- 1Philochem AG, Otelfingen, Switzerland
- 2Department of Chemistry and Applied Biosciences, Swiss Federal Institute of Technology (ETH Zürich), Zurich, Switzerland
- 3Philogen SpA, Monteriggioni, Italy
Certain cytokines synergize in activating anti-cancer immunity at the site of disease and it may be desirable to generate biopharmaceutical agents, capable of simultaneous delivery of cytokine pairs to the tumor. In this article, we have described the cloning, expression and characterization of IL2-XE114-TNFmut, a dual-cytokine biopharmaceutical featuring the sequential fusion of interleukin-2 (IL2) with the XE114 antibody in scFv format and a tumor necrosis factor mutant (TNFmut). The fusion protein recognized the cognate antigen (carbonic anhydrase IX, a marker of hypoxia and of renal cell carcinoma) with high affinity and specificity. IL2-XE114-TNFmut formed a stable non-covalent homotrimeric structure, displayed cytokine activity in in vitro tests and preferentially localized to solid tumors in vivo. The product exhibited a partial growth inhibition of murine CT26 tumors transfected for carbonic anhydrase IX. When administered to Cynomolgus monkey as intravenous injection, IL2-XE114-TNFmut showed the expected plasma concentration of ~1,500 ng/ml at early time points, indicating the absence of any in vivo trapping events, and a half-life of ~2 h. IL2-XE114-TNFmut may thus be considered as a promising biopharmaceutical for the treatment of metastatic clear-cell renal cell carcinoma, since these tumors are known to be sensitive to IL2 and to TNF.
Introduction
Antibody-cytokine fusions (also called “immunocytokines”) represent an emerging class of engineered cytokine products, that may display a superior anti-cancer activity as a consequence of a preferential accumulation at the tumor site, helping spare normal organs (1). An increased density of lymphocyte within the tumor mass typically correlates with a better prognosis, both in mice and in cancer patients (2–5). The targeted delivery of cytokines to the tumor environment may increase the intratumoral density of T-cells and NK cell (2, 4, 6). In this context, IL2 and interleukin-12 (IL12) have proven to be particularly attractive payloads for antibody-based delivery applications (7–9), as these agents can potently activate T-cells and NK cells.
Antibody-cytokine fusions with tumor-homing properties are likely to display their therapeutic action and to increase the therapeutic index of the corresponding cytokine payload as a result of a specific activation and proliferation of tumor-resident CD8+ T cells and of NK cells, which recognize malignant structures (2, 3). For some pro-inflammatory cytokine payload (e.g., IL12), it has been shown that an antibody-based targeted delivery to the tumor may increase therapeutic activity by at least 20-fold, compared to the recombinant cytokine counterpart (10).
Antibody-cytokine fusion proteins in clinical trials for the treatment of cancer include various antibody-IL2 fusions [e.g., hu14.18-IL2 (11), huKS-IL2 (12), L19-IL2 (13), F16-IL2 (14), CEA-IL2v (15), NHS-IL2 (16), DI-Leu16-IL2 (17)], as well as fusions with TNF [e.g., L19-TNF (18)] and with IL12 [BC1-IL12 (19) and NHS-IL12 (20)]. More recently, scientists at Glycart-Roche have described a novel antibody fusion with human 4-1BBL, which has started clinical trials (21). The most advanced product may be represented by a combination of L19-IL2 with L19-TNF, which is currently being investigated in Phase III clinical trials (NCT02938299 and NCT03567889) for the treatment of fully-resectable Stage IIIB, C melanoma (22).
IL2 and TNF are representative examples of cytokines which work well when used in combination. Our group already described in 2010 that the simultaneous administration of L19-IL2 and L19-TNF in an immunocompetent mouse model of neuroblastoma was more active than the products given as single agents. Indeed, the combined use of the two products cured the majority of mice (23). A synergistic benefit for L19-IL2 and L19-TNF was also observed for intralesional administration procedures in mouse models of cancer (24). This work paved the way for the execution of a Phase II clinical trial in melanoma patients (22), which has then triggered Phase III study programs in Europe and in the United States. Tumor necrosis factor and IL2 are synergistic and complementary also from a pharmacodynamic viewpoint. TNF damages the tumor endothelium leading to a rapid hemorrhagic necrosis of the neoplastic mass (4, 6, 25), while IL2 mainly acts by activating NK cells and CD8+ lymphocytes (6, 24, 26).
The synergistic action of IL2 and TNF has stimulated research activities, aimed at incorporating both payloads into the same therapeutic product. We have previously described the production and anti-cancer activity of a dual-cytokine fusion protein (termed IL2-F8-TNFmut), which exhibited a selective accumulation at the tumor site following intravenous administration and a potent anti-cancer activity, particularly against murine soft-tissue sarcomas (6). The therapeutic action of IL2-F8-TNFmut could be potentiated by combination with immune checkpoint inhibitors (27).
Here, were report the cloning, production and characterization (in vitro and in vivo) of a novel fusion protein (termed IL2-XE114-TNFmut), capable of recognizing carbonic anhydrase IX (CAIX) and of simultaneously displaying IL2 and a de-potentiated TNF mutant (TNFmut). CAIX is a membrane protein, which is overexpressed in hypoxia conditions and in various cancer types, including renal cell carcinomas (RCC), urothelial, colorectal, stomach, pancreas, and other cancers (28, 29). This antigen is virtually undetectable in most normal adult tissues, exception made for certain gastrointestinal structures (29). CAIX has been targeted in vivo using both antibody- and small molecule-based products, showing interesting results in imaging studies (30–32).
The product was active in vitro and in vivo and may represent a candidate for the immunotherapy of renal cell carcinoma.
Materials and Methods
Tumor Cell Lines
The human renal cell carcinoma cell line SKRC52 was kindly provided by Professor E. Oosterwijk (Radbound University Nijmegen Medical Center, Nijmegen, the Netherlands). Transfected CT26-CAIX cells were prepared as previously reported (30). CHO cells, CTLL2 cells and L-M fibroblasts were obtained from the ATCC. Cell lines were received between 2017 and 2019, expanded, and stored as cryopreserved aliquots in liquid nitrogen. Cells were grown according the supplier's protocol and kept in culture for no longer than 14 passages. Authentication of the cell lines also including check of post-freeze viability, growth properties, and morphology, test for mycoplasma contamination, isoenzyme assay, and sterility test were performed by the cell bank before shipment.
Mice and Tumor Models
Six to eight-week-old female BALB/c nude mice were obtained from Janvier Labs. Tumor cells were implanted subcutaneously in the flank using 1 × 107 cells (SKRC52), 3 × 106 cells (CT26-CAIX).
Cloning, Expression, and Protein Purification
The fusion protein IL2-XE114-TNFmut contains the antibody XE114 (31) fused to a mutated version of human TNFα (arginine to alanine mutation in the amino acid position 108 of the human TNF gene, corresponding to the position 32 in the soluble form) at the C-terminus by a 15-amino acid linker and to human IL2 at the N-terminus by a 12-amino acid linker (6). The gene encoding for the XE114 antibody and the gene encoding human TNF and human IL2 were PCR amplified, PCR assembled, and cloned into the mammalian expression vector pcDNA3.1(+) (Invitrogen) by a NheI/NotI restriction site as described previously (6).
The fusion proteins used in this study were expressed using transient gene expression in CHO cells as described previously (33, 34) and purified from the cell culture medium to homogeneity by Protein A (Sino Biological) chromatography.
In vitro Characterization
Purified proteins were analyzed by size-exclusion chromatography on a Superdex 200 increase 10/300 GL column on an ÄKTA FPLC (GE Healthcare, Amersham Biosciences). SDS-PAGE was performed with 10% gels (Invitrogen) under reducing and non-reducing conditions. For ESI-MS analysis samples were diluted to about 0.1 mg/mL and LC-MS was performed on a Waters Xevo G2XS Qtof instrument (ESI-ToF-MS) coupled to a Waters Acquity UPLC H-Class System using a 2.1 × 50 mm Acquity BEH300 C4 1.7 μm column (Waters). Differential scanning fluorimetry was performed on an Applied Biosystems StepOnePlus RT-PCR instrument. Protein samples were diluted at 2 μM in PBS in 40 μL and placed in PCR tubes, assay was performed in triplicates. 5x SYPRO ORANGE (Invitrogen, stock 5000x) was added to samples prior to analysis. For thermal stability measurements, the temperature range spanned from 25 to 95°C with a scan rate of 1°C/min. Data analysis was performed in Protein Thermal Shift™ Software version 1.3. The temperature derivative of the melting curve was computed.
Affinity Measurements
Affinity measurements were performed by surface plasmon resonance using BIAcore X100 (BIAcore, GE Healthcare) instrument using a biotinylated CAIX coated streptavidin chip. Samples were injected as serial-dilutions, in a concentration range from 1 mM to 62.5 nM. Regeneration of the chip was performed by HCl 10 mM.
In vitro Biological Activities
The biological activity of TNF was determined by incubation with mouse LM fibroblasts, in the presence of 2 μg/mL actinomycin D (Sigma-Aldrich). In 96-well plates, cells (20,000 per well) were incubated in medium supplemented with actinomycin D and varying concentrations of recombinant human TNF or IL2-XE114-TNFmut. After 24 h at 37°C, cell viability was determined with Cell Titer Aqueous One Solution (Promega). Results were expressed as the percentage of cell viability compared to cells treated with actinomycin D only.
The biological activity of IL2 was determined by its ability to stimulate the proliferation of CTLL2 cells. Cells (25,000 per well) were seeded in 96-well plates in the culture medium supplemented with varying concentrations of the fusion proteins. After incubation at 37°C for 48 h, cell proliferation was determined with Cell Titer Aqueous One Solution (Promega). Results were expressed as the percentage of cell viability compared to untreated cells.
Flow Cytometry
Antigen expression on SKRC52 cells was confirmed by flow cytometry. Cells were centrifuged and washed in cold FACS buffer (0.5% BSA, 2 mM EDTA in PBS) and stained with IL2-XE114-TNFmut (final concentration 10 μg/mL) and detected with rat anti-IL2 (eBioscience 14-7029-85) followed by staining with anti-rat AlexaFluor488 (Invitrogen A21208). IL2-KSF-TNFmut (specific for an irrelevant antigen) was used as negative control.
Immunofluorescence Studies
Antigen expression was confirmed on ice-cold acetone fixed 8 μm cryostat sections of SKRC52 and CT26-CAIX stained with IL2-XE114-TNFmut and IL2-F8-TNFmut (final concentration 5 μg/mL) and detected with rat anti-IL2 (eBioscience 14-7029-85) and anti-rat AlexaFluor488 (Invitrogen A21208). For vascular staining goat anti-CD31 (R&D AF3628) and anti-goat AlexaFluor594 (Invitrogen A11058) antibodies were used. IL2-KSF-TNFmut (specific for an irrelevant antigen) was used as negative control. Slides were mounted with fluorescent mounting medium and analyzed with Axioskop2 mot plus microscope (Zeiss).
For ex vivo immunofluorescence analysis, mice were injected with 50–60 μg IL2-XE114-TNFmut, IL2-F8-TNFmut, or IL2-KSF-TNFmut and sacrificed 24 h after injection. Organs were excised and embedded in cryo-embedding medium (Thermo Scientific) and cryostat section (10 μm) were stained using the following antibodies: rat anti-IL2 (eBioscience 14-7029-85) and anti-rat AlexaFluor488 (Invitrogen A21208). For vascular staining goat anti-CD31 (R&D AF3628) and anti-goat AlexaFluor594 (Invitrogen A11058) antibodies were used. Slides were mounted with fluorescent mounting medium and analyzed with Axioskop2 mot plus microscope (Zeiss).
Mice Therapy Studies
Mice were monitored daily and tumor volume was measured with a caliper (volume = length × width2 × 0.5). When tumors reached a suitable volume (~70–100 mm3), mice were injected into the lateral tail vein with the pharmacological agents. Fusion proteins were dissolved in PBS, also used as negative control, and administered at 30 μg four times every 24 h. Results are expressed as tumor volume in mm3 ± SEM and % mean body weight change ± SEM. For therapy experiments n = 5 mice/group.
Non-human Primate Study
The non-human primate study was performed in accordance with the Directive 2010/63/UE of the European parliament and of the council of 22 September 2010 for the protection of animals used for scientific purposes. Approval for the test site of experimentation: No. E 18-023-01. A total of 3 male naïve Macaca fascicularis (Cynomolgus monkey, Old Java monkey), ~30 months at the time of allocation and estimated to weigh between 2.5 and 4.0 kg were used in this study. Test items were administered by bolus intravenous injection in the cephalic or saphenous vein, at a dose volume of 0.5 mL/kg body weight (corresponding to 0.1 mg/kg), over a period of ~30 s. A flush with 1 mL of physiological saline was administered at the end of the bolus injection. The dose was administered to each animal on the basis of the body weight measured on the day of administration. Blood samples of ~1 mL each were collected from the saphenous or cephalic vein (alternatively from other blood vessels) of all animals at approximately the following 7 time points: before dosing and at 1, 15, and 30 min and 1, 2, and 4 h after treatment. Samples were transferred into serum separator tubes, kept for 30 min in an upright position then centrifuged at room temperature (2,500 g for 10 min) and the serum divided into two polypropylene tubes. Tubes were frozen within 90 min post blood sampling and stored at −80 ± 10°C.
Pharmacokinetics Analysis
Fusion protein concentrations in serum were assessed by AlphaLISA. Briefly, Streptavidin Donor Beads were coated with biotinylated antigen (CAIX for IL2-XE114-TNFmut or EDA for IL2-F8-TNFmut). Acceptor Beads coated with an anti-TNF antibody were used for detection.
Statistical Analysis
Data were analyzed using Prism 7.0 (GraphPad Software, Inc.). Differences in tumor volume between therapeutic groups (until day 14, when n = 5) were evaluated with the two-way ANOVA followed by Bonferroni as post-test. P < 0.05 was considered statistically significant (*P < 0.05, **P < 0.01, ***P < 0.001, ****P < 0.0001).
Results
Figure 1A depicts a schematic representation of a fully-human fusion protein (termed IL2-XE114-TNFmut), featuring a sequential arrangement of IL2, a scFv fragment specific to CAIX [named XE114 (31)] and TNF (Supplementary Figure 1). The protein arrangement is reminiscent of the one previously described for the murine fusion protein IL2-F8-TNFmut (6, 27) (Supplementary Figure 2), but here we used human payloads in order to facilitate clinical translational activities. The TNF moiety was de-potentiated by a single amino acid substitution (R431A), in order to achieve a similar cytokine activity for both IL2 and TNF moieties. ScFv(XE114) has previously been shown to recognize human CAIX with high affinity and kinetic stability (31, 35). IL2-XE114-TNFmut was expressed in mammalian cells and could be purified on Protein A, since the scFv moiety featured a VH domain of the VH3 family (36, 37). The product formed stable non-covalent homotrimers in solution (Figure 1B), as TNF is a trimeric protein, and bound avidly to the cognate antigen in BIAcore assays (Figure 1C). The protein was mainly in a non-glycosylated form, but ~10% of IL2-XE114-TNFmut exhibited a molecular weight increase of 657 Dalton, as a result of O-linked glycosylation (Figure 1D). The product retained intact IL2 activity in an in vitro lymphocyte proliferation assay (Figure 1E), while TNF potency was reduced by ~10-fold, as a result of a single amino acid substitution (6) (Figure 1F). A single band could be detected in SDS-PAGE analysis, both in reducing and in non-reducing conditions (Figure 1G). IL2-XE114-TNFmut bound to SKRC52 renal cell carcinoma cell lines more intensely than IL2-KSF-TNFmut (Figure 1H), which was specific to hen egg lysozyme and was chosen as a negative control of irrelevant specificity in the mouse (38) (Supplementary Figure 3). A multi-step denaturation profile was observed by differential scanning fluorimetry, with a first transition at 49.5°C (Figure 1I).
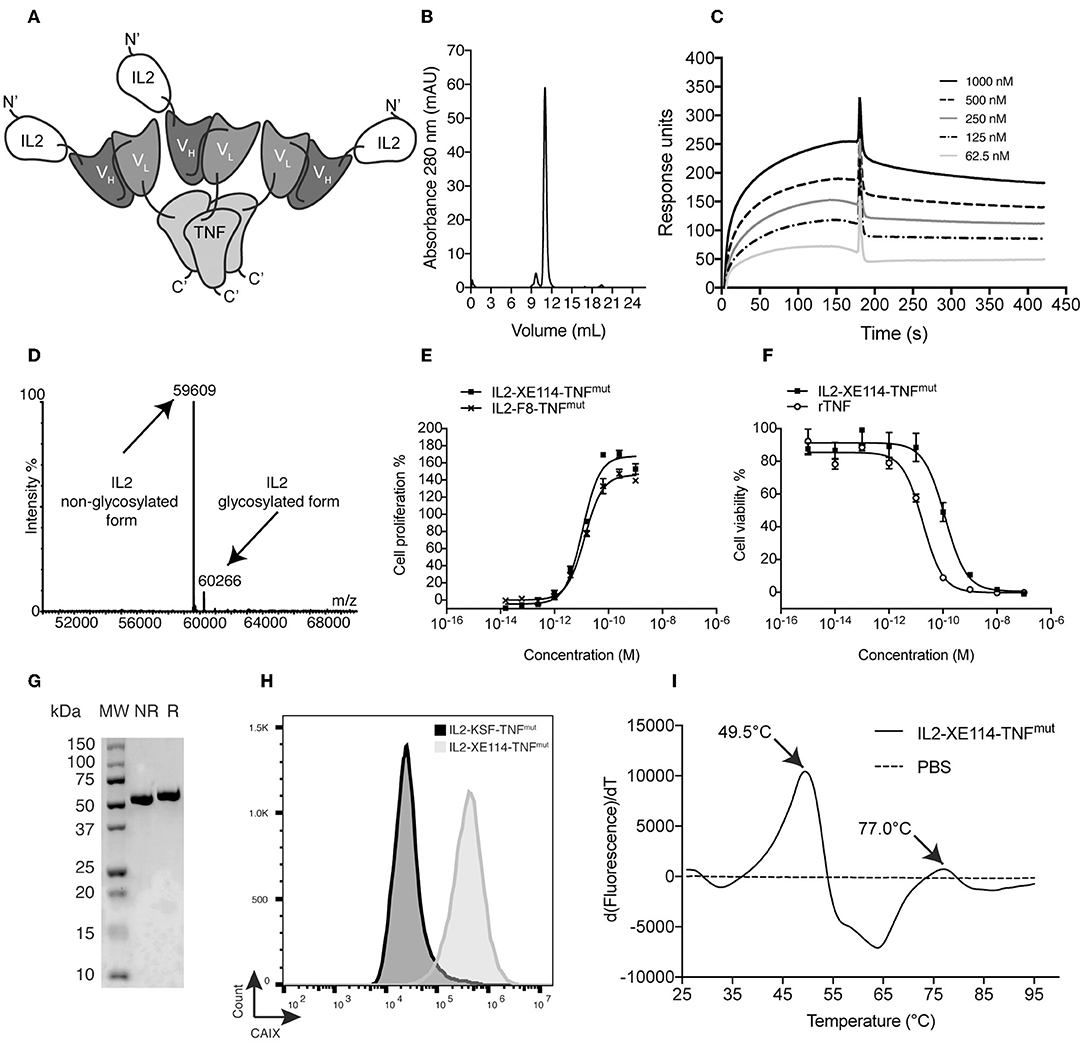
Figure 1. Biochemical characterization of IL2-XE114-TNFmut. (A) Schematic representation of the domain assembly of IL2-XE114-TNFmut in non-covalent homotrimeric format. (B) Size exclusion chromatography profile. (C) BIAcore analysis on CAIX-coated sensor chip. (D) ESI-MS profile. (E) IL2 bioactivity assay on CTLL2 cells. (F) TNF bioactivity assay on L-M fibroblasts. (G) SDS-PAGE analysis; MW, molecular weight; NR, non-reducing conditions; R, reducing conditions. (H) Flow cytometric evaluation of CAIX expression by SKRC52 cells, detected with IL2-XE114-TNFmut. (I) Differential Scanning Fluorimetry on IL2-XE114-TNFmut.
The tumor-homing properties of IL2-XE114-TNFmut were first studied in nude mice, bearing subcutaneously-grafted human SKRC52 renal cell carcinomas (Figure 2). Organs were examined 24 h after intravenous administration of 60 μg of fusion protein, using an immunofluorescence procedure for the detection of the IL2 moiety. A homogenous antigen expression pattern was observed in an in vitro analysis of tumor sections. However, ex vivo, IL2-XE114-TNFmut mainly localized to perivascular tumor cells and failed to homogenously stain the CAIX-positive tumor mass. Similar targeting behaviors have previously been reported for other antibody products, directed against cell surface tumor antigens (31, 39). No detectable antibody uptake could be seen in relevant normal organs (Figure 2). By contrast, no tumor staining and no tumor uptake could be observed for the IL2-KSF-TNFmut negative control protein (Figure 2B).
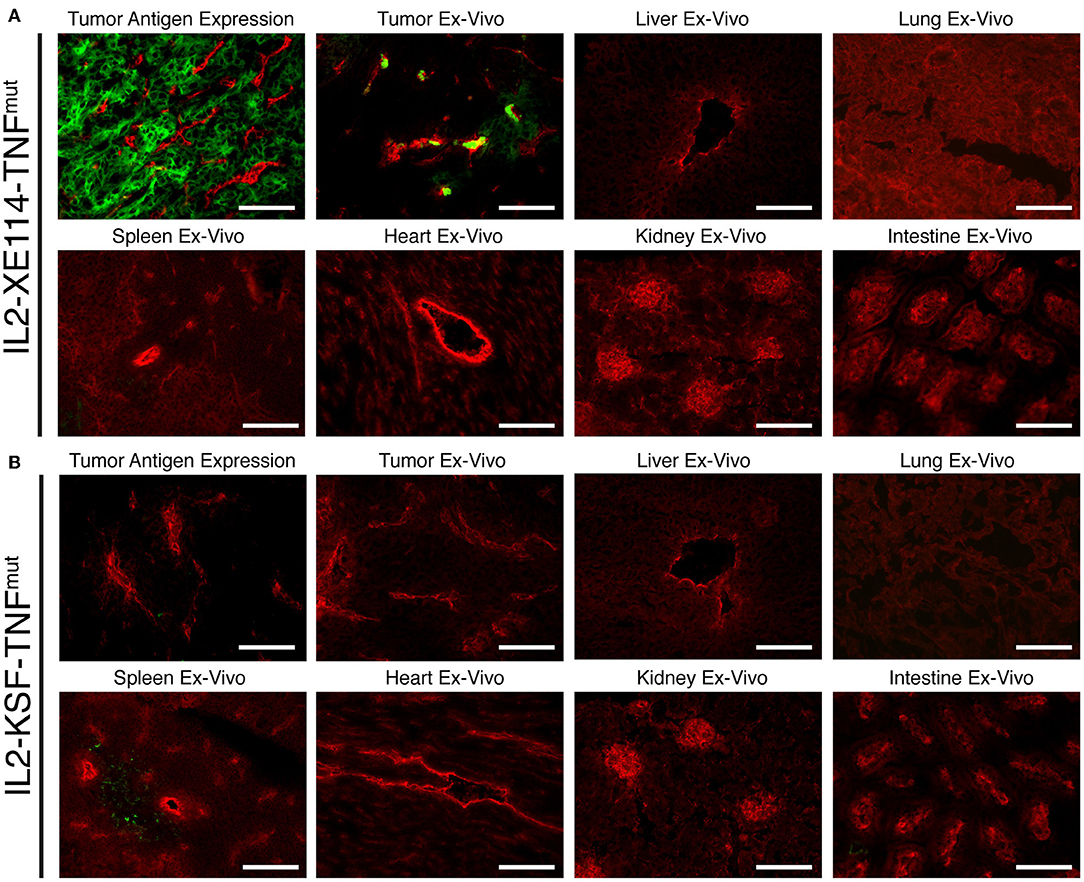
Figure 2. Antigen expression and tumor targeting properties of IL2-XE114-TNFmut. Microscopic fluorescence analysis of CAIX expression on SKRC52 tumor sections (A,B upper left) detected with IL2-XE114-TNFmut or IL2-KSF-TNFmut (negative control). Microscopic fluorescence analysis of organs from SKRC52 tumor bearing mice, 24 h after intravenous administration of IL2-XE114-TNFmut (A) or IL2-KSF-TNFmut (B). Cryosections were stained with anti-IL2 (green, AlexaFluor 488) and anti-CD31 (red, AlexaFluor 594). 20x magnification, scale bars = 100 μm.
In order to get a finer characterization of the tumor-targeting properties of our products, we compared IL2-XE114-TNFmut and IL2-F8-TNFmut (an analog specific to the alternatively-spliced EDA domain of fibronectin) (Supplementary Figure 4) in mice bearing murine CT26 tumors, that had been stably-transfected for CAIX expression on the cell surface (30). Both products exhibited a preferential accumulation at the tumor site, while IL2-KSF-TNFmut failed to localize to the neoplastic mass. However, the IL2-F8-TNFmut yielded a more homogenous pattern of tumor uptake compared to IL2-XE114-TNFmut, in spite of the fact that CAIX was strongly expressed on all tumor cells (Figure 3).
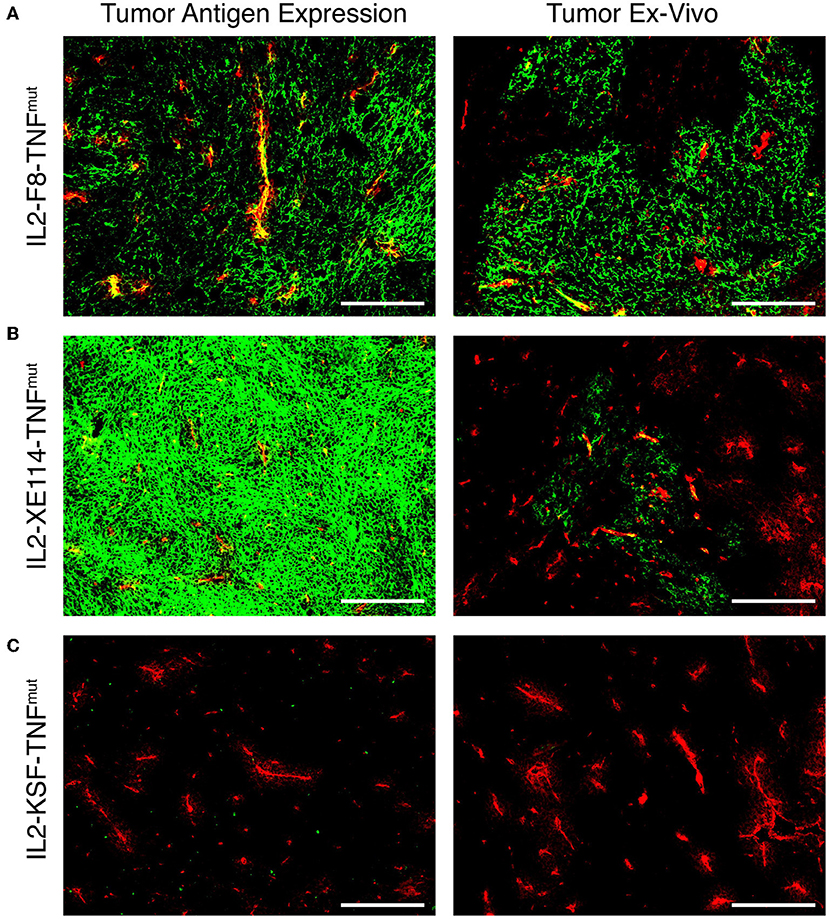
Figure 3. Antigen expression and tumor targeting properties of IL2-XE114-TNFmut. Microscopic fluorescence analysis of CAIX expression on CT26-CAIX tumor sections (A–C) detected with IL2-F8-TNFmut, IL2-XE114-TNFmut, or IL2-KSF-TNFmut (negative control). Microscopic fluorescence analysis of tumors from CT26-CAIX tumor bearing mice, 24 h after intravenous administration of IL2-F8-TNFmut (A), IL2-XE114-TNFmut (B), or IL2-KSF-TNFmut (C). Cryosections were stained with anti-IL2 (green, AlexaFluor 488) and anti-CD31 (red, AlexaFluor 594). 10x magnification, scale bars = 200 μm.
We tested the therapeutic activity of IL2-XE114-TNFmut and IL2-F8-TNFmut in nude mice bearing CAIX-transfected CT26 tumors (Figure 4). Since the human TNF moiety is only partly active in mice, we also studied the therapeutic activity of the mIL2-XE114-mTNFmut and mIL2-F8-mTNFmut analogs, bearing an attenuated version of murine TNF (6, 27) (Supplementary Figures 2, 5). A tumor-growth retardation was observed in this model, which lacked a functional set of T lymphocytes, but still retained natural killer (NK) cells (Figure 4). All products caused a transient reduction in body weight at the dose used (30 μg), which was below the 10% threshold.
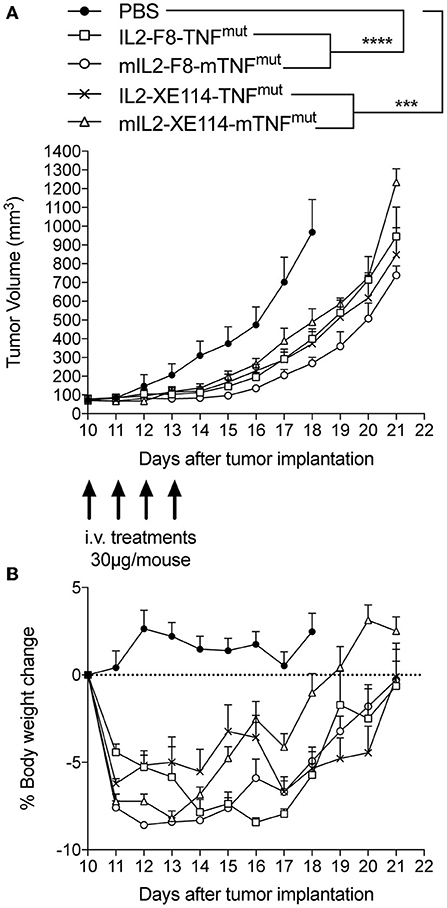
Figure 4. Therapeutic performance of IL2-XE114-TNFmut in BALB/c nude mice bearing CT26-CAIX tumors in comparison with IL2-F8-TNFmut and their murine surrogates (mIL2-XE114-mTNFmut, mIL2-F8-mTNFmut). Treatment started when tumors reached a volume of 70–100 mm3, mice were injected intravenously four times every 24 h with 30 μg of fusion proteins. Results are expressed as tumor volume in mm3 ± SEM (A) and % mean body weight change ± SEM (B). For therapy experiments n = 5 mice/group. n = 4 for group IL2-XE114-TNFmut from day 15. n = 4 for groups mIL2-XE114-mTNFmut and mIL2-F8-mTNFmut from day 19. n = 3 for group mIL2-F8-mTNFmut from day 20 (***P < 0.001, ****P < 0.0001).
Finally, we compared the pharmacokinetic profiles of IL2-XE114-TNFmut and IL2-F8-TNFmut in Cynomolgus monkey, after a single intravenous administration (0.1 mg/kg) (Figure 5). IL2-F8-TNFmut showed a biphasic clearance profile, with a rapid loss of ~2/3 of the protein from circulation, followed by a slower elimination phase. By contrast, IL2-XE114-TNFmut exhibited a slower clearance profile, with a half-life of ~2 h. This pharmacokinetic profile is similar to the one that we have previously observed for other antibody-cytokine fusion proteins, which have progressed to advanced clinical trials (13, 18, 40, 41).
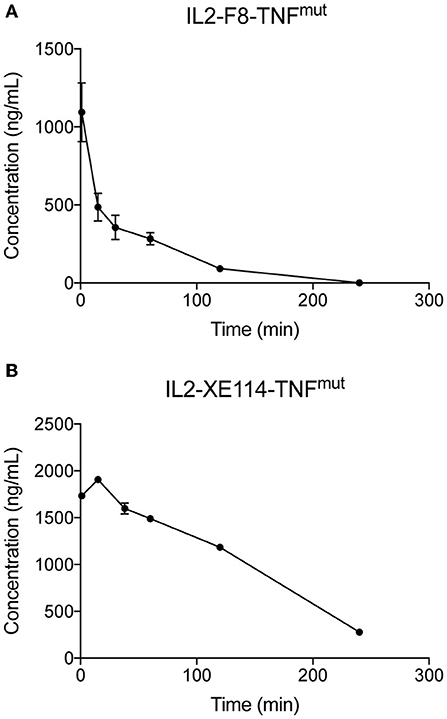
Figure 5. Pharmacokinetics in cynomolgus monkeys. Pharmacokinetics were evaluated in one cynomolgus monkey per group injected once at the dose of 0.1 mg/kg of IL2-F8-TNFmut (A) or IL2-XE114-TNFmut (B). Blood samples were collected before dosing and at 1, 15, and 30 min and 1, 2, and 4 h after treatment.
Discussion
In this work we have described the generation, the in vitro characterization and the in vivo validation of a novel “potency-matched dual-cytokine antibody fusion protein” based on an anti-CAIX antibody fragment simultaneously fused to human IL2 and to a mutant version of human TNF. The product, named IL2-XE114-TNFmut, could be expressed in mammalian cells and purified to homogeneity. The fusion protein was able to recognize CAIX both in vitro and in vivo, when tested on a human renal cell carcinoma cell line (SKRC52, which naturally express the antigen) and in a murine colon carcinoma cell line (CT26-CAIX, which had been transfected with the human antigen).
We have previously reported that the intralesional administration of two immunocytokine products (L19-IL2 and L19-TNF) was able to induce cancer remission both in mouse models of cancer (24) and in patients with stage IIIB/C melanoma (22). However, from an industrial prospective, the development of combination products may cause a duplication of activities and costs. By contrast, the opportunity of incorporate two cytokine payloads into the same antibody moiety may facilitate industrial development.
IL2- and TNF-based products have shown to be synergistically active against various type of malignancies (4, 6, 8, 22–25, 27, 42) by two distinct complementary mechanism of actions. On one hand, TNF is capable of inducing hemorrhagic necrosis and apoptosis of the tumor endothelial cells and also of cancer cells (4, 6, 25, 43, 44). On the other end, IL2 is able to promote a selective boosting of T cell and NK cell activity against cancer cells (6, 24, 26, 45).
The therapeutic activity of IL2-XE114-TNFmut was evaluated in immunocompromised mice bearing human CAIX-transfected CT26 murine tumors. This mouse model, which lacked a functional set of T lymphocytes, but still retained NK cells, was chosen in order to avoid an immune response against the transfected human antigen. This may explain the reason why only an initial tumor growth inhibition was observed. We had previously reported that depletion studies in immunocompetent mice treated with IL2-F8-TNFmut revealed a prominent role of CD4+ and CD8+ T cells in the cancer remission process (6).
Antibodies against cell surface antigens can recognize their cognate antigen with exquisite specificity, but often their penetration into solid malignancies can be suboptimal as a result of a slow extravasation rate due to their relatively large size (39, 46). Indeed, IL2-XE114-TNFmut was found to preferentially localize to perivascular cells in vivo. Similar findings had been reported for antibodies specific to HER2 (39). Interestingly, it has been shown that a small molecule against CAIX could penetrate CAIX-positive tumors more efficiently compared to an antibody against the same target (31).
In spite of a suboptimal penetration in neoplastic lesions, IL2-XE114-TNFmut revealed an acceptable clearance profile, with a half-life of ~2 h in monkeys, which was more favorable compared to the one of IL2-F8-TNFmut. This pharmacokinetic profile is comparable to the one previously observed for other cytokine-fusion proteins based on antibody-fragments (40, 47). By contrast, IL2-F8-TNFmut exhibited a rapid clearance from circulation already at early time-points, possibly indicating a trapping of the F8 antibody in the liver, which was already observed for other F8-based immunocytokines (48).
Renal cell carcinoma (RCC) represents a rare condition that account for ~2% of cancer deaths worldwide (49). The most prominent subtype of RCC (about 70%) is clear cell (ccRCC) (50). In most cases (70%) the tumor is confined to the kidney, but it may disseminate to regional lymph nodes and to visceral organs (50). Surgical resection is the primary treatment option for stage I-III RCC, but postsurgical recurrence is observed with a 5-year relapse rate of 30–40% in patients with stage II or III RCC (51). In the event of recurrence patients are typically treated with conventional chemotherapy (e.g., sunitinib), high dose IL2 or immune-checkpoint inhibitors (e.g., nivolumab and ipilimumab) (52, 53). A phase III clinical trials in patients with advanced renal-cell carcinoma showed that the combination of nivolumab and ipilimumab could increase the overall survival compared to sunitinib alone (53). However, this efficacy was observed only in a small portion of patients, for this reason novel therapeutics for the treatment of RCC may be required.
CAIX is strongly expressed in the majority of ccRCC. The antigen has been extensively validated as a target for ccRCC in preclinical studies and several antibodies against this antigen have been developed by our group and others (54, 55). An humanized monoclonal antibody, named G250 (56) has been used to validate CAIX as a cancer target by nuclear medicine in clinical studies (50, 57). The XE114 antibody fragment used in this study is a fully-human high-affinity antibody (31), which may be considered for clinical development of CAIX-targeted based therapeutics.
The product presented in this study (IL2-XE114-TNFmut) was able to target CAIX in tumor bearing mice and showed a therapeutic effect in immunocompromised animals. Moreover, the favorable pharmacokinetic profile in monkey provide a rational for future clinical investigation. The targeted delivery of cytokine payloads to cell surface antigens may represent a valid alternative to the anchoring of pro-inflammatory payloads to the tumor extracellular matrix. To a certain extent, an immunocytokine able to selectively localize to tumor cell membrane may represent a functional equivalent to a “bispecific antibody” and may be capable of cross-link a tumor cell with a leukocyte (e.g., NK cell, T cell), which displays the cognate cytokine receptor on its surface.
Data Availability Statement
The datasets generated for this study are available on request to the corresponding author.
Ethics Statement
Mice experiments were performed under a project license (license number 04/2018) granted by the Veterinäramt des Kantons Zürich, Switzerland, in compliance with the Swiss Animal Protection Act (TSchG) and the Swiss Animal Protection Ordinance (TSchV). The non-human primate study was performed in accordance with the Directive 2010/63/UE of the European parliament and of the council of 22 September 2010 for the protection of animals used for scientific purposes. Approval for the test site of experimentation: No. E 18-023-01. Written informed consent was obtained from the owners for the participation of their animals in this study.
Author Contributions
RD and DN: conception and design, development of methodology, acquisition, analysis and interpretation of data, writing, review and revision of the manuscript, and study supervision. AV and SG: analysis and interpretation of data. BG, TO, BZ, AS, GB, and MM: technical support.
Funding
We gratefully acknowledge funding from ETH Zürich and the Swiss National Science Foundation (Grant No. 310030_182003/1). This project has received funding from the European Research Council (ERC) under the European Union's Horizon 2020 research and innovation program (grant agreement 670603).
Conflict of Interest
DN is co-founder, shareholder and member of the board of Philogen, a company working on antibody therapeutics.
The remaining authors declare that the research was conducted in the absence of any commercial or financial relationships that could be construed as a potential conflict of interest.
Acknowledgments
We would like to thank Dr. Samuele Cazzamalli for helpful discussions and we gratefully acknowledge Lisa Nadal and Riccardo Corbellari for their help with experimental procedures.
Supplementary Material
The Supplementary Material for this article can be found online at: https://www.frontiersin.org/articles/10.3389/fonc.2019.01228/full#supplementary-material
References
1. Pasche N, Neri D. Immunocytokines: a novel class of potent armed antibodies. Drug Discov Today. (2012) 17:583–90. doi: 10.1016/j.drudis.2012.01.007
2. Neri D. Antibody–cytokine fusions: versatile products for the modulation of anticancer immunity. Cancer Immunol Res. (2019) 7:348–54. doi: 10.1158/2326-6066.CIR-18-0622
3. Neri D, Sondel PM. Immunocytokines for cancer treatment: past, present and future. Curr Opin Immunol. (2016) 40:96–102. doi: 10.1016/j.coi.2016.03.006
4. Probst P, Kopp J, Oxenius A, Colombo MP, Ritz D, Fugmann T, et al. Sarcoma eradication by doxorubicin and targeted TNF relies upon CD8 + T-cell recognition of a retroviral antigen. Cancer Res. (2017) 77:3644–54. doi: 10.1158/0008-5472.CAN-16-2946
5. Mosely SIS, Prime JE, Sainson RCA, Koopmann J-O, Wang DYQ, Greenawalt DM, et al. Rational selection of syngeneic preclinical tumor models for immunotherapeutic drug discovery. Cancer Immunol Res. (2017) 5:29–41. doi: 10.1158/2326-6066.cir-16-0114
6. De Luca R, Soltermann A, Pretto F, Pemberton-Ross C, Pellegrini G, Wulhfard S, et al. Potency-matched dual cytokine–antibody fusion proteins for cancer therapy. Mol Cancer Ther. (2017) 16:2329–39. doi: 10.1158/1535-7163.mct-17-0211
7. Pasche N, Wulhfard S, Pretto F, Carugati E, Neri D. The antibody-based delivery of interleukin-12 to the tumor neovasculature eradicates murine models of cancer in combination with paclitaxel. Clin Cancer Res. (2012) 18: 4092–103. doi: 10.1158/1078-0432.CCR-12-0282
8. Carnemolla B, Borsi L, Balza E, Castellani P, Meazza R, Berndt A, et al. Enhancement of the antitumor properties of interleukin-2 by its targeted delivery to the tumor blood vessel extracellular matrix. Blood. (2002) 99:1659–65. doi: 10.1182/blood.V99.5.1659
9. Johannsen M, Spitaleri G, Curigliano G, Roigas J, Weikert S, Kempkensteffen C, et al. The tumour-targeting human L19-IL2 immunocytokine: Preclinical safety studies, phase I clinical trial in patients with solid tumours and expansion into patients with advanced renal cell carcinoma. Eur J Cancer. (2010) 46:2926–35. doi: 10.1016/j.ejca.2010.07.033
10. Halin C, Rondini S, Nilsson F, Berndt A, Kosmehl H, Zardi L, et al. Enhancement of the antitumor activity of interleukin-12 by targeted delivery to neovasculature. Nat Biotechnol. (2002) 20:264–9. doi: 10.1038/nbt0302-264
11. Osenga KL, Hank JA, Albertini MR, Can J, Sternberg AG, Eickhoff J, et al. A phase I clinical trial of the hu14.18-IL2. (EMD 273063) as a treatment for children with refractory or recurrent neuroblastoma and melanoma: a study of the Children's Oncology Group. Clin Cancer Res. (2006) 12:1657. doi: 10.1158/1078-0432.CCR-05-2000
12. Connor JP, Cristea MC, Lewis NL, Lewis LD, Komarnitsky PB, Mattiacci MR, et al. A phase 1b study of humanized KS-interleukin-2. (huKS-IL2) immunocytokine with cyclophosphamide in patients with EpCAM-positive advanced solid tumors. BMC Cancer. (2013) 13:20. doi: 10.1186/1471-2407-13-20
13. Eigentler TK, Weide B, De Braud F, Spitaleri G, Romanini A, Pflugfelder A, et al. A dose-escalation and signal-generating study of the immunocytokine L19-IL2 in combination with dacarbazine for the therapy of patients with metastatic melanoma. Clin Cancer Res. (2011) 17:7732–42. doi: 10.1158/1078-0432.CCR-11-1203
14. Catania C, Maur M, Berardi R, Rocca A, Di Giacomo AM, Spitaleri G, et al. The tumor-targeting immunocytokine F16-IL2 in combination with doxorubicin: dose escalation in patients with advanced solid tumors and expansion into patients with metastatic breast cancer. Cell Adhes Migr. (2015) 9:14–21. doi: 10.4161/19336918.2014.983785
15. Klein C, Waldhauer I, Nicolini VG, Freimoser-Grundschober A, Nayak T, Vugts DJ, et al. Cergutuzumab amunaleukin. (CEA-IL2v), a CEA-targeted IL-2 variant-based immunocytokine for combination cancer immunotherapy: overcoming limitations of aldesleukin and conventional IL-2-based immunocytokines. Oncoimmunology. (2017) 6:e1277306. doi: 10.1080/2162402X.2016.1277306
16. van den Heuvel MM, Verheij M, Boshuizen R, Belderbos J, Dingemans AMC, De Ruysscher D, et al. NHS-IL2 combined with radiotherapy: preclinical rationale and phase Ib trial results in metastatic non-small cell lung cancer following first-line chemotherapy. J Transl Med. (2015) 13:32. doi: 10.1186/s12967-015-0397-0
17. Bachanova V, Lansigan F, Quick DP, Vlock D, Gillies S, Nakamura R. Remission induction in a phase I/II study of an anti-CD20-interleukin-2 immunocytokine DI-Leu16-IL2 in patients with relapsed B-cell lymphoma. Blood. (2015). 126:1533. doi: 10.1182/blood.V126.23.1533.1533
18. Spitaleri G, Berardi R, Pierantoni C, De Pas T, Noberasco C, Libbra C, et al. Phase I/II study of the tumour-targeting human monoclonal antibody-cytokine fusion protein L19-TNF in patients with advanced solid tumours. J Cancer Res Clin Oncol. (2013) 139:447–55. doi: 10.1007/s00432-012-1327-7
19. Rudman SM, Jameson MB, McKeage MJ, Savage P, Jodrell DI, Harries M, et al. A phase 1 study of AS1409, a novel antibody-cytokine fusion protein, in patients with malignant melanoma or renal cell carcinoma. Clin Cancer Res. (2011) 17:1998–2005. doi: 10.1158/1078-0432.CCR-10-2490
20. Strauss J, Heery CR, Kim JW, Jochems C, Donahue RN, Montgomery AS, et al. First-in-human phase I trial of a tumor-targeted cytokine. (NHS-IL12) in subjects with metastatic solid tumors. Clin Cancer Res. (2019) 25:99–109. doi: 10.1158/1078-0432.CCR-18-1512
21. Claus C, Ferrara C, Xu W, Sam J, Lang S, Uhlenbrock F, et al. Tumor-targeted 4-1BB agonists for combination with T cell bispecific antibodies as off-the-shelf therapy. Sci Transl Med. (2019) 11:eaav5989. doi: 10.1126/scitranslmed.aav5989
22. Danielli R, Patuzzo R, Di Giacomo AM, Gallino G, Maurichi A, Di Florio A, et al. Intralesional administration of L19-IL2/L19-TNF in stage III or stage IVM1a melanoma patients: results of a phase II study. Cancer Immunol Immunother. (2015) 64:999–1009. doi: 10.1007/s00262-015-1704-6
23. Balza E, Carnemolla B, Mortara L, Castellani P, Soncini D, Accolla RS, et al. Therapy-induced antitumor vaccination in neuroblastomas by the combined targeting of IL-2 and TNFα. Int J Cancer. (2010) 127:101–10. doi: 10.1002/ijc.25018
24. Schwager K, Hemmerle T, Aebischer D, Neri D. The immunocytokine L19-IL2 eradicates cancer when used in combination with CTLA-4 blockade or with L19-TNF. J Invest Dermatol. (2013) 133:751–8. doi: 10.1038/jid.2012.376
25. Hemmerle T, Probst P, Giovannoni L, Green AJ, Meyer T, Neri D. The antibody-based targeted delivery of TNF in combination with doxorubicin eradicates sarcomas in mice and confers protective immunity. Br J Cancer. (2013) 109:1206–13. doi: 10.1038/bjc.2013.421
26. Hutmacher C, Gonzalo Núñez N, Liuzzi AR, Becher B, Neri D. Targeted delivery of IL2 to the tumor stroma potentiates the action of immune checkpoint inhibitors by preferential activation of NK and CD8 + T cells. Cancer Immunol Res. (2019) 7:572–58. doi: 10.1158/2326-6066.cir-18-0566
27. De Luca R, Neri D. Potentiation of PD-L1 blockade with a potency-matched dual cytokine–antibody fusion protein leads to cancer eradication in BALB/c-derived tumors but not in other mouse strains. Cancer Immunol Immunother. (2018) 67:1381–91. doi: 10.1007/s00262-018-2194-0
28. Lv P-C, Roy J, Putt KS, Low PS. Evaluation of nonpeptidic ligand conjugates for the treatment of hypoxic and carbonic anhydrase IX-expressing cancers. Mol Cancer Ther. (2017) 16:453–60. doi: 10.1158/1535-7163.MCT-16-0537
29. Hilvo M, Rafajová M, Pastoreková S, Pastorek J, Parkkila S. Expression of carbonic anhydrase IX in mouse tissues. J Histochem Cytochem. (2004) 52:1313–22. doi: 10.1369/jhc.3A6225.2004
30. Cazzamalli S, Ziffels B, Widmayer F, Murer P, Pellegrini G, Pretto F, et al. Enhanced therapeutic activity of non-internalizing small-molecule-drug conjugates targeting carbonic anhydrase IX in combination with targeted interleukin-2. Clin Cancer Res. (2018) 24:3656–67. doi: 10.1158/1078-0432.CCR-17-3457
31. Cazzamalli S, Dal Corso A, Widmayer F, Neri D. Chemically defined antibody- and small molecule-drug conjugates for in vivo tumor targeting applications: a comparative analysis. J Am Chem Soc. (2018) 140:1617–21. doi: 10.1021/jacs.7b13361
32. Cazzamalli S, Dal Corso A, Neri D. Acetazolamide serves as selective delivery vehicle for dipeptide-linked drugs to renal cell carcinoma. Mol Cancer Ther. (2016) 15:2926–35. doi: 10.1158/1535-7163.mct-16-0283
33. Pasche N, Woytschak J, Wulhfard S, Villa A, Frey K, Neri D. Cloning and characterization of novel tumor-targeting immunocytokines based on murine IL7. J Biotechnol. (2011) 154:84–92. doi: 10.1016/j.jbiotec.2011.04.003
34. Rajendra Y, Kiseljak D, Baldi L, Hacker DL, Wurm FM. A simple high-yielding process for transient gene expression in CHO cells. J Biotechnol. (2011) 153:22–6. doi: 10.1016/j.jbiotec.2011.03.001
35. Ziffels B, Stringhini M, Probst P, Fugmann T, Sturm T, Neri D. Antibody-based delivery of cytokine payloads to carbonic anhydrase IX leads to cancer cures in immunocompetent tumor-bearing mice. Mol Cancer Ther. (2019) 18:1544–54. doi: 10.1158/1535-7163.MCT-18-1301
36. Hoogenboom HR, Winter G. By-passing immunisation. Human antibodies from synthetic repertoires of germline VH gene segments rearranged in vitro. J Mol Biol. (1992) 227:381–8. doi: 10.1016/0022-2836(92)90894-P
37. Silacci M, Brack S, Schirru G, Mårlind J, Ettorre A, Merlo A, et al. Design, construction, and characterization of a large synthetic human antibody phage display library. Proteomics. (2005) 5:2340–50. doi: 10.1002/pmic.200401273
38. Schwager K, Villa A, Rösli C, Neri D, Rösli-Khabas M, Moser G. A comparative immunofluorescence analysis of three clinical-stage antibodies in head and neck cancer. Head Neck Oncol. (2011) 3:25. doi: 10.1186/1758-3284-3-25
39. Dennis MS, Jin H, Dugger D, Yang R, McFarland L, Ogasawara A, et al. Imaging tumors with an albumin-binding Fab, a novel tumor-targeting agent. Cancer Res. (2007) 67:254–61. doi: 10.1158/0008-5472.CAN-06-2531
40. Poli GL, Bianchi C, Virotta G, Bettini A, Moretti R, Trachsel E, et al. Radretumab radioimmunotherapy in patients with brain metastasis: a 124I-L19SIP dosimetric PET study. Cancer Immunol Res. (2013) 1:134–43. doi: 10.1158/2326-6066.cir-13-0007
41. Papadia F, Basso V, Patuzzo R, Maurichi A, Di Florio A, Zardi L, et al. Isolated limb perfusion with the tumor-targeting human monoclonal antibody-cytokine fusion protein L19-TNF plus melphalan and mild hyperthermia in patients with locally advanced extremity melanoma. J Surg Oncol. (2013) 107:173–9. doi: 10.1002/jso.23168
42. Borsi L, Balza E, Carnemolla B, Sassi F, Castellani P, Berndt A, et al. Selective targeted delivery of TNFα to tumor blood vessels. Blood. (2003) 102:4384–92. doi: 10.1182/blood-2003-04-1039
43. Wang X, Lin Y. Tumor necrosis factor and cancer, buddies or foes? Acta Pharmacol Sin. (2008) 29:1275–88. doi: 10.1111/j.1745-7254.2008.00889.x
44. van Horssen R. TNF- in cancer treatment: molecular insights, antitumor effects, and clinical utility. Oncologist. (2006) 11:397–408. doi: 10.1634/theoncologist.11-4-397
45. Yang RK, Kalogriopoulos NA, Rakhmilevich AL, Ranheim EA, Seo S, Kim K, et al. Intratumoral hu14.18-IL-2. (IC) induces local and systemic antitumor effects that involve both activated T and NK cells as well as enhanced IC retention. J Immunol. (2012) 189:2656–64. doi: 10.4049/jimmunol.1200934
46. Sievers EL, Senter PD. Antibody-drug conjugates in cancer therapy. Annu Rev Med. (2013) 64:15–29. doi: 10.1146/annurev-med-050311-201823
47. Börjesson PKE, Postema EJ, Roos JC, Colnot DR, Marres HAM, van Schie MH, et al. Phase I therapy study with (186)re-labeled humanized monoclonal antibody BIWA 4. (Bivatuzumab) in patients with head and neck squamous cell carcinoma. Clin Cancer Res. (2003) 9(Pt 2):3961S−72.
48. Bruijnen STG, Chandrupatla DMSH, Giovanonni L, Neri D, Vugts DJ, Huisman MC, et al. F8-IL10: a new potential antirheumatic drug evaluated by a PET-guided translational approach. Mol Pharm. (2019) 16:273–81. doi: 10.1021/acs.molpharmaceut.8b00982
49. Ferlay J, Soerjomataram I, Dikshit R, Eser S, Mathers C, Rebelo M, et al. Cancer incidence and mortality worldwide: sources, methods and major patterns in GLOBOCAN 2012. Int J cancer. (2015) 136:E359–86. doi: 10.1002/ijc.29210
50. Oosterwijk-Wakka JC, Boerman OC, Mulders PFAM, Oosterwijk E. Application of monoclonal antibody G250 recognizing carbonic anhydrase IX in renal cell carcinoma. Int J Mol Sci. (2013) 14:11402–23. doi: 10.3390/ijms140611402
51. Janzen NK, Kim HL, Figlin RA, Belldegrun AS. Surveillance after radical or partial nephrectomy for localized renal cell carcinoma and management of recurrent disease. Urol Clin North Am. (2003) 30:843–52. doi: 10.1016/S0094-0143(03)00056-9
52. Escudier B, Porta C, Schmidinger M, Rioux-Leclercq N, Bex A, Khoo V, et al. Renal cell carcinoma: ESMO Clinical Practice Guidelines for diagnosis, treatment and follow-up. Ann Oncol. (2016) 27: v58–68. doi: 10.1093/annonc/mdw328
53. Motzer RJ, Tannir NM, McDermott DF, Arén Frontera O, Melichar B, Choueiri TK, et al. Nivolumab plus ipilimumab versus sunitinib in advanced renal-cell carcinoma. N Engl J Med. (2018) 378:1277–90. doi: 10.1056/NEJMoa1712126
54. Ahlskog JKJ, Schliemann C, Mårlind J, Qureshi U, Ammar A, Pedley RB, et al. Human monoclonal antibodies targeting carbonic anhydrase IX for the molecular imaging of hypoxic regions in solid tumours. Br J Cancer. (2009) 101:645–57. doi: 10.1038/sj.bjc.6605200
55. Murri-Plesko MT, Hulikova A, Oosterwijk E, Scott AM, Zortea A, Harris AL, et al. Antibody inhibiting enzymatic activity of tumour-associated carbonic anhydrase isoform IX. Eur J Pharmacol. (2011) 657:173–83. doi: 10.1016/j.ejphar.2011.01.063
56. Oosterwdk E, Ruiter DJ, Hoedemaeker PJ, Pauwels EKJ, Jonas U, Zwartendijk I, et al. Monoclonal antibody G 250 recognizes a determinant present in renal-cell carcinoma and absent from normal kidney. Int J Cancer. (1986) 38:489–94. doi: 10.1002/ijc.2910380406
Keywords: immunotherapy, antibody-cytokine fusion proteins, IL2, TNF, EDA domain of fibronectin, CAIX
Citation: De Luca R, Gouyou B, Ongaro T, Villa A, Ziffels B, Sannino A, Buttinoni G, Galeazzi S, Mazzacuva M and Neri D (2019) A Novel Fully-Human Potency-Matched Dual Cytokine-Antibody Fusion Protein Targets Carbonic Anhydrase IX in Renal Cell Carcinomas. Front. Oncol. 9:1228. doi: 10.3389/fonc.2019.01228
Received: 23 August 2019; Accepted: 28 October 2019;
Published: 13 November 2019.
Edited by:
Christian Klein, Roche Innovation Center Zurich, SwitzerlandReviewed by:
Roland E. Kontermann, University of Stuttgart, GermanyJyothi Thyagabhavan Mony, Washington University in St. Louis, United States
Copyright © 2019 De Luca, Gouyou, Ongaro, Villa, Ziffels, Sannino, Buttinoni, Galeazzi, Mazzacuva and Neri. This is an open-access article distributed under the terms of the Creative Commons Attribution License (CC BY). The use, distribution or reproduction in other forums is permitted, provided the original author(s) and the copyright owner(s) are credited and that the original publication in this journal is cited, in accordance with accepted academic practice. No use, distribution or reproduction is permitted which does not comply with these terms.
*Correspondence: Roberto De Luca, cm9iZXJ0by5kZWx1Y2FAcGhpbG9nZW4uY29t; Dario Neri, bmVyaUBwaGFybWEuZXRoei5jaA==