- 1Department of Dermatology, The Second Affiliated Hospital of Xinjiang Medical University, Urumqi, China
- 2Department of Dermatology, Xiangya Hospital, Central South University, Changsha, China
- 3Department of Neurology, Second Affiliated Hospital of Xinjiang Medical University, Urumqi, China
- 4Hunan Key Laboratory of Aging Biology, Xiangya Hospital, Central South University, Changsha, China
- 5National Clinical Research Center for Geriatric Disorders, Xiangya Hospital, Central South University, Changsha, China
Rosacea is significantly associated with dementia, particularly Alzheimer’s disease (AD). However, the common underlying molecular mechanism connecting these two diseases remains limited. This study aimed to reveal the common molecular regulatory networks and identify the potential therapeutic drugs for rosacea and AD. There were 747 overlapped DEGs (ol-DEGs) that were detected in AD and rosacea, enriched in inflammation-, metabolism-, and apoptosis-related pathways. Using the TF regulatory network analysis, 37 common TFs and target genes were identified as hub genes. They were used to predict the therapeutic drugs for rosacea and AD using the DGIdb/CMap database. Among the 113 predicted drugs, melatonin (MLT) was co-associated with both RORA and IFN-γ in AD and rosacea. Subsequently, network pharmacology analysis identified 19 pharmacological targets of MLT and demonstrated that MLT could help in treating AD/rosacea partly by modulating inflammatory and vascular signaling pathways. Finally, we verified the therapeutic role and mechanism of MLT on rosacea in vivo and in vitro. We found that MLT treatment significantly improved rosacea-like skin lesion by reducing keratinocyte-mediated inflammatory cytokine secretion and repressing the migration of HUVEC cells. In conclusion, this study contributes to common pathologies shared by rosacea and AD and identified MLT as an effective treatment strategy for rosacea and AD via regulating inflammation and angiogenesis.
Introduction
Rosacea is a chronic inflammatory skin disease with a global prevalence of over 5% (1). It is clinically characterized by erythema, telangiectasia, papules, or pustules and could be triggered or exacerbated by spicy food and beverages and by physical and psychological stimuli (2). Although immune and neurovascular dysregulation was reported to play an essential role in rosacea, the exact pathogenesis of rosacea remains unclear (3). Due to the lack of effective treatments, rosacea remains an incurable disease for the vast majority of patients (4).
Accumulating studies showed that rosacea develops as a manifestation of systemic illnesses that are linked to metabolic, psychiatric, and neurologic disorders, including Alzheimer’s disease (AD) (5, 6). Thyssen et al. clinically observed an increased risk of AD in patients with rosacea (6). AD is a common neurodegenerative disease, characterized by a progressive deterioration in cognitive and memory abilities, which has become a growing threat to public health (7). Studies showed that dysregulation of the inflammatory system is an essential factor to cognitive impairment in AD (8). Although chronic inflammation and vascular dysfunction are shared in the pathogenesis of both rosacea and AD (9, 10), the detailed molecular mechanism linking AD and rosacea remains limited. The common pathologies shared by rosacea and AD through molecular interaction networks may contribute to the drug discovery for rosacea treatment and AD prevention.
Melatonin (MLT), a neurohormone produced by the pineal gland, has multiple regulatory roles in circadian rhythms, sleep, and neuroendocrine activity (11, 12). In recent years, MLT has been implicated in immunomodulatory, antiangiogenic, and antioxidant activities (13, 14). MLT deficiency could increase the risk of neurodegeneration, immunoregulation disorder, and senescence (15–17). MLT levels have previously been reported to be lower in patients with AD (18, 19), which may contribute to oxidative damage of brain cells of AD patients (20, 21). Beyond that, MLT was reported as an effective treatment for AD, but the mechanisms by which MLT benefits AD are ill-defined. Interestingly, MLT is also widely applied in treating cutaneous diseases, such as atopic dermatitis (22), androgenic alopecia (23), and vitiligo (22). However, the potential therapeutic role of MLT on rosacea and its detailed pharmacological mechanisms still need to be studied.
In the present study, bioinformatics analyses revealed the biological functions, transcription factor (TF) regulatory network, and core targets in both AD and rosacea. Moreover, the network pharmacology approach identified the MLT pharmacological target network and revealed the mechanism that MLT could help in treating AD/rosacea partly by modulating inflammatory and vascular signaling pathways. Finally, the therapeutic role and mechanism of MLT were verified in rosacea.
Materials and Methods
Differentially Expressed Genes in AD and Rosacea
As shown in Figure 1, the data were downloaded from the GEO database (https://www.ncbi.nlm.nih.gov/geo/): 23 tissues (10 hippocampus tissues with AD and 13 control hippocampus tissues) in GSE5281 (GPL570 platform) and 30 tissues (22 hippocampus tissues with AD and 8 control hippocampus tissues) in GSE28146 (GPL570 platform). The 38 rosacea tissues and 20 control tissues in GSE65914 were also downloaded from GEO. The data were standardized and the batch effects were removed using “limma” and “sva” (Figures S1A, S2A). Differentially expressed genes (DEGs) between the disease and control tissues were identified using the R “limma” package with the threshold of |logFC| >0.5 and FDR <0.05.
Gene Ontology and Kyoto Encyclopedia of Genes and Genomes Enrichment Analyses
The Gene Ontology (GO) and Kyoto Encyclopedia of Genes and Genomes (KEGG) enrichment analyses were carried out using R “clusterProfiler”, “enrichplot”, and “ggplot2” packages.
The Protein–Protein Interaction Networks
The protein–protein interaction (PPI) network was constructed using the STRING database (https://string-db.org/) with a combined score >0.7. “cytoHubba” was used to calculate the weight of each gene and explore the hub genes of the PPI network, and “MCODE” was used to identify the representative modules using Cytoscape software 3.8.1 (https://cytoscape.org).
Transcription Factor–Target Networks
The TF–targets were downloaded from the TRRUST database (https://www.grnpedia.org/trrust/). The differently expressed TFs in AD and rosacea, which could regulate DEGs in AD/rosacea, were identified as key TFs. The differently expressed TFs and target genes were used to construct the TF–target networks using Cytoscape software.
DGIbd and Connectivity Map Were Used for Drug Prediction
To prioritize the list of drugs for AD/rosacea, key TF and target genes were used for potential candidate drugs using the DGIbd database (24, 25), and then the Connectivity Map (CMap) database was also used to verify the candidate drugs for AD and rosacea (26).
Prediction of the MLT Pharmacological Target
The potential pharmacological targets of MLT were obtained from accessible online tools, including the Traditional Chinese Medicine Systems Pharmacology Database and Analysis Platform (TCMSP), SwissTargetPrediction, and TargetNet (27). The candidate genes were corrected and identified using the UniProt database (28).
Molecular Docking
The molecular structure of MLT (CID_4091) was downloaded from the PubChem database (https://pubchem.ncbi.nlm.nih.gov/). The protein structures of MMP9_6ESM were downloaded from the PDB database (https://www.rcsb.org/). The maestro software was used for molecular docking analysis as previously described.
Reagents
The synthetic peptide LL37 (LLGDFFRKSKEKIGKEFKRIVQRIKDFLRNLVPRTES) used in this study was purchased from Sangon Biotech (Shanghai, China). All peptides were HPLC purified (purity >95%) and characterized by mass spectrometry. Melatonin was purchased from Sigma Company (USA). Human recombinant human TNF-α was purchased from PeproTech (USA).
Mouse Experiment
Specific pathogen-free female BALB/c mice (6 to 7 weeks old; weight, 20–25 g) were purchased from SLAC Laboratory Animal Co., Ltd. (Shanghai, China). Before the experiment, all the animals were acclimated to experimental conditions for 1 week. The mouse model of rosacea was constructed as described before (29). All the 8-week-old BALB/c mice (7 to 8 weeks old, weight, 20–25g) were divided into four groups (control group, LL37 group, MLT group, LL37 + MLT group) randomly. Mice were sacrificed 12 h after the final injection, and mice dorsal lesion skins were collected. The inflammation severity of the rosacea mice model was evaluated according to the area of skin lesions, erythema, and thickness (30), and the skin tissues were divided into three parts for RNA extraction, HE staining, and immunofluorescence staining, respectively. Once the experiments were not to be carried out immediately, tissues were stored at −80°C until use. All mice were housed in sterile conditions at 23°C ± 2°C (12 h light/dark). All experimental protocols followed the guidelines of animal experimentation and were approved by the Ethical Committee of Xiangya Hospital of Central South University (Approval No. 201611610).
Histological Analysis
Fresh mouse dorsal skin tissues were fixed in 4% paraformaldehyde (PFA), embedded in paraffin, and sectioned at 6 µm. Subsequently, hematoxylin and eosin (H&E) staining was performed on paraffin sections for observation under a light microscope (Olympus, Japan), and the number of dermis-infiltrating cells was then averaged and assessed statistically.
Cell Culture and Treatment
Human immortalized keratinocyte (HaCaT) cells were cultured in complete calcium-free DMEM media (Gibco, USA) containing 10% fetal bovine serum (FBS) (Gibco, USA), 1% L-glutamine (Gibco, USA), and 1% penicillin/streptomycin (Gibco, USA), at 37°C in 5% CO2 in an incubator. When HaCaT cells reached 70% confluence (logarithmic phase HaCaT cells were inoculated into a 24-well plate with cell-climbing slices at the concentration of 1 × 104/well, if HaCaT cells were prepared for immunofluorescence experiments), the culture medium was replaced with serum-free calcium-containing DMEM medium. After HaCaT cells were starved for 12 h, they were then treated with melatonin (1 mM) for 24 h and stimulated with 8 μM LL37 or 100 ng/ml TNF-α for 12 h, and then RNA extraction or immunofluorescence was performed. For the immunoblot experiments, after HaCaT cells reached 70% confluence, they were then treated with melatonin (1 mM) for 6 h and then stimulated with TNF-α (100 ng/ml) for 15 min, and then the cell protein was collected (the other conditions were the same as above).
Human umbilical vein endothelial (HUVEC) cells were cultured in complete RPMI 1640 medium (Thermo Fisher Scientific, USA) containing 10% FBS (Gibco, USA) and 1% penicillin/streptomycin (Gibco, USA) in an incubator (37°C, 5% CO2). For the detailed research methods of chemotaxis and migration assay, refer to the following specific section.
RNA Extraction, Reverse Transcription, and RT-PCR
Total RNA in cells and tissue samples was extracted using the standard RNA extraction method with TRIzol (Invitrogen Life Technologies, USA). After a NanoDrop spectrophotometer determined RNA concentration, reverse transcription was performed on 2 μg RNA, using the Maxima H Minus First-Strand cDNA Synthesis Kit with dsDNase (Thermo Scientific, K1682, USA) to synthesize the cDNA strand, and gene expression was analyzed using an Applied Biosystems ® 7500 machine (Life Technologies, USA) with the qPCR SYBR Green Master Mix (Vazyme Biotech Co., Ltd., Nanjing, China). The primers are shown in Table S11.
Immunofluorescence
Fresh mouse dorsal skin tissues were embedded in optimal cutting temperature compound and 6- to 8-μm frozen sections were cut. Then, the frozen tissues or HaCaT cells were fixed in 4% PFA for 15 min. Afterwards, the samples were permeabilized and blocked using blocking buffer (0.2% Triton-X/5% donkey serum) for 1 h. Next, the skin sections were incubated with different antibodies: rat anti-mouse CD4 antibody (1:100), rat anti-mouse CD31 antibody (1:100), and rat anti-mouse F4/80 antibody (1:100), and these antibodies were purchased from eBioscience. HaCaT cells were incubated with rabbit anti-phospho-NF-kB p65 antibody (1:200, Cell Signaling, USA) at 4°C overnight. Anti-rat Alexa 488 (1:200; Invitrogen, USA) and anti-rabbit Alexa 594 (1:200; Invitrogen, USA) were used as the secondary antibody for staining samples 1 h at room temperature. Finally, all samples were stained with 4′,6-diamidino-2-phenylindole (DAPI) for 5 min to visualize nuclei. Fluorescence images were acquired under a fluorescence microscope (Zeiss, Germany).
Western Blot Assays
Proteins of cells were extracted with RIPA lysis buffer containing protease and phosphatase inhibitor cocktail. Then, the protein concentration was measured by BCA protein assay kits (Thermo Fisher Scientific, USA). Twenty micrograms of total protein was resolved on 10% SDS polyacrylamide gels and transferred onto a polyvinylidene fluoride (PVDF) membrane. After transferring, membranes were blocked with 5% non-fat milk and then the PVDF was incubated with the primary antibodies (rabbit anti-p65, 1:1,000; rabbit anti-phospho-p65, 1:1,000; and rabbit anti-GAPDH, 1:5,000) at 4°C overnight. After washing with TBST, the membranes were incubated with secondary horseradish peroxidase-conjugated antibodies (1:5,000, room temperature for 1 h). Finally, the immunoreactive bands were visualized using a chemiluminescent HRP substrate (Millipore, USA) and imaged using a ChemiDocTM XRS+ System (Bio-Rad, CA, USA). GAPDH expression was used as the endogenous control.
HUVEC Cell Chemotaxis Assay
HUVEC chemotaxis was performed with transwell chambers (8 μm, Millipore, Billerica, MA, USA). In a 24-well culture plate, after being treated with 1 mM MLT or equal volumes of the vehicle solution for 24 h, HUVEC cells were seeded into each upper chamber at a density of 2 × 104 cells with 100 μl serum-free RPMI 1640 medium. Then, the plate was placed in an incubator (37°C, 5% CO2). Non-migratory cells on the upper surface of the membrane were wiped off gently with a cotton swab after the following 24 h. Afterwards, the chamber was fixed with 4% paraformaldehyde for 15 min, and 0.1% crystal violet solution was used to stain the cells on the lower layer for 30 min. Finally, the number of invaded cells was counted under a light microscope (Olympus, Japan).
HUVEC Cell Migration Assay
The HUVEC suspension with a 6 × 105/ml density was inoculated on a six-well plate at 2 ml per well. At a confluency of 95%, the 200-μl pipetting tip was drawn vertically on the culture plate, and the non-adherent cells were rinsed with PBS three times. Then, the images were captured by using an optical microscope (Olympus, Japan) to determine the distance of scratch for 0 h. Following HUVEC incubation with RPMI 1640 medium with 3% FBS co-incubated with 1 mM melatonin or its vehicle in an incubator at 37°C for 24 h, photographs were retaken under the same conditions. The measurement tool of Image-Pro Plus software was used to measure the distance of the cell scratch boundary before and after treatment, and the distance before treatment was subtracted from the distance after treatment, which was the migration distance of the cells at 24 h.
Statistical Analysis
All data were analyzed with SPSS 17.0 statistical software and were presented as means ± SEM. One-way ANOVA analyzed the data between groups, and the LSD method was used for pairwise comparison. *, P < 0.05; **, P < 0.01; and ***, P < 0.001 are considered significant.
Results
The DEGs and PPI network in AD and Rosacea
After integrated bioinformatics analyses for GSE5281 and GSE28146, a total of 5,091 DEGs were identified in AD tissues compared with normal tissues (Figure S1B). The GO analysis of DEGs was mainly related to cellular respiration, ATP metabolic process, and ATP metabolic process (Figure S1C and Table S1). Furthermore, KEGG enrichment analysis disclosed that these DEGs were significantly associated with pathways of neurodegeneration-multiple diseases, tight junction, virus infection, and so on (Figure S1D and Table S2).
Next, we constructed the PPI network and used “cytoHubba” to explore the hub genes (Figure S2). The top 5 representative modules were extracted from the PPI network using “MCODE”, and the module genes were enriched in mRNA splicing, clathrin-mediated endocytosis, G2/M transition, translation, G alpha (q) signaling events, and so on (Table 1).
For rosacea, 3,016 DEGs were identified in rosacea tissues compared with normal tissues (Figure S3B). GO enrichment indicated that rosacea was associated with immune-related biological processes (Figure S3C and Table S3). KEGG enrichment results showed immune-related pathways, the PPAR pathway, virus infection, bacterial infections, metabolism-related pathways, and so on (Figure S3D and Table S4). “cytoHubba” was used to explore the hub genes of the PPI network (Figure S4), and the top 5 representative modules were extracted from the PPI network using “MCODE” (Figure S4). The module genes were enriched in G alpha (i) signaling events, interferon signaling, neutrophil degranulation, cornification, neutrophil degranulation, and so on (Table 2).
The Co-Expressed DEGs and Enriched Pathways in Both AD and Rosacea
To reveal the shared regulatory network, 747 overlapped DEGs (ol-DEGs) were detected in AD and rosacea (Figure 2A). GO enrichment analysis showed that the ol-DEGs were related to immune-related processes and lipid metabolic processes (Figure 2B). The KEGG enrichment results showed that ol-DEGs were related to cytokine/chemokine pathways, infectious/inflammatory disease, and metabolism-related pathways (Figure 2C). Consistent with these results, inflammation-, metabolism-, and apoptosis-related pathways were enriched using the Metascape database (Figure 2D). Moreover, the enrichment analysis showed that ol-DEGs were related to inflammation-, vascular-, and infection-related diseases in DisGeNET (Figure 2E). Transcription factor–target analysis showed that many ol-DEGs were regulated by TFs in the TRRUST database (Figure 2F), indicating that the TF regulatory network may play an essential role in the progression of AD and rosacea.
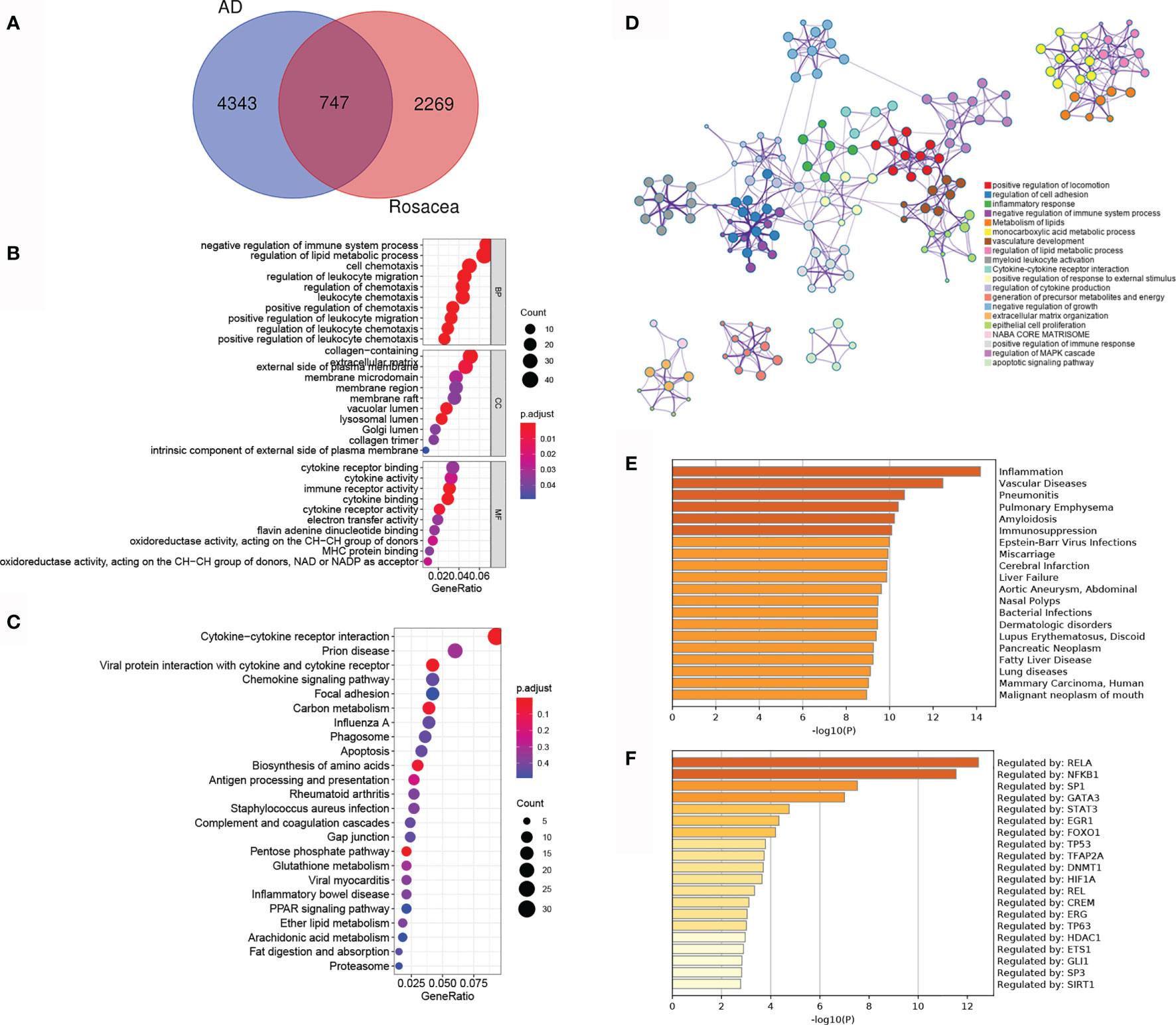
Figure 2 The co-differentially expressed genes (DEGs) in Alzheimer’s disease (AD) and rosacea. (A) The Venn graph of ol-DEGs in both AD and rosacea. (B) GO enrichment analysis of ol-DEGs. (C) The KEGG analysis of co-DEGs. (D) The pathway enrichment analysis of co-DEGs using Metascape. (E) The disease enrichment analysis of ol-DEGs in DisGeNET using Metascape. (F) The transcription factor (TF) enrichment analysis of ol-DEGs in TRRUST using Metascape.
The TF Regulatory Network and Potential Drugs for AD and Rosacea
To further reveal the TF regulatory network in both AD and rosacea, we analyzed the differently expressed TFs with potential targets differently expressed in AD and rosacea. Twenty-four hub TFs are identified in Figure 3A. The TF regulatory network in AD and rosacea is shown in Figure 3B and Table S5. The TFs and targets were enriched in inflammatory-, blood vessel development-, and apoptosis-related signaling pathways (Figure 3C). In Figure 3D, four models were observed to be enriched in atherosclerosis-, IL-17-, proteoglycan-, and inflammatory response-related signaling pathways (Figure 3D and Table 3). These results indicated that inflammatory- and blood vessel-related pathways are crucial during the pathogenesis of AD and rosacea.
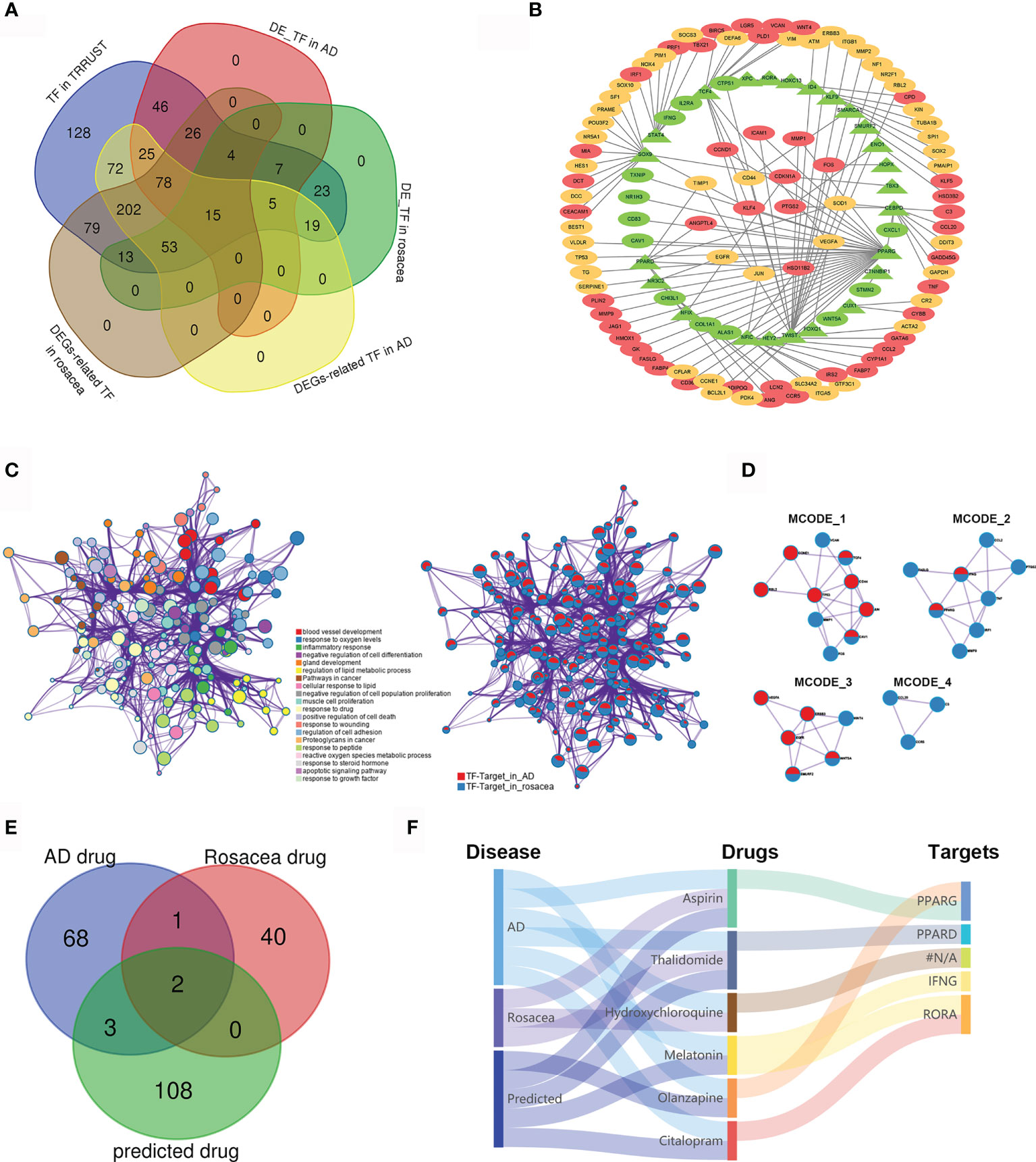
Figure 3 The TF regulatory network and candidate drugs for AD and rosacea. (A) The common TFs in AD and rosacea. (B) The TF regulatory network in AD and rosacea. (C) The enrichment of TF–target genes using the Metascape database. Network of enriched terms colored by cluster ID and the identities of the gene lists. (D) MCODE components identified in TF–targets in rosacea and AD. (E) The Venn diagram revealed the intersection among AD drugs, rosacea drugs, and predicted drugs. (F) The Sankey diagram revealed the correlation between the disease, drugs, and targets.
Next, we analyzed the potential drugs for AD and rosacea. Using the DGIbd database (https://dgidb.org/), 12 of the 37 hub genes were observed as targets of the 113 predicted drugs (Table S6). We compared the 74 drugs for AD, the 44 drugs for rosacea, and the 113 predicted drugs for hub genes. Three drugs (aspirin, thalidomide, and hydroxychloroquine) overlapped in AD and rosacea, and two (aspirin and thalidomide) of these were observed in the predicted drugs (Figure 3E), proving that the candidate drugs for AD/rosacea have high credibility. Moreover, three predicted drugs (MLT, olanzapine, and citalopram) have been used for AD treatment. The Sankey diagram revealed the correlation between disease, drugs, and targets (Figure 3F). These results indicated that the three predicted drugs (MLT, olanzapine, and citalopram) could be effective therapeutic strategies for rosacea. Among these potential drugs, MLT was co-associated both with retinoid-related orphan receptor alpha (RORA) as well as IFN-γ. MLT was predicted as a candidate drug using the CMap database (Table S7). So, MLT was selected for further function assay.
Identifying MLT Targets in AD and Rosacea
Five hundred twenty-nine pharmacological targets of MLT were predicted using the TCMSP, TargetNet, and SwissTargetPrediction databases, and then the repetitive genes were deleted using the UniProt database. An overlap of 128 AD/rosacea TF–targets with MLT pharmacological targets identified 19 intersection genes of MLT against AD/rosacea (Figure 4A and Table S8). GO analyses of the 19 genes indicated that MLT could affect the regulation of inflammatory response, collagen metabolic process, response to oxygen levels, vascular-associated smooth muscle cell proliferation, and so on (Figure 4B and Table S9). Additionally, 51 KEGG pathways were significantly enriched (P-adjusted < 0.05), including the AGE-RAGE signaling pathway in diabetic complications, lipid metabolism and atherosclerosis, NF-kappa B signaling pathway, IL-17 signaling pathway, PPAR signaling pathway, and TNF signaling pathway (Figure 4B and Table S10). Next, the PPI network of the 19 intersection targets was analyzed using STRING (Figure 4C), cytoHubba was used to identify eight core gene targets, namely, CCND1, EGFR, ICAM1, MMP2, MMP9, PTGS2, SERPINE1, and TNF (Figure 4C).
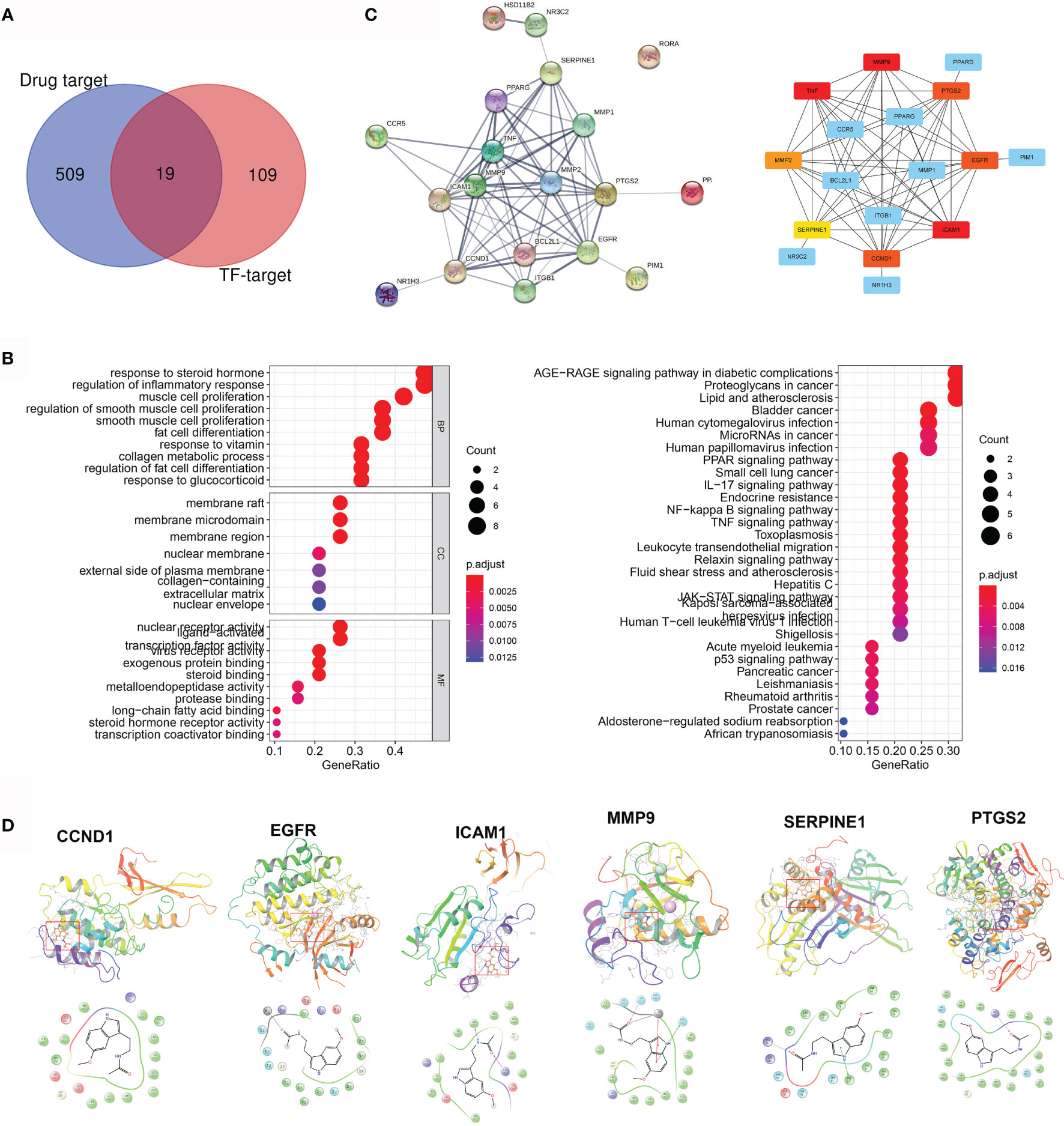
Figure 4 Pharmacological targets of melatonin (MLT) in AD and rosacea. (A) Nineteen intersection genes of MLT against AD/rosacea. (B) GO and KEGG enrichment analysis of the 19 MLT targets. (C) PPI network of the 19 MLT targets. (D) Molecular docking revealed the binding of MLT to its targets.
Next, molecular docking was performed to identify the possible binding between MLT and eight core targets. The result showed that MLT could bind to CCND1, EGFR, ICAM1, MMP9, PTGS2, and SERPINE1 with docking scores of −5.86, −7.16, −6.02, −5.95, −6.52, and −6.81, respectively (Figure 4D).
The Potential MLT Alleviated Rosacea-Like Phenotypes in Mice
To verify the therapeutic effect of MLT on rosacea, rosacea-like mice were treated with MLT for 4 days (29). We found that MLT significantly ameliorated LL37-induced rosacea-like phenotypes (Figure 5A). The redness area, redness score, skin thickness, and inflammatory cell infiltration were dramatically decreased by MLT treatment (Figures 5B–F). Moreover, MLT repressed the expression of proinflammatory cytokines, such as IL-6, TNF-α, TLR2, TGF-β1, MMP9, and VEGF in rosacea-like dermatitis (Figure 5G).
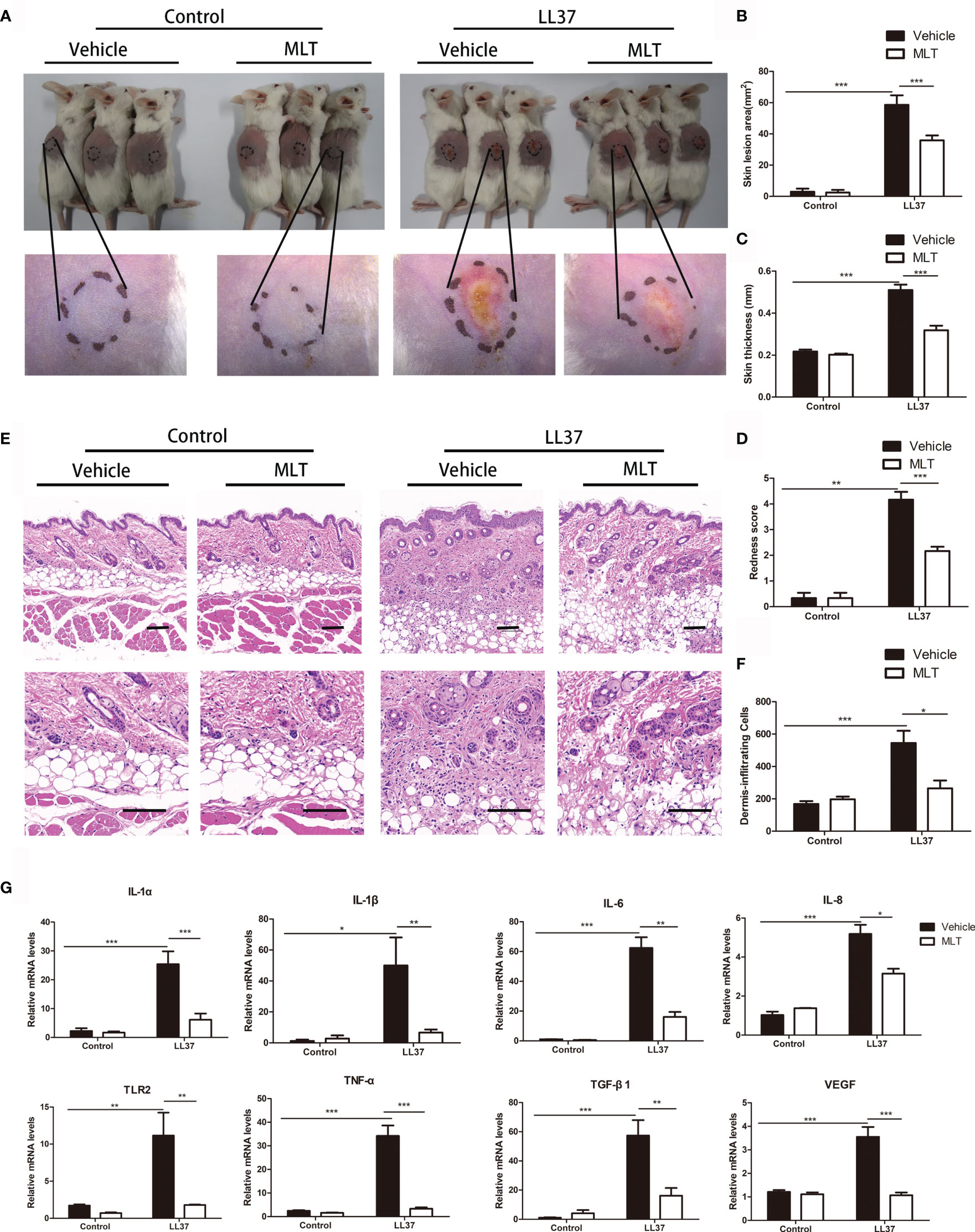
Figure 5 The treatment effects of MLT on rosacea. (A) MLT significantly alleviated rosacea-like phenotype in mice. The effects of MLT on lesion area (B), skin thickness (C), and redness score (D) in rosacea-like mice. (E) H&E staining of rosacea-like lesion. Scale bars: 100 μm. (F) Dermal inflammatory cell infiltration was quantified in rosacea-like mice. (G) The mRNA expression of rosacea-related markers in mice. All results are representative of at least three independent experiments. Data expressed as individual values with mean ± SEM. *P < 0.05, **P < 0.01, ***P < 0.01.
As described in previous studies, the infiltration of CD4+ T cells and Th1/Th17 polarized cells is essential in the pathogenesis of rosacea (31–33). In this study, immunofluorescence and qPCR analysis showed that MLT significantly reduced the infiltration of CD4+ T cells in rosacea-like dermatitis (Figures 6A, B) and suppressed the expression of Th1-related genes (IFN-γ, CCR5, CXCL9, CXCL10) and Th17-related genes (STAT3 and IL-20) (34) in rosacea-like dermatitis (Figure 6C).
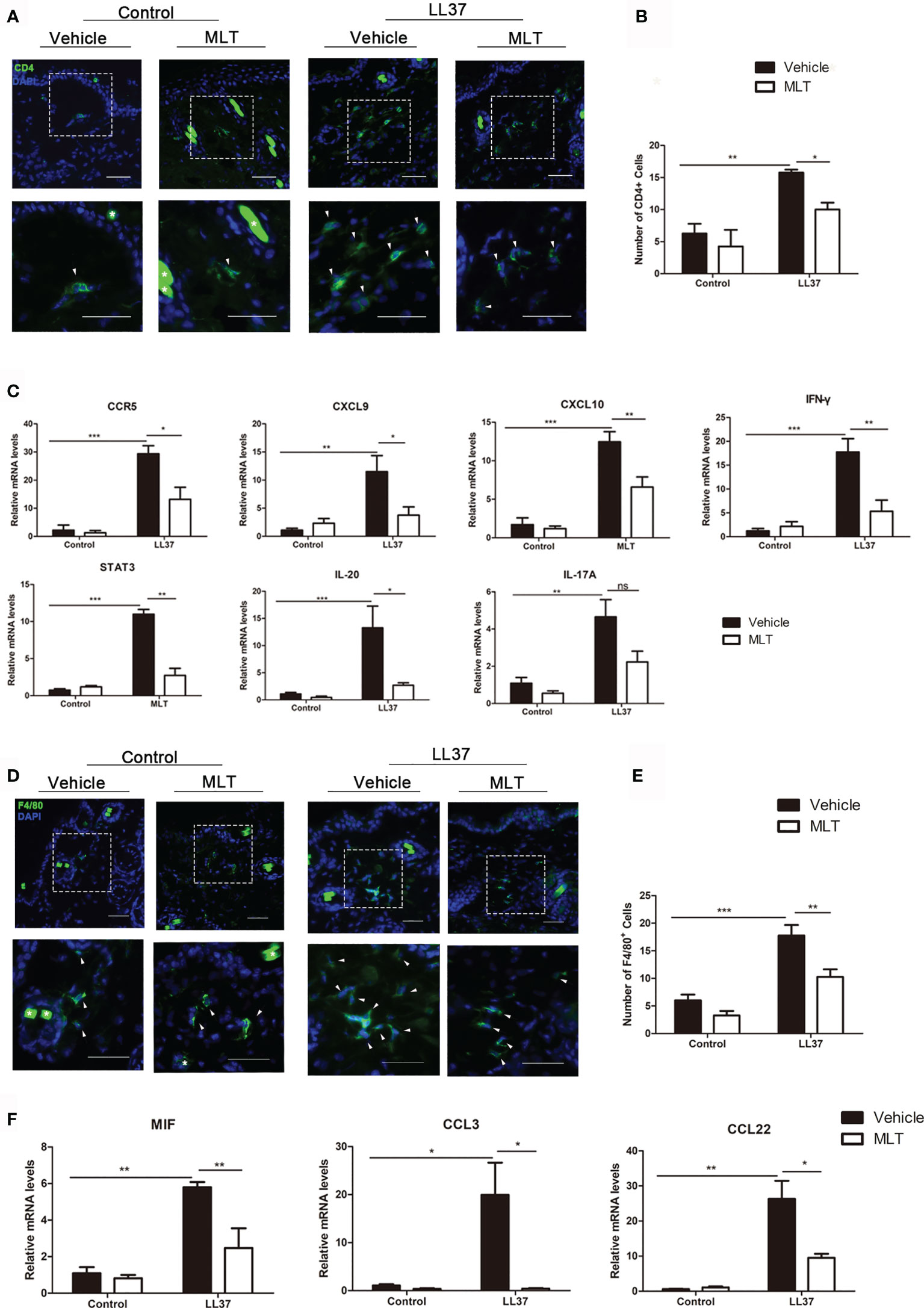
Figure 6 MLT inhibits immune cell infiltration in rosacea-like mice. (A) Immunostaining of CD4+ T cells in rosacea-like mice. Scale bar: 50 μm. (B) The infiltrated CD4+ T cells were quantified. (C) qPCR analysis detected the expression of Th1- and Th17-related genes in rosacea-skin lesions. (D) Immunostaining of macrophages in rosacea-like mice. Scale bar: 50 μm. (E) The infiltration of macrophage cells was quantified in rosacea-like mice. (F) qPCR analysis detected the expression of macrophage-related genes. All results are representative of at least three independent experiments. Data expressed as individual values with mean ± SEM. *P < 0.05, **P < 0.01, ***P < 0.01.
Macrophages have also played an important role in rosacea (35). To investigate whether MLT can inhibit macrophage activity in rosacea-like dermatitis, we detected the infiltration of F4/80+ cells. The number of infiltrated F4/80+ cells was significantly attenuated (Figures 6D, E) and the expression of macrophage-related genes (MIF, CCL3, CCL22, and CCR4) was evidently reduced by MLT treatment in rosacea-like dermatitis (Figure 6F). Collectively, these data suggested that MLT suppressed the immune response in rosacea.
MLT Reduced the Secretion of Inflammatory Factors From Keratinocytes
Studies showed that keratinocyte-mediated secretion of inflammatory chemokines and cytokines plays a pivotal role in the progression of rosacea (29). Here, we explored the effects of MLT on chemokine and cytokine expression in LL37-treated HaCaT cells. The results showed that 1 mM MLT significantly reduced LL37-induced CCL2, CCL20, MMP9, KLK5, IL-6, IL-8 and VEGF-c expression (Figure 7A). What is more, the expression of NF-κB downstream genes (IL-1α and IL-1β) was significantly repressed by MLT. To further assess the precise anti-inflammatory mechanism of MLT, we stimulated the HaCaT cells with TNF-α (100 ng/ml), one of the most potent inducers of NF-κB activation (36). We found that TNF-α induced the mRNA levels of chemokines and cytokines, including CCL2, CCL20, IL-8, KLK5, TGF-β1, TLR2, IL-1α, and IL-1β, which were abrogated by MLT treatment obviously (Figure 7B). Moreover, immunofluorescence results also revealed the inhibition of MLT on TNF-α-induced p65 translocation (Figures 7C, D) and phosphorylation of p65/NF-κB (Figure 7E). Collectively, these data suggest that MLT ameliorated HaCaT-mediated inflammation partly by modulating NF-κB signaling.
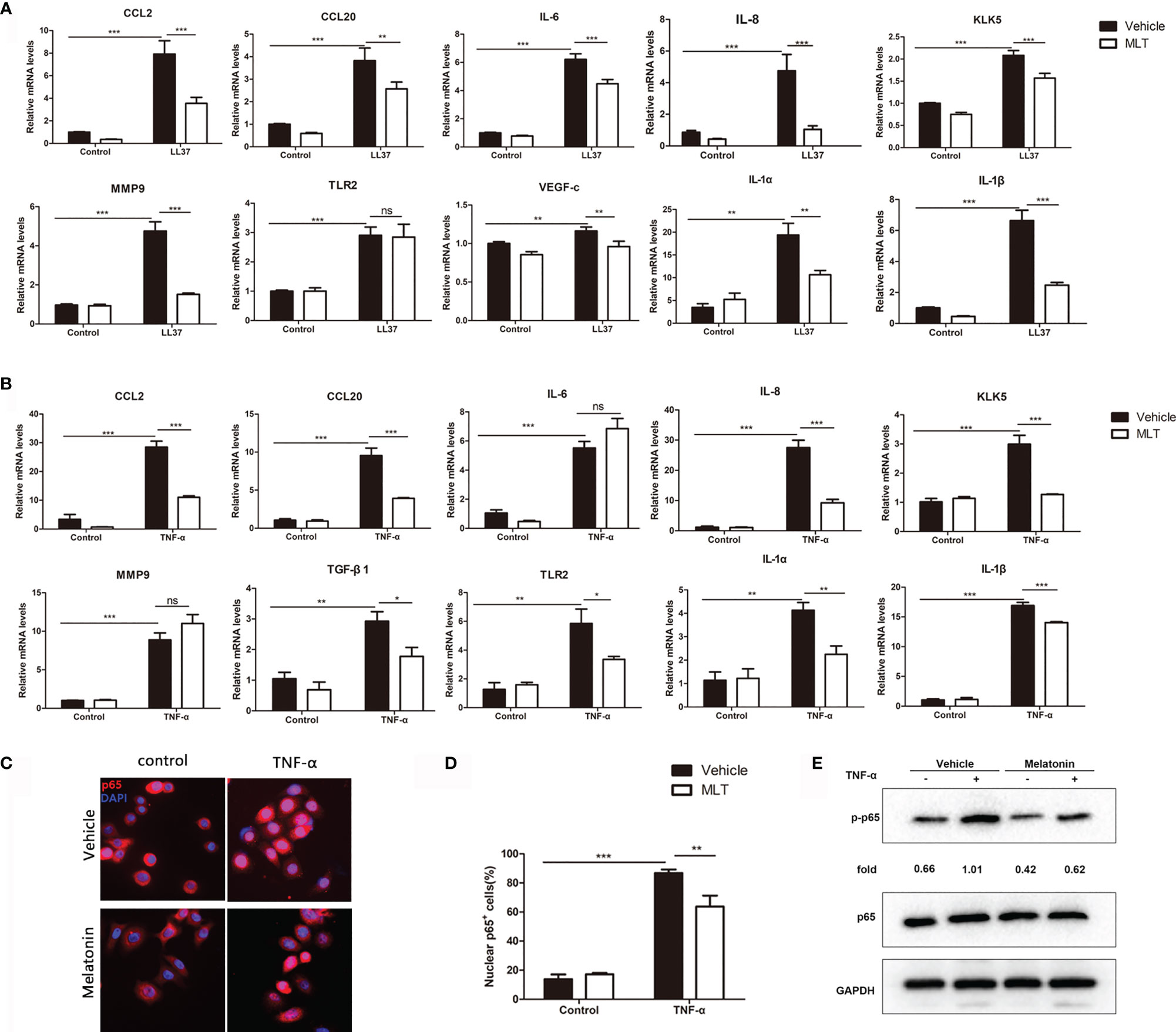
Figure 7 MLT reduced the secretion of inflammatory factors from keratinocyte. qPCR analysis revealed the effects of MLT on the expression of inflammatory cytokines in LL37-treated HaCaT cells (A) and TNF-α-treated HaCaT cells (B). (C) Immunofluorescence analysis revealed the TNF-α-induced p65 translocation. (D) Percentage of p65-positive cells in the nucleus. (E) Immunoblotting of p-p65 and p65 HaCaT cells. All results are representative of at least t independent experiments. Data expressed as individual values with mean ± SEM. *P < 0.05, **P < 0.01, ***P < 0.001. A two-tailed unpaired Student’s t-test was used.
MLT Decreases Angiogenesis by Repressing the Migration and Chemotaxis of HUVEC
We next analyzed the effects of MLT on angiogenesis in rosacea using immunostaining. As shown in Figures 8A, B, the number of CD31+ vessels was significantly reduced by MLT treatment. MLT suppressed LL37-induced HUVEC chemotaxis (Figures 8C, D) and migration (Figures 8E, F). Collectively, these data provide novel evidence that MLT may be a promising therapeutic strategy for vascular dysfunction in rosacea.
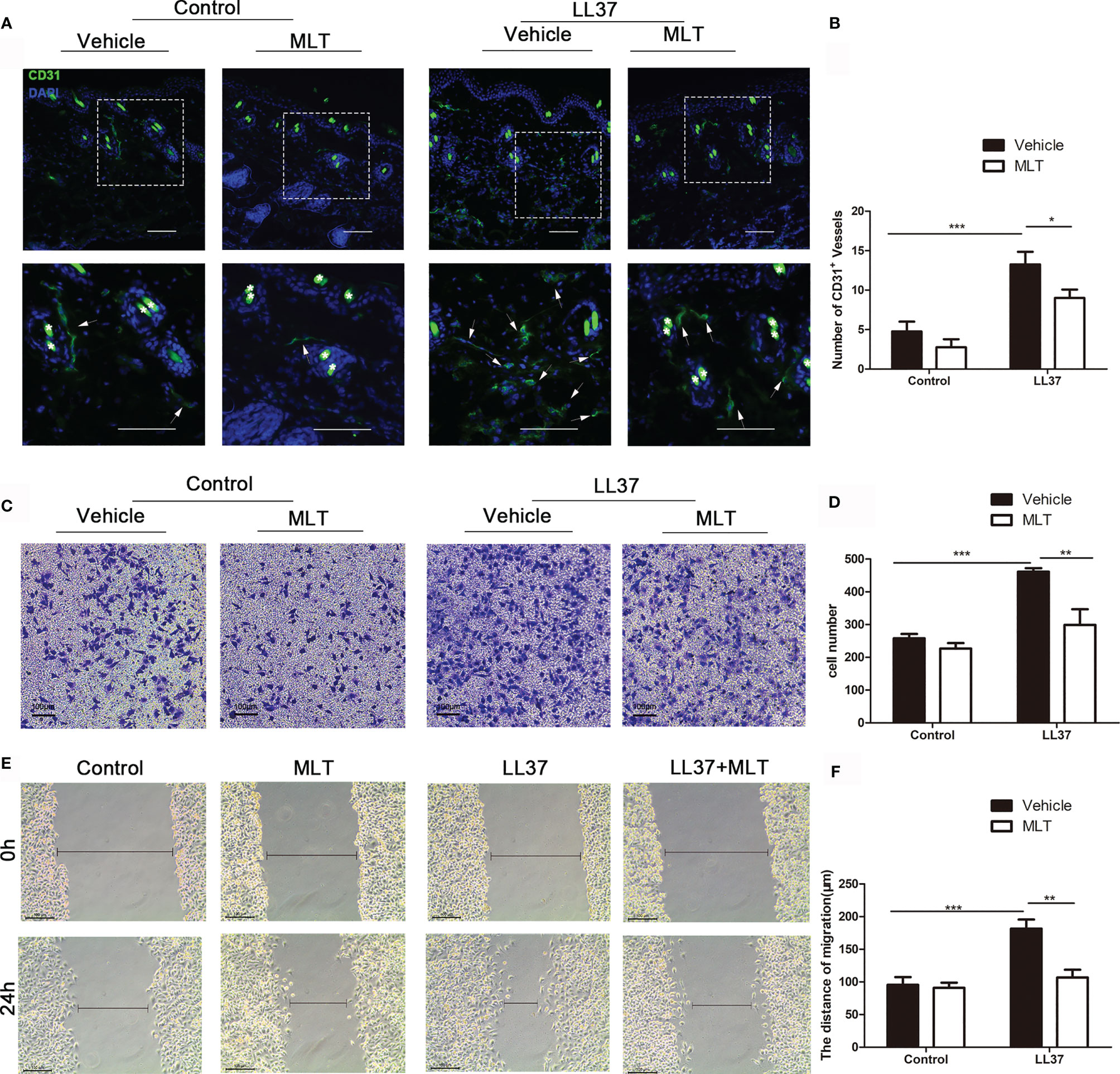
Figure 8 MLT suppresses angiogenesis in rosacea. (A) Immunostaining of CD31+ cells in rosacea-like lesions. Scale bar: 50 μm. (B) The CD31+ microvessels were quantified. (C, D) The transwell assay was used to detect the chemotaxis ability of HUVEC cells. (E, F) The transwell assay was used to detect the migration ability of HUVEC cells. All results are representative of at least three independent experiments. Data expressed as individual values with mean ± SEM. *P < 0.05, **P < 0.01, ***P < 0.01. One-way ANOVA with Bonferroni’s post-hoc test or two-tailed unpaired Student’s t-test was used.
Discussion
Rosacea is a chronic facial inflammatory skin disease, with a global prevalence of over 5% (1). Due to the lack of effective and safe treatments, rosacea may develop as a manifestation of systemic diseases and is significantly associated with metabolic, psychiatric, and neurologic disorders, including AD (37). Therefore, a novel, effective, and safe therapeutic strategy for rosacea is urgently needed. This study revealed the common TF regulatory network in AD/rosacea and identified MLT as a candidate drug for rosacea. The therapeutic effect and mechanism of MLT were verified in vivo and in vitro, and the network pharmacology analysis revealed the MLT-related molecular functions and pharmacological targets for treating AD and rosacea.
Although the exact etiology of rosacea is still uncertain, it is well known that vascular and immunologic dysregulation play a crucial role in rosacea pathogenesis. Chronic neuroinflammation is essential for the pathogenesis of AD significantly (6). Egeberg et al. observed an increased risk of AD in patients with rosacea, partly because of the overlap of proinflammatory mediators between rosacea and AD (6). Consistent with rosacea, vascular dysfunction was also observed in AD (9). In this study, 747 ol-DEGs were detected in AD and rosacea, which were enriched in immune- and metabolism-related pathways. Moreover, the enrichment analysis showed that ol-DEGs were related to inflammation-, vascular-, and infection-related diseases in DisGeNET. These results indicated that inflammatory- and blood vessel-related pathways play a critical role in the pathogenesis of AD and rosacea.
Based on the ol-DEGs, we revealed the TF regulatory network in AD/rosacea, indicating a central role of the 24 TFs in regulating the pathogenesis of AD and rosacea, including PPARG, STAT4, sox9, and RORA. PPARG (PPARγ), a member of the nuclear receptor superfamily, is mainly expressed in adipose tissues and regulates lipid metabolism (38). Recently, studies described the regulation of PPARγ on inflammation by inhibiting cytokines and MMPs and regulating oxidative stress-sensitive pathways and NF-κB pathways (38). Moreover, PPARγ plays a crucial role in the pathogenesis of AD by regulating mitochondrial function, and it is also considered a promising target for pharmacological-based therapies (39). STAT4 is an essential mediator of inflammation by regulating IFN-γ (40, 41), which is closely related to the progression of AD and rosacea (32, 42). RORA, a lipid-sensing nuclear receptor, was reported to have diverse biologic functions, including the regulation of inflammation, lipid metabolism, and angiogenesis (43–46). RORA is distinctly upregulated and reported to play a central role in AD (47). Taken together, these key TFs could be involved in the regulation of inflammation and angiogenesis by targeting their target genes in AD and rosacea. Then, the key TFs and their targets were subsequently submitted to the DGIdb database for predicting the drugs for AD and rosacea, and 113 candidate drugs were identified. In AD and rosacea, two (aspirin and thalidomide) of the three overlapped drugs (aspirin, thalidomide, and hydroxychloroquine) were observed in the predicted drugs, proving that the candidate drugs for AD/rosacea have high credibility. Moreover, three predicted drugs (MLT, olanzapine, and citalopram) have been used for AD treatment. Among these, MLT was found to be the drug that could target both RORA as well as IFN-γ. Moreover, the potential therapeutic effect of MLT on AD and rosacea was also verified using CMap. These findings indicated that MLT could be an effective therapeutic strategy for rosacea.
MLT is a neurohormone that acts as the major regulator of the daily biological rhythm (48) and plays an essential role in diverse physiological processes, including the aging process (13), neuroprotection (49), immune regulation (50), and repressing angiogenesis (51). MLT is safe, has low toxicity, and shows beneficial action against various diseases including AD (52, 53). Hossain et al. showed that MLT can improve sleep quality to mitigate AD neuropathology (54). Our previous study showed that rosacea patients presented poorer sleep quality, which might subsequently aggravate rosacea through regulating inflammatory factors (55). So, we speculate that MLT may relieve rosacea partly by regulating sleep quality.
MLT normally orchestrates daily and seasonal rhythms by acting on the MT(1) and MT(2) receptors (56). Moreover, RORα and RORβ, nuclear MLT receptors, are involved in MLT-mediated regulation of pathological and physiological cardiac hypertrophy (57). Recently, several direct MLT targets and interacting proteins were identified in AD and SARS-CoV-2 treatment, including DAPK1, calmodulin (CALM) 1, and CALM 2 (58, 59). To further reveal the potential molecular mechanism of MLT on AD/rosacea treatment, we used network pharmacology methods to analyze the detailed anti-AD/anti-rosacea mechanisms of MLT. Nineteen genes in the TF regulatory network were identified as potential pharmacological targets of MLT against AD and rosacea. The GO/KEGG enrichment results indicated that anti-AD and anti-rosacea effects exerted by MLT were directed via regulating vascular-associated signaling pathways and inflammation-related signaling pathways, including IL-17, NF-κB, and TNF. Based on the above results, we detected the therapeutic role of MLT on rosacea. The results showed that MLT significantly improved the rosacea-like phenotype in vivo. MLT treatment reduced the CD4+ T-cell and macrophage infiltration and Th1/Th17 polarization partly by repressing keratinocyte-mediated cytokine secretion. Moreover, MLT dramatically suppressed the angiogenesis in the rosacea-like mouse model, partly by inhibiting chemotaxis and migration of HUVECs and VEGF expression. The NF-κB signaling pathway was reported to be activated and functioned as a therapeutic target in AD and rosacea (60–62). MLT was reported to repress the NF-κB signaling pathway in treating neuroinflammation and neurodegeneration (63). In this study, we found that MLT inhibited the activation of the NF-κB pathway in TNF-α-induced inflammatory HaCaT cell, and MLT remarkably decreased the expression of NF-κB downstream genes, IL-1α and IL-1β, in LL37- or TNF-α-treated HaCaT cells. Moreover, TNF-α and IL-17A were also reported as the key cytokines in AD and rosacea (32, 64, 65). Here, we also noted the repression of MLT on IL-17A/TNF-α in rosacea-like mice. These results indicated that MLT attenuates the inflammation of AD/rosacea partly via the NF-κB/IL-17 pathways. Considering the repression of MLT on IL-17, NF-κB, and TNF signaling pathways, MLT could be a potential candidate treatment for patients with autoimmune diseases including psoriasis and vitiligo.
Finally, molecular docking analysis revealed the direct MLT targets, including MMP9, CCND1, EGFR, ICAM1, PTGS2, and SERPINE1, which were inflammation- and angiogenesis-related genes. MMP9 was reported as a key inflammatory factor and as a therapeutic target in both rosacea and AD. Notably, some drugs with an MMP inhibitor function, such as doxycycline and tetracycline, were used for rosacea treatment (66), and the MMP9 inhibitor improved specific neurobehavioral deficits in AD mouse (67). CyclinD1 (CCND1), a prime amplified gene in various cancers (68), was also reported to participate in angiogenesis by repressing the proliferation of endothelial cells (69). ICAM1, an intercellular adhesion molecule, exerts proinflammatory actions via facilitating adhesion and subsequent transmigration of leukocyte (70), increasing the permeability of endothelial and epithelial barrier (71), and promoting immune cell activity (72). The expression of ICAM1 was upregulated in rosacea and AD (73, 74). SERPINE1, a serpin peptidase inhibitor, is related to immune response in gastric cancer (75). PTGS2, also known as COX-2, has a critical role in initiating inflammatory response and angiogenesis (76, 77). Moreover, studies demonstrated the potential relationship between MLT target genes (MMP9, CCND1, EGFR, ICAM1, PTGS2, and SERPINE1) and rosacea-related key genes (IL-6, IL-1β, IFNγ, IL-17, and TNF-α) (78, 79). So, we speculated that MLT repressed the IL-17, NF-κB, and TNF signaling pathways by targeting these pharmacological targets, subsequently leading to inflammation and angiogenesis in AD and rosacea (Figure S5).
Conclusions
In this study, bioinformatics analysis revealed the shared TF regulatory network and the potential drugs for AD and rosacea. Moreover, the potent pharmacological targets and the therapeutic mechanism of MLT against AD/rosacea were identified by network pharmacology and verified in in vivo/in vitro experiments. In conclusion, this study contributes to the common pathologies shared by rosacea and AD and identified MLT as an effective treatment strategy for rosacea and AD via regulating inflammation and angiogenesis.
Data Availability Statement
The original contributions presented in the study are included in the article/Supplementary Material. Further inquiries can be directed to the corresponding authors.
Ethics Statement
All experimental protocols followed the guidelines of animal experimentation and were approved by the Ethical Committee of Xiangya Hospital of Central South University (Approval No. 201611610).
Author Contributions
YZ, HZ, and JL conceived and designed the study. YZ, YL, YW, SY, and SX performed the data analysis and data interpretation. YZ, ZD, and XY conducted the bioinformatics and statistical analyses. YZ, HZ, and JL prepared the manuscript. YZ and HZ performed the cell experiments and animal experiments and data analysis. All authors contributed to the article and approved the submitted version.
Funding
This work was supported by the National Natural Sciences Foundation of Hunan Province (2020JJ5950), the National Natural Science Foundation of China (81703149 and 82073457), and the Science and Technology Innovation Plan of Hunan Province (No. 2018SK2087).
Conflict of Interest
The authors declare that the research was conducted in the absence of any commercial or financial relationships that could be construed as a potential conflict of interest.
Publisher’s Note
All claims expressed in this article are solely those of the authors and do not necessarily represent those of their affiliated organizations, or those of the publisher, the editors and the reviewers. Any product that may be evaluated in this article, or claim that may be made by its manufacturer, is not guaranteed or endorsed by the publisher.
Supplementary Material
The Supplementary Material for this article can be found online at: https://www.frontiersin.org/articles/10.3389/fimmu.2021.756550/full#supplementary-material
References
1. Gether L, Overgaard LK, Egeberg A, Thyssen JP. Incidence and Prevalence of Rosacea: A Systematic Review and Meta-Analysis. Br J Dermatol (2018) 179(2):282–9. doi: 10.1111/bjd.16481
2. Thiboutot D, Anderson R, Cook-Bolden F, Draelos Z, Gallo RL, Granstein RD, et al. Standard Management Options for Rosacea: The 2019 Update by the National Rosacea Society Expert Committee. J Am Acad Dermatol (2020) 82(6):1501–10. doi: 10.1016/j.jaad.2020.01.077
3. Schwab VD, Sulk M, Seeliger S, Nowak P, Aubert J, Mess C, et al. Neurovascular and Neuroimmune Aspects in the Pathophysiology of Rosacea. J Invest Dermatol Symp Proc (2011) 15(1):53–62. doi: 10.1038/jidsymp.2011.6
4. Zhang H, Tang K, Wang Y, Fang R, Sun Q. Rosacea Treatment: Review and Update. Dermatol Ther (2021) 11(1):13–24. doi: 10.1007/s13555-020-00461-0
5. Chen Q, Shi X, Tang Y, Wang B, Xie HF, Shi W, et al. Association Between Rosacea and Cardiometabolic Disease: A Systematic Review and Meta-Analysis. J Am Acad Dermatol (2020) 83(5):1331–40. doi: 10.1016/j.jaad.2020.04.113
6. Egeberg A, Hansen PR, Gislason GH, Thyssen JP. Patients With Rosacea Have Increased Risk of Dementia. Ann Neurol (2016) 79(6):921–8. doi: 10.1002/ana.24645
7. Mann AP, Scodeller P, Hussain S, Braun GB, Mölder T, Toome K, et al. Identification of a Peptide Recognizing Cerebrovascular Changes in Mouse Models of Alzheimer's Disease. Nat Commun (2017) 108(1):1403. doi: 10.1038/s41467-017-01096-0
8. McQuade A, Kang YJ, Hasselmann J, Jairaman A, Sotelo A, Coburn M, et al. Gene Expression and Functional Deficits Underlie TREM2-Knockout Microglia Responses in Human Models of Alzheimer's Disease. Nat Commun (2020) 11(1):5370. doi: 10.1038/s41467-020-19227-5
9. Bandyopadhyay S. Role of Neuron and Glia in Alzheimer's Disease and Associated Vascular Dysfunction. Front Aging Neurosci (2021) 13:653334. doi: 10.3389/fnagi.2021.653334
10. Dekens DW, Eisel ULM, Gouweleeuw L, Schoemaker RG, De Deyn PP, Naudé PJW. Lipocalin 2 as a Link Between Ageing, Risk Factor Conditions and Age-Related Brain Diseases. Ageing Res Rev (2021) 26:101414. doi: 10.1016/j.arr.2021.101414
11. Ferracioli-Oda E, Qawasmi A, Bioch MH. Meta-Analysis: Melatonin for the Treatment of Primary Sleep Disorders. PLoS One (2013) 8(5):1–6. doi: 10.1371/journal.pone.0063773
12. Lok R, Koningsveld MV, Gordijn M, Beersma D, Hut RA. Daytime Melatonin and Light Independently Affect Human Alertness and Body Temperature. J Pineal Res (2019) 67(1):e12583. doi: 10.1111/jpi.12583
13. Majidinia M, Reiter RJ, Shakouri SK, Yousefi B. The Role of Melatonin, a Multitasking Molecule, in Retarding the Processes of Ageing. Ageing Res Rev (2018) 47:198–213. doi: 10.1016/j.arr.2018.07.010
14. Second J, Korganow AS, Jannier S, Puel A, Lipsker D. Rosacea and Demodicidosis Associated With Gain-of-Function Mutation in STAT1. J Eur Acad Dermatol Venereol (2017) 31(12):e542–4. doi: 10.1111/jdv.14413
15. Jauhari A, Baranov SV, Suofu Y, Kim J, Friedlander RM. Melatonin Inhibits Cytosolic Mitochondrial-DNA Induced Neuroinflammatory Signaling in Accelerated Aging and Neurodegeneration. J Clin Invest (2020) 130(6):3124–36. doi: 10.1172/JCI135026
16. Kuklina, Elena M. Melatonin as Potential Inducer of Th17 Cell Differentiation. Med Hypotheses (2014) 83(3):404–6. doi: 10.1016/j.mehy.2014.07.006
17. Guo Q, Dong Y, Cao J, Wang Z, Zhang Z, Chen Y. Developmental Changes of Melatonin Receptor Expression in the Spleen of the Chicken, Gallus Domesticus. Acta Histochem (2015) 117(6):559–65. doi: 10.1016/j.acthis.2015.05.002
18. Wu YH, Feenstra MG, Zhou JN, Liu RY, Toranõ JS, Van Kan HJ, et al. Molecular Changes Underlying Reduced Pineal Melatonin Levels in Alzheimer Disease: Alterations in Preclinical and Clinical Stages. J Clin Endocrinol Metab (2003) 88(12):5898–906. doi: 10.1210/jc.2003-030833
19. Wu YH, Swaab DF. The Human Pineal Gland and Melatonin in Aging and Alzheimer's Disease. J Pineal Res (2005) 38(3):145–52. doi: 10.1111/j.1600-079X.2004.00196.x
20. Rosales-Corral S, Tan DX, Reiter RJ, Valdivia-Velázquez M, Martínez-Barboza G, Acosta-Martínez J, et al. Orally Administered Melatonin Reduces Oxidative Stress and Proinflammatory Cytokines Induced by Amyloid-β Peptide in Rat Brain: A Comparative, In Vivo Study Versus Vitamin C and E. J Pineal Res (2003) 35(2):80–4. doi: 10.1034/j.1600-079X.2003.00057.x
21. Smith MA, Hirai K, Hsiao K, Pappolla MA, Harris PL, Siedlak SL, et al. Amyloid-Beta Deposition in Alzheimer Transgenic Mice Is Associated With Oxidative Stress. J Neurochem (1998) 70(5):2212. doi: 10.1046/j.1471-4159.1998.70052212.x
22. Tsiskarishvili NI, Katsitadze A, Tsiskarishvili NV, Tsiskarishvil TS, Chitanava L. Melatonin Concentration in the Blood of Vitiligo Patients With Stress in Anamnesis. Georgian Med News (2016) 254:47–53.
23. Marseglia L. Atopic Dermatitis: Melatonin as Potential Treatment. J Biol Regul Homeost Agents (2015) 29(2 suppl 1):142–9.
24. Cotto KC, Wagner AH, Feng YY, Kiwala S, Coffman AC, Spies G, et al. DGIdb 3.0: A Redesign and Expansion of the Drug-Gene Interaction Database. Nucleic Acids Res (2018) 46(D1):D1068–d1073. doi: 10.1093/nar/gkx1143
25. Freshour SL, Kiwala S, Cotto KC, Coffman AC, McMichael JF, Song JJ, et al. Integration of the Drug-Gene Interaction Database (DGIdb 4.0) With Open Crowdsource Efforts. Nucleic Acids Res (2021) 49(D1):D1144–51. doi: 10.1093/nar/gkaa1084
26. Subramanian A, Narayan R, Corsello SM, Peck DD, Natoli TE, Lu X, et al. A Next Generation Connectivity Map: L1000 Platform and the First 1,000,000 Profiles. Cell (2017) 171(6):1437–52.e17. doi: 10.1016/j.cell.2017.10.049
27. Wishart DS, Feunang YD, Guo AC, Lo EJ, Marcu A, Grant JR, et al. DrugBank 5.0: A Major Update to the DrugBank Database for 2018. Nucleic Acids Res (2018) 46(D1):D1074–d1082. doi: 10.1093/nar/gkx1037
28. Li R, Li Y, Liang X, Yang L, Su M, Lai KP. Network Pharmacology and Bioinformatics Analyses Identify Intersection Genes of Niacin and COVID-19 as Potential Therapeutic Targets. Brief Bioinform (2021) 22(2):1279–90. doi: 10.1093/bib/bbaa300
29. Yamasaki K, Di Nardo A, Bardan A, Murakami M, Ohtake T, Coda A, et al. Increased Serine Protease Activity and Cathelicidin Promotes Skin Inflammation in Rosacea. Nat Med (2007) 13(8):975–80. doi: 10.1038/nm1616
30. Aroni K, Tsagroni E, Kavantzas N, Patsouris E, Ioannidis E. A Study of the Pathogenesis of Rosacea: How Angiogenesis and Mast Cells may Participate in a Complex Multifactorial Process. Arch Dermatol Res (2008) 300(3):125–31. doi: 10.1007/s00403-007-0816-z
31. Hara-Chikuma M, Satooka H, Watanabe S, Hon Da T, Miyachi Y, Watanabe T, et al. Aquaporin-3-Mediated Hydrogen Peroxide Transport Is Required for NF-κB Signalling in Keratinocytes and Development of Psoriasis. Nat Commun (2015) 6:7454. doi: 10.1038/ncomms8454
32. Buhl T, Sulk M, Nowak P, Buddenkotte J, McDonald I, Aubert J, et al. Molecular and Morphological Characterization of Inflammatory Infiltrate in Rosacea Reveals Activation of Th1/Th17 Pathways. J Invest Dermatol (2015) 135(9):2198–208. doi: 10.1038/jid.2015.141
33. Mengting, Chen, Hongfu, Xie, Zhaohui, San, et al. Thalidomide Ameliorates Rosacea-Like Skin Inflammation and Suppresses NF-κB Activation in Keratinocytes. Biomed Pharmacother (2019) 116:109011–1. doi: 10.1016/j.biopha.2019.109011
34. Sethi A, Kulkarni N, Sonar S, Lal G. Role of miRNAs in CD4 T Cell Plasticity During Inflammation and Tolerance. Front Genet (2013) 4:8–. doi: 10.3389/fgene.2013.00008
35. Steinhoff M, Buddenkotte J, Aubert J, Sulk M, Novak P, Schwab VD, et al. Clinical, Cellular, and Molecular Aspects in the Pathophysiology of Rosacea. J Invest Dermatol Symp Proc (2011) 15(1):2–11. doi: 10.1038/jidsymp.2011.7
36. Sethi G, Tergaonkar V. Potential Pharmacological Control of the NF-κB Pathway. Trends Pharmacol Sci (2009) 30(6):313–21. doi: 10.1016/j.tips.2009.03.004
37. Wollina U. Is Rosacea a Systemic Disease? Clinics Dermatol (2019) 37(6):629–35. doi: 10.1016/j.clindermatol.2019.07.032
38. Linford NJ, Beyer RP, Gollahon K, Krajcik RA, Malloy VL, Demas V, et al. Transcriptional Response to Aging and Caloric Restriction in Heart and Adipose Tissue. Aging Cell (2007) 6(5):673–88. doi: 10.1111/j.1474-9726.2007.00319.x
39. Jamwal S, Blackburn JK, Elsworth JD. PPARγ/PGC1α Signaling as a Potential Therapeutic Target for Mitochondrial Biogenesis in Neurodegenerative Disorders. Pharmacol Ther (2021) 219:107705. doi: 10.1016/j.pharmthera.2020.107705
40. Dong X, Antao OQ, Song W, Sanchez GM, Zembrzuski K, Koumpouras F, et al. Type I Interferon-Activated STAT4 Regulation of Follicular Helper T Cell-Dependent Cytokine and Immunoglobulin Production in Lupus. Arthritis Rheumatol (Hoboken NJ) (2021) 73(3):478–89. doi: 10.1002/art.41532
41. Morinobu A, Gadina M, Strober W, Visconti R, Fornace A, Montagna C, et al. STAT4 Serine Phosphorylation Is Critical for IL-12-Induced IFN-Gamma Production But Not for Cell Proliferation. Proc Natl Acad Sci U S A (2002) 99(19):12281–6. doi: 10.1073/pnas.182618999
42. Yang HS, Zhang C, Carlyle BC, Zhen SY, Trombetta BA, Schultz AP, et al. Plasma IL-12/IFN-γ Axis Predicts Cognitive Trajectories in Cognitively Unimpaired Older Adults. Alzheimers Dement (2021). doi: 10.1002/alz.12399
43. Sun Y, Liu CH, SanGiovanni JP, Evans LP, Tian KT, Zhang B, et al. Nuclear Receptor Rorα Regulates Pathologic Retinal Angiogenesis by Modulating SOCS3-Dependent Inflammation. Proc Natl Acad Sci U S A (2015) 112(33):10401–6. doi: 10.1073/pnas.1504387112
44. Byun JK, Choi YK, Kang YN, Jang BK, Kang KJ, Jeon YH, et al. Retinoic Acid-Related Orphan Receptor Alpha Reprograms Glucose Metabolism in Glutamine-Deficient Hepatoma Cells. Hepatology (Baltimore Md) (2015) 61(3):953–64. doi: 10.1002/hep.27577
45. Sugita K, Steer CA, Martinez-Gonzalez I, Altunbulakli C, Morita H, Castro-Giner F, et al. Type 2 Innate Lymphoid Cells Disrupt Bronchial Epithelial Barrier Integrity by Targeting Tight Junctions Through IL-13 in Asthmatic Patients. J Allergy Clin Immunol (2018) 141(1):300–10.e11. doi: 10.1016/j.jaci.2017.02.038
46. Salimi M, Barlow JL, Saunders SP, Xue L, Gutowska-Owsiak D, Wang X, et al. A Role for IL-25 and IL-33-Driven Type-2 Innate Lymphoid Cells in Atopic Dermatitis. J Exp Med (2013) 210(13):2939–50. doi: 10.1084/jem.20130351
47. Acquaah-Mensah GK, Agu N, Khan T, Gardner A. A Regulatory Role for the Insulin- and BDNF-Linked RORA in the Hippocampus: Implications for Alzheimer's Disease. J Alzheimers Dis (2015) 44(3):827–38. doi: 10.3233/jad-141731
48. Fougerou C, Jaafari N, Bellissant E, Charrier A, Delorme R, Chokron S, et al. Melatonin: Pharmacology, Functions and Therapeutic Benefits. Curr Neuropharmacol (2017) 15(3):434–43. doi: 10.2174/1570159X14666161228122115
49. Vahdani FG, Hantoushzadeh S, Nazari F. Melatonin as Fetal Neuroprotection: Links and Risks. Biomed J (2020) 43(6):496–7. doi: 10.1016/j.bj.2020.10.006
50. Hardeland R. Melatonin and Inflammation-Story of a Double-Edged Blade. J Pineal Res (2018) 65(4):e12525. doi: 10.1111/jpi.12525
51. Ma Q, Reiter RJ, Chen Y. Role of Melatonin in Controlling Angiogenesis Under Physiological and Pathological Conditions. Angiogenesis (2020) 23(2):91–104. doi: 10.1007/s10456-019-09689-7
52. He F, Chou CJ, Scheiner M, Poeta E, Yuan Chen N, Gunesch S, et al. Melatonin- and Ferulic Acid-Based HDAC6 Selective Inhibitors Exhibit Pronounced Immunomodulatory Effects In Vitro and Neuroprotective Effects in a Pharmacological Alzheimer's Disease Mouse Model. J Med Chem (2021) 64(7):3794–812. doi: 10.1021/acs.jmedchem.0c01940
53. Liu B, Huang J, Ashraf A, Rahaman O, Lou J, Wang L, et al. The RNase MCPIP3 Promotes Skin Inflammation by Orchestrating Myeloid Cytokine Response. Nat Commun (2021) 12(1):4105. doi: 10.1038/s41467-021-24352-w
54. Hossain MF, Wang N, Chen R, Li S, Roy J, Uddin MG, et al. Exploring the Multifunctional Role of Melatonin in Regulating Autophagy and Sleep to Mitigate Alzheimer's Disease Neuropathology. Ageing Res Rev (2021) 67:101304. doi: 10.1016/j.arr.2021.101304
55. Wang Z, Xie H, Gong Y, Ouyang Y, Deng F, Tang Y, et al. Relationship Between Rosacea and Sleep. J Dermatol (2020) 47(6):592–600. doi: 10.1111/1346-8138.15339
56. Wang Q, Zhu D, Ping S, Li C, Pang K, Zhu S, et al. Melatonin Recovers Sleep Phase Delayed by MK-801 Through the Melatonin MT(2) Receptor- Ca(2+) -CaMKII-CREB Pathway in the Ventrolateral Preoptic Nucleus. J Pineal Res (2020) 69(3):e12674. doi: 10.1111/jpi.12674
57. Xu L, Su Y, Zhao Y, Sheng X, Tong R, Ying X, et al. Melatonin Differentially Regulates Pathological and Physiological Cardiac Hypertrophy: Crucial Role of Circadian Nuclear Receptor Rorα Signaling. J Pineal Res (2019) 67(2):e12579. doi: 10.1111/jpi.12579
58. Chen D, Mei Y, Kim N, Lan G, Gan CL, Fan F, et al. Melatonin Directly Binds and Inhibits Death-Associated Protein Kinase 1 Function in Alzheimer's Disease. J Pineal Res (2020) 69(2):e12665. doi: 10.1111/jpi.12665
59. Zhou Y, Hou Y, Shen J, Huang Y, Martin W, Cheng F. Network-Based Drug Repurposing for Novel Coronavirus 2019-Ncov/SARS-CoV-2. Cell Discov (2020) 6(1):14. doi: 10.1038/s41421-020-0153-3
60. Yuan X, Li J, Li Y, Deng Z, Zhou L, Long J, et al. Artemisinin, a Potential Option to Inhibit Inflammation and Angiogenesis in Rosacea. Biomed Pharmacother (2019) 117:109181. doi: 10.1016/j.biopha.2019.109181
61. Kaltschmidt B, Kaltschmidt C. NF-kappaB in the Nervous System. Cold Spring Harb Perspect Biol (2009) 1(3):a001271. doi: 10.1101/cshperspect.a001271
62. Lian H, Yang L, Cole A, Sun L, Chiang AC, Fowler SW, et al. NFκB-Activated Astroglial Release of Complement C3 Compromises Neuronal Morphology and Function Associated With Alzheimer's Disease. Neuron (2015) 85(1):101–15. doi: 10.1016/j.neuron.2014.11.018
63. Ali T, Badshah H, Kim TH, Kim MO. Melatonin Attenuates D-Galactose-Induced Memory Impairment, Neuroinflammation and Neurodegeneration via RAGE/NF-K B/JNK Signaling Pathway in Aging Mouse Model. J Pineal Res (2015) 58(1):71–85. doi: 10.1111/jpi.12194
64. Chen J, Liu X, Zhong Y. Interleukin-17a: The Key Cytokine in Neurodegenerative Diseases. Front Aging Neurosci (2020) 12:566922. doi: 10.3389/fnagi.2020.566922
65. How Shing Koy E, Labauge P, Baillet A, Prati C, Marotte H, Pers YM. Immunomodulation With IL-17 and TNF-α in Spondyloarthritis: Focus on the Eye and the Central Nervous System. Ther Adv Musculoskelet Dis (2021) 13:1759720x211025894. doi: 10.1177/1759720x211025894
66. Two AM, Wu W, Gallo RL, Hata TR. Rosacea: Part I. Introduction, Categorization, Histology, Pathogenesis, and Risk Factors. J Am Acad Dermatol (2015) 72(5):749–58; quiz 759-60. doi: 10.1016/j.jaad.2014.08.028
67. Ringland C, Schweig JE, Eisenbaum M, Paris D, Ait-Ghezala G, Mullan M, et al. MMP9 Modulation Improves Specific Neurobehavioral Deficits in a Mouse Model of Alzheimer's Disease. BMC Neurosci (2021) 22(1):39. doi: 10.1186/s12868-021-00643-2
68. Jirawatnotai S, Hu Y, Livingston DM, Sicinski P. Proteomic Identification of a Direct Role for Cyclin D1 in DNA Damage Repair. Cancer Res (2012) 72(17):4289–93. doi: 10.1158/0008-5472.can-11-3549
69. Pi J, Liu J, Zhuang T, Zhang L, Sun H, Chen X, et al. Elevated Expression of Mir302-367 in Endothelial Cells Inhibits Developmental Angiogenesis via CDC42/CCND1 Mediated Signaling Pathways. Theranostics (2018) 8(6):1511–26. doi: 10.7150/thno.21986
70. Pober JS, Kluger MS, Schechner JS. Human Endothelial Cell Presentation of Antigen and the Homing of Memory/Effector T Cells to Skin. Ann N Y Acad Sci (2001) 941:12–25. doi: 10.1111/j.1749-6632.2001.tb03706.x
71. Bui TM, Wiesolek HL, Sumagin R. ICAM-1: A Master Regulator of Cellular Responses in Inflammation, Injury Resolution, and Tumorigenesis. J Leukoc Biol (2020) 108(3):787–99. doi: 10.1002/jlb.2mr0220-549r
72. Proto JD, Doran AC, Gusarova G, Yurdagul A, Sozen E, Subramanian M, et al. Regulatory T Cells Promote Macrophage Efferocytosis During Inflammation Resolution. Immunity (2018) 49(4):666–77. doi: 10.1016/j.immuni.2018.07.015
73. Kulkarni NN, Takahashi T, Sanford JA, Tong Y, Gombart AF, Hinds B, et al. Innate Immune Dysfunction in Rosacea Promotes Photosensitivity and Vascular Adhesion Molecule Expression. J Invest Dermatol (2019) 140(3):645–55. doi: 10.1016/j.jid.2019.08.436
74. Janelidze S, Mattsson N, Stomrud E, Lindberg O, Palmqvist S, Zetterberg H, et al. CSF Biomarkers of Neuroinflammation and Cerebrovascular Dysfunction in Early Alzheimer Disease. Neurology (2018) 91(9):e867–77. doi: 10.1212/wnl.0000000000006082
75. Pei JP, Zhang CD, Yusupu M, Zhang C, Dai DQ. Screening and Validation of the Hypoxia-Related Signature of Evaluating Tumor Immune Microenvironment and Predicting Prognosis in Gastric Cancer. Front Immunol (2021) 12:705511. doi: 10.3389/fimmu.2021.705511
76. Möbius C, Stein HJ, Spiess C, Becker I, Feith M, Theisen J, et al. COX2 Expression, Angiogenesis, Proliferation and Survival in Barrett's Cancer. Eur J Surg Oncol (2005) 31(7):755–9. doi: 10.1016/j.ejso.2005.01.006
77. Binion DG, Otterson MF, Rafiee P. Curcumin Inhibits VEGF-Mediated Angiogenesis in Human Intestinal Microvascular Endothelial Cells Through COX-2 and MAPK Inhibition. Gut (2008) 57(11):1509–17. doi: 10.1136/gut.2008.152496
78. Harden JL, Shih YH, Xu J, Li R, Rajendran D, Hofland H, et al. Paired Transcriptomic and Proteomic Analysis Implicates IL-1β in the Pathogenesis of Papulopustular Rosacea Explants. J Invest Dermatol (2020) 141(4):800–9. doi: 10.1016/j.jid.2020.08.013
Keywords: rosacea, AD, MLT, network pharmacology, inflammation, angiogenesis
Citation: Zhang H, Zhang Y, Li Y, Wang Y, Yan S, Xu S, Deng Z, Yang X, Xie H and Li J (2021) Bioinformatics and Network Pharmacology Identify the Therapeutic Role and Potential Mechanism of Melatonin in AD and Rosacea. Front. Immunol. 12:756550. doi: 10.3389/fimmu.2021.756550
Received: 10 August 2021; Accepted: 28 October 2021;
Published: 23 November 2021.
Edited by:
Kai Fang, University of California, Los Angeles, United StatesReviewed by:
Danuta Gutowska-Owsiak, Intercollegiate Faculty of Biotechnology of University of Gdańsk and Medical University of Gdańsk, PolandUzma Saqib, Indian Institute of Technology Indore, India
Copyright © 2021 Zhang, Li, Wang, Yan, Xu, Deng, Yang, Xie, Zhang and Li. This is an open-access article distributed under the terms of the Creative Commons Attribution License (CC BY). The use, distribution or reproduction in other forums is permitted, provided the original author(s) and the copyright owner(s) are credited and that the original publication in this journal is cited, in accordance with accepted academic practice. No use, distribution or reproduction is permitted which does not comply with these terms.
*Correspondence: Yiya Zhang, eWl5YTAxMDhAY3N1LmVkdS5jbg==; Ji Li, bGlqaV94eUBjc3UuZWR1LmNu
†These authors have contributed equally to this work