- 1Department of Clinical Nutrition, The First Affiliated Hospital of Chengdu Medical College, Chengdu, Sichuan, China
- 2Department of Dermatology, West China Hospital, Sichuan University, Chengdu, China
- 3Department of Nephrology, The First Affiliated Hospital of Chengdu Medical College, Chengdu, Sichuan, China
- 4Department of Vascular Surgery, The First Affiliated Hospital of Chengdu Medical College, Chengdu, Sichuan, China
- 5Department of Neurosurgery, Hospital of Chengdu University of Traditional Chinese Medicine, Chengdu, Sichuan, China
Chronic kidney disease (CKD) is a global health issue, affecting approximately 10% of the population. Hypoalbuminemia, a common complication in advanced CKD, is associated with poor prognosis. This study aimed to investigate the association between a microbiota-friendly dietary scoring system (Dietary Index for Gut Microbiota, DI-GM) and serum albumin levels in patients with CKD. We utilized a cross-sectional cohort from the NHANES 2007–2018, which included 2,947 CKD patients. Multivariable logistic regression and restricted cubic spline models were applied to analyze the relationship between DI-GM scores and serum albumin. Higher DI-GM scores were significantly associated with increased serum albumin levels (β = 0.18 g/L, 95% CI: 0.07–0.28, p = 0.002). Furthermore, each 1-point increase in DI-GM score was linked to a 15% reduction in the odds of hypoalbuminemia (OR: 0.85, 95% CI: 0.74–0.97, p = 0.014). The findings suggest that a high DI-GM diet may have beneficial effects in managing hypoalbuminemia in CKD patients by modulating gut microbiota composition and reducing inflammation. This diet pattern could be a promising dietary intervention for improving clinical outcomes in CKD patients, especially those at risk for malnutrition and inflammation.
Introduction
Chronic kidney disease (CKD) has emerged as a significant global health issue, affecting approximately 10% of the population worldwide, with cases spanning early to advanced stages of the disease, ranging from early to advanced stages of the disease (1, 2).One common complication in CKD is hypoalbuminemia, characterized by low serum albumin levels, which affects about 30% to 50% of patients, especially those in advanced stages (3). Albumin, the most abundant protein in blood plasma, plays an essential role in maintaining colloidal osmotic pressure and antioxidant capacity (4, 5). It also serves as an indicator of chronic inflammation and protein-energy wasting (PEW), a condition that poses additional health risks for CKD patients (6).
The underlying causes of hypoalbuminemia are multifactorial and complex, often involving reduced protein synthesis, increased catabolism, oxidative stress, and nutritional deficiencies (7–9). Research has shown that CKD patients frequently experience significant shifts in gut microbiota, marked by a reduction in beneficial bacteria and an increase in pathogenic species (10–14), contributing to systemic oxidative stress, elevated inflammation (15, 16), and an exacerbation of hypoalbuminemia (4). Furthermore, dietary modifications in CKD, such as low-protein diets and restrictions on fruits and vegetables, may further disrupt gut microbiota (17, 18), perpetuating a vicious cycle of microbial imbalance and inflammation.
Given these concerns, optimizing gut microbiota composition has emerged as a potential therapeutic strategy for addressing hypoalbuminemia in CKD. Dietary management has become critical in this context. The Dietary Index for Gut Microbiota (DI-GM) is a novel dietary assessment tool developed to evaluate diet’s impact on gut health by measuring microbial diversity and short-chain fatty acid production. This index considers the intake of 14 food items, both beneficial (e.g., fermented dairy products, whole grains) and detrimental (e.g., red and processed meats).Studies have positively correlated DI-GM scores with biomarkers indicating gut microbiota diversity, supporting its validity and applicability (19).
Based on existing literature, we hypothesize that the regulation of gut microbiota by the Dietary Index (DI-GM) may be associated with improved serum albumin levels in CKD patients. This study aims to systematically analyze the association between DI-GM and serum albumin levels in CKD patients and explore potential underlying mechanisms. By examining the impact of this dietary index, we hope to provide new evidence for future gut microbiota-related interventions in CKD management.
Materials and methods
Participants
This cross-sectional study utilized data from the NHANES from 2007 to 2018, which was approved by the Institutional Review Board of the National Center for Health Statistics. All participants provided written informed consent, and the use of de-identified public data exempted the study from further ethical review. The study adheres to the Strengthening the Reporting of Observational Studies in Epidemiology (STROBE) guidelines.
We included NHANES participants aged 18 years and older (n=36580). Exclusions were applied for participants with missing values to calculated albumin-to-creatinine ratio (ACR) and estimated glomerular filtration rate (eGFR) data. Those lacking two-day dietary data, missing height or weight data, abnormal energy intake (<800 or >4000 kcal/day for men; <500 or >3500 kcal/day for women), and individuals with a history of cancer or dialysis, pregnant and not meeting the diagnostic criteria for CKD, we also excluded the missing covariate data. The final analytic cohort consisted of 2947 individuals, representing 19,690,044.94 U.S. CKD adults (Figure 1). Sociodemographic variables, lifestyle variables, laboratory variables and dietary data were collected.
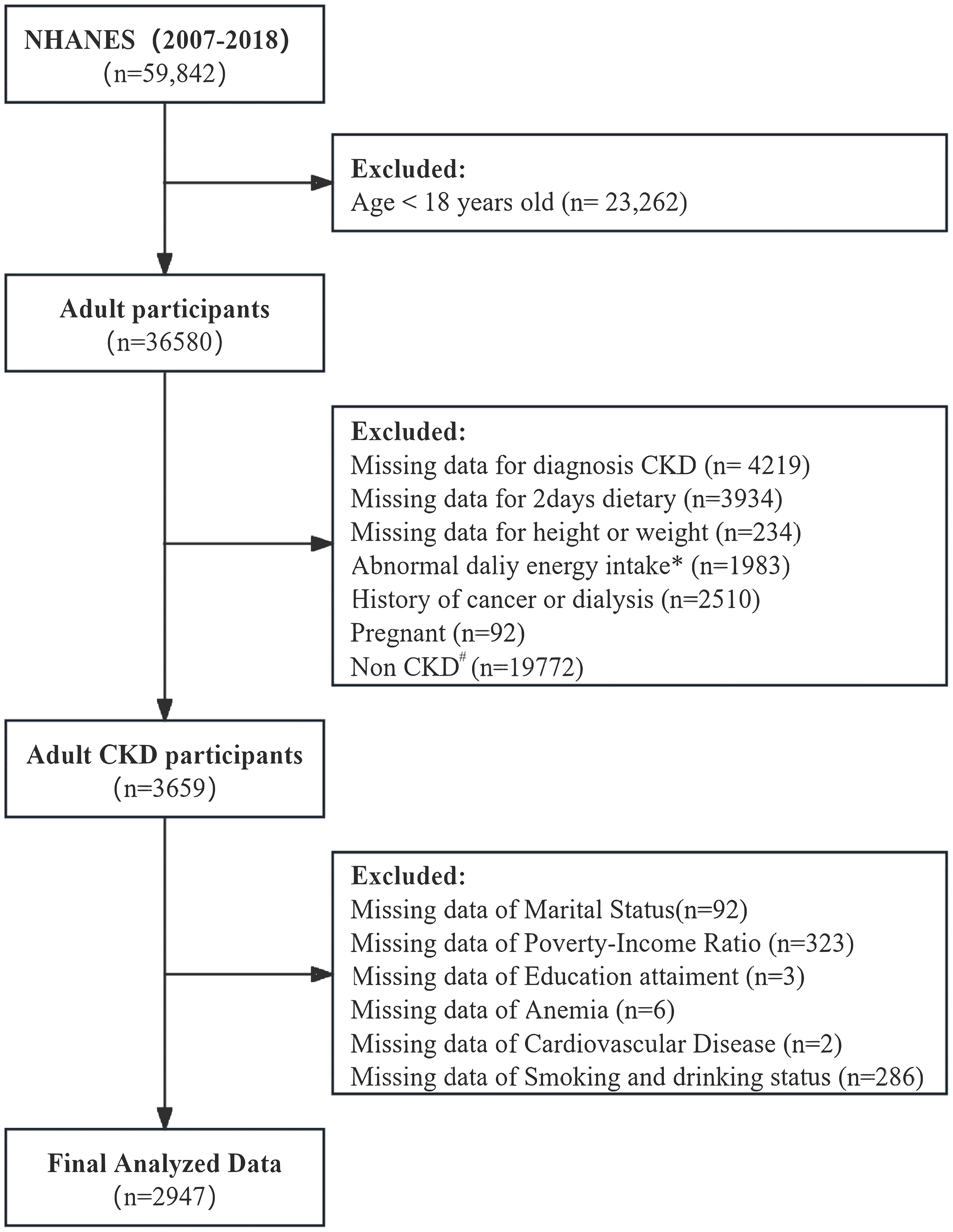
Figure 1. Study Population and flowchart. #: Diagnosis of chronic kidney disease (CKD): estimated glomerular filtration rate (eGFR) <60 mL/min/1.73 m² or urinary albumin-to-creatinine ratio (UACR) ≥30 mg/g. Complete and reliable dietary intake data for calculating DI-GM. *Abnormal dietary records: <800 kcal/day or >4000 kcal/day for men; <500 kcal/day or >3500 kcal/day for women.
Definition
CKD classification was based on a urinary albumin-to-creatinine ratio (≥30 mg/g or 3 mg/mmol) or estimated glomerular filtration rate (eGFR) <60 mL/min/1.73 m² (20). eGFR was calculated using the CKD-EPI- creatinine formula. Given that nutritional interventions typically start at stage G3 (21), we classified G1(eGFR ≥ 90 mL/min/1.73 m²)-G2(eGFR 60–89 mL/min/1.73 m²) as early CKD and G3a-G5(G3a: eGFR 45–59 mL/min/1.73 m², G3b: eGFR 30–44 mL/min/1.73 m², G4: eGFR 15–29 mL/min/1.73 m², G5:eGFR < 15 mL/min/1.73 m²) as advanced CKD. Hypoalbuminemia is defined as a serum albumin level less than 38 g/L (6).
Dietary assessment
Dietary data from NHANES was collected through two 24-hour recalls, spaced 3–10 days, utilizing the USDA’s Automated Multiple-Pass Method. These recalls were conducted at MECs and via telephone, respectively. Standardized tools aided in portion size estimation. The USDA’s FNDDS was employed to code all foods and beverages and their amounts (22). The DI-GM was then constructed using the averaged intake from the two recalls.
DI-GM
To quantify dietary influence on gut microbiota, a scoring system was developed (19). 14 foods or nutrients were identified as components of the DI-GM, including fermented dairy, chickpeas, soybean, whole grains, fiber, cranberries, avocados, broccoli, coffee, and green tea as beneficial components, and red meat, processed meat, refined grains, and high-fat diet (≥40% of energy from fat) as unfavorable components. Each component was scored 0 or 1 based on sex-specific median intakes, participants who met the criteria for healthy intake (above the sex-specific median for beneficial components, or below for unfavorable components) received a score of 1. Those who did not meet these criteria received a 0. The aggregate of these scores produced the DI-GM score, a measure from 0 to 14, where higher values correlate with enhanced gut microbiota health (Supplementary Table S1).
In this study, we divided DI-GM into tertiles based on score ranges: the first tertile, T1(DI-GM scores 0-3, n=600), the second tertile, T2 (DI-GM scores 4-5, n=1331), and the third tertile, T3 (DI-GM scores 6-10, n=1061).
Covariates
Covariates were selected based on existing literature and clinical judgment. Binary logistic regression was used to identify confounders that altered the initial coefficients by more 10%. Multicollinearity was assessed with the variance inflation factor (VIF), with VIF values ≥5 indicating multicollinearity. The final covariates included age, sex, poverty-income ratio (PIR), Education attainment, marital status, body mass index (BMI), smoking and drinking status, hyperlipidemia history, eGFR, urinary albumin, energy intake (kcal/kg/day), and protein intake (g/kg/day), cardiovascular disease (CVD), hyperlipidemia, hypertension and diabetes mellitus (DM).
Statistical analyses
Two-day dietary weights, adjusted for the six survey cycles, were applied to represent the U.S. population aged 18 years and older, accounting for the complex survey design and non-response bias.
Descriptive statistics were used to summarize baseline characteristics, nutrient intake, dietary quality, and frailty incidence across different survey years. Continuous variables were presented as mean ± standard error (SE) for normal distributed data, or as median and interquartile range (IQR) for skewed data, while categorical variables were reported as frequencies and percentages.
Weighted univariable and multivariable logistic regression models were employed to assess the association between DI-GM and serum albumin/hypoalbuminemia across CKD stages, with weighted odds ratios (ORs) and 95% confidence intervals (CIs) calculated before and after adjusting for confounders. Three models were developed to adjusted for (1): age, sex, race (2); age, sex, race, education, marital status, PIR, BMI, smoking status, drinking status; and (3) adding hyperlipidemia, CVD, hypertension, DM, eGFR, urinary albumin, energy, and protein daily intake (adjusted by standard body weight).
A weighted Restricted Cubic Spline (RCS) model with three knots examined the potential non-linear relationship between DI-GM and serum albumin, with 1,000 bootstrap replications for robustness. Subgroup analyses stratified by age, sex, BMI, smoking, CKD stage, diabetes, energy, and protein intake were conducted using multivariable logistic regression to calculate ORs (95% CI). Results were visualized using a forest plot.
We also conducted the further univariable and multivariable analysis of components’ score in the DI-GM and their association with serum albumin levels to find the most important details among the components. We found the unfavorable components score of refined grains was the most related factor, moreover, we further explore the association between refined grain intake daily and serum albumin level with univariable and multivariable logistic regression analysis.
All analyses used weighted data, with statistical significance was set at p<0.05. Statistical analyses were conducted using R (v4.4.1) and Free Statistics software (version 1.9.2; Beijing Free Clinical Medical Technology Co., Ltd.).
Results
A total of 2,947 participants, representing an estimated 19.60 million adults with CKD in the US, were included (Figure 1, Table 1). Baseline characteristics of participants are shown in Table 1. The mean age was 58.21 years, and 40.25% were male. Significant sociodemographic differences were observed across DI-GM tertiles. Non-Hispanic Black individuals comprised 18.01% of the lowest tertile, while Non-Hispanic White individuals accounted for 74.51% of the highest tertile (P < 0.001). Higher education levels were associated with the highest tertile, with 63.89% being college-educated (P <0.001), and the PIR was significantly lower in the lowest tertile (P < 0.001). Regarding lifestyle factors, smoking prevalence was highest in the middle tertile (20.05%) and lowest in the highest tertile (13.47%; P =0.013). For clinical and laboratory variables, albumin levels were highest in the top tertile (42.21 g/L in T3; P = 0.001), whereas serum creatinine and uric acid levels were significantly elevated in the lowest tertile (P = 0.029 and P = 0.015, respectively). Serum bicarbonate levels were significantly lower in the lowest tertile (P =0.003). No significant differences in comorbidity incidence were found among the three tertiles (Table 1).
Dietary data and components of DI-GM
Dietary intake and food group consumption across tertiles of the Dietary Index for Gut Microbiota (DI-GM) in participants with CKD are summarized in Table 2. Energy and protein intake did not differ significantly across tertiles. However, carbohydrate intake increased progressively from the lowest to the highest tertile (P < 0.001), and fiber intake was significantly higher in the highest tertile compared with the other tertiles (P <0.001). Total fat intake and saturated fat intake were highest in the lowest tertile (P = 0.036 and P =0.044, respectively). As DI-GM scores increased, the proportion of individuals with a score of 1 for specific food items also rose (P < 0.01 for all). Scores for all DI-GM components, including avocado, broccoli, chickpea, coffee, cranberry, fermented dairy, fiber, green tea, soybean, whole grains, refined grains, processed meat, and fat, increased significantly across higher DI-GM tertiles (P < 0.001 for all).
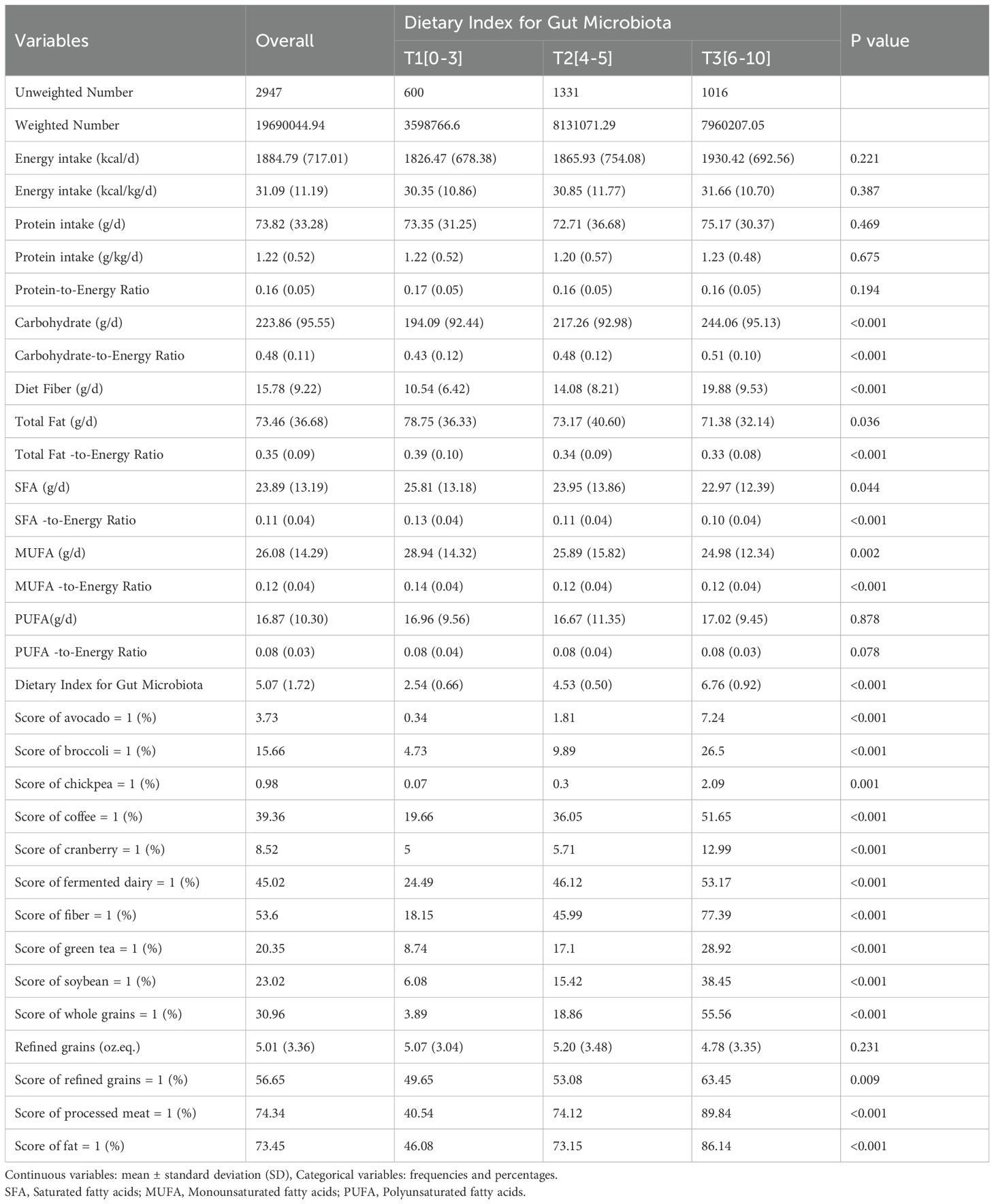
Table 2. Nutrient and food group daily intake across tertiles of the dietary index for gut microbiota in CKD patients.
Association between total DI-GM, the components details and serum albumin levels
Weighted univariable and multivariable analyses showed that each 1-point increase in DI-GM score was significantly associated with higher serum albumin levels across all models. In fully adjusted Model 3, each 1-point increase in DI-GM corresponded to a 0.18 g/L increase in serum albumin (95% CI, 0.07-0.28; P =0.002). When analyzed by tertiles, participants in T2 (DI-GM 4-5) and T3 (DI-GM 6-10) had significantly higher serum albumin levels compared with T1 (DI-GM 0-3), with the most pronounced increase in T3 at 0.82 g/L in Model 3 (95% CI, 0.32-1.33; P = 0.002) (Table 3).
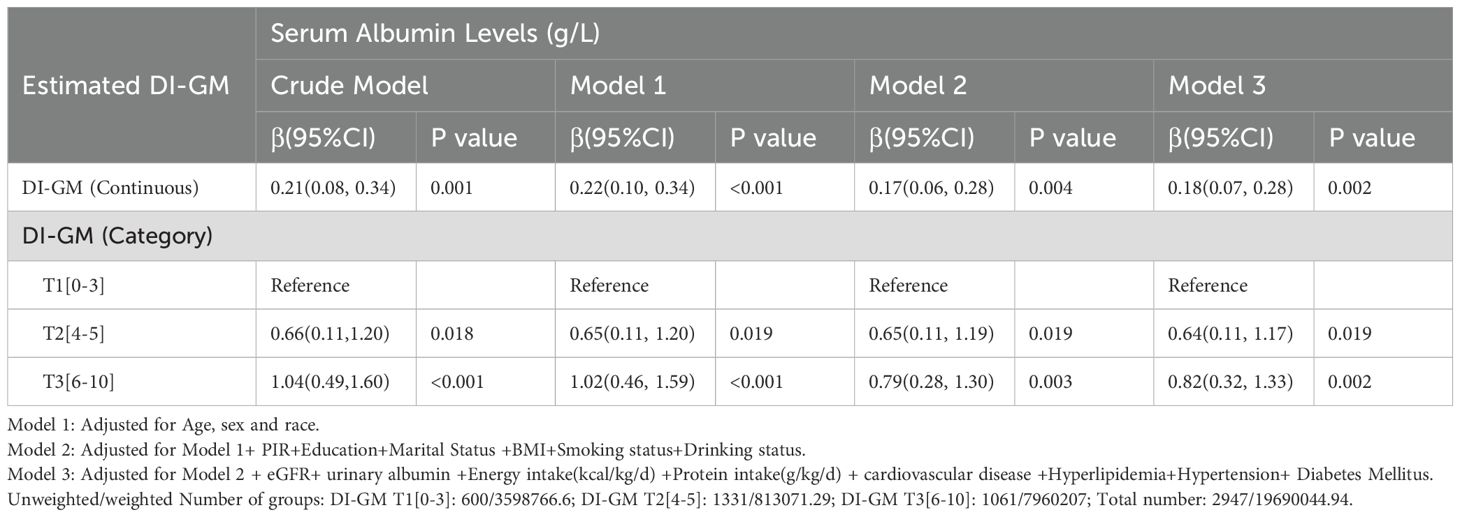
Table 3. Weighted univariable and multivariable analysis of the association between DI-GM and serum albumin levels in CKD patients.
For hypoalbuminemia (serum albumin < 38 g/L), weighted univariable and multivariable analyses indicated that each 1-point increase in DI-GM score was associated with a significant reduction in the odds of hypoalbuminemia across all models. In Model 3, each 1-point increase in DI-GM was associated with a 15% reduction in odds (OR, 0.85; 95% CI, 0.74-0.97; P = 0.014). Categorically, participants in T3 showed the strongest inverse association with hypoalbuminemia, with a 49% reduction in odds compared with T1 in Model 3 (OR, 0.51; 95% CI, 0.29-0.91; P =0.024) (Table 4).
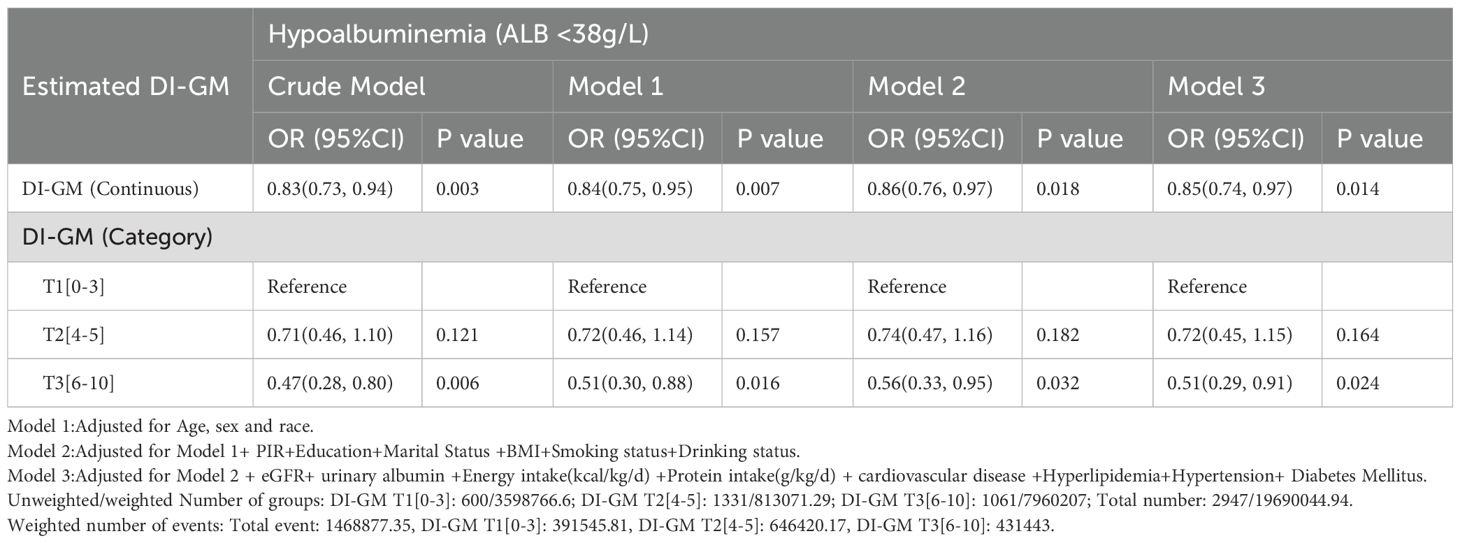
Table 4. Weighted univariable and multivariable analysis of the association between DI-GM and hypoalbuminemia in CKD patients.
The association between individual dietary components of the DI-GM and serum albumin levels showed, in the crude model, a score of 1 for refined grain intake was associated with a 0.56 g/L increase in serum albumin (β = 0.56, 95% CI: 0.13, 0.98, p = 0.01). This association remained significant in the adjusted model, with a 0.41 g/L increase in serum albumin (β = 0.41, 95% CI: 0.02, 0.80, p = 0.041). Regarding other components, in the crude model, only the intake of whole grains above the sex-specific median (i.e., a score of 1) was associated with a 0.51 g/L increase in serum albumin (β = 0.51, 95% CI: 0.02, 1.01, p = 0.042). However, in the adjusted model, this association was no longer significant (β = 0.41, 95% CI: -0.04, 0.85, p = 0.072). (Supplementary Table S2).
Association between score of refined grain, refined grain intake and serum albumin levels
A nuanced association between refined grain intake and serum albumin levels in CKD patients. Got the score of refined grains (score = 1) was positively associated with serum albumin levels across all models, with an increase of 0.41 g/L in the fully adjusted model (95% CI: 0.02, 0.80, p = 0.041). However, when analyzed as a continuous variable (oz. eq.), increased daily refined grain intake was inversely associated with serum albumin levels, with a 0.07 g/L decrease per ounce equivalent in the fully adjusted model (95% CI: -0.13, -0.01, p = 0.032) (Table 5).
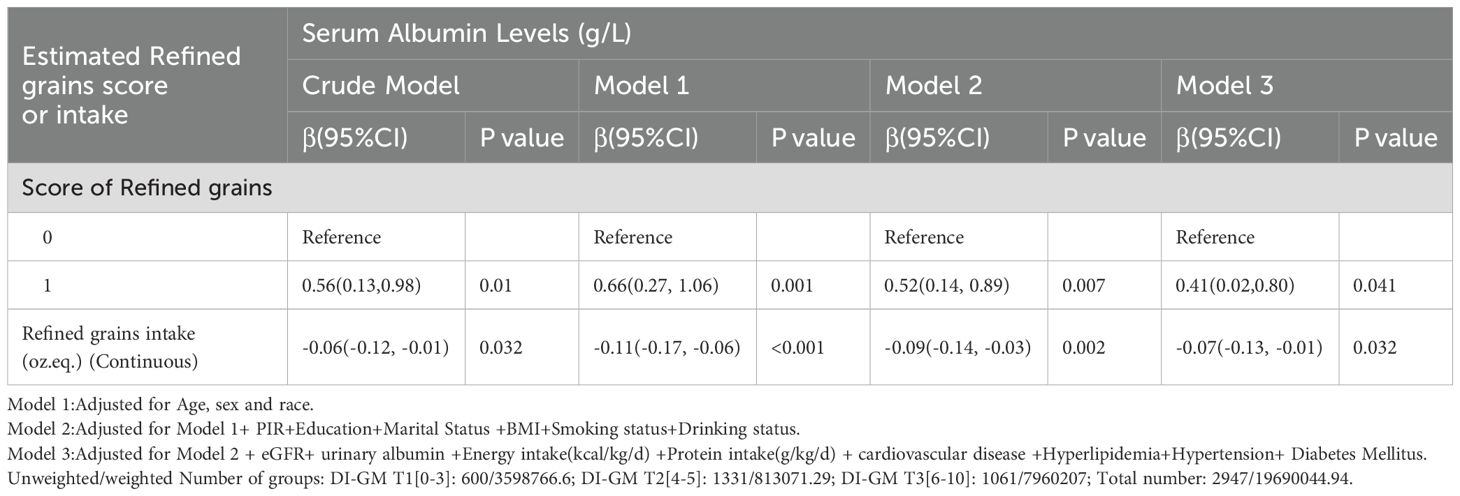
Table 5. Weighted univariable and multivariable analysis of the association between refined grains and serum albumin levels in CKD patients.
Sensitive analysis
We conducted RCS to find the nonlinear relationship between DI-GM, refined grains consumed and serum albumin level. As the DI-GM score increases beyond the reference point (DI-GM = 5), there is a significant increase in serum albumin levels.
A non-linear relationship between refined grains intake and serum albumin levels (p for non-linearity = 0.013, p for overall = 0.006) was also found. At the reference point of approximately 5.28 oz. eq. of refined grains intake per day, albumin levels remain relatively stable. However, increasing intake beyond this point is associated with a decrease in serum albumin levels. (Figure 2).
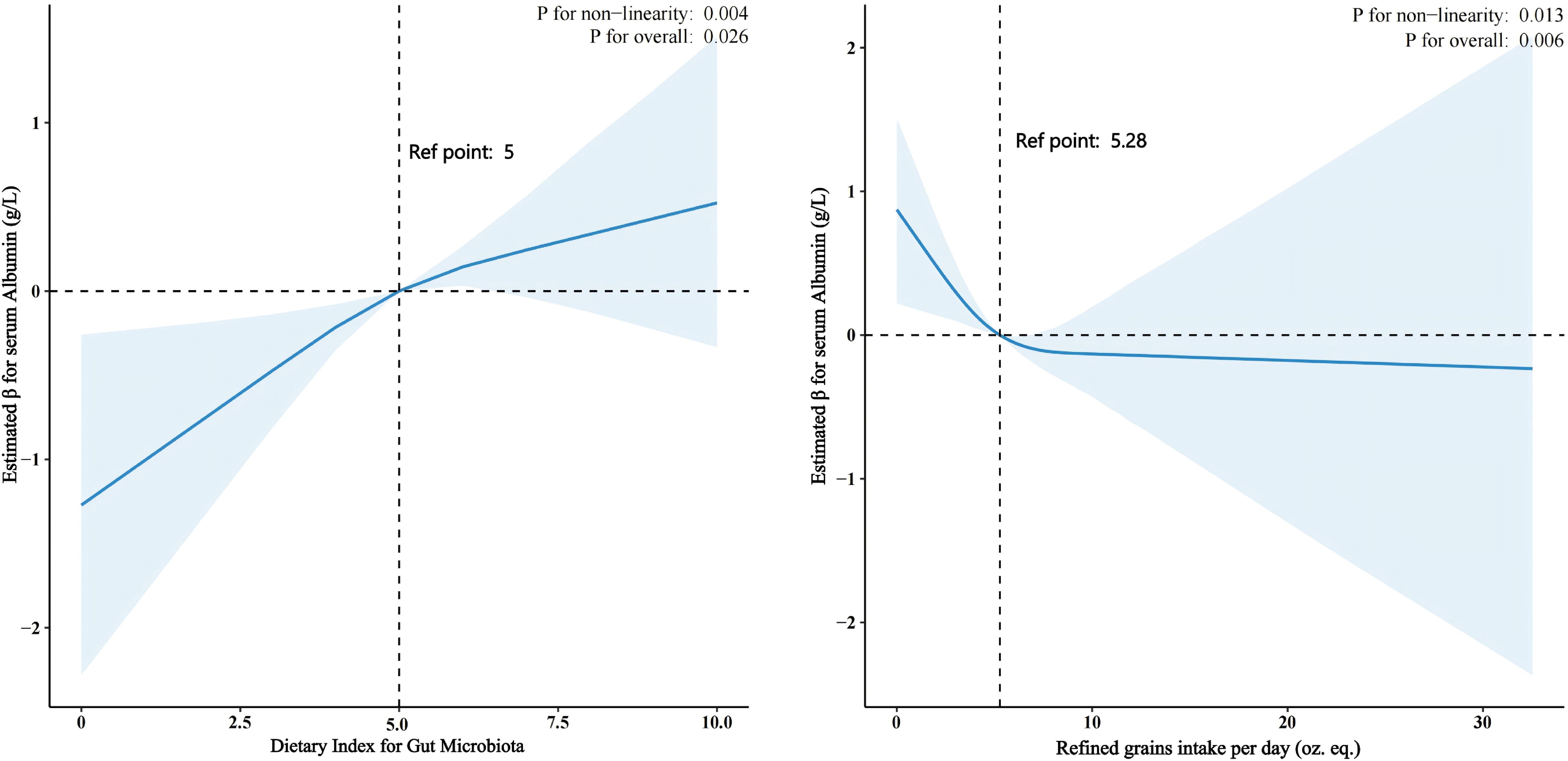
Figure 2. The Relationship Between Dietary Index for Gut Microbiota (DI-GM) and Refined Grains Intake with Serum Albumin Levels in CKD Patients Using Restricted Cubic Splines (RCS). DI-GM and Serum Albumin Levels: An RCS analysis revealed a nonlinear relationship (p = 0.004). Serum albumin levels significantly increase as the DI-GM score exceeds 5. Refined Grain Intake and Serum Albumin Levels: An RCS analysis showed a nonlinear relationship (p = 0.013). At approximately 5.28 oz. eq. of refined grains per day, albumin levels remain stable. However, intake beyond this point is associated with a decrease in serum albumin levels.
The positive association between DI-GM and serum albumin levels was consistent across most subgroups, with stronger effects observed in males, smokers, individuals with lower BMI, and those with diabetes. The significant interaction by smoking status suggests that smoking may modify the relationship between DI-GM and serum albumin. Refined grains intake is consistently associated with lower serum albumin levels across most subgroups, with notable variations in the strength of the association depending on age, BMI, and sex. (Figure 3).
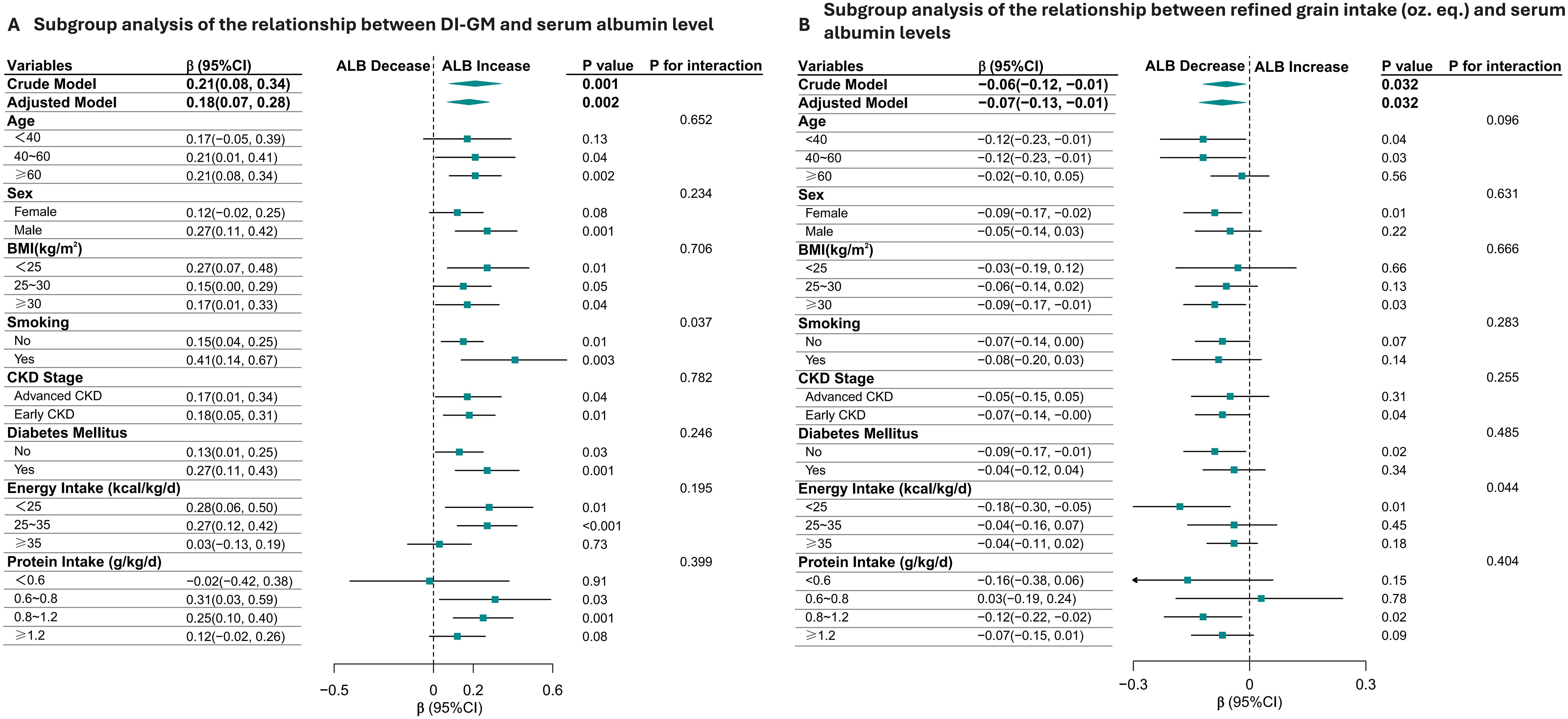
Figure 3. Subgroup Analysis of the relationship Between Dietary Index for Gut Microbiota (DI-GM), daily refined grain intake (oz.eq.) and Serum Albumin Levels in CKD Patients (A, B). The positive association between DI-GM and serum albumin levels was consistent across most subgroups, with stronger effects observed in males, smokers, individuals with lower BMI, and those with diabetes. The significant interaction by smoking status suggests that smoking may modify the relationship between DI-GM and serum albumin (A). Additionally, refined grain intake is consistently associated with lower serum albumin levels across most subgroups (B).
Discussion
In this cross-sectional study, we explored the association between the DI-GM and serum albumin levels in adults with CKD. Our findings indicate a significant positive association between higher DI-GM scores and increased serum albumin levels, with a consistent reduction in the odds of hypoalbuminemia across all models. Additionally, refined grain intake emerged as a key adverse factor, showing a negative relationship with serum albumin levels. These results provide novel insights into the potential role of gut microbiota-targeted dietary interventions in CKD management.
The presence of hypoalbuminemia indicates a poor prognosis and worsened clinical outcomes in patients with CKD, cancer, and infections (23–27), due to its correlation with inflammatory and catabolic processes. Dysbiosis of the gut microbiota is widely reported in CKD (28–30) and has been associated with systemic inflammation, oxidative stress (31–33), and reduced nutrient absorption by nutritional management and complications control (34). These factors collectively contribute to hypoalbuminemia and poor clinical outcomes in CKD patients (35–38). Our study supports previous findings by demonstrating that a high DI-GM diet, rich in fiber, whole grains, and plant-based foods, is associated with improved serum albumin levels. The anti-inflammatory and alkalizing effects of plant-based diets may help mitigate CKD-associated acidosis (39, 40) and chronic inflammation (7, 41), which are critical contributors to hypoalbuminemia.
Among the components of the DI-GM score, refined grains emerged as a notable factor: a score of 1 for refined grains was associated with elevated serum albumin levels (crude model: β=0.56, (95%CI:0.13,0.98); adjusted model: β=0.41, (95%CI:0.02,0.80)). To confirm the robustness of the association between a refined grain score of 1 in the DI-GM and serum albumin, we extended our analysis to investigate daily refined grain intake as a continuous variable. Intriguingly, increased refined grain intake was associated with decreased serum albumin levels. Notably, this inverse association persisted in sensitivity analyses across crude models (β=-0.06, (95%CI: -0.12, -0.01)) and models adjusted for covariates (β=-0.07, (95%CI: -0.13,-0.01)). The negative association between refined grain intake and serum albumin levels highlights the potential harm of diets high in processed carbohydrates. Refined grains may exacerbate gut microbiota dysbiosis by promoting the growth of pathogenic bacteria, leading to increased production of uremic toxins and systemic inflammation. This finding aligns with studies suggesting that diets with a higher ratio of refined to whole grains are associated with CKD progression and worse clinical outcomes (42, 43).
Our findings suggest that the high DI-GM diet alleviates hypoalbuminemia in CKD patients probably through a multi-target mechanism involving gut microbiota-derived metabolites, with significant clinical implications for patient management. At the molecular level, Lactobacillus enrichment (e.g., L. johnsonii) enhances indole-3-aldehyde production to antagonize AhR-mediated renal inflammation and fibrosis (30, 44), while increased short chain fatty acids (SCFAs) (e.g., butyrate) (45) attenuate oxidative stress and NF-κB activation via histone deacetylase inhibition and G protein-coupled receptor109A activation (46). Concurrently, reduced uremic toxins (indoxyl sulfate) and endotoxins (lipopolysaccharide) preserve glomerular filtration barrier integrity by suppressing reactive oxygen species/transforming growth factor- β1 (ROS/TGF-β1) signaling (46). This mechanistic framework is substantiated by broader dietary intervention studies: plant-based diets rich in fiber promote SCFA-producing bacteria (e.g., Roseburia) with anti-inflammatory properties (13, 47–49), whereas red meat-heavy diets exacerbate CKD progression by elevating protein fermentation products (indoxyl sulfate, p-cresyl sulfate) and uremic toxins that intensify inflammation (50–52), while simultaneously decreasing gut microbiota diversity (50).
Clinically, high DI-GM diet implementation represents a practical intervention to improve serum albumin levels, thereby reducing the risk of complications such as protein-energy wasting and cardiovascular events. Most notably, our subgroup analyses revealed that the benefits of this dietary approach were more pronounced in specific high-risk populations—males, smokers, and individuals with diabetes (53, 54)—suggesting opportunities for personalized nutritional strategies. Collectively, these findings underscore that targeted dietary modulation of gut microbiota composition and metabolic output constitutes a critical strategy for mitigating proteinuria and hypoalbuminemia in CKD, highlighting the importance of promoting microbiota-friendly dietary patterns in comprehensive CKD management, particularly for vulnerable patient subgroups.
Furthermore, the lower DI-GM scores observed in non-Hispanic Black individuals and those with lower educational attainment underscore the need for culturally tailored dietary education and interventions. Addressing socioeconomic and racial disparities in dietary quality is essential for improving health outcomes in CKD populations. Culturally appropriate dietary strategies and accessible nutrition education programs should be prioritized to ensure equitable health benefits.
Our study also highlights the role of dietary quality over quantity. Although energy and protein intake did not differ significantly across DI-GM tertiles, higher-quality diets with increased fiber and whole grain intake were associated with improved serum albumin levels. These findings emphasize the importance of dietary composition and nutrient density in managing CKD-related complications.
Despite its strengths, including the use of a nationally representative sample and comprehensive adjustment for confounders, this study has several limitations. First, the cross-sectional design precludes causal inferences between DI-GM and serum albumin levels. Longitudinal studies are needed to confirm these associations and explore underlying mechanisms. Second, dietary data were self-reported, which may introduce recall bias or underreporting, particularly among older adults. Third, while we adjusted for a wide range of confounders, residual confounding cannot be entirely ruled out.
Future research should focus on validating the long-term effects of DI-GM-based diets on serum albumin levels and other clinical outcomes in CKD patients. Interventional studies are also needed to establish causality and assess the feasibility of implementing DI-GM diets in clinical practice. Additionally, exploring the molecular mechanisms underlying the observed associations, such as changes in gut microbiota diversity, SCFA production, and inflammatory pathways, could provide valuable insights into the therapeutic potential of gut-targeted dietary interventions.
In conclusion, a high DI-GM diet may provide a promising dietary approach for managing hypoalbuminemia in CKD patients by modulating gut microbiota composition and reducing systemic inflammation. These findings underscore the importance of personalized dietary interventions in CKD care and suggest potential avenues for future research aimed at optimizing dietary strategies to improve clinical outcomes in this vulnerable population. By prioritizing microbiota-friendly dietary practices, clinicians can improve patient outcomes and address the underlying inflammatory and nutritional challenges associated with CKD.
Data availability statement
The original contributions presented in the study are included in the article/Supplementary Material. Further inquiries can be directed to the corresponding author.
Ethics statement
The studies involving humans were approved by Institutional Review Board of the National Center for Health Statistics. The studies were conducted in accordance with the local legislation and institutional requirements. The participants provided their written informed consent to participate in this study. Written informed consent was obtained from the individual(s) for the publication of any potentially identifiable images or data included in this article.
Author contributions
XW: Conceptualization, Data curation, Formal analysis, Methodology, Supervision, Validation, Visualization, Writing – original draft, Project administration, Software. PW: Conceptualization, Data curation, Formal analysis, Methodology, Supervision, Validation, Visualization, Writing – original draft, Software. FG: Data curation, Supervision, Validation, Writing – review & editing. JZ: Methodology, Supervision, Validation, Writing – review & editing. SM: Conceptualization, Data curation, Formal analysis, Methodology, Project administration, Supervision, Validation, Visualization, Writing – review & editing.
Funding
The author(s) declare that no financial support was received for the research and/or publication of this article.
Acknowledgments
We thank the Free Statistics team for providing technical assistance and valuable data analysis and visualization tools. In addition, Xiaoyan Wang especially wishes to thank all members of the team of Physician Scientist who have given her powerful spiritual support and encouragement. Thanks to Zhang Jing (Second Department of Infectious Disease, Shanghai Fifth People’s Hospital, Fudan University) for his work on the NHANES database. His outstanding work, nhanesR package and webpage, makes it easier for us to explore NHANES database.
Conflict of interest
The authors declare that the research was conducted in the absence of any commercial or financial relationships that could be construed as a potential conflict of interest.
Generative AI statement
The author(s) declare that no Generative AI was used in the creation of this manuscript.
Publisher’s note
All claims expressed in this article are solely those of the authors and do not necessarily represent those of their affiliated organizations, or those of the publisher, the editors and the reviewers. Any product that may be evaluated in this article, or claim that may be made by its manufacturer, is not guaranteed or endorsed by the publisher.
Supplementary material
The Supplementary Material for this article can be found online at: https://www.frontiersin.org/articles/10.3389/fimmu.2025.1546031/full#supplementary-material
Supplementary Table 1 | Components and scoring of the DI-GM (Source: Kase BE, et al. Nutrients. 2024;16(7):1045.).
Supplementary Table 2 | Weighted Univariable and Multivariable Analysis of Dietary Components’ score in the Dietary Index for Gut Microbiota and Their Association with Serum Albumin Levels.
References
1. Kovesdy CP. Epidemiology of chronic kidney disease: an update 2022. Kidney Int Suppl. (2022) 12:7–11. doi: 10.1016/j.kisu.2021.11.003
2. Kalantar-Zadeh K, Jafar TH, Nitsch D, Neuen BL, Perkovic V. Chronic kidney disease. Lancet. (2021) 398:786–802. doi: 10.1016/S0140-6736(21)00519-5
3. Zhang X, Fang Y, Zou Z, Hong P, Zhuo Y, Xu Y, et al. Risk factors for progression of CKD with and without diabetes. J Diabetes Res. (2022) 2022:1–12. doi: 10.1155/2022/9613062
4. Levitt D, Levitt M. Human serum albumin homeostasis: a new look at the roles of synthesis, catabolism, renal and gastrointestinal excretion, and the clinical value of serum albumin measurements. IJGM. (2016) 9:229–55. doi: 10.2147/IJGM.S102819
5. Suzuki Y, Suda K, Matsuyama Y, Era S, Soejima A. Close relationship between redox state of human serum albumin and serum cysteine levels in non-diabetic CKD patients with various degrees of renal function. CN. (2014) 82:320–5. doi: 10.5414/CN108040
6. Hanna RM, Ghobry L, Wassef O, Rhee CM, Kalantar-Zadeh K. A practical approach to nutrition, protein-energy wasting, sarcopenia, and cachexia in patients with chronic kidney disease. Blood Purif. (2020) 49:202–11. doi: 10.1159/000504240
7. Banerjee T, Sebastian A, Frassetto L. Association of diet-dependent systemic acid load, renal function, and serum albumin concentration. J Renal Nutr. (2023) 33:428–34. doi: 10.1053/j.jrn.2023.01.007
8. Kalim S, Zhao S, Tang M, Rhee EP, Allegretti AS, Nigwekar S, et al. Protein carbamylation and the risk of ESKD in patients with CKD. JASN. (2023) 34:876–85. doi: 10.1681/ASN.0000000000000078
9. Kaysen GA, Rathore V, Shearer GC, Depner TA. Mechanisms of hypoalbuminemia in hemodialysis patients. Kidney Int. (1995) 48:510–6. doi: 10.1038/ki.1995.321
10. Zheng HJ, Guo J, Wang Q, Wang L, Wang Y, Zhang F, et al. Probiotics, prebiotics, and synbiotics for the improvement of metabolic profiles in patients with chronic kidney disease: A systematic review and meta-analysis of randomized controlled trials. Crit Rev Food Sci Nutr. (2021) 61:577–98. doi: 10.1080/10408398.2020.1740645
11. Borges NA, Stenvinkel P, Bergman P, Qureshi AR, Lindholm B, Moraes C, et al. Effects of probiotic supplementation on trimethylamine-N-oxide plasma levels in hemodialysis patients: a pilot study. Probiotics Antimicro Prot. (2019) 11:648–54. doi: 10.1007/s12602-018-9411-1
12. Nguyen TTU, Kim HW, Kim W. Effects of probiotics, prebiotics, and synbiotics on uremic toxins, inflammation, and oxidative stress in hemodialysis patients: A systematic review and meta-analysis of randomized controlled trials. JCM. (2021) 10:4456. doi: 10.3390/jcm10194456
13. Yu Z, Zhao J, Qin Y, Wang Y, Zhang Y, Sun S. Probiotics, prebiotics, and synbiotics improve uremic, inflammatory, and gastrointestinal symptoms in end-stage renal disease with dialysis: A network meta-analysis of randomized controlled trials. Front Nutr. (2022) 9:850425. doi: 10.3389/fnut.2022.850425
14. Peters BA, Qi Q, Usyk M, Daviglus ML, Cai J, Franceschini N, et al. Association of the gut microbiome with kidney function and damage in the Hispanic Community Health Study/Study of Latinos (HCHS/SOL). Gut Microbes. (2023) 15:2186685. doi: 10.1080/19490976.2023.2186685
15. Harlacher E, Wollenhaupt J, Baaten CCFMJ, Noels H. Impact of uremic toxins on endothelial dysfunction in chronic kidney disease: A systematic review. IJMS. (2022) 23:531. doi: 10.3390/ijms23010531
16. Watral J, Formanowicz D, Perek B, Kostka-Jeziorny K, Podkowińska A, Tykarski A, et al. Comprehensive proteomics of monocytes indicates oxidative imbalance functionally related to inflammatory response in chronic kidney disease-related atherosclerosis. Front Mol Biosci. (2024) 11:1229648. doi: 10.3389/fmolb.2024.1229648
17. Ranganathan N, Anteyi E. The role of dietary fiber and gut microbiome modulation in progression of chronic kidney disease. Toxins. (2022) 14:183. doi: 10.3390/toxins14030183
18. Di Iorio BR, Rocchetti MT, De Angelis M, Cosola C, Marzocco S, Di Micco L, et al. Nutritional therapy modulates intestinal microbiota and reduces serum levels of total and free indoxyl sulfate and P-cresyl sulfate in chronic kidney disease (Medika study). JCM. (2019) 8:1424. doi: 10.3390/jcm8091424
19. Kase BE, Liese AD, Zhang J, Murphy EA, Zhao L, Steck SE. The development and evaluation of a literature-based dietary index for gut microbiota. Nutrients. (2024) 16:1045. doi: 10.3390/nu16071045
20. Stevens PE, Ahmed SB, Carrero JJ, Foster B, Francis A, Hall RK, et al. KDIGO 2024 clinical practice guideline for the evaluation and management of chronic kidney disease. Kidney Int. (2024) 105:S117–314. doi: 10.1016/j.kint.2023.10.018
21. Piccoli GB, Cederholm T, Avesani CM, Bakker SJL, Bellizzi V, Cuerda C, et al. Nutritional status and the risk of malnutrition in older adults with chronic kidney disease – implications for low protein intake and nutritional care: A critical review endorsed by ERN-ERA and ESPEN. Clin Nutr. (2023) 42:443–57. doi: 10.1016/j.clnu.2023.01.018
22. Ahluwalia N, Dwyer J, Terry A, Moshfegh A, Johnson C. Update on NHANES dietary data: focus on collection, release, analytical considerations, and uses to inform public policy. Adv Nutr. (2016) 7:121–34. doi: 10.3945/an.115.009258
23. Wang X, Chu H, Zhou H. Association between hypoalbuminemia and mortality in patients undergoing continuous renal replacement therapy: A systematic review and meta-analysis. PloS One. (2023) 18:e0283623. doi: 10.1371/journal.pone.0283623
24. Almasaudi AS, Dolan RD, Edwards CA, McMillan DC. Hypoalbuminemia reflects nutritional risk, body composition and systemic inflammation and is independently associated with survival in patients with colorectal cancer. Cancers. (2020) 12:1986. doi: 10.3390/cancers12071986
25. Oster HS, Dolev Y, Kehat O, Weis-Meilik A, Mittelman M. Serum hypoalbuminemia is a long-term prognostic marker in medical hospitalized patients, irrespective of the underlying disease. JCM. (2022) 11:1207. doi: 10.3390/jcm11051207
26. Soetedjo NNM, Iryaningrum MR, Damara FA, Permadhi I, Sutanto LB, Hartono H, et al. Prognostic properties of hypoalbuminemia in COVID-19 patients: A systematic review and diagnostic meta-analysis. Clin Nutr ESPEN. (2021) 45:120–6. doi: 10.1016/j.clnesp.2021.07.003
27. Claps F, Rai S, Mir MC, van Rhijn BWG, Mazzon G, Davis LE, et al. Prognostic value of preoperative albumin-to-fibrinogen ratio (AFR) in patients with bladder cancer treated with radical cystectomy. Urologic Oncology: Semin Original Investigations. (2021) 39:835.e9–835.e17. doi: 10.1016/j.urolonc.2021.04.026
28. Martín-del-Campo F, Avesani CM, Stenvinkel P, Lindholm B, Cueto-Manzano AM, Cortés-Sanabria L. Gut microbiota disturbances and protein-energy wasting in chronic kidney disease: a narrative review. J Nephrol. (2023) 36:873–83. doi: 10.1007/s40620-022-01560-1
29. Evenepoel P, Stenvinkel P, Shanahan C, Pacifici R. Inflammation and gut dysbiosis as drivers of CKD–MBD. Nat Rev Nephrol. (2023) 19:646–57. doi: 10.1038/s41581-023-00736-7
30. Miao H, Liu F, Wang Y-N, Yu X-Y, Zhuang S, Guo Y, et al. Targeting Lactobacillus johnsonii to reverse chronic kidney disease. Sig Transduct Target Ther. (2024) 9:195. doi: 10.1038/s41392-024-01913-1
31. Rysz J, Franczyk B, Ławiński J, Olszewski R, Ciałkowska-Rysz A, Gluba-Brzózka A. The impact of CKD on uremic toxins and gut microbiota. Toxins. (2021) 13:252. doi: 10.3390/toxins13040252
32. Sumida K, Lau WL, Kovesdy CP, Kalantar-Zadeh K, Kalantar-Zadeh K. Microbiome modulation as a novel therapeutic approach in chronic kidney disease. Curr Opin Nephrol Hypertens. (2021) 30:75–84. doi: 10.1097/MNH.0000000000000661
33. Ondrussek-Sekac M, Navas-Carrillo D, Orenes-Piñero E. Intestinal microbiota alterations in chronic kidney disease and the influence of dietary components. Crit Rev Food Sci Nutr. (2021) 61:1490–502. doi: 10.1080/10408398.2020.1761771
34. Vaziri ND, Zhao Y-Y, Pahl MV. Altered intestinal microbial flora and impaired epithelial barrier structure and function in CKD: the nature, mechanisms, consequences and potential treatment. Nephrol Dial Transplant. (2016) 31:737–46. doi: 10.1093/ndt/gfv095
35. Alves FC, Sun J, Qureshi AR, Dai L, Snaedal S, Bárány P, et al. The higher mortality associated with low serum albumin is dependent on systemic inflammation in end-stage kidney disease. PLoS One. (2018) 13:e0190410. doi: 10.1371/journal.pone.0190410
36. Song H, Wei C, Hu H, Wan Q. Association of the serum albumin level with prognosis in chronic kidney disease patients. Int Urol Nephrol. (2022) 54:2421–31. doi: 10.1007/s11255-022-03140-5
37. Cheng T, Wang X, Han Y, Hao J, Hu H, Hao L. The level of serum albumin is associated with renal prognosis and renal function decline in patients with chronic kidney disease. BMC Nephrol. (2023) 24:57. doi: 10.1186/s12882-023-03110-8
38. Zhao BR, Hu XR, Wang WD, Zhou Y. Cardiorenal syndrome: clinical diagnosis, molecular mechanisms and therapeutic strategies. Acta Pharmacol Sin. (2025). doi: 10.1038/s41401-025-01476-z
39. Freeman NS, Turner JM. In the “Plant-based” Era, patients with chronic kidney disease should focus on eating healthy. J Renal Nutr. (2024) 34:4–10. doi: 10.1053/j.jrn.2023.08.010
40. Rebholz CM, Coresh J, Grams ME, Steffen LM, Anderson CAM, Appel LJ, et al. Dietary acid load and incident chronic kidney disease: results from the ARIC study. Am J Nephrol. (2015) 42:427–35. doi: 10.1159/000443746
41. Xu Z, Li L, Jiang L, Zhai Y, Tang Y, Liu D, et al. Association of Dietary Inflammatory Index with CKD progression and estimated glomerular filtration rate in the American CKD population: A cross-sectional study. PloS One. (2024) 19:e0297916. doi: 10.1371/journal.pone.0297916
42. Xu Z-H, Qiu C-S, Qi J, Tang X-L, Li H-M, Zhang L-W, et al. Association between whole grain intake and chronic kidney disease. J Nutr. (2024) 154:1262–70. doi: 10.1016/j.tjnut.2024.02.013
43. Mazidi M, Katsiki N, Mikhailidis DP, Banach M. A higher ratio of refined grain to whole grain is associated with a greater likelihood of chronic kidney disease: a population-based study. Br J Nutr. (2019) 121:1294–302. doi: 10.1017/S0007114518003124
44. Miao H, Wang Y, Yu X, Zou L, Guo Y, Su W, et al. Lactobacillus species ameliorate membranous nephropathy through inhibiting the aryl hydrocarbon receptor pathway via tryptophan-produced indole metabolites. Br J Pharmacol. (2024) 181:162–79. doi: 10.1111/bph.16219
45. Zheng L, Luo M, Zhou H, Chen J. Natural products from plants and microorganisms: Novel therapeutics for chronic kidney disease via gut microbiota regulation. Front Pharmacol. (2022) 13:1068613. doi: 10.3389/fphar.2022.1068613
46. Li X-J, Shan Q-Y, Wu X, Miao H, Zhao Y-Y. Gut microbiota regulates oxidative stress and inflammation: a double-edged sword in renal fibrosis. Cell Mol Life Sci. (2024) 81:480. doi: 10.1007/s00018-024-05532-5
47. Du X, Wu J, Gao C, Tan Q, Xu Y. Effects of resistant starch on patients with chronic kidney disease: A systematic review and meta-analysis. J Diabetes Res. (2022) 2022:1–11. doi: 10.1155/2022/1861009
48. de Paiva BR, Esgalhado M, Borges NA, Kemp JA, Alves G, Leite PEC, et al. Resistant starch supplementation attenuates inflammation in hemodialysis patients: a pilot study. Int Urol Nephrol. (2020) 52:549–55. doi: 10.1007/s11255-020-02392-3
49. Esgalhado M, Kemp JA, Azevedo R, Paiva BR, Stockler-Pinto MB, Dolenga CJ, et al. Could resistant starch supplementation improve inflammatory and oxidative stress biomarkers and uremic toxins levels in hemodialysis patients? A pilot randomized controlled trial. Food Funct. (2018) 9:6508–16. doi: 10.1039/C8FO01876F
50. Wan Y, Wang F, Yuan J, Li J, Jiang D, Zhang J, et al. Effects of dietary fat on gut microbiota and faecal metabolites, and their relationship with cardiometabolic risk factors: a 6-month randomised controlled-feeding trial. Gut. (2019) 68:1417–29. doi: 10.1136/gutjnl-2018-317609
51. Takkavatakarn K, Phannajit J, Udomkarnjananun S, Tangchitthavorngul S, Chariyavilaskul P, Sitticharoenchai P, et al. Association between indoxyl sulfate and dialysis initiation and cardiac outcomes in chronic kidney disease patients. IJNRD. (2022) 15:115–26. doi: 10.2147/IJNRD.S354658
52. Rossi M, Johnson DW, Xu H, Carrero JJ, Pascoe E, French C, et al. Dietary protein-fiber ratio associates with circulating levels of indoxyl sulfate and p-cresyl sulfate in chronic kidney disease patients. Nutrition Metab Cardiovasc Dis. (2015) 25:860–5. doi: 10.1016/j.numecd.2015.03.015
53. Yan S, Ma Z, Jiao M, Wang Y, Li A, Ding S. Effects of smoking on inflammatory markers in a healthy population as analyzed via the gut microbiota. Front Cell Infect Microbiol. (2021) 11:633242. doi: 10.3389/fcimb.2021.633242
Keywords: CKD, dietary index for gut microbiota, serum albumin, hypoalbuminemia, dietary pattern
Citation: Wang X, Wen P, Gao F, Zhao J and Miao S (2025) Microbiota-friendly diet ameliorates hypoalbuminemia in chronic kidney disease: evidence from NHANES. Front. Immunol. 16:1546031. doi: 10.3389/fimmu.2025.1546031
Received: 16 December 2024; Accepted: 07 April 2025;
Published: 06 May 2025.
Edited by:
Sandip K. Wagh, Sandip University, IndiaReviewed by:
Lin Chen, Northwest University, ChinaLuca Ongaro, Royal Free Hospital, United Kingdom
Haoxian Tang, First Affiliated Hospital of Shantou University Medical College, China
Copyright © 2025 Wang, Wen, Gao, Zhao and Miao. This is an open-access article distributed under the terms of the Creative Commons Attribution License (CC BY). The use, distribution or reproduction in other forums is permitted, provided the original author(s) and the copyright owner(s) are credited and that the original publication in this journal is cited, in accordance with accepted academic practice. No use, distribution or reproduction is permitted which does not comply with these terms.
*Correspondence: Shuchuan Miao, bWlhb3NodWNodWFuMEAxMjYuY29t
†These authors have contributed equally to this work and share first authorship