- 1Department of Health Inspection and Quarantine, School of Public Health, Cheeloo College of Medicine, Shandong University, Jinan, China
- 2Department of Clinical Laboratory Medicine, The First Affiliated Hospital of Shandong First Medical University & Shandong Provincial Qianfoshan Hospital, Shandong Medicine and Health Key Laboratory of Laboratory Medicine, Jinan, Shandong, China
- 3Department of Pathogen Biology, School of Clinical and Basic Medicine, Shandong First Medical University and Shandong Academy of Medical Sciences, Jinan, China
- 4School of Stomatology, Shandong First Medical University and Shandong Academy of Medical Sciences, Jinan, China
Gender differences exist in the susceptibility, incidence, progression, and prognosis of diseases caused by viral infections. These differences are influenced by various factors, including lifestyle and habits between males and females. Some reports have pointed out that estrogen plays an important immune-modulatory role in many viral infections. In certain viral infections, estrogen exhibits a protective and regulatory effect, while in others, it has a synergistic and promoting effect. The action of estrogen and its receptors affect the occurrence and prognosis of viral infections to some extent. This article reviews the correlation and the related mechanisms between estrogen and viral infections.
1 Introduction
Pathogenic microorganism infections, particularly viral infections, pose a significant threat to human health (1). In recent years, a number of emerging infectious diseases caused by viral infections, including novel coronaviruses, influenza viruses, and others, have emerged, leading to a major public health crisis (2, 3). Although many factors lead to infection, epidemiological data showed that there are gender differences in many pathogenic microorganism infections (4–7). In some viral infection, men showed higher infection susceptibility and worse infection prognosis compared with women. While various factors, such as variations in immune responses and lifestyle habits between genders, may contribute to this phenomenon, growing research suggests that estrogen plays a critical role in viral infections and the associated molecular mechanisms.
This review provides a summary of current research on estrogen-related viral infections and the associated molecular mechanisms.
2 Estrogen
Estrogen is a sex steroid hormone that plays a crucial role in the development of female secondary sexual characteristics and the regulation of the reproductive system in adult women. It also demonstrates a wide range of functions and impacts in various aspects (8).Several studies had indicated that virus infection modulated the estrogen expression in host cells (9). Furthermore, estrogen levels impact the host’s susceptibility and immune response to viruses (10). The types of estrogen in the human body include estrone (E1), estradiol (E2), estriol (E3) and estetrol (E4), with estradiol being the most active (8, 11).Estrogen exerts its biological effects primarily by binding to estrogen receptors (ERs). The classical ERs is a nuclear receptor consisting of ERα and ERβ isoforms. Additionally, estrogen can also bind to membrane receptors and exert indirect transcriptional regulatory functions through the second messenger (12).
3 Estrogen and virus
3.1 Human immunodeficiency virus
HIV, a single-stranded RNA virus belonging to the lentivirus genus of the family Retroviridae (13), causes destruction of the human immune system, particularly CD4+ lymphocytes. This leads to a decrease or loss of immune function and renders the body highly vulnerable to other pathogenic microorganisms. HIV infection is caused by one of two types of retroviruses, HIV-1 and HIV-2. HIV-1 is responsible for the majority of global HIV infections.
Global data suggests that females are more susceptible to HIV-1 infection compared to males, with the reproductive tract mucosa serving as the portal of entry for HIV (14, 15). Estrogen therapy enhances the natural protective barrier of the reproductive tract tissues, effectively preventing the transmission of HIV-1 (16). Mechanisms of estrogen action include increased production of cervical mucus, reduced cervical ectopy, and alteration of vaginal bacterial communities, thereby enhancing anti-HIV infectivity (17).
Studies have found that, compared to males, females exhibit stronger interferon-inducible gene expression in response to HIV-1 infection at the same viral load levels, indicating a more robust immune activation (18). Estrogen plays a crucial role in regulating innate immune function, as evidenced by 17β-estradiol therapy enhancing TLR7-dependent IFNα production in postmenopausal women dendritic cells, thus preventing systemic infection (18). Interestingly, estrogen exhibits protective effects against HIV-1 infection, while progesterone increases susceptibility to HIV-1 (16, 19). Experiments have shown that female macaques exposed to vaginal SIV are less susceptible during the follicular phase of the menstrual cycle (when estrogen levels are higher) compared to the luteal phase (when progesterone levels are higher). During the proliferative phase of the menstrual cycle, elevated progesterone levels typically suppress the immune system, providing opportunities for virus invasion and colonization. Therefore, the high levels of progesterone during the luteal phase may lead to a “susceptibility window” for HIV infection, increasing the risk of vaginal transmission in menstruating women (20, 21). Furthermore, peak estrogen levels downregulate adhesion molecules, reducing the recruitment of inflammatory T cells and macrophages. Progesterone increases the expression of CCR5 on human cervical CD4+ T cells, making females more susceptible (21).
E2 and lactobacilli alleviate the adverse effects of HIV-1 on barrier function of epithelial cells in the reproductive tract and pro-inflammatory cytokine production (22). Estrogen regulates innate immune and inflammatory responses in the female genital tract (FGT), playing a predominant role in reducing local inflammation, and exerting significant anti-inflammatory effects on epithelial cells, such as reducing the secretion of TNF-α, IL-1α, and IL-8 (22, 23). Lactobacilli provide nonspecific defense in the vagina, promoting the immune competence of FGT. Estrogen stimulates the maturation and proliferation of vaginal epithelial cells and accumulation of glycogen, favoring the proliferation of lactobacilli. Lactobacilli produce lactic acid and lower pH to resist HIV invasion (22–24).
Local application of estrogen can also increase the production of keratin proteins in the male foreskin epithelial cells and increase the thickness of the protective layer of epithelial cells, thereby reducing the viral load in contact with Langerhans cells (25, 26), thus contributing to enhanced resistance to HIV infection in males.
HIV also infects astrocytes and microglia, causing neurotoxicity. Infected glial cells can release viral proteins, leading to neuronal damage and resulting in cognitive impairment in patients (13, 27). HIV-1 Tat protein activates HIV-1 LTR-directed gene expression by directly interacting with the transcriptional activation response (TAR) element in the long terminal repeat (LTR) and other cis-acting elements in LTR, thereby promoting HIV-1 infection (28, 29). Studies have shown that physiological levels of E2 and ER-α agonists can inhibit Tat-induced HIV LTR transcription in human astrocytes (27, 30). E2 also regulates neuronal growth by mediating neurite outgrowth and reducing cell apoptosis signals induced by Tat via Erβ (31). Furthermore, exposure to HIV has been shown to decrease the expression of tight junction proteins such as ZO-1, claudin-5, and occludin, thereby disrupting the blood-brain barrier (32). Estrogen can maintain the integrity of the blood-brain barrier by inducing the expression of Sonic hedgehog signaling protein through the activation of the ERα pathway (33, 34).
Estrogen can also alter neuronal anti-apoptotic signaling pathways in a receptor-dependent manner to promote neuronal survival, provide nutritional support to neurons and glial cells, promote the expression of nerve growth factor and its receptor, inhibit neuronal inflammation, and exert antioxidant and anti-inflammatory effects (35–37). The mechanism of estrogen action is illustrated in area A of Figure 1.
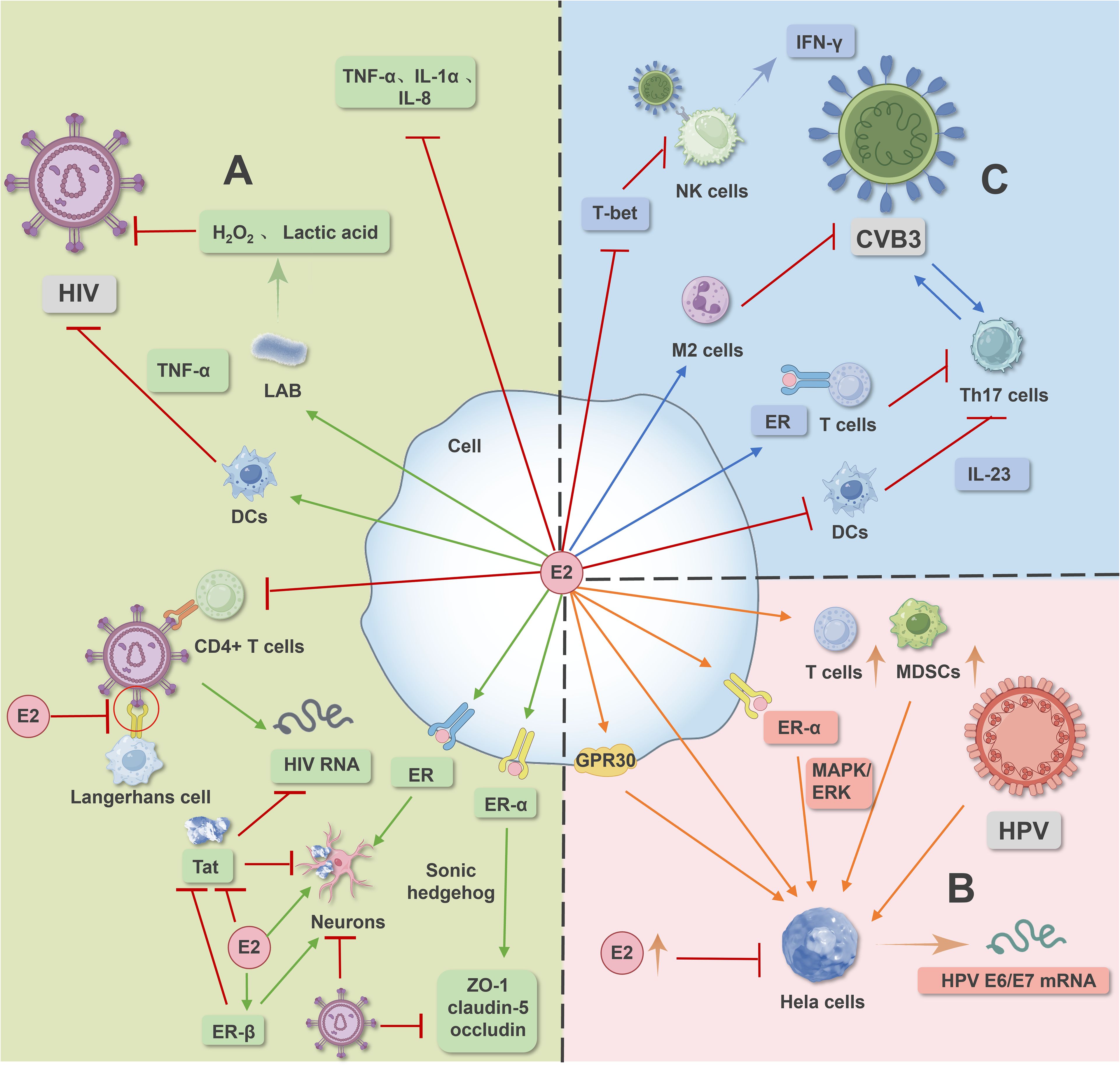
Figure 1. Image 1 illustrates the signaling pathways mediated by estrogen and its receptors in HIV, HPV, and CVB virus infections. In HIV infection (A), estrogen and its receptors modulate signaling pathways to counteract HIV virus-induced infection. In HPV infection (B), estrogen and its receptor-mediated signaling pathways promote human papillomavirus infection and facilitate the occurrence and development of cervical cancer. In CVB virus infection (C), estrogen and its receptor-mediated signaling pathways, along with macrophage polarization and dendritic cell inhibition, regulate CVB infection.
3.2 Human papilloma virus
HPV, a DNA virus belonging to the family Papillomaviridae, is associated with various skin infections and is a major etiological factor in cervical cancer. This virus encodes potent oncogenes E6 and E7 (38–40). Despite numerous types of papillomaviruses associated with reproductive tract lesions and malignancies, mere HPV infection alone is insufficient to induce malignant transformation (41, 42).
During HPV infection, estrogen and its receptors play pivotal roles in the occurrence and progression of cervical cancer. Studies indicate that elevated estrogen levels in women with high-risk HPV infections can promote the onset and progression of cervical cancer, with pregnant women diagnosed with cervical cancer having a higher risk of mortality (43–45). Estrogen stimulates HPV16 transcription and increases E6/E7 mRNA levels in cervical cancer cells. Moreover, transfection and immortalization of cells with HPV16 increase 16α-hydroxyestrone, a mechanism through “autocrine” means to prolong estrogen action and enhance cell proliferation. The synergistic effects of HPV and 16α-hydroxylation promote cervical cancer cell proliferation. Progesterone-responsive elements are also observed in the viral promoter region of the HPV16 genome, where progesterone can increase HPV mRNA levels and stimulate viral replication (42, 46–48). The loss of p53, in conjunction with prolonged estrogen exposure and cooperation with HPV oncogenes, promotes the development of cervical and mammary cancers, as well as squamous cell carcinomas in the genital tract (49–51). HPV infection is also recognized as one of the risk factors for head and neck squamous cell carcinoma (HNSCC), with male sex hormones exhibiting potential toxic effects in the development and progression of HNSCC (52).
ERα is essential for the progression and maintenance of cervical cancer in mice, mediating estrogen-stimulated MAPK/ERK signaling pathways, thereby promoting invasion, migration, and proliferation of cervical cancer cell lines. Blocking estrogen receptors can prevent and treat cervical cancer (53–56). Estrogen can also mediate G protein-coupled receptor 30 (GPR30) involvement in the progression of cervical adenocarcinoma (57). GPR30 expression in normal endometrial glands, E2 through GPR30 increases proliferation of cervical columnar and glandular cancer cells, and induces genomic instability exacerbating HPV infection (58). In addition to directly promoting the growth of cervical epithelial cells, estradiol also enhances the function of stromal and infiltrating immunosuppressive cells such as Tregs, MDSCs, and cancer-associated fibroblasts, indirectly promoting tumor growth (45). However, studies have also shown that exogenous high concentrations of estradiol can inhibit the proliferation of HeLa cells and induce apoptosis in HPV-positive cervical cancer cells (45, 59). Dihydrotestosterone can slow down the proliferation of transduced androgen receptor W12 cells and promote the expression of squamous differentiation-related genes, thereby helping to slow the development of cervical epithelial neoplasia (60).The mechanism of estrogen action is illustrated in area B of Figure 1.
3.3 Coxsackie virus B
CV, known as Coxsackievirus, belongs to the genus enterovirus. It is a non-enveloped, linear, single-stranded RNA virus, which is classified into two subtypes: group A and group B (61). Group A consists of one serotype, while group B consists of six serotypes: CVB1, CVB2, CVB3, CVB4, CV-B5, and CV-B6 (62).
Viral myocarditis is typically caused by viral infections, with one of the most common pathogenic viruses being Coxsackievirus B3 (CVB3), which exhibits gender disparities (63, 64). In males, the incidence and mortality rates of CVB3 infection are higher, possibly due to testosterone enhancement and estrogen suppression, leading to the systemic progression of the disease (65, 66). Following CVB3 infection, male mice generate a virus-specific soluble T-cell response, thereby accelerating disease progression, which may be associated with testosterone levels, while estrogen can protect female mice from exacerbating responses to CVB infection by stimulating antibody production (65).
In males infected with CVB3, T cells play a significant role in the pathogenesis of CVB3-induced myocarditis. More Th17 cells are produced in male mice infected with CVB3, correlating with viral load. Th17 cells adversely affect myocardial cells by promoting virus replication and the production of pro-inflammatory cytokines (TNF-α and IL-1β), exacerbating CVB3-induced myocarditis (66). Compared to testosterone’s negligible effect on Th17 cell induction, estrogen can suppress CVB3-induced Th17 cells by directly interacting with ER-α on T cells or indirectly impairing IL-23 produced by dendritic cells (66, 67).
CVB3 stimulation induces NK cell production of IFN-γ, with male mice being more susceptible to CVB3-induced myocarditis. High doses of estradiol during the menstrual cycle can inhibit NK activity and its mediated cytotoxicity (68). Estrogen affects the production of IFN-γ in NK cells stimulated by CVB3 by downregulating the expression of Th1-specific T-box transcription factor (T-bet), thereby reducing cellular infiltration in male mice and preventing further myocarditis progression (63, 69).
In CVB3-induced myocarditis, there are gender differences in macrophage infiltration phenotypes. M1-type macrophages can produce pro-inflammatory responses and related factors, while M2-type macrophages can produce anti-inflammatory responses and repair damaged tissues (70). Male mice mainly exhibit M1 phenotype macrophage infiltration, while female mice mainly exhibit M2 phenotype macrophage infiltration, and high levels of IFN-γ can also inhibit M2 polarization in male mice (71). Studies have shown that E2 can polarize macrophages towards the M2 phenotype, thereby slowing down the progression of diseases induced by CVB infection (72). Additionally, autophagy can improve cardiac function by limiting myocardial cell death during acute ischemia-reperfusion injury (73–75), with studies indicating that GPR30 can regulate autophagy via the AKT/mTOR signaling pathway to protect myocardial cells (76). The mechanism of estrogen action is illustrated in area C of Figure 1.
3.4 Hepatitis virus
Hepatitis viruses are the primary pathogens responsible for viral hepatitis. Among them, HAV, HBV, HCV, HDV, and HEV constitute the major global burden of viral hepatitis (77, 78). Research has identified a correlation between estrogen and the infections caused by Hepatitis B virus (HBV), Hepatitis C virus (HCV) and Hepatitis E virus (HEV) (79).
HBV infection leads to hepatocellular degeneration and necrosis, accompanied by varying degrees of inflammatory cell infiltration, which may progress to hepatitis, cirrhosis, and even hepatocellular carcinoma (HCC) (80). Epidemiological investigations indicate that the incidence and prognosis of HBV-related cirrhosis (HBV-LC) and hepatocellular carcinoma (HBV-HCC) are more severe in males and postmenopausal females, possibly due to changes in estrogen levels (79, 81, 82).
HBV affects mitochondrial function to generate reactive oxygen species (ROS) altering the redox state of cells and activate transcription factors to influence virus replication (83). Studies have shown that ER-α can reduce the DNA binding capacity of HNF-4α to the core region of enhancer I, thereby reducing viral load and decreasing the incidence of liver cancer in females infected with HBV (80, 84, 85). Additionally, E2 can protect damaged liver cells by inhibiting the activity of NADH/NADPH oxidase, thereby preventing the secretion of reactive oxygen species (ROS) and TGF-β (81, 86).
Estrogen can enhance the immune activity of NK cells in women infected with HBV through multiple pathways, helping the body clear the virus (87). E2 can also regulate cytokines, attenuate the induction of redox-sensitive transcription factors, and alleviate HBV-related liver damage to slow disease progression (81, 88). The sodium taurocholate cotransporting polypeptide (NTCP) is a functional receptor for HBV infection of liver cells (89). Estrogen can downregulate the expression of NTCP on the surface of liver cells in HBV patients, thereby inhibiting the ability of HBV to infect liver cells (90).
E2 is also considered a protective factor for the progression of fibrosis in patients with chronic hepatitis (91). HBV infection activates hepatic stellate cells, leading to their transformation into myofibroblast-like cells (92). E2 can inhibit the activation of hepatic stellate cells (HSCs) and thus suppress liver fibrosis (81). Chronic HBV infection can cause hepatic steatosis, and the accumulation of hepatic lipids can affect liver fibrosis, inflammation, apoptosis, and cancer (81, 93, 94). Estrogen prevents liver fibrosis caused by hepatic steatosis by altering fat distribution in men to more closely resemble the subcutaneous fat distribution in women (95).
HBV-related HCC is a severe consequence of chronic HBV infection, and estrogen plays an important role in the development of HCC (80). Constitutive activation of the transcription factor STAT3 is observed in the liver tissues and HCC cell lines of nearly half of HCC patients, and it is an important factor inducing cell death (96, 97). Estrogen plays an anticancer or antitumor role by mediating ER-α to inhibit the activation of NF-κB and the release of IL-6, and by attenuating the sustained activity of STAT3 in liver cells (80). In addition, ER-α can inhibit the activity of STAT3 by enhancing protein tyrosine phosphatase receptor O (PTPRO) and the tumor suppressor gene SORBS3α on chromosome 8. The NLR family pyrin domain containing 3 (NLRP3) inflammasome is a critical factor in HCC tissue (98). NLRP3 inflammasome is regulated by the ERβ/mitogen-activated protein kinase (MAPK) pathway, and E2 regulates this pathway to inhibit the proliferation, migration, and colony formation ability of HCC cells (80). Furthermore, estrogen can inhibit the activation of inflammasomes and the transformation activation of tumor-associated macrophages through its receptors, thereby suppressing the growth of HCC (99, 100).
Hepatitis C virus (HCV) is a positive-sense RNA virus that infects the host and leads to the development of hepatitis C. The progression of liver fibrosis and cirrhosis associated with HCV infection is closely related to the development of chronic liver diseases, ultimately culminating in hepatocellular carcinoma (101, 102). Studies have demonstrated that females infected with HCV exhibit higher rates of viral clearance and slower disease progression, indicating a protective role of estrogen in the chronic course of hepatitis C (103, 104). Some protective mechanisms parallel those observed in chronic hepatitis B virus infection: estrogen can inhibit the proliferation and fibrosis of hepatic stellate cells (105).
17β-Estradiol can regulate hepatic cell type I interferon to control HCV infection and mediate ERα inhibition of mature HCV viral particle production (79, 104, 106). Additionally, 17β-estradiol can interfere with the HCV lifecycle through its intracellular receptors, disrupting both early (viral entry) and late (assembly/release) stages of the HCV lifecycle, significantly reducing particle release (107). G protein-coupled estrogen receptor 1 (GPER) is a membrane receptor that specifically binds estrogen and exerts rapid effects on target cells (12). HCV can enter cells via the tight junction protein occludin. Estrogen can bind to GPER, inducing metalloproteinase 9 (MMP) cleavage of tight junction protein occludin to inhibit HCV entry into hepatocytes (108). Estrogen also activates the sterol regulatory element-binding protein (SREBP) pathway, which may interfere with the viral release stage (109). SREBP can regulate microsomal triglyceride transfer protein (MTP), affecting the proper release of infectious particles and regulating apolipoprotein on newly formed particles (110–112).
Research suggests that E2 prevents the accumulation of monocytes and macrophages and suppresses the production of pro-inflammatory cytokines during the course of persistent liver damage caused by HCV through ER. Conversely, progesterone enhances the accumulation of inflammatory response cells and their cytokine production to counteract the positive effects of E2 (113). Testosterone also accelerates the progression of hepatitis C. A cross-sectional study involving male veterans with chronic HCV infection revealed that elevated serum total testosterone increases the risk of advanced HCV infection activity and liver fibrosis (114).
Hepatitis E virus (HEV) is a positive-sense RNA virus belonging to the family Hepeviridae, widely recognized as a major etiological agent of acute viral hepatitis worldwide (115). Infection with HEV can lead to high mortality rates and diffuse hepatic failure, with elevated levels of estrogen during pregnancy associated with increased HEV titers, exacerbating the infection (116, 117). Elevated estrogen levels and its metabolites exert inhibitory effects on hepatocytes, rendering them more susceptible to hepatic dysfunction/failure upon exposure to infectious pathogens (118–120).
In pregnant women infected with HEV, elevated estrogen levels result in placental dysfunction, leading to preterm birth, low birth weight, and fetal death (121, 122). Studies indicate that in HEV infection, estrogen and ERα sustained activation interact with HEV and enhance infection through the cAMPK-PKA-CREB and PI3K-AKT-mTOR signaling pathways, thereby increasing HEV mRNA and protein levels (116, 119, 123).
Furthermore, estradiol inhibits IFN-mediated antiviral activity within the liver and mediates ERα suppression of the host’s innate immune response during HEV infection to promote HEV infection (117, 122–124). Progesterone also influences the progression of hepatitis E. Pregnant women with fulminant liver failure exhibit elevated levels of progesterone and hepatitis E virus RNA. Studies have reported that progesterone can stimulate ROS production, promote HSCs activation, transforming growth factor expression, and extracellular collagen formation, which may also be critical factors in promoting hepatocellular carcinoma development (125). The mechanism of estrogen action is illustrated in Figure 2.
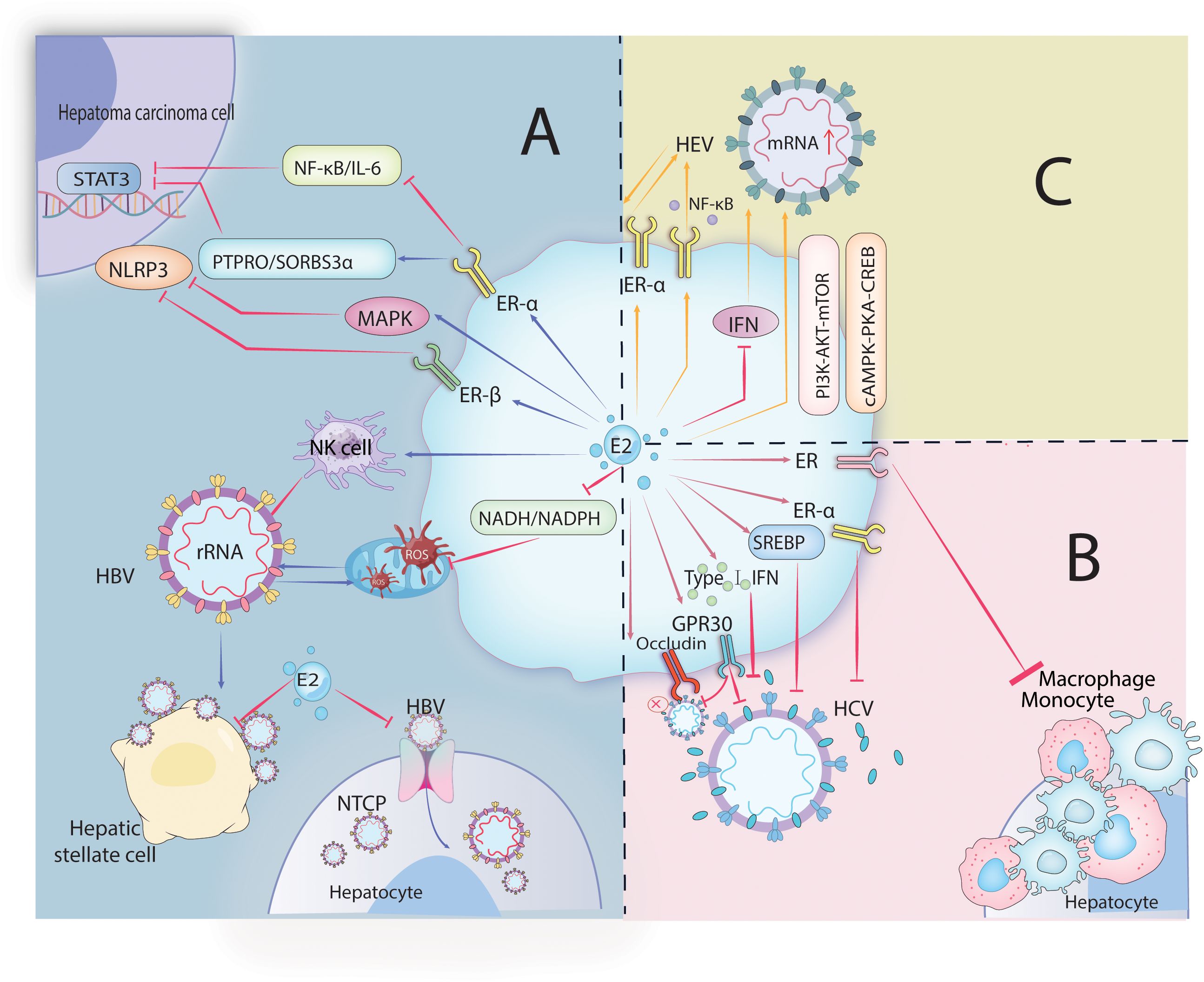
Figure 2. Image 2 depicts the role of estrogen in hepatitis virus infections. HBV, HCV, and HEV. In HBV infection (A), estrogen-mediated receptors inhibit HBV entry into cells and its viral replication, as well as transcription factor activity in hepatoma cells. In HCV infection (B), estrogen and its receptors regulate signaling pathways and interfere with interferon affecting the release of viral particles and inflammatory cell aggregation. In HEV infection (C), estrogen-mediated receptor-regulated signaling pathways promote HEV infection.
3.5 Influenza A virus
Influenza virus is a common respiratory virus belonging to the Orthomyxoviridae family, categorized into four types: A, B, C, and D. Influenza A virus (IAV) is highly pathogenic, causing damage to lung epithelial cells and potentially leading to severe pneumonia, respiratory failure, and acute respiratory distress syndrome (126–130). Female prognosis with IAV infection tends to be worse, often associated with decreased body temperature and significant weight loss (131–134). Despite similar viral titers between genders in lung tissues, females exhibit higher levels of pro-inflammatory cytokines and chemokines, such as TNF-α, IFN-γ, IL-6, and CCL2 (133, 135–137). These inflammatory responses may lead to cytokine storms, significantly increasing morbidity and mortality associated with IAV infection (138–142).
IAV infection also impairs ovarian function and decreases estrogen and progesterone levels in female mice, with pregnancy being a risk factor for severe outcomes related to influenza (132, 143). The role of estrogen in IAV infection is mediated through pro-inflammatory responses, where low concentrations of estradiol promote excessive inflammation and disease progression (144). However, high-dose estradiol exerts anti-inflammatory effects by inhibiting NF-kB transcriptional activity and mediating the ERα signaling pathway, suppressing the transcription of pro-inflammatory genes and cytokine production, thereby reducing the production of lung TNF-α and CCL2 and improving survival rates (145).
Physiological concentrations of progesterone can also promote faster repair of damaged lung tissues to prevent fatal and sublethal IAV infections (132). The mechanism primarily involves progesterone promoting higher concentrations of anti-inflammatory cytokines and lower concentrations of pro-inflammatory cytokines, reducing nitric oxide production, while maintaining macrophages and dendritic cells in a less activated state, thereby exerting protective effects during IAV infection (146–149).
Studies have shown that in lung tissues infected with the influenza virus, males exhibit faster resolution of inflammation and tissue repair compared to females, possibly due to elevated levels of certain growth factor proteins and testosterone levels in males (150). Testosterone can promote the contraction of pneumonia inflammatory monocytes during the peak of the disease and control the frequency of lung-specific CD8+ T cells and eosinophils, thereby improving the outcome of IAV infection (151).
3.6 Severe acute respiratory syndrome coronavirus 2
SARS-CoV-2, a member of the Coronaviridae family, is an RNA virus with high transmissibility and pathogenicity. It emerged in late 2019, precipitating the global COVID-19 pandemic. COVID-19 manifests as pulmonary inflammation and acute respiratory distress syndrome, posing significant threats to human health and public safety (152, 153). Epidemiological data indicate gender disparities in the incidence and mortality rates of COVID-19, with males exhibiting higher rates of infection, mortality, and disease severity compared to females (154). Studies suggest that estrogen and its receptors contribute to this gender difference, exerting effects through various mechanisms such as modulating the cellular entry of SARS-CoV-2, the immune response, and influencing coagulation function (155).
Angiotensin-converting enzyme 2 (ACE2) is expressed in various epithelial cells, including those in the lungs and respiratory tract, where it regulates fluid balance and the renin-angiotensin-aldosterone system (RAAS) (154, 156). SARS-CoV-2 can bind to ACE2 to enter cells, concurrently downregulating ACE2 expression, leading to an imbalance between ACE2 and Ang II levels, affecting the human RAAS system (157). Additionally, the spike protein of SARS-CoV-2 interacts with ACE2, facilitating membrane fusion and releasing viral RNA by binding to ACE2 on the host cell surface, thereby accelerating the progression of respiratory diseases (154, 158). Estrogen-responsive elements are present in the promoter region of ACE2, exerting their effects by regulating ACE2 gene expression, resulting in lower ACE2 expression levels in the lungs of females compared to males, thereby reducing interactions with the viral spike protein (159, 160). ACE2 glycosylation can enhance the stability and affinity of virus spike protein binding to host cell receptors. Estrogen, by binding to almost all sites including ACE2 glycan, inhibits host viral infection, reducing receptor surface energy, making it less prone to interact with the virus (161).
Estrogen also exerts anti-inflammatory effects by modulating innate and adaptive immunity, thereby inhibiting COVID-19 progression. SARS-CoV-2 infection leads to increased levels of Th1 cytokines interferons, inflammatory cytokines, and monocyte chemoattractant protein-1 (MCP-1) (162, 163). These cytokine storms activate monocytes/macrophages and neutrophils, leading to multi-organ failure, acute respiratory distress syndrome, and disseminated intravascular coagulation (158). Studies indicate that estrogen therapy attenuates MCP-1 expression in the blood, alleviates endothelial dysfunction, thereby inhibiting the occurrence of inflammatory responses and improving trauma outcomes (164). Infected lung epithelial cells produce IL-6, IL-1β, and IFN-I/III, which induce inflammatory programs in macrophages and recruit inflammatory monocytes, granulocytes, and lymphocytes from circulating blood, causing inflammatory responses (165). Estrogen receptors (ERs) are transcription factors crucial in regulating immune cell development and both innate and adaptive immune pathways (166, 167). High concentrations of estrogen upregulate ER signaling, suppress cytokine storms, and clear accumulated inflammatory cells (168).
In vitro experiments suggest that estrogen therapy can also regulate the NF-κB signaling pathway and the production of IL-6 and TNF-α (164, 166). E2 downregulates the expression of monocyte chemotactic factor receptors 2 (CCR2) and 3 (CXCR3) in mice, reducing monocyte recruitment (169). Moreover, estrogen inhibits the production of pro-inflammatory cytokines (including IL-6, IL-1β, and TNF-α) by monocytes and macrophages, exerting innate immune anti-inflammatory effects, while also preventing the migration of neutrophils and monocytes, among other innate immune cells, to the inflammatory area, thereby slowing disease progression (153).
Most severe COVID-19 patients exhibit reduced T lymphocytes. E2 promotes extrathymic T cell differentiation, affecting thymic tissue cell growth, and plays a role in immune system regulation. Specifically, estrogen alters the number and function of peripheral blood T lymphocytes through the sex hormone receptor pathway and conveys relevant information to the immune system (170). Maintenance of immune function typically relies on moderate lymphocyte apoptosis and Th1/Th2 balance, where Th1 and Th2 mutually inhibit each other (171). COVID-19 pathogenesis is complex, but characterized by Th1/Th17 immune responses. Overactivation of Th1/Th17 cells leads to the release of pro-inflammatory cytokines, resulting in lung injury (172). Th2-type immunity and regulatory T cells (Tregs) have the ability to alleviate systemic inflammation and complications. Estrogen therapy reduces the secretion of Th1 cytokine IFN-γ and promotes the secretion of Th2 cytokine IL-4, thereby correcting Th1/Th2 imbalance and enhancing the body’s ability to combat extracellular microbial infections (173). Tregs limit immune pathological reactions in the lungs of respiratory virus-infected patients. Tregs are significantly reduced in COVID-19 patients, estrogen promoting Treg cell expansion playing a protective role in SARS-CoV-2 infection (174, 175).
In terms of cellular immunity, estrogen stimulates plasma cells to produce immunoglobulins, regulates B cell maturation, upregulates the expression of pro-B cell survival mediators, and downregulates the expression of pro-B cell apoptosis mediators, thereby slowing SARS-CoV-2 infection (176). Estrogen also regulates the number of eosinophils and increases their migration, adhesion, survival, and degranulation in vivo and in vitro, affecting adaptive immunity (169, 177). Eosinophils can produce antiviral molecules and participate in the adaptive immune response caused by SARS-CoV-2 (178).
Due to the highly inflammatory response, platelet activation, endothelial dysfunction, and congestion, COVID-19 patients are prone to thrombotic diseases (179). Estrogen activates endothelial nitric oxide synthase transcription by binding to classical ER or GPR30, upregulating nitric oxide expression, maintaining endothelial homeostasis, and vasodilation (180). In vitro experiments have also shown that estrogen inhibits platelet aggregation by promoting the efflux or reuptake activity of Ca2+ (181).
SARS-CoV-2 infection is related not only to estrogen but also to testosterone and progesterone. High levels of testosterone promote virus entry, adversely affecting male resistance to COVID-19 (182). Transmembrane serine protease 2 (TMPRSS2) can cleave ACE2 and promote virus entry into host cells (183, 184). Androgen receptor activity is necessary for TMPRSS2 gene transcription, with high levels of testosterone upregulating its expression, making males more susceptible to COVID-19 (185). Unlike testosterone, progesterone has a protective effect in SARS-CoV-2 infection. Progesterone can promote CD4+ T cell bias from Th1 to Th2, promote anti-inflammatory responses, and regulate Siglec receptor to exert direct antiviral effects (186, 187). The mechanism of estrogen action is illustrated in area B of supplementary figure.
4 Conclusion
Gender-based disparities in disease incidence and mortality following viral infections have been extensively documented, with variations in hormonal profiles between males and females and the differential involvement of hormones in response mechanisms (7, 188). Hormones also exhibit synergistic and antagonistic interactions. Evidence suggests estrogen’s regulatory role in human susceptibility to viruses and immune responses (189, 190). Disparities in estrogen levels between genders and the pathways mediated by hormones may be pivotal factors in the divergent outcomes post-viral infection. As previously mentioned, males demonstrate heightened susceptibility, severe illness, and poorer prognoses across most viral infections. Estrogen exerts protective effects post-infection, attenuating disease onset and progression. However, recent studies have unveiled estrogen’s synergistic role with human papillomavirus (HPV) in promoting cervical carcinogenesis. Furthermore, elevated estrogen levels and ERα activation during pregnancy facilitate hepatitis E virus (HEV) infection in females. The protective effects of estrogen are typically realized through immunomodulation and anti-inflammatory actions (145, 189). Estrogen also influences macrophage classification, promoting an increase in M2 macrophage numbers, thereby aiding in disease control (191). Estrogen typically necessitates ER binding to modulate the estrogen receptor signaling pathway, thereby further regulating other signaling pathways. Apart from its anti-inflammatory effects, estrogen exhibits varied protective effects against different viral infections.
Numerous clinical studies have substantiated the therapeutic potential of exogenous estrogen therapy. Exogenous estrogen or ERα agonist therapy may be necessary for diseases where estrogen confers beneficial effects in viral infections (192–194). Conversely, ERα antagonists may hold therapeutic benefits for HPV, HEV, and other viruses. Future research should delve into elucidating the specific biological mechanisms of estrogen in viral infections, exploring improved estrogen therapy methods, including dosage, administration routes, and more appropriate targets, while balancing these aspects with other health risks.
Author contributions
XW: Conceptualization, Data curation, Formal Analysis, Funding acquisition, Investigation, Methodology, Project administration, Resources, Software, Supervision, Validation, Visualization, Writing – original draft, Writing – review & editing. LH: Conceptualization, Data curation, Formal Analysis, Funding acquisition, Investigation, Methodology, Project administration, Resources, Software, Supervision, Validation, Visualization, Writing – original draft, Writing – review & editing. HL: Writing – original draft, Writing – review & editing. KR: Writing – original draft, Writing – review & editing. WM: Writing – original draft, Writing – review & editing. YZ: Writing – original draft, Writing – review & editing. PG: Conceptualization, Data curation, Formal Analysis, Funding acquisition, Investigation, Methodology, Project administration, Resources, Software, Supervision, Validation, Visualization, Writing – original draft, Writing – review & editing.
Funding
The author(s) declare that financial support was received for the research and/or publication of this article. This work was funded by Shandong Provincial Natural Science Foundation (No. ZR2022QH039), National Natural Science Youth Foundation (No. 82402627) and Youth Innovation Science and Technology Support Plan for Universities in Shandong Province (No. 2024KJJ009).
Acknowledgments
We thank Zhujun Shao from the State Key Laboratory for Infectious Disease Prevention and Control, National Institute for Communicable Disease Control for kindly and helpful discussions.
Conflict of interest
The authors declare that the research was conducted in the absence of any commercial or financial relationships that could be construed as a potential conflict of interest.
Generative AI statement
The author(s) declare that no Generative AI was used in the creation of this manuscript.
Publisher’s note
All claims expressed in this article are solely those of the authors and do not necessarily represent those of their affiliated organizations, or those of the publisher, the editors and the reviewers. Any product that may be evaluated in this article, or claim that may be made by its manufacturer, is not guaranteed or endorsed by the publisher.
Supplementary material
The Supplementary Material for this article can be found online at: https://www.frontiersin.org/articles/10.3389/fimmu.2025.1556728/full#supplementary-material
Supplementary Figure 1 | The role of estrogen in influenza virus and SARS-CoV-2 infections. In influenza virus A (IVA) infection (A), low concentrations of estrogen promote the release of inflammatory factors, while high concentrations of estrogen, mediated by its receptors, inhibit the release of inflammatory factors through signaling pathways. In SARS-CoV-2 infection (B), estrogen and its receptor regulate signaling pathways and immune cells and proteins to counteract viral infection.
References
1. Cadwell K. The virome in host health and disease. Immunity. (2015) 42:805–13. doi: 10.1016/j.immuni.2015.05.003
2. Rothan HA and Byrareddy SN. The epidemiology and pathogenesis of coronavirus disease (Covid-19) outbreak. J Autoimmun. (2020) 109:102433. doi: 10.1016/j.jaut.2020.102433
3. Abdelrahman Z, Li M, and Wang X. Comparative review of sars-cov-2, sars-cov, mers-cov, and influenza a respiratory viruses. Front Immunol. (2020) 11:552909. doi: 10.3389/fimmu.2020.552909
4. Takahashi T, Ellingson MK, Wong P, Israelow B, Lucas C, Klein J, et al. Sex differences in immune responses that underlie covid-19 disease outcomes. Nature. (2020) 588:315–20. doi: 10.1038/s41586-020-2700-3
5. Tan Y, Shilts MH, Rosas-Salazar C, Puri V, Fedorova N, Halpin RA, et al. Influence of sex on respiratory syncytial virus genotype infection frequency and nasopharyngeal microbiome. J Virol. (2023) 97:e0147222. doi: 10.1128/jvi.01472-22
6. Polishchuk I, Stavi V, Awesat J, Ben Baruch Golan Y, Bartal C, Sagy I, et al. Sex differences in infective endocarditis. Am J Med Sci. (2021) 361:83–9. doi: 10.1016/j.amjms.2020.08.021
7. Wilkinson NM, Chen HC, Lechner MG, and Su MA. Sex differences in immunity. Annu Rev Immunol. (2022) 40:75–94. doi: 10.1146/annurev-immunol-101320-125133
8. Alderman MH 3rd and Taylor HS. Molecular mechanisms of estrogen action in female genital tract development. Differentiation; Res Biol Diversity. (2021) 118:34–40. doi: 10.1016/j.diff.2021.01.002
9. Li CL, Yeh KH, Liu WH, Chen CL, Chen DS, Chen PJ, et al. Elevated P53 promotes the processing of mir-18a to decrease estrogen receptor-A in female hepatocellular carcinoma. Int J Cancer. (2015) 136:761–70. doi: 10.1002/ijc.29052
10. Navaneethan U, Al Mohajer M, and Shata MT. Hepatitis E and pregnancy: understanding the pathogenesis. Liver international: Off J Int Assoc Study Liver. (2008) 28:1190–9. doi: 10.1111/j.1478-3231.2008.01840.x
11. Bronowicka-Kłys DE, Lianeri M, and Jagodziński PP. The role and impact of estrogens and xenoestrogen on the development of cervical cancer. Biomedicine pharmacotherapy = Biomedecine pharmacotherapie. (2016) 84:1945–53. doi: 10.1016/j.biopha.2016.11.007
12. Revankar CM, Cimino DF, Sklar LA, Arterburn JB, and Prossnitz ER. A transmembrane intracellular estrogen receptor mediates rapid cell signaling. Science. (2005) 307:1625–30. doi: 10.1126/science.1106943
13. Fanales-Belasio E, Raimondo M, Suligoi B, and Buttò S. Hiv virology and pathogenetic mechanisms of infection: A brief overview. Ann Ist Super Sanita. (2010) 46:5–14. doi: 10.4415/ann_10_01_02
14. Du R and Huang J. Machine learning revealed a novel ferroptosis-based classification for diagnosis in antiretroviral therapy-treated hiv patients with defective immune recovery. AIDS Res Hum retroviruses. (2024) 40:90–100. doi: 10.1089/aid.2022.0138
15. Hladik F and Hope TJ. Hiv infection of the genital mucosa in women. Curr HIV/AIDS Rep. (2009) 6:20–8. doi: 10.1007/s11904-009-0004-1
16. Hel Z, Stringer E, and Mestecky J. Sex steroid hormones, hormonal contraception, and the immunobiology of human immunodeficiency virus-1 infection. Endocrine Rev. (2010) 31:79–97. doi: 10.1210/er.2009-0018
17. Mingjia L and Short R. How oestrogen or progesterone might change a woman’s susceptibility to hiv-1 infection. Aust New Z J obstetrics gynaecology. (2002) 42:472–5. doi: 10.1111/j.0004-8666.2002.00472.x
18. Ziegler S and Altfeld M. Sex differences in hiv-1-mediated immunopathology. Curr Opin HIV AIDS. (2016) 11:209–15. doi: 10.1097/coh.0000000000000237
19. Wessels JM, Felker AM, Dupont HA, and Kaushic C. The relationship between sex hormones, the vaginal microbiome and immunity in hiv-1 susceptibility in women. Dis Models Mech. (2018) 11. doi: 10.1242/dmm.035147
20. Smith SM, Baskin GB, and Marx PA. Estrogen protects against vaginal transmission of simian immunodeficiency virus. J Infect Dis. (2000) 182:708–15. doi: 10.1086/315776
21. Goode D, Aravantinou M, Jarl S, Truong R, Derby N, Guerra-Perez N, et al. Sex hormones selectively impact the endocervical mucosal microenvironment: implications for hiv transmission. PloS One. (2014) 9:e97767. doi: 10.1371/journal.pone.0097767
22. Dizzell S, Nazli A, Reid G, and Kaushic C. Protective effect of probiotic bacteria and estrogen in preventing hiv-1-mediated impairment of epithelial barrier integrity in female genital tract. Cells. (2019) 8. doi: 10.3390/cells8101120
23. Vitali D, Wessels JM, and Kaushic C. Role of sex hormones and the vaginal microbiome in susceptibility and mucosal immunity to hiv-1 in the female genital tract. AIDS Res Ther. (2017) 14:39. doi: 10.1186/s12981-017-0169-4
24. Smith SB and Ravel J. The vaginal microbiota, host defence and reproductive physiology. J Physiol. (2017) 595:451–63. doi: 10.1113/jp271694
25. Pask AJ, McInnes KJ, Webb DR, and Short RV. Topical oestrogen keratinises the human foreskin and may help prevent hiv infection. PloS One. (2008) 3:e2308. doi: 10.1371/journal.pone.0002308
26. Hladik F, Sakchalathorn P, Ballweber L, Lentz G, Fialkow M, Eschenbach D, et al. Initial events in establishing vaginal entry and infection by human immunodeficiency virus type-1. Immunity. (2007) 26:257–70. doi: 10.1016/j.immuni.2007.01.007
27. Wilson ME, Allred KF, Bisotti AJ, Bruce-Keller A, Chuahan A, and Nath A. Estradiol negatively regulates hiv-ltr promoter activity in glial cells. AIDS Res Hum retroviruses. (2006) 22:350–6. doi: 10.1089/aid.2006.22.350
28. Wang M, Yang W, Chen Y, Wang J, Tan J, and Qiao W. Cellular relb interacts with the transactivator tat and enhance hiv-1 expression. Retrovirology. (2018) 15:65. doi: 10.1186/s12977-018-0447-9
29. Ali A and Banerjea AC. Curcumin inhibits hiv-1 by promoting tat protein degradation. Sci Rep. (2016) 6:27539. doi: 10.1038/srep27539
30. Heron PM, Turchan-Cholewo J, Bruce-Keller AJ, and Wilson ME. Estrogen receptor alpha inhibits the estrogen-mediated suppression of hiv transcription in astrocytes: implications for estrogen neuroprotection in hiv dementia. AIDS Res Hum retroviruses. (2009) 25:1071–81. doi: 10.1089/aid.2009.0065
31. McLaurin KA, Moran LM, Booze RM, and Mactutus CF. Selective estrogen receptor B Agonists: A therapeutic approach for hiv-1 associated neurocognitive disorders. J Neuroimmune Pharmacol. (2020) 15:264–79. doi: 10.1007/s11481-019-09900-y
32. McRae M. Hiv and viral protein effects on the blood brain barrier. Tissue barriers. (2016) 4:e1143543. doi: 10.1080/21688370.2016.1143543
33. Kameda C, Nakamura M, Tanaka H, Yamasaki A, Kubo M, Tanaka M, et al. Oestrogen receptor-alpha contributes to the regulation of the hedgehog signalling pathway in eralpha-positive gastric cancer. Br J Cancer. (2010) 102:738–47. doi: 10.1038/sj.bjc.6605517
34. Alvarez JI, Dodelet-Devillers A, Kebir H, Ifergan I, Fabre PJ, Terouz S, et al. The hedgehog pathway promotes blood-brain barrier integrity and cns immune quiescence. Science. (2011) 334:1727–31. doi: 10.1126/science.1206936
35. Wilson ME, Dimayuga FO, Reed JL, Curry TE, Anderson CF, Nath A, et al. Immune modulation by estrogens: role in cns hiv-1 infection. Endocrine. (2006) 29:289–97. doi: 10.1385/endo:29:2:289
36. Liu Z, Mu S, and Wang X. Effect of estrogen on recovering the injured nervous system. Pakistan J pharmaceutical Sci. (2015) 28:1471–5.
37. De Marinis E, Marino M, and Ascenzi P. Neuroglobin, estrogens, and neuroprotection. IUBMB Life. (2011) 63:140–5. doi: 10.1002/iub.426
38. Oyouni AAA. Human papillomavirus in cancer: infection, disease transmission, and progress in vaccines. J infection Public Health. (2023) 16:626–31. doi: 10.1016/j.jiph.2023.02.014
39. Chung SH, Wiedmeyer K, Shai A, Korach KS, and Lambert PF. Requirement for estrogen receptor alpha in a mouse model for human papillomavirus-associated cervical cancer. Cancer Res. (2008) 68:9928–34. doi: 10.1158/0008-5472.Can-08-2051
40. Brianti P, De Flammineis E, and Mercuri SR. Review of hpv-related diseases and cancers. New microbiologica. (2017) 40:80–5.
41. de Villiers EM. Relationship between steroid hormone contraceptives and hpv, cervical intraepithelial neoplasia and cervical carcinoma. Int J Cancer. (2003) 103:705–8. doi: 10.1002/ijc.10868
42. Ramachandran B. Functional association of oestrogen receptors with hpv infection in cervical carcinogenesis. Endocrine-related Cancer. (2017) 24:R99–r108. doi: 10.1530/erc-16-0571
43. Brake T and Lambert PF. Estrogen contributes to the onset, persistence, and Malignant progression of cervical cancer in a human papillomavirus-transgenic mouse model. Proc Natl Acad Sci United States America. (2005) 102:2490–5. doi: 10.1073/pnas.0409883102
44. Wang JT, Gao ES, Cheng YY, Yan JW, and Ding L. Analysis on synergistic action between estrogen, progesterone and human papillomaviruses in cervical cancer. Zhonghua liu xing bing xue za zhi = Zhonghua liuxingbingxue zazhi. (2005) 26:370–3.
45. SJ R. The immune microenvironment in human papilloma virus-induced cervical lesions-evidence for estrogen as an immunomodulator. Front Cell infection Microbiol. (2021) 11:649815. doi: 10.3389/fcimb.2021.649815
46. Coelho FR, Prado JC, Pereira Sobrinho JS, Hamada G, Landman G, Pinto CA, et al. Estrogen and progesterone receptors in human papilloma virus-related cervical neoplasia. Braz J Med Biol Res = Rev Bras pesquisas medicas e biologicas. (2004) 37:83–8. doi: 10.1590/s0100-879x2004000100012
47. Mitrani-Rosenbaum S, Tsvieli R, and Tur-Kaspa R. Oestrogen stimulates differential transcription of human papillomavirus type 16 in siha cervical carcinoma cells. J Gen Virol. (1989) 70:2227–32. doi: 10.1099/0022-1317-70-8-2227
48. Auborn KJ, Woodworth C, DiPaolo JA, and Bradlow HL. The interaction between hpv infection and estrogen metabolism in cervical carcinogenesis. Int J Cancer. (1991) 49:867–9. doi: 10.1002/ijc.2910490611
49. Arbeit JM, Howley PM, and Hanahan D. Chronic estrogen-induced cervical and vaginal squamous carcinogenesis in human papillomavirus type 16 transgenic mice. Proc Natl Acad Sci United States America. (1996) 93:2930–5. doi: 10.1073/pnas.93.7.2930
50. Elson DA, Riley RR, Lacey A, Thordarson G, Talamantes FJ, and Arbeit JM. Sensitivity of the cervical transformation zone to estrogen-induced squamous carcinogenesis. Cancer Res. (2000) 60:1267–75.
51. Shai A, Pitot HC, and Lambert PF. P53 loss synergizes with estrogen and papillomaviral oncogenes to induce cervical and breast cancers. Cancer Res. (2008) 68:2622–31. doi: 10.1158/0008-5472.Can-07-5266
52. Čonkaš J, Sabol M, and Ozretić P. ‘Toxic masculinity’: what is known about the role of androgen receptors in head and neck squamous cell carcinoma. Int J Mol Sci. (2023) 24. doi: 10.3390/ijms24043766
53. Chung SH and Lambert PF. Prevention and treatment of cervical cancer in mice using estrogen receptor antagonists. Proc Natl Acad Sci United States America. (2009) 106:19467–72. doi: 10.1073/pnas.0911436106
54. Au WW, Abdou-Salama S, and Al-Hendy A. Inhibition of growth of cervical cancer cells using a dominant negative estrogen receptor gene. Gynecologic Oncol. (2007) 104:276–80. doi: 10.1016/j.ygyno.2006.10.015
55. Son J, Park JW, Lambert PF, and Chung SH. Requirement of estrogen receptor alpha DNA-binding domain for hpv oncogene-induced cervical carcinogenesis in mice. Carcinogenesis. (2014) 35:489–96. doi: 10.1093/carcin/bgt350
56. Sun Q, Liang Y, Zhang T, Wang K, and Yang X. Er-A36 mediates estrogen-stimulated mapk/erk activation and regulates migration, invasion, proliferation in cervical cancer cells. Biochem Biophys Res Commun. (2017) 487:625–32. doi: 10.1016/j.bbrc.2017.04.105
57. Akimoto T, Takasawa A, Takasawa K, Aoyama T, Murata M, Osanai M, et al. Estrogen/gpr30 signaling contributes to the Malignant potentials of er-negative cervical adenocarcinoma via regulation of claudin-1 expression. Neoplasia (New York NY). (2018) 20:1083–93. doi: 10.1016/j.neo.2018.08.010
58. Ogawa M, Hashimoto K, Kitano S, Yamashita S, Toda A, Nakamura K, et al. Estrogen induces genomic instability in high-risk hpv-infected cervix and promotes the carcinogenesis of cervical adenocarcinoma. Biochem Biophys Res Commun. (2023) 659:80–90. doi: 10.1016/j.bbrc.2023.04.009
59. Kim CJ, Um SJ, Kim TY, Kim EJ, Park TC, Kim SJ, et al. Regulation of cell growth and hpv genes by exogenous estrogen in cervical cancer cells. Int J gynecological cancer: Off J Int Gynecological Cancer Soc. (2000) 10:157–64. doi: 10.1046/j.1525-1438.2000.00016.x
60. Matsumoto T, Suzuki T, Nakamura M, Yamamoto M, Iizuka T, Ono M, et al. Androgen promotes squamous differentiation of atypical cells in cervical intraepithelial neoplasia via an elf3-dependent pathway. Cancer Med. (2023) 12:10816–28. doi: 10.1002/cam4.5824
61. Tariq N and Kyriakopoulos C. Group B coxsackie virus. In: Statpearls. StatPearls Publishing LLC, Treasure Island (FL (2023). ) ineligible companies. Disclosure: Chris Kyriakopoulos declares no relevant financial relationships with ineligible companies.: StatPearls Publishing Copyright © 2023.
62. Muehlenbachs A, Bhatnagar J, and Zaki SR. Tissue tropism, pathology and pathogenesis of enterovirus infection. J Pathol. (2015) 235:217–28. doi: 10.1002/path.4438
63. Zhou N, Yue Y, and Xiong S. Sex hormone contributes to sexually dimorphic susceptibility in cvb3-induced viral myocarditis via modulating ifn-Γ(+) nk cell production. Can J Cardiol. (2018) 34:492–501. doi: 10.1016/j.cjca.2018.01.002
64. Koenig A, Sateriale A, Budd RC, Huber SA, and Buskiewicz IA. The role of sex differences in autophagy in the heart during coxsackievirus B3-induced myocarditis. J Cardiovasc Trans Res. (2014) 7:182–91. doi: 10.1007/s12265-013-9525-5
65. Huber SA, Job LP, and Auld KR. Influence of sex hormones on coxsackie B-3 virus infection in balb/C mice. Cell Immunol. (1982) 67:173–9. doi: 10.1016/0008-8749(82)90210-6
66. Li Z, Yue Y, and Xiong S. Distinct th17 inductions contribute to the gender bias in cvb3-induced myocarditis. Cardiovasc pathology: Off J Soc Cardiovasc Pathol. (2013) 22:373–82. doi: 10.1016/j.carpath.2013.02.004
67. Lélu K, Laffont S, Delpy L, Paulet PE, Périnat T, Tschanz SA, et al. Estrogen receptor A Signaling in T lymphocytes is required for estradiol-mediated inhibition of th1 and th17 cell differentiation and protection against experimental autoimmune encephalomyelitis. J Immunol (Baltimore Md: 1950). (2011) 187:2386–93. doi: 10.4049/jimmunol.1101578
68. Hao S, Zhao J, Zhou J, Zhao S, Hu Y, and Hou Y. Modulation of 17beta-estradiol on the number and cytotoxicity of nk cells in vivo related to mcm and activating receptors. Int Immunopharmacol. (2007) 7:1765–75. doi: 10.1016/j.intimp.2007.09.017
69. Yovel G, Shakhar K, and Ben-Eliyahu S. The effects of sex, menstrual cycle, and oral contraceptives on the number and activity of natural killer cells. Gynecologic Oncol. (2001) 81:254–62. doi: 10.1006/gyno.2001.6153
70. Yunna C, Mengru H, Lei W, and Weidong C. Macrophage M1/M2 polarization. Eur J Pharmacol. (2020) 877:173090. doi: 10.1016/j.ejphar.2020.173090
71. Li K, Xu W, Guo Q, Jiang Z, Wang P, Yue Y, et al. Differential macrophage polarization in male and female balb/C mice infected with coxsackievirus B3 defines susceptibility to viral myocarditis. Circ Res. (2009) 105:353–64. doi: 10.1161/circresaha.109.195230
72. Lee CC, Chen SY, and Lee TM. 17β-oestradiol facilitates M2 macrophage skewing and ameliorates arrhythmias in ovariectomized female infarcted rats. J Cell Mol Med. (2022) 26:3396–409. doi: 10.1111/jcmm.17344
73. Gottlieb RA and Mentzer RM Jr. Cardioprotection through autophagy: ready for clinical trial? Autophagy. (2011) 7:434–5. doi: 10.4161/auto.7.4.14442
74. Kanamori H, Takemura G, Goto K, Maruyama R, Ono K, Nagao K, et al. Autophagy limits acute myocardial infarction induced by permanent coronary artery occlusion. Am J Physiol Heart Circ Physiol. (2011) 300:H2261–71. doi: 10.1152/ajpheart.01056.2010
75. Przyklenk K, Undyala VV, Wider J, Sala-Mercado JA, Gottlieb RA, and Mentzer RM Jr. Acute induction of autophagy as a novel strategy for cardioprotection: getting to the heart of the matter. Autophagy. (2011) 7:432–3. doi: 10.4161/auto.7.4.14395
76. Zhang S, Ma J, Wang X, Zhao D, Zhang J, Jiang L, et al. Gpr30 alleviates pressure overload-induced myocardial hypertrophy in ovariectomized mice by regulating autophagy. Int J Mol Sci. (2023) 24. doi: 10.3390/ijms24020904
77. Lanini S, Ustianowski A, Pisapia R, Zumla A, and Ippolito G. Viral hepatitis: etiology, epidemiology, transmission, diagnostics, treatment, and prevention. Infect Dis Clinics North America. (2019) 33:1045–62. doi: 10.1016/j.idc.2019.08.004
78. Tong S. Hepatitis B virus, a sex hormone-responsive virus. Gastroenterology. (2012) 142:696–9. doi: 10.1053/j.gastro.2012.02.036
79. Ruggieri A, Gagliardi MC, and Anticoli S. Sex-dependent outcome of hepatitis B and C viruses infections: synergy of sex hormones and immune responses? Front Immunol. (2018) 9:2302. doi: 10.3389/fimmu.2018.02302
80. Yang ST, Han JQ, Wang YD, Shen C, and Zhao CY. Estrogen and its receptors in hepatitis B virus-related hepatocellular carcinoma. Zhonghua Gan Zang Bing Za Zhi. (2019) 27:232–5. doi: 10.3760/cma.j.issn.1007-3418.2019.03.014
81. Shimizu I, Kohno N, Tamaki K, Shono M, Huang HW, He JH, et al. Female hepatology: favorable role of estrogen in chronic liver disease with hepatitis B virus infection. World J gastroenterology. (2007) 13:4295–305. doi: 10.3748/wjg.v13.i32.4295
82. Yu MW, Yang YC, Yang SY, Cheng SW, Liaw YF, Lin SM, et al. Hormonal markers and hepatitis B virus-related hepatocellular carcinoma risk: A nested case-control study among men. J Natl Cancer Institute. (2001) 93:1644–51. doi: 10.1093/jnci/93.21.1644
83. Kim YS, Seo HW, and Jung G. Reactive oxygen species promote heat shock protein 90-mediated hbv capsid assembly. Biochem Biophys Res Commun. (2015) 457:328–33. doi: 10.1016/j.bbrc.2014.12.110
84. Karaahmet F, Coban S, and Yuksel I. Impact of endogenous activity of oestrogen in patient with hepatitis B virus infection. Liver international: Off J Int Assoc Study Liver. (2015) 35:1782. doi: 10.1111/liv.12796
85. Wang SH, Yeh SH, Lin WH, Yeh KH, Yuan Q, Xia NS, et al. Estrogen receptor A Represses transcription of hbv genes via interaction with hepatocyte nuclear factor 4A. Gastroenterology. (2012) 142:989–98.e4. doi: 10.1053/j.gastro.2011.12.045
86. Jalil S, Grady JJ, Lee C, and Anderson KE. Associations among behavior-related susceptibility factors in porphyria cutanea tarda. Clin gastroenterology hepatology: Off Clin Pract J Am Gastroenterological Assoc. (2010) 8:297–302.e1. doi: 10.1016/j.cgh.2009.11.017
87. Macek Jilkova Z, Decaens T, Marlu A, Marche H, Jouvin-Marche E, and Marche PN. Sex differences in spontaneous degranulation activity of intrahepatic natural killer cells during chronic hepatitis B: association with estradiol levels. Mediators Inflammation. (2017) 2017:3214917. doi: 10.1155/2017/3214917
88. Wang SH, Chen PJ, and Yeh SH. Gender disparity in chronic hepatitis B: mechanisms of sex hormones. J gastroenterology hepatology. (2015) 30:1237–45. doi: 10.1111/jgh.12934
89. Yan H, Zhong G, Xu G, He W, Jing Z, Gao Z, et al. Sodium taurocholate cotransporting polypeptide is a functional receptor for human hepatitis B and D virus. Elife. (2012) 1:e00049. doi: 10.7554/eLife.00049
90. Le Vee M, Lecureur V, Moreau A, Stieger B, and Fardel O. Differential regulation of drug transporter expression by hepatocyte growth factor in primary human hepatocytes. Drug Metab Dispos. (2009) 37:2228–35. doi: 10.1124/dmd.109.028035
91. Yan Z, Tan W, Xu B, Dan Y, Zhao W, Deng C, et al. A cis-acting regulatory variation of the estrogen receptor A (Esr1) gene is associated with hepatitis B virus-related liver cirrhosis. Hum Mutat. (2011) 32:1128–36. doi: 10.1002/humu.21544
92. You H, Wang X, Ma L, Zhang F, Zhang H, Wang Y, et al. Insights into the impact of hepatitis B virus on hepatic stellate cell activation. Cell communication signaling: CCS. (2023) 21:70. doi: 10.1186/s12964-023-01091-7
93. Ohata K, Hamasaki K, Toriyama K, Matsumoto K, Saeki A, Yanagi K, et al. Hepatic steatosis is a risk factor for hepatocellular carcinoma in patients with chronic hepatitis C virus infection. Cancer. (2003) 97:3036–43. doi: 10.1002/cncr.11427
94. Powell EE, Jonsson JR, and Clouston AD. Steatosis: co-factor in other liver diseases. Hepatology (Baltimore Md). (2005) 42:5–13. doi: 10.1002/hep.20750
95. Elbers JM, Asscheman H, Seidell JC, and Gooren LJ. Effects of sex steroid hormones on regional fat depots as assessed by magnetic resonance imaging in transsexuals. Am J Physiol. (1999) 276:E317–25. doi: 10.1152/ajpendo.1999.276.2.E317
96. Fuke H, Shiraki K, Sugimoto K, Tanaka J, Beppu T, Yoneda K, et al. Jak inhibitor induces S phase cell-cycle arrest and augments trail-induced apoptosis in human hepatocellular carcinoma cells. Biochem Biophys Res Commun. (2007) 363:738–44. doi: 10.1016/j.bbrc.2007.09.049
97. Xin H, Wang M, Tang W, Shen Z, Miao L, Wu W, et al. Hydrogen sulfide attenuates inflammatory hepcidin by reducing il-6 secretion and promoting sirt1-mediated stat3 deacetylation. Antioxid Redox Signal. (2016) 24:70–83. doi: 10.1089/ars.2015.6315
98. Wei Q, Mu K, Li T, Zhang Y, Yang Z, Jia X, et al. Deregulation of the nlrp3 inflammasome in hepatic parenchymal cells during liver cancer progression. Lab investigation; A J Tech Methods Pathol. (2014) 94:52–62. doi: 10.1038/labinvest.2013.126
99. Wei Q, Guo P, Mu K, Zhang Y, Zhao W, Huai W, et al. Estrogen suppresses hepatocellular carcinoma cells through erβ-mediated upregulation of the nlrp3 inflammasome. Lab investigation; A J Tech Methods Pathol. (2015) 95:804–16. doi: 10.1038/labinvest.2015.63
100. Yang W, Lu Y, Xu Y, Xu L, Zheng W, Wu Y, et al. Estrogen represses hepatocellular carcinoma (Hcc) growth via inhibiting alternative activation of tumor-associated macrophages (Tams). J Biol Chem. (2012) 287:40140–9. doi: 10.1074/jbc.M112.348763
101. Iyer JK, Kalra M, Kaul A, Payton ME, and Kaul R. Estrogen receptor expression in chronic hepatitis C and hepatocellular carcinoma pathogenesis. World J gastroenterology. (2017) 23:6802–16. doi: 10.3748/wjg.v23.i37.6802
102. Lin MV, King LY, and Chung RT. Hepatitis C virus-associated cancer. Annu Rev Pathol. (2015) 10:345–70. doi: 10.1146/annurev-pathol-012414-040323
103. Di Martino V, Lebray P, Myers RP, Pannier E, Paradis V, Charlotte F, et al. Progression of liver fibrosis in women infected with hepatitis C: long-term benefit of estrogen exposure. Hepatology (Baltimore Md). (2004) 40:1426–33. doi: 10.1002/hep.20463
104. Barbaglia MN, Harris JM, Smirnov A, Burlone ME, Rigamonti C, Pirisi M, et al. 17β-oestradiol protects from hepatitis C virus infection through induction of type I interferon. Viruses. (2022) 14. doi: 10.3390/v14081806
105. Yasuda M, Shimizu I, Shiba M, and Ito S. Suppressive effects of estradiol on dimethylnitrosamine-induced fibrosis of the liver in rats. Hepatology (Baltimore Md). (1999) 29:719–27. doi: 10.1002/hep.510290307
106. Hayashida K, Shoji I, Deng L, Jiang DP, Ide YH, and Hotta H. 17β-estradiol inhibits the production of infectious particles of hepatitis C virus. Microbiol Immunol. (2010) 54:684–90. doi: 10.1111/j.1348-0421.2010.00268.x
107. Magri A, Barbaglia MN, Foglia CZ, Boccato E, Burlone ME, Cole S, et al. 17,B-estradiol inhibits hepatitis C virus mainly by interference with the release phase of its life cycle. Liver international: Off J Int Assoc Study Liver. (2017) 37:669–77. doi: 10.1111/liv.13303
108. Ulitzky L, Lafer MM, KuKuruga MA, Silberstein E, Cehan N, and Taylor DR. A new signaling pathway for hcv inhibition by estrogen: gpr30 activation leads to cleavage of occludin by mmp-9. PloS One. (2016) 11:e0145212. doi: 10.1371/journal.pone.0145212
109. Lopez D, Sanchez MD, Shea-Eaton W, and McLean MP. Estrogen activates the high-density lipoprotein receptor gene via binding to estrogen response elements and interaction with sterol regulatory element binding protein-1a. Endocrinology. (2002) 143:2155–68. doi: 10.1210/endo.143.6.8855
110. Sato R, Miyamoto W, Inoue J, Terada T, Imanaka T, and Maeda M. Sterol regulatory element-binding protein negatively regulates microsomal triglyceride transfer protein gene transcription. J Biol Chem. (1999) 274:24714–20. doi: 10.1074/jbc.274.35.24714
111. Gastaminza P, Cheng G, Wieland S, Zhong J, Liao W, and Chisari FV. Cellular determinants of hepatitis C virus assembly, maturation, degradation, and secretion. J Virol. (2008) 82:2120–9. doi: 10.1128/jvi.02053-07
112. Paul D, Madan V, and Bartenschlager R. Hepatitis C virus rna replication and assembly: living on the fat of the land. Cell Host Microbe. (2014) 16:569–79. doi: 10.1016/j.chom.2014.10.008
113. Yuan Y, Shimizu I, Shen M, Aoyagi E, Takenaka H, Itagaki T, et al. Effects of estradiol and progesterone on the proinflammatory cytokine production by mononuclear cells from patients with chronic hepatitis C. World J gastroenterology. (2008) 14:2200–7. doi: 10.3748/wjg.14.2200
114. White DL, Tavakoli-Tabasi S, Kuzniarek J, Pascua R, Ramsey DJ, and El-Serag HB. Higher serum testosterone is associated with increased risk of advanced hepatitis C-related liver disease in males. Hepatology (Baltimore Md). (2012) 55:759–68. doi: 10.1002/hep.24618
115. Kenney SP and Meng XJ. Hepatitis E virus genome structure and replication strategy. Cold Spring Harb Perspect Med. (2019) 9. doi: 10.1101/cshperspect.a031724
116. Gong S, Hao X, Bi Y, Yang C, Wang W, Mickael HK, et al. Hepatitis E viral infection regulates estrogen signaling pathways: inhibition of the campk-pka-creb and pi3k-akt-mtor signaling pathways. J Med Virol. (2021) 93:3769–78. doi: 10.1002/jmv.26641
117. Sooryanarain H, Heffron CL, Mahsoub HM, Hassebroek AM, Wang B, Tian D, et al. Modulation of socs3 levels via stat3 and estrogen-erαp66 signaling during hepatitis E virus replication in hepatocellular carcinoma cells. J Virol. (2022) 96:e0100822. doi: 10.1128/jvi.01008-22
118. McGovern BH, Ditelberg JS, Taylor LE, Gandhi RT, Christopoulos KA, Chapman S, et al. Hepatic steatosis is associated with fibrosis, nucleoside analogue use, and hepatitis C virus genotype 3 infection in hiv-seropositive patients. Clin Infect Dis. (2006) 43:365–72. doi: 10.1086/505495
119. Yang C, Yu W, Bi Y, Long F, Li Y, Wei D, et al. Increased oestradiol in hepatitis E virus-infected pregnant women promotes viral replication. J viral hepatitis. (2018) 25:742–51. doi: 10.1111/jvh.12865
120. Jilani N, Das BC, Husain SA, Baweja UK, Chattopadhya D, Gupta RK, et al. Hepatitis E virus infection and fulminant hepatic failure during pregnancy. J gastroenterology hepatology. (2007) 22:676–82. doi: 10.1111/j.1440-1746.2007.04913.x
121. Singh S, Daga MK, Kumar A, Husain SA, and Kar P. Role of oestrogen and its receptors in hev-associated feto-maternal outcomes. Liver international: Off J Int Assoc Study Liver. (2019) 39:633–9. doi: 10.1111/liv.13928
122. Mahsoub HM, Heffron CL, Hassebroek AM, Sooryanarain H, Wang B, LeRoith T, et al. Fetal loss in pregnant rabbits infected with genotype 3 hepatitis E virus is associated with altered inflammatory responses, enhanced virus replication, and extrahepatic virus dissemination with positive correlations with increased estradiol level. mBio. (2023) 14:e0041823. doi: 10.1128/mbio.00418-23
123. Bi Y, Yang C, Yu W, Zhao X, Zhao C, He Z, et al. Pregnancy serum facilitates hepatitis E virus replication in vitro. J Gen Virol. (2015) 96:1055–61. doi: 10.1099/vir.0.000054
124. Prusty BK, Hedau S, Singh A, Kar P, and Das BC. Selective suppression of nf-kbp65 in hepatitis virus-infected pregnant women manifesting severe liver damage and high mortality. Mol Med. (2007) 13:518–26. doi: 10.2119/2007-00055.Prusty
125. Itagaki T, Shimizu I, Cheng X, Yuan Y, Oshio A, Tamaki K, et al. Opposing effects of oestradiol and progesterone on intracellular pathways and activation processes in the oxidative stress induced activation of cultured rat hepatic stellate cells. Gut. (2005) 54:1782–9. doi: 10.1136/gut.2005.053278
126. Ducatez MF, Pelletier C, and Meyer G. Influenza D virus in cattle, France, 2011-2014. Emerg Infect Dis. (2015) 21:368–71. doi: 10.3201/eid2102.141449
127. Ferguson L, Eckard L, Epperson WB, Long LP, Smith D, Huston C, et al. Influenza D virus infection in mississippi beef cattle. Virology. (2015) 486:28–34. doi: 10.1016/j.virol.2015.08.030
128. Dong Y, Wang L, Burgner DP, Miller JE, Song Y, Ren X, et al. Infectious diseases in children and adolescents in China: analysis of national surveillance data from 2008 to 2017. Bmj. (2020) 369:m1043. doi: 10.1136/bmj.m1043
129. Meischel T, Villalon-Letelier F, Saunders PM, Reading PC, and Londrigan SL. Influenza a virus interactions with macrophages: lessons from epithelial cells. Cell Microbiol. (2020) 22:e13170. doi: 10.1111/cmi.13170
130. Uyeki TM and Peiris M. Novel avian influenza a virus infections of humans. Infect Dis Clinics North America. (2019) 33:907–32. doi: 10.1016/j.idc.2019.07.003
131. Robinson DP, Lorenzo ME, Jian W, and Klein SL. Elevated 17β-estradiol protects females from influenza a virus pathogenesis by suppressing inflammatory responses. PloS Pathog. (2011) 7:e1002149. doi: 10.1371/journal.ppat.1002149
132. Creisher PS, Seddu K, Mueller AL, and Klein SL. Biological sex and pregnancy affect influenza pathogenesis and vaccination. Curr topics Microbiol Immunol. (2023) 441:111–37. doi: 10.1007/978-3-031-35139-6_5
133. Vom Steeg LG and Klein SL. Sex and sex steroids impact influenza pathogenesis across the life course. Semin immunopathology. (2019) 41:189–94. doi: 10.1007/s00281-018-0718-5
134. Peretz J, Pekosz A, Lane AP, and Klein SL. Estrogenic compounds reduce influenza a virus replication in primary human nasal epithelial cells derived from female, but not male, donors. Am J Physiol Lung Cell Mol Physiol. (2016) 310:L415–25. doi: 10.1152/ajplung.00398.2015
135. Lorenzo ME, Hodgson A, Robinson DP, Kaplan JB, Pekosz A, and Klein SL. Antibody responses and cross protection against lethal influenza a viruses differ between the sexes in C57bl/6 mice. Vaccine. (2011) 29:9246–55. doi: 10.1016/j.vaccine.2011.09.110
136. Larcombe AN, Foong RE, Bozanich EM, Berry LJ, Garratt LW, Gualano RC, et al. Sexual dimorphism in lung function responses to acute influenza a infection. Influenza other respiratory viruses. (2011) 5:334–42. doi: 10.1111/j.1750-2659.2011.00236.x
137. Hoffmann J, Otte A, Thiele S, Lotter H, Shu Y, and Gabriel G. Sex differences in H7n9 influenza a virus pathogenesis. Vaccine. (2015) 33:6949–54. doi: 10.1016/j.vaccine.2015.08.044
138. Guan Y, Poon LL, Cheung CY, Ellis TM, Lim W, Lipatov AS, et al. H5n1 influenza: A protean pandemic threat. Proc Natl Acad Sci United States America. (2004) 101:8156–61. doi: 10.1073/pnas.0402443101
139. Szretter KJ, Gangappa S, Lu X, Smith C, Shieh WJ, Zaki SR, et al. Role of host cytokine responses in the pathogenesis of avian H5n1 influenza viruses in mice. J Virol. (2007) 81:2736–44. doi: 10.1128/jvi.02336-06
140. Kobasa D, Jones SM, Shinya K, Kash JC, Copps J, Ebihara H, et al. Aberrant innate immune response in lethal infection of macaques with the 1918 influenza virus. Nature. (2007) 445:319–23. doi: 10.1038/nature05495
141. Kash JC, Tumpey TM, Proll SC, Carter V, Perwitasari O, Thomas MJ, et al. Genomic analysis of increased host immune and cell death responses induced by 1918 influenza virus. Nature. (2006) 443:578–81. doi: 10.1038/nature05181
142. de Jong MD, Simmons CP, Thanh TT, Hien VM, Smith GJ, Chau TN, et al. Fatal outcome of human influenza a (H5n1) is associated with high viral load and hypercytokinemia. Nat Med. (2006) 12:1203–7. doi: 10.1038/nm1477
143. Littauer EQ and Skountzou I. Hormonal regulation of physiology, innate immunity and antibody response to H1n1 influenza virus infection during pregnancy. Front Immunol. (2018) 9:2455. doi: 10.3389/fimmu.2018.02455
144. Robinson DP, Huber SA, Moussawi M, Roberts B, Teuscher C, Watkins R, et al. Sex chromosome complement contributes to sex differences in coxsackievirus B3 but not influenza a virus pathogenesis. Biol sex Dif. (2011) 2:8. doi: 10.1186/2042-6410-2-8
145. Straub RH. The complex role of estrogens in inflammation. Endocrine Rev. (2007) 28:521–74. doi: 10.1210/er.2007-0001
146. Butts CL, Shukair SA, Duncan KM, Bowers E, Horn C, Belyavskaya E, et al. Progesterone inhibits mature rat dendritic cells in a receptor-mediated fashion. Int Immunol. (2007) 19:287–96. doi: 10.1093/intimm/dxl145
147. Jones LA, Kreem S, Shweash M, Paul A, Alexander J, and Roberts CW. Differential modulation of tlr3- and tlr4-mediated dendritic cell maturation and function by progesterone. J Immunol (Baltimore Md: 1950). (2010) 185:4525–34. doi: 10.4049/jimmunol.0901155
148. Kyurkchiev D, Ivanova-Todorova E, Hayrabedyan S, Altankova I, and Kyurkchiev S. Female sex steroid hormones modify some regulatory properties of monocyte-derived dendritic cells. Am J Reprod Immunol (New York NY: 1989). (2007) 58:425–33. doi: 10.1111/j.1600-0897.2007.00526.x
149. Su L, Sun Y, Ma F, Lü P, Huang H, and Zhou J. Progesterone inhibits toll-like receptor 4-mediated innate immune response in macrophages by suppressing nf-kappab activation and enhancing socs1 expression. Immunol Lett. (2009) 125:151–5. doi: 10.1016/j.imlet.2009.07.003
150. Vermillion MS, Ursin RL, Kuok DIT, Vom Steeg LG, Wohlgemuth N, Hall OJ, et al. Production of amphiregulin and recovery from influenza is greater in males than females. Biol sex Dif. (2018) 9:24. doi: 10.1186/s13293-018-0184-8
151. Abu-Raya B, Michalski C, Sadarangani M, and Lavoie PM. Maternal immunological adaptation during normal pregnancy. Front Immunol. (2020) 11:575197. doi: 10.3389/fimmu.2020.575197
152. Hu B, Guo H, Zhou P, and Shi ZL. Characteristics of sars-cov-2 and covid-19. Nat Rev Microbiol. (2021) 19:141–54. doi: 10.1038/s41579-020-00459-7
153. Mauvais-Jarvis F, Klein SL, and Levin ER. Estradiol, progesterone, immunomodulation, and covid-19 outcomes. Endocrinology. (2020) 161. doi: 10.1210/endocr/bqaa127
154. Majdic G. Could sex/gender differences in ace2 expression in the lungs contribute to the large gender disparity in the morbidity and mortality of patients infected with the sars-cov-2 virus? Front Cell infection Microbiol. (2020) 10:327. doi: 10.3389/fcimb.2020.00327
155. Cattrini C, Bersanelli M, Latocca MM, Conte B, Vallome G, and Boccardo F. Sex hormones and hormone therapy during covid-19 pandemic: implications for patients with cancer. Cancers. (2020) 12. doi: 10.3390/cancers12082325
156. Ashraf UM, Abokor AA, Edwards JM, Waigi EW, Royfman RS, Hasan SA, et al. Sars-cov-2, ace2 expression, and systemic organ invasion. Physiol Genomics. (2021) 53:51–60. doi: 10.1152/physiolgenomics.00087.2020
157. Hoffmann M, Kleine-Weber H, Schroeder S, Krüger N, Herrler T, Erichsen S, et al. Sars-cov-2 cell entry depends on ace2 and tmprss2 and is blocked by a clinically proven protease inhibitor. Cell. (2020) 181:271–80.e8. doi: 10.1016/j.cell.2020.02.052
158. Letko M, Marzi A, and Munster V. Functional assessment of cell entry and receptor usage for sars-cov-2 and other lineage B betacoronaviruses. Nat Microbiol. (2020) 5:562–9. doi: 10.1038/s41564-020-0688-y
159. Stilhano RS, Costa AJ, Nishino MS, Shams S, Bartolomeo CS, Breithaupt-Faloppa AC, et al. Sars-cov-2 and the possible connection to ers, ace2, and rage: focus on susceptibility factors. FASEB journal: Off Publ Fed Am Societies Exp Biol. (2020) 34:14103–19. doi: 10.1096/fj.202001394RR
160. Bunders MJ and Altfeld M. Implications of sex differences in immunity for sars-cov-2 pathogenesis and design of therapeutic interventions. Immunity. (2020) 53:487–95. doi: 10.1016/j.immuni.2020.08.003
161. Aguilar-Pineda JA, Albaghdadi M, Jiang W, Vera-Lopez KJ, Nieto-Montesinos R, Alvarez KLF, et al. Structural and functional analysis of female sex hormones against sars-cov-2 cell entry. Int J Mol Sci. (2021) 22. doi: 10.3390/ijms222111508
162. Hasanvand A. Covid-19 and the role of cytokines in this disease. Inflammopharmacology. (2022) 30:789–98. doi: 10.1007/s10787-022-00992-2
163. Soni B, Kabra R, and Singh S. Quantitative insight into immunopathology of sars-cov-2 infection. J interferon cytokine research: Off J Int Soc Interferon Cytokine Res. (2021) 41:244–57. doi: 10.1089/jir.2020.0156
164. Youn JY, Zhang Y, Wu Y, Cannesson M, and Cai H. Therapeutic application of estrogen for covid-19: attenuation of sars-cov-2 spike protein and il-6 stimulated, ace2-dependent nox2 activation, ros production and mcp-1 upregulation in endothelial cells. Redox Biol. (2021) 46:102099. doi: 10.1016/j.redox.2021.102099
165. Vabret N, Britton GJ, Gruber C, Hegde S, Kim J, Kuksin M, et al. Immunology of covid-19: current state of the science. Immunity. (2020) 52:910–41. doi: 10.1016/j.immuni.2020.05.002
166. Harding AT and Heaton NS. The impact of estrogens and their receptors on immunity and inflammation during infection. Cancers. (2022) 14. doi: 10.3390/cancers14040909
167. Shepherd R, Cheung AS, Pang K, Saffery R, and Novakovic B. Sexual dimorphism in innate immunity: the role of sex hormones and epigenetics. Front Immunol. (2020) 11:604000. doi: 10.3389/fimmu.2020.604000
168. Suba Z. Prevention and therapy of covid-19 via exogenous estrogen treatment for both male and female patients. J Pharm Pharm Sci. (2020) 23:75–85. doi: 10.18433/jpps31069
169. Kadel S and Kovats S. Sex hormones regulate innate immune cells and promote sex differences in respiratory virus infection. Front Immunol. (2018) 9:1653. doi: 10.3389/fimmu.2018.01653
170. Vadakedath S, Kandi V, Mohapatra RK, Pinnelli VBK, Yegurla RR, Shahapur PR, et al. Immunological aspects and gender bias during respiratory viral infections including novel coronavirus disease-19 (Covid-19): A scoping review. J Med Virol. (2021) 93:5295–309. doi: 10.1002/jmv.27081
171. Shang Q, Yu X, Sun Q, Li H, Sun C, and Liu L. Polysaccharides regulate th1/th2 balance: A new strategy for tumor immunotherapy. Biomedicine pharmacotherapy = Biomedecine pharmacotherapie. (2024) 170:115976. doi: 10.1016/j.biopha.2023.115976
172. Rojas M, Rodríguez Y, Monsalve DM, Acosta-Ampudia Y, Camacho B, Gallo JE, et al. Convalescent plasma in covid-19: possible mechanisms of action. Autoimmun Rev. (2020) 19:102554. doi: 10.1016/j.autrev.2020.102554
173. Priyanka HP and Nair RS. Neuroimmunomodulation by estrogen in health and disease. AIMS Neurosci. (2020) 7:401–17. doi: 10.3934/Neuroscience.2020025
174. Qin C, Zhou L, Hu Z, Zhang S, Yang S, Tao Y, et al. Dysregulation of immune response in patients with coronavirus 2019 (Covid-19) in wuhan, China. Clin Infect Dis. (2020) 71:762–8. doi: 10.1093/cid/ciaa248
175. Leon MA, Wemlinger SM, Larson NR, Ruffalo JK, Sestak JO, Middaugh CR, et al. Soluble antigen arrays for selective desensitization of insulin-reactive B cells. Mol pharmaceutics. (2019) 16:1563–72. doi: 10.1021/acs.molpharmaceut.8b01250
176. Chakraborty B, Byemerwa J, Krebs T, Lim F, Chang CY, and McDonnell DP. Estrogen receptor signaling in the immune system. Endocrine Rev. (2023) 44:117–41. doi: 10.1210/endrev/bnac017
177. Tamaki M, Konno Y, Kobayashi Y, Takeda M, Itoga M, Moritoki Y, et al. Expression and functional roles of G-protein-coupled estrogen receptor (Gper) in human eosinophils. Immunol Lett. (2014) 160:72–8. doi: 10.1016/j.imlet.2014.03.012
178. Ma Q, Hao ZW, and Wang YF. The effect of estrogen in coronavirus disease 2019. Am J Physiol Lung Cell Mol Physiol. (2021) 321:L219–l27. doi: 10.1152/ajplung.00332.2020
179. Bikdeli B, Madhavan MV, Jimenez D, Chuich T, Dreyfus I, Driggin E, et al. Covid-19 and thrombotic or thromboembolic disease: implications for prevention, antithrombotic therapy, and follow-up: jacc state-of-the-art review. J Am Coll Cardiol. (2020) 75:2950–73. doi: 10.1016/j.jacc.2020.04.031
180. Reyes-García J, Montaño LM, Carbajal-García A, and Wang YX. Sex hormones and lung inflammation. Adv Exp Med Biol. (2021) 1304:259–321. doi: 10.1007/978-3-030-68748-9_15
181. Breithaupt-Faloppa AC, Correia CJ, Prado CM, Stilhano RS, Ureshino RP, and Moreira LFP. 17β-estradiol, a potential ally to alleviate sars-cov-2 infection. Clinics (Sao Paulo). (2020) 75:e1980. doi: 10.6061/clinics/2020/e1980
182. Ghare Naz MS, Banaei M, Dashti S, and Tehrani FR. An overview of sex hormones in relation to sars-cov-2 infection. Future Virol. (2021). doi: 10.2217/fvl-2021-0058
183. Lucas JM, Heinlein C, Kim T, Hernandez SA, Malik MS, True LD, et al. The androgen-regulated protease tmprss2 activates a proteolytic cascade involving components of the tumor microenvironment and promotes prostate cancer metastasis. Cancer Discov. (2014) 4:1310–25. doi: 10.1158/2159-8290.Cd-13-1010
184. Heurich A, Hofmann-Winkler H, Gierer S, Liepold T, Jahn O, and Pöhlmann S. Tmprss2 and adam17 cleave ace2 differentially and only proteolysis by tmprss2 augments entry driven by the severe acute respiratory syndrome coronavirus spike protein. J Virol. (2014) 88:1293–307. doi: 10.1128/jvi.02202-13
185. Stopsack KH, Mucci LA, Antonarakis ES, Nelson PS, and Kantoff PW. Tmprss2 and covid-19: serendipity or opportunity for intervention? Cancer Discov. (2020) 10:779–82. doi: 10.1158/2159-8290.Cd-20-0451
186. Piccinni MP, Giudizi MG, Biagiotti R, Beloni L, Giannarini L, Sampognaro S, et al. Progesterone favors the development of human T helper cells producing th2-type cytokines and promotes both il-4 production and membrane cd30 expression in established th1 cell clones. J Immunol (Baltimore Md: 1950). (1995) 155:128–33. doi: 10.4049/jimmunol.155.1.128
187. Gordon DE, Jang GM, Bouhaddou M, Xu J, Obernier K, White KM, et al. A sars-cov-2 protein interaction map reveals targets for drug repurposing. Nature. (2020) 583:459–68. doi: 10.1038/s41586-020-2286-9
188. Griesbeck M, Scully E, and Altfeld M. Sex and gender differences in hiv-1 infection. Clin Sci (London England: 1979). (2016) 130:1435–51. doi: 10.1042/cs20160112
189. Kovats S. Estrogen receptors regulate innate immune cells and signaling pathways. Cell Immunol. (2015) 294:63–9. doi: 10.1016/j.cellimm.2015.01.018
190. Ramírez-de-Arellano A, Gutiérrez-Franco J, Sierra-Diaz E, and Pereira-Suárez AL. The role of estradiol in the immune response against covid-19. Hormones (Athens Greece). (2021) 20:657–67. doi: 10.1007/s42000-021-00300-7
191. Shu Z, Zhang G, Zhu X, and Xiong W. Estrogen receptor A Mediated M1/M2 macrophages polarization plays a critical role in nash of female mice. Biochem Biophys Res Commun. (2022) 596:63–70. doi: 10.1016/j.bbrc.2022.01.085
192. Hashemzadeh M, Haseefa F, Peyton L, Park S, and Movahed MR. The effects of estrogen and hormone replacement therapy on platelet activity: A review. Am J Blood Res. (2022) 12:33–42.
193. Costa AJ, Lemes RMR, Bartolomeo CS, Nunes TA, Pereira GC, Oliveira RB, et al. Overexpression of estrogen receptor gper1 and G1 treatment reduces sars-cov-2 infection in beas-2b bronchial cells. Mol Cell Endocrinol. (2022) 558:111775. doi: 10.1016/j.mce.2022.111775
Keywords: estrogen, gender difference, immune response, viral infection, molecular mechanism
Citation: Wei X, Hong L, Liang H, Ren K, Man W, Zhao Y and Guo P (2025) Estrogen and viral infection. Front. Immunol. 16:1556728. doi: 10.3389/fimmu.2025.1556728
Received: 08 January 2025; Accepted: 28 April 2025;
Published: 16 May 2025.
Edited by:
Ajay Pradhan, AstraZeneca, SwedenReviewed by:
Anna Ruggieri, National Institute of Health (ISS), ItalyCopyright © 2025 Wei, Hong, Liang, Ren, Man, Zhao and Guo. This is an open-access article distributed under the terms of the Creative Commons Attribution License (CC BY). The use, distribution or reproduction in other forums is permitted, provided the original author(s) and the copyright owner(s) are credited and that the original publication in this journal is cited, in accordance with accepted academic practice. No use, distribution or reproduction is permitted which does not comply with these terms.
*Correspondence: Pengbo Guo, ODc3MjczNjIxQHFxLmNvbQ==
†These authors have contributed equally to this work and share first authorship