Abrasion Induced Volume Loss in Porcine Teeth Measured Using X-Ray Microtomography
- 1School of Mechanical and Design Engineering, University of Portsmouth, Portsmouth, United Kingdom
- 2Glaxosmithkline Consumer Healthcare, Oral Health Innovation, Brentford, United Kingdom
- 3School of Engineering, London South Bank University, London, United Kingdom
Teeth are complex biological structures consisting primarily of enamel and dentin. The mechanical loading of teeth due to the action of brushing provides a complex interaction between applied forces and the biological structure, resulting in volume loss of dental tissue. The volume loss as a resulting of using an ex situ brushing of teeth was measured using x-ray microcomputed tomography (XCT) and corresponding image analyses. Three-dimension reconstructions of teeth reveal a considerably loss of dentin at a rate of over 30 times that of enamel during brushing, as would be expected by the consideration of the corresponding structures. The approach here is flexible and can be applied to a range of brushing, teeth, and environmental conditions.
Introduction
Teeth are a complex biological structure composed primarily of enamel and dentin. The enamel forms the outermost layer of the tooth and is formed of highly ordered apatite prisms (Fincham et al., 1999). These prisms are combined to form enamel rods, which provides a high degree of mechanical performance (Imbeni et al., 2005). Dentin is a collagen and mineral based biocomposite that forms the second major component and comprises the bulk of the tooth volume (Fratzl, 2008). Dentin has a pronounced tubular structure, where the tubules run from the tooth pulp chamber to the dentin-enamel junction. The tubules have a diameter on the order of several micrometers, narrowing in diameter as they approach the surface of the dentin or the dentin-enamel junction (Fratzl, 2008). The primary component of dentin is the intertubular dentin that is a dense fibrillar structure of mainly mineralized collagen (Zaslansky et al., 2006). The intertubular dentin surrounds the highly mineralized peritubular dentin, which in turn surrounds the dentin tubules (Jud et al., 2015). Peritubular dentin is reported to contain no collagen and is composed of highly developed hydroxyapatite crystals (Fratzl, 2008). The main function of dentin is to provide mechanical support for the enamel layer in order to facilitate the translation of the forces from the enamel through to the jaw bone (Zaslansky et al., 2006). The enamel and dentin are designed to work in conjunction, and be resistant to the repeated forces experienced within the oral cavity mainly from mastication (Montoya et al., 2016). The surface of the dentin is covered by a mineralised layer known as the cementum. The cementum generally covers the dentin surface up to the gum line where it meets the enamel (Nanci, 2014). The cementum serves as the binding point between the tooth and the periodontal ligament, which anchors the tooth to the alveolar bone (Bosshardt and Selvig, 1997). The interface between the cementum and enamel is known as the cementoenamel junction. Generally at this junction the cementum will directly interface with the enamel, slightly overlap or in some cases leave a gap of exposed root surface (Arambawatta et al., 2009).
The inherent resistance to mechanical stresses of the enamel is highlighted by the mechanical properties. Enamel has significantly higher mechanical properties when compared to dentin. The elastic modulus of human dental enamel is in the range of 63 to 98 GPa, whereas the dentin has an elastic modulus between 19 and 24 GPa (Fong et al., 2000; Habelitz et al., 2001; Marshall et al., 2001, 2003; Kinney et al., 2003; Inoue et al., 2009; Bertassoni et al., 2011; Yilmaz and Schneider, 2016). The trend is similar when examining the reported hardness values of enamel (2.7 to 4.8 GPa) and dentin (0.55 to 0.81 GPa) (Habelitz et al., 2001; Marshall et al., 2001; Cuy et al., 2002; Low, 2004; Inoue et al., 2009; Sui et al., 2014). Compared to both the enamel and dentin, the cementum has reduced mechanical properties. The elastic modulus of the cementum present in human teeth has been reported to range from 2.3 to 16 GPa (Ho et al., 2004, 2009; Srivicharnkul et al., 2005).
Unlike many biological structures, teeth do not undergo major remodeling following damage or tissue loss (Fratzl, 2008), which means that understanding their wear behavior is important for maintaining healthy teeth. Tooth brushing is widely considered the most common method for routine dental cleaning to maintain healthy teeth (Addy and Hunter, 2003). Brushing of the teeth with the addition of a tooth paste is performed to mechanically remove dental plaque (Dyer et al., 2000). Dental plaques contribute to the development of caries and lesions on the teeth (Hicks et al., 2003). Brushing with a toothpaste has been identified as a contributor to the wear of teeth through abrasion of the tooth surface (Tellefsen et al., 2011). Low mechanical loading of the tooth from brushing, considered as a “normal” brushing condition, is advantageous as little abrasive damage occurs to the enamel such that damage is repaired by the remineralization process of the enamel that occurs within the oral cavity (Wiegand et al., 2007). Understanding damage within the tooth from brushing conditions is therefore important in providing routes to limit this damage and provide repair from remineralization.
A wide range of abrasive forces are applied to the tooth during brushing that are highly dependent on the user and the type of toothbrush, which are grouped into manual or powered, that are used. Studies quantifying the brushing mechanism are limited but some works have measured the brushing force applied by patients using strain gauges attached directly to the toothbrush. Specifically, Ganss et al. reported that patients with a manual tooth brush apply a mean force of 2.4 ± 0.8 N with a maximum force of 5.5 ± 1.9 N (Ganss et al., 2009). Wiegand et al. indicated patients with a manual toothbrush applied a mean force of 1.6 N and mean force of 0.9 N with a powered toothbrush (Wiegand et al., 2013). In both studies the patients were uninstructed regarding brushing force or technique. These studies show that the brushing forces applied to the tooth are varied but can be relatively high, which can lead to abrasion of the tooth surface when combined with a toothpaste (Kielbassa et al., 2005).
Many studies have investigated the effects of tooth brushing on the wear of dental tissue in conjunction with the effects of erosion (Attin et al., 1997; Hooper et al., 2003; Wiegand et al., 2007; Lima et al., 2013; Bizhang et al., 2016). The erosion is included in these studies to replicate the effects of acid mediated softening of the dental tissue, as sound dental tissue is considered as not susceptible to abrasive wear alone (Kielbassa et al., 2005). The softening by acidic substances or foods increases the susceptibility of the dental tissue to abrasive damage. In addition to the erosive damage leading to increased wear, gingival recession is one route that can lead to exposure of the underlying dentin (Bizhang et al., 2016). Dentin has reduced mechanical resistance to abrasion when compared to enamel, and has been shown to be susceptible to significant abrasive damage following an erosive attack (Bizhang et al., 2016).
To study the wear characteristics of enamel and dentin, the abrasive wear is conducted over a period repeated brushing cycles on polished sections of dental material. These abrasive wear studies often exclude the presence of the cementoenamel junction and cementum on the surface of the dentin or completely remove the layer prior to beginning the study. The total wear is often assessed using profilometry over a specific region of the brushed area to measure the mean change or peak change in the height of the sample within the brushed region, which provides a one dimension characterization of the damage (Dyer et al., 2000; Hooper et al., 2003; Kielbassa et al., 2005; Wiegand et al., 2013; Arnold et al., 2016; Bizhang et al., 2016). The one dimensional analysis of the wear provides a convenient method of drawing comparisons between varying conditions applied between the brush and the tooth surface, but provides less information about the overall changes occurring within the tooth structure during the wear process.
Extending the analysis to three dimensions would provide extensive information on changes in tooth volume due to the applied brushing forces. X-ray microcomputed tomography (XCT) is a powerful technique to acquire images of an entire volume at the micrometer length scale. The ability of XCT to accurately evaluate volume changes in teeth due to brushing forces has not been fully explored and evidence within the literature of quantifying small, micrometer scale, volume loss in teeth using XCT is lacking. X-ray tomography techniques have been exploited to investigate large scale abrasion wear in teeth (Kofmehl et al., 2010) but does not consider loading from a contacting toothbrush. We believe state-of-the-art x-ray microscopy techniques are not fully realized as a potential method for exploring volumetric wear in dental materials. The aim of this work is therefore to investigate the abrasive wear of tooth brushing on teeth using XCT. A particular emphasis is on quantifying the total volume loss within the enamel and dentin as a result of ex situ brushing using only the movement applied by a (powered) sonic toothbrush.
Methods
Porcine teeth were extracted from the lower jaw of a pig and cleaned under running water to remove any excess soft tissue. The individual teeth were then mounted to aluminum tubing using epoxy resin (Devcon 5 minute epoxy, ITW Devcon, IE) in order to secure the teeth in the sample holders for the XCT. Aluminum phantoms, ~0.2 mm in diameter, were adhered to the surface of the tooth adjacent to the region of interest using epoxy resin. The phantoms provide a reference point on the sample during image processing.
Tooth brushing was performed using a powered sonic toothbrush (Philips Sonicare Easyclean, Philips, USA) and a toothpaste slurry with a ratio of 1:3 toothpaste (Sensodyne Daily Care, GSK, UK) to water. The toothbrush was secured on a ring stand and static weights were attached to the main handle of the toothbrush. The force applied to the tooth through the toothbrush head was 2.8 N. The toothbrush head was immersed in the toothpaste slurry then attached to the toothbrush prior to brushing. Brushing consisted of contacting the long length of the tooth approximately at the gum-line position and activating the toothbrush. The experimental setup for tooth brushing is shown in Figure 1. Using this region of interested ensured that both enamel, present at the surface of the tooth above the gum-line, and dentin, present at the surface of the tooth below the gum-line, were examined in this work to explore differences in their response to the brushing. Each tooth was brushed at 2 min time intervals for up to 20 min, with the tooth rinsed using deionized water, dried using laboratory wipes and imaged prior to the subsequent brushing interval. A total of three teeth were imaged and exposed to the tooth brushing cycles.
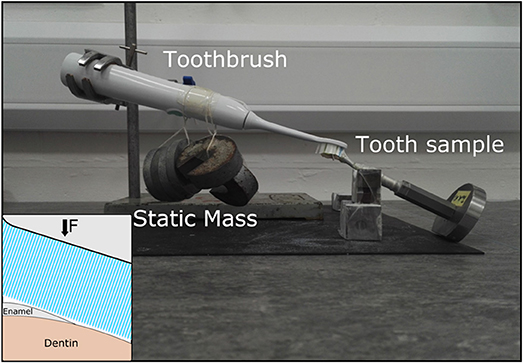
Figure 1. Optical image of the ex situ brushing set up. Static weights are applied to the central portion of the electric toothbrush. The tooth sample is mounted in the XCT sample holder and stabilized by a steel v-block. A schematic of the brushing location is shown in the bottom left hand corner of the image.
XCT was conducted using an x-ray microscope (Versa 510, Carl Zeiss, USA/EU) operating at a voltage of 50 kV with a power of 4 W and a Zeiss LE2 filter positioned directly after the x-ray source to filter the x-ray spectrum. One thousand and six hundred and one projections were collected over 360°at an interval of 0.22° with an isotropic voxel size of 3 μm for each tomography reconstruction. An exposure time of 5–6 s was used for each projection. The exposure time was selected individually for each sample and maintained throughout the study. Two initial scans were conducted for each sample; between the initial scans no changes were made to the experimental equipment or the position of the sample. The projections were reconstructed using software incorporating a filtered back projection algorithm (Scout and Scan, Carl Zeiss, USA/EU). For each dataset the center shift was manually found, the standard beam hardening correction was applied with a value of 0.2–0.7 depending on the specific sample and a smoothing correction of 0.5–0.7 used. The beam hardening and smoothing corrections were kept constant for each individual sample.
The reconstructed datasets were filtered using a non-local means denoising filter in ImageJ (NIST, USA) (Darbon et al., 2008; Buades et al., 2011). The filtered datasets were registered to the initial scans using the manual rigid registration module in MeVisLab (MeVis Medical Solutions AG, DE). The aluminum phantoms were used as key features for the registration process. Registration of the datasets ensured that the center of each scan was aligned and a consistent region of interest (ROI) could be selected from each dataset.
The specific ROI from each dataset were segmented through pixel classification using the ilastik software (Sommer et al., 2011). Pixel classification was carried out using 15 features: Gaussian Smoothing (σ = 0.3 px−3.5 px), Laplacian of Gaussian (σ = 0.7 px−5.0 px), Structure Tensor Eigenvalues (σ = 0.7 px−5.0 px). Each dataset was segmented individually using three labels. The labels were selected to represent the enamel, dentin, and air present in the images. Each label is represented by a single color value within the segmented data, resulting in a dataset containing only three pixel color values. The volume of each label was calculated by counting the number of pixels for each specific color within each slice of the ROI. The total number of pixels for each color within a dataset were counted using a custom python script. The volume was then calculated by multiplying the number of pixels by the voxel size of the images.
Results
Porcine teeth were successfully imaged using XCT, focusing mainly on the region of the tooth surface that transitions from enamel to dentin. Figure 2 shows the 3D rendered volume of an imaged tooth and highlights the presence of both the enamel and the dentin within the imaged volume. A plane section of the imaged volume is shown in Figure 3, where the enamel and dentin can be distinguished clearly. Figure 3A also highlights the cementum at the surface of the tooth, which is indicated by the change in the image morphology. The interface between the cementum and dentin is not clear from the XCT images, but was confirmed using light microscopy (Supplementary Figure 1). The light microscopy images indicated that the cementum in these samples connects directly to the enamel and there is no overlap of the two layers. The artifacts present within Figure 3 are likely caused by the presence of the aluminum phantom adhered to the tooth surface. Figure 3 shows the plane section that has been produced using a non-local means denoising filter and indicates that the filter has reduced the overall noise while maintaining the features of the sample.
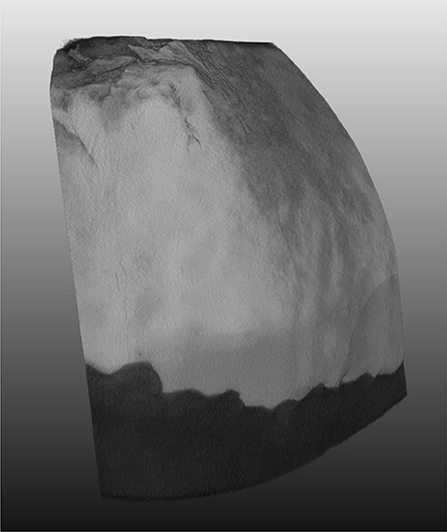
Figure 2. 3D volume rendering of the scanned region of the tooth. A greyscale lookup table is applied to the rendering, showing the enamel in the lighter coloration and the dentin the darker coloration.
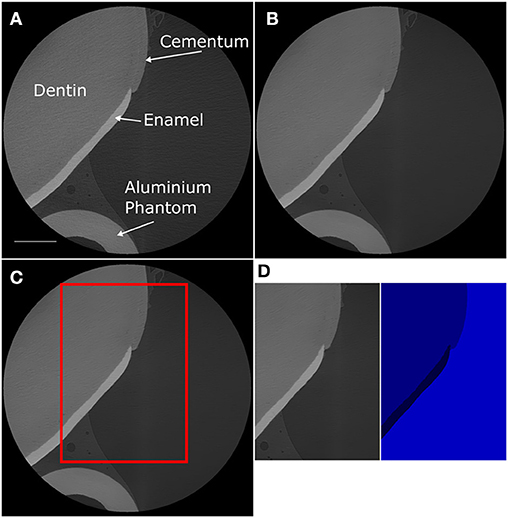
Figure 3. 2D virtual slices of the 3D tomography data captured via XCT. (A) Initial reconstructed slice. (B) Reconstructed slice following application of a NLM denoising filter. (C) The red box highlights the ROI selected for calculating the volume of enamel and dentin. (D) The selected region of interest and the resulting segmented data.
The filtered image datasets were segmented to accurately distinguish between the enamel, dentin and air in order to quantify the volume of each phase present. The volume of the enamel and dentin present within each sample at each time point is recorded in Table 1. The table also displays the volume data for the initial repeat scans. Table 1 shows the change in volume at each time point against the initial reference scan, labeled initial scan 1, which shows an increase in the volume lost in both the enamel and dentin over the course of the total brushing time. The size of the ROI varies for each sample, therefore to draw comparisons between each samples the data is normalized against the total measured volume of the ROI in the initial scan. The total volume of the ROI and the normalized volume at each time point are displayed in Table 1. The average change in the volume of each phase as a function of the applied brushing time can therefore be observed and is plotted in Figure 4. A linear regression fit to the data to describe the rate of volume loss within each phase was made.
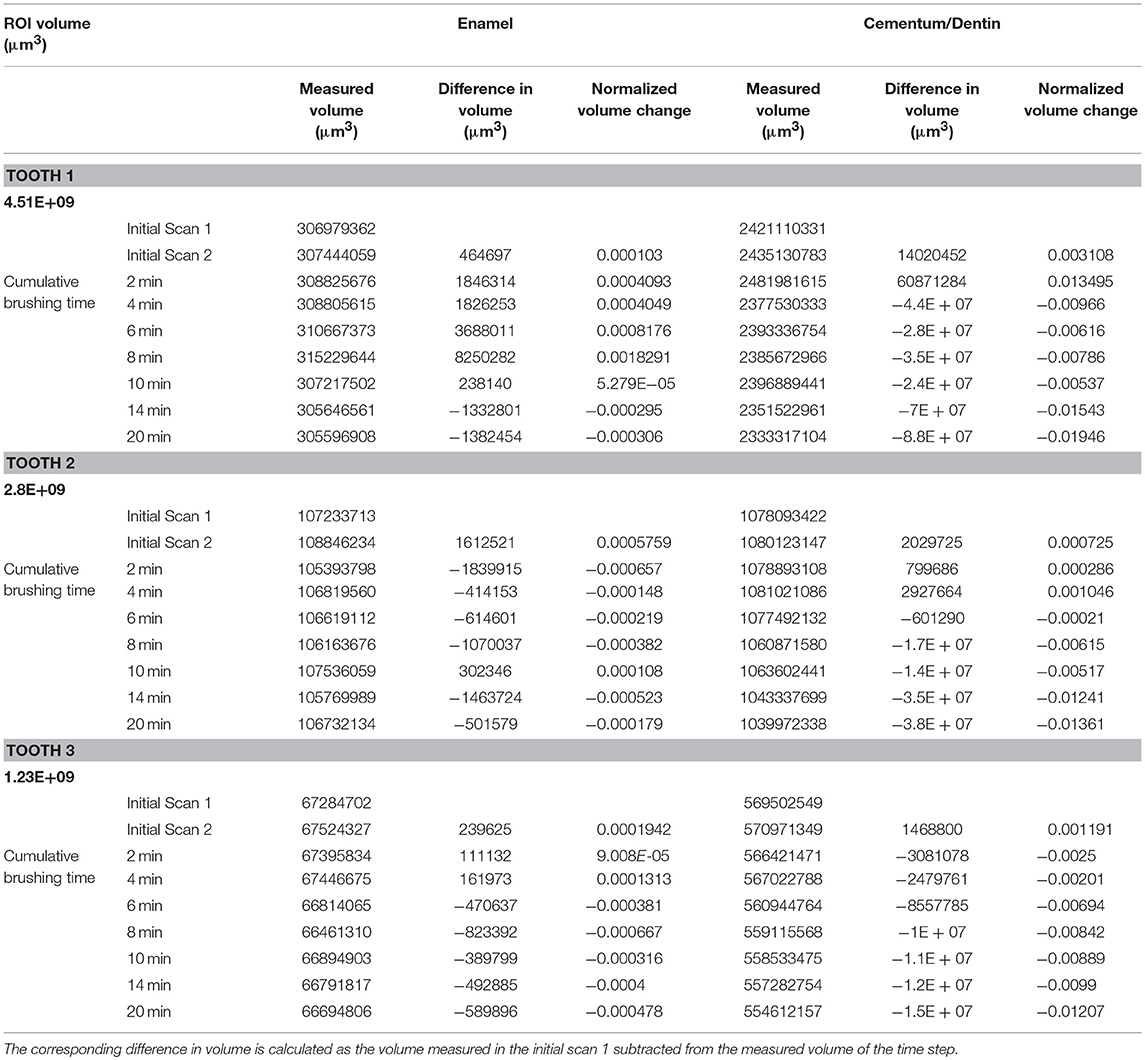
Table 1. List of the volumetric data calculated from the XCT images captured for each tooth sample over 10 brushing time points.
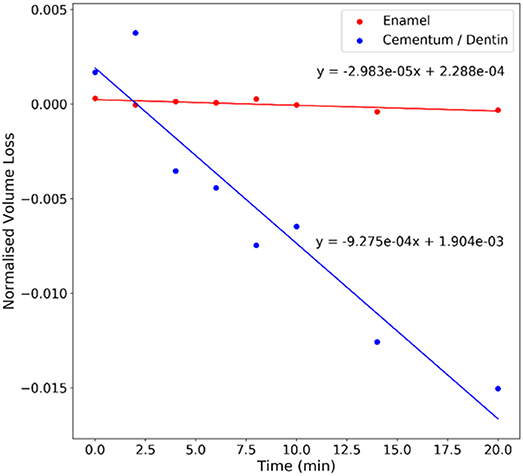
Figure 4. Plot of the average normalized changes in volume for the enamel and cementum/dentin vs. the cumulative brushing time (in minutes).
Discussion
In the XCT images the cementum at the surface of the tooth can be identified but the interface between the cementum and the dentin cannot be consistently differentiated. The lack of contrast between the cementum and dentin is likely due to the similiarity of mineral concentration for each material. Dentin has ~60% hydroxyapatite, where cementum has between 45 and 50% (Ho et al., 2004; Srivicharnkul et al., 2005; Goldberg, 2011; Yamaguchi et al., 2016) The high mineral content of the enamel, over 90% (Habelitz et al., 2001; Goldberg et al., 2014; Yilmaz and Schneider, 2016), results in significant contrast to the cementum and dentin. Therefore, it is not possible to indicate when the abrasive behavior of the tooth brushing has completely removed the cementum and then begun to remove the underlying dentin. The removal of the cementum from the tooth surface exposes the dentin and the openings of the dentin tubules, which has been shown to lead to hypersensitivity (Addy and Hunter, 2003; Arnold et al., 2016). As a result of this, the discussion here considers the dentin and cementum as one material component of the tooth and the enamel as another.
The plots in Figure 4 indicate a substantial increase in the volume of the cementum/dentin lost when compared to the enamel. This difference in rate of volume loss was expected as the enamel has greater mechanical properties and is a biological structure that is designed to withstand long term exposure to wear forces. An initial increase in the normalized volume changes in both the enamel and dentin is noted and is expected to be due to initial deposition of material or redeposition of loose material within the ROI, which is absent as removal of the tooth material becomes active.
The increased wear resistance observed in the enamel is consistent with the literature, where brushing of enamel produces minimal volume loss when compared to the dentin and also holds for acid softened/eroded dentin and enamel (Wiegand et al., 2007, 2013). The consistency with previous work can be evaluated by comparing the ratio of the rate of dentin volume loss to rate of enamel volume loss. Wiegand et al. showed, using profilometry, that brushing of enamel and dentin with powered sonic toothbrushes resulted in an average dentin to enamel volume loss ratio of 12.6:1 (Wiegand et al., 2013). The data we present here indicates an average cementum/dentin to enamel volume loss ratio of 35.48:1, which indicates a more rapid volume loss compared to the literature. The ratio was calculated using the slope from the linear regression of the volume loss vs. time data for each sample. This increase in the ratio appears to be related to the applied force during brushing, where increasing the applied force by a factor of three increased the ratio of volume loss by a similar factor. The force applied in this work was ~2.8 N, while Wiegand et al. applied 0.9 N with the sonic toothbrush (Wiegand et al., 2013). The increase of the ratio with force indicates that the increase in cementum/dentin volume loss observed here is not dependent on the material properties, and is potentially related to the experimental methodology. The work presented by Wiegand et al. used controlled sample geometry, whereas in this work a geometry more representative of in vivo brushing was employed to take advantage of the XCT imaging capabilities. The irregular geometry used in this work may lead to increased contact stresses arising at the surface during brushing that may not be observed using polished blocks used for profilometric studies. In addition, the sample preparation techniques employed in other works, namely mechanical surface polishing, may preferentially remove weakly bound or damaged material prior to the wear testing. Specifically, the polishing in Wiegand et al. removed 200 μm from the surface of the enamel and dentin block faces (Wiegand et al., 2013), thus potentially removing weaker material, and the cementum, that could be lost more easily due to brushing. Polishing is also expected to defects from the surface that act as initiation sites for damage or volume removal. Future work should therefore examine the interaction of the toothbrush head with the dentin and enamel surfaces using in situ XCT to provide better insight into how the contact stresses are being applied to an irregular tooth surface.
Summary
The volume change of teeth following ex situ tooth brushing was successfully measured using XCT to observe the wear characteristics of teeth. The rate of volume loss for enamel was observed to be lower than that of the cementum/dentin within these teeth samples, consistent with the literature of brushing performed on dental material. Specifically, the rate of volume loss of cementum/dentin was found to be over 30 times higher than for enamel. The methodology for experimentally measuring the volume loss using x-ray imaging and corresponding image analyses provide robust approaches for assessing a wide range of brushing interactions with teeth in relevant 3-dimensional space.
Ethics Statement
All porcine material was obtained from a local butcher. Ethics approval was not required as per the University of Portsmouth's guidelines and national regulations.
Author Contributions
AK, AB, and SK devised the experiments. AK carried out the experimental work. Data analysis was carried out by AK, AB, and SK. All authors contributed to the writing of the manuscript.
Funding
The work was supported by the GSK grant Multiscale imaging and mechanical evaluation of biomaterials in the oral cavity.
Conflict of Interest Statement
The authors declare that the research was conducted in the absence of any commercial or financial relationships that could be construed as a potential conflict of interest.
Supplementary Material
The Supplementary Material for this article can be found online at: https://www.frontiersin.org/articles/10.3389/fmech.2018.00012/full#supplementary-material
Supplementary Figure 1. Light microscopy image of the cross section of a porcine tooth used in this study. The image shows the presence of the enamel, dentin, cementum and cementoenamel junction. Scale bar is 0.1 mm.
References
Addy, M., and Hunter, M. L. (2003). Can tooth brushing damage your health? Effects on oral and dental tissues. Int. Dent. J. 53, 177–186. doi: 10.1111/j.1875-595X.2003.tb00768.x
Arambawatta, K., Peiris, R., and Nanayakkara, D. (2009). Morphology of the cemento-enamel junction in premolar teeth. J. Oral Sci. 51, 623–627. doi: 10.2334/josnusd.51.623
Arnold, W. H., Gröger, C., Bizhang, M., and Naumova, E. A. (2016). Dentin abrasivity of various desensitizing toothpastes. Head Face Med. 12:16. doi: 10.1186/s13005-016-0113-1
Attin, T., Koidl, U., Buchalla, W., Schaller, H. G., Kielbassa, A. M., and Hellwig, E. (1997). Correlation of microhardness and wear in differently eroded bovine dental enamel. Arch. Oral Biol. 42, 243–250. doi: 10.1016/0003-9969(06)00073-2
Bertassoni, L. E., Habelitz, S., Pugach, M., Soares, P. C., Marshall, J., William, G., et al. (2011). Following remineralization of dentin. Scanning 32, 312–319. doi: 10.1002/sca.20199
Bizhang, M., Riemer, K., Arnold, W. H., Domin, J., and Zimmer, S. (2016). Influence of bristle stiffness of manual toothbrushes on eroded and sound human dentin - an in vitro study. PLoS ONE 11:e0153250. doi: 10.1371/journal.pone.0153250
Bosshardt, D. D., and Selvig, K. A. (1997). Dental cementum: the dynamic tissue covering of the root. Periodontol. 2000 13, 41–75. doi: 10.1111/j.1600-0757.1997.tb00095.x
Buades, A., Coll, B., and Morel, J. (2011). Non-local means denoising. Image Process. Line 1, 208–212. doi: 10.5201/ipol.2011.bcm_nlm
Cuy, J. L., Mann, A. B., Livi, K. J., Teaford, M. F., and Weihs, T. P. (2002). Nanoindentation mapping of the mechanical properties of human molar tooth enamel. Arch. Oral Biol. 47, 281–291. doi: 10.1016/S0003-9969(02)00006-7
Darbon, J., Cunha, A., Chan, T. F., Osher, S., and Jensen, G. J. (2008). “Fast nonlocal filtering applied to electron cryomicroscopy,” in 2008 5th IEEE International Symposium on Biomedical Imaging: From Nano to Macro, Proceedings, ISBI (Paris), 1331–1334. doi: 10.1109/ISBI.2008.4541250
Dyer, D., Addy, M., and Newcombe, R. G. (2000). Studies in vitro of abrasion by different manual toothbrush heads and a standard toothpaste. J. Clin. Periodontol. 27, 99–103. doi: 10.1034/j.1600-051x.2000.027002099.x
Fincham, A. G., Moradian-Oldak, J., and Simmer, J. P. (1999). The structural biology of the developing dental enamel matrix. J. Struct. Biol. 126, 270–299. doi: 10.1006/jsbi.1999.4130
Fong, H., Sarikaya, M., White, S. N., and Snead, M. L. (2000). Nano-mechanical properties profiles across dentin–enamel junction of human incisor teeth. Mater. Sci. Eng. C 7, 119–128. doi: 10.1016/S0928-4931(99)00133-2
Fratzl, P. (ed.). (2008). Collagen: Structure and Mechanics. Boston, MA: Springer US. doi: 10.1007/978-0-387-73906-9
Ganss, C., Schlueter, N., Preiss, S., and Klimek, J. (2009). Tooth brushing habits in uninstructed adults - Frequency, technique, duration and force. Clin. Oral Investig. 13, 203–208. doi: 10.1007/s00784-008-0230-8
Goldberg, M. (2011). Dentin structure composition and mineralization. Front. Biosci. E3:281. doi: 10.2741/e281
Goldberg, M., Kellermann, O., Dimitrova-Nakov, S., Harichane, Y., and Baudry, A. (2014). Comparative studies between mice molars and incisors are required to draw an overview of enamel structural complexity. Front. Physiol. 5:359. doi: 10.3389/fphys.2014.00359
Habelitz, S., Marshall, S., Marshall, G., and Balooch, M. (2001). Mechanical properties of human dental enamel on the nanometre scale. Arch. Oral Biol. 46, 173–183. doi: 10.1016/S0003-9969(00)00089-3
Hicks, J., Garcia-Godoy, F., and Flaitz, C. (2003). Biological factors in dental caries: role of saliva and dental plaque in the dynamic process of demineralization and remineralization (part 1). J. Clin. Pediatr. Dent. 28, 47–52. doi: 10.17796/jcpd.28.1.yg6m443046k50u20
Ho, S. P., Balooch, M., Goodis, H. E., Marshall, G. W., and Marshall, S. J. (2004). Ultrastructure and nanomechanical properties of cementum dentin junction. J. Biomed. Mater. Res. 68A, 343–351. doi: 10.1002/jbm.a.20061
Ho, S. P., Senkyrikova, P., Marshall, G. W., Yun, W., Wang, Y., Karan, K., et al. (2009). Structure, chemical composition and mechanical properties of coronal cementum in human deciduous molars. Dent. Mater. 25, 1195–1204. doi: 10.1016/j.dental.2009.04.005
Hooper, S., West, N. X., Pickles, M. J., Joiner, A., Newcombe, R. G., and Addy, M. (2003). Investigation of erosion and abrasion on enamel and dentine: a model in situ using toothpastes of different abrasivity. J. Clin. Periodontol. 30, 802–808. doi: 10.1034/j.1600-051X.2003.00367.x
Imbeni, V., Kruzic, J. J., Marshall, G. W., Marshall, S. J., and Ritchie, R. O. (2005). The dentin-enamel junction and the fracture of human teeth. Nat. Mater. 4, 229–232. doi: 10.1038/0nmat1323
Inoue, T., Saito, M., Yamamoto, M., Debari, K., Kou, K., and Nishimura, F. (2009). Comparison of nanohardness between coronal and radicular intertubular dentin Dent. Mater. J. 28, 295–300. doi: 10.4012/dmj.28.295
Jud, C., Schaff, F., Zanette, I., Wolf, J., Fehringer, A., and Pfeiffer, F. (2015). Dentinal tubules revealed with X-ray tensor tomography. Dent. Mater. 32, 1189–1195. doi: 10.1016/j.dental.2016.06.021
Kielbassa, A. M., Gillmann, L., Zantner, C., Meyer-Lueckel, H., Hellwig, E., and Schulte-Mönting, J. (2005). Profilometric and microradiographic studies on the effects of toothpaste and acidic gel abrasivity on sound and demineralized bovine dental enamel. Caries Res. 39, 380–386. doi: 10.1159/000086844
Kinney, J. H., Marshall, S. J., and Marshall, G. W. (2003). The mechanical properties of human dentin: a critical review and re-evaluation of the dental literature. Crit. Rev. Oral Biol. Med. 14, 13–29. doi: 10.1177/154411130301400103
Kofmehl, L., Schulz, G., Deyhle, H., Filippi, A., Hotz, G., Berndt-Dagassan, D., et al. (2010). Computed tomography to quantify tooth abrasion. Proc. SPIE 7804, 1–10. doi: 10.1117/12.859278
Lima, J. P. M., Melo, M. A. S., Passos, V. F., Braga, C. L. N., Rodrigues, L. K. A., and Santiago, S. L. (2013). Dentin erosion by whitening mouthwash associated to toothbrushing abrasion: a focus variation 3D scanning microscopy study. Microsc. Res. Tech. 76, 904–908. doi: 10.1002/jemt.22246
Low, I. M. (2004). Depth-profiling of crystal structure, texture, and microhardness in a functionally graded tooth enamel. J. Am. Ceram. Soc. 87, 2125–2131. doi: 10.1111/j.1151-2916.2004.tb06369.x
Marshall, G. W., Balooch, M., Gallagher, R. R., Gansky, S. A., and Marshall, S. J. (2001). Mechanical properties of the dentinoenamel junction: AFM studies of nanohardness, elastic modulus, and fracture. J. Biomed. Mater. Res. 54, 87–95. doi: 10.1002/1097-4636(200101)54:1<87::AID-JBM10>3.0.CO;2-Z
Marshall, S. J., Balooch, M., Habelitz, S., Balooch, G., Gallagher, R., and Marshall, G. W. (2003). The dentin - enamel junction - a natural, multilevel interface. J. Eur. Ceram. Soc. 23, 2897–2904. doi: 10.1016/S0955-2219(03)00301-7
Montoya, C., Arola, D., and Ossa, E. A. (2016). Importance of tubule density to the fracture toughness of dentin. Arch. Oral Biol. 67, 9–14. doi: 10.1016/j.archoralbio.2016.03.003
Nanci, A. (2014). Ten Cate's Oral Histology - E-Book: Development, Structure, and Function. Elsevier Health Sciences. Available online at: https://books.google.co.uk/books?id=I8fsAwAAQBAJ
Sommer, C., Straehle, C., Kothe, U., and Hamprecht, F. A. (2011). “Ilastik: interactive learning and segmentation toolkit,” in Proceedings - IEEE International Symposium on Biomedical Imaging (Chicago, IL), 230–233. doi: 10.1109/ISBI.2011.5872394
Srivicharnkul, P., Kharbanda, O. P., Swain, M. V., Petocz, P., and Darendeliler, M. A. (2005). Physical properties of root cementum: part 3. Hardness and elastic modulus after application of light and heavy forces. Am. J. Orthod. Dentofac. Orthop. 127, 168–176. doi: 10.1016/j.ajodo.2003.12.021
Sui, T., Sandholzer, M. A., Le Bourhis, E., Baimpas, N., Landini, G., and Korsunsky, A. M. (2014). Structure-mechanical function relations at nano-scale in heat-affected human dental tissue. J. Mech. Behav. Biomed. Mater. 32, 112–124. doi: 10.1016/j.jmbbm.2013.12.014
Tellefsen, G., Liljeborg, A., Johannsen, A., and Johannsen, G. (2011). The role of the toothbrush in the abrasion process. Int. J. Dent. Hyg. 9, 284–290. doi: 10.1111/j.1601-5037.2011.00505.x
Wiegand, A., Burkhard, J. P. M., Eggmann, F., and Attin, T. (2013). Brushing force of manual and sonic toothbrushes affects dental hard tissue abrasion. Clin. Oral Investig. 17, 815–822. doi: 10.1007/s00784-012-0788-z
Wiegand, A., Köwing, L., and Attin, T. (2007). Impact of brushing force on abrasion of acid-softened and sound enamel. Arch. Oral Biol. 52, 1043–1047. doi: 10.1016/j.archoralbio.2007.06.004
Yamaguchi, M., Yao-Umezawa, E., Tanimoto, Y., Shimizu, M., Kikuta, J., Hikida, T., et al. (2016). Individual variations in the hardness and elastic modulus of the human cementum. J. Hard Tissue Biol. 25, 345–350. doi: 10.2485/jhtb.25.345
Yilmaz, E. D., and Schneider, G. A. (2016). Mechanical behavior of enamel rods under micro-compression. J. Mech. Behav. Biomed. Mater. 63, 183–194. doi: 10.1016/j.jmbbm.2016.06.017
Keywords: X-ray microtomography, tooth, mechanical processes, structural biology, abrasion
Citation: Kao AP, Khan S and Barber AH (2018) Abrasion Induced Volume Loss in Porcine Teeth Measured Using X-Ray Microtomography. Front. Mech. Eng. 4:12. doi: 10.3389/fmech.2018.00012
Received: 08 January 2018; Accepted: 06 September 2018;
Published: 27 September 2018.
Edited by:
Federico Bosia, Università degli Studi di Torino, ItalyReviewed by:
Victor E. Arana-Chavez, Universidade de São Paulo, BrazilUrszula Stachewicz, AGH University of Science and Technology, Poland
Copyright © 2018 Kao, Khan and Barber. This is an open-access article distributed under the terms of the Creative Commons Attribution License (CC BY). The use, distribution or reproduction in other forums is permitted, provided the original author(s) and the copyright owner(s) are credited and that the original publication in this journal is cited, in accordance with accepted academic practice. No use, distribution or reproduction is permitted which does not comply with these terms.
*Correspondence: Asa H. Barber, barbera8@lsbu.ac.uk