang Z.-Q. (2011). Phylum Arthropoda von Siebold 1848. Zootaxa 3148, 99–103. doi: 10.11646/zootaxa.3148.1.14
Zhao F., Caron J. B., Bottjer D. J., Hu S., Yin Z., Zhu M. (2014). Diversity and species abundance patterns of the Early Cambrian (Series 2, Stage 3) Chengjiang Biota from China. Paleobiology 40, 50–69. doi: 10.1666/12056
Keywords: Euarthropoda, development, niche differentiation, Ordovician, Morocco, marrellida
Citation: Laibl L, Gueriau P, Saleh F, Pérez-Peris F, Lustri L, Drage HB, Bath Enright OG, Potin GJ-M and Daley AC (2023) Early developmental stages of a Lower Ordovician marrellid from Morocco suggest simple ontogenetic niche differentiation in early euarthropods. Front. Ecol. Evol. 11:1232612. doi: 10.3389/fevo.2023.1232612
Received: 31 May 2023; Accepted: 25 August 2023;
Published: 25 September 2023.
Edited by:
Tae-Yoon Park, Korea Polar Research Institute, Republic of KoreaReviewed by:
Dongjing Fu, Northwest University, ChinaJames Holmes, University of New England, Australia
Copyright © 2023 Laibl, Gueriau, Saleh, Pérez-Peris, Lustri, Drage, Bath Enright, Potin and Daley. This is an open-access article distributed under the terms of the Creative Commons Attribution License (CC BY). The use, distribution or reproduction in other forums is permitted, provided the original author(s) and the copyright owner(s) are credited and that the original publication in this journal is cited, in accordance with accepted academic practice. No use, distribution or reproduction is permitted which does not comply with these terms.
*Correspondence: Lukáš Laibl, lukaslaibl@gmail.com; Allison C. Daley, allison.daley@unil.ch