- 1Health Program, International Livestock Research Institute, Nairobi, Kenya
- 2Department of Biochemistry, Biotechnology and Microbiology, Kenyatta University, Nairobi, Kenya
- 3Institute of Infection, Veterinary and Ecological Sciences, University of Liverpool, Liverpool, United Kingdom
- 4Department of Veterinary and Animal Sciences, University of Copenhagen, Frederiksberg C, Denmark
- 5Department of Disease Control, London School of Hygiene and Tropical Medicine, London, United Kingdom
Introduction: Antimicrobial resistance (AMR) is a global health priority. This systematic review summarizes the prevalence of AMR in enteric pathogens originating from the community, specifically among ≤10-year-old children in low-and middle-income countries (LMICs). In addition, it presents the proportions of pooled resistance in Campylobacter spp., Escherichia coli, Shigella spp., and Salmonella spp. (CESS) to clinically relevant antibiotics.
Methods: Six online repositories, namely PubMed, Medline, Web of Science, Cochrane Library, CABI, and EMBASE were searched for articles published between January 2005 and September 2024. Random-effects meta-analysis models were constructed to estimate the pooled AMR proportions for CESS pathogens, and a subgroup analysis by region was also carried out.
Results: A total of 64 publications from 23 LMICs met our inclusion criteria. The pooled estimates of E. coli AMR for clinically important antibiotics were as follows: sulfamethoxazole/trimethoprim (SXT) 71% [95%CI: 57–82%]; ampicillin (AMP) 56% [95%CI: 44–67%]; ciprofloxacin (CIP) 10% [95%CI: 5–20%]; and ceftriaxone (CRO) 8% [95%CI: 2–31%]. The proportions of AMR detected in Shigella spp. were AMP 76% [95%CI: 60–87%]; nalidixic acid (NA) 9% [95%CI: 2–31%]; CIP 3% [95%CI: 0–15%]; and CRO 2% [95%CI: 0–19%]. The proportions of Salmonella spp. AMR were AMP 55% [95%CI: 35–73%] and SXT 25% [95%CI: 15–38%]. The proportions of Campylobacter spp. AMR were erythromycin (ERY) 33% [95%CI: 12–64%] and CIP 27% [95%CI: 8–61%]. There was high variability in the regional subgroup analysis, with high interstudy and regional heterogeneity I2 ≥ 75%.
Conclusion: Our results shed light on drug-resistant enteric bacterial pathogens in young children, providing evidence that CESS pathogens are becoming increasingly resistant to clinically important antimicrobials. Regional differences in resistance patterns between these community isolates highlight the need for strong national and regional surveillance to detect regional variations and inform treatment and appropriate antibiotic stewardship programs. The limitations of our findings include high regional variability, significant interstudy heterogeneity, and underrepresentation of certain LMICs.
Systematic review registration: https://inplasy.com/inplasy-2024-2-0051/, registration number: INPLASY202420051.
1 Introduction
Antimicrobial resistance (AMR) is a significant threat to global health, contributing to an estimated 1.27 million deaths worldwide in 2019, with the highest mortality rates reported in sub-Saharan Africa and South Asia (Barber and Sutherland, 2017; Murray et al., 2022). AMR aggravates health complications and increases the risk of mortality and economic burden undermining childhood survival in low- and middle-income countries (LMICs) (Huynh et al., 2015; Sulis et al., 2022). LMICs are often characterized by limited access to healthcare, inadequate sanitation and hygiene, and a high burden of infectious diseases, such as pneumonia, diarrhea, and malaria (Barber and Sutherland, 2017; Hendriksen et al., 2019), driving both prophylactic and therapeutic antimicrobial use (Omulo et al., 2015).
A key factor in addressing the challenge of AMR is surveillance (World Health Organization, 2017a). Community AMR surveillance involves monitoring the prevalence and patterns of AMR in community to facilitate early detection of resistant strains and allow timely interventions to prevent further spread (Kasolo et al., 2013). However, comprehensive population-based AMR surveillance data are lacking in both LMICs and high-income countries (HICs), thus casting doubt on the accuracy of global estimates of AMR burden (Gandra et al., 2020). LMICs that have developed national action plans for AMR often rely on fragmented and non-representative surveillance data from larger urban hospitals, with obvious gaps in human population- or community-based AMR data; thus, their usefulness to healthcare policymakers is limited (Otto et al., 2022; Iskandar et al., 2021). High-quality AMR surveillance data help monitor treatment guidelines by assessing the effectiveness of current recommendations and making necessary adjustments. Through surveillance, high-risk areas or vulnerable populations such as children can be identified in order to guide the implementation of infection control to prevent the spread of resistant strains.
Variability has been reported in the capability to conduct AMR surveillance in low-resource settings due to challenges such as weak laboratory infrastructure, limited resources, overreliance on donor funding, and a lack of qualified staff and training (Iskandar et al., 2021). The Global Antimicrobial Resistance Surveillance System (GLASS) has endeavored to support evidence-based and standardized surveillance worldwide (Gandra et al., 2020). Following this GLASS initiative, national AMR surveillance systems have been established in some South and Southeast Asian countries, including Bangladesh, Cambodia, India, Laos, Nepal, Pakistan, Thailand, and Vietnam (Gandra et al., 2020; World Health Organization, 2017b; Aggarwal et al., 2012; World Health Organization, 2016). Although GLASS initiatives support standardized global surveillance, reports on community circulation of bacteria with AMR remain limited, particularly in Africa, where only 23 of 54 countries have implemented national AMR systems (Okolie et al., 2022).
Previous reviews have focused on the burden associated with AMR in healthcare-acquired infections (HAIs), reporting a higher burden in LMICs than in HICs (Ayobami et al., 2022; Allegranzi et al., 2011). However, there is a paucity of comprehensive data on the prevalence and distribution of AMR in community-acquired enteric bacteria, especially in vulnerable ≤10-year-old children in resource-limited settings. Enteric pathogens, namely E. coli, Salmonella, Shigella, and Campylobacter, were investigated in this review since they are among the predominant bacterial causes of diarrhea in children (CDC, 2022; Guarino et al., 2018). Diarrhea remains the second leading cause of infant mortality, preceded by pneumonia (Liu et al., 2012). In LMICs, inappropriate use of antimicrobials to manage diarrhea and other childhood diseases in community settings contributes to AMR (Farthing et al., 2013). Antimicrobial use not only alters the gut microbiome but also selects for bacteria with AMR, turning innocuous gut commensals into reservoirs of AMR determinants that can be transmitted to pathogens or across epidemiological compartments through prolonged fecal shedding (World Health Organization, 2005b). Due to its abundance in the gut, ease of isolation, widespread distribution, and significant genetic diversity, E. coli has been used as an indicator of overall AMR patterns within community, providing insights into the prevalence and distribution of resistance.
Thus, this review addresses critical gaps in the literature by estimating pooled proportions of AMR in clinically important, community-acquired enteric bacteria in ≤10-year-old children across LMICs. By analyzing resistance patterns across geographical regions, we aim to identify the gaps in AMR surveillance and highlight areas where action is required to mitigate the burden of AMR in resource-limited settings.
2 Methods
This systematic review and meta-analysis was conducted in accordance with the Preferred Reporting Items for Systematic Reviews and Meta-analyses (PRISMA) guidelines (Figure 1) and in compliance with the PRISMA checklist (Supplementary Table 1) (Shamseer et al., 2015). The study protocol was registered on INPLASY (INPLASY202420051) to ensure full compliance with best practices in research transparency and protocol registration.
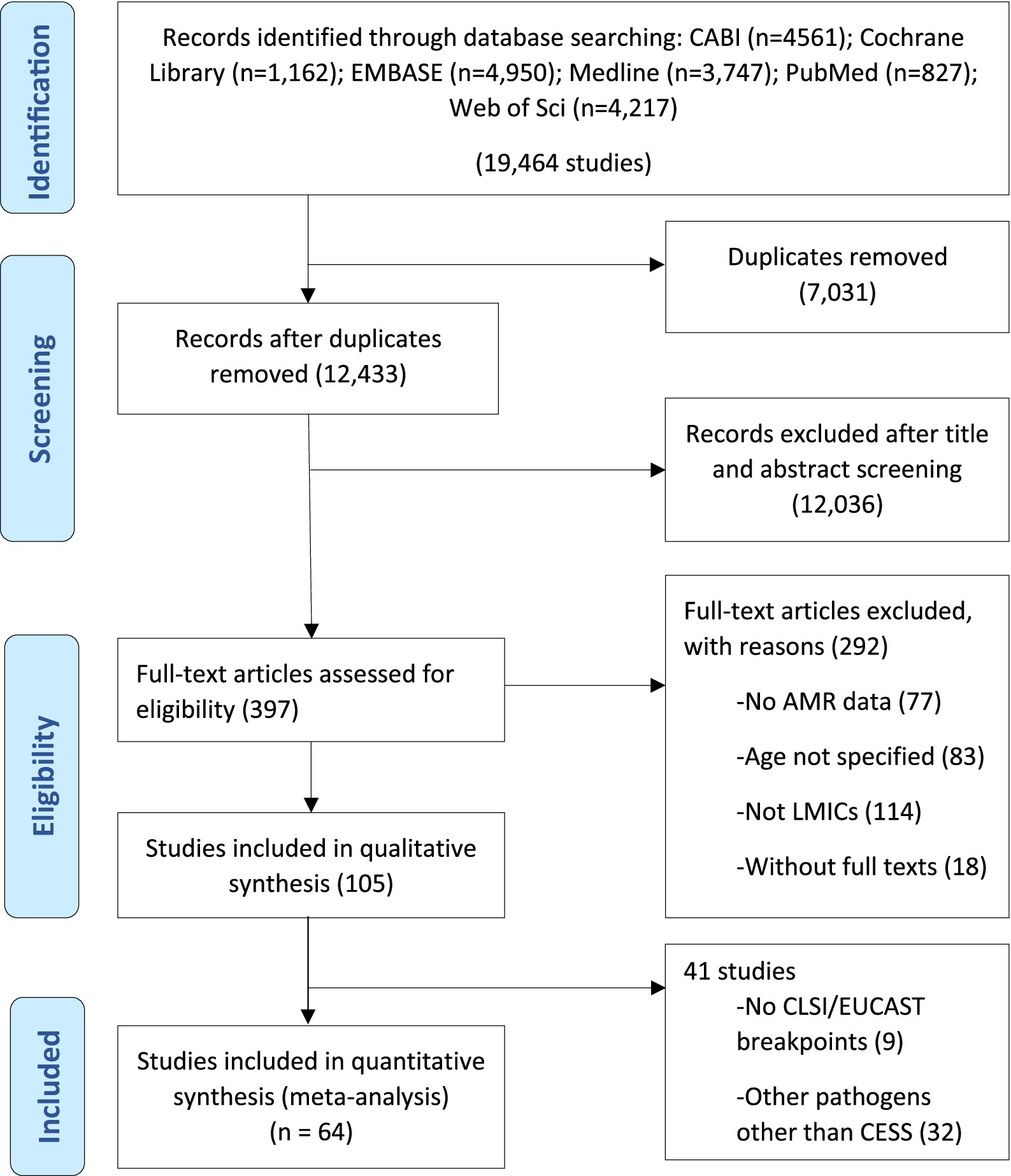
Figure 1. Systematic selection for inclusion of studies on community-acquired antimicrobial resistance in children in low- and middle-income countries.
2.1 Search strategy and selection criteria
As defined by the World Bank, various combinations of search terms related to child descriptors, AMR, and LMICs were used to search numerous databases, including PubMed, Medline, Web of Science, Cochrane Library, CABI, and EMBASE (World Bank, 2019). The initial database searches were conducted between July 14 and August 5, 2020, and revised searches were performed between September 20 and September 22, 2024. The complete search strategy is provided in Supplementary File 1. Articles were screened for eligibility using the following inclusion criteria: (1) original articles published or accepted in a peer-reviewed journal between January 2005 and September 2024; (2) participants (≤10-year-old children) residing in an LMIC; (3) cross-sectional or longitudinal design with baseline data; and (4) having measured AMR in bacterial isolates obtained from children in non-clinical or outpatient settings or from samples collected within 48 h of hospital admission, to minimize the inclusion of healthcare-acquired infections. The year 2005 was purposefully selected because it was the year the World Health Organization began emphasizing AMR as a critical public health challenge (World Health Organization, 2005b). Studies published since 2005 are more likely to reflect contemporary diagnostic, treatment, and surveillance practices, making them more relevant to our research objectives. Including data up to September 2024 ensured that the review captures the most up-to-date evidence, reflecting the current status of AMR in community-acquired enteric pathogens, particularly in LMICs. Case reports and review papers were excluded. Non-English articles were translated using Google Translate, and key sections (Methods and Results) were cross-checked for accuracy. The identified articles were shared among four co-authors (NO, LO, AK, and LA) who independently screened the titles and abstracts and reviewed the full texts of potentially relevant articles. Disagreements between the reviewers were resolved through consensus.
2.2 Data extraction
Data were extracted by the same four independent reviewers (NO, LO, AK, and LA) using a predefined and standardized data extraction form. The extracted information included (1) year of publication, (2) first author’s name, (3) study location (country/region), (4) study setting (hospital, community, or both), (5) year of sample collection, (6) participant demographics (age and sex) and health status, (7) sample size, (8) sample type, (9) bacterial species isolated, (10) number of isolates, (11) study design, (12) antimicrobial susceptibility testing (AST) method, and (13) prevalence of AMR (i.e., the number of resistant isolates). The quality of the articles included in the review was assessed using the Newcastle Ottawa Quality Assessment Scale (NOS) adapted for cross-sectional study design (Feeley, 2020). This assessment was conducted using three parameters: the selection of the sample, the comparability of the tests, and the level of exposure. The selection parameter was based on the representativeness of the sample, the definition of the community, and the sample size. The comparability parameter was based on the bacterial isolation and identification method and the AST method. The exposure parameter was based on the number of AMR isolates. A study with an overall score of ≥7 out of 10 was considered to be of good quality and was included in the analysis to obtain a revised pooled estimate.
2.3 Statistical analysis
The primary outcome of our analysis was the proportion of bacterial isolates that showed resistance to an antimicrobial. We examined the prevalence of AMR in different enteric bacterial species, such as E. coli, Shigella spp., Salmonella spp., Campylobacter spp., Vibrio cholerae, and Enterococcus spp. However, the meta-analysis focused on the four most reported enteric bacterial pathogens: Campylobacter spp., E. coli, Shigella spp., and Salmonella spp. (hereafter referred to as CESS). The isolate–drug combinations included drugs commonly reported in the articles and indicated for first- or second-line empiric treatment. These drugs included ciprofloxacin (CIP), gentamicin (CN), sulfamethoxazole/trimethoprim (SXT), ceftriaxone (CRO), ampicillin (AMP), amoxicillin/clavulanate (AMC), nalidixic acid (NA), azithromycin (AZM), erythromycin (ERY), and tetracycline (TET).
All data analyses were performed using R (version 4.2.2). Meta-analyses of the proportions of the tested bacteria resistant to each antimicrobial were conducted using the “metaprop” function of the “dmetar” and “meta” packages. Meta-analyses were stratified by isolate–drug combination and geographical region (n ≥ 2 studies). Due to the high levels of heterogeneity (I2 > 75%) between studies, a random-effects model was used to pool effect sizes (i.e., pooled proportion of pathogens resistant to a particular antimicrobial). Random-effects meta-analysis is a statistical approach that accounts for variability between studies and provides a more generalized effect estimate across different populations. AMR proportions were pooled using a generalized linear mixed-effects model (GLMM) with logit-transformed proportions, using the following equation:
represents the pooled proportion estimate, which is the combined estimate of the proportion of the resistance of E. coli to an antibiotic (e.g., AMP) across all included studies;
refers to the number of studies included in the meta-analysis, each study contributing an estimate of the proportion of resistance;
is the estimated proportion of the resistance of E. coli to AMP in study . It is calculated by dividing the number of resistant isolates by the total number of isolates in study ; and.
represents the weight assigned to study . In GLMM, weights are derived from the estimated variances of the proportion estimates, considering both within-study and between-study variability.
The GLMM incorporates both fixed and random effects to model the variability in proportion estimates across studies. It allows for the estimation of study-specific effects (fixed effects) and the overall pooled effect (random effects). Using Equation 1, a weighted sum of the individual study proportion estimates is calculated, where each estimate is weighted by its corresponding weight . The weights are derived from the GLMM framework, which accounts for the heterogeneity between studies. The denominator is the sum of weights, ensuring that is appropriately scaled.
Variability between studies was assessed through heterogeneity tests using the I2 statistic, which was calculated using the “dmetar” package (Smellie, 2006). An I2 index greater than 50% indicated moderate to substantial heterogeneity (Higgins and Thompson, 2002). Influence analysis was conducted to identify the impact of outliers on the overall effect estimate, following the approach outlined by Viechtbauer et al. (2015). Outliers have extremely small and large effects that significantly differ from the overall effect based on 95% CI. To find and remove outlier studies and to measure the pooled effect size without them, the “find.outliers” function was used. Furthermore, Egger’s regression test for funnel plot asymmetry was performed to measure publication bias using the following equation:
3 Results
Electronic searches yielded 19,464 publications, which was reduced to 12,433 after the removal of duplicates (Figure 1). After screening and exclusion, 105 publications met our inclusion criteria. Out of these 105 publications, 64 provided data on the four most commonly reported enteric bacteria (E. coli, Shigella, Salmonella, and Campylobacter) and were included in the meta-analyses (Figure 1). Each individual bacterial species reported in these 64 articles was treated as a unique study. Therefore, this resulted in a total of 93 studies (representing 8,082 isolates). Among these 93 studies, 44 focused on E. coli (n = 5,941 isolates), 23 on Shigella (n = 836 isolates), 18 on Salmonella (n = 690 isolates), and 8 on Campylobacter (n = 615 isolates). Sub-Saharan Africa had the highest number of eligible studies (n = 37), followed by Asia (n = 31), North Africa/Middle East (n = 14), Central and South America (n = 10), and North America (n = 1). Ethiopia had the highest number of eligible studies (n = 18), followed by Kenya (n = 11) and Iran (n = 10) (Figure 2). A summary of the characteristics of the included studies is presented in Table 1.
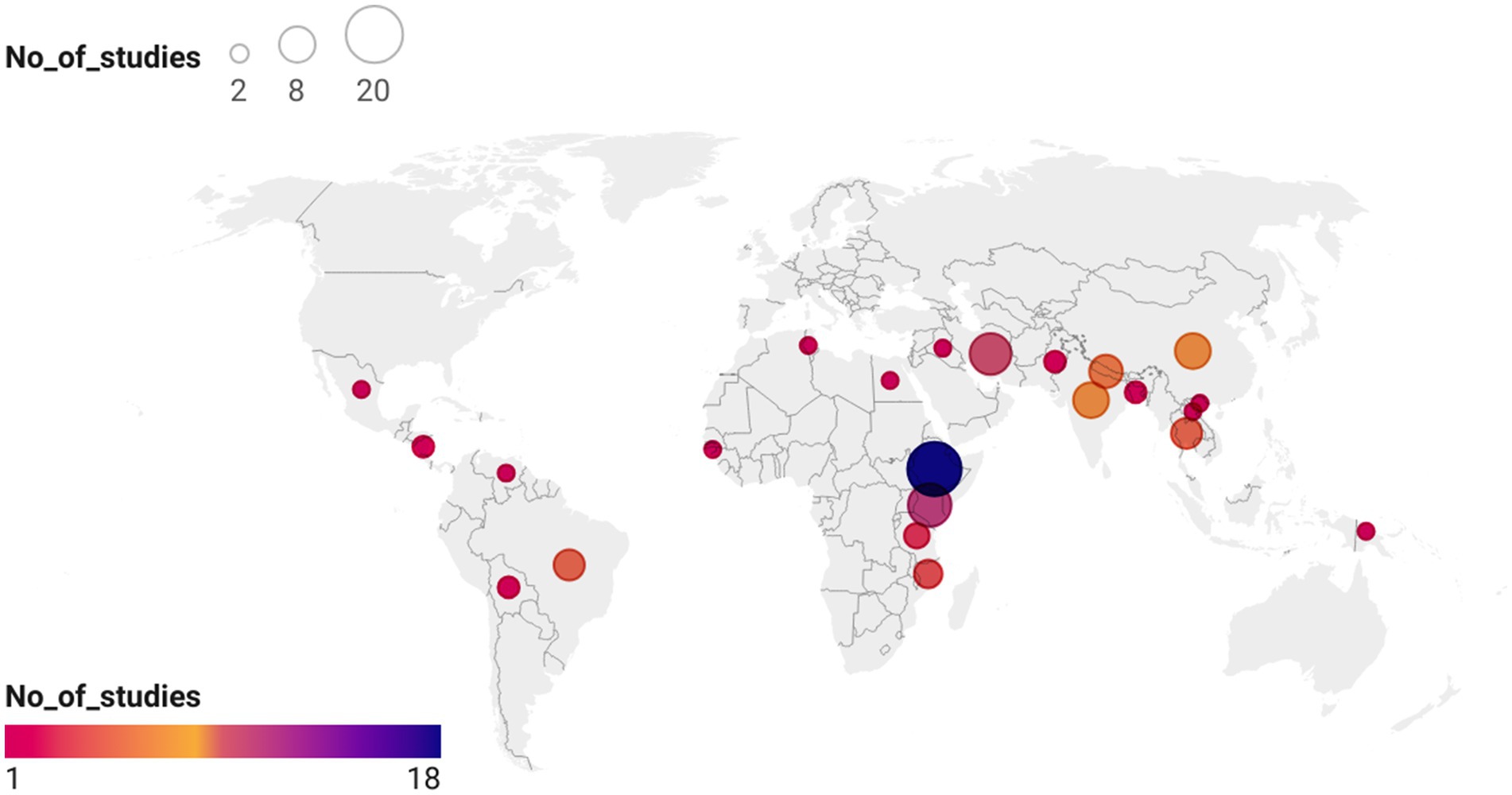
Figure 2. Locations by the country of 64 publications included in this systematic review. The basemap of the world was created using datawrapper: https://datawrapper.dwcdn.net/p5txr/1/. The basemap shape file for the world was downloaded from https://www.datawrapper.de/maps/choropleth-map.
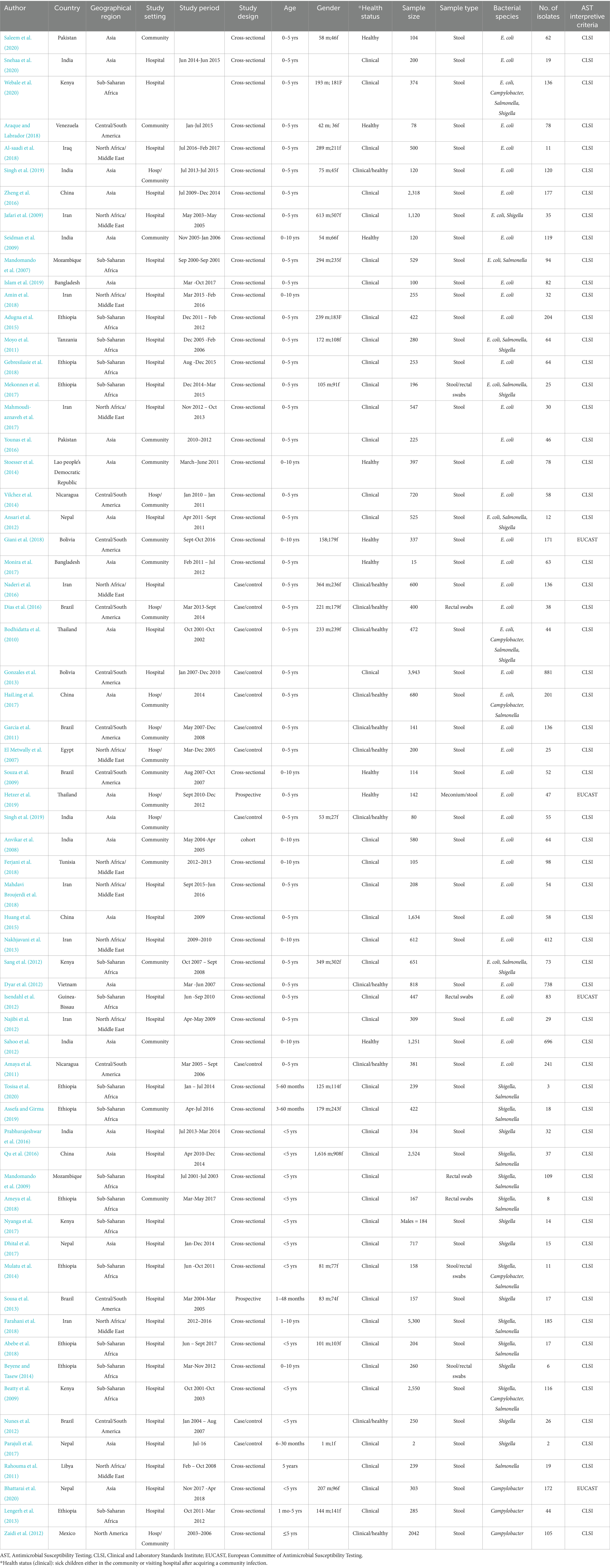
Table 1. Characteristics of 64 articles included in the meta-analysis of community-acquired AMR in under-10-year-old children in low- and middle-income countries.
The 64 selected publications included observational studies comprising 50 cross-sectional, 11 case–control, and 3 cohort studies. The antimicrobials tested against these bacteria were clinically relevant and included AMP, AMC, TET, SXT, CIP, NA, CN, CRO, and chloramphenicol (C). Only two publications examined all four bacterial species (Bodhidatta et al., 2010; Webale et al., 2020), while seven publications examined three of the four species (Moyo et al., 2011; Mekonnen et al., 2017; Ansari et al., 2012; HaiLing et al., 2017; Sang et al., 2012; Mulatu et al., 2014; Beatty et al., 2009). In addition, nine publications examined two bacterial species each (Jafari et al., 2009; Mandomando et al., 2007; Tosisa et al., 2020; Assefa and Girma, 2019; Qu et al., 2016; Mandomando et al., 2009; Ameya et al., 2018; Farahani et al., 2018; Abebe et al., 2018), while the remaining 46 publications examined only one pathogen (Singh et al., 2019; Anvikar et al., 2008; Mahdavi Broujerdi et al., 2018; Huang et al., 2015; Nakhjavani et al., 2013; Saleem et al., 2020; Snehaa et al., 2020; Araque and Labrador, 2018; Al-Saadi et al., 2018; Zheng et al., 2016; Seidman et al., 2009; Islam et al., 2019; Amin et al., 2018; Adugna et al., 2015; GebreSilasie et al., 2018; Mahmoudi-aznaveh et al., 2017; Younas et al., 2016; Stoesser et al., 2014; Vilchez et al., 2014; Giani et al., 2018; Monira et al., 2017; Naderi et al., 2016; Dias et al., 2016; Gonzales et al., 2013; Garcia et al., 2011; El Metwally et al., 2007; Souza et al., 2009; Hetzer et al., 2019; Dyar et al., 2012; Isendahl et al., 2012; Najibi et al., 2012; Sahoo et al., 2012; Amaya et al., 2011; Prabhurajeshwar et al., 2016; Nyanga et al., 2017; Dhital et al., 2017; Sousa et al., 2013; Beyene and Tasew, 2014; Nunes et al., 2012; Parajuli et al., 2017; Rahouma et al., 2011; Bhattarai et al., 2020; Lengerh et al., 2013; Zaidi et al., 2012; Singh et al., 2019; Ferjani et al., 2018). A comprehensive overview of meta-analysis results is presented in Supplementary Table 2.
3.1 Escherichia coli
Our meta-analysis identified high levels of E. coli resistance to key antibiotics, with notable regional variations. Resistance to AMP was the highest overall, with a pooled proportion of 71%, reaching 89% in North Africa/Middle East. Substantial resistance to SXT (56%) and TET (51%) was observed globally, while resistance to CIP (10%) and CRO (8%) remained low but showed regional hotspots, particularly in North Africa/Middle East and Asia.
There were 32 studies with a total of 4,425 E. coli isolates that reported resistance to AMP. The overall pooled proportion of AMP resistance was 71%, which increased to 75% after the removal of 11 outlier studies (Figure 3).
Regional subgroup analyses revealed a pooled AMP resistance proportion of 89% for North Africa/Middle East, 81% for sub-Saharan Africa, 71% for Asia, and 52% for Central and South America (Figure 4A). There were 33 studies with a total of 5,198 E. coli isolates studied for resistance to SXT. The pooled SXT resistance proportion was 56%, which decreased to 54% after the removal of 14 outlier studies. Regional subgroup analyses revealed a pooled SXT resistance proportion of 73% for sub-Saharan Africa, 57% for North Africa/Middle East, 50% for Asia, and 48% for Central and South America (Figure 4B). Although CIP resistance was lower overall (10%), it was notably higher in North Africa/Middle East (20%) than in other regions, emphasizing the need for targeted interventions in these areas (Figure 4C). There were 18 studies with a total of 1,865 E. coli isolates studied for resistance to CRO. The pooled CRO resistance proportion was 8%, which decreased to 4% after the removal of three outlier studies. The pooled CRO resistance proportion was 18% in Asia, 13% in North Africa/Middle East, 5% in sub-Saharan Africa, and 1% in Central and South America (Figure 4D). A total of 21 studies investigated 2,080 E. coli isolates studied for resistance to AMC, reporting a pooled resistance proportion of 20%, which decreased to 17% after 10 outliers were removed. Regional subgroup analysis revealed moderate resistance to AMC, with the highest prevalence in North Africa/Middle East (51%) and the lowest in Asia (7%). Detailed statistics are provided in Supplementary Figure 1A. CN resistance was investigated by 33 studies covering 3,111 E. coli isolates. The pooled CN resistance proportion was 12%, which decreased to 10% after the removal of 13 outliers. Subgroup analysis showed a CN resistance of 22% among Asian isolates, while CN resistance of E. coli isolates from sub-Saharan Africa, North Africa/Middle East, and Central and South America was 18, 8, and 2%, respectively (Supplementary Figure 1B). There were 28 studies with a total of 4,658 E. coli isolates investigated for TET resistance. The pooled TET resistance proportion was 51%, which increased to 54% after the removal of nine outliers. Regional subgroup analysis revealed that E. coli showed a higher TET resistance in Asia (56%) than in North Africa/Middle East (51%), sub-Saharan Africa (49%), and Central and South America (39%) (Supplementary Figure 1C).
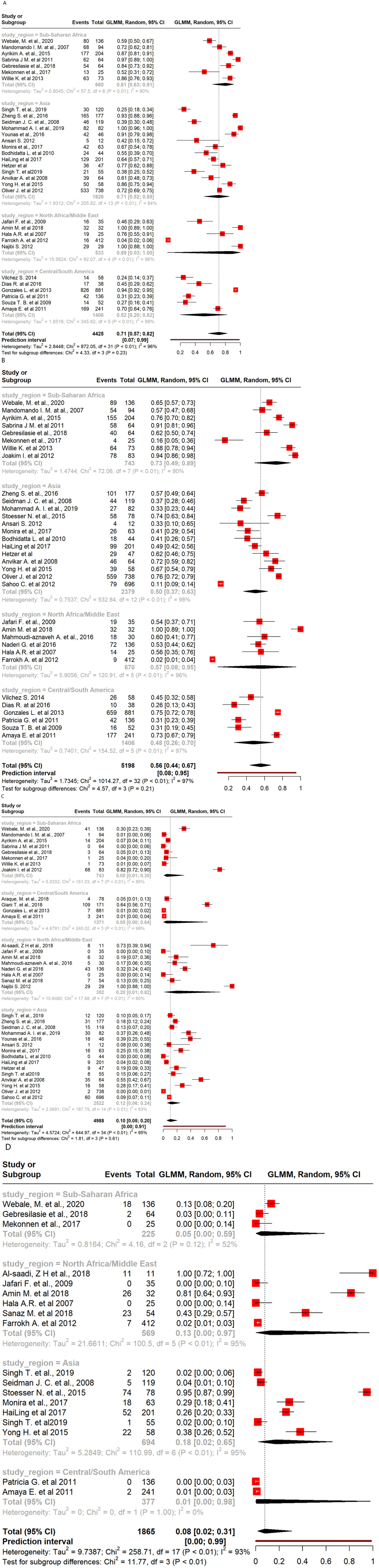
Figure 4. Forest plot of E. coli antimicrobial resistance across regions: (A) ampicillin; (B) sulfamethoxazole/trimethoprim; (C) ciprofloxacin; and (D) ceftriaxone.
3.2 Shigella
Meta-analysis of Shigella isolates revealed large regional differences in resistance to the tested antibiotics. Resistance to AMP was highest overall with a pooled estimate of 76%, with the highest proportion in North Africa/Middle East (85%) followed by sub-Saharan Africa (79%). Resistance to CIP, CRO, and NA was low overall (<10%), but higher in specific regions, such as Asia for CIP (28%) and NA (53%).
There were 20 studies with a total of 525 Shigella isolates that reported resistance to CIP. The overall pooled proportion of CIP resistance was 3% (Figure 3), which decreased to 2% after the removal of three outlier studies (Figure 3). Regional subgroup AMR analysis showed a CIP resistance of 28% in Asia and 3% in sub-Saharan Africa (Figure 5A). There were 16 studies that analyzed 635 Shigella isolates for resistance to NA. The overall pooled NA resistance proportion was 9%, which decreased to 5% when three outliers were removed (Supplementary Table 2). Regional subgroup analysis showed higher resistance estimates for Asian isolates (53%) than for those from North Africa/Middle East (11%) and sub-Saharan Africa (6%) (Figure 5B). There were 20 studies that analyzed 771 isolates for AMP resistance. The pooled estimate proportion for AMP resistance was 76%, which remained the same after three outliers were removed. Regional subgroup analysis showed a pooled AMP resistance proportion of 85% for North Africa/Middle East, 79% for sub-Saharan Africa, and 74% for Asia (Figure 5C). Of the 367 isolates from 11 studies that analyzed CRO resistance, a pooled resistance proportion of 2% was observed, which decreased to 1% after one outlier was removed. Of the 238 isolates investigated from eight studies in sub-Saharan Africa, the pooled proportion for CRO resistance was 4% (Figure 5D). Three studies analyzed 39 isolates showing AZM resistance, resulting in an overall resistance proportion of 29%. No outlier was removed since none was detected as shown in Supplementary Table 2.
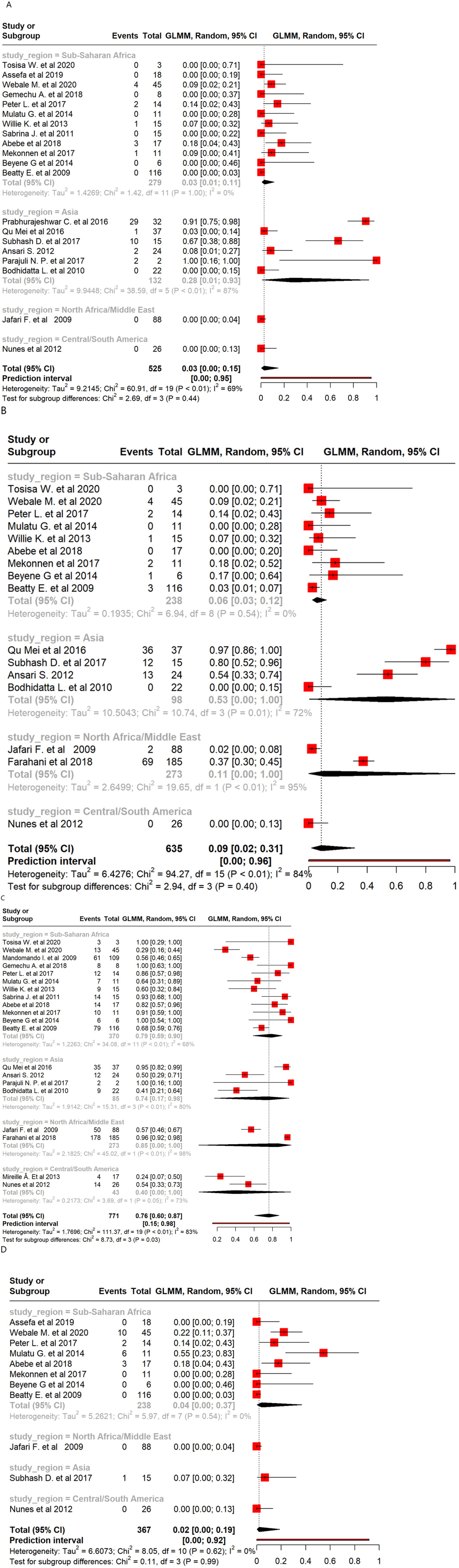
Figure 5. Forest plot of Shigella antimicrobial resistance across regions: (A) ciprofloxacin; (B) nalidixic acid; (C) ampicillin; and (D) ceftriaxone.
3.3 Salmonella
Meta-analysis of resistance shown by Salmonella revealed moderate levels of resistance to the antibiotics and some regional differences. Resistance was highest for AMP (55%) and SXT (25%), with sub-Saharan Africa showing higher resistance than Asia in both cases. C resistance was also more prevalent in sub-Saharan Africa than in Asia. CIP resistance was minimal overall (1%), with no resistance observed in sub-Saharan Africa.
There were 16 studies that analyzed 642 Salmonella isolates for AMP resistance. The overall pooled proportion of AMP resistance was 55% (Figure 3), which increased to 58% after the removal of one outlier study (Figure 3). Regional subgroup analysis showed that sub-Saharan Africa had a higher pooled AMP resistance proportion than Asia (66% vs. 46%) (Figure 6A). Of the 533 isolates from 15 studies that analyzed resistance to SXT, a pooled resistance proportion of 25% was reported, which increased to 26% after the removal of two outlier studies. Regional subgroup analysis showed that sub-Saharan Africa had higher SXT resistance than Asia (33% vs. 20%) (Figure 6B). Of the 457 isolates from 15 studies that analyzed C resistance, a pooled resistance proportion of 23% was reported, which decreased to 21% after one outlier was removed (Supplementary Figure 2A). Regional subgroup analysis showed that sub-Saharan Africa had higher C resistance than Asia (28% vs. 15%). The pooled proportion of CIP resistance was 1% from 15 studies that analyzed 461 isolates. When six outliers were removed, 100% CIP susceptibility was found, as shown in Supplementary Table 2. Regional subgroup analysis showed 2% resistance in Asian isolates and 100% susceptibility among sub-Saharan African isolates (Supplementary Figure 2B).
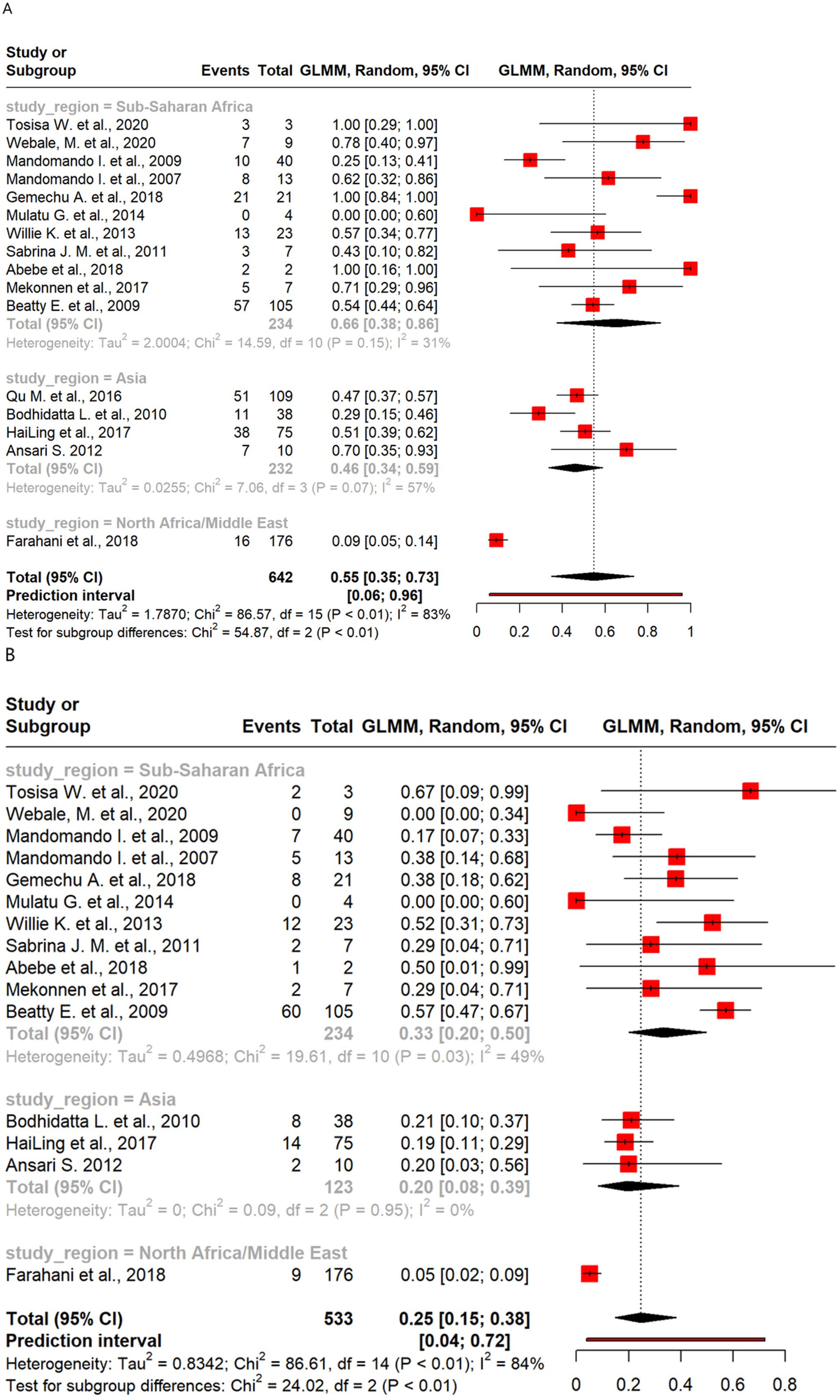
Figure 6. Forest plot of Salmonella antimicrobial resistance across regions: (A) ampicillin and (B) sulfamethoxazole/trimethoprim.
3.4 Campylobacter
Meta-analysis of resistance shown by Campylobacter to the antibiotics highlighted notable regional differences, with higher ERY resistance observed in sub-Saharan Africa (44%) than in Asia (37%). CIP resistance was more prevalent in Asia (57%) than in sub-Saharan Africa (9%), while AMP resistance was similar across regions. CN resistance was higher in sub-Saharan Africa (33%) than in Asia (13%).
Of the 489 isolates from seven studies that analyzed ERY resistance, a pooled resistance proportion of 33% was reported, which increased to 35% after the removal of two outlier studies. Studies from sub-Saharan Africa reported higher ERY resistance than those from Asia (44% vs. 37%) (Figure 7A). Eight studies covering 615 isolates that analyzed resistance to CIP reported a pooled resistance estimate of 27%, which decreased to 21% after the removal of one outlier study. Subgroup analysis showed higher resistance in Asia (57%) than in sub-Saharan Africa (9%) (Figures 3, 7B). There were five studies with 368 Campylobacter isolates that analyzed AMP resistance, which reported a pooled AMP resistance proportion of 53%, with no outlier removal. AMP resistance among Asian isolates was 58%, while that among sub-Saharan African isolates was 51% (Supplementary Figure 3A). The pooled proportion of CN resistance across the regions was 13% out of the 489 isolates investigated across seven studies, without outlier removal, with sub-Saharan Africa showing higher resistance than Asia (33% vs. 13%) (Supplementary Figure 3B).
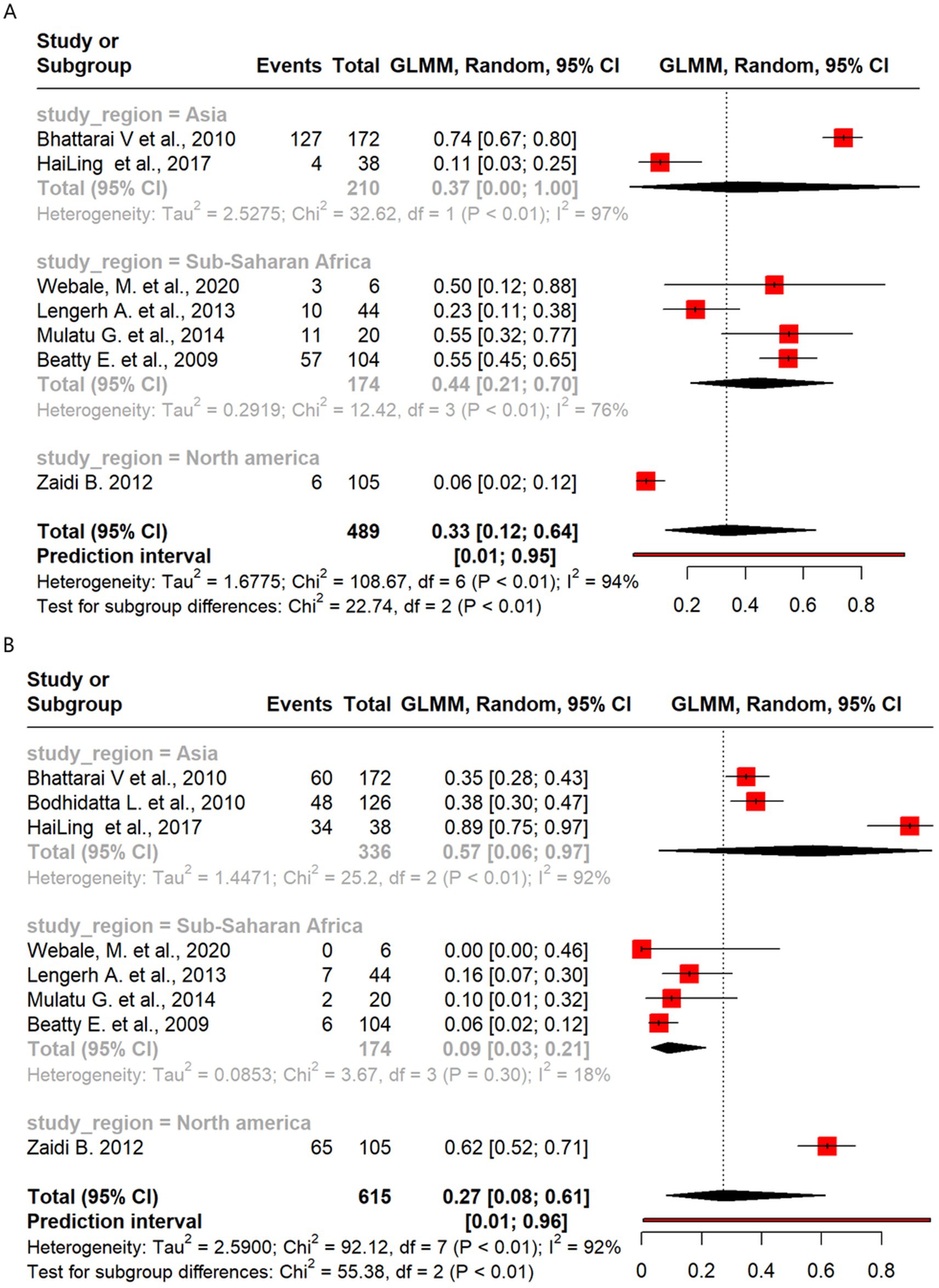
Figure 7. Forest plot of Campylobacter antimicrobial resistance across regions: (A) erythromycin and (B) ciprofloxacin.
In summary, the analysis revealed moderate (I2 > 50%) to high level (I2 > 75%) of heterogeneity between the results, with no evidence of small study effects or publication bias overall (Supplementary Table 3 and Supplementary Figure 4).
4 Discussion
This meta-analysis illustrates the level of pooled AMR in key bacterial enteric pathogens, which may lead to potential treatment failures of certain antimicrobials (Murray et al., 2022).
4.1 Escherichia coli
Our analysis of the 64 studies from LMICs showed that enteric E. coli were resistant to critically important antimicrobials at rates ranging from 8 to 71%. The pooled CRO resistance of 8% shows that these isolates could be Extended-spectrum beta lactamase (ESBL) producers. CRO is a third-generation cephalosporin and therefore infections with this third-generation cephalosporin (3GC)-resistant pathogens would render treatment with AMP or AMC ineffective (Abayneh et al., 2018). CRO is classified as Highest Priority Critically Important Antimicrobial (HPCIA) by WHO, and the resistance of E. coli to this 3GC poses a significant human health challenge (World Health Organization, 2019). Although it is not clear why E. coli shows higher CRO resistance in Asia than in other regions, the use of CRO as an alternative therapy in the treatment of diarrhea where other antibiotics fail has been reported in some developing Asian countries (Gu et al., 2015). This increase in resistance may be associated with the global increase in antibiotic consumption, a 65% rise between 2000 and 2015 (Klein et al., 2018). Notably, four out of six countries with the highest antibiotic consumption rates were LMICs (namely Tunisia, Algeria, Romania, and Turkey). Furthermore, overall antibiotic consumption has increased by 114% in LMICs, led by India, Pakistan, and China. The higher consumption of broad-spectrum penicillins, cephalosporins, quinolones, and macrolides by LMICs than by HICs could contribute to the increased development of resistance, including against antibiotics like CRO (Klein et al., 2018; Wieters et al., 2024). Higher CRO resistance, as reported in clinical E. coli, could therefore be associated with increased usage in clinical settings (Gelaw et al., 2022). ESBL-encoding genes are mostly plasmid borne and circulate in bacterial clones by horizontal transfer. ESBL-E not only circulates among community-onset and hospital infections but has also been reported in more than 60% of healthy children and adults with minimal antimicrobial exposure (Bartoloni et al., 2009). Therefore, fecal E. coli is a useful indicator of the spread of community-acquired AMR genes (Woerther et al., 2013; Zhang et al., 2015; Hijazi et al., 2016; Rolain, 2013; World Health Organization, 2020; United Nations Environment Programme, 2023).
Resistance to AMP among E. coli isolates ranged from 89% in North Africa/Middle East, 81% in sub-Saharan Africa, 71% in Asia, to 62% in Central and South America. This high level of AMP resistance is consistent with reports from various studies conducted in South Africa and Peru investigating AMP resistance in diarrheagenic E. coli (DEC) isolates from affected children (Omolajaiye et al., 2020; Ochoa et al., 2009). The lower AMP resistance observed in Central and South America could be due to prioritizing nutritional therapy and considering antibiotic use only in the treatment of persistent diarrhea (Dias et al., 2016). However, the 93% AMP resistance observed among Bolivian E. coli isolates was associated with inappropriate antimicrobial use (Gonzales et al., 2013). The AMP resistance in this study (like AMC) is concerning since this is the only WHO-recommended pediatric oral antibiotic for outpatient treatment of bacterial infections without access to hospital-based care. However, AMC is not the best option for the treatment of diarrhea as it is largely an oral formulation quickly absorbed in the gut (Okomo et al., 2019). These results on the resistance of E. coli to AMP are consistent with those reported in the review of commensal E. coli isolates collected from healthy community participants of 0–77 years of age in LMICs, indicating that AMR pathogens in healthy people may be acting as AMR reservoirs (Nji et al., 2021). Regional differences in AMP resistance shown by high between-region heterogeneity (I2 > 75%) were statistically significant (p < 0.01), which is reflective of high variability in sample sizes, number of isolates, and resistance prevalence across the included studies.
Resistance patterns across regions showed reduced sensitivity to SXT (56% resistance proportion), which is one of the recommended treatment choices for ESBL-E (Figure 4B). This finding was consistent with the SXT resistance proportions of E. coli reported in a West African review of patients attending a hospital outpatient department (Bernabé et al., 2017). Since SXT is largely used in the treatment of diarrhea, the SXT resistance reported poses a significant health concern in humans (Guarino et al., 2018). The higher SXT resistance reported in Africa than in other regions could be associated with its prophylactic use to reduce morbidity and mortality among HIV-positive patients (Morpeth et al., 2008; Said et al., 2022). TET resistance (54%) is of particular interest since TET is contraindicated in children (Hetzer et al., 2019); therefore, TET resistance in humans may be due to zoonotic transfer from animals and/or co-selection or cross resistance, e.g., through efflux pumps (Nji et al., 2021).
4.2 Shigella
Shigellosis, which is typically self-limiting, sometimes requires antimicrobial therapy to avoid complications, reduce dysenteric discharge, and stop prolonged fecal shedding. CIP, a fluoroquinolone like NA, is a high-priority critically important antibiotic recommended as the first-line treatment of shigellosis, especially in adult patients (WHO, 2005a). In our study, a pooled CIP resistance proportion of 3% out of 525 isolates was identified. Regional subgroup analysis revealed that Shigella isolates from Asia were more resistant to CIP (28% out of 132 isolates) than those from other regions, which ranged from 0 to 3%. The study by The et al. (2016) found that all CIP-resistant Shigella they isolated belonged to one clade, with Asia being the likely primary source. This review confirms that Asian Shigella is more likely to be CIP resistant. This may be due to specific genetic characteristics associated with efflux-pump-mediated fluoroquinolone resistance observed in S. flexneri (Azmi et al., 2014). This also reflects the global trend in Shigella resistance with the emergence of extensively drug-resistant (XDR) Shigella sonnei reported in clinical samples in Europe, where CIP resistance is documented at 42.3%, suggesting global spread (Lefèvre et al., 2023). Other fluoroquinolone-resistant Shigella depict a similar trend as shown by NA-resistant Shigella from Asia in this study (Figure 5B). This observed quinolone resistance may not be directly associated with use since quinolones are not prescribed to children but widely used in veterinary medicine. Thus, fluoroquinolone resistance reported in this review could be spreading to children from older people or foods of animal origin (Hijazi et al., 2016; González and Araque, 2013; Clermont et al., 2000). Due to the emerging CIP resistance, WHO recommends amidinopenicillins (pivmecillinam) or CRO as second-line therapies for CIP-resistant shigellosis (WHO, 2005a; Azmi et al., 2014). CRO efficacy in Shigella infection treatment is shown by the low pooled resistance proportion in this review (2% out of 367 isolates). However, inappropriate use (dose, frequency, or duration) in more than 60% of patients that ever get CRO in Africa could further lead to the emergence and spread of resistance (Bishaw et al., 2021). Due to AMP resistance across regions, as also depicted in this study (76% out of 771 isolates), WHO highlighted AMP as one of the antimicrobials that is inappropriate for shigellosis treatment (WHO, 2005a; Williams and Berkley, 2018).
4.3 Salmonella
Salmonella is an archetypal zoonotic Enterobacterale implicated in self-limiting diarrheal disease in humans, with children aged ≤5 years particularly affected (Park et al., 2021). SXT, C, and AMP are the most used antibiotics in the empiric treatment of salmonellosis. The resistance proportion of Salmonella to SXT, C, and AMP was mostly higher in sub-Saharan African studies (33, 28, and 66%) than in Asian studies (20, 15, and 46%), respectively. The huge increase in antimicrobial consumption in Africa and Asia could be the reason for this reported resistance (Murray et al., 2022; Browne et al., 2021). This could result in the use of other antimicrobials that are not specifically indicated for salmonellosis treatment, such as CIP and CRO. Park et al. (2021) reported the spread of CIP- and CRO-resistant Salmonella typhimurium ST313 and Enteritidis ST11 in several countries in Africa using genotypic methods. They attributed the resistance to the growing use of these antimicrobials to treat febrile illnesses in Africa. However, our study showed low resistance to CIP (1% out of 461 isolates) and CRO (0%), which may be because the review focused on studies reporting lab-based phenotypic AMR profiles of the isolates as opposed to complete AMR genotypes.
4.4 Campylobacter
In this meta-analysis, eight studies analyzed the AMR of Campylobacter from 615 isolates. ERY and CN resistance proportions were higher in sub-Saharan African isolates than in Asian isolates, whereas AMP and CIP resistance proportions were higher in Asian than in sub-Saharan African isolates. In studies that isolated mixed CESS isolates, Campylobacter spp. was the most predominant etiology of resistance to commonly prescribed antimicrobials (Mulatu et al., 2014). Hlashwayo et al. (2021) investigated the resistance of Campylobacter isolated from all age groups in Africa and reported that its resistance to ERY was 43% (vs. 33% in this study), TET was 43% (vs. 43%), CIP was 16% (vs. 27%), NA was 36% (vs. 35%), and CN was 35% (vs. 13%). These resistance patterns are consistent with ours, and inappropriate use of antimicrobials in LMICs could partly explain this phenomenon. TET resistance observed in our study may have included zoonotic transfer from animals and/or co-selection or cross-resistance through efflux pumps and mobile genetic elements, such as transposons (Nji et al., 2021). Campylobacter is commonly isolated from poultry, and the use of CIP and other quinolones in poultry farming is widely practiced (Coulidiaty et al., 2021). Therefore, CIP resistance in these community Campylobacter isolates from children could be due to zoonotic transfer.
The highest AMR proportion among these CESS infections was observed against AMP, with a pooled resistance proportion higher than 50%. This suggests that antimicrobials recommended for empiric treatment in children are losing their efficacy. AMP and CIP resistance proportions are far higher than those of clinical isolates reported in HICs that practice judicious antimicrobial use (AMP 8.2%, CIP 2.1%) but consistent with AMR clinical isolates reported in Ghana (Asare et al., 2022).
This systematic review demonstrates that CRO is largely effective in treating enteric bacterial infections in children. However, the emerging CRO resistance observed in the community isolates of E. coli and Shigella could explain the level of non-efficacy of AMP and AMC across CESS. This could as well be associated with inappropriate CRO use in hospital settings in Africa (Clermont et al., 2000). These resistance patterns are comparable with those reported in hospital settings (Dias et al., 2016; Ochoa et al., 2009). The evident misuse of CRO in African hospitals and CRO and CIP prescriptions for febrile illnesses in LMICs could further increase resistance (Wieters et al., 2024; Bishaw et al., 2021; Park et al., 2021). SXT resistance presented in our study is consistent with those reported in clinical cases of DEC and other enteric bacteria (Bernabé et al., 2017). Although quinolones are not prescribed for pediatric acute gastroenteritis cases, CIP and NA resistance may have resulted due to transmission from older relatives or via foods of animal origin due to common CIP usage in veterinary practice; the same holds for C and TET resistance, where co-selection is a possible phenomenon (Ananchaipattana et al., 2014). The evidence from this review underpins the need to conduct interventions not only in hospital settings but also in community. The current policies with regard to outpatient management of diarrhea in children should focus on appropriate antimicrobial stewardship and alternative interventions such as water, sanitation and hygiene (WASH) programs that could be effective in curbing the emergence and spread of AMR in LMICs (Simiyu et al., 2020; Awiti et al., 2019; Simiyu et al., 2023; Fuhrmeister et al., 2023). However, these approaches should be context specific since they are likely to be influenced by socioeconomic status in LMICs.
Previously published reviews have focused on HAI infections (Ayobami et al., 2022; Allegranzi et al., 2011; Le Doare et al., 2015). They have reported the highest prevalence of Klebsiella pneumonia resistance to AMP (93.8%) and CN (68.8%), with overall Enterobacteriaceae resistance to AMP at 79.6%. Our findings of E. coli AMP resistance across regions (52–89%) are consistent with these reports, highlighting the importance of community surveillance in predicting bacteria with AMR and mitigating the future spread of resistance to clinical settings.
The regional disparities in pooled AMR prevalence estimates observed in this study can be attributed to several factors, including differences in healthcare access, antibiotic usage policies, and regulatory frameworks (Iskandar et al., 2021). Regions with limited healthcare infrastructure, such as parts of sub-Saharan Africa, often experience higher AMR due to over-the-counter access to antibiotics, poor adherence to treatment regimens, and limited diagnostic capacity (Omulo et al., 2015; Omulo et al., 2017). The high resistance proportions observed in North Africa/Middle East and Asia may be associated with increased antibiotic use over the past decade. These findings highlight the need for region-specific interventions, including improving healthcare infrastructure, strengthening antibiotic policies, and raising public awareness to mitigate AMR effectively.
Our study assessed pooled AMR prevalence in community-acquired enteric bacteria, namely E. coli, Salmonella, Shigella, and Campylobacter. While our focus was on pooled AMR prevalence from community-acquired infections, understanding colonization prevalence, especially for E. coli, is crucial. The ubiquitous presence of E. coli in the gastrointestinal tract underscores the importance of colonization prevalence data. Unlike Salmonella, Shigella, and Campylobacter, high colonization prevalence of E. coli may not directly correlate with AMR prevalence. However, it can significantly determine the risk of AMR development and transmission.
4.5 Limitations
We observed a high variance in resistance proportions across the individual study estimates. Heterogeneity between studies and between regions was in most cases I2 ≥ 75%. This high heterogeneity is likely attributable to differences in study design, setting, sampling design, and bacterial isolation and identification methods, as well as variations in AST methods. Since studies conducted after 2005 were included, it is highly likely that some studies used outdated Clinical and Laboratory Standards Institute (CLSI) and European Committee of Antimicrobial Susceptibility Testing (EUCAST) breakpoints. This could introduce bias as we were unable to reinterpret or adjust resistance data based on the latest breakpoints due to the reporting of results as the percentage or the number of resistant isolates rather than disk inhibition zones or minimum inhibitory concentration values. We included only studies that used laboratory-based phenotypic AST methods whose data are interpretable using CLSI or EUCAST, thus losing out on more robust methods that reported AMR genotypes. This might have limited the robustness of the findings by overlooking the genetic mechanisms of resistance. Furthermore, the 64 studies included in this systematic review and meta-analysis came from 23 countries (against a total of 82 LMICs) and thus may not be representative of all WHO geographical regions. Regions with limited research infrastructure or fewer published studies may be underrepresented, leading to gaps in data coverage and potential underestimation of resistance in these areas.
Studies published before 2005 were excluded to ensure alignment with WHO’s intensified efforts to address AMR as a global public health threat that started around this period. Excluding studies with older data or different methodologies helps maintain consistency and relevance in the reported findings as older studies may not reflect current testing standards or resistance patterns. However, this exclusion may limit historical context and trends, potentially underestimating the evolution of resistance over time.
To address these constraints future research should focus on harmonizing AST methodologies, incorporating genotypic data for a more comprehensive understanding of resistance mechanisms, and ensuring broader geographical representation by including studies from underrepresented regions. Expanding databases to include data reported in various formats could also help mitigate publication bias and enhance the generalizability of findings.
5 Conclusion
The present systematic review provides evidence of the prevalence of resistant enteric bacteria in children. It provides evidence that these CESS pathogens are increasingly becoming insensitive to clinically important antimicrobials. Regional differences in resistance patterns among these community isolates underpin the need to strengthen local and regional AMR surveillance systems to understand these reported differences. This would then inform clinical practice and the development of appropriate stewardship measures. Since the research and development pipeline of novel antimicrobials is lacking, there is an urgent need for the management of antimicrobials to prolong their use and explore alternative therapies such as vaccine development and use that reduce febrile illnesses, thus negating the need for antibiotic prescription.
Data availability statement
The original contributions presented in the study are included in the article/Supplementary material, further inquiries can be directed to the corresponding author.
Author contributions
NO: Conceptualization, Data curation, Formal analysis, Investigation, Methodology, Validation, Visualization, Writing – original draft, Writing – review & editing. DM: Formal analysis, Methodology, Visualization, Writing – review & editing. AM: Methodology, Supervision, Writing – review & editing. JW: Data curation, Writing – review & editing, Methodology. AK: Data curation, Investigation, Writing – review & editing. LO: Data curation, Investigation, Writing – review & editing. JOW: Data curation, Investigation, Writing – review & editing. CM: Writing – review & editing. LA: Writing – review & editing, Data curation, Investigation. JN: Supervision, Writing – review & editing. OC: Funding acquisition, Supervision, Writing – review & editing. EC: Conceptualization, Funding acquisition, Resources, Supervision, Writing – review & editing.
Funding
The author(s) declare that financial support was received for the research and/or publication of this article. This work was supported by the Bill & Melinda Gates Foundation (BMGF) and the Foreign, Commonwealth and Development Office (FCDO) of the UK Government (INV-008449); and the CGIAR One Health Initiative “Protecting Human Health Through a One Health Approach,” which was supported by contributors of the CGIAR Trust Fund (https://www.cgiar.org/funders/).
Conflict of interest
The authors declare that the research was conducted in the absence of any commercial or financial relationships that could be construed as a potential conflict of interest.
The author(s) declared that they were an editorial board member of Frontiers, at the time of submission. This had no impact on the peer review process and the final decision.
Generative AI statement
The authors declare that no Gen AI was used in the creation of this manuscript.
Publisher’s note
All claims expressed in this article are solely those of the authors and do not necessarily represent those of their affiliated organizations, or those of the publisher, the editors and the reviewers. Any product that may be evaluated in this article, or claim that may be made by its manufacturer, is not guaranteed or endorsed by the publisher.
Supplementary material
The Supplementary material for this article can be found online at: https://www.frontiersin.org/articles/10.3389/fmicb.2025.1539160/full#supplementary-material
References
Abayneh, M., Tesfaw, G., and Abdissa, A. (2018). Isolation of extended-Spectrum β-lactamase-(ESBL-) producing Escherichia coli and Klebsiella pneumoniae from patients with community-onset urinary tract infections in Jimma University specialized hospital, Southwest Ethiopia. Can. J. Infect. Dis. Med. Microbiol. 2018:6159. doi: 10.1155/2018/4846159
Abebe, W., Earsido, A., Taye, S., Assefa, M., Eyasu, A., and Godebo, G. (2018). Prevalence and antibiotic susceptibility patterns of Shigella and salmonella among children aged below five years with Diarrhoea attending Nigist Eleni Mohammed memorial hospital, South Ethiopia. BMC Pediatr. 18:241. doi: 10.1186/s12887-018-1221-9
Adugna, A., Kibret, M., Abera, B., Nibret, E., and Adal, M. (2015). Antibiogram of E. coli serotypes isolated from children aged under five with acute diarrhea in Bahir Dar town. Afr. Health Sci. 15, 656–664. doi: 10.4314/ahs.v15i2.45
Aggarwal, A., Mehta, S., Gupta, D., Sheikh, S., Pallagatti, S., Singh, R., et al. (2012). Clinical and immunological erythematosus patients characteristics in systemic lupus Maryam. J. Dent. Educ. 76, 1532–1539. doi: 10.4103/ijmr.IJMR
Allegranzi, B., Nejad, S., Combescure, C., Graafmans, W., Attar, H., Donaldson, L., et al. (2011). Burden of endemic health-care-associated infection in developing countries: systematic review and meta-analysis. Lancet 377, 228–241. doi: 10.1016/S0140-6736(10)61458-4
Al-Saadi, Z. H., Tarish, A. H., and Saeed, E. A. (2018). Phenotypic detection and antibiotics resistance pattern of local serotype of E. coli O157:H7 from children with acute diarrhea in hilla city/Iraq. J. Pharm. Sci. Res. 10, 604–609.
Amaya, E., Reyes, D., Vilchez, S., Paniagua, M., Mollby, R., Nord, C. E., et al. (2011). Antibiotic resistance patterns of intestinal Escherichia coli isolates from Nicaraguan children. J. Med. Microbiol. 60, 216–222. doi: 10.1099/jmm.0.020842-0
Ameya, G., Tsalla, T., Getu, F., and Getu, E. (2018). Antimicrobial susceptibility pattern, and associated factors of salmonella and Shigella infections among under five children in Arba Minch, South Ethiopia. Ann. Clin. Microbiol. Antimicrob. 17:1. doi: 10.1186/s12941-018-0253-1
Amin, M., Sirous, M., Javaherizadeh, H., Motamedifar, M., Saki, M., Veisi, H., et al. (2018). Antibiotic resistance pattern and molecular characterization of extended-spectrum beta-lactamase producing enteroaggregative Escherichia coli isolates in children from Southwest Iran. Infect. Drug Resist. 11, 1097–1104. doi: 10.2147/IDR.S167271
Ananchaipattana, C., Hosotani, Y., Kawasaki, S., Bari, M., Yamaguchi, K., and Inatsu, Y. (2014). Serotyping, RAPD grouping and antibiotic susceptibility testing of Salmonella enterica isolated from retail foods in Thailand. Food Sci. Technol. Res. 20, 905–913. doi: 10.3136/fstr.20.905
Ansari, S., Sherchand, J., Parajuli, K., Mishra, S., Dahal, R., Shrestha, S., et al. (2012). Bacterial etiology of acute diarrhea in children under five years of age. J Nepal Health Res Counc 10, 218–223
Anvikar, A. R., Dolla, C., Dutta, S., Rao, V. G., Gadge, V. S., Shukla, G. P., et al. (2008). Role of Escherichia coli in acute diarrhoea in tribal preschool children of Central India. Paediatr. Perinat. Epidemiol. 22, 40–46. doi: 10.1111/j.1365-3016.2007.00892.x
Araque, M., and Labrador, I. (2018). Prevalence of fecal carriage of CTX-M-15 beta-lactamase-producing Escherichia coli in healthy children from a rural andean village in Venezuela. Osong. Public Health Res. Perspect. 9, 9–15. doi: 10.24171/j.phrp.2018.9.1.03
Asare, K., Amoah, S., Coomson, C., Banson, C., Yaro, D., Mbata, J., et al. (2022). Antibiotic-resistant pathogenic bacterial isolates from patients attending the outpatient department of university of Cape Coast hospital, Ghana: a retrospective study between 2013–2015. PLoS Global Public Health 2, –e0000417. doi: 10.1371/journal.pgph.0000417
Assefa, A., and Girma, M. (2019). Prevalence and antimicrobial susceptibility patterns of Salmonella and Shigella isolates among children aged below five years with diarrhea attending robe general hospital and Goba referral hospital, south East Ethiopia. Trop. Dis. Travel Med. Vaccines 5:19. doi: 10.1186/s40794-019-0096-6
Awiti, J., Mumma, O., Cumming, O., Simiyu, S., Czerniewska, A., Aseyo, R., et al. (2019). Infant food hygiene and childcare practices in context: findings from an urban informal settlement in Kenya. Am J Trop Med Hyg 102, 220–222. doi: 10.4269/ajtmh.19-0279
Ayobami, O., Brinkwirth, S., Eckmanns, T., Markwart, R., Ayobami, O., Brinkwirth, S., et al. (2022). Antibiotic resistance in hospital-acquired ESKAPE- E infections in low- and lower-middle-income countries: a systematic review and meta-analysis. Emerg Microbes Infect 11, 443–451. doi: 10.1080/22221751.2022.2030196
Azmi, I., Khajanchi, B., Akter, F., Hasan, T., Shahnaij, M., Akter, M., et al. (2014). Fluoroquinolone resistance mechanisms of Shigella flexneri isolated in Bangladesh. PLoS One 9:e102533. doi: 10.1371/journal.pone.0102533
Barber, S., and Sutherland, N. (2017). O'Neill review into antibiotic resistance. House of Commons Library 1, 1–41.
Bartoloni, A., Pallecchi, L., Rodríguez, H., Fernandez, C., Mantella, A., Bartalesi, F., et al. (2009). Antibiotic resistance in a very remote Amazonas community. Int. J. Antimicrob. Agents 33, 125–129. doi: 10.1016/j.ijantimicag.2008.07.029
Beatty, M., Ochieng, J., Chege, W., Kumar, L., Okoth, G., Shapiro, R., et al. (2009). Sporadic paediatric diarrhoeal illness in urban and rural sites in Nyanza Province, Kenya. East Afr. Med. J. 86, 387–398. doi: 10.4314/eamj.v86i8.54159
Bernabé, K., Langendorf, C., Ford, N., Ronat, J., and Murphy, R. (2017). Antimicrobial resistance in West Africa: a systematic review and meta-analysis. Int. J. Antimicrob. Agents 50, 629–639. doi: 10.1016/j.ijantimicag.2017.07.002
Beyene, G., and Tasew, H. (2014). Prevalence of intestinal parasite, Shigella and salmonella species among diarrheal children in Jimma health center, Jimma Southwest Ethiopia: a cross sectional study. Ann. Clin. Microbiol. Antimicrob. 13:10. doi: 10.1186/1476-0711-13-10
Bhattarai, V., Sharma, S., Rijal, K. R., and Banjara, M. R. (2020). Co-infection with campylobacter and rotavirus in less than 5 year old children with acute gastroenteritis in Nepal during 2017-2018. BMC Pediatr. 20:20. doi: 10.1186/s12887-020-1966-9
Bishaw, B., Tegegne, G., and Berha, A. (2021). Appropriate use of ceftriaxone in sub-saharan Africa: a systematic review. Infect. Drug Resist. 14, 3477–3484. doi: 10.2147/IDR.S329996
Bodhidatta, L., McDaniel, P., Sornsakrin, S., Srijan, A., Serichantalergs, O., and Mason, C. (2010). Case-control study of diarrheal disease etiology in a remote rural area in Western Thailand. Am. J. Trop. Med. Hyg. 83, 1106–1109. doi: 10.4269/ajtmh.2010.10-0367
Browne, A., Chipeta, M., Haines-Woodhouse, G., Kumaran, E., Hamadani, B., Zaraa, S., et al. (2021). Global antibiotic consumption and usage in humans, 2000–18: a spatial modelling study. Lancet Planet Health 5, e893–e904. doi: 10.1016/S2542-5196(21)00280-1
CDC. Centers for Disease Control and Prevention, Escherichia coli. CDC; (2022). Available online at: https://www.cdc.gov/ecoli/index.html (accessed May 19, 2023)
Clermont, O., Bonacorsi, S., and Bingen, E. (2000). Rapid and simple determination of the Escherichia coli phylogenetic group. Appl. Environ. Microbiol. 66, 4555–4558. doi: 10.1128/AEM.66.10.4555-4558.2000
Coulidiaty, A., Sanou, A., Houngbedji, C., Djibougou, D., Dicko, A., Kobo, G., et al. (2021). Prevalence and sensitivity to antibiotics of campylobacter spp. in chicken, farmers and soil in Bobo-Dioulasso, Burkina Faso. Pan African medical journal one. Health 4, 1–12. doi: 10.11604/pamj-oh.2021.4.8.28089
Dhital, S., Sherchand, J. B., Pokharel, B. M., Parajuli, K., Mishra, S. K., Sharma, S., et al. (2017). Antimicrobial susceptibility pattern of Shigella spp. isolated from children under 5 years of age attending tertiary care hospitals, Nepal along with first finding of ESBL-production. BMC. Res. Notes 10:192. doi: 10.1186/s13104-017-2512-1
Dias, R., dos Santos, B., dos Santos, L., Vieira, M., Yamatogi, R., Mondelli, A., et al. (2016). Diarrheagenic Escherichia coli pathotypes investigation revealed atypical enteropathogenic E-coli as putative emerging diarrheal agents in children living in Botucatu, Sao Paulo state, Brazil. Apmis 124, 299–308. doi: 10.1111/apm.12501
Dyar, O. J., Hoa, N. Q., Trung, N. V., Phuc, H. D., Larsson, M., Chuc, N. T. K., et al. (2012). High prevalence of antibiotic resistance in commensal Escherichia coli among children in rural Vietnam. BMC Infect. Dis. 12:12. doi: 10.1186/1471-2334-12-92
El Metwally, H. A. R., Ibrahim, H. A. H., El-Athamna, M. N., and Amer, M. A. (2007). Multiplex PCR for detection of diarrheagenic Escherichia coli in Egyptian children. J. Med. Sci. 7, 255–262. doi: 10.3923/jms.2007.255.262
Farahani, N. N., Jazi, F. M., Nikmanesh, B., Asadolahi, P., Kalani, B. S., and Amirmozafari, N. (2018). Prevalence and antibiotic susceptibility patterns of salmonella and Shigella species isolated from pediatric diarrhea in Tehran. Archives of pediatric. Infect. Dis. 6, In Press:328. doi: 10.5812/pedinfect.57328
Farthing, M., Salam, M., Lindberg, G., Dite, P., Khalif, I., Salazar-Lindo, E., et al. (2013). Acute diarrhea in adults and children: a global perspective. J. Clin. Gastroenterol. 47, 12–20. doi: 10.1097/MCG.0b013e31826df662
Feeley, T. (2020). Assessing study quality in meta-analysis. Hum. Commun. Res. 46, 334–342. doi: 10.1093/hcr/hqaa001
Ferjani, S., Saidani, M., Maamar, E., Harbaoui, S., Hamzaoui, Z., Hosni, H., et al. (2018). Escherichia coli colonizing healthy children in Tunisia: high prevalence of extra-intestinal pathovar and occurrence of non-extended-spectrum-beta-lactamase-producing ST131 clone. Int. J. Antimicrob. Agents 52, 878–885. doi: 10.1016/j.ijantimicag.2018.07.015
Fuhrmeister, E., Harvey, A., Nadimpalli, M., Gallandat, K., Ambelu, A., Arnold, B., et al. (2023). Evaluating the relationship between community water and sanitation access and the global burden of antibiotic resistance: an ecological study. Lancet Microbe 4, e591–e600. doi: 10.1016/S2666-5247(23)00137-4
Gandra, S., Alvarez-Uria, G., Turner, P., Joshi, J., Limmathurotsakul, D., and van Doorn, H. (2020). Antimicrobial resistance surveillance in low-and middle-income countries: Progress and challenges in eight south Asian and southeast Asian countries. Clin. Microbiol. Rev. 33, 1–29. doi: 10.1128/CMR.00048-19
Garcia, P. G., Silva, V. L., and Diniz, C. G. (2011). Occurrence and antimicrobial drug susceptibility patterns of commensal and Diarrheagenic Escherichia coli in fecal microbiota from children with and without acute diarrhea. J. Microbiol. 49, 46–52. doi: 10.1007/s12275-011-0172-8
GebreSilasie, Y. M., Tullu, K. D., and Yeshanew, A. G. (2018). Resistance pattern and maternal knowledge, attitude and practices of suspected Diarrheagenic Escherichia coli among children under 5 years of age in Addis Ababa, Ethiopia: cross sectional study. Antimicrob. Resist. Infect. Control 7:7. doi: 10.1186/s13756-018-0402-5
Gelaw, L. Y., Bitew, A. A., Gashey, E. M., and Ademe, M. N. (2022). Ceftriaxone resistance among patients at GAMBY teaching general hospital. Sci. Rep. 12:12000. doi: 10.1038/s41598-022-16132-3
Giani, T., Sennati, S., Antonelli, A., Di Pilato, V., di Maggio, T., Mantella, A., et al. (2018). High prevalence of carriage of mcr-1-positive enteric bacteria among healthy children from rural communities in the Chaco region, Bolivia, September to October 2016. Euro surveillance 23:115. doi: 10.2807/1560-7917.ES.2018.23.45.1800115
Gonzales, L., Joffre, E., Rivera, R., Sjoling, A., Svennerholm, A., and Iniguez, V. (2013). Prevalence, seasonality and severity of disease caused by pathogenic Escherichia coli in children with diarrhoea in Bolivia. J. Med. Microbiol. 62, 1697–1706. doi: 10.1099/jmm.0.060798-0
González, F., and Araque, M. (2013). Association of transferable quinolone resistance determinant qnrB19 with extended-spectrum β -lactamases in salmonella give and salmonella Heidelberg in Venezuela. Int. J. Microbiol. 2013:8185. doi: 10.1155/2013/628185
Gu, B., Zhou, M., Ke, X., Pan, S., Cao, Y., Huang, Y., et al. (2015). Comparison of resistance to third-generation cephalosporins in Shigella between Europe-America and Asia-Africa from 1998 to 2012. Epidemiol. Infect. 143, 2687–2699. doi: 10.1017/S0950268814003446
Guarino, A., Bruzzese, E., and Giannattasio, A. (2018). Antibiotic treatment of acute gastroenteritis in children. F1000 Faculty Rev 7:193. doi: 10.12688/f1000research.12328.1
HaiLing, C., Ling, Z., YanLing, G., JieHao, C., XiangShi, W., Zheng, H., et al. (2017). A hospital-based case-control study of diarrhea in children in Shanghai. Pediatr. Infect. Dis. J. 36, 1057–1063. doi: 10.1097/INF.0000000000001562
Harrer, M., Cuijpers, P., Furukawa, T. A., and Ebert, D. D. (2021). Doing meta-analysis with R: a hands-on guide Boca Raton. FL and London: Chapman & Hall/CRC Press.
Hendriksen, R., Lukjancenko, O., Munk, P., Hjelmso, M., Verani, J., Ng'eno, E., et al. (2019). Pathogen surveillance in the informal settlement, Kibera, Kenya, using a metagenomics approach. PLoS One 14, e0222531–e0222515. doi: 10.1371/journal.pone.0222531
Hetzer, B., Orth-Holler, D., Wurzner, R., Kreidl, P., Lackner, M., Muller, T., et al. (2019). Enhanced acquisition of antibiotic-resistant intestinal E. coli during the first year of life assessed in a prospective cohort study. Antimicrob. Resist. Infect. Control 8, 1–13. doi: 10.1186/s13756-019-0522-6
Higgins, J., and Thompson, S. (2002). Quantifying heterogeneity in a meta-analysis. Stat. Med. 21, 1539–1558. doi: 10.1002/sim.1186
Hijazi, S., Fawzi, M., Ali, F., and Abd El Galil, K. (2016). Prevalence and characterization of extended-spectrum beta-lactamases producing Enterobacteriaceae in healthy children and associated risk factors. Ann. Clin. Microbiol. Antimicrob. 15, 3–9. doi: 10.1186/s12941-016-0121-9
Hlashwayo, D., Sigaúque, B., Noormahomed, E., Afonso, S., Mandomando, I., and Bila, C. (2021). A systematic review and meta-analysis reveal that campylobacter spp. and antibiotic resistance are widespread in humans in sub-Saharan Africa. PLoS One 16:e0245951. doi: 10.1371/journal.pone.0245951
Huang, Y., Shan, X. F., Deng, H. J., Huang, Y. J., Mu, X. P., Huang, A. L., et al. (2015). Epidemiology, antimicrobial resistance and beta-lactamase genotypic features of Enteropathogenic Escherichia coli isolated from children with diarrhea in southern China. Jpn. J. Infect. Dis. 68, 239–243. doi: 10.7883/yoken.JJID.2014.120
Huynh, B.-T., Padget, M., Garin, B., Herindrainy, P., Kermorvant-Duchemin, E., Watier, L., et al. (2015). Burden of bacterial resistance among neonatal infections in low income countries: how convincing is the epidemiological evidence? BMC Infect. Dis. 15, 1–13. doi: 10.1186/s12879-015-0843-x
Isendahl, J., Turlej-Rogacka, A., Manjuba, C., Rodrigues, A., Giske, C. G., and Naucler, P. (2012). Fecal carriage of ESBL-producing E. coli and K. pneumoniae in children in Guinea-Bissau: a hospital-based cross-sectional study. PLoS One 7:e51981. doi: 10.1371/journal.pone.0051981
Iskandar, K., Molinier, L., Hallit, S., Sartelli, M., Hardcastle, T., Haque, M., et al. (2021). Surveillance of antimicrobial resistance in low- and middle-income countries: a scattered picture. Antimicrob. Resist. Infect. Control 10, 63–19. doi: 10.1186/s13756-021-00931-w
Islam, M. A., Amin, M. B., Roy, S., Asaduzzaman, M., Islam, M. R., Navab-Daneshmand, T., et al. (2019). Fecal colonization with multidrug-resistant E-coli among healthy infants in rural Bangladesh. Front. Microbiol. 10:10. doi: 10.3389/fmicb.2019.00640
Jafari, F., Garcia-Gil, L. J., Salmanzadeh-Ahrabi, S., Shokrzadeh, L., Aslani, M. M., Pourhoseingholi, M. A., et al. (2009). Diagnosis and prevalence of enteropathogenic bacteria in children less than 5 years of age with acute diarrhea in Tehran children's hospitals. J. Infect. 58, 21–27. doi: 10.1016/j.jinf.2008.10.013
Kasolo, F., Yahaya, A., Ndihokubwayo, J., Impouma, B., Oxenford, C., and Cognat, S. (2013). Guide for establishing laboratory-based surveillance for antimicrobial resistance. Brazzaville, Republic of Congo: World Health Organization Regional Office for Africa, 1–25.
Klein, E. Y., Van Boeckel, T. P., Martinez, E. M., Pant, S., Gandra, S., Levin, S. A., et al. (2018). Global increase and geographic convergence in antibiotic consumption between 2000 and 2015. Proc. Natl. Acad. Sci. USA 115, E3463–E3470. doi: 10.1073/pnas.1717295115
Le Doare, K., Bielicki, J., Heath, P. T., and Sharland, M. (2015). Systematic review of antibiotic resistance rates among gram-negative bacteria in children with sepsis in resource-limited countries. J Pediatric Infect Dis Soc 4, 11–20. doi: 10.1093/jpids/piu014
Lefèvre, S., Njamkepo, E., Feldman, S., Ruckly, C., Carle, I., Lejay-Collin, M., et al. (2023). Rapid emergence of extensively drug-resistant Shigella sonnei in France. Nat. Commun. 14:462. doi: 10.1038/s41467-023-36222-8
Lengerh, A., Moges, F., Unakal, C., and Anagaw, B. (2013). Prevalence, associated risk factors and antimicrobial susceptibility pattern of campylobacter species among under five diarrheic children at Gondar University hospital, Northwest Ethiopia. BMC Pediatr. 13:13. doi: 10.1186/1471-2431-13-82
Liu, L., Johnson, H., Cousens, S., Perin, J., Scott, S., Lawn, J., et al. (2012). Global, regional, and national causes of child mortality: an updated systematic analysis for 2010 with time trends since 2000. Lancet 379, 2151–2161. doi: 10.1016/S0140-6736(12)60560-1
Mahdavi Broujerdi, S., Roayaei Ardakani, M., Rezatofighi, S. E., Borujerdi, S. M., Ardakani, M. R., and Rezatofighi, S. E. (2018). Characterization of diarrheagenic Escherichia coli strains associated with diarrhea in children, Khouzestan, Iran. J. Infect Dev. Count. 12, 649–656. doi: 10.3855/jidc.9538
Mahmoudi-aznaveh, A., Bakhshi, B., and Najar-peerayeh, S. (2017). The trend of enteropathogenic Escherichia coli towards atypical multidrug resistant genotypes. J. Chemother. 29, 1–7. doi: 10.1080/1120009X.2016.1154683
Mandomando, I., Jaintilal, D., Pons, M. J., Valles, X., Espasa, M., Mensa, L., et al. (2009). Antimicrobial susceptibility and mechanisms of resistance in Shigella and salmonella isolates from children under five years of age with diarrhea in rural Mozambique. Antimicrob. Agents Chemother. 53, 2450–2454. doi: 10.1128/AAC.01282-08
Mandomando, I., Sigauque, B., Valles, X., Espasa, M., Sanz, S., Sacarlal, J., et al. (2007). Epidemiology and clinical presentation of shigellosis in children less than five years of age in rural Mozambique. Pediatr. Infect. Dis. J. 26, 1059–1061. doi: 10.1097/INF.0b013e31812e55a2
Mekonnen, M., Geda, B., Teklemariam, Z., Weldegebreal, F., and Balakrishnan, S. (2017). Prevalence of childhood diarrhea and associated risk factors in Dire Dawa, eastern Ethiopia. J. Public Health 26, 29–37. doi: 10.1007/s10389-017-0843-y
Monira, S., Shabnam, S. A., Ali, S. I., Sadique, A., Johura, F. T., Rahman, K. Z., et al. (2017). Multi-drug resistant pathogenic bacteria in the gut of young children in Bangladesh. Gut Pathogens 9:19. doi: 10.1186/s13099-017-0170-4
Morpeth, S., Thielman, N., Ramadhani, H., Hamilton, J., Ostermann, J., Kisenge, P., et al. (2008). Effect of trimethoprim-sulfamethoxazole prophylaxis on antimicrobial resistance of fecal Escherichia coli in HIV-infected patients in Tanzania. JAIDS 47, 585–591. doi: 10.1097/QAI.0b013e31816856db
Moyo, S., Gro, N., Matee, M., Kitundu, J., Myrmel, H., Mylvaganam, H., et al. (2011). Age specific aetiological agents of diarrhoea in hospitalized children aged less than five years in Dar Es Salaam, Tanzania. BMC Pediatr. 11, 1–6. doi: 10.1186/1471-2431-11-19
Mulatu, G., Beyene, G., and Zeynudin, A. (2014). Prevalence of Shigella, salmonella and campylobacter species and their susceptibility patters among under five children with diarrhea in Hawassa town, South Ethiopia. Ethiop. J. Health Sci. 24, 101–108. doi: 10.4314/ejhs.v24i2.1
Murray, C., Ikuta, K., Sharara, F., Swetschinski, L., Aguilar, G., Gray, A., et al. (2022). Global burden of bacterial antimicrobial resistance in 2019: a systematic analysis. Lancet 399, 629–655. doi: 10.1016/S0140-6736(21)02724-0
Naderi, G., Haghi, F., Zeighami, H., Hemati, F., and Masoumian, N. (2016). Distribution of pathogenicity island (PAI) markers and phylogenetic groups in diarrheagenic and commensal Escherichia coli from young children. Gastroenterol. Hepatol. Bed Bench 9, 316–324
Najibi, S., Bakhshi, B., Fallahzad, S., Pourshafie, M. R., Katouli, M., Sattari, M., et al. (2012). Distribution of class 1 integrons among enteropathogenic Escherichia coli. Can. J. Microbiol. 58, 637–643. doi: 10.1139/w2012-035
Nakhjavani, F. A., Emaneini, M., Hosseini, H., Iman-Eini, H., Aligholi, M., Jabalameli, F., et al. (2013). Molecular analysis of typical and atypical enteropathogenic Escherichia coli (EPEC) isolated from children with diarrhoea. J. Med. Microbiol. 62, 191–195. doi: 10.1099/jmm.0.046516-0
Nji, E., Kazibwe, J., Hambridge, T., Joko, C., Larbi, A., Damptey, L., et al. (2021). High prevalence of antibiotic resistance in commensal Escherichia coli from healthy human sources in community settings. Sci. Rep. 11, 3372–3311. doi: 10.1038/s41598-021-82693-4
Nunes, M. R., Magalhaes, P. P., Penna, F. J., Nunes, J. M., and Mendes, E. N. (2012). Diarrhea associated with Shigella in children and susceptibility to antimicrobials. J. Pediatr. 88, 125–128. doi: 10.2223/JPED.2131
Nyanga, P., Onyuka, J., Webale, M., Were, T., and Budambula, V. (2017). Escherichia coli pathotypes and Shigella sero-groups in diarrheic children in Nairobi city, Kenya. Gastroenterol Hepatol Bed Bench 10, 220–228
Ochoa, T., Ruiz, J., Molina, M., Del Valle, L., Vargas, M., Gil, A., et al. (2009). High frequency of antimicrobial drug resistance of Diarrheagenic Escherichia coli in infants in Peru. Am. J. Trop. Med. Hyg. 81, 296–301. doi: 10.4269/ajtmh.2009.81.296
Okolie, O., Igwe, U., Ismail, S., Ighodalo, U., and Adukwu, E. (2022). Systematic review of surveillance systems for AMR in Africa. J. Antimicrob. Chemother. 78, 31–51. doi: 10.1093/jac/dkac342
Okomo, U., Akpalu, E., Doare, K., Roca, A., Cousens, S., Jarde, A., et al. (2019). Articles Aetiology of invasive bacterial infection and antimicrobial resistance in neonates in sub-Saharan Africa: a systematic review and meta-analysis in line with the STROBE-NI reporting guidelines. Lancet Infect. Dis. 19, 1219–1234. doi: 10.1016/S1473-3099(19)30414-1
Omolajaiye, S., Afolabi, K., and Iweriebor, B. (2020). Pathotyping and antibiotic resistance profiling of Escherichia coli isolates from children with acute diarrhea in amatole district municipality of eastern cape, South Africa. BioMed Res. Int. 2020, 1–10. doi: 10.1155/2020/4250165
Omulo, S., Thumbi, S., Lockwood, S., Verani, J., Bigogo, G., Masyongo, G., et al. (2017). Evidence of superficial knowledge regarding antibiotics and their use: results of two cross-sectional surveys in an urban informal settlement in Kenya. PLoS One 12:e0185827. doi: 10.1371/journal.pone.0185827
Omulo, S., Thumbi, S., Njenga, M., and Call, D. (2015). A review of 40 years of enteric antimicrobial resistance research in eastern Africa: what can be done better? Antimicrob. Resist. Infect. Control 4, 1–13. doi: 10.1186/s13756-014-0041-4
Otto, S., Haworth-Brockman, M., Miazga-Rodriguez, M., Wierzbowski, A., and Saxinger, L. (2022). Integrated surveillance of antimicrobial resistance and antimicrobial use: evaluation of the status in Canada (2014-2019). Can. Vet. J. 113, 11–22. doi: 10.17269/s41997-021-00600-w
Parajuli, N. P., Joshi, G., Pardhe, B. D., Shakya, J., Bhetwal, A., Shakya, S., et al. (2017). Shigellosis caused by CTX-M type ESBL producing Shigella flexneri in two siblings of rural Nepal: first case report from the country. Case Rep. Infect. Dis. 2017, 1–6. doi: 10.1155/2017/1862320
Park, S., Pham, D., Pak, G., Panzner, U., Maria Cruz Espinoza, L., Von Kalckreuth, V., et al. (2021). The genomic epidemiology of multi-drug resistant invasive non-typhoidal salmonella in selected sub-Saharan African countries. BMJ Glob. Health 6:e005659. doi: 10.1136/bmjgh-2021-005659
Prabhurajeshwar, C., Desai, P., and Chandrakanth, R. K. (2016). Molecular evaluation of high fluoroquinolone resistant genes in endemic cases of shigellosis, northeast part of Karnataka, India. Ann. Global Health 82, 832–839. doi: 10.1016/j.aogh.2016.09.009
Qu, M., Lv, B., Zhang, X., Yan, H. Q., Huang, Y., Qian, H. K., et al. (2016). Prevalence and antibiotic resistance of bacterial pathogens isolated from childhood diarrhea in Beijing, China (2010-2014). Gut Pathogens 8:31. doi: 10.1186/s13099-016-0116-2
Rahouma, A., Klena, J. D., Krema, Z., Abobker, A. A., Treesh, K., Franka, E., et al. (2011). Enteric pathogens associated with childhood diarrhea in Tripoli-Libya. Am. J. Trop. Med. Hyg. 84, 886–891. doi: 10.4269/ajtmh.2011.11-0116
Rolain, J. (2013). Food and human gut as reservoirs of transferable antibiotic resistance encoding genes. Front. Microbiol. 4, 1–10. doi: 10.3389/fmicb.2013.00173
Sahoo, K. C., Tamhankar, A. J., Sahoo, S., Sahu, P. S., Klintz, S. R., and Lundborg, C. S. (2012). Geographical variation in antibiotic-resistant Escherichia coli isolates from stool, cow-dung and drinking water. Int. J. Environ. Res. Public Health 9, 746–759. doi: 10.3390/ijerph9030746
Said, M., Msanga, D., Mtemisika, C., Silago, V., Mirambo, M., and Mshana, S. (2022). Extended Spectrum β-lactamase producing lactose fermenting bacteria colonizing children with human immunodeficiency virus, sickle cell disease and diabetes mellitus in Mwanza City, Tanzania: a cross-sectional study. Trop. Med. Infect. Dis. 7:144. doi: 10.3390/tropicalmed7080144
Saleem, A. F., Allana, A., Hale, L., Diaz, A., Salinas, R., Salinas, C., et al. (2020). The gut of healthy infants in the community as a reservoir of ESBL and carbapenemase-producing bacteria. Antibiotics 9:9. doi: 10.3390/antibiotics9060286
Sang, W., Oundo, V., and Schnabel, D. (2012). Prevalence and antibiotic resistance of bacterial pathogens isolated from childhood diarrhoea in four provinces of Kenya. J. Infect. Dev. Ctries. 6, 572–578. doi: 10.3855/jidc.2196
Seidman, J. C., Anitha, P., Kanungo, R., Bourgeois, A. L., and Coles, C. L. (2009). Risk factors for antibiotic-resistant E. coli in children in a rural area. Epidemiol. Infect. 137, 879–888. doi: 10.1017/S0950268808001519
Shamseer, L., Moher, D., Clarke, M., Ghersi, D., Liberati, A., Petticrew, M., et al. (2015). Preferred reporting items for systematic review and meta-analysis protocols (prisma-p) 2015: elaboration and explanation. BMJ 350, 1–25. doi: 10.1136/bmj.g7647
Simiyu, S., Aseyo, E., Anderson, J., Cumming, O., Baker, K., Dreibelbis, R., et al. (2023). A mixed methods process evaluation of a food hygiene intervention in low-income informal Neighbourhoods of Kisumu, Kenya. Matern. Child Health J. 27, 824–836. doi: 10.1007/s10995-022-03548-6
Simiyu, S., Czerniewska, A., Aseyo, E., Baker, K., Cumming, O., Mumma, J., et al. (2020). Designing a food hygiene intervention in low-income, peri-urban context of Kisumu, Kenya: application of the trials of improved practices methodology. Am J Trop Med Hyg 102, 1116–1123. doi: 10.4269/ajtmh.19-0629
Singh, T., Singh, P. K., Dar, S. A., Haque, S., Akhter, N., and Das, S. (2019). Changing paradigm of antibiotic resistance amongst Escherichia coli isolates in Indian pediatric population. PLoS One 14, –e0213850. doi: 10.1371/journal.pone.0213850
Singh, T., Singh, P. K., Das, S., Wani, S., Jawed, A., and Dar, S. A. (2019). Transcriptome analysis of beta-lactamase genes in diarrheagenic Escherichia coli. Sci. Rep. 9:3626. doi: 10.1038/s41598-019-40279-1
Smellie, W. (2006). Testing pitfalls and summary of guidance in lipid management. Br. Med. J. 333, 83–86. doi: 10.1136/bmj.333.7558.83
Snehaa, K., Singh, T., Dar, S. A., Haque, S., Ramachandran, V. G., Saha, R., et al. (2020). Typical and atypical enteropathogenic Escherichia coli in children with acute diarrhoea: changing trend in East Delhi. Biom. J. 44, 471–478. doi: 10.1016/j.bj.2020.03.011
Sousa, M. A. B., Mendes, E. N., Collares, G. B., Peret, L. A., Penna, F. J., Magalhaes, P. P., et al. (2013). Shigella in Brazilian children with acute diarrhoea: prevalence, antimicrobial resistance and virulence genes. Memorias Do Instituto Oswaldo Cruz 108, 30–35. doi: 10.1590/S0074-02762013000100005
Souza, T. B., Morais, M. B., Tahan, S., Melli, L., Rodrigues, M. S. C., and Scaletsky, I. C. A. (2009). High prevalence of antimicrobial drug-resistant diarrheagenic Escherichia coli in asymptomatic children living in an urban slum. J. Infect. 59, 247–251. doi: 10.1016/j.jinf.2009.08.007
Stoesser, N., Xayaheuang, S., Vongsouvath, M., Phommasone, K., Elliott, I., Elias, C. D., et al. (2014). Colonization with Enterobacteriaceae producing ESBLs in children attending pre-school childcare facilities in the Lao People's Democratic Republic. J. Antimicrob. Chemother. 70, 1893–1897. doi: 10.1093/jac/dkv021
Sulis, G., Sayood, S., and Gandra, S. (2022). Antimicrobial resistance in low- and middle-income countries: current status and future directions. Expert Rev. Anti-Infect. Ther. 20, 147–160. doi: 10.1080/14787210.2021.1951705
The, H., Rabaa, M., Thanh, D., De Lappe, N., Cormican, M., Valcanis, M., et al. (2016). South Asia as a reservoir for the global spread of ciprofloxacin-resistant Shigella sonnei: a cross-sectional study. PLoS Med. 13:e1002055. doi: 10.1371/journal.pmed.1002055
Tosisa, W., Mihret, A., Ararsa, A., Eguale, T., and Abebe, T. (2020). Prevalence and antimicrobial susceptibility of salmonella and Shigella species isolated from diarrheic children in ambo town. BMC Pediatr. 20:91. doi: 10.1186/s12887-020-1970-0
United Nations Environment Programme. Bracing for superbugs: Strengthening environmental action in the one health response to antimicrobial resistance (2023). Available online at: https://www.unep.org/resources/superbugs/environmental-action (accessed February 20, 2023)
Viechtbauer, W., López-López, J., Sánchez-Meca, J., and Marín-Martínez, F. (2015). A comparison of procedures to test for moderators in mixed-effects meta-regression models. Psychol. Methods 20, 360–374. doi: 10.1037/met0000023
Vilchez, S., Becker-Dreps, S., Amaya, E., Perez, C., Paniagua, M., Reyes, D., et al. (2014). Characterization of enterotoxigenic Escherichia coli strains isolated from Nicaraguan children in hospital, primary care and community settings. J. Med. Microbiol. 63, 729–734. doi: 10.1099/jmm.0.066779-0
Webale, M., Wanjala, C., Guyah, B., Shaviya, N., Munyekenye, G., Nyanga, P., et al. (2020). Epidemiological patterns and antimicrobial resistance of bacterial diarrhea among children in Nairobi City, Kenya. Gastroenterol Hepatol Bed Bench 13, 238–246
WHO. World Health Organization: guidelines for the control of shigellosis, including epidemics due to Shigella dysenteriae type 1 (2005a). Available online at: https://iris.who.int/handle/10665/20291 (accessed February 6, 2024)
Wieters, I., Johnstone, S., Makiala-Mandanda, S., Poda, A., Akoua-Koffi, C., Abu Sin, M., et al. (2024). Reported antibiotic use among patients in the multicenter ANDEMIA infectious diseases surveillance study in sub-saharan Africa. Antimicrob. Resist. Infect. Control 13:9. doi: 10.1186/s13756-024-01365-w
Williams, P., and Berkley, J. (2018). Guidelines for the treatment of dysentery (shigellosis): a systematic review of the evidence. J Paediatr 38, S50–S65. doi: 10.1080/20469047.2017.1409454
Woerther, P.-L., Burdet, C., Chachaty, E., and Andremont, A. (2013). Trends in human fecal carriage of extended-spectrum β-lactamases in the community: toward the globalization of CTX-M. Clin. Microbiol. Rev. 26, 744–758. doi: 10.1128/CMR.00023-13
World Bank. World Bank, New country classifications by income level: 2019-2020 (2019). Available online at: https://blogs.worldbank.org/en/opendata/new-country-classifications-income-level-2019-2020 (accessed August 2, 2020)
World Health Organization. Antimicrobial resistance: a threat to global health security. Rational use of medicines by prescribers and patients: report by the secretariat (A58/14) Fifty-eighth World Health Assembly (2005b). Available online at: https://apps.who.int/gb/archive/pdf_files/WHA58/A58_14-en.pdf (accessed February 22, 2023)
World Health Organization. World health organization: joint external evaluation of IHR Core capacities of Pakistan (2016). Available online at: https://apps.who.int/iris/bitstream/handle/10665/254614/WHO-WHE-CPI-2017.9-eng.pdf (accessed March 24, 2022)
World Health Organization. World Health Organization: global action plan on antimicrobial resistance (2017a). Available online at: https://apps.who.int/iris/bitstream/handle/10665/193736/9789241509763_eng.pdf (accessed April 27, 2022)
World Health Organization. World Health Organization: joint external evaluation of IHR Core capacities of the People's Republic of Bangladesh (2017b). Available online at: https://iris.who.int/bitstream/handle/10665/254275/WHO-HSE-GCR-2016.23-eng.pdf?sequence=1&isAllowed=y (accessed May 19, 2023)
World Health Organization. World health organization list of critically important antimicrobials (2019). Available online at: https://apps.who.int/iris/bitstream/handle/10665/312266/9789241515528-eng.pdf (accessed October 2, 2023)
World Health Organization. World Health Organization: GLASS methodology for surveillance of national antimicrobial consumption (2020). Available online at: https://iris.who.int/bitstream/handle/10665/336215/9789240012639-eng.pdf (accessed February 22, 2023)
Younas, M., Siddiqui, F., Noreen, Z., Bokhari, S. S., Gomez-Duarte, O. G., Wren, B. W., et al. (2016). Characterization of enteropathogenic Escherichia coli of clinical origin from the pediatric population in Pakistan. Trans. R. Soc. Trop. Med. Hyg. 110, 414–420. doi: 10.1093/trstmh/trw047
Zaidi, M. B., McDermott, P. F., Campos, F. D., Chim, R., Leon, M., Vazquez, G., et al. (2012). Antimicrobial-resistant campylobacter in the food chain in Mexico. Foodborne Pathog. Dis. 9, 841–847. doi: 10.1089/fpd.2012.1127
Zhang, H., Zhou, Y., Guo, S., and Chang, W. (2015). High prevalence and risk factors of fecal carriage of CTX-M type extended-spectrum beta-lactamase-producing Enterobacteriaceae from healthy rural residents of Taian, China. Front. Microbiol. 6, 1–5. doi: 10.3389/fmicb.2015.00239
Keywords: antimicrobial resistance, low-and middle-income countries, enteric bacteria, community, children
Citation: Okumu NO, Muloi DM, Moodley A, Watson J, Kiarie A, Ochieng L, Wasonga JO, Mutisya C, Alumasa L, Ngeranwa JJN, Cumming O and Cook EAJ (2025) Antimicrobial resistance in community-acquired enteric pathogens among children aged ≤ 10-years in low-and middle-income countries: a systematic review and meta-analysis. Front. Microbiol. 16:1539160. doi: 10.3389/fmicb.2025.1539160
Edited by:
Santi M. Mandal, Indian Institute of Technology Kharagpur, IndiaReviewed by:
Yabing Li, Michigan State University, United StatesAlidehou Jerrold Agbankpe, Institut Pasteur de Guinée, Guinea
Phénix Constant Essè Assogba, Research Unit in Applied Microbiology and Pharmacology of Natural Substances, Benin
Bashar Gulumbe, Federal University, Birnin Kebbi, Nigeria
Copyright © 2025 Okumu, Muloi, Moodley, Watson, Kiarie, Ochieng, Wasonga, Mutisya, Alumasa, Ngeranwa, Cumming and Cook. This is an open-access article distributed under the terms of the Creative Commons Attribution License (CC BY). The use, distribution or reproduction in other forums is permitted, provided the original author(s) and the copyright owner(s) are credited and that the original publication in this journal is cited, in accordance with accepted academic practice. No use, distribution or reproduction is permitted which does not comply with these terms.
*Correspondence: Noah O. Okumu, Ti5Pa3VtdUBjZ2lhci5vcmc=