- 1Department of Translational Research and New Technologies in Medicine and Surgery, University of Pisa, Pisa, Italy
- 2Laboratory of Pharmaceutical Microbiology, Ghent University, Ghent, Belgium
- 3Department of Biomedical Sciences, University of Cagliari, Cagliari, Italy
Antimicrobial peptides (AMPs) are promising templates for the development of novel antibiofilm drugs. Despite the large number of studies on screening and optimization of AMPs, only a few of these evaluated the antibiofilm activity in physiologically relevant model systems. Potent in vitro activity of AMPs often does not translate into in vivo effectiveness due to the interference of the host microenvironment with peptide stability/availability. Hence, mimicking the complex environment found in biofilm-associated infections is essential to predict the clinical potential of novel AMP-based antimicrobials. In the present study, we examined the antibiofilm activity of the semi-synthetic peptide lin-SB056-1 and its dendrimeric derivative (lin-SB056-1)2-K against Pseudomonas aeruginosa in an in vivo-like three-dimensional (3-D) lung epithelial cell model and an in vitro wound model (consisting of an artificial dermis and blood components at physiological levels). Although moderately active when tested alone, lin-SB056-1 was effective in reducing P. aeruginosa biofilm formation in association with 3-D lung epithelial cells in combination with the chelating agent EDTA. The dimeric derivative (lin-SB056-1)2-K demonstrated an enhanced biofilm-inhibitory activity as compared to both lin-SB056-1 and the lin-SB056-1/EDTA combination, reducing the number of biofilm-associated bacteria up to 3-Log units at concentrations causing less than 20% cell death. Biofilm inhibition by (lin-SB056-1)2-K was reported both for the reference strain PAO1 and cystic fibrosis lung isolates of P. aeruginosa. In addition, using fluorescence microscopy, a significant decrease in biofilm-like structures associated with 3-D cells was observed after peptide exposure. Interestingly, effectiveness of (lin-SB056-1)2-K was also demonstrated in the wound model with a reduction of up to 1-Log unit in biofilm formation by P. aeruginosa PAO1 and wound isolates. Overall, combination treatment and peptide dendrimerization emerged as promising strategies to improve the efficacy of AMPs, especially under challenging host-mimicking conditions. Furthermore, the results of the present study underlined the importance of evaluating the biological properties of novel AMPs in in vivo-like model systems representative of specific infectious sites in order to make a more realistic prediction of their therapeutic success, and avoid the inclusion of unpromising peptides in animal studies and clinical trials.
Introduction
The opportunistic pathogen Pseudomonas aeruginosa is known to play a role in many types of infections, including lung infections, wound infections, otitis media and medical device-associated infections (Taylor et al., 2014; Rybtke et al., 2015). P. aeruginosa infections range from minor external infections to serious and life-threatening conditions, such as in individuals with CF (Gellatly and Hancock, 2013). Intrinsic and acquired resistance to many conventional antibiotics makes the treatment of P. aeruginosa infections particularly challenging (Breidenstein et al., 2011). The ability of P. aeruginosa to form biofilms further complicates the problem of antimicrobial resistance causing high rates of failure in antibiotic therapy and chronic infection of the host (Parsek and Singh, 2003; Mulcahy et al., 2014). Therefore, the identification of novel antimicrobial agents that can effectively suppress P. aeruginosa biofilm-related infections is a research priority.
Over the last years, AMPs have gained increasing attention as candidates for the development of innovative antimicrobial drugs. Due to their main mechanism of action, which relies on the permeabilization of bacterial membranes, AMPs exhibit broad-spectrum activity, a low tendency to induce resistance, and a high potential to target metabolically dormant cells that are found at elevated rate within microbial biofilms (Batoni et al., 2016b; Grassi et al., 2017a). A number of AMPs have been tested for their antibiofilm activity (BaAMPs database1), demonstrating a variable effectiveness in interfering with the different stages of biofilm formation (Di Luca et al., 2015; de la Fuente-Núñez et al., 2016). Despite the large number of studies reporting the screening and optimization of AMPs, only a few of these evaluated the antibiofilm activity in clinically relevant model systems (Lashua et al., 2016; Crabbé et al., 2017; de Breij et al., 2018). Nevertheless, the specific environmental conditions found at the site of infection have been shown to influence both biofilm architecture and susceptibility and to interfere with the activity and stability of AMPs (Bjarnsholt et al., 2013; Crabbé et al., 2017). For this reason, potent in vitro activity of AMPs does not necessarily translate into in vivo effectiveness, resulting in a limited predictive value (Mardirossian et al., 2017; Dostert et al., 2018). Therefore, the screening of AMPs in conditions mimicking the host microenvironment and the in vivo host–pathogen interactions represents an essential step to assess the clinical potential of novel AMP-based antimicrobials.
The semi-synthetic peptide lin-SB056-1 has been recently designed as a beta-strand with a perfect pattern of alternating hydrophilic and hydrophobic residues in the primary structure in order to maximize bacterial membrane interaction and killing (Manzo et al., 2015). The regular amphipathic profile of lin-SB056-1 significantly enhanced the antibacterial properties of the peptide, which displayed a rapid and strong activity against a broad spectrum of Gram-positive and Gram-negative bacteria, including multidrug-resistant clinical isolates of P. aeruginosa (Maisetta et al., 2017). In addition to exerting a marked antibiofilm activity in standard conditions, lin-SB056-1 in combination with EDTA demonstrated the ability to almost completely inhibit the formation of P. aeruginosa biofilm-like structures in an artificial sputum medium mimicking the lung environment of CF patients (Maisetta et al., 2017). Since dendrimeric peptides are known to exhibit enhanced antimicrobial activity as compared to their monomeric counterparts (Lind et al., 2015; Pires et al., 2015), lin-SB056-1 has been further optimized by generating a two-branched polypeptide with a lysine linker and a lipidic tail (den-SB056-1) (Batoni et al., 2016a). Interestingly and differently from the monomeric structure, den-SB056-1 has been demonstrated to preserve high affinity for negatively charged membranes and to retain its antibacterial activity even in the presence of physiological salt concentrations (Batoni et al., 2016a). Since the inhibition of AMPs by salts represents a severe limitation to their applicability (Maisetta et al., 2008), maintenance of activity at high ionic strength makes the dendrimeric derivative particularly promising for the use in physiological conditions (Polcyn et al., 2013). In the current study, a slightly different dimeric derivative of lin-SB056-1, referred to as (lin-SB056-1)2-K, has been obtained starting from den-SB056-1 through the elimination of the lipidic moiety. Indeed, a recent study has reported that the lipidic tail does not play a fundamental role in the membrane-binding affinity as well as in the antimicrobial properties of the peptide (Manzo et al., 2016).
In the present study, combination treatment (i.e., lin-SB056-1 used in combination with EDTA) and peptide dendrimerization [i.e., design of (lin-SB056-1)2-K] were investigated as two promising strategies to improve the antibacterial and antibiofilm activity of lin-SB056-1 against P. aeruginosa under in vivo-like conditions. In particular, the antimicrobial properties of the lin-SB056-1/EDTA combination and/or (lin-SB056-1)2-K were assessed in two representative model systems of biofilm-associated infections, namely an in vivo-like three-dimensional (3-D) lung epithelial cell model and an artificial wound model. Organotypic 3-D lung models have been previously developed by using the RWV bioreactor technology, which allows to replicate aspects of the in vivo lung infection with P. aeruginosa biofilms (Carterson et al., 2005; Barrila et al., 2010; Crabbé et al., 2014, 2017). In order to mimic chronic wound infections, we exploited an in vitro wound model reflecting the nutritional conditions found in wound beds, and resembling the actual surface on which bacterial aggregates are formed in vivo (Brackman and Coenye, 2016; Trivedi et al., 2017). Overall, the results demonstrated the ability of the lin-SB056-1/EDTA combination to retain its biofilm-inhibitory activity in the presence of the host cellular component that is known to markedly influence the effectiveness of both antibiotics and AMPs (Moreau-Marquis et al., 2008; Crabbé et al., 2017). Furthermore, the dendrimeric peptide (lin-SB056-1)2-K displayed an enhanced ability in inhibiting P. aeruginosa biofilm formation as compared to both lin-SB056-1 and the lin-SB056-1/EDTA combination, thereby underlining the importance of the rational peptide optimization to obtain valid templates to be developed as novel antibiofilm agents. Importantly, the present study highlighted the need of including physiologically relevant model systems in the preclinical testing of candidate antibiofilm peptides in order to assess their potential, but also their limitations.
Materials and Methods
AMPs and EDTA
Lin-SB056-1 (KWKIRVRLSA-NH2) and (lin-SB056-1)2-K ([KWKIRVRLSA]2-K) were synthetized by Peptide Protein Research (Fareham, England). Analysis of the synthetic peptides by high performance liquid chromatography and mass spectrometry revealed purity over 98%. Peptides were diluted in milli-Q water to obtain a stock solution of 2 mg/mL and stored at -80°C. Disodium EDTA was purchased from Sigma-Aldrich (St. Louis, MO, United States). A stock solution of EDTA (50 mM, pH 8) was prepared in milli-Q water, sterilized by filtration and stored at 4°C.
Bacterial Strains and Culture Conditions
The reference strain P. aeruginosa PAO1 (ATCC 15692) was used in this study as well as clinical isolates of P. aeruginosa (AA2 and AA44) from the lungs of patients with CF (Cullen et al., 2015). P. aeruginosa burn wound isolates (2091 and 2549) were obtained from Ghent University Hospital (Ghent, Belgium) (Zhang et al., 2018). For experiments involving fluorescence imaging of host-associated biofilms, the previously reported GFP-expressing strain of P. aeruginosa PAO1 was used (Crabbé et al., 2017). All strains were stored in a cryovial bead storage system (Microbank, Pro-Lab Diagnostics, Metropolitan Borough of Wirral, United Kingdom) at -80°C. For the preparation of the inoculum, beads from the frozen cultures were spread on TSA (LabM) and incubated overnight at 37°C. Liquid cultures were prepared starting from colonies isolated on agar plates. For all studies, P. aeruginosa PAO1, GFP-tagged P. aeruginosa PAO1 and P. aeruginosa clinical isolates from CF patients (AA2 and AA44) were aerobically cultured in LB at 37°C, 250 rpm. P. aeruginosa clinical isolates from wounds (2091 and 2549) were aerobically grown in TSB (LabM) at 37°C.
Determination of Minimal Inhibitory Concentration (MIC)
The susceptibility of P. aeruginosa PAO1 and CF clinical isolates to lin-SB056-1 and (lin-SB056-1)2-K was assessed in terms of MIC values according to the standard microdilution method (Clinical and Laboratory Standards Institute [CLSI], 2018). Bacteria were grown in Mueller-Hinton Broth (MHB, Oxoid, Basingstoke, United Kingdom) until exponential phase and diluted in the same medium to reach a final density of 1 × 107 CFU/mL. A volume of 10 μL of the bacterial suspensions was added to 90 μL of MHB in the absence (viability control) or in the presence of the peptides at different concentrations (1.2–77 μM). MIC values of both peptides were also determined in the culture medium used to grow 3-D lung epithelial cells (i.e., GTSF-2 medium, as described in Section “Biofilm Model Systems”). MIC values were defined as the lowest concentration of each peptide resulting in the complete inhibition of visible growth after 24 h of incubation at 37°C.
Bactericidal Activity in GTSF-2 Medium
Bactericidal activity of lin-SB056-1, used alone and in combination with EDTA, was evaluated against P. aeruginosa PAO1 in GTSF-2 medium, as described previously (Maisetta et al., 2017). Bacteria were grown in LB until exponential phase and diluted in GTSF-2 medium to reach a final density of 1 × 107 CFU/mL. A volume of 10 μL of the bacterial suspensions was added to 90 μL of GTSF-2 medium containing lin-SB056-1 (19.25 and 38.5 μM), EDTA (0.3–1.25 mM) and different peptide/EDTA combinations. Bacteria suspended in GTSF-2 medium were used as viability control. Samples were incubated statically for 1.5 h (37°C, 5% CO2), subsequently diluted 10-fold in a PSS (0.9% NaCl) and plated on TSA for the CFU count.
Biofilm Model Systems
A 3-D lung epithelial cell model and an artificial wound model were exploited to assess the biofilm-inhibitory activity of the AMPs under investigation. The 3-D lung model was generated by growing the human adenocarcinoma alveolar epithelial cell line A549 (ATCC CCL-185) on microcarrier beads in RWV bioreactors (Synthecon, Houston, TX, United States), as previously described (Carterson et al., 2005; Crabbé et al., 2017). 3-D cultures were generated in GTSF-2 medium (GE Healthcare, Diegem, Belgium) supplemented with 10% heat-inactivated FBS (Thermo Fisher Scientific, Waltham, MA, United States) and 1% penicillin/streptomycin (Thermo Fisher Scientific) and were used for infection studies after 11–14 days of culture in the RWV (37°C, 5% CO2). Immediately prior to use, 3-D A549 cells were washed once with HBSS and resuspended in GTSF-2 medium (without FBS and antibiotics) at a final density of 1 × 106 cells/mL.
The artificial wound model included a dermis-like scaffold and blood components at physiological concentrations. A two-layered artificial dermis composed of HA (Lifecore Biomedical, Chaska, MN, United States) and collagen (type-I collagen from rat tail) (Col; Sigma-Aldrich) with dimensions of approximately 1 cm3 was prepared as previously reported (Brackman et al., 2016). The upper layer of the artificial dermis consisted of chemically cross-linked high-molecular-weight HA (HMW-HA), while the lower layer was prepared from a mixed solution of HMW-HA, low-molecular-weight HA (LMW-HA) and heat-denatured Col. The biphasic spongy sheet was obtained by lyophilisation, subsequently irradiated with a UV lamp to induce cross-linking between Col molecules and finally sterilized at 110°C for 1 h. At the time of use, artificial dermis was immersed into a WLM consisting of BB (LabM) supplemented with 50% heparinized bovine plasma (Sigma-Aldrich) and 5% freeze-thaw laked horse blood (Biotrading, Mijdrecht, Netherlands) (Brackman et al., 2016).
Cytotoxicity in 3-D Lung Epithelial Cell Model
Cytotoxicity of the tested compounds toward 3-D A549 cells was assessed by measuring the enzymatic activity of cytosolic LDH released from dead cells. Upon maturation of the 3-D aggregates (11–14 days), a volume of 250 μL of the 3-D lung epithelial cells (containing 2.5 × 105 cells) was transferred into flat-bottom 48-well plates. Lin-SB056-1 (9.6–77 μM), used alone and in combination with EDTA (0.3–1.25 mM), and (lin-SB056-1)2-K (9.6–77 μM) were added to the 3-D cells and incubated statically for 4 h at 37°C, 5% CO2. 3-D aggregates incubated in GTSF-2 medium alone were used as negative (cell viability) control, while cells lysed with 1% Triton-X100 served as positive (death) control. Following incubation, cell culture medium was collected and centrifuged at 2500 × g for 15 min in order to remove cell debris. The amount of LDH in 3-D cell supernatants was quantified using a LDH activity assay kit (Sigma-Aldrich) following manufacturer’s instructions. The cytotoxic effect was determined according to the following formula: Cytotoxicity (%) = [(LDH activity tested compound – LDH activity negative control)/(LDH activity positive control – LDH activity negative control)] × 100.
Biofilm-Inhibitory Activity in 3-D Lung Epithelial Cell Model
The ability of lin-SB056-1, alone and in combination with EDTA, to inhibit biofilm formation in association with 3-D cells was evaluated against P. aeruginosa PAO1. Biofilm-inhibitory activity of (lin-SB056-1)2-K was assessed against both P. aeruginosa PAO1 and an isogenic pair of early and late clinical CF strains isolated from the same patient (AA2 and AA44) (Cullen et al., 2015). Bacteria were cultured overnight in LB (37°C, 250 rpm), suspended in GTSF-2 medium and added to 3-D A549 cells (2.5 × 105 cells/well) into 48-well plates at a multiplicity of infection (MOI) of 15. Biofilm formation was determined in the absence (negative control) or presence of different concentrations of lin-SB056-1 (9.6–77 μM), used alone and in combination with EDTA (0.3 and 0.6 mM), and (lin-SB056-1)2-K (9.6–77 μM). After 4 h of incubation (37°C, 5% CO2), host-associated biofilm formation was quantified through plating and CFU counting as described previously (Crabbé et al., 2017). To this end, 3-D cells were transferred into new 48-well plates and rinsed twice with HBSS (Thermo Fisher Scientific) in order to remove non-attached bacteria. To assess bacterial adhesion to 3-D cells, biofilms were exposed to 0.1% Triton X-100, disrupted by vigorous pipetting, serially diluted in PSS and plated on TSA.
Light and Fluorescence Microscopy
The effect of the tested compounds on morphology and integrity of 3-D A549 cells was determined through light microscopy using EVOS XL Core Imaging System (Life Technologies, Carlsbad, CA, United States). Qualitative evaluation of antibiofilm activity against GFP-tagged P. aeruginosa PAO1 in the 3-D lung epithelial cell model was performed by fluorescence microscopy using EVOS FL Auto Imaging System (Life Technologies). Light and fluorescence microscopy images were acquired with 20 and 40x objectives (total magnification of 599 and 1200x, respectively) and appropriate filter cubes. Imaging of 3-D aggregates was carried out following 4 h of incubation with the tested compounds both in the absence and presence of bacteria.
Hemolysis Assay
Hemolytic activity of lin-SB056-1 and (lin-SB056-1)2-K was tested against hRBCs as previously described (Grassi et al., 2017c). Briefly, a suspension of hRBCs (5%, v/v) was mixed with various concentrations of the peptides (1.2 to 77 μM) into round-bottom polystyrene 96-well microplates. hRBCs suspended in PBS alone were used as negative control (0% hemolysis), while cells lysed with 0.1% (v/v) Triton X-100 were taken as positive control (100% hemolysis). After 1 h of incubation (37°C, static), microplates were centrifuged at 1000 × g for 10 min, 4°C. Supernatants were transferred into new plates and optical density at 450 nm (OD450) was measured using a microplate reader. The hemolytic activity was quantified according to the following formula: Hemolysis (%) = [(OD peptide – OD negative control)/(OD positive control – OD negative control)] × 100.
Biofilm-Inhibitory Activity in Artificial Wound Model
The ability of (lin-SB056-1)2-K to inhibit biofilm formation in the artificial wound model was evaluated against P. aeruginosa PAO1 and two clinical isolates from wound infections. Bacteria were cultured overnight in TSB (37°C, static), washed and diluted in PSS to a final density of 1 × 106 CFU/mL. Artificial dermis was placed into flat-bottom 24-well plates and soaked with 500 μL of WLM. A volume of 10 μL of the bacterial suspension was spotted on the top of each artificial dermis. Following inoculation, (lin-SB056-1)2-K at a final concentration of 19.25 μM (100 μL) was added to the artificial dermis. Finally, additional WLM (400 μL) was added around the artificial dermis in order to avoid dehydration and plates were incubated at 37°C for 16 h. Based on preliminary experiments, such incubation time was found to ensure adequate P. aeruginosa biofilm formation without significant degradation of the artificial dermis by bacterial secreted factors. After the incubation, the artificial dermis was rinsed once in PSS to remove non-adherent cells and placed in glass tubes containing 10 mL of PSS. Biofilm-forming bacteria were detached from the artificial dermis through three cycles of vortexing (30 s) and sonication (30 s; Branson 3510, Branson Ultrasonics Corporation, Danbury, CT, United States), serially diluted in PSS and pour-plated on TSA to determine the number of CFU.
Statistical Analysis
All the experiments were performed at least in biological triplicate, unless otherwise specified. Statistical analysis was carried out using SPSS statistics software, version 25 (SPSS, Chicago, IL, United States). Normal distribution of the data was verified using the Shapiro–Wilk test. In the case of normally distributed data, differences between mean values were evaluated with the Student’s t-test for independent samples. Non-normally distributed data were analyzed using a Mann–Whitney test and Kruskal–Wallis test followed by Bonferroni post hoc test. In the case of combination studies, differences between the effect of the lin-SB056-1/EDTA combination and the sum of the effects of its individual components (i.e., lin-SB056-1 and EDTA) were analyzed using a Student’s t-test for independent samples. A p-value < 0.05 was considered statistically significant.
Results
Bactericidal Activity of lin-SB056-1 in Combination With EDTA in Host Cell Culture Medium
It is known that culture media can strongly affect the biological properties of AMPs (Dorschner et al., 2006; Ersoy et al., 2017). Therefore, the bactericidal activity of lin-SB056-1, alone and in combination with EDTA, was evaluated in the host cell culture medium (i.e., GTSF-2 medium) in order to identify the most promising peptide/EDTA combinations to be included in the subsequent antibiofilm activity studies. The antibacterial activity against planktonic P. aeruginosa PAO1 was assessed after 1.5 h of incubation taking into account the rapid killing kinetics of lin-SB056-1 (Maisetta et al., 2017). The peptide alone was able to reduce the number of viable bacteria by 3-Log units at a concentration of 38.5 μM (Figure 1A). In addition, a significantly increased bactericidal activity was observed when the peptide was used in combination with sub-inhibitory concentrations of EDTA (Figure 1B). In particular, the combination of lin-SB056-1 at 38.5 μM with EDTA (0.3 to 1.25 mM) resulted in the reduction of the initial bacterial inoculum to the limit of detection (10 CFU/mL) (Figure 1A). A statistically significant difference was also obtained by comparing the Log reduction of the lin-SB056-1/EDTA combination with the sum of the Log reductions caused by the individual compounds (data not shown).
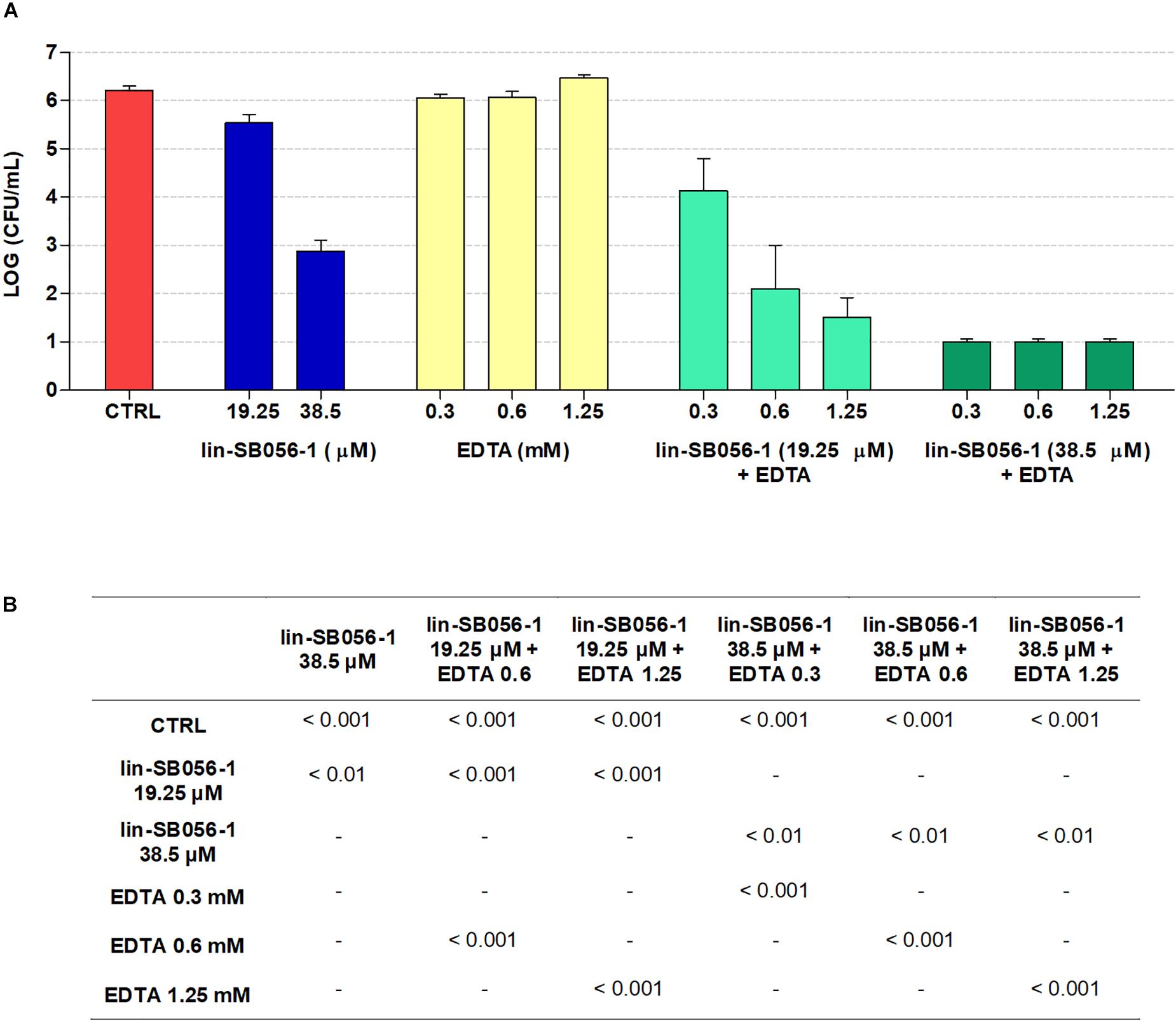
Figure 1. Bactericidal activity of lin-SB056-1, alone and in combination with EDTA, against planktonic cells of Pseudomonas aeruginosa PAO1 in GTSF-2 medium after 1.5 h of incubation. (A) Bactericidal activity of the lin-SB056-1/EDTA combinations was assessed by CFU count. Control (CTRL) represents untreated bacteria. A number of 10 CFU/mL was taken as detection limit. Data are reported as mean ± standard error of at least three independent experiments. (B) p-Values obtained by one-way ANOVA followed by Bonferroni post hoc test; only statistically significant comparisons are reported.
Cytotoxicity of lin-SB056-1 in Combination With EDTA in the 3-D Lung Epithelial Cell Model
The effect of lin-SB056-1, used alone and in combination with EDTA, on host cell viability was evaluated in the 3-D lung epithelial cell model. Lin-SB056-1 alone did not significantly affect host cell viability at any of the tested concentrations, as determined through the measurement of LDH release (Figure 2A). Similarly, no cytotoxic effect was observed when EDTA was used alone in the concentration range 0.3–1.25 mM. When used in combination with EDTA, the peptide exerted enhanced cytotoxicity toward 3-D cells although the fraction of dead cells was below 22% for all tested peptide/EDTA combinations, with the exception of the combination of 38.5 μM lin-SB056-1 and 1.25 mM EDTA (approximately 50% cell death) (Figure 2A). Furthermore, due to its chelating properties, the effect of EDTA on host cell adhesion was assessed through the microscopic observation of 3-D aggregates. Although EDTA was non-toxic when used alone (Figure 2A), at the concentration of 1.25 mM, it caused a morphological change of 3-D structures with the appearance of round-shaped cells and noticeable cell detachment from the scaffolds (Figure 2B). Nevertheless, morphology of 3-D cells treated with lower concentrations of EDTA (0.3 and 0.6 mM) was similar to the untreated control (Figure 2B), even when used in combination with the peptide (data not shown). Accordingly, subsequent biofilm studies were performed by selecting peptide/EDTA combinations able to reduce the number of planktonic bacteria by at least 3-Log units with as low as possible effect on cell viability (less than 22% cell death) and adhesion (i.e., lin-SB056-1 at 19.25 and 38.5 μM combined with EDTA at 0.3 and/or 0.6 mM).
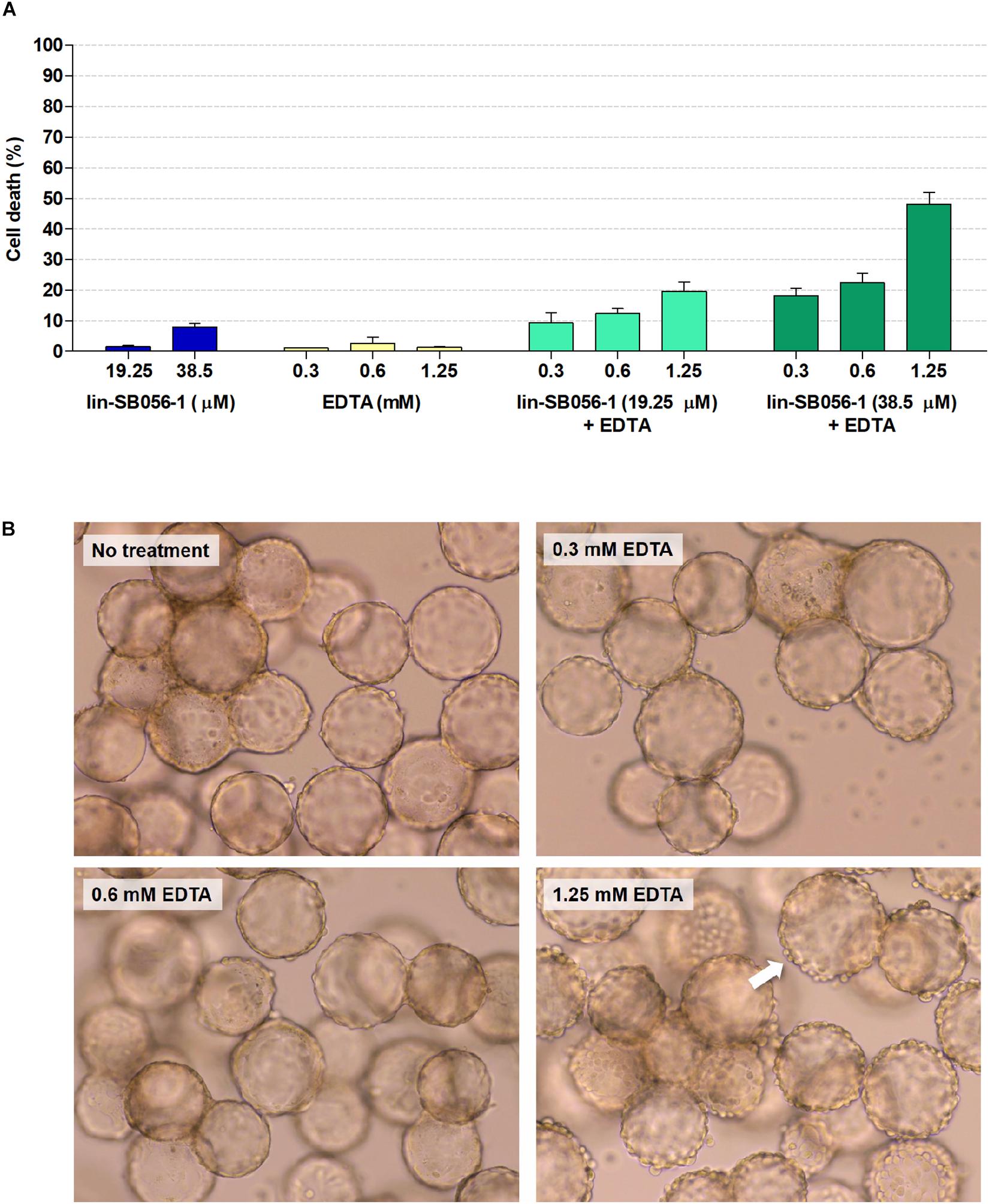
Figure 2. Cytotoxicity of lin-SB056-1 and EDTA, used alone and in combination, toward 3-D lung epithelial cells after 4 h of incubation. (A) Cell viability was evaluated by LDH-release assay. Control (CTRL) represents untreated 3-D cells. Data are reported as mean ± standard error of at least three independent experiments. (B) Effect of EDTA on cell adhesion to microcarrier bead scaffolds was evaluated by optical microscopy. Images were obtained at a magnification of 1200×. White arrow indicates round-shaped cells detaching from the surface of microcarrier beads.
Biofilm-Inhibitory Activity of lin-SB056-1 in Combination With EDTA in the 3-D Lung Epithelial Cell Model
The peptide/EDTA combinations selected according to bactericidal activity and cytotoxicity studies were tested for their ability to inhibit biofilm formation by P. aeruginosa PAO1 in the 3-D lung epithelial cell model after 4 h of incubation. Incubation time for the evaluation of the antibiofilm activity was selected taking into account that P. aeruginosa PAO1 is able to form microcolonies on the 3-D lung epithelium starting from 2 h of incubation (Crabbé et al., 2017). When assayed alone, lin-SB056-1 (38.5 μM) resulted in a decrease of approximately 0.5 Log-unit (3.3 fold) in the number of biofilm-forming bacteria compared to the untreated control (Figure 3). Among all the tested combinations, the most powerful biofilm-inhibitory effect in terms of viable count reduction (approximately 1 Log [10 fold] as compared to the control) was obtained by combining lin-SB056-1 at 38.5 μM with EDTA at 0.3 and 0.6 mM (Figure 3). At such concentrations, a statistically significant reduction in the CFU number was observed between the combination and the peptide or EDTA used alone (Figure 3). A statistically significant difference (p < 0.05, independent samples t-test) was also obtained by comparing the Log reduction of the lin-SB056-1/EDTA combination with the sum of the Log reductions caused by the individual compounds.
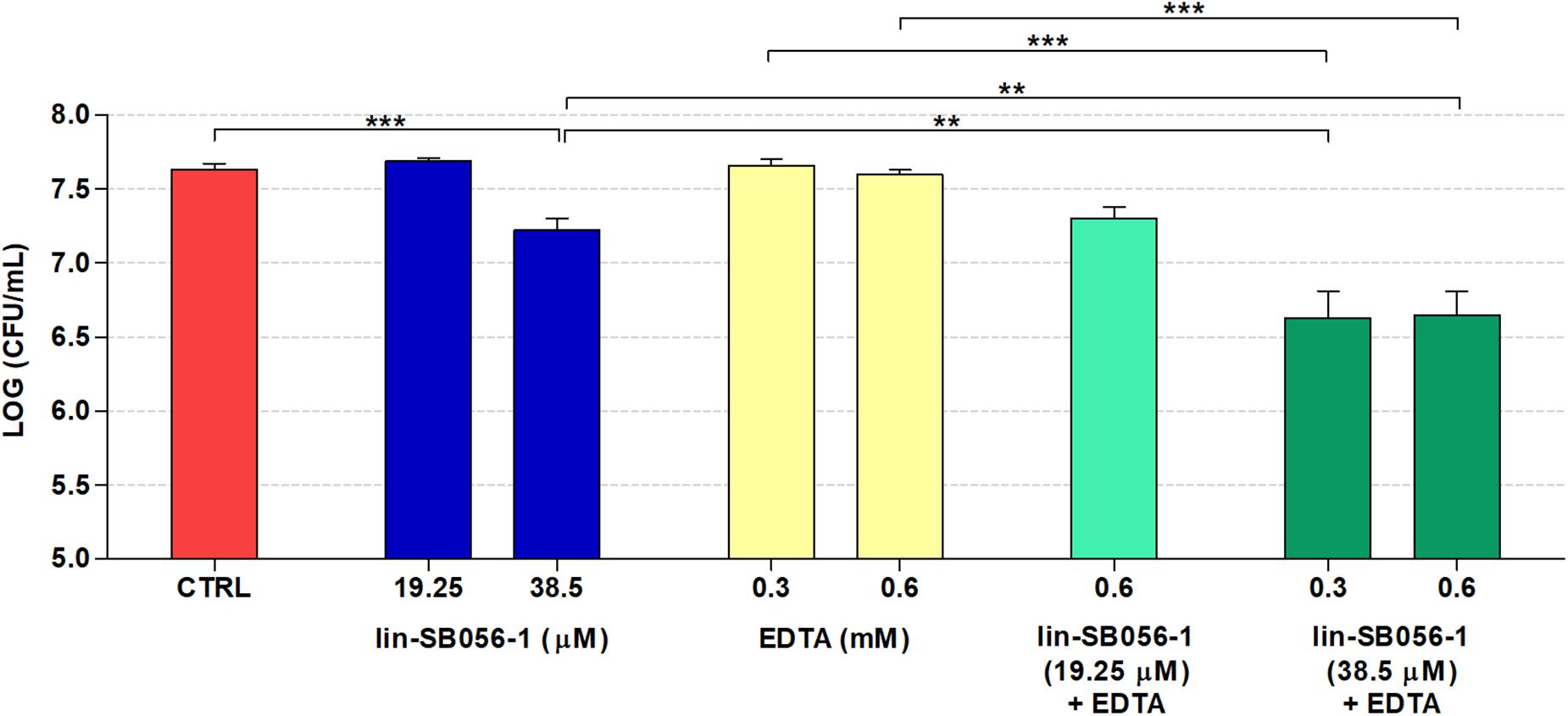
Figure 3. Biofilm-inhibitory activity of lin-SB056-1, alone and in combination with EDTA, against P. aeruginosa PAO1 in the 3-D lung epithelial cell model after 4 h of incubation. Control (CTRL) represents untreated bacteria. Data are reported as mean ± standard error of at least three independent experiments. ∗∗p < 0.01; ∗∗∗p < 0.001 (Kruskal–Wallis test followed by Bonferroni post hoc test).
Activity of (lin-SB056-1)2-K in the 3-D Lung Epithelial Cell Model
The biofilm-inhibitory activity and cytotoxicity of lin-SB056-1 were compared to those exerted by its dendrimeric derivative (lin-SB056-1)2-K in order to explore the possibility to obtain an improved antibiofilm effect in the 3-D lung epithelial cell model. The antibacterial activity of the two peptides was preliminarily compared in terms of MIC values, evaluated in MHB and GTSF-2 medium. Interestingly, although (lin-SB056-1)2-K was found to exhibit 2 to 4-fold higher MIC values in MHB than lin-SB056-1 against P. aeruginosa PAO1 and CF lung isolates (AA2 and AA44), it exerted an enhanced antibacterial activity in GTSF-2 medium (Table 1). Accordingly, (lin-SB056-1)2-K demonstrated a significantly improved ability to inhibit biofilm formation by P. aeruginosa PAO1 as compared to its linear counterpart in the 3-D lung epithelial cell model (Figure 4A). Although dendrimerization of the peptide was associated with an overall increase in cytotoxicity toward 3-D A549 cells (Figure 4B), (lin-SB056-1)2-K at a concentration causing less than 20% cell death (19.25 μM) (Figure 4B) reduced the number of biofilm-forming bacteria by 2-Log units (100 fold) (Figure 4A). Thus, when compared at the same cut-off of cytotoxicity (20% cell death) (Figure 4B), the dendrimeric form of the peptide (at 19.25 μM) caused an additional 1.5-Log unit reduction in the CFU count as compared to its linear counterpart (at 38.5 μM) (Figure 4A).
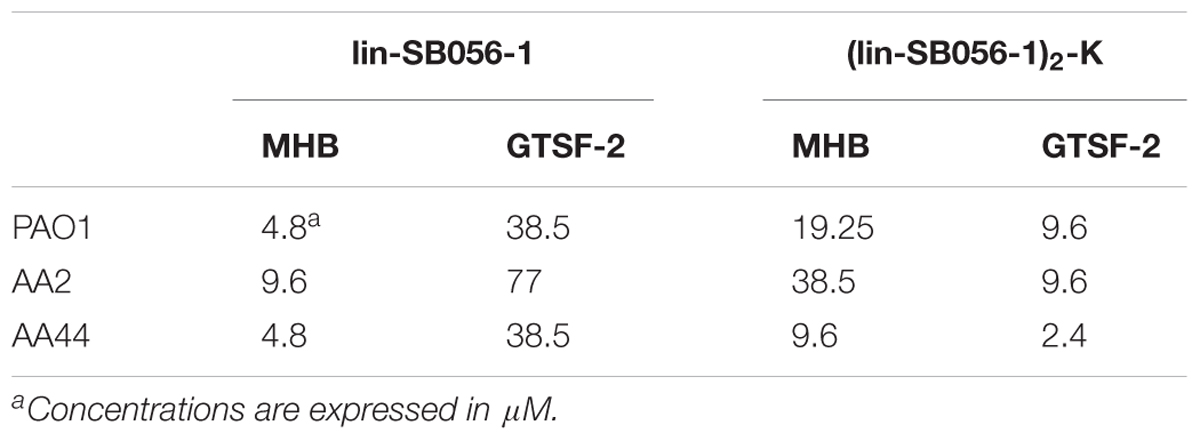
Table 1. Minimal inhibitory concentration (MIC) values of lin-SB056-1 and (lin-SB056-1)2-K against P. aeruginosa PAO1 and CF clinical isolates evaluated in MHB and GTSF-2 medium.
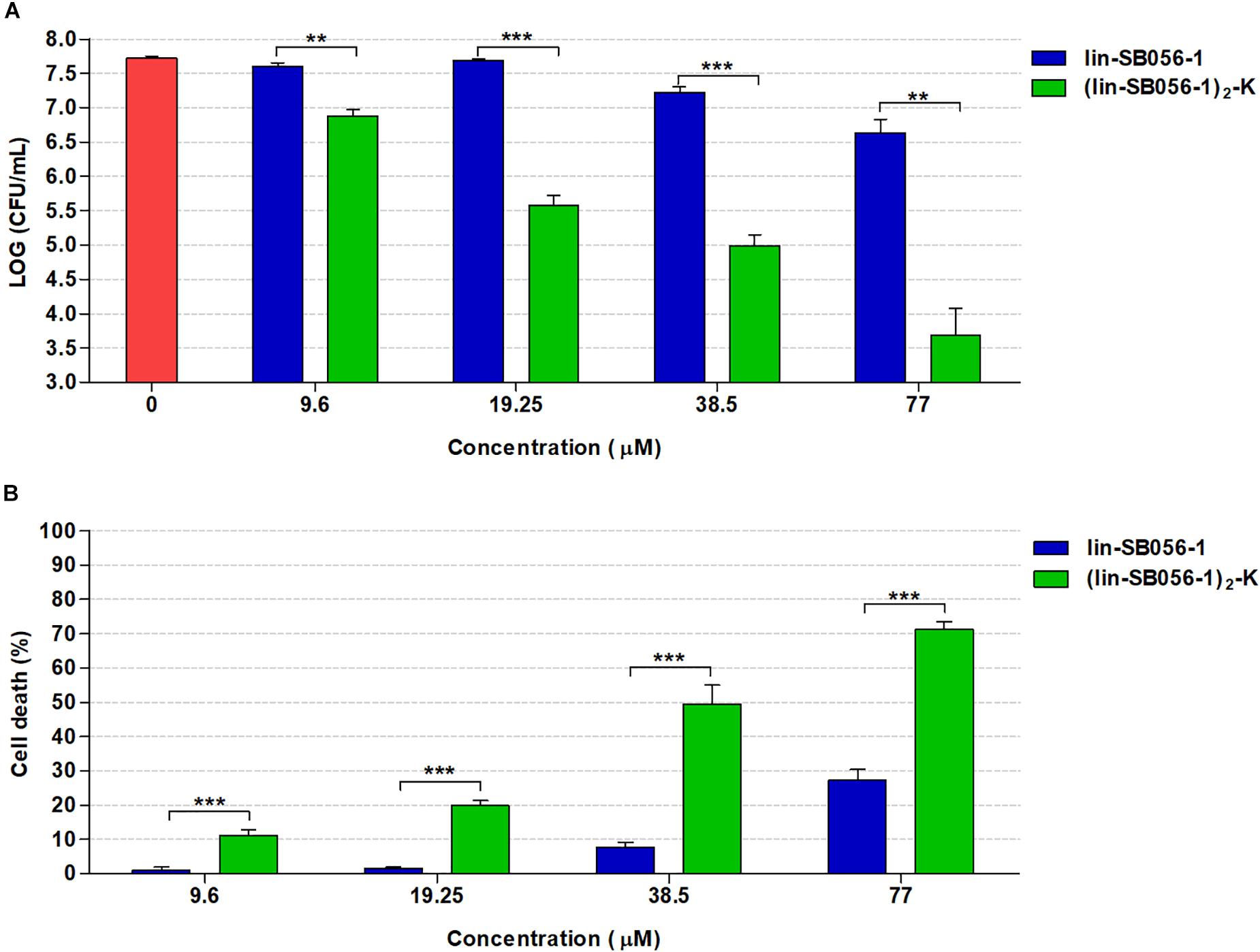
Figure 4. Comparison of the biofilm-inhibitory activity and cytotoxicity of lin-SB056-1 and (lin-SB056-1)2-K in the 3-D lung epithelial cell model after 4 h of incubation. (A) Biofilm-inhibitory activity against P. aeruginosa PAO1 was assessed by CFU count. Data are reported as mean ± standard error of at least three independent experiments. ∗∗p < 0.01; ∗∗∗p < 0.001 (Kruskal–Wallis test followed by Bonferroni post hoc test). (B) Viability of 3-D lung epithelial cells was evaluated by LDH-release assay. Data are reported as mean ± standard error of at least three independent experiments. ∗∗∗p < 0.001 (one-way ANOVA followed by Bonferroni post hoc test).
Higher levels of cytotoxicity of the dendrimeric peptide over the monomeric counterpart were also observed in a standard hemolysis assay. Nevertheless, at concentrations exerting significant antibiofilm activity (up to 19.25 μM), (lin-SB056-1)2-K caused less than 5% hemolysis (Supplementary Figure S1).
Importantly, (lin-SB056-1)2-K also exhibited a marked ability to inhibit biofilm formation by an early (AA2) and a late (AA44) clinical isolate of P. aeruginosa from a CF patient. In particular, at the concentration of 19.25 μM, the peptide reduced the number of CFU up to 3-Log units (1000 fold) as compared to the untreated control (Figure 5).
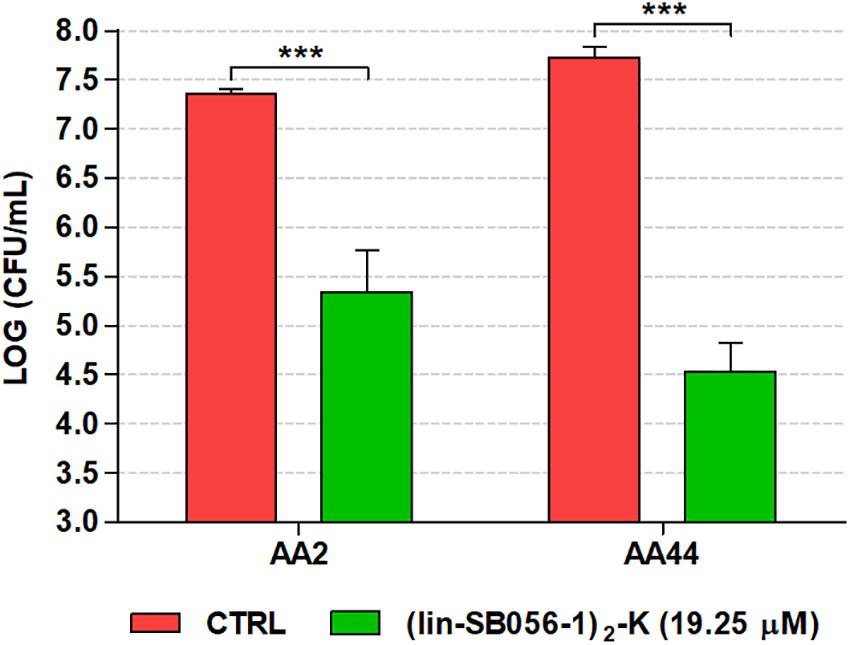
Figure 5. Biofilm-inhibitory activity of (lin-SB056-1)2-K (19.25 μM) against CF isolates of P. aeruginosa in the 3-D lung epithelial cell model after 4 h of incubation. Control (CTRL) represents untreated bacteria. Data are reported as mean ± standard error of at least three independent experiments. ∗∗∗p < 0.001 (independent samples t-test or Mann–Whitney test).
Qualitative assessment of the antibiofilm activity of (lin-SB056-1)2-K in the 3-D lung epithelial cell model was performed by monitoring the formation of fluorescent biofilm-like structures by GFP-producing P. aeruginosa PAO1. A remarkable decrease in the amount of biofilm-like structures associated with 3-D lung epithelial cells was observed after the treatment with (lin-SB056-1)2-K at 19.25 μM (Figure 6).
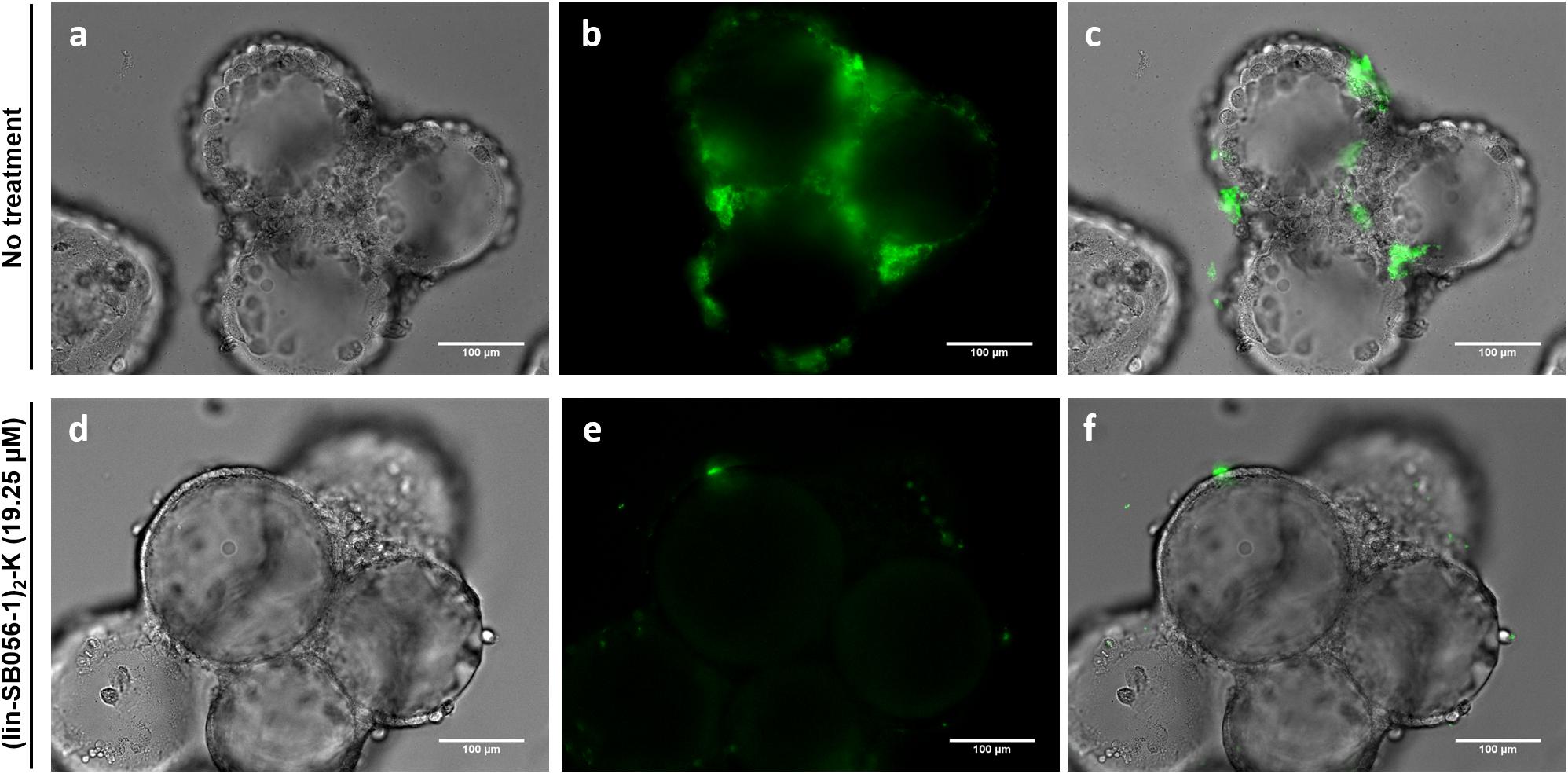
Figure 6. Qualitative evaluation of the biofilm-inhibitory activity of (lin-SB056-1)2-K (19.25 μM) against GFP-expressing P. aeruginosa PAO1 in the 3-D lung epithelial cell model after 4 h of incubation. Light microscopy (a,d), epifluorescence (b,e), and overlaid images (c,f) were obtained at a magnification of 599×. Scale bar corresponds to 100 μm.
Biofilm-Inhibitory Activity of (lin-SB056-1)2-K in Artificial Wound Model
The efficacy of (lin-SB056-1)2-K against forming biofilms of P. aeruginosa was also investigated in an in vitro chronic wound model consisting of a dermis-like scaffold embedded in physiological levels of blood components. At 19.25 μM, (lin-SB056-1)2-K displayed a significant biofilm-inhibitory activity against both P. aeruginosa PAO1 and the wound clinical isolate 2091, reducing the number of viable bacteria up to 1 Log-unit (10 fold) after 16 h of incubation (Figure 7). Although the peptide resulted in a reduction of approximately twofold in the CFU count of the clinical strain 2549, the difference did not reach statistical significance as compared to the control (p = 0.058, independent samples t-test).
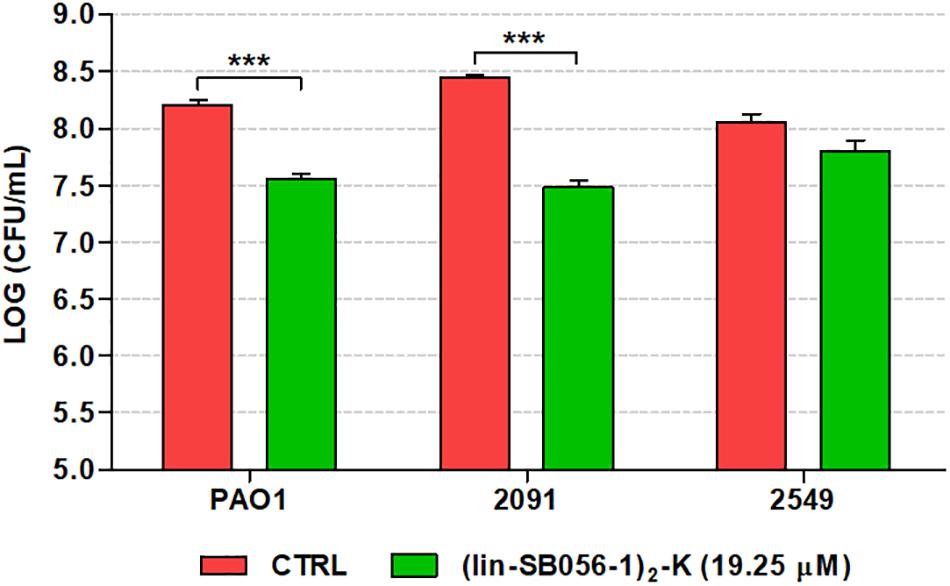
Figure 7. Biofilm-inhibitory activity of (lin-SB056-1)2-K (19.25 μM) against P. aeruginosa PAO1 and wound clinical isolates in the in vitro artificial wound model after 16 h of incubation. Control (CTRL) represents untreated bacteria. Data are reported as mean ± standard error of at least three independent experiments. ∗∗∗p < 0.001 (independent samples t-test).
Discussion
Over the last years, an increasing awareness of the role of biofilms in the establishment of chronic infections has led to a growing interest in the identification and development of novel antibiofilm strategies. High tolerance of biofilms to available antibiotics has stimulated interest in AMPs as potential antibiofilm agents (Batoni et al., 2011; Di Luca et al., 2015; de la Fuente-Núñez et al., 2016). Nevertheless, most of the studies assessing the antibiofilm activity of candidate AMPs have been performed using in vitro models in which the conditions are very different from those found in vivo at the site of infection (Lebeaux et al., 2013; Roberts et al., 2015). In particular, the electrostatic interaction of AMPs with bacterial membranes can be dramatically reduced by the presence of salts at physiological levels, proteases, macromolecules of the body fluids as well as host cells (Dorschner et al., 2006; Hancock and Sahl, 2006; Maisetta et al., 2008). Furthermore, many of these in vitro models rely on the formation of surface-attached biofilms characterized by a biomass order of magnitude larger than in vivo biofilms. Indeed, it has been reported that in vitro biofilms can reach dimensions of approximately 1 to 10 cm2 with a thickness up to 300 μm, whereas biofilm aggregates in chronic infections have been shown to mainly range from 5 to 200 μm in diameter (Bjarnsholt et al., 2013). In addition, different gene expression patterns and susceptibility to most antimicrobials have been reported for in vitro and in vivo-like biofilms (Caceres et al., 2014; Crabbé et al., 2017). For example, P. aeruginosa biofilms formed on CF bronchial epithelial cells have been found to exhibit enhanced tolerance to tobramycin as compared to biofilms grown on plastic surfaces (Anderson et al., 2008; Moreau-Marquis et al., 2008). Therefore, conclusions drawn from standard laboratory techniques may not be directly translated to human infections, and physiologically relevant biofilm model systems are desirable as a complementary tool for the evaluation of novel AMP-based antimicrobial agents (Pompilio et al., 2015; Bock et al., 2018; Dostert et al., 2018).
To address this issue, we exploited an in vivo-like 3-D lung epithelial cell model and an artificial wound model in order to assess the therapeutic potential of the semi-synthetic peptide lin-SB056-1 and its dendrimeric derivative (lin-SB056-1)2-K in the context of P. aeruginosa biofilm infections. Besides mimicking key aspects of the lung tissue, P. aeruginosa has been previously reported to form biofilms in the 3-D lung epithelial cell model (Crabbé et al., 2017), which is relevant for the phenotype of this opportunistic pathogen found during chronic lung infections (Alhede et al., 2011; Bjarnsholt et al., 2013; Ciofu et al., 2015). Additionally, the presence of host cells has been shown to markedly influence the ability of different antimicrobial agents to inhibit the formation of P. aeruginosa biofilms. Significant differences in the efficacy of conventional antibiotics (amikacin, colistin, gentamicin, and tobramycin) and the antibiofilm peptide DJK-5 have been observed between biofilms associated with 3-D lung epithelial cells and biofilms formed on plastic surfaces (Crabbé et al., 2017). Interestingly, the 3-D lung epithelial cell model offers the possibility to assess in parallel the antibiofilm activity and the cytotoxicity of antimicrobials toward cell types representative of the site of infection. In the case of AMPs, the tendency to employ hemolytic activity as preferred parameter for the high-throughput screening of cytotoxicity has markedly limited the accurate evaluation of their potential adverse effects. Indeed, the degree to which AMPs permeabilize human erythrocytes may not realistically reflect their selective toxicity against specific cell types in complex biomatrices in vivo (Yeaman and Yount, 2003; Bacalum and Radu, 2015). In addition to the lung environment, we aimed at recreating the physiological conditions encountered in chronic wounds by employing an artificial dermis embedded in a blood-based medium (Sun et al., 2008; Brackman et al., 2016). Despite the absence of a biotic surface, the artificial wound model closely reflects in vivo wound beds by resembling the nutritional conditions found in wound exudates and the dermal surface on which bacteria can adhere and form biofilm-like structures (Brackman et al., 2016; Trivedi et al., 2017).
In a previous study, we have demonstrated the effectiveness of the co-treatment with lin-SB056-1 and EDTA in preventing P. aeruginosa biofilms in an abiotic environment similar to the CF airway (Maisetta et al., 2017). Combination treatments represent a promising strategy to enhance the efficacy of AMPs against bacterial biofilms, especially under in vivo-like conditions that are known to interfere with peptide activity (Walkenhorst, 2016; Grassi et al., 2017b). In particular, the use of the chelating agent EDTA has been shown to improve the activity of different peptides due to its disaggregating effect on the biofilm extracellular matrix and its perturbing action on the outer membrane of Gram-negative bacteria (Banin et al., 2006; Grassi et al., 2017c). Furthermore, the ability of EDTA to decrease mucus viscosity has been hypothesized to promote the mobility and killing activity of lin-SB056-1 in the presence of artificial sputum (Gustafsson et al., 2012; Maisetta et al., 2017). In the present study, the synergistic interaction between lin-SB056-1 and EDTA has also been confirmed in the 3-D lung epithelial cell model. Indeed, lin-SB056-1 in combination with sub-inhibitory concentrations of EDTA significantly reduced the formation of P. aeruginosa biofilms in association with the airway epithelium.
In order to further improve the therapeutic potential of lin-SB056-1, we investigated the antibiofilm properties of the peptide’s dimeric derivative (lin-SB056-1)2-K in the same 3-D lung infection model. Interestingly, (lin-SB056-1)2-K was remarkably more effective than its monomeric counterpart in preventing the formation of P. aeruginosa biofilm-like structures in association with 3-D lung epithelial cells. Although in agreement with peptides’ activity observed in GTSF-2, these results contrast with MIC values obtained in MHB, where (lin-SB056-1)2-K consistently exhibited MIC values higher than lin-SB056-1 against both PAO1 strain and CF lung isolates of P. aeruginosa. This observation indicates that medium composition may greatly affect peptide activity, suggesting that the widely used MHB might not be an appropriate medium to screen the antibacterial potency of candidate AMPs (Dostert et al., 2018). The significant difference between the MIC values obtained in MHB and GTSF-2 could be due to differential peptide binding to specific medium components, such as complex carbohydrates (e.g., starch) that are present in MHB but not in GTSF-2, and/or to proteins (Lelkes et al., 1997). Different amounts of cations and carbonate in these media could also influence peptide activity in a manner that is difficult to forecast (Dorschner et al., 2006; Wu et al., 2008).
It has been suggested that the concentration at which a peptide exerts its antibiofilm activity is a parameter that could provide valuable information about its mechanism of action (Batoni et al., 2016a; Pletzer et al., 2016). Under the test conditions, both lin-SB056-1 and (lin-SB056-1)2-K inhibited adhesion/biofilm formation in association with host cells at concentrations equal and/or higher than their MIC values in GTSF-2 medium, thereby suggesting that they may act by a “classical” antibacterial effect rather than via a biofilm-specific mechanism of action.
As expected, along with the enhancement of the biofilm-inhibitory activity, dimerization of lin-SB056-1 led to an overall increase in the cytotoxicity of the original peptide against both 3-D lung epithelial cells and human erythrocytes. An increase in peptide cytotoxicity following modification is not surprising as it is widely accepted that there is a direct relationship between the antimicrobial and the cytotoxic properties of AMPs (Matsuzaki, 2009; Takahashi et al., 2010; Bolt et al., 2017). Nevertheless, in the 3-D lung epithelial cell model, a net percentage of cell death lower than 20% was observed at concentrations of (lin-SB056-1)2-K that significantly inhibited biofilm formation (up to 1000-fold reduction in the number of biofilm-forming bacteria) by both the reference strain PAO1 and two CF clinical isolates of P. aeruginosa. Interestingly, when evaluated at the same cut-off of cytotoxicity (20% cell death), (lin-SB056-1)2-K resulted in a superior antibiofilm activity also compared to lin-SB056-1 used in combination with EDTA, causing an additional reduction of 1 to 1.5-Log units in the CFU number as compared to the selected lin-SB056-1/EDTA combinations (i.e., 19.25 μM lin-SB056-1 in combination with 0.6 mM EDTA, and 38.5 μM lin-SB056-1 in combination with 0.3 mM EDTA).
Overall, our findings underline the superiority of the dendrimeric arrangement over the monomeric structure for the maintenance of the antimicrobial activity in complex and physiologically relevant environments. Interestingly, the biofilm-inhibitory activity of (lin-SB056-1)2-K was also maintained against different P. aeruginosa strains in the artificial wound model under very challenging in vivo-like conditions due to the presence of high concentrations of plasma/blood, which are known to impact the activity of peptides (Maisetta et al., 2008). It is commonly recognized that dendrimeric peptides exhibit enhanced antimicrobial activity as compared to their monomeric counterparts due to the higher local concentration of bioactive units and the reduced susceptibility to proteolytic degradation (Lind et al., 2015; Pires et al., 2015). In addition, it is likely that (lin-SB056-1)2-K is less influenced than lin-SB056-1 by the presence of physiological salt concentrations, as reported for the original dimeric structure from which it was derived (i.e., den-SB056-1) (Batoni et al., 2016a).
Although (lin-SB056-1)2-K at the active concentrations exhibited a certain degree of cytotoxicity against both erythrocytes and lung epithelial cells, different strategies could be exploited in the attempt to reduce peptide adverse effects against mammalian cells, while maintaining its antimicrobial properties. A number of different approaches have been described to control cell selectivity of AMPs, including modulation of a range of physicochemical parameters (e.g., hydrophobicity, helicity, and amphipathicity), design of pro-peptides, and insertion of D-amino acids and/or non-natural residues in the peptide primary sequence (Matsuzaki, 2009; Takahashi et al., 2010; Forde et al., 2016). Among these strategies, peptide entrapment in suitable nanocarriers able to deliver the active molecules to the infection site, while increasing their stability and minimizing side effects, appears one of the most promising approaches, and is currently under evaluation in our laboratory (Piras et al., 2015; Sandreschi et al., 2016).
Conclusion
In order to narrow the gap between in vitro and in vivo observations, research on AMPs should take into account the influence of the host environment on biofilm phenotype and peptide activity. Several studies have extensively questioned the usefulness of currently performed antibiotic susceptibility testing due to their limited clinical predictive value (Hurley et al., 2012; Hancock, 2015; Coenye et al., 2018). Analysis of antimicrobial activity in host-mimicking conditions represents a convenient approach to make a more realistic prediction of the therapeutic success of AMPs, thereby avoiding testing unpromising peptides in costly animal studies and clinical trials (Dorschner et al., 2006; Dostert et al., 2018). Although clinical development of novel drugs requires evidence of in vivo efficacy in animal models, preliminary in vitro evaluation in physiologically relevant biofilm model systems may facilitate the identification of effective AMP-based antimicrobials and reduce animal testing. For instance, the ability of (lin-SB056-1)2-K to preserve a significant antibiofilm activity in different challenging host-mimicking environments suggested that such peptide might be a promising template for the development of novel treatment strategies against P. aeruginosa infections.
Author Contributions
AC, GB, LG, and TC conceived, designed, and drafted the study. LG, LO, PR, and SVdB contributed to the acquisition, analyzed and interpreted the data. AC, AR, GB, GM, LG, LO, PR, SE, SVdB, and TC critically revised the study and gave the final approval.
Funding
This work was funded by an Odysseus grant of the Research Foundation Flanders (G.0.E53.14N) to AC, by a grant from the University of Pisa (PRA 2017_18) to GB and by the Ph.D. Program in Clinical and Translational Sciences of the University of Pisa. LG was supported by a scholarship from the University of Pisa (Ph.D. Program in Clinical and Translational Sciences).
Conflict of Interest Statement
The authors declare that the research was conducted in the absence of any commercial or financial relationships that could be construed as a potential conflict of interest.
Supplementary Material
The Supplementary Material for this article can be found online at: https://www.frontiersin.org/articles/10.3389/fmicb.2019.00198/full#supplementary-material
Abbreviations
AMP, antimicrobial peptide; BB, Bolton broth; CF, cystic fibrosis; CFUs, colony-forming units; Col, collagen; EDTA, ethylenediaminetetraacetic acid; FBS, fetal bovine serum; HA, hyaluronic acid; HBSS, Hanks’ balanced salt solution; hRBCs, human red blood cells; LB, Luria-Bertani broth; LDH, lactate dehydrogenase; MHB, Mueller-Hinton broth; MIC, minimal inhibitory concentration; PSS, physiological saline solution; RWV, rotating-wall vessel; TSA, tryptone soy agar; TSB, tryptone soy broth; WLM, wound-like medium.
Footnotes
References
Alhede, M., Nørskov Kragh, K., Qvortrup, K., Allesen-Holm, M., van Gennip, M., Christensen, L. D., et al. (2011). Phenotypes of non-attached Pseudomonas aeruginosa aggregates resemble surface attached biofilm. PLoS One 6:e27943. doi: 10.1371/journal.pone.0027943
Anderson, G. G., Moreau-Marquis, S., Stanton, B. A., and O’Toole, G. A. (2008). In vitro analysis of tobramycin-treated Pseudomonas aeruginosa biofilms on cystic fibrosis-derived airway epithelial cells. Infect. Immun. 76, 1423–1433. doi: 10.1128/IAI.01373-07
Bacalum, M., and Radu, M. (2015). Cationic antimicrobial peptides cytotoxicity on mammalian cells: an analysis using therapeutic index integrative concept. Int. J. Pept. Res. Ther. 21, 47–55. doi: 10.1007/s10989-014-9430-z
Banin, E., Brady, K. M., and Greenberg, E. P. (2006). Chelator-induced dispersal and killing of Pseudomonas aeruginosa cells in a biofilm. Appl. Environ. Microbiol. 72, 2064–2069. doi: 10.1128/AEM.72.3.2064-2069.2006
Barrila, J., Radtke, A. L., Crabbé, A., Sarker, S. F., Herbst-Kralovetz, M. M., Ott, C. M., et al. (2010). Organotypic 3D cell culture models: using the rotating wall vessel to study host-pathogen interactions. Nat. Rev. Microbiol. 8, 791–801. doi: 10.1038/nrmicro2423
Batoni, G., Casu, M., Giuliani, A., Luca, V., Maisetta, G., Mangoni, M. L., et al. (2016a). Rational modification of a dendrimeric peptide with antimicrobial activity: consequences on membrane-binding and biological properties. Amino Acids 48, 887–900. doi: 10.1007/s00726-015-2136-5
Batoni, G., Maisetta, G., Brancatisano, F. L., Esin, S., and Campa, M. (2011). Use of antimicrobial peptides against microbial biofilms: advantages and limits. Curr. Med. Chem. 18, 256–279. doi: 10.2174/092986711794088399
Batoni, G., Maisetta, G., and Esin, S. (2016b). Antimicrobial peptides and their interaction with biofilms of medically relevant bacteria. Biochim. Biophys. Acta 1858, 1044–1060. doi: 10.1016/j.bbamem.2015.10.013
Bjarnsholt, T., Alhede, M., Alhede, M., Eickhardt-Sorensen, S. R., Moser, C., Kuhl, M., et al. (2013). The in vivo biofilm. Trends Microbiol. 21, 466–474. doi: 10.1016/j.tim.2013.06.002
Bock, L. J., Hind, C. K., Sutton, J. M., and Wand, M. E. (2018). Growth media and assay plate material can impact on the effectiveness of cationic biocides and antibiotics against different bacterial species. Lett. Appl. Microbiol. 66, 368–377. doi: 10.1111/lam.12863
Bolt, H. L., Eggimann, G. A., Jahoda, C. A. B., Zuckermann, R. N., Sharples, G. J., and Cobb, S. L. (2017). Exploring the links between peptoid antibacterial activity and toxicity. MedChemComm 8, 886–896. doi: 10.1039/c6md00648e
Brackman, G., and Coenye, T. (2016). In vitro and in vivo biofilm wound models and their application. Adv. Exp. Med. Biol. 897, 15–32. doi: 10.1007/5584_2015_5002
Brackman, G., Garcia-Fernandez, M. J., Lenoir, J., De Meyer, L., Remon, J. P., De Beer, T., et al. (2016). Dressings loaded with cyclodextrin-hamamelitannin complexes increase Staphylococcus aureus susceptibility toward antibiotics both in single as well as in mixed biofilm communities. Macromol. Biosci. 16, 859–869. doi: 10.1002/mabi.201500437
Breidenstein, E. B., de la Fuente-Núñez, C., and Hancock, R. E. (2011). Pseudomonas aeruginosa: all roads lead to resistance. Trends Microbiol. 19, 419–426. doi: 10.1016/j.tim.2011.04.005
Caceres, S. M., Malcolm, K. C., Taylor-Cousar, J. L., Nichols, D. P., Saavedra, M. T., Bratton, D. L., et al. (2014). Enhanced in vitro formation and antibiotic resistance of non-attached Pseudomonas aeruginosa aggregates through incorporation of neutrophil products. Antimicrob. Agents Chemother. 58, 6851–6860. doi: 10.1128/AAC.0351414
Carterson, A. J., Höner zu Bentrup, K., Ott, C. M., Clarke, M. S., Pierson, D. L., Vanderburg, C. R., et al. (2005). A549 lung epithelial cells grown as three-dimensional aggregates: alternative tissue culture model for Pseudomonas aeruginosa pathogenesis. Infect. Immun. 73, 1129–1140. doi: 10.1128/IAI.73.2.1129-1140.2005
Ciofu, O., Tolker-Nielsen, T., Jensen, P. Ø, Wang, H., and Høiby, N. (2015). Antimicrobial resistance, respiratory tract infections and role of biofilms in lung infections in cystic fibrosis patients. Adv. Drug Deliv. Rev. 85, 7–23. doi: 10.1016/j.addr.2014.11.017
Clinical and Laboratory Standards Institute [CLSI]. (2018). Methods for Dilution Antimicrobial Susceptibility Tests for Bacteria that. (grow) Aerobically; Approved Standard–Tenth Edition. Wayne, PA: CLSI.
Coenye, T., Goeres, D., Van Bambeke, F., and Bjarnsholt, T. (2018). Should standardized susceptibility testing for microbial biofilms be introduced in clinical practice? Clin. Microbiol. Infect. 24, 570–572. doi: 10.1016/j.cmi.2018.01.003
Crabbé, A., Ledesma, M. A., and Nickerson, C. A. (2014). Mimicking the host and its microenvironment in vitro for studying mucosal infections by Pseudomonas aeruginosa. Pathog. Dis. 71, 1–19. doi: 10.1111/2049-632X.12180
Crabbé, A., Liu, Y., Matthijs, N., Rigole, P., De La Fuente-Nùñez, C., Davis, R., et al. (2017). Antimicrobial efficacy against Pseudomonas aeruginosa biofilm formation in a three-dimensional lung epithelial model and the influence of fetal bovine serum. Sci. Rep. 7:43321. doi: 10.1038/srep43321
Cullen, L., Weiser, R., Olszak, T., Maldonado, R. F., Moreira, A. S., Slachmuylders, L., et al. (2015). Phenotypic characterization of an international Pseudomonas aeruginosa reference panel: strains of cystic fibrosis (CF) origin show less in vivo virulence than non-CF strains. Microbiology 161, 1961–1977. doi: 10.1099/mic.0.000155
de Breij, A., Riool, M., Cordfunke, R. A., Malanovic, N., de Boer, L., Koning, R. I., et al. (2018). The antimicrobial peptide SAAP-148 combats drug-resistant bacteria and biofilms. Sci. Transl. Med. 10:eaan4044. doi: 10.1126/scitranslmed.aan4044
de la Fuente-Núñez, C., Cardoso, M. H., de Souza Cândido, E., Franco, O. L., and Hancock, R. E. (2016). Synthetic antibiofilm peptides. Biochim. Biophys. Acta 1858, 1061–1069. doi: 10.1016/j.bbamem.2015.12.015
Di Luca, M., Maccari, G., Maisetta, G., and Batoni, G. (2015). BaAMPs: the database of biofilm-active antimicrobial peptides. Biofouling 31, 193–199. doi: 10.1080/08927014.2015.1021340
Dorschner, R. A., Lopez-Garcia, B., Peschel, A., Kraus, D., Morikawa, K., Nizet, V., et al. (2006). The mammalian ionic environment dictates microbial susceptibility to antimicrobial defense peptides. FASEB J. 20, 35–42. doi: 10.1096/fj.05-4406com
Dostert, M., Belanger, C. R., and Hancock, R. E. W. (2018). Design and assessment of anti-biofilm peptides: steps toward clinical application. J. Innate Immun. 22, 1–12. doi: 10.1159/000491497
Ersoy, S. C., Heithoff, D. M., Barnes, L., Tripp, G. K., House, J. K., Marth, J. D., et al. (2017). Correcting a fundamental flaw in the paradigm for antimicrobial susceptibility testing. eBioMedicine 20, 173–181. doi: 10.1016/j.ebiom.2017.05.026
Forde,É., Schütte, A., Reeves, E., Greene, C., Humphreys, H., Mall, M., et al. (2016). Differential in vitro and in vivo toxicities of antimicrobial peptide prodrugs for potential use in cystic fibrosis. Antimicrob. Agents Chemother. 60, 2813–2821. doi: 10.1128/AAC.0015716
Gellatly, S. L., and Hancock, R. E. (2013). Pseudomonas aeruginosa: new insights into pathogenesis and host defenses. Pathog. Dis. 67, 159–173. doi: 10.1111/2049-632X.12033
Grassi, L., Di Luca, M., Maisetta, G., Rinaldi, A. C., Esin, S., Trampuz, A., et al. (2017a). Generation of persister cells of Pseudomonas aeruginosa and Staphylococcus aureus by chemical treatment and evaluation of their susceptibility to membrane-targeting agents. Front. Microbiol. 8:1917. doi: 10.3389/fmicb.2017.01917
Grassi, L., Maisetta, G., Esin, S., and Batoni, G. (2017b). Combination strategies to enhance the efficacy of antimicrobial peptides against bacterial biofilms. Front. Microbiol. 8:2409. doi: 10.3389/fmicb.2017.02409
Grassi, L., Maisetta, G., Maccari, G., Esin, S., and Batoni, G. (2017c). Analogs of the frog-skin antimicrobial peptide temporin 1Tb exhibit a wider spectrum of activity and a stronger antibiofilm potential as compared to the parental peptide. Front. Chem. 5:24. doi: 10.3389/fchem.2017.00024
Gustafsson, J. K., Ermund, A., Ambort, D., Johansson, M. E., Nilsson, H. E., Thorell, K., et al. (2012). Bicarbonate and functional CFTR channel are required for proper mucin secretion and link cystic fibrosis with its mucus phenotype. J. Exp. Med. 209, 1263–1272. doi: 10.1084/jem.20120562
Hancock, R. E. W. (2015). Rethinking the antibiotic discovery paradigm. eBioMedicine 2, 629–630. doi: 10.1016/j.ebiom.2015.07.010
Hancock, R. E. W., and Sahl, H. G. (2006). Antimicrobial and host-defense peptides as new anti-infective therapeutic strategies. Nat. Biotechnol. 24, 1551–1557. doi: 10.1038/nbt1267
Hurley, M. N., Ariff, A. H., Bertenshaw, C., Bhatt, J., and Smyth, A. R. (2012). Results of antibiotic susceptibility testing do not influence clinical outcome in children with cystic fibrosis. J. Cyst. Fibros. 11, 288–292. doi: 10.1016/j.jcf.2012.02.006
Lashua, L. P., Melvin, J. A., Deslouches, B., Pilewski, J. M., Montelaro, R. C., and Bomberger, J. M. (2016). Engineered cationic antimicrobial peptide (eCAP) prevents Pseudomonas aeruginosa biofilm growth on airway epithelial cells. J. Antimicrob. Chemother. 8, 2200–2207. doi: 10.1093/jac/dkw143
Lebeaux, D., Chauhan, A., Rendueles, O., and Beloin, C. (2013). From in vitro to in vivo models of bacterial biofilm-related infections. Pathogens 2, 288–356. doi: 10.3390/pathogens2020288
Lelkes, P. I., Ramos, E., Nikolaychik, V. V., Wankowski, D. M., Unsworth, B. R., and Goodwin, T. J. (1997). GTSF-2: a new, versatile cell culture medium for diverse normal and transformed mammalian cells. In Vitro Cell. Dev. Biol. Anim. 33, 344–351. doi: 10.1007/s11626-997-0004-7
Lind, T. K., Polcyn, P., Zielinska, P., Cárdenas, M., and Urbanczyk-Lipkowska, Z. (2015). On the antimicrobial activity of various peptide-based dendrimers of similar architecture. Molecules 20, 738–753. doi: 10.3390/molecules20010738
Maisetta, G., Di Luca, M., Esin, S., Florio, W., Brancatisano, F. L., Bottai, D., et al. (2008). Evaluation of the inhibitory effects of human serum components on bactericidal activity of human beta defensin 3. Peptides 29, 1–6. doi: 10.1016/j.peptides.2007.10.013
Maisetta, G., Grassi, L., Esin, S., Serra, I., Scorciapino, M. A., Rinaldi, A. C., et al. (2017). The semi-synthetic peptide lin-SB056-1 in combination with EDTA exerts strong antimicrobial and antibiofilm activity against Pseudomonas aeruginosa in conditions mimicking cystic fibrosis sputum. Int. J. Mol. Sci. 18:1994. doi: 10.3390/ijms18091994
Manzo, G., Scorciapino, M. A., Wadhwani, P., Bürck, J., Montaldo, N. P., Pintus, M., et al. (2015). Enhanced amphiphilic profile of a short β-stranded peptide improves its antimicrobial activity. PLoS One 10:e0116379. doi: 10.1371/journal.pone.0116379
Manzo, G., Serra, I., Pira, A., Pintus, M., Ceccarelli, M., Casu, M., et al. (2016). The singular behavior of a β-type semi-synthetic two branched polypeptide: three-dimensional structure and mode of action. Phys. Chem. Chem. Phys. 18, 30998–31011. doi: 10.1039/c6cp05464a
Mardirossian, M., Pompilio, A., Degasperi, M., Runti, G., Pacor, S., Di Bonaventura, G., et al. (2017). D-BMAP18 antimicrobial peptide is active in vitro, resists to pulmonary proteases but loses its activity in a murine model of Pseudomonas aeruginosa lung infection. Front. Chem. 5:40. doi: 10.3389/fchem.2017.00040
Matsuzaki, K. (2009). Control of cell selectivity of antimicrobial peptides. Biochim. Biophys. Acta 1788, 1687–1692. doi: 10.1016/j.bbamem.2008.09.013
Moreau-Marquis, S., Bomberger, J. M., Anderson, G. G., Swiatecka-Urban, A., Ye, S., O’Toole, G. A., et al. (2008). The DeltaF508-CFTR mutation results in increased biofilm formation by Pseudomonas aeruginosa by increasing iron availability. Am. J. Physiol. Lung Cell. Mol. Physiol. 295, L25–L37. doi: 10.1152/ajplung.00391.2007
Mulcahy, L. R., Isabella, V. M., and Lewis, K. (2014). Pseudomonas aeruginosa biofilms in disease. Microb. Ecol. 68, 1–12. doi: 10.1007/s00248-013-0297-x
Parsek, M. R., and Singh, P. K. (2003). Bacterial biofilms: an emerging link to disease pathogenesis. Annu. Rev. Microbiol. 57, 677–701. doi: 10.1146/annurev.micro.57.030502.090720
Piras, A. M., Maisetta, G., Sandreschi, S., Gazzarri, M., Bartoli, C., Grassi, L., et al. (2015). Chitosan nanoparticles loaded with the antimicrobial peptide temporin B exert a long-term antibacterial activity in vitro against clinical isolates of Staphylococcus epidermidis. Front. Microbiol. 6:372. doi: 10.3389/fmicb.2015.00372
Pires, J., Siriwardena, T. N., Stach, M., Tinguely, R., Kasraian, S., Luzzaro, F., et al. (2015). In vitro activity of the novel antimicrobial peptide dendrimer G3KL against multidrug-resistant Acinetobacter baumannii and Pseudomonas aeruginosa. Antimicrob. Agents Chemother. 12, 7915–7918. doi: 10.1128/AAC.01853-15
Pletzer, D., Coleman, S. R., and Hancock, R. E. (2016). Anti-biofilm peptides as a new weapon in antimicrobial warfare. Curr. Opin. Microbiol. 33, 35–40. doi: 10.1016/j.mib.2016.05.016
Polcyn, P., Zielinska, P., Zimnicka, M., Troć, A., Kalicki, P., Solecka, J., et al. (2013). Novel antimicrobial peptide dendrimers with amphiphilic surface and their interactions with phospholipids - insights from mass spectrometry. Molecules 18, 7120–7144. doi: 10.3390/molecules18067120
Pompilio, A., Crocetta, V., Pomponio, S., Fiscarelli, E., and Di Bonaventura, G. (2015). In vitro activity of colistin against biofilm by Pseudomonas aeruginosa is significantly improved under “cystic fibrosis-like” physicochemical conditions. Diagn. Microbiol. Infect. Dis. 82, 318–325. doi: 10.1016/j.diagmicrobio.2015.01.006
Roberts, A. E., Kragh, K. N., Bjarnsholt, T., and Diggle, S. P. (2015). The limitations of in vitro experimentation in understanding biofilms and chronic infection. J. Mol. Biol. 427, 3646–3661. doi: 10.1016/j.jmb.2015.09.002
Rybtke, M., Hultqvist, L. D., Givskov, M., and Tolker-Nielsen, T. (2015). Pseudomonas aeruginosa biofilm infections: community structure, antimicrobial tolerance and immune response. J. Mol. Biol. 427, 3628–3645. doi: 10.1016/j.jmb.2015.08.016
Sandreschi, S., Piras, A. M., Batoni, G., and Chiellini, F. (2016). Perspectives on polymeric nanostructures for the therapeutic application of antimicrobial peptides. Nanomedicine 11, 1729–1744. doi: 10.2217/nnm-2016-0057
Sun, Y., Dowd, S. E., Smith, E., Rhoads, D. D., and Wolcott, R. D. (2008). In vitro multispecies lubbock chronic wound biofilm model. Wound Repair. Regen. 16, 805–813. doi: 10.1111/j.1524-475X.2008.00434.x
Takahashi, D., Shukla, S. K., Prakash, O., and Zhang, G. (2010). Structural determinants of host defense peptides for antimicrobial activity and target cell selectivity. Biochimie 92, 1236–1241. doi: 10.1016/j.biochi.2010.02.023
Taylor, P. K., Yeung, A. T., and Hancock, R. E. (2014). Antibiotic resistance in Pseudomonas aeruginosa biofilms: towards the development of novel anti-biofilm therapies. J. Biotechnol. 191, 121–130. doi: 10.1016/j.jbiotec.2014.09.003
Trivedi, U., Madsen, J. S., Rumbaugh, K. P., Wolcott, R. D., Burmølle, M., and Sørensen, S. J. (2017). A post-planktonic era of in vitro infectious models: issues and changes addressed by a clinically relevant wound like media. Crit. Rev. Microbiol. 43, 453–465. doi: 10.1080/1040841X.2016.1252312
Walkenhorst, W. F. (2016). Using adjuvants and environmental factors to modulate the activity of antimicrobial peptides. Biochim. Biophys. Acta 1858, 926–935. doi: 10.1016/j.bbamem.2015.12.034
Wu, G., Ding, J., Li, H., Li, L., Zhao, R., Shen, Z., et al. (2008) Effects of cations and pH on antimicrobial activity of thanatin and s-thanatin against Escherichia coli ATCC25922 and B. subtilis ATCC 21332. Curr. Microbiol. 57, 552–557. doi: 10.1007/s00284-008-9241-6
Yeaman, M. R., and Yount, N. Y. (2003). Mechanisms of antimicrobial peptide action and resistance. Pharmacol. Rev. 55, 27–55. doi: 10.1124/pr.55.1.2
Keywords: antimicrobial peptides, dendrimeric peptide, combination treatment, biofilm, Pseudomonas aeruginosa, 3-D lung epithelial cell model, artificial wound model
Citation: Grassi L, Batoni G, Ostyn L, Rigole P, Van den Bossche S, Rinaldi AC, Maisetta G, Esin S, Coenye T and Crabbé A (2019) The Antimicrobial Peptide lin-SB056-1 and Its Dendrimeric Derivative Prevent Pseudomonas aeruginosa Biofilm Formation in Physiologically Relevant Models of Chronic Infections. Front. Microbiol. 10:198. doi: 10.3389/fmicb.2019.00198
Received: 12 November 2018; Accepted: 24 January 2019;
Published: 08 February 2019.
Edited by:
Fabian Cieplik, Universitätsklinikum Regensburg, GermanyReviewed by:
Bjoern O. Schroeder, University of Gothenburg, SwedenDaniel Pletzer, The University of British Columbia, Canada
César de la Fuente, Massachusetts Institute of Technology, United States
Copyright © 2019 Grassi, Batoni, Ostyn, Rigole, Van den Bossche, Rinaldi, Maisetta, Esin, Coenye and Crabbé. This is an open-access article distributed under the terms of the Creative Commons Attribution License (CC BY). The use, distribution or reproduction in other forums is permitted, provided the original author(s) and the copyright owner(s) are credited and that the original publication in this journal is cited, in accordance with accepted academic practice. No use, distribution or reproduction is permitted which does not comply with these terms.
*Correspondence: Lucia Grassi, bHVjaWEuZ3Jhc3NpQG1lZC51bmlwaS5pdA==