- Department of Evolutionary Neuroethology, Max-Planck-Institute for Chemical Ecology, Jena, Germany
The search for resources occupies a major part of the time and energy budget of many insects. In this context, many insects display constancy behavior, in which they learn to return to the same, predictably rewarding resource repeatedly. Flower constancy is one such behavior, where nectar-feeding insects restrict foraging visits to a few plant species, sometimes overlooking potentially better-rewarding plants. This phenomenon is well documented in multiple species and is believed to be an optimal strategy to maximize benefits, while minimizing energy and time expenditure. Oviposition constancy is a similar behavior, where insects, dependent on previous experience, restrict their visits to a few types of potential oviposition sites. In contrast to flower constancy, the prevalence of oviposition constancy and the rationale behind this behavior are relatively unknown. An improved understanding of oviposition constancy can act as a lens into the evolutionary history of local insect adaptations, it can help gauge the impact of climate change on insect plant interactions, and it can aid the design of crop-pest management strategies. In this review, we discuss the potential benefits of oviposition constancy in insects and the extent of plasticity occurring in host-plant choice for oviposition.
Introduction
Nectar feeding is a common feature among insects and typically constitutes a symbiotic relationship with the flower, which gets pollinated. The tobacco sphinx moth Manduca sexta e.g. displays an innate preference for Datura wrightii flowers (Riffell et al., 2008), while the armyworm Helicoverpa armigera prefers flowers of several horticultural crops (Jallow et al., 2004). Despite these innate preferences, the insects, especially in the absence of their preferred flowers, feed on less preferred plants. Having fed on flowers of a given plant species, many insect species, however, display flower constancy, defined as the tendency to restrict foraging visits to these flowers, often bypassing better rewarding ones. This has been suggested to be a result from cognitive limitations (Chittka et al., 1999). The feeding insects thus learn the features of one, reliable food source and benefit from such a system by becoming more efficient and fast in search and handling behavior. Flower constancy has been observed in e.g. bees (Grant, 1950; Waser, 1986; Goulson, 2000; Grüter and Ratnieks, 2011), butterflies (Goulson et al., 1997b) and hoverflies (Goulson and Wright, 1998).
This review deals with a similar, however less well investigated phenomenon, the experienced-based change of insect oviposition preference, which can result in oviposition constancy. Before we talk about such oviposition learning, let us first summarize what is known about innate oviposition preferences in insects.
Most insect females exhibit innate oviposition preferences for host-plants with specialists having a strong innate preference for only few hosts. For instance, the monarch butterfly Danaus plexippus exemplifies a robust preference for milkweed Asclepias (Ladner and Altizer, 2005). The plants defend themselves with glycosides against herbivores (Larrea et al., 2022). However, the monarch has adapted to withstand this defence and by that not only circumvents competition with other herbivores but even accumulates these glycosides for its own protection against predators. Similarly, the diamond back moth Plutella xylostella is able to detoxify poisonous glucosinolates from Brassica plants and prefers to lay its eggs on those otherwise well protected plants (Renwick et al., 2006; Liu et al., 2020; Ghosh et al., 2022). On the other hand, generalists have a wider innate preference such as Spodoptera littoralis for cotton, cow pea, Egyptian clover, cabbage and maize (Thöming et al., 2013) or the Aster leafhoppers ovipositing on vegetable crops, grasses, clovers (Hagel et al., 1973), and cereals (Romero et al., 2020). An extreme generalist is Helicoverpa armigera, which oviposits on many crop species but has also been reported on 67 wild species from about 30 plant families (Zalucki et al., 1986; Fitt, 1989; Jallow et al., 2004). While specialists are thought to be better adapted to the defense mechanisms of their hosts, generalists probably profit from not being so dependent on the presence of one specific plant. Even if their most preferred plant is absent, they still are flexible enough to switch to other hosts. However, apart from these strict specialists and super wide generalists, most species range somewhere in-between. Helicoverpa assulta e.g., is regarded as oligophagous, as it oviposits on a narrow range of solanaceous plants (Fitt, 1989; Mitter et al., 1993; Kunjun et al., 2006; Lee et al., 2006). Similarly, Manduca sexta is mainly specialized on solanaceous plants but also oviposits on plants of the genus Proboscidea. Despite accepting several host plants, the insects, however, often prefer one plant over the other. H. assulta prefer tobacco over sunflower over hot pepper (Liu et al., 2010), Manduca has been reported to prefer potato, tobacco, and Datura plants over other potential hosts (Garvey et al., 2020). Interestingly, whether or not a plant is preferred is not necessarily directly correlated with its nutritional value for the offspring, but also with potential protection against competition and predation (Zhang et al., 2019; Garvey et al., 2020; Zhang et al., 2022). Although e.g. Manduca sexta larvae grow slower on tobacco plants, which protect themselves with nicotine, the accumulation of this poison within the larvae seems to protect them against parasitoid wasps (Garvey et al., 2020). Female insects thus always need to make a trade-off between the best nutritional value and a potential enemy-free space.
Comparing constancy in insects: flower/foraging behavior vs. oviposition
Foraging constancy
In the context of foraging behavior, insects often employ a constancy strategy, akin to the fidelity observed in flower visits by bees like Apis mellifera (Grüter and Ratnieks, 2011), and skipper butterflies such as Thymelicus flavus (Goulson et al., 1997a; Goulson et al., 1997b). This strategy involves learning the specific characteristics of flowers that have been successfully foraged from and subsequently targeting those same flowers for future visits (Figure 1). By adhering to this behavior despite potential distractions, insects can efficiently gather nectar and pollen from reliable host plants. However, it is worth noting that this constancy strategy can limit their exploration of potentially more rewarding plants, even if they are more easily accessible (Waser, 1986).
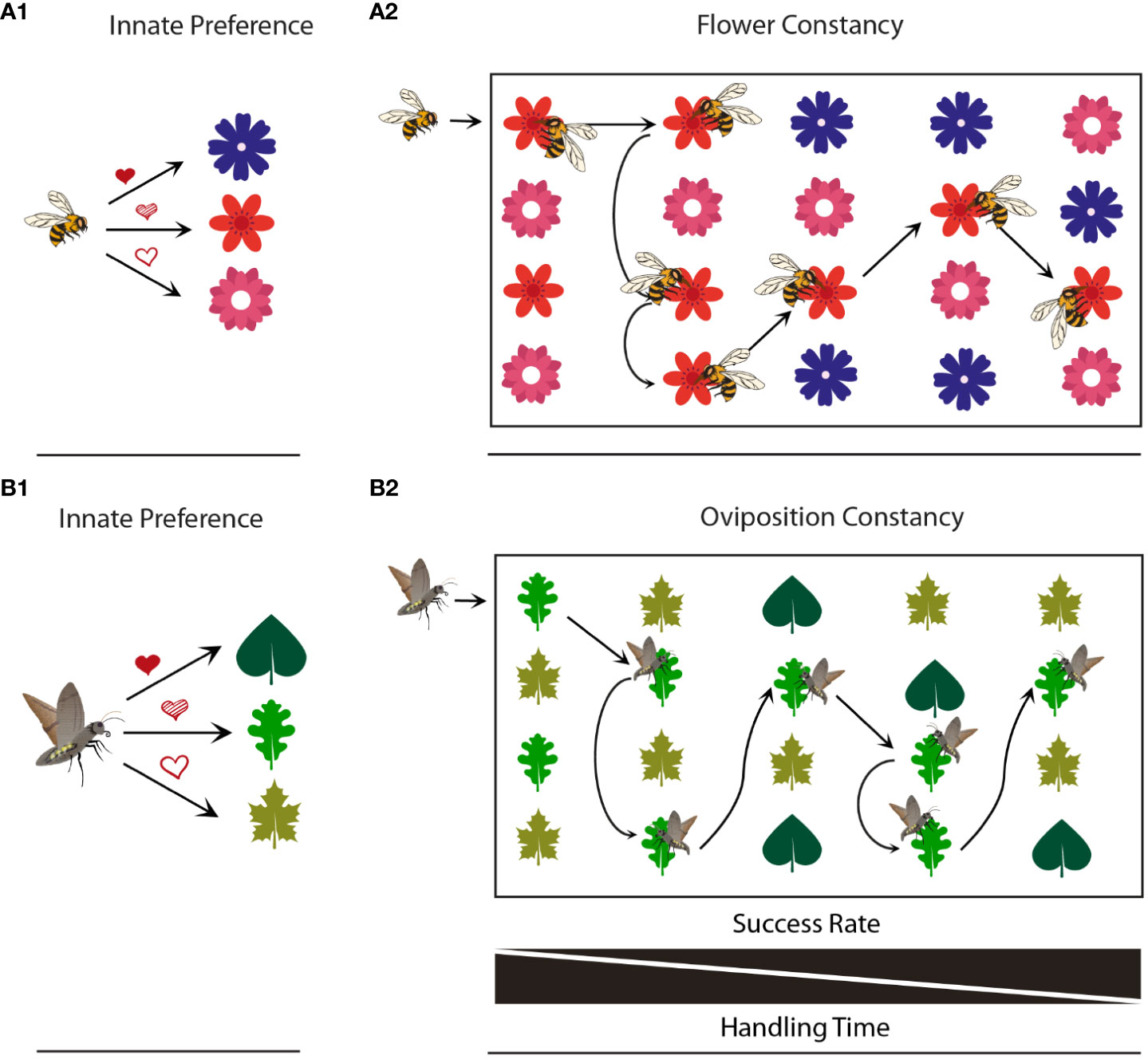
Figure 1 Comparison of flower and oviposition constancy. (A1) Flower preferences of a bee (fully shaded heart, highest preference; partially shaded heart, intermediate preference; unshaded heart, lowest preference). (A2) Flower constancy: In the absence of preferred flowers, a bee might forage on less preferred ones and later might stick to this choice. (B1) Host plant preferences of an ovipositing moths (for meaning of hearts see above). (B2) Oviposition constancy: In the absence of preferred hosts, a moth might oviposit on less preferred ones and later might stick to this choice.
The concept of flower constancy, first noted by Aristotle in honeybee workers (Apis mellifera), has since been observed in various insect pollinators. Flower constancy benefits plants by preventing pollen loss to other species and avoiding the blockage of stigmas with pollen from different species. However, it is essential to consider the benefits received by the pollinators themselves. In some cases, flower constancy may come at a clear cost. For instance, a honeybee that exhibits flower constancy while foraging in a field with multiple plant species in bloom might miss out on more energetically rewarding opportunities if it focuses solely on one plant species. In certain experimental scenarios, honeybee foragers show constancy towards a floral type that offers lower rewards compared to an alternative that is simultaneously available. This constancy is independent of the energetic value provided by flowers of other colors (Wells and Wells, 1986). The learning investment hypothesis suggests that flower constancy is the optimal strategy because switching from one flower species to another would require a period of reduced efficiency as the insects need to learn how to extract pollen or nectar from the new species (Waser, 1986; Chittka et al., 1997). As bees live in eusocial societies, one needs also to consider that an individual honey bee might make “mistakes” about flower choice and constancy on its own, but that its behavior might be adaptive when looking from the colony perspective. The hawkmoth Manduca sexta feeds (among others) from Nicotiana flowers, but naïve moths hardly profit from the first flower visit, as hovering in front of the flower consumes almost as much energy as the moths can gain from the nectar reward and the moths in addition often fail to insert their proboscis (Haverkamp et al., 2016). However, a recent study on hawkmoths’ learning abilities concerning specific flower types revealed that moths achieved higher success rates with experience (Adam et al., 2021). Obviously hawkmoths, similar to butterflies (Kandori and Ohsaki, 1996) and bumblebees (Laverty, 1994; Chittka et al., 1997), improve their foraging efficiency when repeatedly foraging on a single flower type.
Additionally, apart from the increase in efficiency in flower visitation, there is a prevailing hypothesis suggesting that flower constancy is not rigidly maintained when rewards are low (Greggers and Menzel, 1993). In situations where recently encountered rewards from a particular flower species are unsatisfactory, insects have two choices: either leave the patch and seek out another of the same species or remain within the patch but switch to an alternative species. Previous studies have indicated that bees often opt to fly to a different patch when they have recently experienced inadequate rewards and no alternative plant species is available. However, other studies have demonstrated that bees (Grüter et al., 2011), bumblebees (Chittka et al., 1997) and butterflies (Goulson et al., 1997a) exhibit a higher likelihood of switching to a different species when they receive rewards lower than the average.
A study using honey bees and artificial flower patches assessed the bees’ responses to mixed-color flower patches and variations in reward quantity, quality, and frequency associated with flower morphology. The results showed that each honey bee visiting a patch with randomly distributed blue and yellow flowers exhibited constancy to one color, even though this behavior did not result in the maximum reward. When e.g. reward quantity or quality differed between the two flower morphs, each bee remained constant to one color, despite the suboptimal nature of this behavior. Even when reward frequency was higher in one flower morph than the other, each bee maintained constancy to one color, despite the behavior’s failure to maximize reward or minimize uncertainty (Wells and Wells, 1983; Hill et al., 1997). A study by Menzel and coauthors (Menzel et al., 1993) interpreted flower constancy as an indication of the capacity to learn and utilize various floral features, including colors, odors, and patterns, as predictive cues for floral rewards. However, as individual animals have been shown to flexibly adjust their foraging constancy, other authors regard flower constancy rather as a successful foraging strategy (Grüter and Ratnieks, 2011). Anyhow, there seems to be a strong selection for exhibiting flower constancy that is influenced by a complex interaction of innate preference, the present set of floral choices, and the provided rewards (Latty and Trueblood, 2020) that is still not fully understood.
Oviposition constancy
Constancy behavior in the context of foraging has been extensively studied in insects, while only a few studies deal with the corresponding behavior in the context of oviposition, i.e. oviposition constancy, where an insect turns its oviposition preference towards a host it has already experienced (Figure 1). One of the classic examples for oviposition-related learning comes from the studies performed by Smid & coworkers, on the two closely related parasitoids, Cotesia glomerata and C. rubecula (Smid et al., 2007). These parasitoid wasps oviposit on the larvae of Pieris butterflies. The wasp uses the odor from the host plants as navigational cues to locate the larvae of the butterfly.
A high density of caterpillars obviously aids the wasp’s navigation towards the host plant as plants with a high density of caterpillars emit a stronger plant odor. As a result, naïve parasitoid wasps responded differently to odor from plants infested by different numbers of caterpillars (Geervliet et al., 1998). However, Geervliet and colleagues could show that after gaining experience, C. glomerata wasps became more responsive even to the weak odor emitted by host plants with a low density of caterpillars. Evidently, learning during oviposition increased the wasps’ subsequent responsiveness to plant odors.
In addition, Cotesia wasps are also able to associate the odor of a specific plant species, on which they found a caterpillar, with successful oviposition. Although naïve wasps search for their host caterpillars on different plants, after finding them, on e.g. Brussels sprouts, their preference for the odor of this plant increases (Geervliet et al., 1996). Much to the advantage of the wasp, Cotesia glomerata, its main butterfly host, the large white Pieris brassicae, simplifies the wasp’s task by depositing eggs in clusters, which increases the probability for the wasp to find multiple caterpillars nearby on the same plant species. In contrast, the closely related wasp Cotesia rubecula parasitizes the small white, Pieris rapae, which lays individual eggs on widely spaced Brassiceae host plants. Cotesia glomerata after localizing a caterpillar, can hence expect more caterpillars on the same plant near-by, while for C. rubecula the chance to find additional caterpillars on a given plant is lower. This might also be the reason for the different learning capabilities of the two wasp species. C. glomerata wasps learn the plant odor after a single oviposition event, while the C. rubecula wasps need several oviposition events to form the association with the plant odor (Bleeker et al., 2006). While both wasp species can finally learn the association, it also takes fewer learning trials for C. glomerata than for C. rubecula to form a long-term memory of this association (Smid et al., 2007). Hence, we can conclude that parasitoid wasps learn oviposition cues, but to which extent they do so is dictated by their life history. The chance to locate numerous caterpillars on a given plant perhaps governed the evolution of a very fast learning process including a fast memory consolidation in C. glomerata, while its close relative C. rubecula evolved a different strategy.
Cotesia wasps are, however, not the only parasitoid wasps whose learning capabilities have been studied. Another well-investigated species is Leptopilina boulardi, whose females oviposit in Drosophila larvae. The wasps locate these larvae by probing fruits with their ovipositors, and like Cotesia wasps they are able to learn the association of specific odors with the oviposition event. (Kaiser et al., 2003) tested the influence of the number of conditioning trials on short- and long-term memory. To do so the authors exposed naïve female wasps to banana odor while they had inserted their ovipositor into a Drosophila larva. This experience could result in wasps probing a substrate with their ovipositor whenever they smell banana again. In a set of experiments, the authors exposed the wasps to this oviposition-training procedure either once, five-times or ten-times. The differentially trained wasps were afterwards tested regarding their short-, mid-, or long-term memory by testing the wasps’ probing response to banana odor either 12 minutes, 2 hours, 24 hours, or even 48 hours after the last training trial. While short-term memory (i.e. 12 minutes after the training) existed already after a single training trial (though became better after 5 trials), it needed at least 5 trials to generate long-term memory up to 24 hours after training. However, even after repeated training, any knowledge about the training odor was gone when the wasps were tested 48 hours after the last training.
In conclusion, parasitoid wasps – be it Cotesia wasps ovipositing in butterfly larvae or Leptopilina wasps ovipositing in Drosophila larvae – learn olfactory cues they are exposed to during oviposition and follow those cues during subsequent host search. By repeatedly targeting plants or fruits, where they have already found caterpillars or fly larvae, the wasps exhibit a behavior that resembles flower constancy and can, hence, be called oviposition constancy. Although parasitoid wasps are a classical example for oviposition learning, they might not be the most straightforward one, as the wasps do not necessarily have to learn host cues, but rather the cues of plants, where the host can be found on.
Nonetheless, the phenomenon of experience-dependent oviposition constancy extends beyond parasitoid wasps and has also been observed in lepidopterans and their host plants. A comprehensive investigation conducted by (Stanton, 1984) delved into the intricacies of host-plant selection among three species of sulphur butterflies belonging to the Colias genus: C. periphyle, C. meadii, and C. alexandra. Colias butterflies are specialized in their preference for legume plants, with each species exhibiting a distinct range of innately preferred legume species. Stanton’s observations revealed that females of C. periphyle and C. meadii exhibited a more diverse oviposition behavior, laying eggs on as many as six different legume species. In contrast, females of C. alexandra displayed a high degree of specialization, exclusively depositing eggs on a single legume species. Additionally, C. alexandra displayed an exceptionally focused host-plant search, relying on only a few specific cues.
These butterflies organized their activities into distinct foraging bouts and oviposition bouts, i.e. alternatingly visited several flowers in a row for nectar feeding and afterwards several leaves for ovipositing, before they started foraging again. Stanton noted that during oviposition bouts, females occasionally landed on non-legume species that resembled suitable hosts. Here, they probed the plants using tarsal drumming and abdominal curling but refrained from laying eggs. Intriguingly, the frequency of such errors decreased within the oviposition bouts, indicating an improved ability to identify suitable host-plants based on very recent experiences. Evidently, the learning of host cues occurred rapidly and resulted in an immediate increase in host-localization accuracy.
Interestingly, this heightened accuracy diminished after subsequent foraging bouts, implying that the acquired information was only retained on a very short-term basis. Furthermore, Stanton’s findings revealed that the female C. alexandra, which is a specialist on a single host, made fewer errors throughout the oviposition bouts, suggesting that specialization on fewer hosts could enhance the efficiency in localizing host plants.
In various insects, we have observed that oviposition constancy often results from a mix of innate preferences and learnt preferences. Such behavior was also evident in the apple maggot fly, Rhagoletis pomonella, as shown by Prokopy and coauthors (Prokopy et al., 1982). The study revealed that these flies could modify their future host choices based on past egg-laying experiences with apple or hawthorn hosts. This learning capacity notably led to the flies’ complete avoidance of hawberries when they had previously laid eggs on apples. Obviously, these flies form associations between specific physical or chemical cues of host fruit and the act of laying eggs. This associative learning then significantly influences their future oviposition choices. This finding implies that the apple maggot flies are not just passively selecting sites but actively learning and adjusting their behavior based on previous experiences.
In a recent study (Nataraj et al., 2021) we found such oviposition learning also in the hawkmoth Manduca sexta. These moths mainly oviposit on Solanaceous plants but even within the Solanaceae they prefer some species over others. Naïve ovipositing M. sexta moths favor e.g. Datura plants over Nicotiana plants. However, when a moth, in the absence of Datura plants, oviposits on a Nicotiana plant, its innate preference for Datura vanishes. Subsequently, when presented with a choice, the moth will opt to lay its next eggs on Nicotiana again. Interestingly, this change in preference happens already after a single egg laid and, contrary to the before-mentioned example of Colias butterflies (Stanton, 1984), can persist at least until the next day.
Why does ovipositing on Nicotiana induce a change in preference in female hawkmoths? The moth is unable to directly observe, whether its offspring succeeds on this plant. Hence, directly experiencing the offspring’s success or failure cannot be involved in the moth’s learning process. However, could the act of laying an egg still provide the moth with any information regarding the likelihood of its offspring succeeding on this particular plant? During the oviposition event, moths engage in extensive probing of the plant, utilizing not only their antennae (Yamamoto et al., 1969) but also making contact with their tarsae, their proboscis (Chew and Robbins, 1984; Städler, 2002; Städler and Reifenrath, 2009), and their ovipositor (Seada et al., 2016). These organs are known to possess both chemical and mechanical sensors (Ozaki et al., 2011; Seada et al., 2018; Koutroumpa et al., 2021). Such probing behavior may provide the moth with valuable insights into the quality of the plant. If the plant is deemed suitable for egg-laying, it might be advantageous for the moth to learn the plant’s odor, thereby potentially increasing its efficiency in locating other plants of similar quality. Indeed, moths that have once oviposited on a given plant species can exhibit an increased oviposition efficiency. Manduca females that had already oviposited on Datura in a training phase, in the later test phase identified and reached a provided Datura leaf significantly faster (Nataraj et al., 2021). Therefore, despite not receiving direct feedback regarding its offspring’s performance on a given plant, a moth might profit from oviposition learning by being able to make a faster decision, that might result in energy saving and reduced exposure to potential predators. In addition, it is well established that many insects choose their host based on volatile cues (Bruce et al., 2005). These cues, however usually are complex blends where rather not the presence or absence of individual components but their relative amounts differ between host and non-host plants. Given the numerous plant-derived compounds that contribute to a potential host-plant blend and the infinitive number of potential blend compositions, identifying a host plant can become a difficult task. Accordingly, oviposition learning was also discussed as a potential strategy to mitigate limitations in host plant choice accuracy (Carrasco et al., 2015).
In this section, all the mentioned case studies have something in common: they stress how important it is to understand the oviposition-learning paradigm. While the relationship between flower or flower odor and nectar reward is well-established and extensively studied in insect foraging learning, the process through which insects acquire the knowledge to seek out oviposition sites remains largely unexplored. In each of the aforementioned case studies, it becomes apparent that insects memorize plant or fruit odors as a mechanism for locating their specific hosts, whether they are butterfly caterpillars, Drosophila larvae, or host plants. This association of odor with oviposition-related behavior highlights the pivotal role of oviposition-related behavior as a form of reward within this learning paradigm. In the subsequent section, we will delve into a more comprehensive examination of this reward mechanism.
Prior studies have established that insects are capable of learning and retaining memory for both foraging (Menzel, 1999; Giurfa, 2007) and oviposition behavior (Stanton, 1984; Mery and Kawecki, 2003; Nataraj et al., 2021). The reward for flower learning is evident, as it provides access to energy-rich nectar (Figure 2). Hence, one could consider this as “Pavlovian Conditioning” in which an insect associates flower stimuli with the rewarding nectar or pollen (Dupuy et al., 2006; Menzel, 1999; Giurfa, 2007).
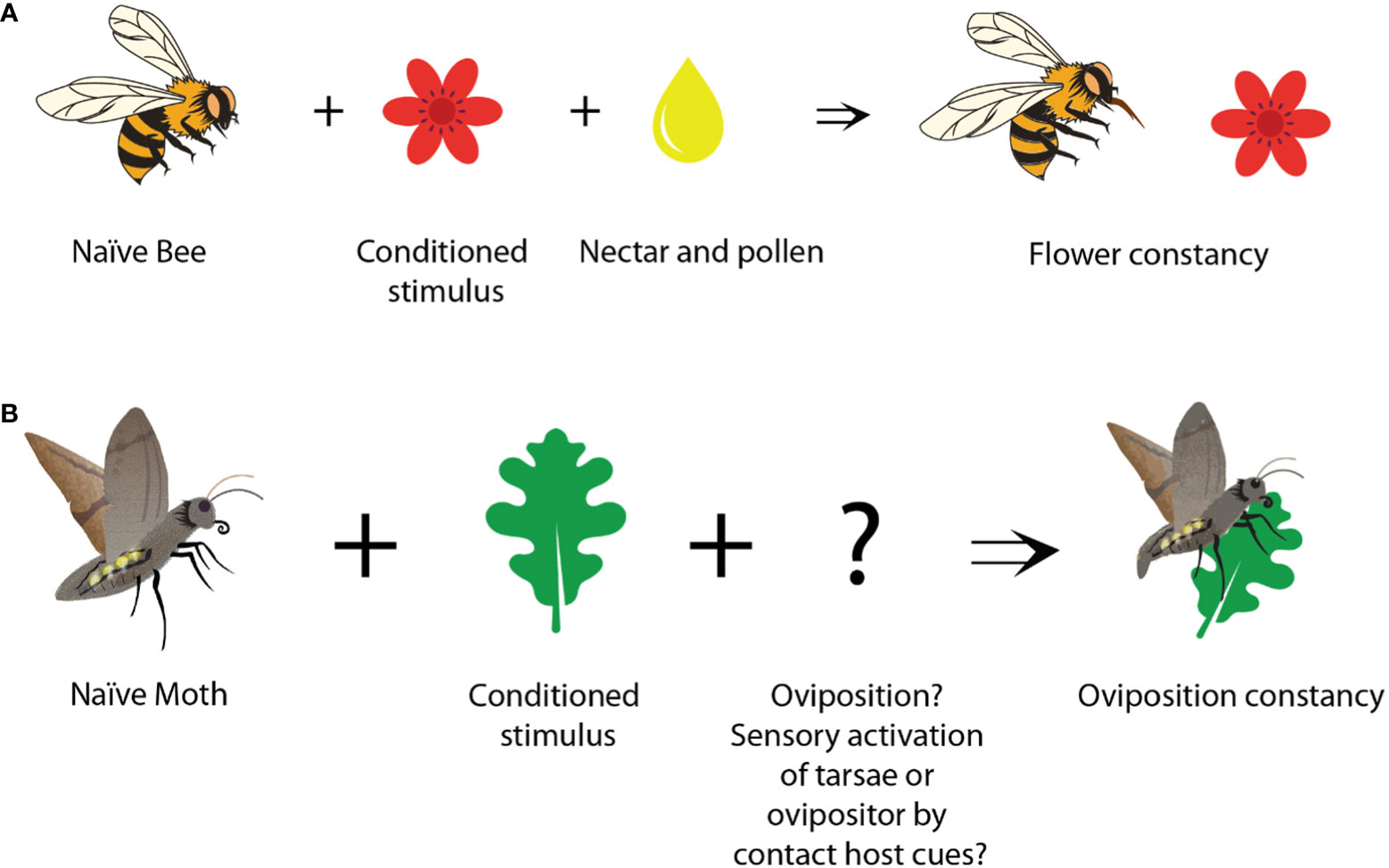
Figure 2 Appetitive learning of feeding and oviposition cues. (A) Flowers become more attractive to bees, after bees have visited them and were rewarded with nectar or pollen. (B) A host plant can become more attractive to a moth, after it has oviposited on it. The unconditioned stimulus (reward) in this process remains open.
The reward that reinforces host stimuli during oviposition learning, however, is less apparent (Figure 2). As in all the mentioned studies on oviposition learning (Stanton, 1984; Kaiser et al., 2003; Smid et al., 2007; Nataraj et al., 2021) the female insects targeted the learned host cues over distance, volatile host cues seem to act as the conditioned cues. At the same time, it is conceivable that the act of oviposition itself is intrinsically rewarding (Lewis and Takasu, 1990; Takasu and Lewis, 1993; Takasu and Lewis, 2003; Smid et al., 2007). Given the critical importance of reproduction for survival, behaviors that promote successful mating are pleasurable and tend to be favorably reinforced. For example, in Drosophila, successful copulation is inherently rewarding for male flies, and under such circumstances, ejaculation by male flies can be considered a form of reward (Zer-Krispil et al., 2018). Similarly, during oviposition, insects might form long-term appetitive memories associated with host chemicals, just because they were detected shortly before or during the oviposition process. Whether the act of oviposition is really needed, or whether the activation of sensory neurons on the tarsae (Städler, 2002) or ovipositor (Seada et al., 2016) is already sufficient, might, however, differ between the studied species. Identifying the specific sources that finally act as unconditioned rewards and induce oviposition learning is a difficult task. However, (Smid et al., 2007) could show, that already the presence of larval cues (e.g. larval feces or silk) was enough to induce the learning of plant cues in the parasitoid wasp Cotesia. Hence, oviposition itself was not needed to learn oviposition related cues. In another parasitoid, Microplitis croceipes, again oviposition was not necessarily needed, as these wasps learned host-related cues better, when their ovipositor was brought in contact with the haemolymph of the parasitized larvae (Takasu and Lewis, 2003). Obviously, many elements interact in complex ways that finally can lead to insect oviposition constancy.
Finally, it will be interesting to identify the neuronal circuits that are involved in the establishment of oviposition constancy. It is well established that the mushroom body calyces are they main centers of associative learning in insects (Heisenberg, 1998). Most of our knowledge regarding the neuronal tissues involved in the establishment of memory results from rather artificial experiments, where a formerly neutral stimulus becomes associated either with an electric shock as punishment or with a sugar reward (Adam et al., 2021). One can, however, speculate that the same tissues are also involved in a more natural situation, when e.g. host cues are learned during oviposition. Apart from the involved neuronal tissue it will also be interesting to decipher the neurochemical mechanisms that coordinate these behaviors. Biogenic amines, specifically dopamine and octopamine, are at the forefront of this discussion. Octopamine has been shown to govern oviposition behavior in the diamond back moth (Li et al., 2020). At the same time octopaminergic neurons within the antennal lobe, i.e. the first olfactory neuropil, are involved in the associative learning of feeding-related volatile cues in the tobacco hawkmoth (Riffell et al., 2013), the honeybee (Hammer and Menzel, 1998), and Drosophila (Schwaerzel et al., 2003). Dopamine, however, seems to be involved rather in aversive memory (Schwaerzel et al., 2003). From this, one could assume, that rather an octopaminergic circuit is involved in the establishment of oviposition constancy. However, more recent findings revealed that also dopaminergic neurons can be involved in the formation of appetitive memory (Cognigni et al., 2018) and experience-dependent oviposition decisions at least in flies (Vijayan et al., 2022). Furthermore, both octopamine and dopamine receptors turned out to be involved in the learning of oviposition cues in the parasitic wasp Nasonia vitripennis (Lenschow et al., 2018). It will therefore be interesting to investigate further, whether a conserved circuit and/or a conserved neurochemical mechanism govern oviposition learning in different insects.
Regardless of which sensory cues finally drive and which circuits finally process oviposition constancy, it can be hypothesized that this behavior is adaptive as it helps females to minimize their efforts spent on unsuccessful oviposition attempts and to focus their limited resources on hosts they have experienced before and have already judged carefully to be suitable for their offspring.
Author contributions
NN: Conceptualization, Visualization, Writing – original draft. BH: Conceptualization, Supervision, Writing – review & editing. MK: Conceptualization, Supervision, Visualization, Writing – review & editing.
Funding
The author(s) declare financial support was received for the research, authorship, and/or publication of this article. This work was funded by the Max Planck Society and by a DAAD grant to NN.
Acknowledgments
With profound gratitude, the first author dedicates this acknowledgment to her late friend Alexander Hausmann. His insightful discussions were invaluable to her research, and this overdue recognition is a heartfelt tribute to the wisdom and guidance he so generously imparted.
Conflict of interest
The authors declare that the research was conducted in the absence of any commercial or financial relationships that could be construed as a potential conflict of interest.
Publisher’s note
All claims expressed in this article are solely those of the authors and do not necessarily represent those of their affiliated organizations, or those of the publisher, the editors and the reviewers. Any product that may be evaluated in this article, or claim that may be made by its manufacturer, is not guaranteed or endorsed by the publisher.
References
Adam E., Hansson B. S., Knaden M. (2021). Moths sense but do not learn flower odors with their proboscis during flower investigation. J. Exp. Biol. 224 (17), 1–11. doi: 10.1242/jeb.242780
Bleeker M., Smid H., Steidle J., Kruidhof H., Van Loon J., Vet L. (2006). Differences in memory dynamics between two closely related parasitoid wasp species. Anim. Behav. 71 (6), 1343–1350. doi: 10.1016/j.anbehav.2005.09.016
Bruce T. J. A., Wadhams L. J., Woodcock C. M. (2005). Insect host location: a volatile situation. Trens Plant Sci. 10 (6), 269–274. doi: 10.1016/j.tplants.2005.04.003
Carrasco D., Larsson M. C., Anderson P. (2015). Insect host plant selection in complex environments. Curr. Opin. Insect Sci. 8, 1–7. doi: 10.1016/j.cois.2015.01.014
Chittka L., Gumbert A., Kunze J. (1997). Foraging dynamics of bumble bees: correlates of movements within and between plant species. Behav. Ecol. 8 (3), 239–249. doi: 10.1093/beheco/8.3.239
Chittka L., Thomson J., Waser N. (1999). Flower Constancy, Insect Psychology, and Plant Evolution. Animal Behav. 86, 361–377. doi: 10.1007/s001140050636
Cognigni P., Felsenberg J., Waddell S. (2018). Do the right thing: neural network mechanisms of memory formation, expression and update in. Drosophila. Curr. Opin. Neurobiol. 49, 51–58. doi: 10.1016/j.conb.2017.12.002
Dupuy F., Sandoz J.-C., Giurfa M., Josens R. (2006). Individual olfactory learning in Camponotus ants. Animal Behav. 76, 1081–1091. doi: 10.1016/j.anbehav.2006.03.011
Fitt G. P. (1989). The ecology of Heliothis species in relation to agroecosystems. Annu. Rev. entomol. 34 (1), 17–53. doi: 10.1146/annurev.en.34.010189.000313
Garvey M. A., Creighton J. C., Kaplan I. (2020). Tritrophic interactions reinforce a negative preference–performance relationship in the tobacco hornworm (Manduca sexta). Ecol. Entomol. 45 (4), 783–794. doi: 10.1111/een.12852
Geervliet J. B., Ariëns S., Dicke M., Vet L. E. (1998). Long-distance assessment of patch profitability through volatile infochemicals by the parasitoids Cotesia glomerata and C. rubecula (Hymenoptera: Braconidae). Biol. Control 11 (2), 113–121. doi: 10.1006/bcon.1997.0585
Geervliet J. B., Vet L. E., Dicke M. (1996). Innate responses of the parasitoids Cotesia glomerata and C. rubecula (Hymenoptera: Braconidae) to volatiles from different plant-herbivore complexes. J. Insect Behav. 9, 525–538. doi: 10.1007/BF02213877
Ghosh E., Sasidharan A., Ode P. J., Venkatesan R. (2022). Oviposition preference and performance of a specialist herbivore is modulated by natural enemies, larval odors, and immune status. J. Chem. Ecol. 48 (7-8), 670–682. doi: 10.1007/s10886-022-01363-5
Giurfa M. (2007). Behavioral and neural analysis of associative learning in the honeybee: a taste from the magic well. J. Comp. Physiol. A 193 (8), 801–824. doi: 10.1007/s00359-007-0235-9
Goulson D. (2000). Are insects flower constant because they use search images to find flowers? Oikos 88 (3), 547–552. doi: 10.1034/j.1600-0706.2000.880311.x
Goulson D., Ollerton J., Sluman C. (1997a). Foraging strategies in the small skipper butterfly, Thymelicus flavus: when to switch? Anim. Behav. 53 (5), 1009–1016. doi: 10.1006/anbe.1996.0390
Goulson D., Stout J. C., Hawson S. A. (1997b). Can flower constancy in nectaring butterflies be explained by Darwin’s interference hypothesis? Oecologia 112 (2), 225–231. doi: 10.1007/s004420050304
Goulson D., Wright N. P. (1998). Flower constancy in the hoverflies Episyrphus balteatus (Degeer) and Syrphus ribesii (L.) (Syrphidae). Behav. Ecol. 9 (3), 213–219. doi: 10.1093/beheco/9.3.213
Grant V. (1950). The flower constancy of bees. Botanical Rev. 16 (7), 379–398. doi: 10.1007/BF02869992
Greggers U., Menzel R. (1993). Memory dynamics and foraging strategies of honeybees. Behav. Ecol. Sociobiol. 32, 17–29. doi: 10.1007/BF00172219
Grüter C., Moore H., Firmin N., Helanterä H., Ratnieks F. L. W. (2011). Flower constancy in honey bee workers (Apis mellifera) depends on ecologically relistic rewards. J. Expe. Biol. 214, 1397–1402. doi: 10.1242/jeb.050583
Grüter C., Ratnieks F. L. W. (2011). Flower constancy in insect pollinators: Adaptive foraging behaviour or cognitive limitation? Commun. Integr. Biol. 4 (6), 633–636. doi: 10.4161/cib.16972
Hagel G., Landis B., Ahrens M. (1973). Aster leafhopper: source of infestation, host plant preference, and dispersal. J. Economic Entomol. 66 (4), 877–881. doi: 10.1093/jee/66.4.877
Hammer M., Menzel R. (1998). Multiple sites of associative odor learning as revealed by local brain microinjections of octopamine in honeybees. Learn. Memory 5 (1), 146–156. doi: 10.1101/lm.5.1.146
Haverkamp A., Bing J., Badeke E., Hansson B. S., Knaden M. (2016). Innate olfactory preferences for flowers matching proboscis length ensure optimal energy gain in a hawkmoth. Nat. Commun. 7 (1), 11644. doi: 10.1038/ncomms11644
Heisenberg M. (1998). What do the mushroom bodies do for the insect brain? An Introduction. Learn. Memory 5, 1–10. doi: 10.1101/lm.5.1.1
Hill P. S. M., Wells P. H., Wells H. (1997). Spontaneous flower constancy and learning in honey bees as a function of colour. Anim. Behav. 54 (3), 615–627. doi: 10.1006/anbe.1996.0467
Jallow M. F. A., Paul Cunningham J., Zalucki M. P. (2004). Intra-specific variation for host plant use in Helicoverpa armigera (Hübner) (Lepidoptera: Noctuidae): implications for management. Crop Prot. 23 (10), 955–964. doi: 10.1016/j.cropro.2004.02.008
Kaiser L., Pérez-Maluf R., Sandoz J. C., Pham-Delègue M. H. (2003). Dynamics of odour learning in Leptopilina boulardi, a hymenopterous parasitoid. Anim. Behav. 66 (6), 1077–1084. doi: 10.1006/anbe.2003.2302
Kandori I., Ohsaki N. (1996). The learning abilities of the white cabbage butterfly, Pieris rapae, foraging for flowers. Res. population Ecol. 38, 111–117. doi: 10.1007/BF02514977
Koutroumpa F. A., Monsempes C., François M.-C., Severac D., Montagné N., Meslin C., et al. (2021). Description of chemosensory genes in unexplored tissues of the moth Spodoptera littoralis. Front. Ecol. Evol. 9, 678277. doi: 10.3389/fevo.2021.678277
Kunjun W., Peiyu G., Yongming R. (2006). Is tomato plant the host of the oriental tobacco budworm, Helicoverpa assulta (Guenée)? Kun Chong xue bao. Acta Entomologica Sin. 49 (3), 421–427.
Ladner D. T., Altizer S. (2005). Oviposition preference and larval performance of North American monarch butterflies on four Asclepias species. Entomologia Experimentalis Applicata 116 (1), 9–20. doi: 10.1111/j.1570-7458.2005.00308.x
Larrea M. I. S. A., Larrea M. D. S. A., Olivos-Oré L. A. (2022). “Plants, poisonous (Animals),” in Reference module in biomedical sciences (Amsterdam: Elsevier).
Latty T., Trueblood J. S. (2020). How do insects choose flowers? A review of multi-attribute flower choice and decoy effects in flower-visiting insects. J. Anim. Ecol. 89, 2750–2769. doi: 10.1111/1365-2656.13347
Laverty T. M. (1994). Bumble bee learning and flower morphology. Anim. Behav. 47 (3), 531–545. doi: 10.1006/anbe.1994.1077
Lee H.-S., Hieu T. T., Ahn Y.-J. (2006). Oviposition-stimulating activity of (E)-capsaicin identified in Capsicum annuum fruit and related compounds towards Helicoverpa assulta (Lepidoptera: Noctuidae). Chemoecology 16, 153–157. doi: 10.1007/s00049-006-0341-0
Lenschow M., Cordel M., Pokorny T., Mair M. M., Hofferberth J., Ruther J. (2018). The post-mating switch in the pheromone response of Nasonia females is mediated by dopamine and can be reversed by appetitive learning. Front. Behav. Neurosci. 12, 14. doi: 10.3389/fnbeh.2018.00014
Lewis W., Takasu K. (1990). Use of learned odours by a parasitic wasp in accordance with host and food needs. Nature 348 (6302), 635–636.
Li F., Li K., Wu L.-J., Fan Y.-L., Liu T.-X. (2020). Role of biogenic amines in oviposition by the diamondback moth, Plutella xylostella L. Front. Physiol. 11, 475. doi: 10.3389/fphys.2020.00475
Liu Z., Scheirs J., Heckel D. G. (2010). Host plant flowering increases both adult oviposition preference and larval performance of a generalist herbivore. Environ. Entomol. 39 (2), 552–560. doi: 10.1603/en09129
Liu X.-L., Zhang J., Yan Q., Miao C.-L., Han W.-K., Hou W., et al. (2020). The molecular basis of host selection in a crucifer-specialized moth. Curr. Biol. 30 (22), 4476–4482. e4475. doi: 10.1016/j.cub.2020.08.047
Menzel R. (1999). Memory dynamics in the honeybee. J. Comp. Physiol. A 185 (4), 323–340. doi: 10.1007/s003590050392
Menzel R., Greggers U., Hammer M. (1993). “Functional organization of appetitive learning and memory in a generalist pollinator, the honey bee,” in Insect learning: ecology and evolutionary perspectives (New York: Springer), 79–125.
Mery F., Kawecki T. J. (2003). A fitness cost of learning ability in Drosophila melanogaster. Proc. Biol. Sci. 270 (1532), 2465–2469. doi: 10.1098/rspb.2003.2548
Mitter C., Poole R. W., Matthews M. (1993). Biosystematics of the heliothinae (Lepidoptera: noctuidae). Annu. Rev. Entomol. 38 (1), 207–225. doi: 10.1146/annurev.en.38.010193.001231
Nataraj N., Adam E., Hansson B. S., Knaden M. (2021). Host plant constancy in ovipositing Manduca sexta. J. Chem. Ecol. 47 (12), 1042–1048. doi: 10.1007/s10886-021-01309-3
Ozaki K., Ryuda M., Yamada A., Utoguchi A., Ishimoto H., Calas D., et al. (2011). A gustatory receptor involved in host plant recognition for oviposition of a swallowtail butterfly. Nat. Commun. 2 (1), 542. doi: 10.1038/ncomms1548
Prokopy R. J., Averill A. L., Cooley S. S., Roitberg C. A. (1982). Associative learning in egglaying site selection by apple maggot flies. Science 218 (4567), 76–77. doi: 10.1126/science.218.4567.76
Renwick J. A. A., Haribal M., Gouinguené S., Städler E. (2006). Isothiocyanates stimulating oviposition by the diamondback moth, Plutella xylostella. J. Chem. Ecol. 32 (4), 755–766. doi: 10.1007/s10886-006-9036-9
Riffell J. A., Alarcón R., Abrell L., Davidowitz G., Bronstein J. L., Hildebrand J. G. (2008). Behavioral consequences of innate preferences and olfactory learning in hawkmoth–flower interactions. Proc. Natl. Acad. Sci. 105 (9), 3404–3409. doi: 10.1073/pnas.0709811105
Riffell J. A., Lei H., Abrell L., Hildebrand J. G. (2013). Neural basis of a pollinator’s buffet: olfactory specialization and learning in Manduca sexta. Science 339 (6116), 200–204. doi: 10.1126/science.1225483
Romero B., Olivier C., Wist T., Prager S. M. (2020). Oviposition behavior and development of aster leafhoppers (Hemiptera: Cicadellidae) on selected host plants from the Canadian prairies. J. Economic Entomol. 113 (6), 2695–2704. doi: 10.1093/jee/toaa243
Schwaerzel M., Monastirioti M., Scholz H., Friggi-Grelin F., Birman S., Heisenberg M. (2003). Dopamine and octopamine differentiate between aversive and appetitive olfactory memories in Drosophila. J. Neurosci. 23 (33), 10495–10502. doi: 10.1523/JNEUROSCI.23-33-10495.2003
Seada M. A., Ignell R., Al Assiuty A. N., Anderson P. (2018). Functional characterization of the gustatory sensilla of tarsi of the female polyphagous moth Spodoptera littoralis. Front. Physiol. 9, 1606. doi: 10.3389/fphys.2018.01606
Seada M. A., Ignell R., Anderson P. (2016). Morphology and distribution of ovipositor sensilla of female cotton leaf worm Spodoptera littoralis (Lepidoptera: Noctuidae), and evidence for gustatory function. Entomological Sci. 19 (1), 9–19. doi: 10.1111/ens.12160
Smid H. M., Wang G., Bukovinszky T., Steidle J. L., Bleeker M. A., van Loon J. J., et al. (2007). Species-specific acquisition and consolidation of long-term memory in parasitic wasps. Proc. R. Soc. B: Biol. Sci. 274 (1617), 1539–1546. doi: 10.1098/rspb.2007.0305
Städler E. (2002). “Plant chemical cues important for egg deposition by herbivorous insects,” in Chemoecology of insect eggs and egg deposition. Eds. Hilker M., Meiners T. (Berlin, Germany: Blackwell Verlag), 171–204.
Städler E., Reifenrath K. (2009). Glucosinolates on the leaf surface perceived by insect herbivores: review of ambiguous results and new investigations. Phytochem. Rev. 8 (1), 207–225. doi: 10.1007/s11101-008-9108-2
Stanton M. L. (1984). Short-term learning and the searching accuracy of egg-laying butterflies. Anim. Behav. 32 (1), 33–40. doi: 10.1016/S0003-3472(84)80321-8
Takasu K., Lewis W. (1993). Host-and food-foraging of the parasitoid Microplitis croceipes: learning and physiological state effects. Biol. Control 3 (1), 70–74. doi: 10.1006/bcon.1993.1011
Takasu K., Lewis W. (2003). Learning of host searching cues by the larval parasitoid Microplitis croceipes. Entomologia Experimentalis Applicata 108 (2), 77–86. doi: 10.1046/j.1570-7458.2003.00070.x
Thöming G., Larsson M. C., Hansson B. S., Anderson P. (2013). Comparison of plant preference hierarchies of male and female moths and the impact of larval rearing hosts. Ecology 94 (8), 1744–1752. doi: 10.1890/12-0907.1
Vijayan V., Wang Z., Chandra V., Chakravorty A., Li R., Sarbanes S. L., et al. (2022). An internal expectation guides Drosophila egg-laying decisions. Sci. Adv. 8 (43), eabn3852. doi: 10.1126/sciadv.abn3852
Waser N. M. (1986). Flower constancy: definition, cause, and measurement. Am. Nat. 127 (5), 593–603. doi: 10.1086/284507
Wells H., Wells P. H. (1983). Honey bee foraging ecology: optimal diet, minimal uncertainty or individual constancy? J. Anim. Ecol. 829-836. doi: 10.2307/4457
Wells H., Wells P. H. (1986). Optimal diet, minimal uncertainty and individual constancy in the foraging of honey bees, Apis mellifera. J. Anim. Ecol. 55, 881–891. doi: 10.2307/4422
Yamamoto R. T., Jenkins R. Y., McClusky R. K. (1969). Factors determining the selection of plants for oviposition by the tobacco hornworm Manduca sexta. Entomologia Experimentalis Applicata 12 (5), 504–508. doi: 10.1111/j.1570-7458.1969.tb02548.x
Zalucki M., Daglish G., Firempong S., Twine P. (1986). The biology and ecology of Heliothis armigera and Heliothis punctigera (Lepidoptera, Noctuidae) in Australia - What do we know. Aust. J. Zool. 34 (6), 779–814. doi: 10.1071/ZO9860779
Zer-Krispil S., Zak H., Shao L., Ben-Shaanan S., Tordjman L., Bentzur A., et al. (2018). Ejaculation induced by the activation of Crz neurons is Rewarding to Drosophila males. Curr. Biol. 28 (9), 1445–1452.e1443. doi: 10.1016/j.cub.2018.03.039
Zhang J., Bisch-Knaden S., Fandino R. A., Yan S., Obiero G. F., Grosse-Wilde E., et al. (2019). The olfactory coreceptor IR8a governs larval feces-mediated competition avoidance in a hawkmoth. Proc. Natl. Acad. Sci. 116 (43), 21828–21833. doi: 10.1073/pnas.1913485116
Keywords: oviposition learning, insect learning, behavior, host choice, plasticity
Citation: Nataraj N, Hansson BS and Knaden M (2024) Learning-based oviposition constancy in insects. Front. Ecol. Evol. 12:1351400. doi: 10.3389/fevo.2024.1351400
Received: 06 December 2023; Accepted: 08 January 2024;
Published: 24 January 2024.
Edited by:
Joachim Ruther, University of Regensburg, GermanyReviewed by:
Torsten Meiners, Julius Kühn-Institut, GermanyCopyright © 2024 Nataraj, Hansson and Knaden. This is an open-access article distributed under the terms of the Creative Commons Attribution License (CC BY). The use, distribution or reproduction in other forums is permitted, provided the original author(s) and the copyright owner(s) are credited and that the original publication in this journal is cited, in accordance with accepted academic practice. No use, distribution or reproduction is permitted which does not comply with these terms.
*Correspondence: Markus Knaden, bWtuYWRlbkBpY2UubXBnLmRl