- 1Laboratorio Nazionale di Riferimento per il Controllo Delle Contaminazioni Batteriche dei Molluschi Bivalvi, Istituto Zooprofilattico Sperimentale Dell’Umbria e Delle Marche “Togo Rosati”, Ancona, Italy
- 2Istituto Zooprofilattico Sperimentale Dell’Umbria e Delle Marche “Togo Rosati”, Perugia, Italy
- 3National Reference Centre for Whole Genome Sequencing of Microbial Pathogens: Data-Base and Bioinformatics Analysis (GENPAT), Istituto Zooprofilattico Sperimentale Dell’Abruzzo e del Molise “G. Caporale”, Teramo, Italy
- 4Centro di Referenza per il Controllo Microbiologico e Chimico dei Molluschi Bivalvi Vivi, Istituto Zooprofilattico Sperimentale Dell’Umbria e Delle Marche “Togo Rosati”, Ancona, Italy
The spread of extended-spectrum β-lactamase (ESBL)-producing Escherichia coli is a major public health issue. Bivalves are filter-feeder animals capable of bioaccumulating the microorganisms present in water. This physiological characteristic makes them both good indicators of environmental contamination and possible carriers of pathogenic bacteria, including those resistant to antimicrobials. The aim of this study was to investigate the occurrence of ESBL-producing E. coli in clams (n = 308) collected from harvesting areas of the Central Adriatic Sea between 2018 and 2019. ESBL- /class C β-lactamase (AmpC)- producing E. coli and Escherichia spp. were isolated by streaking over the surface of MacConkey agar plates supplemented with cefotaxime enriched broths of the initial shellfish suspension. E. coli and Escherichia spp. resistant to cefotaxime were screened for ESBL production by using the double disk synergy test. Susceptibility to different antimicrobials and confirmation of ESBL-production were determined by the minimum inhibitory concentration (MIC) test. Isolates were further characterized by whole genome sequencing (WGS) and bioinformatic analysis of genomes with different tools. Overall, ESBL-producing E. coli were isolated from 3% of the samples. Of 13 ESBL- and ESBL−/AmpC-producing Escherichia spp. (n = 11 E. coli, n = 1 E. marmotae, n = 1 E. ruysiae) isolates, 13 were resistant to ampicillin and cefotaxime, 9 to sulfamethoxazole, 6 to tetracycline and nalidixic acid, 4 to trimethoprim, and 3 to ceftazidime, cefoxitin, ciprofloxacin, and chloramphenicol. Moreover, the majority (8/11) of the ESBL-producing E. coli isolates were multidrug-resistant. WGS showed that the isolates predominantly carried the blaCTX-M-15 gene (3/11) and blaCTX-M-14 and blaCTX-M-1 (2/11 each). The AmpC β-lactamase CMY-2 was found in two isolates. Phylogroup A was the most prevalent (5/11), followed by phylogroups D (4/11), F (1/11), and B2 (1/11). Ten different sequence types (STs) were identified. Occurrence at sampling sites ranged between 0 and 27%. To identify associations between the occurrence of ESBL-producing E. coli and E. coli levels, samples were divided into two groups, with E. coli at >230 MPN/100 g and E. coli at ≤230 MPN/100 g. ESBL-producing E. coli isolates were significantly more commonly recovered in samples with higher E. coli levels (14%) than in those with lower levels of E. coli (2%). Moreover, the majority (3/4) of the potentially pathogenic strains were isolated in samples with higher E. coli levels. These findings provided evidence for the bacterial indicator of fecal contamination, E. coli, as an index organism for ESBL-producing E. coli isolates in bivalves.
Introduction
Antimicrobial resistance (AMR) is one of the most significant public health threats (World Health Organization, 2021), responsible for hundreds of thousands of estimated deaths annually worldwide (O’Neill, 2016).
Third/fourth/fifth-generation cephalosporins are the highest priority critically important antimicrobials (HPCIAs) in human medicine (World Health Organization, 2019), and Enterobacteriaceae producers of extended-spectrum β-lactamases (ESBLs) are on the critical-priority WHO list of antibiotic-resistant bacteria for research and development of new antibiotics (Tacconelli et al., 2018). An 8-fold increase in the intestinal carriage rate of ESBL Escherichia coli in the community has occurred globally over the past two decades (Bezabih et al., 2021), and the global and regional human intestinal carriage of ESBL E. coli is increasing in both community and healthcare settings (Bezabih et al., 2022). The spread of ESBL poses a serious threat to public health; therefore, it is important to investigate sources and transmission routes and encourage studies contributing to the “One-Health” approach.
Human- and animal-gut bacteria, including those resistant to antimicrobials, can reach marine environments through various routes (e.g., runoff from land, sewage systems, and feces from birds and wild animals), with the potential to contaminate seafood products. Bivalves are invertebrate filter-feeder animals capable of bioaccumulating microorganisms present in the surrounding waters. Thus, they are good indicators of environmental contamination and may act as possible carriers of bacteria derived from fecal pollution (Lee and Silk, 2013), including those that are resistant to antimicrobials (Albini et al., 2022).
In the European Union, regulation concerning the sanitary safety of live bivalve mollusks (Anonymous, 2004, 2019) stipulates that classified production areas shall be periodically monitored to check the microbiological quality of shellfish by using the bacteriological indicator of fecal contamination, E. coli. The latter is a genetically diverse species that comprises non-pathogenic gut commensals and strains responsible for intestinal and extra-intestinal disease. Enterotoxigenic E. coli (ETEC) strains are able to bind and colonize the intestinal epithelium and also produce various enterotoxins, of which heat-labile and heat-stable toxins and/or enteroaggregative heat-stable toxin 1 (EAST1) lead to diarrhea. Extra-intestinal pathogenic E. coli (ExPEC) are non-commensal E. coli isolates capable of causing extra-intestinal disease due to the possession of pathogenic virulence factors (Russo and Johnson, 2000). Escherichia coli isolates containing at least two genes coding virulence factors (papA and/or papC, sfa/foc, afa/draBC, kpsM II, and iutA) are defined as ExPEC (Peirano et al., 2013).
Antimicrobial resistance monitoring programs in the EU are focused on terrestrial animals (Aerts et al., 2019). Studies on the occurrence of ESBL-producing E. coli in bivalves are limited. The prevalence of ESBL-producing E. coli has been investigated in retail bivalves in studies conducted in European or North African countries (Boss et al., 2016; Vu et al., 2018; Sola et al., 2022). In European studies, ESBL- or AmpC-producing E. coli isolates were not recovered in retail oysters sampled in Switzerland (Boss et al., 2016), and ESBL-producing Enterobacteriaceae were isolated in 20% of bivalve samples collected at retail in Berlin (Vu et al., 2018). In another study conducted in Tunisia, bivalves were sampled in unrelated markets in different regions, and ESBL-producing Enterobacterales (mostly E. coli) were cultured from 1.6% of clam pools (Sola et al., 2022). Other studies investigated the occurrence of ESBL-producing E. coli isolates in bivalves from production areas (Rees et al., 2015; Bueris et al., 2022). Hence, there are relatively few studies assessing the occurrence of ESBL-producing E. coli in bivalves from production areas over different seasons, and none of them were carried out in Italy. Furthermore, to our knowledge, previous studies have not investigated the relationship between levels of the bacterial indicator of fecal contamination, E. coli, and ESBL-producing E. coli presence in bivalve mollusks.
Clam is a major commercial species in Italy, and among EU countries, Italy is the main producer, accounting for 77% of farmed clams in the EU, reaching 24,452 tons in 2020 (European Commission, 2022). As clams are grown in coastal waters, they can also represent a sentinel species in determining AMR in the marine environment.
The aim of this study was to investigate the occurrence of ESBL-producing E. coli in clams collected from harvesting areas of the Central Adriatic Sea between 2018 and 2019 and study the correlation between ESBL-producing E. coli and levels of E. coli, the bacterial indicator of fecal contamination of bivalve mollusks. Moreover, EBSL- and ESBL-/AmpC-producing E. coli isolates from bivalves were characterized phenotypically, for susceptibility to antimicrobials, and genotypically, by whole-genome sequencing (WGS), to assign them to a serotype, phylogroup, sequence type (ST), and identify the presence of resistance and virulence genes and mutations that confer antimicrobial resistance.
Materials and methods
Sampling
A total of 308 samples of clams (Venus gallina), collected from 28 sampling sites of harvesting areas located along the coast of the region of Marche (Supplementary Appendixes 1, 2), were analyzed for the presence of E. coli-producing ESBL and/or class C β-lactamase (AmpC). Of these, 127 were from bivalve mollusk harvesting areas classified as B, which requires a post-harvest treatment before being placed on the market to meet E. coli health parameters. The remaining 181 samples were from areas classified as A.
Monitoring for the presence of E. coli ESBL/AmpC was performed approximately each month between 2018 and 2019 for the majority (25) of the areas.
Bivalve mollusks were externally cleaned with running potable water; then, the flesh and liquor of the bivalve mollusks were aseptically collected, diluted, homogenized, and further diluted in a 0.1% sterile peptone water to achieve a final suspension of 1:10. Subsequent decimal dilutions were prepared in a 0.1% sterile peptone solution. E. coli enumeration on bivalve mollusks was performed by a most probable number (MPN) method according to ISO 16649-3 (Anonymous, 2015).
ESBL-/AmpC-producing E. coli were isolated by streaking over the surface of MacConkey (MC) agar plates supplemented with 1 μg/mL of cefotaxime (Sigma Aldrich-Merck KGaA, Darmstadt, Germany) enriched broths of the initial shellfish suspension in double-strength mineral-modified glutamate (MMGB) broth from the E. coli enumeration method. Inoculated MC agar plates with cefotaxime were incubated at 37° C ± 1° C for 24 h ± 2 h in aerobic conditions. Two colonies showing typical characteristics of E. coli were randomly selected from each sample and isolated in trypticase soy agar (TSA, Biolife, Italy).
Escherichia coli identification
Presumptive colonies of E. coli were analyzed by PCR for the uidA gene with primers uidA-277F and uidA-934R1 and by matrix-assisted laser desorption ionization–time-of-flight mass spectrometry (MALDI-TOF MS Biotyper, Bruker Daltonics) analysis.
Antimicrobial susceptibility testing and ESBL/AmpC phenotype
For the isolates identified as E. coli, disk diffusion susceptibility tests (EUCAST, 2017a) were conducted for nine antibiotics (Supplementary Appendix 2). Inhibition diameter sizes were interpreted by using the EUCAST breakpoint tables (EUCAST, 2018), except for nalidixic acid and tetracycline, for which CLSI breakpoint values were used (CLSI, 2019).
E. coli isolates resistant to cefotaxime were screened for ESBL production by using the double disk synergy test (DDST) (EUCAST, 2017b).
For each sample, according to antimicrobial resistance screening results, one E. coli isolate was selected and further investigated for the determination of the minimum inhibitory concentration (MIC) for different antimicrobial classes and for the confirmation of ESBL production. If differences were observed in the antimicrobial susceptibility profiles of E. coli isolates from the same sample, each isolate underwent MIC tests. MIC tests were performed with Sensititre EU Surveillance Salmonella/E. coli EUVSEC Plates and Sensititre EU Surveillance ESBL EUVSEC2 Plates (Thermo Fisher Scientific), according to the Thermo Scientific Sensititre Plate Guide for Antimicrobial Susceptibility Testing (Thermo Fisher Scientific).
Clinical breakpoints provided by the Clinical and Laboratory Standards Institute (CLSI, 2021) were used for the interpretation of MICs (S: susceptible, I: intermediate, SDD: susceptible-dose dependent, and R: resistant) of the following antimicrobials: ampicillin (AMP), cefepime (FEP), cefotaxime (FOT), cefoxitin (FOX), ceftazidime (TAZ), ertapenem (ETP), imipenem (IMI), meropenem (MER), colistin (COL), gentamicin (GEN), ciprofloxacin (CIP), trimethoprim (TMP), tetracycline (TET), chloramphenicol (CHL), sulfamethoxazole (SXT), and nalidixic acid (NAL). For tigecycline (TGC) and temocillin (TRM), for which no clinical breakpoints were available from CLSI, EUCAST clinical breakpoint tables (EUCAST, 2023) were used for MIC interpretation (S: susceptible and R: resistant). In the case of azithromycin (AZI), for which no clinical breakpoint was defined, the epidemiological cutoff (ECOFF) value of 16 mg/L (EUCAST, 2023) was used for the classification of E. coli isolates as susceptible/non-susceptible. Isolates were considered ESBL if ≥8-fold reduction was observed in the MIC of any of the cephalosporins (cefotaxime or ceftazidime) combined with clavulanic acid compared with the MIC of that cephalosporin alone (EUCAST, 2017b). Isolates resistant to cefoxitin and cefepime, negative to the synergy test, were characterized as ESBL based on genetic characterization.
Multidrug resistance (MDR) was considered when isolates were resistant to three or more antimicrobial classes (Magiorakos et al., 2012).
DNA extraction and whole-genome sequencing
Genomic DNAs were extracted from 1 mL of logarithmic phase broth cultures from pure E. coli cultures by using the QIAamp DNA Mini Kit (Qiagen Inc., Hilden, Germany) following the manufacturer’s protocol for Gram-negative bacterial organisms. DNA was quantified with the Qubit fluorometer (QubitTM DNA HS Assay, Life Technologies, Thermo Fisher Scientific Inc.). DNA libraries were prepared by using the Nextera DNA Flex Library Prep Kit (Illumina Inc., San Diego, CA), according to the manufacturer’s manual, loaded onto NextSeq 500/550 Mid Output Reagent Cartridge v2, 300 cycles kit (Illumina Inc., San Diego, CA) and then sequenced on an Illumina NextSeq 500 platform, to generate 150 bp paired-end reads.
Sequence analysis
Raw data were checked for quality, trimmed using Trimmomatic v0.36 (Bolger et al., 2014), and assembled using SPAdes genome assembler v3.11.1 (Bankevich et al., 2012). Quality checks of raw data and assembled genomes are reported in the Supplementary Appendix 3, 4.
The assembled genomes were analyzed by online tools available at the Center for Genomic Epidemiology (CGE), Technical University of Denmark.2 Briefly, the FASTA files were analyzed using the following CGE databases: ResFinder (v.4.1) for antimicrobial resistance genes (ARGs) and chromosomal point mutations associated with resistance (Camacho et al., 2009; Zankari et al., 2017; Bortolaia et al., 2020), multilocus sequence typing (MLST v.2.0.9) for defining the ST (Lemee et al., 2004; Bartual et al., 2005; Wirth et al., 2006; Jaureguy et al., 2008; Camacho et al., 2009; Griffiths et al., 2010; Larsen et al., 2012), PlasmidFinder (2.0.1) for plasmid replicons (Camacho et al., 2009; Carattoli et al., 2014), VirulenceFinder (2.0.3) for virulence determinants (Camacho et al., 2009; Joensen et al., 2015; Malberg Tetzschner et al., 2020), and SeroTypeFinder (2.0) for serotyping (Joensen et al., 2015). Ribosomal multilocus sequence typing (rMLST, last updated 13 September 2022) at the Public databases for molecular typing and microbial genome diversity (PubMLST) was used for species identification (Jolley et al., 2012).
The presence of chromosomal mutations was evaluated based on the criteria that one single chromosomal mutation in the gyrA gene confers low-level resistance to quinolones, and several mutations in DNA gyrase genes (gyrA and gyrB) and topoisomerase IV genes (parC and parE) are required to increase the level of quinolone resistance in Enterobacteriaceae (Correia et al., 2017). ARG or plasmid replicons were considered present if length coverage and identity to the reference sequence were 100% and ≥ 95%, respectively. Virulence genes were considered present if length coverage and identity to the reference sequence were 100% and ≥ 90%, respectively.
Escherichia coli phylogroup and Escherichia clade assignment was performed in silico (Beghain et al., 2018) with ClermonTyping 21.03.3
To gain insight into the chromosomal or plasmid location of ESBL- /AmpC-encoding genes, assembled genomes were analyzed by MOB-suite (Robertson and Nash, 2018; v.3.0.3) to predict plasmid- and chromosome-derived sequences. Contigs harboring ESBL/AmpC-encoding genes were analyzed with ResFinder (v.4.1), PlasmidFinder (v2.0.1), and MobileElementFinder (v1.0.3; Johansson et al., 2021).
The raw sequencing data have been submitted to NCBI’s Sequence Read Archive (SRA) repository (BioProject: PRJNA882336, BioSample accessions SAMN30930934 to SAMN30930946).
Statistical analysis
Quantitative E. coli results were divided into two groups based on the level of fecal contamination (E. coli ≤ 230 MPN/100 g and E. coli > 230 MPN/100 g). Statistical analysis was performed with Fisher’s test (Stata 16.1®), and values of p < 0.05 were considered statistically significant.
To study the seasonality of ESBL E. coli in clams, samples were categorized as summer (21st of June to 22nd of September)–autumn (23rd of September to 20th of December), and winter (21st of December to 20th of March)–spring (21st of March to 20th of June), according to the season of collection.
Results
Occurrence of ESBL-and ESBL/AmpC-producing Escherichia coli and other ESBL-producing Escherichia species isolates in clam samples
Overall, ESBL-producing E. coli isolates were cultured from 10 (3%, C.I.: 2–6%) of the 308 clam samples collected between July 2018 and November 2019 from the 28 sampling points. Of these, six (2%, C.I.: 0.7–4%) and three (1%, C.I.: 0.2–3%) samples harbored ESBL- or ESBL-/AmpC-producing E. coli isolates, respectively, while both types of isolates were recovered from one sample. Of note, other ESBL-producing Escherichia species were isolated, specifically E. ruysiae from one sample, and E. marmotae, from another sample, which also harbored an isolate of ESBL-/AmpC-producing E. coli. The latter species were presumably identified as E. coli, by PCR for the uidA gene and MALDI-TOF, and subsequently as E. marmotae and E. ruysiae by rMLST (100% with 53 exact matches) of WGS data.
Antimicrobial resistance phenotype of ESBL- and ESBL-/AmpC-producing Escherichia coli and other ESBL-producing Escherichia spp.
Distribution of MIC values among the 13 Escherichia spp. isolates is reported in Table 1. Overall, all 13 ESBL- and ESBL-/AmpC-producing Escherichia spp. (n = 11 E. coli, n = 1 E. marmotae, n = 1 E. ruysiae) isolates showed resistance to ampicillin and cefotaxime, while 2 and 3 isolates were resistant to cefepime and ceftazidime, respectively, and 4 isolates had intermediate susceptibility to ceftazidime. Resistance and intermediate susceptibility to cefoxitin were found in 3 and 1 of the 13 isolates, respectively. Moreover, resistance to non-beta-lactam antibiotics was also observed to nalidixic acid (6/13), tetracycline (6/13), chloramphenicol (3/13), trimethoprim (4/13), sulfamethoxazole (9/13), gentamicin (2/13), and azithromycin (2/13). Of note, resistance to ciprofloxacin was found in three of the isolates. All (13 out of 13) isolates showed susceptibility to carbapenems (ertapenem, imipenem, and meropenem). Additionally, all isolates were susceptible to tigecycline, colistin, and temocillin. The majority (8 out of 11) of the ESBL- or ESBL-/AmpC-producing E. coli isolates were MDR (Table 2). The ESBL-producing E. marmotae was resistant to ampicillin and cefotaxime (Table 2), whereas the E. ruysiae was resistant to ampicillin, cefotaxime, and sulfamethoxazole and intermediate- and susceptible-dose dependent to ceftazidime and cefoperazone (Table 2).
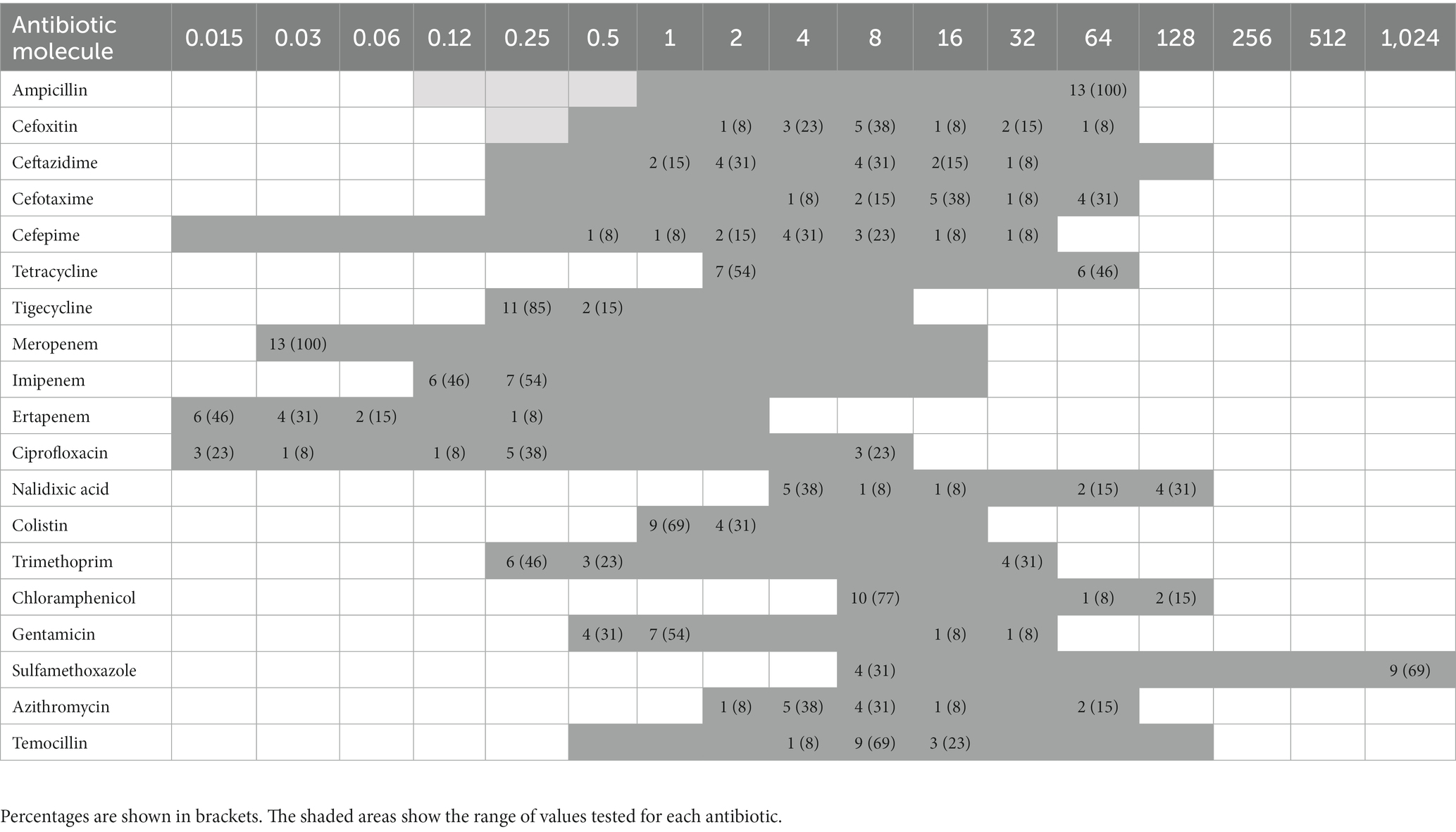
Table 1. Distribution of MIC (minimum inhibitory concentration) values among the 13 ESBL- or ESBL-/AmpC-producing Escherichia coli (11 isolates) and ESBL-producing E. marmotae (1 isolate) and E. ruysiae (1 isolate) from clams.
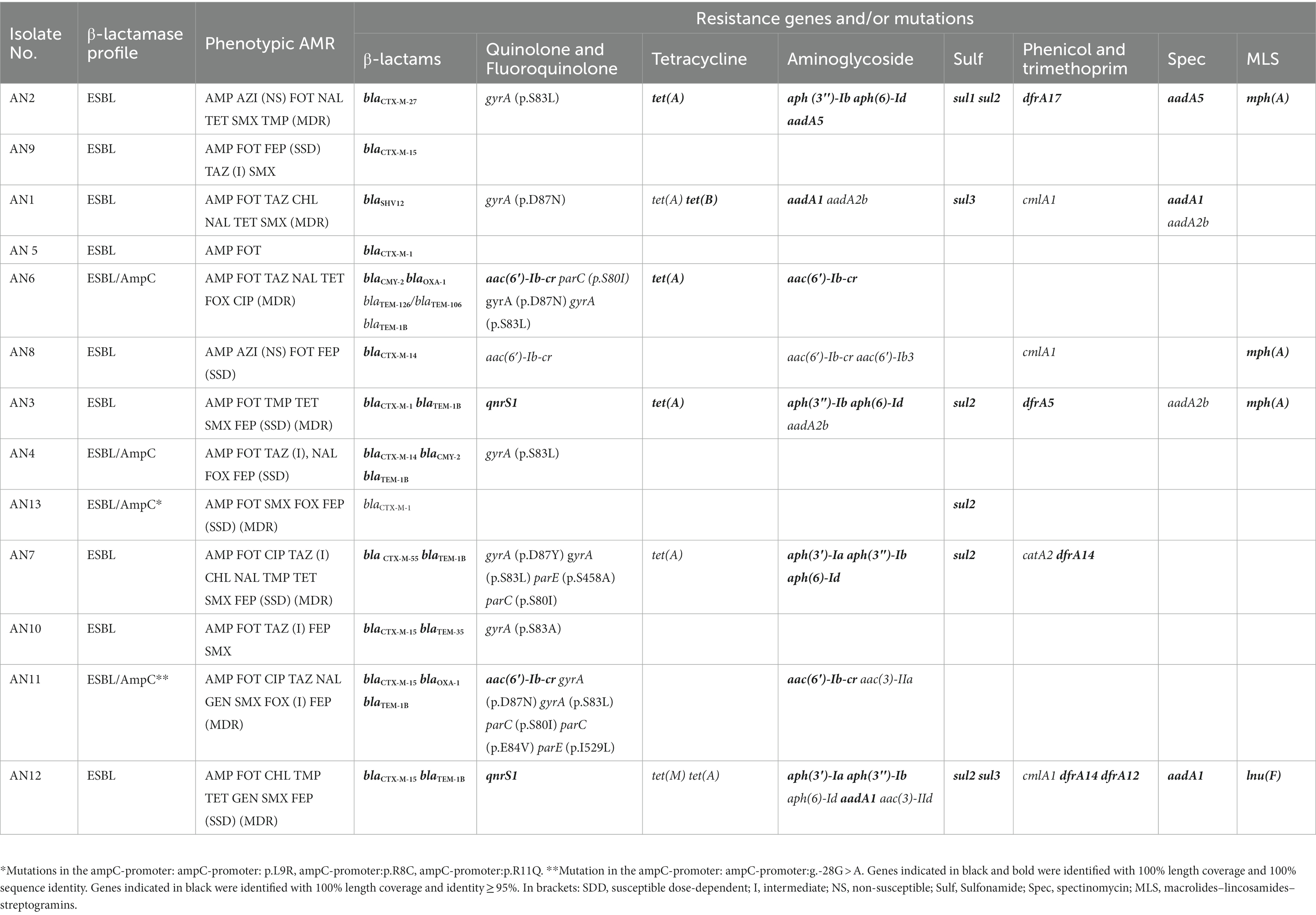
Table 2. Phenotypic antimicrobial resistance (AMR) profile, resistance genes, and gene mutations for AMR of ESBL- and ESBL-/AmpC-producing Escherichia spp. isolates from clams.
Genomic analysis of the ESBL- and ESBL-/AmpC-producing Escherichia coli
Among the 11 sequenced E. coli isolates, ARGs were detected for beta-lactams (n = 11), fluoroquinolones (n = 5), tetracyclines (n = 6), aminoglycosides (n = 8), sulphonamides (n = 6), phenicols (n = 4), trimethoprim (n = 4), spectinomycin (n = 4), macrolides (n = 3), and lincosamide (n = 1; Table 2).
ESBLs were encoded in 3 out of 11 E. coli isolates from clams by the blaCTX-M-15 gene; other common CTX-M variants were blaCTX-M-14 and blaCTX-M-1 (2 out of 11 isolates each), whereas blaCTX-M27 and blaCTX-M55 were each present in 1 of the 11 isolates (Table 2). Other ESBL-producing genes found in the E. coli isolates from clams were the blaSHV12 gene (1 out of 11 isolates) and one blaTEM gene that had a sequence identity of 99.8% to blaTEM-106 (859/861 bp) and blaTEM-126 (859/861 bp), respectively. The combination of an ESBL (CTX-M-14 or a TEM enzyme with a gene sequence identity of 99.8% to blaTEM-106 and blaTEM-126) with a plasmidic AmpC β-lactamase (CMY-2) was found in two out of four of the isolates (Table 2). The remaining AmpC-producing E. coli isolates had mutations in the AmpC promoter (p.L9R, p.R8C, and p.R11Q; g.-28G > A, Table 2). The identified fluoroquinolone resistance genes were aac(6′)-Ib-cr and qnrS1 in three and two isolates, respectively. Moreover, seven isolates had at least one point mutation known to mediate quinolone resistances in the chromosomal gyrA, while three isolates also possessed at least one additional mutation in the genes parC/parE. Tetracycline resistance genes tetA, tetB, and tetM were found in 6, 1, and 1 of the 11 isolates, respectively.
By the MOB-suite analysis of assembled genomes, 9 of the 11 contigs harboring ESBL-encoding genes and the two contigs harboring AmpC-encoding genes were classified as plasmid-derived sequences (Table 3; Supplementary Appendix 5). The presence of mobile elements and other antibiotic resistance genes in the same contigs harboring ESBL-/AmpC-encoding genes was also investigated and is reported in Table 3.
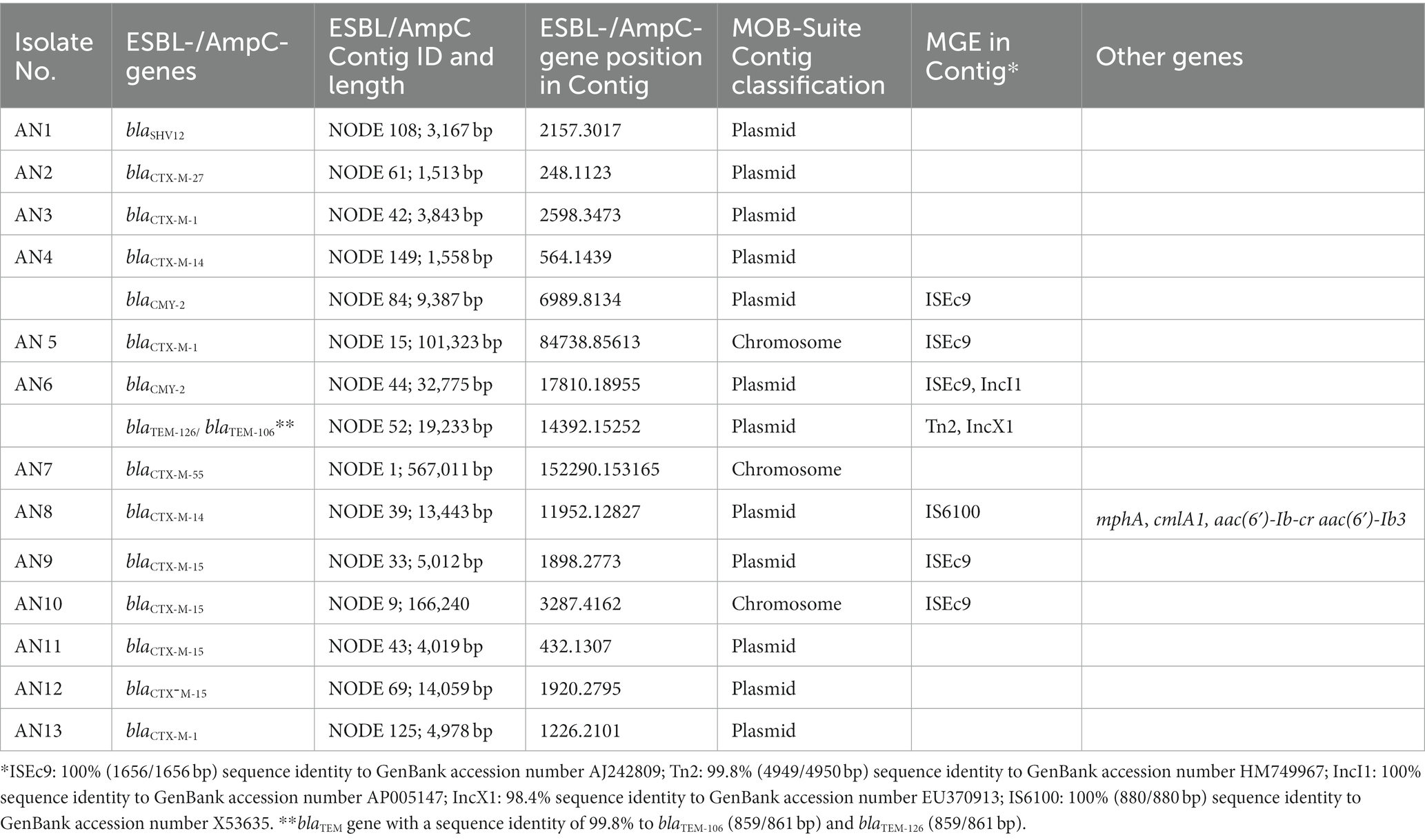
Table 3. Predicted genomic location (plasmid or chromosome) of ESBL- and AmpC-encoding genes using the MOB-Suite and presence of mobile genetic elements (MGEs) and other antimicrobial resistance genes in the same contig.
ESBL- and ESBL/AmpC-producing E. coli strains were diverse in serotype and fimbriae (Table 4). Most (9 out of 11) of the E. coli isolates were included in 6 different clonal complexes (Table 4); of these, CC10 and CC69 were present in 3 and 2 of the 11 ESBL and ESBL/AmpC E. coli isolates, respectively. Notably, the pandemic extra-intestinal pathogenic E. coli (ExPEC) ST131 clone (clonal complex CC131) was detected. Four phylogenetic groups (Table 5) were identified, of which phylogroup A was the most prevalent (45%), followed by phylogroup D (36%).
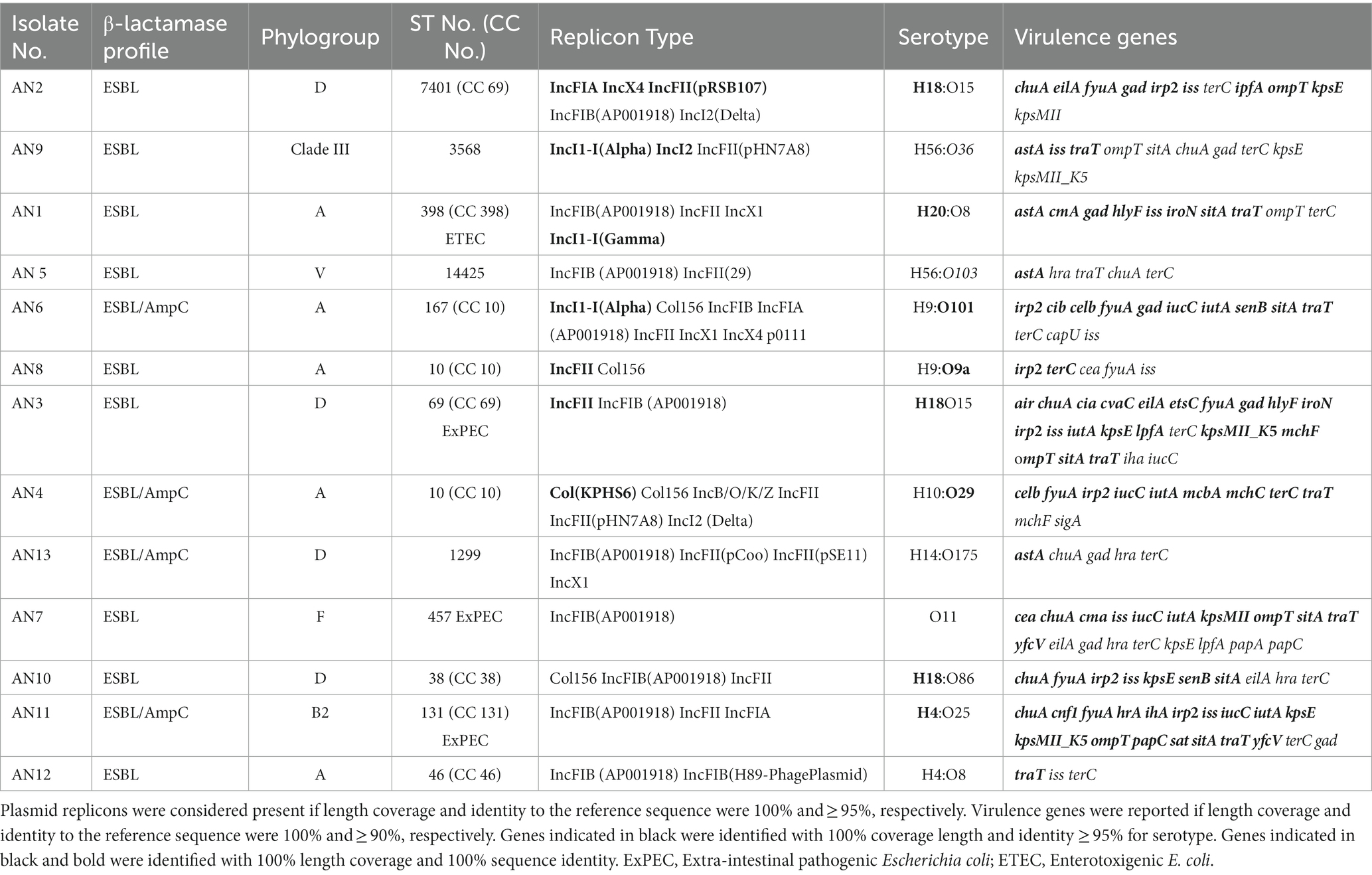
Table 4. Phylogroup, sequence type (ST), clonal complex (CC), replicon type, serotype, and virulence genes of ESBL- and ESBL-/AmpC-producing Escherichia spp. isolates from clams.
Most strains harbored a broad virulence repertoire; moreover, in 3 out of 11 of the ESBL- and EBSL-/AmpC-producing E. coli isolates, at least two genes encoding for ExPEC virulence factors (papA and/or papC, kpsM II, iutA, afa/draBC, and sfa/foc) were identified (Table 4).
Genomic analysis of the ESBL-producing Escherichia spp.
Among the 13 sequenced Escherichia spp., two isolates of ESBL producers were identified by rMLST (100% with 53 exact matches each) as E. marmotae and E. ruysiae and assigned by phylogroup analysis to clades V and III, respectively. The E. marmotae strain harbored the astA gene for the heat-stable enterotoxin 1 and other virulence genes (Table 4), whereas the ESBL was encoded by the blaCTX-M-1 (Table 2). The latter was predicted to be located in a chromosome-derived sequence (Table 3, AN5). A new ST (14425) was identified in the E. marmotae strain (Table 4). The E. ruysiae strain was assigned to ST 3568 by MLST analysis. Several putative virulence genes were predicted from the genome sequence of the E. ruysiae strain, including the enterotoxin astA gene (Table 4). The ESBL was encoded by the blaCTX-M-15 gene (Table 2), which was located in a contig classified as a plasmid-derived sequence (Table 3, AN9).
Occurrence of ESBL- and ESBL-/AmpC-producing Escherichia coli isolates and seasonality at sampling points
Over the studied period, the prevalence of ESBL- (including ESBL- and AmpC-)-producing E. coli isolates (Figure 1) ranged from 0% (no isolation) at 21 (75%) of the 28 sampling sites to 27% (3 out of 11 samples) in an area that was not suitable for the direct human consumption of bivalve mollusks.
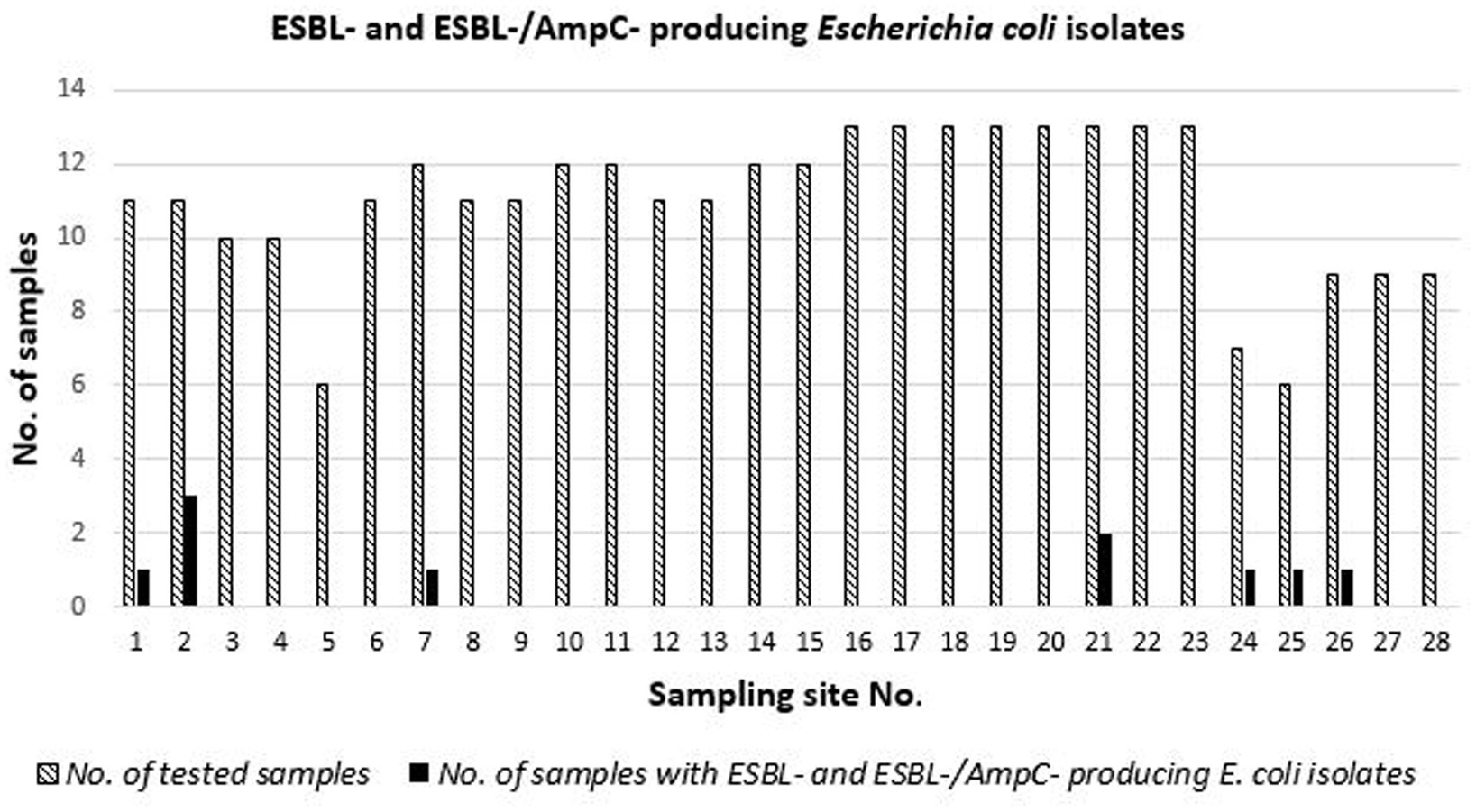
Figure 1. Occurence of ESBL-producing E. coli isolotes at 28 sampling sites of the Central Adriatic in 308 samples of clams collected between July 2018 and November 2019.
Considering the seasonality, ESBL-producing E. coli were isolated in 3 of 85 (4%, C.I.95 1–10%), 3 of 62 (5%, C.I.95 1–14%), and 4 of 54 (7%, C.I.95 2–18%) of the samples collected in autumn, winter, and spring, respectively. Furthermore, ESBL-producing E. coli were not isolated from 107 samples of clams collected during the summer season.
The occurrence of ESBL-/ESBL- and AmpC-producing E. coli STs at the sampling sites is reported in Table 6. Variability of STs and resistance to antimicrobials were found in strains isolated from clams sampled over time. One site (sampling point 2) had the greatest variability, with isolates of different STs harboring different ESBL-producing genes (Table 6).
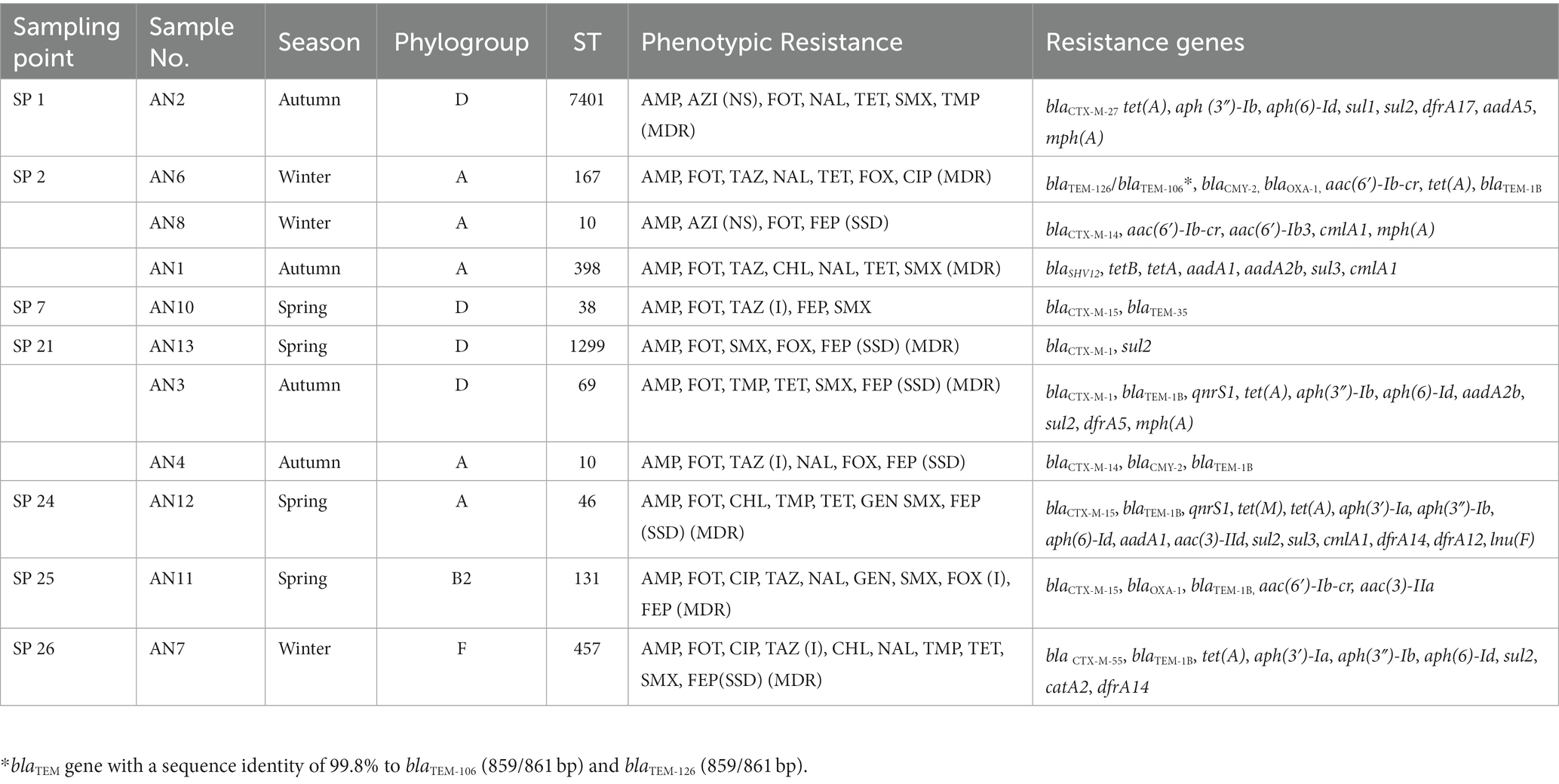
Table 6. ESBL- (ESBL-/ESBL- and AmpC-) producing E. coli recovered from 308 samples of clams collected from harvesting areas of the Central Adriatic between July 2018 and November 2019.
Escherichia coli Levels and ESBL-producing Escherichia isolates in clams
The fecal indicator E. coli was quantified (MPN/100 g) in the 308 clam samples. E. coli numbers of clam samples were grouped into two E. coli contamination levels (<230 MPN/100 g and ≥ 230 MPN/100 g) and were further divided depending on the detection or not of ESBL-producing E. coli isolates (Table 7). ESBL-producing E. coli isolates were significantly more likely to be present (p = 0.008) among clam samples with E. coli > 230 MPN/100 g (4 out of 28 samples, 14% C.I.95: 5–33%) than in those with E. coli ≤ 230 MPN/100 g (6 out of 280 samples, 2% C.I.95: 1–5%).
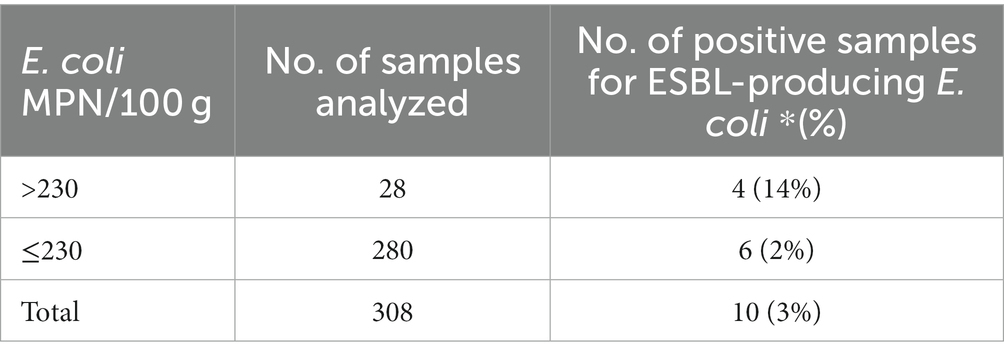
Table 7. Presence/absence of ESBL- (ESBL- and ESBL-/AmpC-) producing E. coli isolates in 308 samples of clams according to the levels of E. coli (<230 MPN/100 g and ≥ 230 MPN/100 g).
The ETEC and two of the three ExPEC strains were isolated from samples with E. coli contamination levels >230 MPN/100 g. The majority (7 out of 11, 63%) of ESBL- and ESBL/AmpC-producing E. coli isolates were recovered from samples of areas classified as not suitable for direct human consumption.
Discussion
Gut bacteria from humans or terrestrial animals, including those resistant to antimicrobials, can enter aquatic environments through various routes. Thus, bivalves, which are filter-feeder animals, may act as possible carriers of bacteria derived from fecal pollution. Antimicrobial resistance monitoring programs in the EU focus on terrestrial animals, while studies on the presence of ESBL-producing bacteria in seafood products are limited.
We report here on the presence of ESBL-producing E. coli strains in bivalves collected in Italy between 2018 and 2019 from sampling points of production areas of the Central Adriatic Sea. To our knowledge, this is one of the few studies performed worldwide to investigate the occurrence of ESBL-producing E. coli strains in bivalves from production areas (Rees et al., 2015; Bueris et al., 2022). Moreover, this study investigated the relationship between levels of E. coli, the bacterial indicator of fecal contamination, and the presence of E. coli strains with an ESBL phenotype in bivalves. Overall, E. coli producers of ESBL were recovered from 3% of clam samples collected at several sampling sites in the studied period; of these, 2% and 1% harbored ESBL- and ESBL-/AmpC-producing E. coli isolates, respectively. Previous studies in bivalve production areas were conducted in other countries and were limited in the sample collection period (Rees et al., 2015; Bueris et al., 2022). In a study conducted in Canada (Rees et al., 2015), with a study period of 2 months, ESBL-producing E. coli isolates were not recovered from oysters harvested from sampling points of an open oyster fishery and a restricted zone for bivalves (Rees et al., 2015). In another study from Brazil, ceftriaxone-resistant E. coli isolates with an ESBL phenotype were cultured from edible bivalves (oysters and brown mussels) collected from three locations of a polluted area on the South American Atlantic coast (Bueris et al., 2022). Other studies reporting on the prevalence of ESBL-producing E. coli in bivalves were performed at retail in European countries (Boss et al., 2016; Vu et al., 2018). In the latter studies, E. coli producers of ESBL or AmpC were not recovered from retail sampled oysters (n = 10) in Switzerland (Boss et al., 2016), whereas in Germany, ESBL-producing Enterobacteriaceae were isolated in 20% of bivalve samples collected at retail level in Berlin, with the bivalves originating from several European countries, including Italy (Vu et al., 2018). Another study from North Africa reported a prevalence of 1.6% of ESBL-producing Enterobacterales (mostly E. coli) among 215 analyzed pools of 5 clams (Ruditapes decussatus), which were sampled in unrelated markets in four different regions of Tunisia (Sola et al., 2022).
All ESBL- or ESBL-/AmpC-producing E. coli isolated in this study from bivalves were resistant to cefotaxime, a third-generation cephalosporin, and, to a lesser extent, to ceftazidime (27%) and fourth-generation cephalosporin cefepime (18%). Moreover, resistance to fluoroquinolones, another antibiotic class recognized as the highest priority critically important antimicrobials (HPCIAs) in human medicine (World Health Organization, 2019), was recorded in 27% of the ESBL- or ESBL-/AmpC-producing E. coli isolates.
Other studies have reported the proportion of third- or fourth-generation cephalosporin-resistant isolates of E. coli relative to the total number of isolates recovered from bivalve samples from production areas (Vignaroli et al., 2016; Grevskott et al., 2017; Miotto et al., 2019; Jeong et al., 2021). However, a screening method of a selective medium with an antibiotic to isolate third-generation cephalosporin-resistant E. coli strains was not applied in these studies; therefore, the prevalence of ESBL in samples collected at harvesting areas was not determined. In a systematic review and meta-analysis on antimicrobial resistance in marine bivalves from our group, resistance to third/fourth/fifth-generation cephalosporins and fluoroquinolones was recorded in approximately 10% of E. coli isolates, while resistance to carbapenems was not reported in E. coli strains from bivalves (Albini et al., 2022). Accordingly, resistance to carbapenems (ertapenem, imipenem, and meropenem) was not found in the ESBL- and ESBL-/AmpC-producing E. coli strains we isolated from bivalves.
CTX-M-type enzymes are the most common global ESBL in E. coli; among these, CTX-M-15 is the most frequent, followed by CTX-M-14 (Peirano and Pitout, 2019). Noteworthy, a recent study performed in Italy has reported CTX-M types as prevalent in both ExPEC human and animal isolates, and among these, the CTX-M-15 enzyme is largely predominant in human isolates and in a consistent percentage of the isolates from different animal species (Giufrè et al., 2021). According to the same study, the second most common CTX-M enzyme in Italian isolates was CTX-M-27 in humans and CTX-M-1 in animals. Previous studies performed on bivalve mollusks reported that the ESBL phenotype in E. coli was predominantly due to the presence of blaCTX-M genes (Vu et al., 2018; Bueris et al., 2022; Sola et al., 2022), with the most frequent one detected in isolates from clams sampled at retail in Tunisia being blaCTX-M-15, followed by blaCTX-M-1 and blaCTX-M-14 (Sola et al., 2022). In agreement with these studies, our analysis of ESBL genes revealed that the most prevalent gene found in E. coli isolates from bivalves of the Central Adriatic Sea in the studied period was the blaCTX-M-15 gene, followed by blaCTX-M-14 and blaCTX-M-1, whereas blaCTX-M27 and blaCTX-M55 were less represented. Interestingly, other ESBL-producing genes were found in a minor percentage of the E. coli isolates from clams (blaSHV12 and blaTEM genes). Additionally, 18% of ESBL-producing E. coli isolates from clams possessed the blaCMY-2 gene for the plasmidic class C β-lactamases. Of the latter, only the CMY-2 enzyme was identified in clams. Analysis of the chromosomal or plasmid location evidenced that the majority of ESBL- /AmpC-encoding genes were harbored in sequences classified as plasmid-derived by the MOB suite. The genomic location of ESBL- /AmpC-encoding genes on plasmids in E. coli from the marine environment is worrying from a public health perspective as plasmids play an important role in the horizontal transfer of resistance genes. Moreover, mobile genetic elements such as insertion sequences (ISEcp1, synonym of ISEc9, and IS6100) and transposons (Tn2) were also found in the same contigs of the blaCTX-M (in five isolates) and the blaCMY-2 genes (in two isolates).
Phylogenetic group analysis showed that phylogroup A was the most prevalent (45%) in ESBL- and ESBL/AmpC-producing E. coli isolates, followed by other phylogroups that include isolates associated with human extra-intestinal infections (D, F, and B2). Among the sequenced ESBL- and ESBL-/AmpC-producing E. coli isolates, a high genomic diversity (10 different STs in 11 isolates) was observed, yet some clinically important STs were identified (ST131, ST38, ST10, ST69, ST457, and ST398). Of these, E. coli ST131, ST69, and ST457 showed ExPEC status and carried blaCTX-M variants. The E. coli ST131 isolate from clams was MDR and had genomic features of clade C of the pandemic E. coli ST131 lineage (Denamur et al., 2021), which is the most prevalent ExPEC clonal group isolated in extra-intestinal infections in humans (Nicolas-Chanoine et al., 2014). The ST131 clone has previously been reported in water environments (Colomer-Lluch et al., 2013; Nicolas-Chanoine et al., 2014; Jørgensen et al., 2017), influent (Nicolas-Chanoine et al., 2014; Jørgensen et al., 2017), effluent treated wastewaters of water treatment plants (Zhi et al., 2020; Sekizuka et al., 2022), and bivalves (Vignaroli et al., 2016; Bueris et al., 2022; Sola et al., 2022). The ST457 is a broad host range, globally disseminated diverse E. coli lineage that can cause human extra-intestinal disease (Nesporova et al., 2021). A study focusing on ST457 evidenced that Australian human clinical and silver gull strains were closely related, suggesting that ST457 was an emerging ESBL lineage with reservoirs in wildlife and food-producing animals (Nesporova et al., 2021). ST38, ST10, and ST69 found in clams in a study conducted in Italy were reported to be more frequently detected in both human and animal isolates (Giufrè et al., 2021). Moreover, ST10 was one of the most represented STs among isolates from cattle and pigs, while ST 69 was also largely represented in isolates from pigs (Giufrè et al., 2021).
In this study, other ESBL-producing Escherichia spp., E. marmotae (Liu et al., 2015) and E. ruysiae (Van der Putten et al., 2021), were identified. Phylogroup analysis had previously assigned these species to Escherichia cryptic clades V and III, respectively. Previous studies on Escherichia cryptic clades have speculated that these may represent environmentally adapted Escherichia lineages that may be more abundant outside the gastrointestinal tract of the host (Walk et al., 2009; Ingle et al., 2011). Cryptic lineages of Escherichia were unlikely to be detected in human fecal samples and were more abundant in animal feces, ranging from 3% to 8% in non-human mammals to 8–28% in birds (Clermont et al., 2011). E. marmotae isolated from the feces of wild rodents (Marmota himalayana) has been reported as a potential invasive pathogen (Liu et al., 2019). In addition, human-invasive infections caused by E. marmotae have recently been described, and this Escherichia species has only recently been identified as a new common pathogen because it can be easily misidentified as E. coli in routine diagnostic laboratories (Sivertsen et al., 2022). Like animal isolates from wild rodents (Liu et al., 2019) and clinical isolates from human-invasive infections (Sivertsen et al., 2022), the ESBL-producing isolate of E. marmotae from clams harbored the enterotoxin-encoding gene astA. Moreover, the E. coli in silico serotyper evidenced that it contained the fliC-H56 flagellar antigen gene, as did the human clinical isolates (Sivertsen et al., 2022). Available data on E. marmotae isolates from different sources showed infrequent occurrences of antimicrobial resistance (Sivertsen et al., 2022); isolates from reported human-invasive infections were phenotypically susceptible to tested antimicrobials, and resistance genes were not identified in their genomes (Sivertsen et al., 2022). Differently from these findings, the E. marmotae strain from clams was an ESBL-producing isolate, phenotypically resistant to cefotaxime, which possessed the blaCTX-M-1 gene.
Escherichia ruysiae sp. nov. was proposed by van der Putten et al. (2021) as a novel species, encompassing Escherichia cryptic clades III and IV. The strain of E. ruysiae was isolated from the fecal material of an international traveler, harbored the blaCTX-M-14 gene, and was of cryptic clade IV (van der Putten et al., 2021). Differently from the human isolate, the isolate of ESBL-producing E. ruysiae from clams in this study harbored the blaCTX-M-15 gene, the enterotoxin-encoding gene astA, and belonged to cryptic clade III.
In the EU, the microbiological safety of bivalve mollusks is based on the classification and monitoring of production areas. Assessing the sources and types of fecal contamination in the vicinity of the areas and how these affect mollusk production areas, combined with the quantitative monitoring of the fecal indicator organism E. coli, is critical to providing an estimate of the risk of contamination of an area by microbial pathogens.
To the best of our knowledge, this study was the first to investigate the correlation between the presence of ESBL-producing E. coli and the bacterial indicator of fecal contamination of E. coli in bivalve mollusks. Over the studied period, ESBL (including ESBL-/AmpC-)-producing E. coli were not isolated in clams from most (75%) of the studied sampling sites (n = 28), while the highest frequency of isolation (27%) was observed in an area requiring post-harvest treatment to reduce microbiological contamination before human consumption. A significant correlation was found between the indicator of fecal contamination by E. coli above 230 MPN/100 g and the presence of ESBL-producing E. coli. Thus, this study provided evidence of E. coli in molluscan shellfish as an index of the potential presence of ESBL-producing E. coli isolates, which are bacteria resistant to a critically important class of highest-priority antimicrobials.
Considering seasonality, ESBL-producing E. coli were not isolated in clam samples collected in summer but in other seasons (8% in spring, 5% in winter, and 4% in autumn), although these differences in prevalence were not found to be significant. One area had the greatest variability in STs and ESBL-producing genes, whereas the blaCTX-M-1 variant was present in one specific area. These findings can be explained by the variability in the presence and type of pollution sources affecting the microbiological contamination of bivalve mollusk areas and by environmental effects (e.g., rainfalls, winds, and tidal currents) on pollution sources. A large number of uncertainties exist with respect to the sources and transmission routes of antimicrobial-resistant bacteria and antimicrobial-resistance genes in food-producing environments.
In future studies, we will investigate the presence in bivalves of ESBL-producing E. coli, other antimicrobial-resistant bacteria, and antimicrobial-resistance genes of importance in human health in relation to the sources and types of pollution, seasonal variations, and climatic factors that influence production areas.
In conclusion, this study presents novel observations on the prevalence, seasonality, genomic, and phenotypic characteristics of ESBL-producing E. coli isolates in bivalves from production areas. ESBL-producing E. coli isolates were significantly more likely to be present among clam samples with higher levels of E. coli contamination (> 230 MPN/100 g) than among those with lower levels (≤ 230 MPN/100 g). Furthermore, potentially pathogenic ESBL-producing E. coli strains (ETEC and ExPEC) were isolated mainly in samples with E. coli contamination levels above 230 MPN/100 g. These findings provided evidence in support of E. coli as an index organism for the presence of ESBL-producing E. coli.
Data availability statement
The dataset is available in Appendix 1 of Supplementary Material. Sequencing data are published in online repositories and accession numbers are specified in the material and methods section.
Author contributions
FL: study conception. FL, CM, FB, FM, EA, LC, and CC: methodology. FL, CM, and FB: validation and study design. LS, GA, SP, EA, and FM: laboratory analysis. FL, AR, and FM: bioinformatics analysis. LC: isolate preparation for NGS analysis. CC and SP: NGS sequencing. FB: statistical analysis. FL, FB, EA, and FM: data curation. AD and FL: resources, project administration, and funding acquisition. FL: writing–original draft preparation. CM, FB, EA, and FM: writing–reviewing and editing supervision. All authors contributed to the article and approved the submitted version.
Funding
This study was supported by the Italian Ministry of Health through project IZSUM RC006/2016 and regional funding through project RCIS12019.
Acknowledgments
The authors thank colleagues in the food control microbiology laboratories for technical assistance. The authors are grateful to Dr. Enrico Buenaventura for his helpful comments and his review of the manuscript before submission.
Conflict of interest
The authors declare that the research was conducted in the absence of any commercial or financial relationships that could be construed as a potential conflict of interest.
Publisher’s note
All claims expressed in this article are solely those of the authors and do not necessarily represent those of their affiliated organizations, or those of the publisher, the editors and the reviewers. Any product that may be evaluated in this article, or claim that may be made by its manufacturer, is not guaranteed or endorsed by the publisher.
Supplementary material
The Supplementary material for this article can be found online at: https://www.frontiersin.org/articles/10.3389/fmicb.2023.1219008/full#supplementary-material
Footnotes
1. ^STEC Reference Center, http://www.shigatox.net/new/tools/ecmlst.html.
References
Aerts, M., Battisti, A., Hendriksen, R., Kempf, I., Teale, C., Tenhagen, B. A., et al. (2019). Technical specifications on harmonised monitoring of antimicrobial resistance in zoonotic and indicator bacteria from food-producing animals and food. EFSA J. 17:e05709. doi: 10.2903/j.efsa.2019.5709
Albini, E., Orso, M., Cozzolino, F., Sacchini, L., Leoni, F., and Magistrali, C. F. (2022). A systematic review and meta-analysis on antimicrobial resistance in marine bivalves. Front. Microbiol. 13:1040568. doi: 10.3389/fmicb.2022.1040568
Anonymous (2004). Regulation (EC) no 854/2004 of the European Parliament and of the council of 29 April 2004 laying down specific rules for the organisation of official controls on products of animal origin intended for human consumption. Off. J. Eur. Union L226, 83–127.
Anonymous (2015). ISO 16649-3:2015. Microbiology of the food chain - Horizontal method for the enumeration of beta-glucuronidase-positive Escherichia coli - Part 3: Detection and most probable number technique using 5-bromo-4-chloro-3-indolyl-ß-D-glucuronide.
Anonymous (2019). Commission implementing regulation (EU) 2019/627 of 15 March 2019 laying down uniform practical arrangements for the performance of official controls on products of animal origin intended for human consumption in accordance with regulation (EU) 2017/625. Off. J. Eur. Union L131, 51–100.
Bankevich, A., Nurk, S., Antipov, D., Gurevich, A. A., Dvorkin, M., Kulikov, A. S., et al. (2012). SPAdes: a new genome assembly algorithm and its applications to single-cell sequencing. J. Comput. Biol. 19, 455–477. doi: 10.1089/cmb.2012.0021
Bartual, S. G., Seifert, H., Hippler, C., Domı, M. A., Wisplinghoff, H., and Rodrı, F. (2005). Development of a multilocus sequence typing scheme for characterization of clinical isolates of Acinetobacter baumannii. J. Clin. Microbiol. 43, 4382–4390. doi: 10.1128/JCM.43.9.4382
Beghain, J., Bridier-Nahmias, A., Le Nagard, H., Denamur, E., and Clermont, O. (2018). ClermonTyping: an easy-to-use and accurate in silico method for Escherichia genus strain phylotyping. Microb. Genom. 4:e000192. doi: 10.1099/mgen.0.000192
Bezabih, Y. M., Bezabih, A., Dion, M., Batard, E., Teka, S., Obole, A., et al. (2022). Comparison of the global prevalence and trend of human intestinal carriage of ESBL-producing Escherichia coli between healthcare and community settings: a systematic review and meta-analysis. JAC Antimicrob. Resist. 4:dlac048. doi: 10.1093/jacamr/dlac048
Bezabih, Y. M., Sabiiti, W., Alamneh, E., Bezabih, A., Peterson, G. M., Bezabhe, W. M., et al. (2021). The global prevalence and trend of human intestinal carriage of ESBL-producing Escherichia coli in the community. J. Antimicrob. Chemother. 76, 22–29. doi: 10.1093/jac/dkaa399
Bolger, A. M., Lohse, M., and Usadel, B. (2014). Trimmomatic: a flexible trimmer for Illumina sequence data. Bioinformatics 30, 2114–2120. doi: 10.1093/bioinformatics/btu170
Bortolaia, V., Kaas, R. S., Ruppe, E., Roberts, M. C., Schwarz, S., Cattoir, V., et al. (2020). ResFinder 4.0 for predictions of phenotypes from genotypes. J. Antimicrob. Chemother. 75, 3491–3500. doi: 10.1093/jac/dkaa345
Boss, R., Overesch, G., and Baumgartner, A. (2016). Antimicrobial resistance of Escherichia coli, enterococci, Pseudomonas aeruginosa, and Staphylococcus aureus from raw fish and seafood imported into Switzerland. J. Food Prot. 79, 1240–1246. doi: 10.4315/0362-028X.JFP-15-463
Bueris, V., Sellera, F. P., Fuga, B., Sano, E., Carvalho, M. P. N., Couto, S. C. F., et al. (2022). Convergence of virulence and resistance in international clones of WHO critical priority enterobacterales isolated from marine bivalves. Sci. Rep. 12:5707. doi: 10.1038/s41598-022-09598-8
Camacho, C., Coulouris, G., Avagyan, V., Ma, N., Papadopoulos, J., Bealer, K., et al. (2009). BLAST+: architecture and applications. BMC Bioinformatics 10:421. doi: 10.1186/1471-2105-10-421
Carattoli, A., Zankari, E., Garciá-Fernández, A., Larsen, M. V., Lund, O., Villa, L., et al. (2014). In silico detection and typing of plasmids using plasmidfinder and plasmid multilocus sequence typing. Antimicrob. Agents Chemother. 58, 3895–3903. doi: 10.1128/AAC.02412-14
Clermont, O., Gordon, D. M., Brisse, S., Walk, S. T., and Denamur, E. (2011). Characterization of the cryptic Escherichia lineages: rapid identification and prevalence. Environ. Microbiol. 13, 2468–2477. doi: 10.1111/j.1462-2920.2011.02519.x
CLSI. (2019). Performance standards for antimicrobial susceptibility testing. CLSI supplement M100. 29th Edn. Wayne, PA: CLSI.
CLSI. (2021). Performance standards for antimicrobial susceptibility testing M100. 31st Edn. Wayne, PA: CLSI.
Colomer-Lluch, M., Mora, A., López, C., Mamani, R., Dahbi, G., Marzoa, J., et al. (2013). Detection of quinolone-resistant Escherichia coli isolates belonging to clonal groups O25B:H4-B2-ST131 and O25B:H4-D-ST69 in raw sewage and river water in Barcelona, Spain. J. Antimicrob. Chemother. 68, 758–765. doi: 10.1093/jac/dks477
Correia, S., Poeta, P., Hébraud, M., Capelo, J. L., and Igrejas, G. (2017). Mechanisms of quinolone action and resistance: where do we stand? J. Med. Microbiol. 66, 551–559. doi: 10.1099/jmm.0.000475
Denamur, E., Clermont, O., Bonacorsi, S., and Gordon, D. (2021). The population genetics of pathogenic Escherichia coli. Nat. Rev. Microbiol. 19, 37–54. doi: 10.1038/s41579-020-0416-x
EUCAST. (2017a). Antimicrobial susceptibility testing EUCAST disk diffusion method, pp. 1–21. Available at: https://www.eucast.org/fileadmin/src/media/PDFs/EUCAST_files/Disk_test_documents/2017_manuals/Manual_v_6.0_EUCAST_Disk_Test_final.pdf.
EUCAST. (2017b). The European committee on antimicrobial susceptibility testing. EUCAST guidelines for detection of resistance mechanisms and specific resistances of clinical and/or epidemiological importance, pp. 1–43. Available at: https://www.eucast.org/fileadmin/src/media/PDFs/EUCAST_files/Resistance_mechanisms/EUCAST_detection_of_resistance_mechanisms_170711.pdf.
EUCAST. (2018). EUCAST breakpoint tables for interpretation of MICs and zone diameters. Available at: https://www.eucast.org/fileadmin/src/media/PDFs/EUCAST_files/Breakpoint_tables/v_8.1_Breakpoint_Tables.pdf.
EUCAST. (2023). European committee on antimicrobial susceptibility testing breakpoint tables for interpretation of MICs and zone diameters, p. 77. Available at: https://www.eucast.org/fileadmin/src/media/PDFs/EUCAST_files/Breakpoint_tables/v_13.0_Breakpoint_Tables.pdf.
European Commission. (2022). The EU fish market. Luxembourg: Publications Office of the European Union.
Giufrè, M., Mazzolini, E., Cerquetti, M., Brusaferro, S., Accogli, M., Agnoletti, F., et al. (2021). Extended-spectrum β-lactamase-producing Escherichia coli from extraintestinal infections in humans and from food-producing animals in Italy: a ‘one health’ study. Int. J. Antimicrob. Agents 58:106433. doi: 10.1016/j.ijantimicag.2021.106433
Grevskott, D. H., Svanevik, C. S., Sunde, M., Wester, A. L., and Lunestad, B. T. (2017). Marine bivalve mollusks as possible indicators of multidrug-resistant Escherichia coli and other species of the Enterobacteriaceae family. Front. Microbiol. 8:24. doi: 10.3389/fmicb.2017.00024
Griffiths, D., Fawley, W., Kachrimanidou, M., Bowden, R., Crook, D. W., Fung, R., et al. (2010). Multilocus sequence typing of Clostridium difficile. J. Clin. Microbiol. 48, 770–778. doi: 10.1128/JCM.01796-09
Ingle, D. J., Clermont, O., Skurnik, D., Denamur, E., Walk, S. T., and Gordon, D. M. (2011). Biofilm formation by and thermal niche and virulence characteristics of Escherichia spp. Appl. Environ. Microbiol. 77, 2695–2700. doi: 10.1128/AEM.02401-10
Jaureguy, F., Landraud, L., Passet, V., Diancourt, L., Frapy, E., Guigon, G., et al. (2008). Phylogenetic and genomic diversity of human bacteremic Escherichia coli strains. BMC Genomics 9:560. doi: 10.1186/1471-2164-9-560
Jeong, S. H., Kwon, J. Y., Shin, S. B., Choi, W. S., Lee, J. H., Kim, S. J., et al. (2021). Antibiotic resistance in shellfish and major inland pollution sources in the drainage basin of Kamak Bay, Republic of Korea. Environ. Monit. Assess. 193:471. doi: 10.1007/s10661-021-09201-z
Joensen, K. G., Tetzschner, A. M. M., Iguchi, A., Aarestrup, F. M., and Scheutz, F. (2015). Rapid and easy in silico serotyping of Escherichia coli isolates by use of whole-genome sequencing data. J. Clin. Microbiol. 53, 2410–2426. doi: 10.1128/JCM.00008-15
Johansson, M. H. K., Bortolaia, V., Tansirichaiya, S., Aarestrup, F. M., Roberts, A. P., and Petersen, T. N. (2021). Detection of mobile genetic elements associated with antibiotic resistance in Salmonella enterica using a newly developed web tool: MobileElementFinder. J. Antimicrob. Chemother. 76, 101–109. doi: 10.1093/jac/dkaa390
Jolley, K. A., Bliss, C. M., Bennett, J. S., Bratcher, H. B., Brehony, C., Colles, F. M., et al. (2012). Ribosomal multilocus sequence typing: universal characterization of bacteria from domain to strain. Microbiology 158, 1005–1015. doi: 10.1099/mic.0.055459-0
Jørgensen, S. B., Søraas, A. V., Arnesen, L. S., Leegaard, T. M., Sundsfjord, A., and Jenum, P. A. (2017). A comparison of extended spectrum β-lactamase producing Escherichia coli from clinical, recreational water and wastewater samples associated in time and location. PLoS One 12:e018657. doi: 10.1371/journal.pone.0186576
Larsen, M. V., Cosentino, S., Rasmussen, S., Friis, C., Hasman, H., Marvig, R. L., et al. (2012). Multilocus sequence typing of total-genome-sequenced bacteria. J. Clin. Microbiol. 50, 1355–1361. doi: 10.1128/JCM.06094-11
Lee, R. J., and Silk, R. (2013). Sources of variation of Escherichia coli concentrations in bivalve molluscs. J. Water Health 11, 78–83. doi: 10.2166/wh.2012.114
Lemee, L., Dhalluin, A., Pestel-Caron, M., Lemeland, J. F., and Pons, J. L. (2004). Multilocus sequence typing analysis of human and animal Clostridium difficile isolates of various toxigenic types. J. Clin. Microbiol. 42, 2609–2617. doi: 10.1128/JCM.42.6.2609-2617.2004
Liu, S., Feng, J., Pu, J., Xu, X., Lu, S., Yang, J., et al. (2019). Genomic and molecular characterisation of Escherichia marmotae from wild rodents in Qinghai-Tibet plateau as a potential pathogen. Sci. Rep. 9:10619. doi: 10.1038/s41598-019-46831-3
Liu, S., Jin, D., Lan, R., Wang, Y., Meng, Q., Dai, H., et al. (2015). Escherichia marmotae sp. nov., isolated from faeces of Marmota himalayana. Int. J. Syst. Evol. Microbiol. 65, 2130–2134. doi: 10.1099/ijs.0.000228
Magiorakos, A. P., Srinivasan, A., Carey, R. B., Carmeli, Y., Falagas, M. E., Giske, C. G., et al. (2012). Multidrug-resistant, extensively drug-resistant and pandrug-resistant bacteria: an international expert proposal for interim standard definitions for acquired resistance. Clin. Microbiol. Infect. 18, 268–281. doi: 10.1111/j.1469-0691.2011.03570.x
Malberg Tetzschner, A. M., Johnson, J. R., Johnston, B. D., Lund, O., and Scheutzd, F. (2020). In silico genotyping of Escherichia coli isolates for extraintestinal virulence genes by use of whole-genome sequencing data. J. Clin. Microbiol. 58, e01269–e01220. doi: 10.1128/JCM.01269-20
Miotto, M., Ossai, S. A., Meredith, J. E., Barretta, C., Kist, A., Prudencio, E. S., et al. (2019). Genotypic and phenotypic characterization of Escherichia coli isolated from mollusks in Brazil and the United States. Microbiology 8:e00738. doi: 10.1002/mbo3.738
Nesporova, K., Wyrsch, E. R., Valcek, A., Bitar, I., Chaw, K., Harris, P., et al. (2021). Escherichia coli sequence type 457 is an emerging extended-spectrum-β-lactam-resistant lineage with reservoirs in wildlife and food-producing animals. Antimicrob. Agents Chemother. 65:e01118. doi: 10.1128/AAC.01118-20
Nicolas-Chanoine, M. H., Bertrand, X., and Madec, J. Y. (2014). Escherichia coli ST131, an intriguing clonal group. Clin. Microbiol. Rev. 27, 543–574. doi: 10.1128/CMR.00125-13
O’Neill, J. (2016). Tackling drug-resistant infections globally: Final report and recommendations. The review on antimicrobial resistance. Available at: https://www.amr-review.org/sites/default/files/160518_Finalpaper_withcover.pdf.
Peirano, G., Mulvey, G. L., Armstrong, G. D., and Pitout, J. D. D. (2013). Virulence potential and adherence properties of Escherichia coli that produce CTX-M and NDM β-lactamases. J. Med. Microbiol. 62, 525–530. doi: 10.1099/jmm.0.048983-0
Peirano, G., and Pitout, J. D. D. (2019). Extended-spectrum β-lactamase-producing Enterobacteriaceae: update on molecular epidemiology and treatment options. Drugs 79, 1529–1541. doi: 10.1007/s40265-019-01180-3
Rees, E. E., Davidson, J., Fairbrother, J. M., St Hilaire, S., Saab, M., and McClure, J. T. (2015). Occurrence and antimicrobial resistance of Escherichia coli in oysters and mussels from Atlantic Canada. Foodborne Pathog. Dis. 12, 164–169. doi: 10.1089/fpd.2014.1840
Robertson, J., and Nash, J. H. E. (2018). MOB-suite: software tools for clustering, reconstruction and typing of plasmids from draft assemblies. Microb. Genom. 4:e0002. doi: 10.1099/mgen.0.000206
Russo, T. A., and Johnson, J. R. (2000). Proposal for a new inclusive designation for extraintestinal pathogenic isolates of Escherichia coli: ExPEC. J. Infect. Dis. 181, 1753–1754. doi: 10.1086/315418
Sekizuka, T., Tanaka, R., Hashino, M., Yatsu, K., and Kuroda, M. (2022). Comprehensive genome and plasmidome analysis of antimicrobial resistant bacteria in wastewater treatment plant effluent of Tokyo. Antibiotics 11:1283. doi: 10.3390/antibiotics11101283
Sivertsen, A., Dyrhovden, R., Tellevik, M. G., Bruvold, T. S., Nybakken, E., Skutlaberg, D. H., et al. (2022). Escherichia marmotae–a human pathogen easily misidentified as Escherichia coli. Microbiol. Spectr. 10:e020352. doi: 10.1128/spectrum.02035-21
Sola, M., Mani, Y., Saras, E., Drapeau, A., Grami, R., Aouni, M., et al. (2022). Prevalence and characterization of extended-spectrum β-lactamase-and carbapenemase-producing Enterobacterales from Tunisian seafood. Microorganisms 10:1364. doi: 10.3390/microorganisms10071364
Tacconelli, E., Carrara, E., Savoldi, A., Harbarth, S., Mendelson, M., Monnet, D. L., et al. (2018). Discovery, research, and development of new antibiotics: the WHO priority list of antibiotic-resistant bacteria and tuberculosis. Lancet Infect. Dis. 18, 318–327. doi: 10.1016/S1473-3099(17)30753-3
Van der Putten, B. C. L., Matamoros, S., Mende, D. R., Scholl, E. R., and Schultsz, C. (2021). Escherichia ruysiae sp. nov., a novel gram-stain-negative bacterium, isolated from a faecal sample of an international traveller. Int. J. Syst. Evol. Microbiol. 71:004609. doi: 10.1099/ijsem.0.004609
Vignaroli, C., Di Sante, L., Leoni, F., Chierichetti, S., Ottaviani, D., Citterio, B., et al. (2016). Multidrug-resistant and epidemic clones of Escherichia coli from natural beds of Venus clam. Food Microbiol. 59, 1–6. doi: 10.1016/j.fm.2016.05.003
Vu, T. T. T., Alter, T., Roesler, U., Roschanski, N., and Huehn, S. (2018). Investigation of extended-spectrum and AmpC β -lactamase-producing Enterobacteriaceae from retail seafood in Berlin, Germany. J. Food Prot. 81, 1079–1086. doi: 10.4315/0362-028X.JFP-18-029
Walk, S. T., Alm, E. W., Gordon, D. M., Ram, J. L., Toranzos, G. A., Tiedje, J. M., et al. (2009). Cryptic lineages of the genus Escherichia. Appl. Environ. Microbiol. 75, 6534–6544. doi: 10.1128/AEM.01262-09
Wirth, T., Falush, D., Lan, R., Colles, F., Mensa, P., Wieler, L. H., et al. (2006). Sex and virulence in Escherichia coli: an evolutionary perspective. Mol. Microbiol. 60, 1136–1151. doi: 10.1111/j.1365-2958.2006.05172.x
World Health Organization. (2019). Critically important antimicrobials for human medicine, 6th revision. Available at: https://www.who.int/publications/i/item/9789241515528.
World Health Organization. (2021). Global antimicrobial resistance and use surveillance system (GLASS) report 2021. Available at: https://apps.who.int/iris/rest/bitstreams/1350455/retrieve.
Zankari, E., Allesøe, R., Joensen, K. G., Cavaco, L. M., Lund, O., and Aarestrup, F. M. (2017). PointFinder: a novel web tool for WGS-based detection of antimicrobial resistance associated with chromosomal point mutations in bacterial pathogens. J. Antimicrob. Chemother. 72, 2764–2768. doi: 10.1093/jac/dkx217
Keywords: bivalves, Escherichia coli, ESBL, antimicrobial resistance, CTX-M, clam, Escherichia ruysiae, Escherichia marmotae
Citation: Leoni F, Sacchini L, Pieralisi S, Angelico G, Magistrali CF, Cucco L, Massacci FR, Albini E, Duranti A, Cammà C, Secondini B, Rinaldi A and Barchiesi F (2023) Occurrence and temporal distribution of extended-spectrum β-lactamase-producing Escherichia coli in clams from the Central Adriatic, Italy. Front. Microbiol. 14:1219008. doi: 10.3389/fmicb.2023.1219008
Edited by:
Benoit Doublet, Institut National de Recherche Pour L’agriculture, L’alimentation et L’environnement (INRAE), FranceReviewed by:
Agnese Lupo, Unité Antibiorésistance et Virulence Bactériennes, ANSES Site de Lyon, FranceWejdene Mansour, University of Sousse, Tunisia
Copyright © 2023 Leoni, Sacchini, Pieralisi, Angelico, Magistrali, Cucco, Massacci, Albini, Duranti, Cammà, Secondini, Rinaldi and Barchiesi. This is an open-access article distributed under the terms of the Creative Commons Attribution License (CC BY). The use, distribution or reproduction in other forums is permitted, provided the original author(s) and the copyright owner(s) are credited and that the original publication in this journal is cited, in accordance with accepted academic practice. No use, distribution or reproduction is permitted which does not comply with these terms.
*Correspondence: Francesca Leoni, Zi5sZW9uaUBpenN1bS5pdA==