- 1Department of Plant and Soil Sciences, University of Pretoria, Pretoria, South Africa
- 2Range and Forage Division, Agricultural Research Council (ARC), Irene, Pretoria, South Africa
Introduction: Bush encroachment has a negative impact on the vegetation structure, ecosystem functions, and services of savanna rangelands. Woody plant encroachment creates an imbalance in the grass-to-tree ratio, leading to a decline in ecosystem services, including grazing capacity and soil nutrients.
Methods: This study aimed to evaluate the vegetation structure, diversity, and relationships of tree species across four different growth stages in the Marikana Thornveld. Three sites were identified at the Roodelpaat experimental farm, where six 1-hectare plots were established. In each plot, nine 10 m × 10 m subplots were set up to identify mature woody plants, shrubs, and saplings. Seedlings were identified within three 1.69 m² quadrats nested within each subplot.
Results and discussion: The study identified a total of 9,028 individual woody plants, including 158 seedling species, 161 saplings, 159 shrubs, and 154 mature woody plant species. The regression line plotted seedling density against the densities of mature trees, shrubs, and saplings, with b values significantly less than 1, indicating a negative impact of mature trees, shrubs, and saplings on the seedling layer. Seedling abundance displayed a non-linear relationship with mature trees, indicating a 4.75% representation of seedling abundance within the mature tree layer. Woody seedling species exhibited the highest abundance across four growth stages. Overall, across all woody plant species at different growth stages, there was a general decline in the woody density class, resulting in a J-shaped curve pattern. Seedlings and mature trees exhibited the highest diameter at breast height (dbh) proportions among individual woody plants from the first to the third DBH size classes, followed by a decline.
Conclusion: Generally, these results highlight a weak relationship between mature woody plant species and the understory layer, including shrubs, saplings, and seedlings. Consequently, mature woody plants cannot predict the establishment of understory woody plants or the recruitment of seedlings as a cause of bush encroachment.
1 Introduction
1.1 Background
Woody plant encroachment has been increasing worldwide for centuries at varying rates across different continents. Moreover, this has led to an alarming negative effect on the grassland-savanna biome (Sala and Maestre, 2014; Stevens et al., 2016; Archer et al., 2017; García Criado et al., 2020). Not fully acknowledging the role of human activities has led to a significant loss of the grassland-savanna biome (Ripley et al., 2022). Ecosystem degradation due to woody plant encroachment is a widespread issue in savanna ecosystems and requires thorough scientific investigation by rangeland ecologists (Belay et al., 2013; Ellis et al., 2019). The rise of woody plant encroachment in open savannas decreases grazing carrying capacity and subsequently affects grazing animals (Kimaro et al., 2019; Hare et al., 2020). This is because increased woody plant encroachment is typically characterized by thorny and unpalatable species, which are not favored by browsing animals (Kgosikoma and Mogotsi, 2013). Thus, in some areas, the savanna biome has transformed into a thicket-like biome due to the encroachment of woody plant species (Kimaro et al., 2019). The rapid encroachment of woody plants into savanna rangelands has been reported as a significant indirect threat to profitable beef enterprises based on natural rangelands (Dalle et al., 2006; Lunt et al., 2010).
Several studies on woody plant encroachment have previously focused on biotic and abiotic factors, either individually or in combination, to understand the main contributors to this phenomenon (Holdrege et al., 2021). The areas most impacted by the encroachment of woody plant species are rangelands primarily utilized as grazing lands for livestock and/or game animals, owing to their grass cover and tree species that are acceptable for grazing and browsing animals (Kgosikoma and Mogotsi, 2013). Consequently, the quality of rangelands has deteriorated because of overgrazing, inadequate management practices, and the impacts of climate change (Ratajczak et al., 2011). Alterations in vegetation structure and species composition impact the sustainability of livestock production and ecosystem services (Mussa et al., 2016). Additionally, rangeland degradation due to bush encroachment seems to weaken ecosystem integrity and reduce grazing capacity (Sankaran et al., 2008). Once 75% of the total of the savanna rangeland is covered by woody plant species, that rangeland is declared bush encroached (Oba et al., 2000; Bond and Midgley, 2001; Kellner et al., 2022).
Encroaching woody plant species may include native trees and/or shrubs that inhabit grasslands and savanna grazing areas (Kgosikoma and Mogotsi, 2013). In South Africa, bush encroachment has reached a scale that demands scientific intervention (O’Connor and Chamane, 2012). Kraaij and Ward (2006) reported that the extent of bush encroachment in South Africa has reached an alarming level, with approximately 20 million hectares of land declared as bush encroached by woody plant species. Additionally, Higgins and Scheiter (2012) and Moncrieff et al. (2014) reported similar findings, indicating that bush encroachment is one of the most recognized rangeland issues in South Africa, particularly in the savanna regions. This problem is likely to escalate further over time, depending on the measures taken to reverse its aggressive inversion in savanna regions. As part of restoration programs, new technologies have been integrated with traditional rangeland techniques used in the long-term monitoring of these areas. Hudak and Wessman (1998) demonstrated a technique for mapping bush densities across a savanna landscape using historical aerial photographs from 1955, 1970, and 1984 to understand the factors that could be driving bush density in savanna rangelands.
However, restoring and rehabilitating areas affected by bush encroachment require advanced technologies to monitor changes in vegetation, especially given the impact that bush encroachment has on savanna rangelands (Maphanga et al., 2022). Currently, rangeland assessment and monitoring primarily rely on comparing the existing species composition to an expected successional end-state defined by the ecological condition of the site (D’Odorico et al., 2012). For instance, historically, rangeland assessment and monitoring have primarily relied on fieldwork-based surveys, which are often time-consuming, costly, and occasionally inaccurate (Mutanga et al., 2016). These methods frequently require specialized technicians to perform laboratory analyses of plant and soil chemicals in order to assess forage quality. (Zhao et al., 2016; Zhang et al., 2016). Therefore, an integrated assessment that utilizes satellite remote sensing techniques along with fieldwork methods is essential (Jindo et al., 2021). These techniques aim to offer significant guidelines for monitoring savanna rangelands affected by woody plant species and to ensure sustainable management that enhances savanna rangeland productivity. Therefore, it is essential to have skills in remote sensing tools and ecology in order to provide valuable support to policymakers. This enhances the savanna’s carrying capacity and investigates the dynamics of woody plant species and ecosystem properties in a cost-effective manner.
The study focuses on assessing woody vegetation to analyze the effects and dynamics of bush encroachment in savanna rangelands. This is to examine the changes in vegetation that lead to bush encroachment and its relationship with understory establishment. Subsequently, the data from this study are also valuable for validating the predictions of existing models that forecast savanna rangeland productivity after bush encroachment. Moreover, despite the negative ecological impacts of bush encroachment, there have been very few studies examining how woody plant encroachment affects the successful development of the understory woody plant layer and its relationship with mature trees (Archer et al., 2017). Thus, this study aims to highlight the intraspecific interactions among woody plant species in the Marikana Thornveld.
2 Materials and methods
2.1 Study site
The study was conducted at the Roodeplaat Experimental Farm (25°36′S, 29°2’08′E, altitude 1,182 m) of the Agricultural Research Council (ARC), which encompasses over 2,067 hectares of natural vegetation outside Pretoria, South Africa (Figure 1). The average rainfall is 687 mm, with the most rainfall occurring during austral summer (November to February). The daily maximum summer temperature ranges from 20°C to 29°C, while the winter temperature can range from 2°C to 16°C. The soil types in the study area consist of vertisols, ferralsols, and luvisols. The vegetation type of the study site is Marikana Thornveld, characterized by open Vachellia karroo and Senegalia caffra veld types (Mucina and Rutherford, 2006). The farm is primarily dominated by the tree species Vachellia karroo, Senegalia caffra, Ziziphus mucronata, and Combretum zeyheri. The most dominant grass species include Panicum maximum, Setaria sphacelata, Digitaria eriantha, Themeda triandra, Heteropogon contortus, and several weak annual species such as Aristida congesta subspecies congesta, and subspecies barbicollis (van Rooyen, 1983). On the farm, three sites were designated to evaluate the composition of woody species and the changing patterns of these species on the farm. All three sites were marked within heavily encroached areas where the density of woody plants exceeded the acceptable threshold of 2,500 plants per hectare, displaying a similar species composition. Invasive alien plant species are found in high densities in localized areas, especially along drainage lines. The common invasive plants in the study area include Tagetes minuta, Bidens pilosa, Lantana camara, and Pontederia crassipes (Rutherford and Powrie, 2012). The study site has only been grazed and browsed by a very small number of antelopes, such as Tragelaphus strepsiceros.
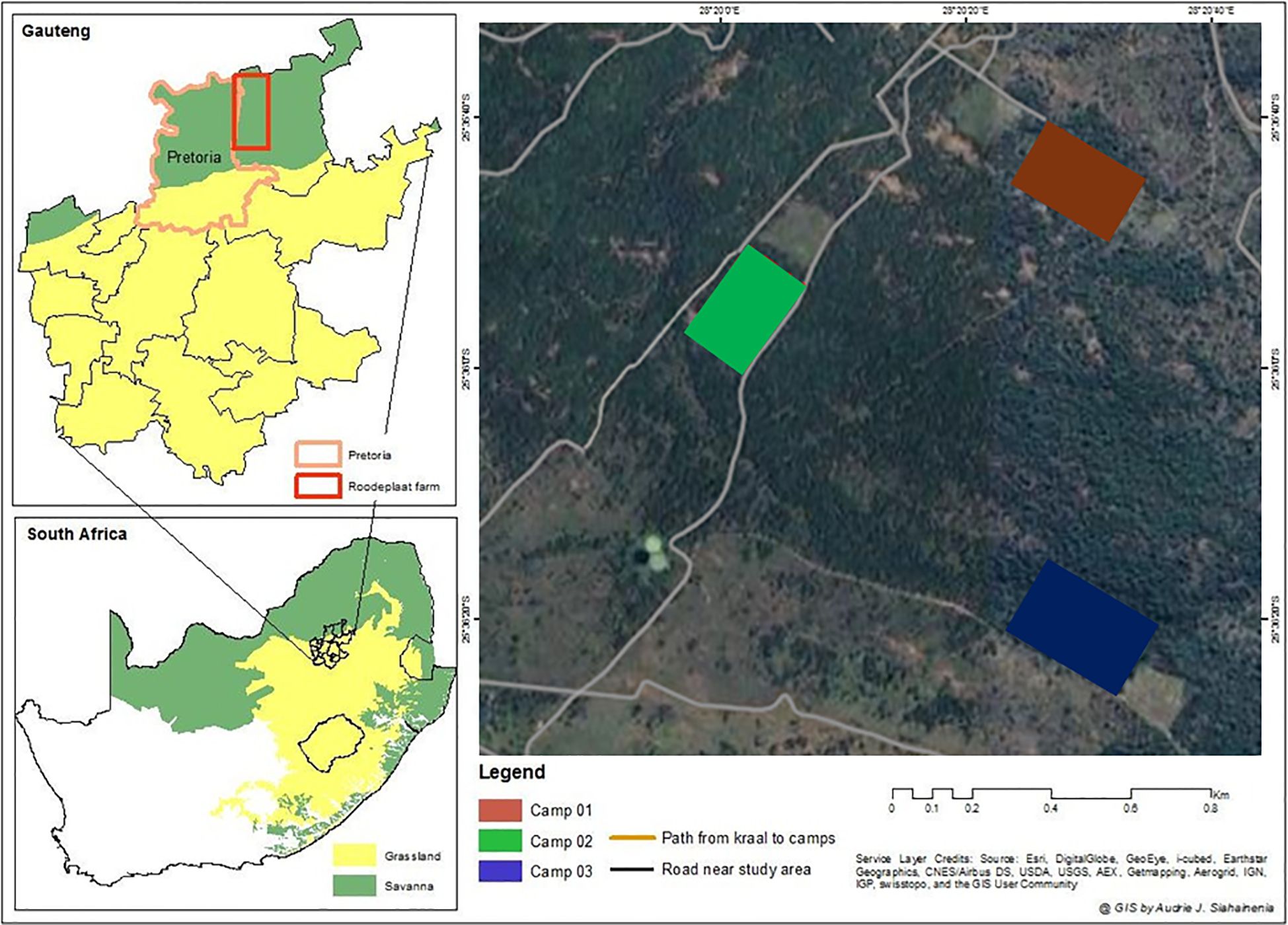
Figure 1. The map displays the location of the Roodeplaat Experimental Farm, which covers an area of 2,067 hectares in Gauteng Province, South Africa, just outside Pretoria. In the bottom right corner, there is a Google Map image of the study area at Roodeplaat, with three sites represented by red squares on the map.
2.2 Data collection
2.2.1 Study design
For this study, vegetation assessments were conducted during the growing season from October 2020 to February 2022. Three bush-encroached sites measuring 320 m × 223 m were randomly selected. A completely randomized design (CRD) was conducted using aerial photographs downloaded from the United States Geological Survey Earth Explorer from 2020, focusing on three sites measuring 320 m × 223 m that depicted areas affected by bush encroachment. All three sites featured comparable woody vegetation types, similar soil nutrient compositions, and alike topography. The sites were chosen based on aerial photographs and the degree of encroachment by woody plant species. Coordinates were recorded to position the sites on the ground. Three sites were randomly chosen and six 1-hectare plots per site were marked based on their level of encroachment. In each of the six 100 m × 100 m plots across the three sites, nine 10 m × 10 m subplots were established for identifying woody plant species, including mature trees, shrubs, and saplings. In each of the nine 10 m × 10 m subplots, three 1.3 m × 1.3 m quadrats were placed in the corner, center, and opposite corner for woody seedling identification (Figure 2). All woody plants within these 10 m × 10 m subplots were identified. Four growth stages of woody plant species based on maximum height were measured, and snag/rotting logs for which the original height was not known were excluded. In each subplot, tree height, density/abundance, and stem diameter parameters were measured. All unknown woody plant species encountered during identification were further examined by ecologists, and a few unidentified individual plant specimens were sent to the South African National Biodiversity Institute (SANBI) herbarium for additional identification. Subsequently, all woody plant species were identified. However, individual woody plants and seedlings without leaves were excluded from this study.
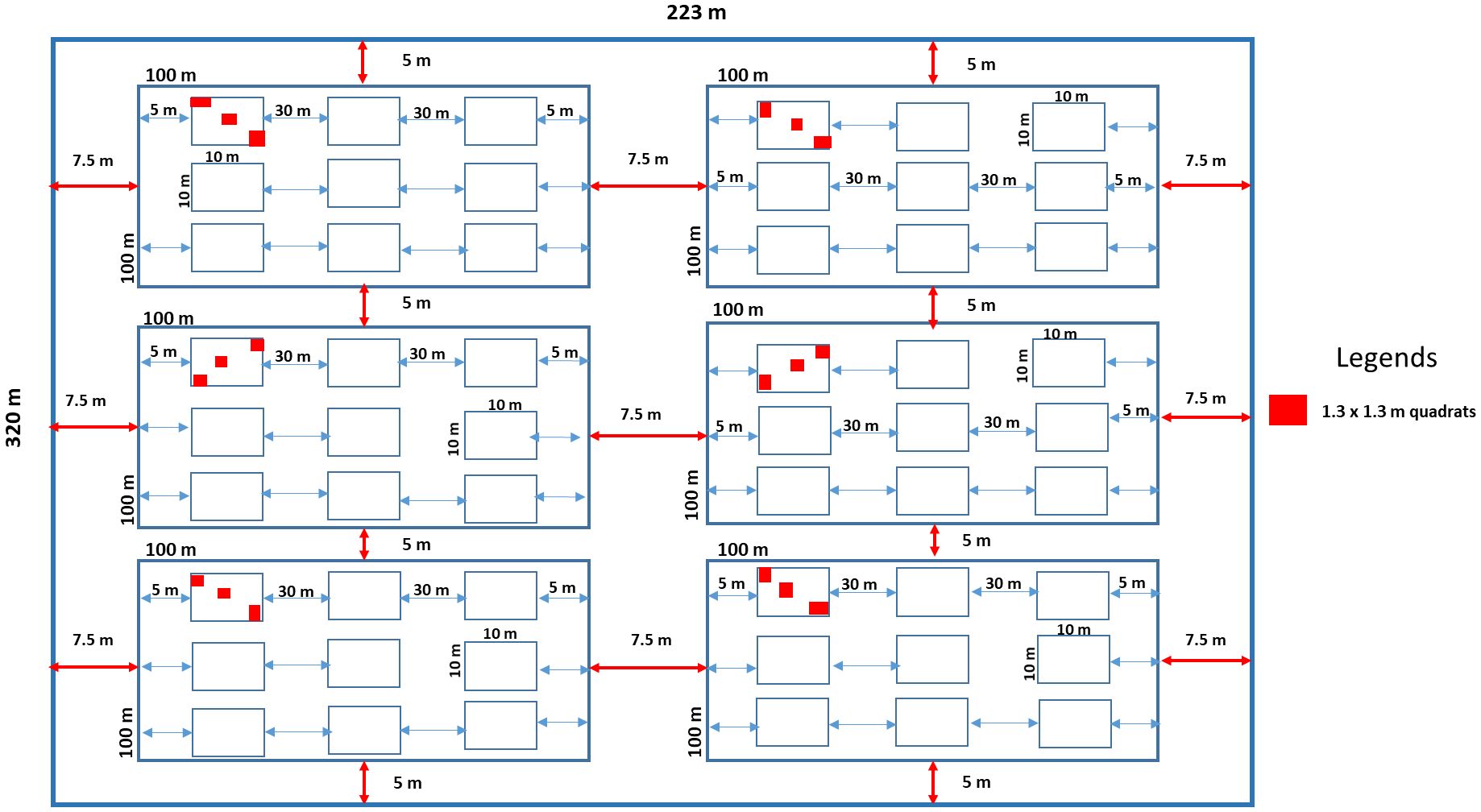
Figure 2. Sampling design for assessing woody species in the Marikana Thornveld at the Roodeplaat Experimental Farm outside Pretoria.
2.3 Data analysis
2.3.1 Assessment of wood plant species vegetation
Woody plants were systematically categorized into different height classes to classify age groups. This was followed by calculating the diversity, richness, and evenness of the woody species. Seedlings ≤ 0.3 m in height and with a diameter at breast height (DBH) of less than 1.0 to ≤ 2.50 cm were identified and measured within each 1.69 m² quadrat. Saplings, shrubs, and mature woody plants were also measured, with a DBH of ≥ 2.50 cm, and heights ranging from ≥ 0.3 to 2 m for saplings, > 2 to 3.5 m for shrubs, and > 3.5 to 10 m for mature trees. All individual woody species with a DBH greater than 5 cm were sampled within the 10 m × 10 m sub-plots using a measuring tape, a 4-m calibrated measuring rod, and forestry 550 hypsometers for trees over 4 m (Table 1) (van der Maarel, 1979). The variation in species abundance among the seedling layer, mature trees, shrubs, and sapling tree species was assessed. We modeled the relationship between seedling abundance and the abundance of mature trees, shrubs, and saplings.
The relationship between woody seedlings and mature trees, shrubs, and sapling tree woody species abundance was assessed through regression analysis using Equation 1 as:
where S is the number of identified woody seedlings, R is the number of mature trees in the 2020–2022 census and α is the mean abundance-independent seedling abundance per mature trees, shrub, and sapling tree abundance while b captures the effect of species abundance on per capita seedling abundance. This means that if the b value is equal to 1, there is no effect of mature trees, shrubs, and sapling tree abundance on seedling abundance. If b is less than 1, it indicates a negative effect; however, a b value greater than 1 indicates a positive effect of mature trees, shrubs, and sapling trees on seedling abundance. This means that an increase in the abundance of mature trees, shrubs, and saplings leads to an increase in woody seedling abundance, resulting in a positive effect. Conversely, a decrease has a negative effect. The power function “b” was determined by log-transforming both sides of Equation 1 to create a straight-line Equation 2:
The linear regression analyses were performed using SigmaPlot 13.0 (Systat Software, 2013).
We calculated estimates of diversity for the seedling layer and for all saplings, shrubs, and mature trees. Collected data were analyzed to calculate the species richness index and alpha diversity indices. As a measure of evenness, we calculated the Shannon–Wiener probability of intraspecific encounter (Shannon and Wiener, 1963), which is related to rarefaction and is also independent of sample size (Olszewski, 2004).
2.3.2 Woody plant species diversity
The diversity of woody plant species was calculated using the Shannon–Wiener diversity index formula using Equation 3.
Here H’ is the Shannon–Weiner diversity index, S is the species richness, Pi is the number of individuals of each species, and the natural logarithm of Pith individual species. The evenness of woody species was further quantified using the Shannon–Wiener evenness index, where J represents the species evenness using Equation 4:
2.3.3 Vegetation structure of the Marikana Thornveld
The structural parameters, specifically density and diameter at breast height, were measured. The density of woody plants is used to analyze the structure of the vegetation in the Marikana Thornveld using Equation 5:
Here, D is the woody plant species density, N is the total number of individual woody plants, and A is the area in which woody plant species were identified. Woody plant density was calculated within 10 x 10 m subplots and subsequently extrapolated to plants per hectare. Diameter at breast height was categorized into four DBH classes and organized in ascending order to evaluate the structural patterns of the Marikana Thornveld (Table 1). The DBH was calculated from mature woody plant species, shrubs, saplings, and seedlings by measuring the woody species’ circumferences using Equation 6.
where DBH (in cm) is the diameter at breast height, C is the circumference, and Π is Pi, which is equal to 3.14. Furthermore, the importance value index was calculated through summation of the following three parameters measured i.e., relative frequency (RF), and relative density (RD) (Kent, 2011).
2.3.4 Regeneration vegetation status of younger plants (i.e. saplings and seedlings) and mature woody plants
The regeneration patterns of woody plant species were determined by the density ratios of all woody species size classes (i.e., the ratio of seedlings to saplings, seedlings to shrubs, seedlings to mature trees, saplings to shrubs, saplings to mature trees, and finally shrubs to mature trees). All counts of seedlings, saplings, shrubs, and mature trees were presented in hectares.
2.3.5 Statistical analysis
All analyses were conducted using SigmaPlot statistical software. A univariate analysis was performed to test for normality and equality of variances through Shapiro–Wilk tests. Consequently, all the datasets collected underwent Shapiro–Wilk tests to determine if they were normally distributed; the p-value of the Shapiro–Wilk test was greater than 0.05, indicating that the datasets were normally distributed. Before analysis, the woody plant density was log10 transformed to meet the assumptions of the analysis of variance (ANOVA) test. Data for mature woody plants, shrub density, saplings, and seedling woody plant density were log10 transformed prior to regression analysis. Mature woody plant abundance and mature tree density underwent log and log10 transformations, respectively. A one-way ANOVA was performed to assess the relationship between woody seedling plant species density and the density of mature woody plant species, shrub density, sapling density, and young (saplings and seedlings) plant density using the general linear model (GLM). The diversity of woody plant species was calculated using Fisher’s alpha index and the Shannon–Wiener diversity index (H′). Species composition frequency analyses were conducted using XLSTAT software (version 2024.5, Addinsoft, New York, USA).
3 Results
3.1 Representation of seedling abundance in the abundance of adult trees (mature trees and shrubs)
A total of 9,028 woody plant individuals were recorded, including 158 seedlings, 161 saplings, 159 shrubs, and 154 mature woody plants, in three sites measuring 320 m × 223 m during the 2020–2022 woody plant species survey (Table 2). The relative abundance of adult trees (mature trees and shrub plant species) accounted for 16% of the variation in the abundance of young woody plant species (saplings and seedlings), with r² = 0.16, p ≤ 0.001 (Figure 3B). However, when assessing the relationship between seedling abundance and mature trees at the subplot level, the results indicated a weak correlation between seedling abundance and the abundance of mature woody plant species (Figure 3A). Generally, the slope of the regression line and the correlation coefficient for seedling abundance had no impact on mature tree species abundance (Figure 3A). However, when compared to adult trees (mature trees and shrub density) and young trees (sapling and seedling density), as shown in Figure 3B, there was a slight increase in the relationship among the stages of tree growth. The number of mature trees in the Marikana Thornveld represented only 4.75% of the seedling abundance (r² = 0.0475, df = 134, p < 0.0001). However, the regression line fitted for the log-transformed data was significantly less than 1, with a b value of 0.1535 representing the negative effect of mature woody plants on seedling abundance. The slope of the regression line was significantly less than 1, indicating that the number of seedlings per mature tree declines with increasing reproductive mature tree abundance (Figure 3A).
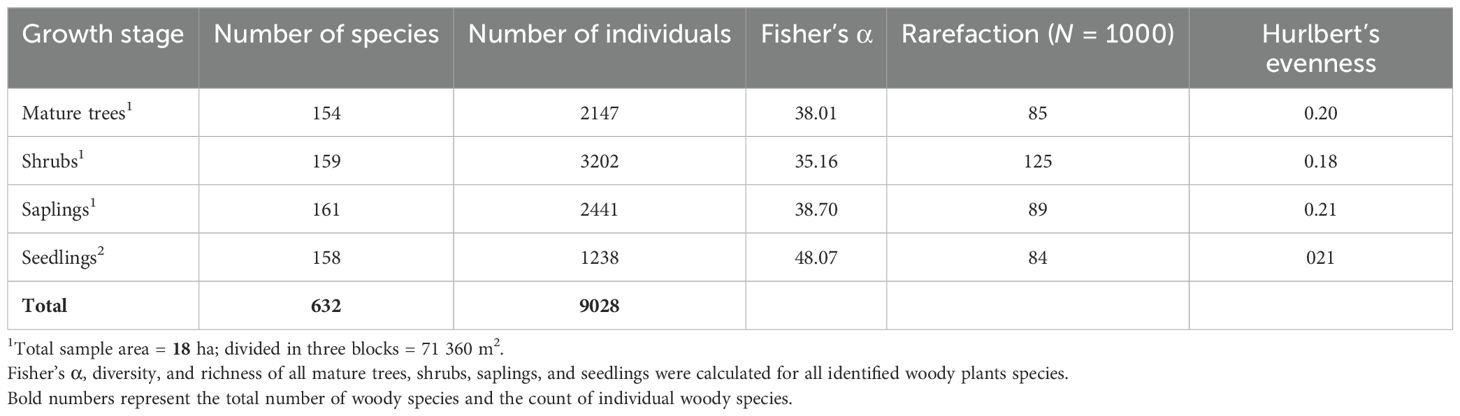
Table 2. The number of woody plant species and individuals at each growth stage encountered during the 2020-2022 identification of woody species was analyzed.
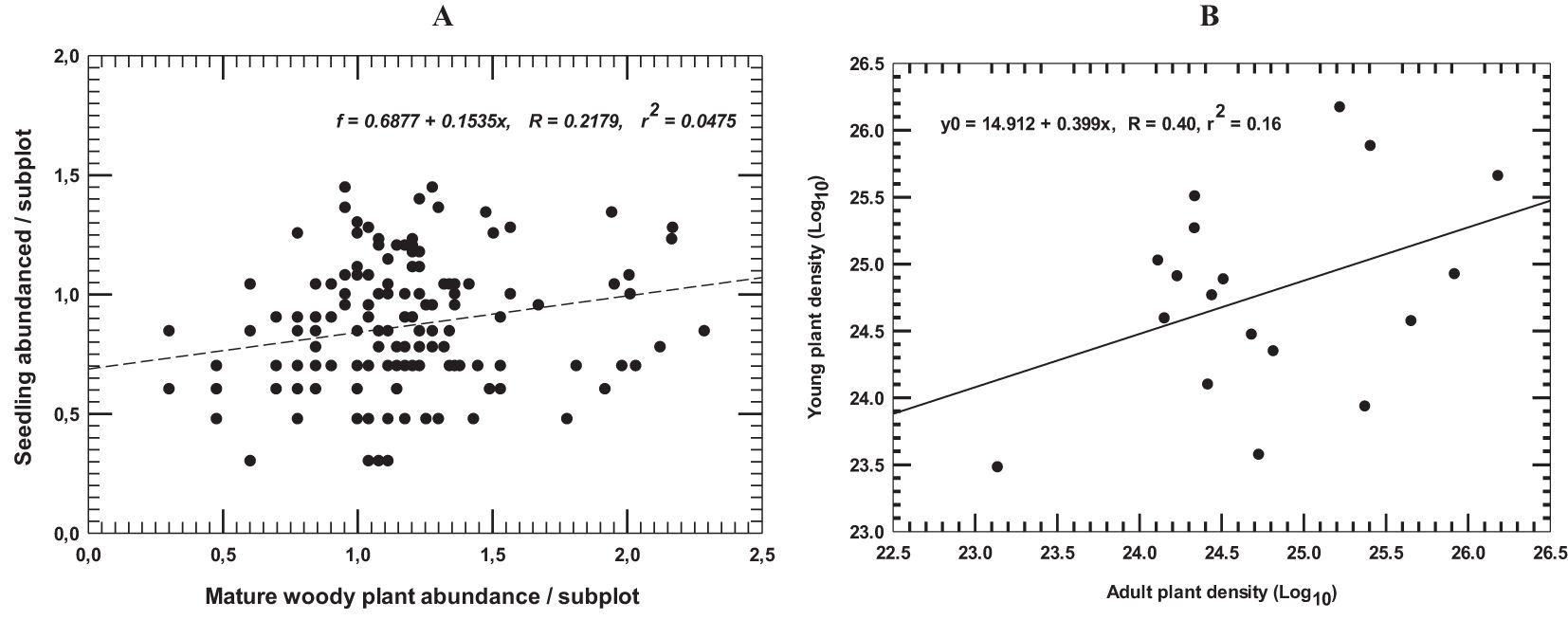
Figure 3. (A) The relationship between seedling abundance and mature woody plant abundance. (B) The relationship between adult plant density (mature trees and shrubs) and young plant density (sapling and seedling density) in the Marikana Thornveld.
The slope of the regression line for the log-transformed data for woody plant density was significantly less than 1, with b values of 0.58 (r² = 0.58), 3.23 (r² = 0.61), and 0.64 (r² = 0.39) for per capita seedling abundance for mature tree, sapling, and shrub abundance, respectively, at a 95% confidence interval (Figure 4). This indicated that as the abundance of mature trees, saplings, and shrubs increased, there was a general decline in seedling abundance (Figures 4a, c). However, the b value shown in Figure 4b was greater than 1 (b = 3.23, r² = 0.61), indicating a positive effect of per capita seedling abundance on the abundance of saplings’ woody plant species. Furthermore, there was a positive correlation between seedling abundance and mature tree, sapling, and shrub abundance, with R values of 0.75, 0.78, and 0.68, respectively (Figure 4). Figure 5 demonstrates a very poor relationship between seedling density and mature tree, shrub, and sapling density. The results in Figure 5 illustrate that the assessed relationship exists at a site level. However, the overall findings indicate that mature tree, shrub, and sapling density has no effect on seedling establishment.
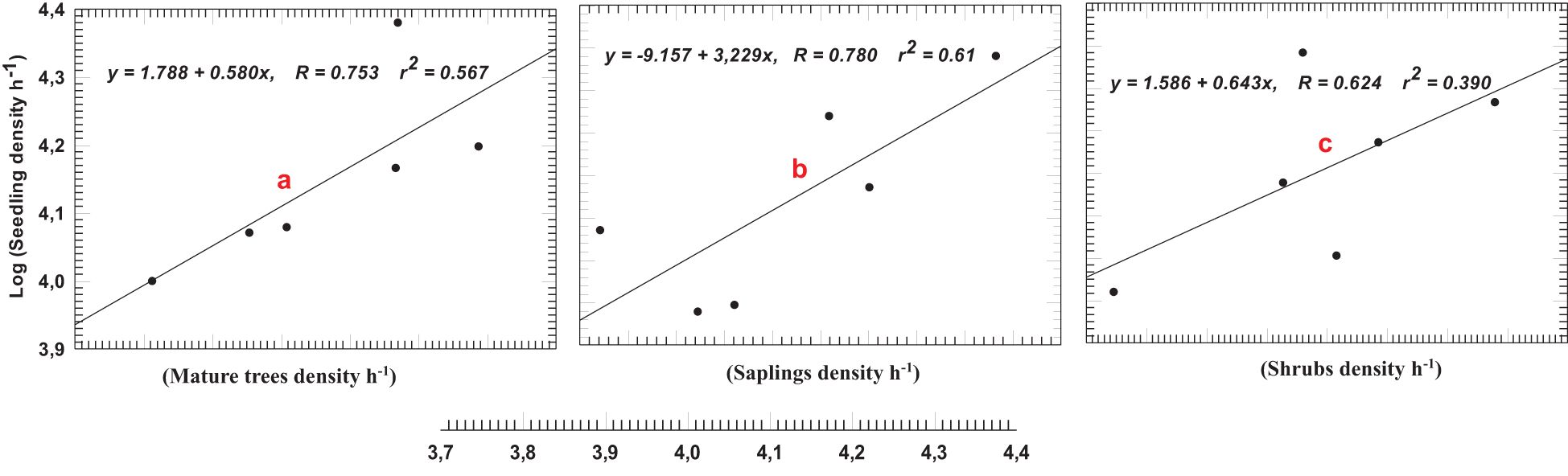
Figure 4. Each graph illustrates the relationship between seedlings and mature tree, sapling, and shrub densities at the site level. Lower-case letters represent each graph.
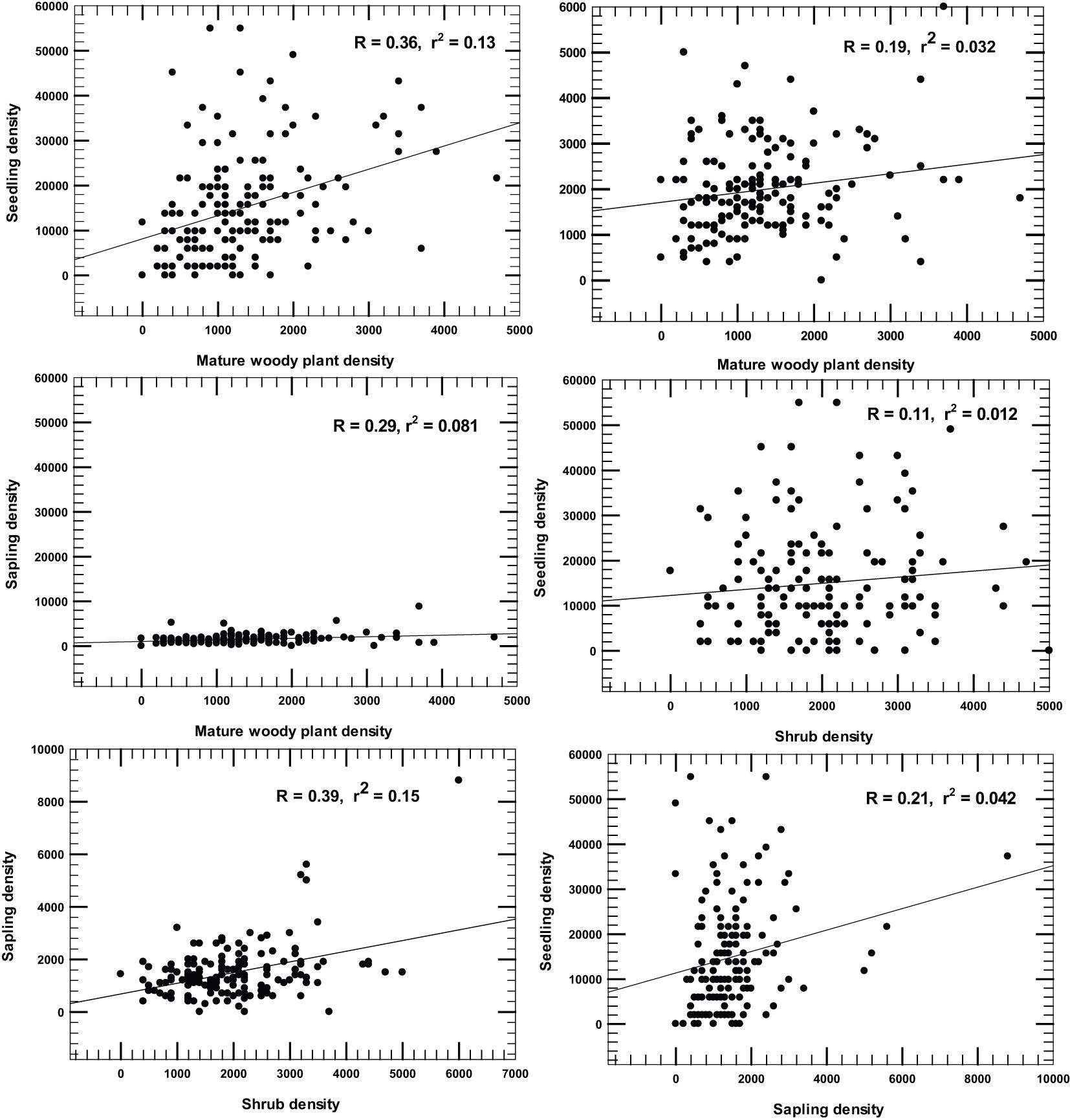
Figure 5. Each of these graphs illustrates the relationship between woody plant density and different growth stages at the site level.
3.2 Species diversity and the evenness of woody seedlings and adult trees
According to both Fisher’s alpha values and estimates of diversity based on rarefaction, tree species diversity was lower in the shrub layer compared to all other growth stages. However, the seedling layer recorded the highest Fisher’s alpha index. This indicates that, even though the number of individuals in the seedling layer was low, the ratio of the total number of individuals to the number of species exceeded 1, which reflects a completeness of taxa and individuals. Although species evenness did not differ significantly, a noticeable decline was observed in the shrub layer (Table 2).
H’ and evenness (J) were used to evaluate the diversity of woody species at the study site across various growth stages. The results in Table 3 also showed that the shrub and sapling layer had high species diversity compared to that of mature trees and the seedling layer, with 5.05 and 5.12, and 4.89 and 4.75, respectively. Compared to adult trees, the seedling layer showed moderate to low species richness and evenness (Rutherford and Powrie, 2013) (Table 3).
3.3 Vegetation structure of the Marikana Thornveld
3.3.1 Density
In the Marikana Thornveld, the density of wood plant species for mature trees, shrubs, saplings, and seedlings was recorded as 3,322 ha-1, 6,256 ha-1, 9,978 ha-1, and 4,136 individual plants ha-1, respectively. Woody plant density was further calculated based on the individual woody plant species across various growth stages in the Marikana Thornveld. The highest density of mature tree species was recorded for Ehretia rigida at 7,233 plants per hectare, followed by Dichrostachys cinerea with 6,633 plants per hectare, and Combretum zeyheri with 6,133 plants per hectare. Some woody plant species exceeded the encroachment threshold of 2,500 plants per hectare, ranging from 2,700 to 9,978 plants per hectare. In contrast, Euclea natalensis (19 per hectare), Senegalia mellifera (219 per hectare), Searsia dentata, Olea europaea, Millettia grandis, and Combretum molle (315 per hectare) were the least dense species in the Marikana Thornveld (Table 4).
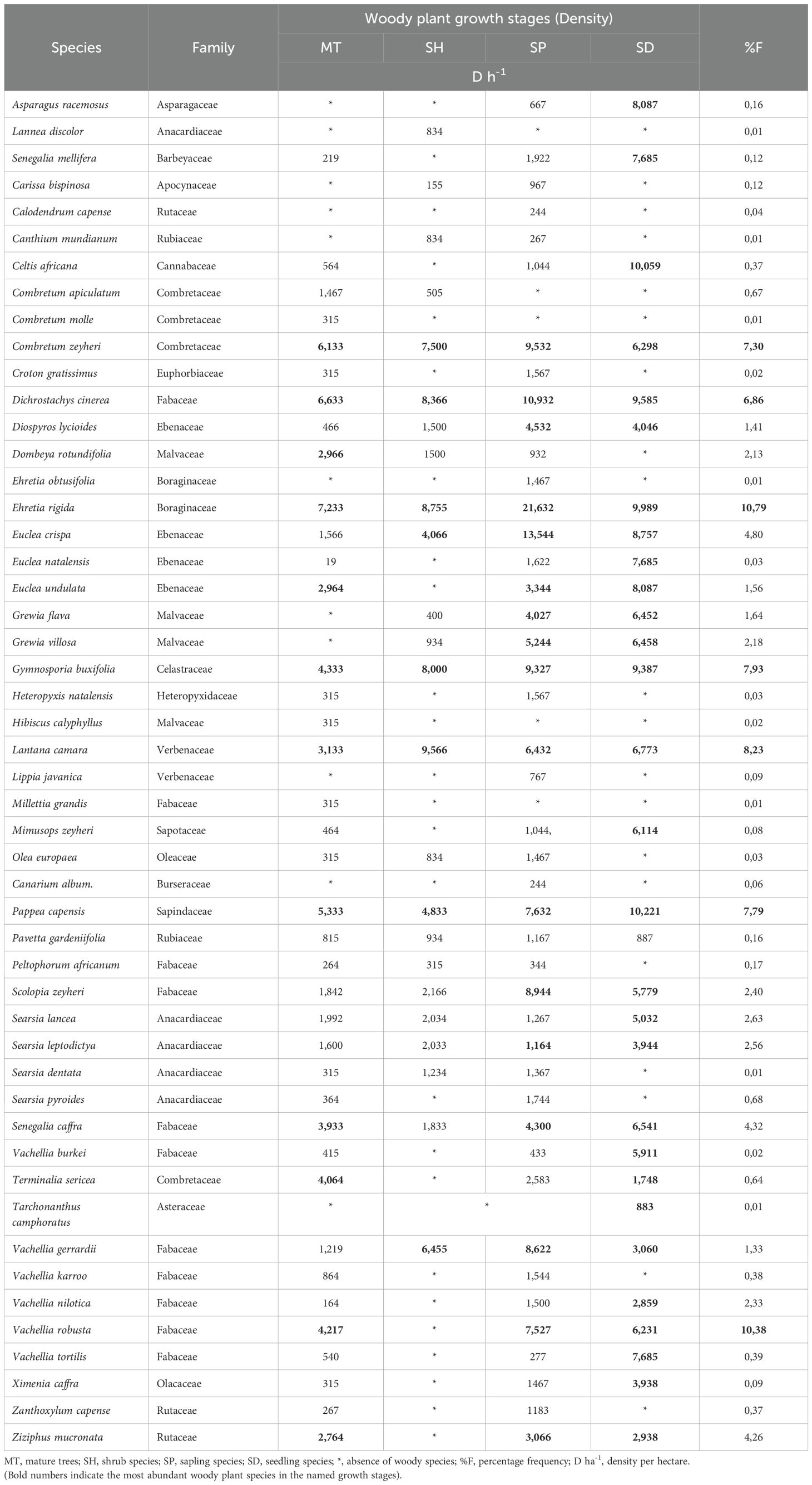
Table 4. Density and percentage frequency of woody plant species in the 2020–2022 census in the Marikana Thornveld.
The most abundant woody shrub species recorded was Lantana camara (9,566 plants ha-1), followed by Ehretia rigida (7,233 ha-1). The least abundant species recorded were Carissa bispinosa (155 ha-1), Grewia flava (400 ha-1), and Combretum apiculatum (505 ha-1). This study also noted several common woody plant species for both saplings and seedlings, with the most dominant being Ehretia rigida (6,326 ha-1; 9,989 ha-1), Combretum zeyheri (6,375 ha-1; 6,298 ha-1), and Gymnosporia buxifolia (6,327 ha-1; 9,387 ha-1). Overall, Ehretia rigida, Dichrostachys cinerea, Gymnosporia buxifolia, Combretum zeyheri, Pappea capensis, Lantana camara, and Vachellia robusta were the most abundant species recorded across all growth stages. There were also common to moderate species abundances compared to those recorded at all growth stages (Table 4).
3.3.2 Density classes
Across the various woody plant species at different growth stages, there was a general decline in woody species density class that formed a J-shaped pattern. The formation of this pattern can be attributed to several events related to plant succession. For example, mature trees and shrubs may have reached their mature state. Therefore, the mortality rate from plant diseases and competition for natural resources can result in a decline in wood density classes due to the death of older trees and the establishment of snags (dead trees). The density classes of seedlings and saplings also formed a J-shaped curve pattern (Figure 6).
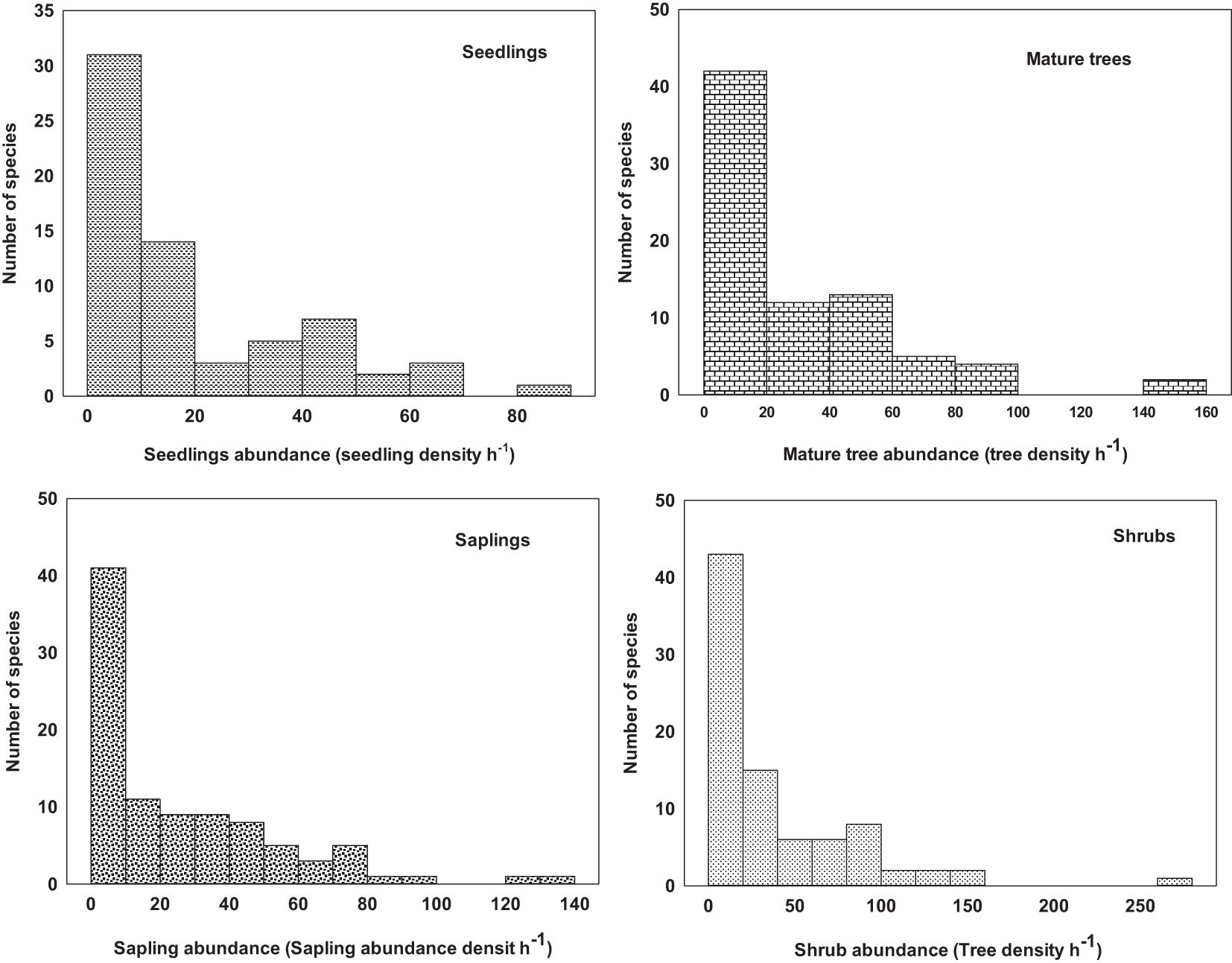
Figure 6. Density classes of woody plant species across various tree growth stages in the Marikana Thornveld, Pretoria.
3.3.3 DBH size-class distribution
Seedlings and mature trees are the only group among the four growth stages that form a J-shaped pattern. The first one to three DBH size classes exhibited the highest proportion of individual wood species abundance per hectare, followed by a noticeable decline. In contrast to these results, the highest DBH size class for shrubs and saplings was recorded in the last DBH class, accounting for approximately ± 600 to 1200 individuals per hectare per hour (Figure 7).
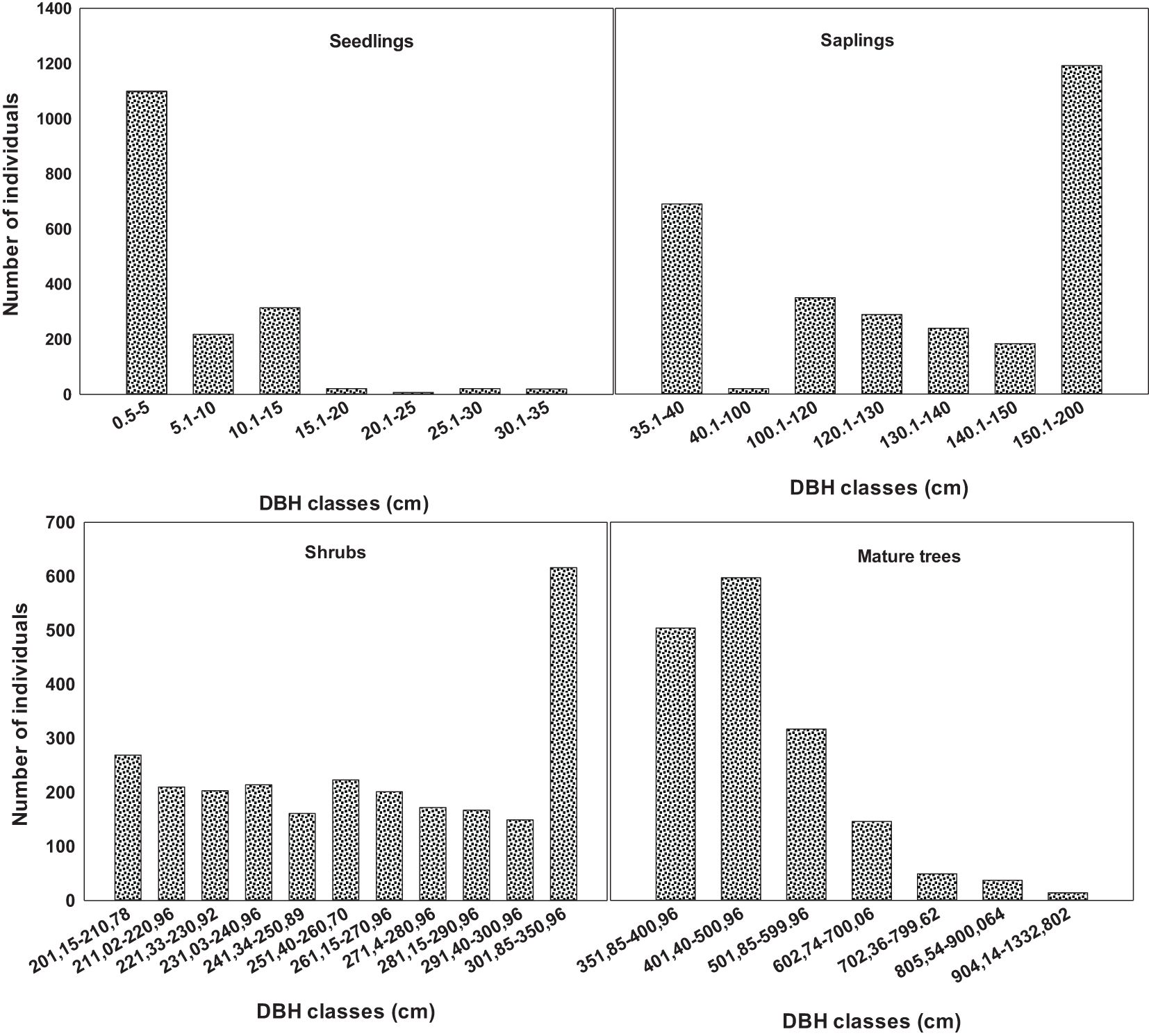
Figure 7. Distribution of individual DBH size classes for woody plant species abundance across various growth stages in the Marikana Thornveld, Pretoria.
4 Discussion
4.1 Woody plant density and changing aspects of seedlings and adult trees
Woody plant density exceeding 2,400 TE ha-1 or having woody plant cover greater than or equal to 40% of the total area is considered bush encroached (Stevens et al., 2017) and has the potential to pose significant environmental risks, particularly to the composition and structure of natural vegetation (Tiawoun et al., 2022). Other research results indicate that an area with a woody plant density exceeding 2,500 TE ha-1 reflects a moderately encroached condition (Richter et al., 2009; Abate et al., 2012). Furthermore, our findings are supported by Gemedo-Dalle et al. (2006), who indicated that an area with a woody plant density exceeding the 2,400 ha-1 threshold is considered bush encroached. In our study, the total density of woody plants across all species and growth stages surpassed 2,400 TE ha-1, indicating that the Marikana Thornveld is significantly encroached upon (O’Connor et al., 2014). The study results indicated a high density of woody plant species, primarily Ehretia rigida at 7,233 plants per hectare, followed by Dichrostachys cinerea with 6,633 plants per hectare and Combretum zeyheri with 6,133 plants per hectare. Therefore, the elevated density values of these woody plant species suggest that their ecological impact in the Marikana Thornveld is significantly problematic. Ayele et al. (2024) reported similar results in Church Forest, documenting a total of 5,923 saplings and 6,136 seedlings per hectare, along with 83 and 66 woody plant species recorded, respectively. The data on species abundance and density varied significantly by growth stage and individual woody plant species. These differences may stem from various factors, including the environmental adaptations of specific species, population pressure, and management practices, such as the underutilization of woody shrub species by browsing animals (Tiawoun et al., 2022a).
Additionally, when we grouped identified woody plant species by their ecological growth stages, our results showed the highest density of woody plants for saplings at 9,978 ha-1, followed by shrub species at 6,256 ha-1, mature trees at 3,322 ha-1, and seedling species at 3,136 ha-1. Consequently, the high abundance of saplings and shrub species clearly indicates that the Marikana Thornveld is in a state of regenerating woody vegetation. Thus, as a component of bush management, applying fire is frequently suggested for controlling bush, particularly in the shrub and sapling layer (Balch et al., 2013). However, for successful burning to control the understory bush layer, at least ≥4,000 kg ha-1 of dry herbaceous material is required to ensure the efficacy of burning (Trollope 2004). Ward (2005) explains the possibilities that lead to occurrences where these changes are realized when resource allocation and certain management practices, such as fire, interact. Furthermore, numerous studies have linked the encroachment of woody plant species to changing fire regimes, overgrazing, soil moisture, nutrients, and global climate change (Ward, 2005; Bond, 2008; van Auken, 2009; Stevens et al., 2016). The prolonged absence of fire promotes woody plant encroachment by providing a secure environment for the development of woody plant seedlings and saplings into fire-resistant growth stages (Trollope, 1980; Bond et al., 2003). Therefore, it is not surprising that our results show a high density of woody plant species, particularly shrubs and saplings. This is due to the fact that prescribed fire applications at our site have been recorded since 2010, resulting in more than 10 years without fire as a control tool for bush encroachment. Additionally, the rapid increase in woody plant species has negative effects that lead to a decline in herbaceous plant species (Angassa and Oba, 2008; Devine et al., 2017) due to competition for available resources among grasses and woody species (Strohbach et al., 2015). Woody plants and herbaceous vegetation coexist; however, once the threshold of 2,500 woody plants per hectare is exceeded, the resulting changes completely suppress grass growth and establishment (Sankaran et al., 2005; Tiawoun et al., 2022b). This creates a hostile environment for herbaceous plants to access water and nutrients in the soil profile (Boldrin et al., 2022).
Both of these reported woody plant species encroachment thresholds (2,400 plants ha-1 and 2,500 plants ha-1) (Gemedo-Dalle et al., 2006) were lower than the density values reported in our research findings. The results for both saplings and shrub species showed high woody density values, which could be due to the fact that woody plants of their height can evade natural disturbances such as trampling and competition (shading, nutrient availability, etc) (Muturi et al., 2013). Our research findings indicate that all the surveyed sites experienced bush encroachment, with several dominant woody species including Ehretia rigida, Dichrostachys cinerea, Gymnosporia buxifolia, Combretum zeyheri, Pappea capensis, Lantana camara, and Vachellia robusta. These findings align with the research conducted by Malan et al. (2021) on wood plant densities, particularly concerning the two most encroaching species: Senegalia mellifera and Dichrostachys cinerea. Consequently, the increase in woody plant density leads to greater complexity in ecosystem functions and a transition from a simple single-stratum to a multiple-stratum ecosystem (Archer, 1998).
4.2 Species abundance in the seedling layer versus adult trees (mature trees, shrubs, and saplings)
The abundance of adult plants represented approximately a quarter or less of the total seedling abundance in the Marikana Thornveld. Seedling abundance showed no positive relationship with adult tree abundance; consequently, adult tree abundance had a negative effect on the number of seedlings per reproductive adult in the Marikana Thornveld. The poor representation of seedlings beneath the adult tree layer can be attributed to seed predation and seedling mortality (Holík and Janík, 2022). The abundance of seedlings and saplings in African savannah ecosystems is the primary factor contributing to bush thickening (Chidumayo, 2013).
4.3 Species diversity and evenness
The species diversity index includes both species richness and the abundance of the identified woody species. The diversity of woody species plays a crucial role in conserving woody plant species as it guides ecological processes, management efforts, and the protection of specific species (Wakjira, 2006). The species diversity in the Marikana Thornveld, Roodeplaat, South Africa, demonstrated a wide range of woody plant species. This veld type includes mature trees, shrubs, saplings, and seedlings. There were differences in the Shannon–Wiener diversity index between mature trees and seedlings, with values of H′ = 4.89 and H′ = 4.75, respectively, and between shrubs and saplings, with H′ = 5.05 and H′ = 5.12, respectively. Lower diversity values were noted for seedlings, possibly due to seed predation by birds and/or insects before germination. Consequently, there is low or no establishment of various new tree species in the seedling layer (Abunie and Dalle, 2018). Moreover, low species diversity in the seedling layer may result from poor seed viability in the soil bank. However, greater woody species diversity was noted in the shrubs and saplings compared to the mature and seedling layers (Wassie et al., 2010). Only shrub species exhibited low species evenness, which was due to a high diversity component (Rutherford and Powrie, 2013) (Table 3).
4.4 Species distribution and vegetation structure
Tree species grow and develop until they reach a stage of reproduction, and as they increase in size, both the DBH classes and woody density also change (Kebede et al., 2016). In all growth stages observed in this study across different density classes, a J-shaped pattern emerged. This pattern can be attributed to several factors, such as anthropogenic activities like logging, senescence, diseases, and fire suppression, particularly affecting adult trees (Tebabala et al., 2024). However, for seedlings and saplings, the J-shaped pattern can be attributed to factors such as trampling, competition for sunlight, and heavy browsing by herbivores. Additionally, regenerating ecosystems can develop varying density-class distributions due to changes in specific characteristics of the secondary ecosystem environment (Yigeremu et al., 2022).
DBH class increased with larger DBH size classes across all woody plants in the four growth stages. This pattern indicates a normal population and a healthy ecosystem environment (Gurmessa et al., 2012). Additionally, Senbeta et al. (2014) reported that this shape demonstrates an ecosystem with a positive potential to regenerate and recruit normally. Therefore, the DBH classes for all woody species across different growth stages suggest that the ecosystem environment still has remnants to support regeneration, even as bush encroachment accelerates.
5 Conclusion and future directions
The study aimed to generate information and understanding of the interaction between mature woody plant species and the understory woody plant layer to create an effective woody plant encroachment control management plan. The results indicated that the density of mature trees and the understory woody plant layer exhibited little to no relationship, confirming that seedling woody plant species were not adequately represented in the adult tree layer (which includes mature trees, shrubs, and saplings). Therefore, the notably high density of saplings and shrubs is entirely due to the re-establishment of existing woody species in our study area. This suggests that mature woody plant species should not be considered a central element of the management plan for controlling the establishment of the understory layer. However, special attention should be given to saplings and shrub species that contribute to woody plant encroachment in the Marikana Thornveld. The decrease in seedling density in relation to adult tree density indicates a negative correlation between the two variables. Thus, adult trees cannot adequately predict seedling recruitment or abundance, nor the end-successional state of the Marikana Thornveld. Saplings and shrubs displayed a high density of woody plant species; consequently, diversity index values were also elevated, reflecting variation among the identified saplings and shrubs. However, the results suggested that the lack of fire application on the existing woody plant species was unnecessary; therefore, fire as a management tool should be used judiciously to control sapling and shrub abundance in the Marikana Thornveld. The J-shaped pattern formed by various DBH classes indicates a common presence of many small-diameter trees (saplings and shrubs) and relatively few large-diameter trees throughout the veld type, which contributes to bush encroachment. Overall, the research results suggest that the understory vegetation layer should be eradicated during bush management control to maintain an open savanna ecosystem.
Data availability statement
The raw data supporting the conclusions of this article will be made available by the authors, without undue reservation.
Author contributions
MM: Conceptualization, Data curation, Formal analysis, Investigation, Methodology, Resources, Software, Validation, Visualization, Writing – original draft, Writing – review & editing. IM: Conceptualization, Formal analysis, Software, Supervision, Validation, Visualization, Writing – review & editing. TT: Conceptualization, Data curation, Formal analysis, Funding acquisition, Project administration, Resources, Supervision, Writing – review & editing. AR: Conceptualization, Data curation, Formal analysis, Software, Supervision, Visualization, Writing – review & editing.
Funding
The author(s) declare that financial support was received for the research and/or publication of this article. This study was supported by Agricultural Research Council Range and Forage (ARC- National Department of Agriculture, grant number API012403000089).
Acknowledgments
The authors are grateful to the Agricultural Research Council for financially supporting this study and a special thanks to the University of Pretoria for availing its resources to the authors to complete the study. A special thanks also goes to Mthunzi Mndela, Nothando Ngcobo, Nchaupa Johannes Rasekgokga, and Piet Monegi for their technical support during the inception and data collection of the study.
Conflict of interest
The authors declare that the research was conducted in the absence of any commercial or financial relationships that could be construed as a potential conflict of interest.
Publisher’s note
All claims expressed in this article are solely those of the authors and do not necessarily represent those of their affiliated organizations, or those of the publisher, the editors and the reviewers. Any product that may be evaluated in this article, or claim that may be made by its manufacturer, is not guaranteed or endorsed by the publisher.
References
Abate T., Ebro A., Nigatu L. (2012). Evaluation of woody vegetation in the rangeland of Southeast Ethiopia. Int. Res. J. Agric. Sci. Soil Sci. 2, 113–126.
Abunie A. A., Dalle G. (2018). Woody species diversity, structure, and regeneration status of Yemrehane Kirstos Church Forest of Lasta Woreda, North Wollo Zone, Amhara region, Ethiopia. Int. J. For. Res. 1–17, 1–18. doi: 10.1155/2018/5302523
Angassa A., Oba G. (2008). Herder perceptions on impacts of range enclosures, crop farming, fire ban, and bush encroachment on the rangelands of Borana, Southern Ethiopia. Hum. Ecol. 36, 201–215. doi: 10.1007/s10745-007-9156-z
Archer M. (1998). “Social Theory and the Analysis of Society,” in Knowing the Social World. Ed. Williams T. M. (Open University Press, Buckingham).
Archer S. R., Andersen E. M., Predick K. I., Schwinning S., Steidl R. J., Woods S. R. (2017). “Woody plant encroachment: Causes and consequences,” in Rangeland Systems. Ed. Briske D. (Springer, Cham, Switzerland), 25–84.
Ayele A., Seid A., Mekonnen A. B., Wassie W. A., Yemata G., Yihune E., et al. (2024). Woody species diversity, structure, and regeneration status of the church forests in West Gojjam Zone, Northwestern Ethiopia. Trees Forests People 16, 100770. doi: 10.1016/j.tfp.2024.100570
Balch J. K., Tara J. M., Brando P. M., Nepstad D. C., Curran L. M. (2013). Effects of high-frequency understorey fires on woody plant regeneration in southeastern Amazonian forests. Philos. Trans. R. Soc. B: Biol. Sci. 368, 0962–8436. doi: 10.1098/rstb.2012.0157
Belay T. A., Totland Ø., Moe S. R. (2013). Ecosystem responses to woody plant encroachment in a semiarid savanna rangeland. Plant Ecol. 214, 1211–1222. doi: 10.1007/s11258-013-0245-3
Boldrin D., Knappett J. A., Leung A. K., Brown J. L., Loades K. W., Bengough A. G. (2022). Modifying soil properties with herbaceous plants for natural flood risk reduction. Ecol. Eng. 180, 106668. doi: 10.1016/j.ecoleng.2022.106668
Bond W. J. (2008). What limits trees in C4 grasslands and savannas? Annu. Rev. ecol. evol. system. 641-659, 641–659. doi: 10.1146/annurev.ecolsys.39.110707.173411
Bond W. J., Midgley J. J. (2001). Ecology of sprouting in woody plants: the persistence niche. Trends Ecol. Evol. 16, 45–51. doi: 10.1016/S0169-5347(00)02033-4
Bond W. J., Midgley G. F., Woodward F. I. (2003). The importance of low atmospheric CO2 and fire in promoting the spread of grasslands and savannas. Global Change Biol. 9, 973–982. doi: 10.1046/j.1365-2486.2003.00577.x
Chidumayo E. N. (2013). Forest degradation and recovery in a miombo woodland landscape in Zambia: 22 years of observations on permanent sample plots. For. Ecol. Manage. 291, 154–161. doi: 10.1016/j.foreco.2012.11.031
D’Odorico P., Carr J. A., Laio F., Ridolfi L. (2012). Spatial organization and drivers of the virtual water trade: A community-structure analysis. Environ. Res. Lett. 7, 34007. doi: 10.1088/1748-9326/7/3/034007
Dalle G., Maass B. L., Isselstein J. (2006). Encroachment of woody plants and its impact on pastoral livestock production in the © 2013 Proceedings of the 22nd International Grassland Congress Borana lowlands, southern Oromia, Ethiopia. Afr. J. Ecol. 44, 237–246. doi: 10.1111/j.1365-2028.2006.00638.x
Devine A. P., Mcdonald R. A., Quaife T., Maclean I. M. (2017). Determinants of woody encroachment and cover in African savannas. Oecologia 183, 939–951. doi: 10.1007/s00442-017-3807-6
Ellis E., Pascual C. U., Mertz O. (2019). Ecosystem services and nature’s contribution to people: negotiating diverse values and trade-offs in land systems. Curr. Opin. Environ. Sustain. 38, 86–94. doi: 10.1016/j.cosust.2019.05.001
García Criado M., Myers-Smith I. H., Bjorkman A. D., Lehmann C. E., Stevens N. (2020). Woody plant encroachment intensifies under climate change across tundra and savanna biomes. Global Ecol. Biogeogr. 29, 925–943. doi: 10.1111/geb.13072
Gemedo-Dalle T., Maass B. L., Isselstein J. (2006). Rangeland condition and trend in the semi-arid Borana lowlands, southern Oromia, Ethiopia. Afr. J. Range Forage Sci. 23, 49–58. doi: 10.2989/10220110609485886
Gurmessa F., Soromessa T., Kelbessa E. (2012). Structure and regeneration status of komto afromontane moist forest, east wollega zone, west ethiopia. J. Res. 23, 205–216.
Hare M. L., Xu X., Wang Y., Gedda A. I. (2020). The effects of bush control methods on encroaching woody plants in terms of die-off and survival in Borana rangelands, southern Ethiopia. Pastoralism 10, 16. doi: 10.1186/s13570-020-00171-4
Higgins S. I., Scheiter S. (2012). Atmospheric CO2 forces abrupt vegetation shifts locally, but not globally. Nature 488, 209–212. doi: 10.1038/nature11238
Holdrege M. C., Beard K. H., Kulmatiski A. (2021). Woody plant growth increases with precipitation intensity in a cold semiarid system. Ecology 102, e03212. doi: 10.1002/ecy.3212
Holík J., Janík D. (2022). Seed and seedling predation by vertebrates mediates the effects of adult trees in two temperate tree species. Oecologia 199, 625–636. doi: 10.1007/s00442-022-05203-x
Hudak A. T., Wessman C. A. (1998). “Textural analysis of aerial photography to characterize large-scalel and cover change,” in Proceedings, 1997 ESRI Users Conference, SanDiego.
Jindo K., Kozan O., Iseki K., Maestrini B., van Evert F. K., Wubengeda Y., et al. (2021). Potential utilization of satellite remote sensing for field-based agricultural studies chem. Biol. Technol. Agric. 8, 58. doi: 10.1186/s40538-021-00253-4
Kebede B., Soromessa T., Kelbessa E. (2016). Floristic composition and community types of Gedo dry evergreen montane forest, West Shewa, Ethiopia. Acta Ecol. Sin. 36, 392–400. doi: 10.1016/j.chnaes.2016.07.001
Kellner K. F., Parsons A. W., Kays R., Millspaugh J. J., Rota C. T. (2022). A two-species occupancy model with a continuous-time detection process reveals spatial and temporal interactions. J. Agricult Biol. Environ. Stat. 321–338. doi: 10.1007/s13253-021-00482
Kent M. (2011). Vegetation description and data analysis: a practical approach (2nd ed.) (John Wiley & Sons).
Kgosikoma O. E., Mogotsi K. (2013). Understanding the causes of bush encroachment in Africa: The key to effective management of savanna grasslands. Trop. Grasslands-Forrajes Tropic. 1, 215–220. doi: 10.17138/TGFT(1)215-219
Kimaro H. S., Asenga A. M., Linus Munishi L., Treydt A. C. (2019). Woody encroachment extent and its associated impacts on plant and herbivore species occurrence in maswa game reserve, Tanzania. Environ. Natural Resour. Res. 9, 1927–0488. doi: 10.5539/enrr.v9n3p63
Kraaij T., Ward D. (2006). Effects of rain, nitrogen, fire, and grazing on tree recruitment and early survival in bush-encroached savanna, South Africa. Plant Ecol. 186, 235–246. doi: 10.1007/s11258-006-9125-4
Lunt I. D., Winsemius L. M., McDonald S. P., Morgan J. W., Dehaan R. L. (2010). How widespread is woody plant encroachment in temperate Australia? Changes in woody vegetation cover in lowland woodland and coastal ecosystems in Victoria from 1989 to 2005. J. Biogeogr. 37, 722–732. doi: 10.1111/j.1365-2699.2009.02255.x
Malan P. W., Tiawoun M. A. P., Molatlhegi K. S., Materechera S. A. (2021). Effect of encroaching woody plant species on soil nutrients and selected soil chemical properties in communally managed semiarid savanna grazing lands in the North-West province, South Africa. South Afr. J. Plant Soil Environ. 38, 27–35. doi: 10.1080/02571862.2020.1829117
Maphanga T., Dube T., Shoko C., Sibanda M. (2022). Advancements in the satellite sensing of the impacts of climate and variability on bush encroachment in savannah rangelands. Remote Sens. Applic.: Soc. Environ. 25, 100689. doi: 10.1016/j.rsase.2021.100689
Moncrieff G. R., Scheiter S., Bond W. J., Higgins S. I. (2014). Increasing atmospheric CO2 overrides the historical legacy of multiple stable biome states in Africa. New Phytol. 201, 908–915. doi: 10.1111/nph.12551
Mucina L., Rutherford M. (2006). Vegetation map of South Africa, Lesotho, and Swaziland. Strelitzia 19 (Pretoria: South African National Biodiversity Institute).
Mussa M., Ebro A., Nigatu L. (2016). Impact of woody plant species on soil physicochemical properties along grazing gradients in rangelands of eastern Ethiopia. Trop. Subtrop. Agroecosys. 19, 343–355. doi: 10.56369/tsaes.2254
Mutanga O., Dube T., Ahmed F. (2016). Progress in remote sensing: vegetation monitoring in South Africa. Geograph. J. 98, 461–471. doi: 10.1080/03736245.2016.1208586
Muturi G. M., Poorter L., Mohren G. M. J., Kigomo B. N. (2013). Ecological impacts of Prosopis species invasion in Turkwel riverine forest, Kenya. J. Arid Environ. 92, 89–97. doi: 10.1016/j.jaridenv.2013.01.010
O’Connor T. G., Chamane S. C. (2012). Bush clump succession in grassland in the Kei Road region of the Eastern Cape, South Africa. Afr. J. Range Forage Sci. 29, 133–146. doi: 10.2989/10220119.2012.744776
O’Connor T. G., Puttick J. R., Hoffman M. T. (2014). Bush encroachment in southern Africa: changes and causes. Afr. J. Range Forage Sci. 31, 67–88. doi: 10.2989/10220119.2014.939996
Oba G., Post E., Syvertsen P. O., Stenseth N. C. (2000). Bush cover and range condition assessments in relation to landscape and grazing in southern Ethiopia. Landscape Ecol. 15, 535–546. doi: 10.1023/A:1008106625096
Olszewski T. D. (2004). A unified mathematical framework for the measurement of richness and evenness within and among multiple communities. Oikos 104, 377–387. doi: 10.1111/j.0030-1299.2004.12519.x
Ratajczak Z., Nippert J., Hartman J., Ocheltree T. (2011). Positive feedback amplifies rates of woody encroachment in mesic tallgrass prairie. Ecosphere 2, 121. doi: 10.1890/ES11-00212.1
Richter C. G. F., Snyman H. A., Smit G. N. (2009). The influence of tree density on the grass layer of three semi-arid savanna types of southern Africa. Afr. J. Range Forage Sci. 9, 103–109. doi: 10.2989/10220110109485762
Ripley B. S., Raubenheimer S. L., Perumal L., Anderson M., Mostert E., Kgope B. S., et al. (2022). CO2 fertilization enhances resilience to browsing in the recruitment phase of an encroaching savanna tree. Funct. Ecol. 36, 3223–3233. doi: 10.1111/1365-2435.14215
Rutherford M. C., Powrie L. W. (2012). Impacts of high utilization pressure on biodiversity components in Colophospermum mopane savanna. Afr. J. Range Forage Sci. 29, 1–11. doi: 10.2989/10220119.2012.687039
Rutherford M. C., Powrie L. W. (2013). Impacts of heavy grazing on plant species richness: A comparison across rangeland biomes of South Africa. South Afr. J. Bot. 87, 146–156. doi: 10.1016/j.sajb.2013.03.020
Sala O. E., Maestre F. T. (2014). Grass–woodland transitions: determinants and consequences for ecosystem functioning and provisioning of services. J. Ecol. 102, 1357–1362. doi: 10.1111/1365-2745.12326
Sankaran M., Hanan N. P., Scholes R. J., Ratnam J., Augustine D. J., Cade B. S., et al. (2005). Determinants of woody cover in African savannas. Nature 438, 846–849. doi: 10.1038/nature04070
Sankaran M., Ratnam J., Hanan N. (2008). Woody cover in African savannas: the role of resources, fire, and herbivory, Glob. Ecol. biogeogr. 17, 236–245. doi: 10.1111/j.1466-8238.2007.00360.x
Senbeta F., Schmitt C., Woldemariam T., Boehmer H. J., Denich M. (2014). Plant diversity, vegetation structure and the relationship between plant communities and environmental variables in the Afromontane forests of Ethiopia. SINET 37, 113–130.
Shannon C. E., Wiener W. (1963). The mathematical theory of communication (Urbana: University of Illinois Press).
Stevens N., Erasmus B. F. N., Archibald S., Bond W. J. (2016). Woody encroachment over 70 years in South African savannahs: overgrazing, global change or extinction aftershock? Philos. Trans. R. Soc. 371, 20150437. doi: 10.1098/rstb.2015.0437
Stevens N., Lehmann C. E. R., Murphy B. P., Durigan G. (2017). Savanna woody encroachment is widespread across three continents. Global Change Biol. 23, 235–244. doi: 10.1111/gcb.13409
Strohbach M. W., Kohler M. L., Dauber J., Klimek S. (2015). High nature value farming: from indication to conservation. Ecol. Indic. 57, 557–563. doi: 10.1016/j.ecolind.2015.05.021
Tebabala W. M., Kassa G. M., Anteneh E. A. (2024). Structural patterns and regeneration status of woody plant species in the forest of Northwest Ethiopia. Sustain. Environ. 10. doi: 10.1080/27658511.2024.2345439
Tiawoun M. A. P., Malan P. W., Comole A. A. (2022a). Effects of soil properties on the distribution of woody plants in communally managed rangelands in Ngaka Modiri Molema District, North-West Province, South Africa. Ecologies 3, 361–375. doi: 10.3390/ecologies3030027
Tiawoun M. A. P., Malan P. W., Comolli A. A. (2022b). Composition and structural patterns of encroaching woody plant species along riparian zones of the Molopo River, North-West Province, South Africa. Alvino Abraham Comole. South Afr. J. Bot. 147, 652–658. doi: 10.1016/j.sajb.2022.02.039
Trollope W. (1980). Controlling bush encroachment with fire in the savanna areas of South Africa. Proc. Annu. Congress. Grassland Soc. South. Afr. 15, 173–177. doi: 10.1080/00725560.1980.9648907
Trollope W. S. W. (2004). “Prescribed burning in african grasslands and savannas for domestic livestock systems,” (Grassroots: Newsletter of the Grassland Society of southern Africa).
van Auken O. (2009). Causes and consequences of woody plant encroachment into western North American grasslands. J. Environ. Manage. 90, 2931–2942. doi: 10.1016/j.jenvman.2009.04.023
van der Maarel E. (1979). Transformation of cover-abundance values in phytosociology and its effects on community. Vegetation 39, 97–114. doi: 10.1007/BF00052021
van Rooyen N. (1983). A homogeneity index based on species diversity in Sour Bushveld. Bothalia 14, 299–301. doi: 10.4102/abc.v14i2.1174
Wakjira F. S. (2006). Biodiversity and ecology of Afromontane rainforests with wild Coffea arabica L. population in Ethiopia (. Germany: Cuvillier Verlag Göttingen). Center for Development Research, University of Bonn. Ecology and Development Series No. 38.
Ward D. (2005). Do we understand the causes of bush encroachment in African savannas? Afr. J. Range Forage Sci. 101–105. doi: 10.2989/10220110509485867
Wassie A., Sterck F. J., Bongers F. (2010). Species and structural diversity of church forests in a fragmented ethiopian highland landscape. J. Veg. Sci. 21, 938e948.
Yigeremu A., Woldearegay M., Mansour A. (2022). Woody species diversity, composition, and regeneration status of Abbo Sacred Forest, Southern Ethiopia. Sci. World J. 1–9, 1–10. doi: 10.1155/2022/9112578
Zhang J., Yang C., Song H., Hoffmann W. C., Zhang D., Zhang G. (2016). Evaluation of an airborne remote sensing platform consisting of two consumer-grade cameras for crop identification. Remote Sens. 8, 257. doi: 10.3390/rs8030257
Keywords: species density, tree growth stages, vegetation structure, patterns of woody species, diversity indices
Citation: Mangwane M, Madakadze IC, Tjelele TJ and Ramoelo A (2025) Patterns of mature woody plant species encroachment on vegetation structure, density, and diversity of the understory layer across the Marikana Thornveld. Front. Ecol. Evol. 13:1498429. doi: 10.3389/fevo.2025.1498429
Received: 18 September 2024; Accepted: 31 March 2025;
Published: 06 May 2025.
Edited by:
Leonardo Montagnani, Free University of Bozen-Bolzano, ItalyReviewed by:
Dongjie Zhang, Shandong University of Aeronautics, ChinaZerihun Kebebew, Jimma University, Ethiopia
Teshome Beza, Hawassa University, Ethiopia
Copyright © 2025 Mangwane, Madakadze, Tjelele and Ramoelo. This is an open-access article distributed under the terms of the Creative Commons Attribution License (CC BY). The use, distribution or reproduction in other forums is permitted, provided the original author(s) and the copyright owner(s) are credited and that the original publication in this journal is cited, in accordance with accepted academic practice. No use, distribution or reproduction is permitted which does not comply with these terms.
*Correspondence: Mziwanda Mangwane, dTE0Mzg3MDI3QHR1a3MuY28uemE=