- 1Department of Oncology, First Affiliated Hospital, College of Medicine, Zhejiang University, Hangzhou, China
- 2HaploX Biotechnology, Shenzhen, China
- 3Department of Radiotherapy, The Eighth Medical Center of the Chinese PLA General Hospital, Beijing, China
Immunotherapy by immune checkpoint inhibitors (ICIs) has showed outstanding efficacy in the treatment of advanced non-small cell lung cancer (NSCLC). The combination of immunotherapy with anti-angiogenic therapy exhibited enhanced efficacy in multiline treatment. However, the potential biomarkers for predicting and monitoring the therapeutic response of the combined therapy remain undefined. In this study, we performed a pilot study by prospectively recruiting 22 advanced NSCLC patients who failed to previous lines of chemotherapy, chemoradiotherapy, TKI therapy, surgery, or any combination of the therapies, and investigated the prognostic factors for patients who received anti-PD-1 (Camrelizumab) and anti-angiogenic (Apatinib) combined therapy. The objective response rate (ORR) assessed by an independent radiology review was 22.7%, and the median progression-free survival (PFS) was 5.25 months. We found that high concentration of circulating-free DNA (cfDNA) (HR = 27.75, P = 0.003), MIKI67 mutation (HR = 114.11, P = 0.009) and gene variations related to hyper-progressive disease (HPD) (HR = 36.85, P = 0.004) were independent risk factors and exhibited significant correlation with PFS. Circulating tumor DNA (ctDNA) mutational status was also a predicting indicator for PFS. In contrast, the blood tumor mutational burden (bTMB) could not stratify the clinical benefit in this combined therapy (HR = 0.81, P = 0.137). Furthermore, we found that the variant allele fraction (VAF) of mutations in ctDNA was sensitive indicators of therapeutic response and therefore can be used to monitor the tumor relief or progression. In conclusion, cfDNA concentration, MIKI67 mutations and HPD-related mutations were independent risk factors and PFS predictors for multiline combined anti-angiogenic/ICI combined therapy. ctDNA may be a novel monitoring biomarker for therapeutic response and predicting biomarker for prognosis in future combined therapy involving PD-1 blockade.
Introduction
Immune checkpoint inhibitors (ICIs), such as programmed death 1 (PD-1)/programmed death ligand 1 (PD-L1) inhibitors have made a significant breakthrough in lung cancer treatment (1–3). Antibody blockade of the PD pathway rectifies immunodeficiency in tumor microenvironment, and empowers CD8+ T cells with the capability to kill tumor cells efficiently (4, 5). Along with success in clinical trials of immunotherapy, many more therapeutic options have appeared for lung cancer patients (6–12).
In patients with previously treated lung cancer, especially patients with wild-type driver genes, ICIs have substantially improved clinical prognosis compared with chemotherapy, as evidenced by an improvement of overall survival (OS) from 9.6 to 13.8 months in OAK cohorts (13). However, the achieved objective response rate (ORR) was less than 20% for anti-PD-1/PD-L1 antibody monotherapy in second-line or higher settings, including Nivolumab, Pembrolizumab, and Atezolizumab (6, 13, 14). Therefore, there is an urgent need to explore appropriate methods for improving the efficacy of immunotherapy and selecting patients potentially benefit from immunotherapy at multiline levels.
Many studies have focused on combination strategies to improve clinical efficacy of ICIs. The combination of Atezolizumab with Bevacizumab, Carboplatin, and Paclitaxel (ABCP) had significantly improved OS (19.2 months vs. 14.7 months) and PFS (8.3 months vs. 6.8 months) for metastatic non-squamous non-small cell lung cancer (NSCLC) in the phase III IMpower150 trial (9). Evidence has also suggested that anti-PD-1 and anti-vascular endothelial growth factor (VEGF) combined therapy may be a more favorable treatment option than any single reagent for NSCLC patients who had failed on the first-line or later treatment, and this therapeutic response was not affected by VEGF mutational status (15–17). CheckMate 227, another phase III trial in advanced NSCLC, suggested that Nivolumab plus Ipilimumab resulted in a longer OS independent of the PD-L1 expression level (18). This could be because the differential immune effects of CTLA-4 vs. PD-1 inhibition recruited effective antitumor immunity from the peripheral compartment, which is increasingly recognized as an important mechanism of response to immunotherapy (19–21). The combination of Pembrolizumab with chemotherapy exhibited superior ORR (57.9% vs. 38.4%), OS (15.9 months vs. 11.3 months) and PFS (6.4 months vs. 4.8 months) compared with placebo group for previously untreated metastatic, squamous NSCLC, demonstrated by the phase III KEYNOTE-407 trial (22). Similarly, in patients with treatment-naïve metastatic non-squamous NSCLC without EGFR or ALK mutations, the combination of standard chemotherapy with Pembrolizumab resulted in significantly longer OS (1-year OS rate: 69.2% vs. 49.4%) and PFS (8.8 months vs. 4.9 months) compared with placebo in KEYNOTE-189 trial (11). Moreover, PEMBRO-RT, a multicenter, randomized phase II study for metastatic NSCLC patients after chemotherapy failure, indicated that the ORR at 12 weeks was improved from 18 to 36% when radiotherapy was combined with Pembrolizumab, although the improvement did not reach statistical significance (P = 0.07) (23).
All the above clinical trials suggest that immunotherapy combined with chemotherapy or radiotherapy improved clinical efficacy in NSCLC patients. However, most randomized phase III clinical trials using combined immunotherapy were designed for first-line therapy, and there are few data for NSCLC patients in second-line or higher settings. In this study, we therefore performed a pilot study by recruiting a total of 22 NSCLC patients who failed to previous lines of chemotherapy, chemoradiotherapy, TKI therapy, surgery, or any combination of the therapies, and investigated the clinical efficacy of Camrelizumab, a humanized, high-affinity IgG4-kappa mAb against PD-1, combined with Apatinib, a small molecular drug targeting vascular endothelial growth factor receptor-2 (VEGFR-2). Since the traditional biomarkers for immunotherapy, such as the PD-L1 expression and tumor mutation burden (TMB) appeared to have limited predicting values in several clinical trials using combined immunotherapy (11, 12, 22, 24, 25), we investigated the values of a few new biomarkers in predicting and monitoring the therapeutic response and prognosis in second-line or higher settings when ICI was combined with anti-angiogenic therapy.
Materials and Methods
Ethic Approval by Participating Hospitals
All study plans and protocols for the study were submitted to the ethics/licensing committee of the First Affiliated Hospital of Zhejiang University for review and approval before the start of the study, and were approved by the corresponding committee. Confirmation of approval for clinical study was received from the ethics board (approval number: 2018-775-1) before the start of the study. All experiments, methods, procedures and personnel training were carried out in accordance with relevant guidelines and regulations of participating hospitals and laboratories.
Study Design, Patients, and Samples
Patients recruited in this study were advanced NSCLC patients who received at least one line of treatment (chemotherapy or target therapy) and exhibited disease progression, and were recommended for subsequent therapy using Camrelizumab (or SHR-1210, Hengrui Medicine, Jiangsu, China) (200 mg q2w) combined with Apatinib (Hengrui Medicine, Jiangsu, China) (250 mg qd). Blood samples were collected at the First Affiliated Hospital of Zhejiang University. All patients received written informed consent for the use of clinical samples. Patient information was kept anonymous for confidentiality.
A total of 22 advanced lung cancer patients were recruited in this study between July 1, 2018 and October 31, 2019. The baseline characteristics of these patients are described in Table 1, and the detailed information has been included in Table 2, Supplementary Tables S2, S3. The median age of these patients was 61.5 years old (33∼73 years old). Seventeen patients (77.3%) were male and five (22.7%) were female, and all the female patients (n = 5) were non-smokers, whereas 15 (88.2%) male patients had more than 20 pack years of smoking history. The number of lung adenocarcinoma patients (n = 10) was approximately equal to the patients harboring squamous cell carcinoma (n = 12). Seventeen patients (77.3%) were diagnosed with stage IV lung cancer, and five patients were at stage IIIB. Fourteen patients (63.6%) underwent this combined immunotherapy as second-line therapy, and the remaining eight patients (36.4%) received this therapy as third-line or higher treatments. The clinical information of all patients is summarized in Table 1. Disease control rate (DCR) and progression free survival (PFS) was used to assess the response of therapy. The expression of PD-L1 was determined before the first-line therapy on primary tumors and not on recurrent or resistant tumors, and immunohistochemistry was performed with Dako PDl-L1 IHC 22C3 pharmDx [Agilent Technology (China) Co., Ltd.] using the 22C3 antibody.
Sample Preparation, Library Construction, Targeted Sequencing, and Data Processing
Blood samples from patients were collected in Ethylene Diamine Tetraacetic Acid (EDTA) tubes and centrifuged at 1600 g for 10 min and at 4°C. The supernatants were further centrifuged at 10,000 × g for 10 min at 4°C, and plasma was harvested and stored at −80°C until further use. Circulating tumor DNA (ctDNA) was extracted from 3 to 3.5 ml plasma using the QIAamp Circulating Nucleic Acid kit (Qiagen, Inc., Valencia, CA, United States) according to the manufacturer’s instructions. Blood cell fragments (including peripheral blood lymphocytes and red cells) were preserved at −20°C for further study. We applied the RelaxGene blood DNA system (Tiangen Biotech) to extract genomic DNA from peripheral blood lymphocytes (PBLs) as the normal control for mutation calling from cancer tissues and ctDNA. DNA was quantified with the Qubit 2.0 Fluorometer and the Qubit dsDNA HS assay kit (Thermo Fisher Scientific, Inc., Waltham, MA, United States) according to manufacturer’s instructions. Fragmented genomic DNA underwent end-repairing, A-tailing and ligation with indexed adapters sequentially, followed by size selection using Agencourt AMPure XP beads (Beckman Coulter Inc., Brea, CA, United States), and DNA fragments were used for library construction using the KAPA Library Preparation kit (Kapa Biosystems, Inc., Wilmington, MA, United States) according to the manufacturer’s protocol. Hybridization-based target enrichment was carried out with HaploX pan-cancer gene panel (605 cancer-relevant genes, HaploX Biotechnology, gene list is provided in Supplementary Table S1) for cancer tissue sequencing. Seven to eight polymerase chain reaction (PCR) cycles, depending on the amount of DNA used, were performed by pre-capture ligation-mediated PCR (Pre-LM-PCR) Oligos (Kapa Biosystems, Inc.) in 50 μl reactions. ctDNA sequencing was then performed on the Illumina Novaseq 6000 system according to the manufacturer’s recommendations at an average depth of 20,000×.
Data which meet the following criteria were chosen for subsequent analysis: the ratio of remaining data filtered by fastq in raw data is ≥85%; the proportion of Q30 bases is ≥85%; the ratio of reads on the reference genome is ≥85%; target region coverage ≥98%; average effective sequencing depth in ctDNA is ≥ 3000×. The called somatic variants need to meet the following criteria: the read depth at a position is ≥20×; the variant allele fraction (VAF) is ≥0.5% for ctDNA and ≥2% for PBL genomic DNA; somatic-P-value ≤0.01; strand filter ≥1. VAF were calculated for Q30 bases. The copy number variation (CNVs) was detected by CNVkit version 0.9.31. Further analyses of genomic alterations were also performed, including single nucleotide variants (SNVs), CNVs, insertion/deletion (Indels), fusions, and structural variation.
Statistical Analysis
Statistical analysis was performed and figures were plotted with Graphpad Prism 5.0 software (GraphPad Software, Inc., La Jolla, CA, United States). Student t-test was performed when two groups were compared, and ANOVA and post hoc tests were performed when three or more groups were compared. Chi-square test, calibrated Chi-square test or Fisher exact probability test were performed when rate or percentage was compared for significance. Figures for mutation spectrum were made with the R software2. Univariate and multivariate analyses were performed using the SPSS 17.0 software (IBM China Company Limited, Beijing, China). P < 0.05 was regarded as statistically significant.
Results
Genetic Alterations Were Capable of Predicting the Therapeutic Response and Prognosis of the Multiline Anti-angiogenic/ICI Combined Therapy
Twenty-two patients involved in this study received Camrelizumab combined with Apatinib therapy (Table 1). Fourteen patients received the combined treatment as the second-line therapy, while the rest as the third to sixth line therapy. The ORR and DCR assessed by an independent radiology review were 28.6% (4/14) and 64.3% (9/14), respectively, for the second-line therapy, and were 12.5% (1/8) and 50.0% (4/8), respectively, for the third to sixth line therapy (Table 2). All patients were followed up until disease progression (PD) or the end of this study. The progression-free survival (PFS) for all patients ranged from 1.4 to 15.5 months (median at 5.25 months). Data in Table 2 showed a significantly longer PFS in patients with DCR than those with PD at the second-line therapy (P = 0.010, unpaired t-test), and similar trend can also be observed with patients at third to sixth line therapy (P = 0.088, unpaired t-test), while no significant difference was observed in PFS between patients with second-line therapy and patients with third to sixth line therapy (Table 2). We stratified all patients into the ADC group and SCC group, and examined the potential correlation between the above factors and patients’ response (DCR or PD). It can be observed from Supplementary Table S2 that the significant difference in PFS between patients with DCR and PD can be observed in SCC patients at second-line level (P = 0.04), while a trend of better PFS was also observed with ADC at second-line level (P = 0.15).
The mutational spectrum of circulating-free DNA (cfDNA) was established using the pre-therapeutic blood samples (Figure 1A). Mutations were detected in cfDNA of 15 patients while not detected in 7 patients. The NGS sequencing yielded a total of 80 somatic SNVs, 19 small insertions/deletions (indels), and 4 CNVs. TP53 (41%), FAT1 (14%), and KMT2D (14%) exhibited the highest mutational frequencies (Figure 1A). Few patients carried TKI-related driver gene mutations, including EGFR (one patient), BRAF (one patient), PIK3CA (one patient), and ERBB2 (one patient).
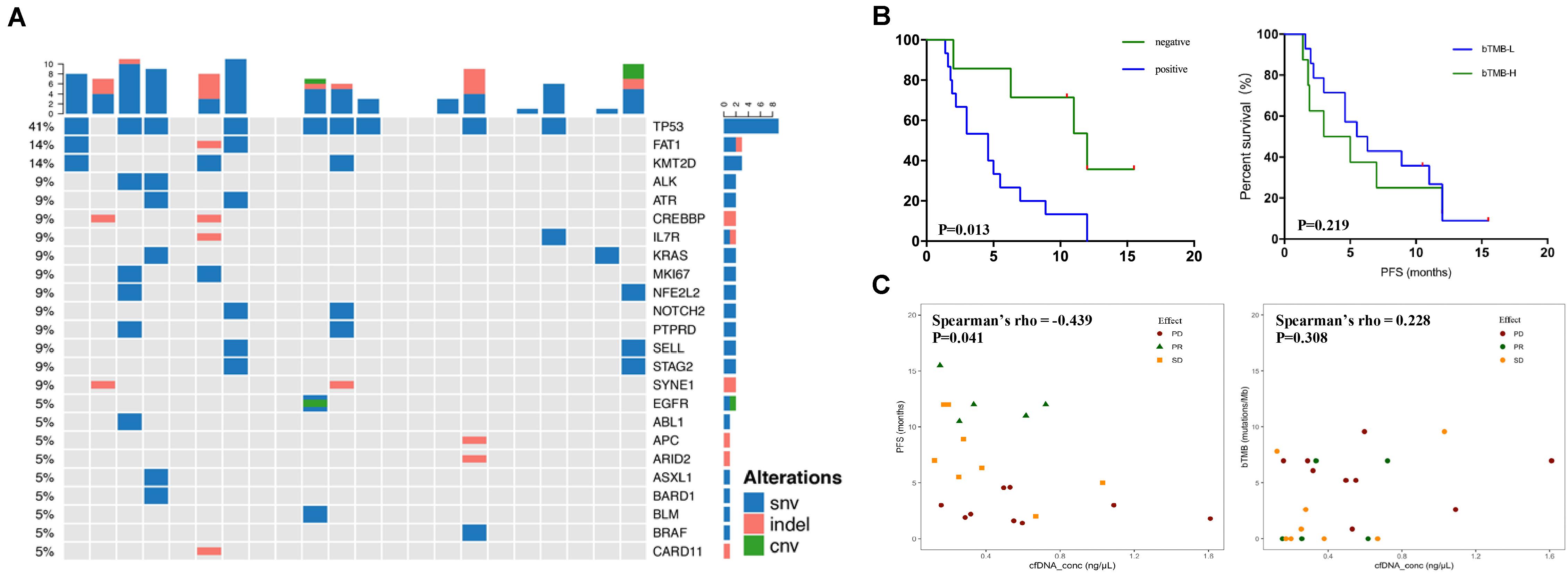
Figure 1. The relationship between blood mutational landscape and therapeutic response. (A) The pre-therapeutic blood ctDNA mutational landscape. (B) Keplan–Meier survival analysis of patients with negative or positive ctDNA mutation detection (left panel, 7 patients with negative and 15 patients with positive ctDNA mutation detection) or patients with low or high bTMB levels (right panel, 8 patients with high and 14 patients with low bTMB levels). (C) Correlation between cfDNA concentration and PFS (left panel) or bTMB (right panel).
We further explored the potential predicting efficacy of genetic variations on PFS and therapeutic response (Figures 1B,1C). Patients with PR and SD were combined into DCR group due to the limited number of patients. It was found that patients with no detectable ctDNA mutations (ctDNA-negative group) showed significantly better PFS than patients with ctDNA mutations (ctDNA-positive group) (P = 0.017, Fisher exact probability test), suggesting that the pre-therapeutic ctDNA mutations were predictive for PFS (Figure 1B and Table 2). Further analysis on ADC and SCC individually revealed that the significant correlation between ctDNA mutational status and response was mainly reflected in ADC (P = 0.008, Supplementary Table S2). In contrast, the pre-therapeutic blood TMB (bTMB) level was not able to predict the PFS, when the 66.7 percentile bTMB value (6.96 mutations/Mb) was used to discriminate TMB-high (TMB-H) from TMB-low (TMB-L) (Figure 1B, Table 2 and Supplementary Table S3). This observation indicates that the predictive capability of bTMB may be compromised in the second-line or multiline combined immunotherapy. Further correlation analysis showed that PFS had significant correlation with the blood cfDNA concentration (Spearman’s rho = −0.439, P = 0.041), in which patients with better therapeutic response (PR or SD) correlated with longer PFS and lower cfDNA concentration, while no significant correlation can be found between bTMB and blood cfDNA concentration (Figure 1C and Supplementary Table S3). Furthermore, it appeared that gender, pathological types, smoking history, cancer stage, line of therapy, and the presence of TKI-related driver gene mutations did not affect the therapeutic response (DCR or PD) (Table 2). The correlation between PD-L1 expression and PFS (Supplementary Figure S1A), bTMB (Supplementary Figure S1B), ctDNA concentration (Supplementary Figure S1C), and response (Supplementary Figure S1D) were investigated, and no clear correlation was identified. In addition, PD-L1 expression levels, whether grouped by positive or negative expression (P = 0.18) or by 50% threshold (P = 0.39), did not show significant stratification on response (Supplementary Figures S1E,F).
The relationship between gene variations and PFS was investigated in detail. Kaplan–Meier survival analysis showed that patients with MIKI67 mutations (Log-rank test P = 0.022) or CREBBP mutations (Log-rank test P = 0.049) exhibited significantly worse PFS than those without mutations (Figures 2A,B). In contrast, no such difference was observed in patients with or without TP53 mutations (Figure 2C). Interestingly, we found that patients who carried mutations in genes related to hyper-progressive diseases (HPDs) (one patient carried EGFR amplification and one patient carried FGF4 amplification) showed significantly worse PFS compared with those without such mutations (Log-rank test P = 0.028) (Figure 2D), while no significant difference in PFS was found between patients with or without TKI-related driver gene mutations (Figure 2E). Finally, no significant difference was found between patients with second-line therapy and those with third- to sixth-line therapy (Figure 2F and Table 2).
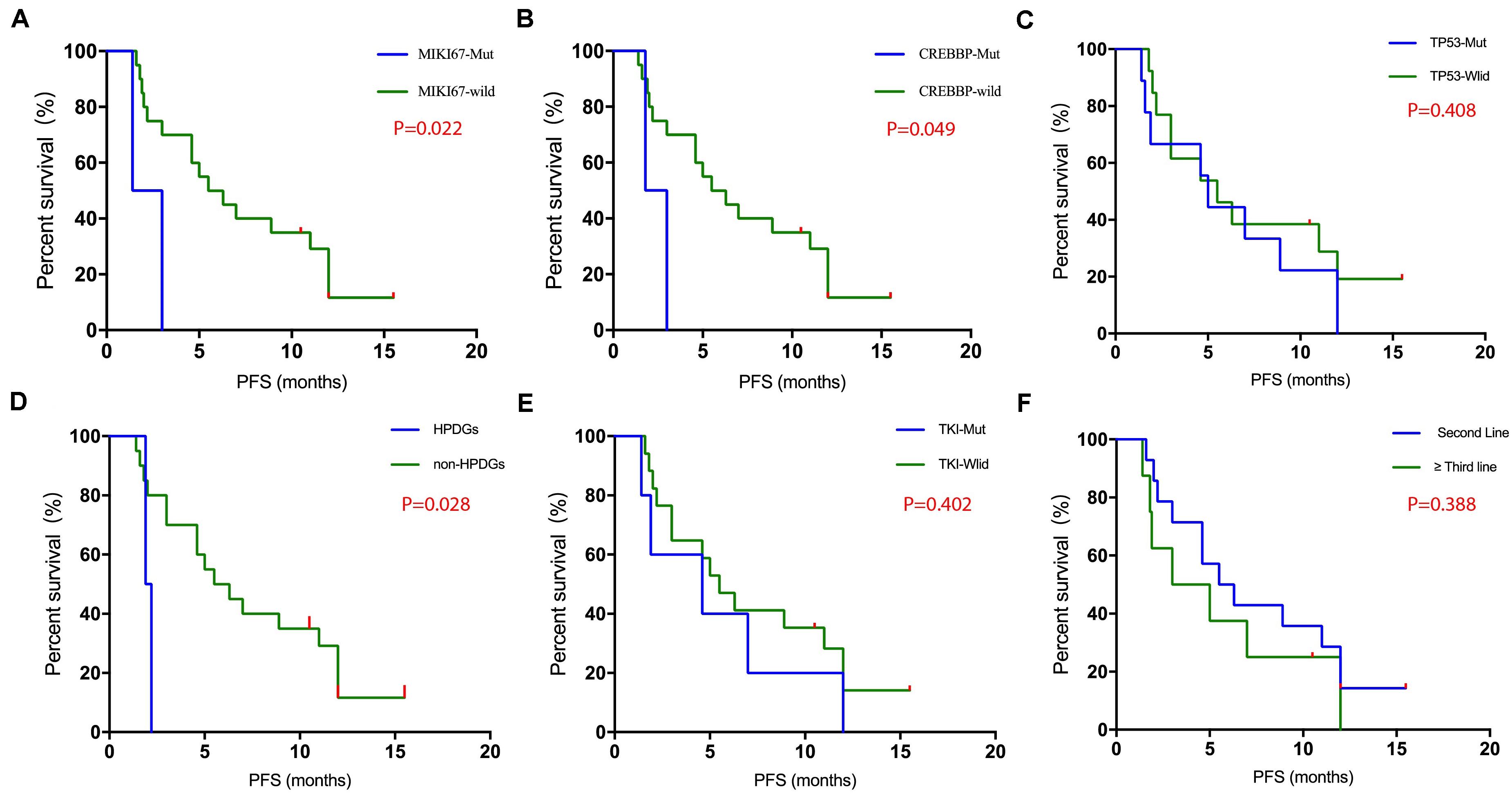
Figure 2. Keplan–Meier survival analysis of various mutational status. The mutational status of MIKI67 (A, 2 muts and 20 WTs), CREBBP (B, 2 muts and 20 WTs), TP53 (C, 12 muts and 10 WTs), HPD genes (D, 2 with HPDG alterations and 20 without), TKI-relevant mutations (E, 4 with TKI-related mutations and 18 without), and lines of therapy (F, 12 at second-line and 10 at higher lines) were compared and shown as indicated.
Multivariate Cox regression analysis (Figure 3) was performed using variables with P ≤ 0.3 as described above (Figures 1B,C, 2). The concentration of cfDNA was found to be an independent predictor of PFS (HR = 27.75, P = 0.003), in which higher cfDNA concentration correlated with poorer outcomes (Figure 3). Similarly, patients harboring mutations in MIKI67 (HR = 114, P = 0.009) or genes relating to HPDs (HR = 36.85, P = 0.004) were also significantly associated with shorter PFS. Meanwhile, due to the limited number of patients, the ctDNA-negative group did not show more benefit from this combined immunotherapy compared with the ctDNA-positive group, although a trend of difference was observed (HR = 0.19, P = 0.068). As expected, bTMB could not predict the clinical benefits in this combined therapy.
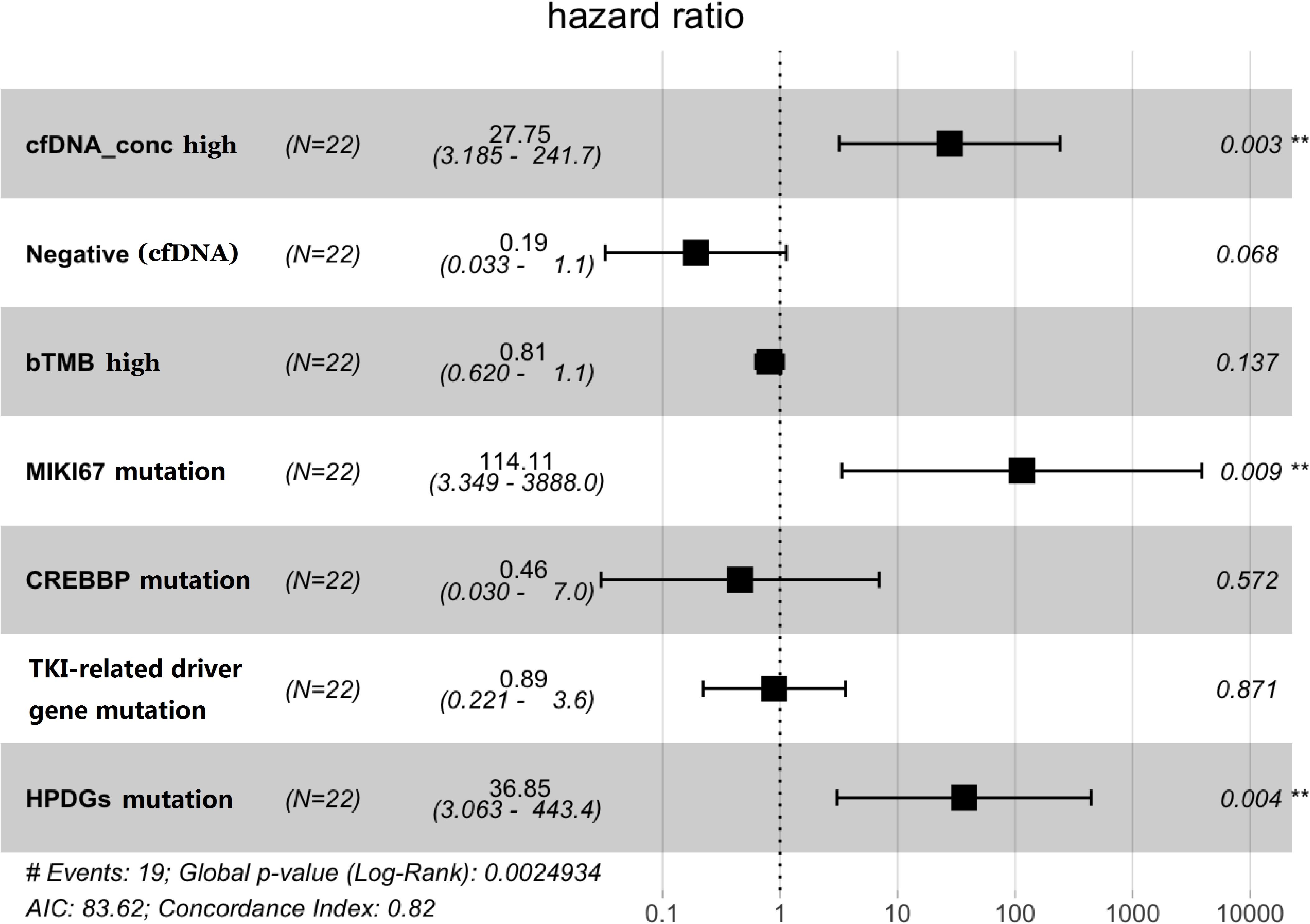
Figure 3. The results of multivariate Cox regression analysis for various potential risk factors. cfDNA concentration (P = 0.003), MIKI67 mutations (P = 0.009), and HPD gene mutations (P = 0.004) were identified as independent risk factors, while a trend of significance can be observed with ctDNA mutations (P = 0.068). Negative (cfDNA) indicates that no mutations in any gene were found in the 605 gene-panel test.
ctDNA Is Capable of Monitoring the Therapeutic Responses of the Multiline Anti-angiogenic/ICI Combined Therapy
We further investigated the potential of blood ctDNA in therapeutic response monitoring. Plasma samples were obtained before and after treatment at key assessment points of therapeutic response in parallel with imaging examination. Figure 4 shows several examples of patients with distinct therapeutic responses. We found that the changes in variant allelic frequency (VAF) of mutations exhibited identical trend to the changes in target tumor maximal diameter, whether in progressed disease (patient A), partial response (patient B), or in stable disease (patient D). More interestingly, we observed that the VAF of ctDNA decreased significantly in one patients following combined immunotherapy without significant change in tumor size (patient C), but exhibited and a clear cavity inside the tumor. The patient was progression free for 12 months and achieved PR ultimately. These results indicate that dynamics of ctDNA may be more sensitive than imaging examination to monitor the therapeutic response to combined immunotherapy. Furthermore, the regression tree analysis has been performed. PFS, cfDNA concentration, ctDNA detection (negative or positive), bTMB, MIKI67 mutation, CREBBP mutation, TKI-related driver gene mutation, HPDG mutation, TP53 mutation, and lines of therapy were set as the independent variable and the patient risk (determined by the significant factors in Figure 3) was set as the dependent variable. Although ctDNA detection appeared to be the only significant factor that may predict the risk of patients, the test was not conclusive as the number of patients involved was limited.
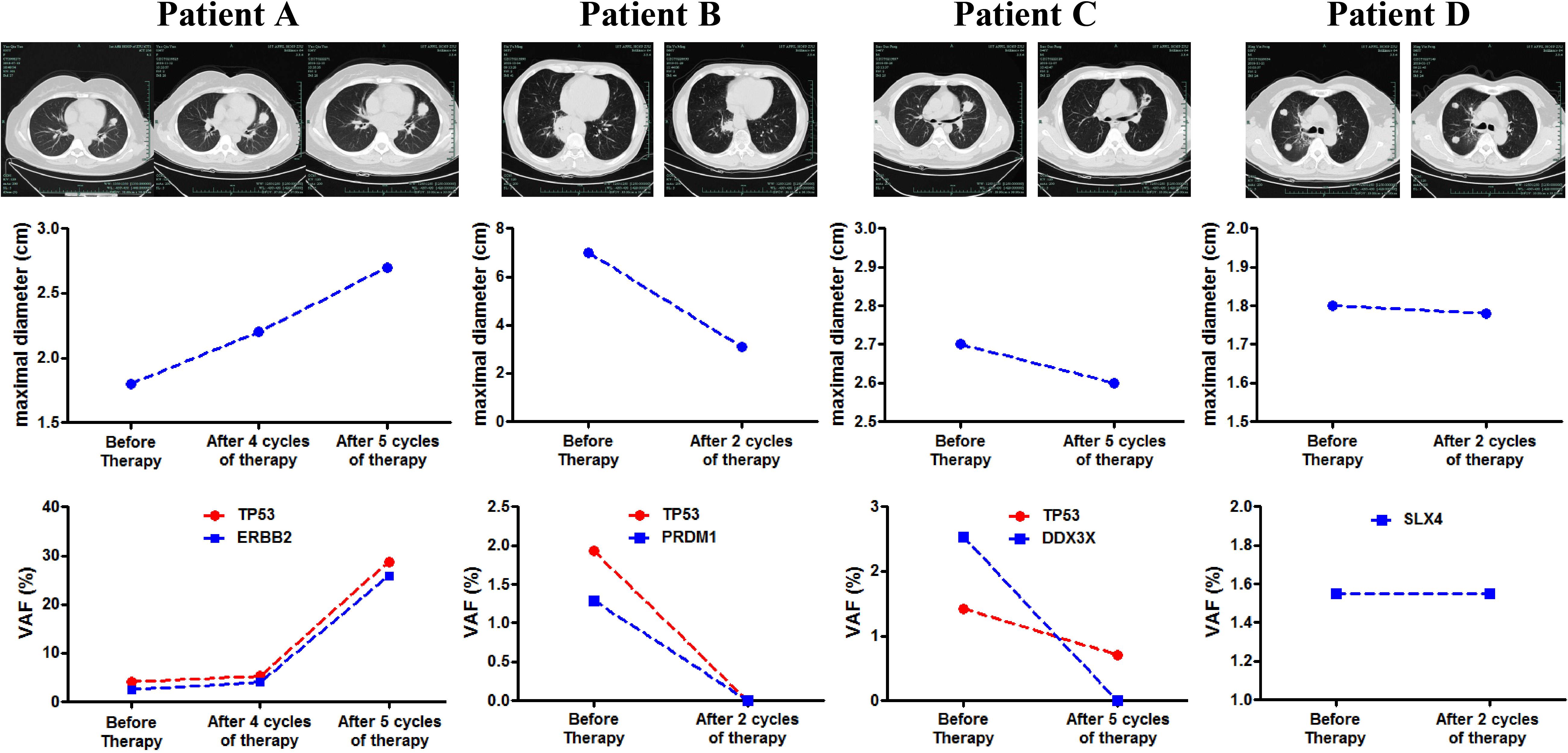
Figure 4. Correlation between tumor diameter change and ctDNA mutational change following the multiline anti-angiogenic/ICI combined therapy. The CT images, tumor diameter changes and VAF changes of blood ctDNA mutations of four patients (A–D) were illustrated.
Discussion
In this study, we prospectively enrolled 22 patients with advanced NSCLC and studied the therapeutic responses and prognostic factors of the combined anti-angiogenic/ICI therapy at second-line and multiline levels. Previous research reported that antiangiogenic drugs can exert immune activation by inhibiting VEGF, promoting dendritic cell maturation, increasing T cell infiltration, and reprogramming the immune microenvironment (26). Therefore, anti-vascular drugs may reverse VEGF-mediated immunosuppression, thereby enhancing the antitumor activity of immunotherapy. Compared with the therapeutic responses of second- or multi-line PD-1 blockade previously reported (13, 14), PD-1 blockade combined with anti-angiogenic drugs had obvious clinical advantages, significantly improving ORR and PFS. This combined therapy could have better ORR, DCR, and PFS in the second-line treatment than the multiline treatment. In this study, we found a trend that the PFS of patients with second-line therapy may be better than that of the 3rd—6th lines of therapy, which supported previous observations. We also found that cfDNA concentration, MIKI67 mutations, and HPD-related gene mutations and potentially ctDNA mutations, were independent risk factors for therapeutic response prediction, while age, gender, lung cancer subtypes, smoking history, and bTMB did not affect the response.
Previous studies have shown that ctDNA was able to predict PFS in the first-line or multi-line immunotherapy of NSCLC (1, 27, 28). Similar capability were confirmed in colorectal cancer (29, 30), breast cancer (31, 32), liver cancer (33), and gastric cancer (34, 35). In this study, we found that ctDNA was also capable of predicting the response of NSCLC to multiline anti-angiogenic/ICI combined therapy. Patients with positive ctDNA exhibited worse prognosis (PFS) than patients with negative ctDNA. This may be due to fact that higher tumor load in ctDNA-positive patients led to poor treatment response and poor tumor remission. It can be concluded from previous and our studies that the survival of ctDNA-positive patients will be worse than ctDNA-negative patients, regardless of single-agent or combined immunotherapy, and regardless of first-line or multi-line therapy. In addition, we also found that the VAF of ctDNA mutations was a good indicator of response of the combined therapy. In this study, prospective collections of blood samples before and after treatment and tests using NGS panel allowed the tracking of multiple genes with fluctuating VAF found in ctDNA. High concordance was found between the change of VAF and the tumor size. Due to the limited number of mutations that can be detected in ctDNA, our observation suggested that targeting a few mutations with high VAF in ctDNA may be sufficient to monitor the therapeutic response. We also found that ctDNA may be more sensitive and have predicting efficacy compared with imaging studies. This is supported by previous reports showing that ctDNA can predict tumor recurrence or remission much earlier than imaging examination (36), which is important for decision-making in therapeutic strategy selection. Therefore, we speculate that if ctDNA is used in combination with imaging, they can better monitor and predict the response and prognosis of patients than using one method alone. In addition, we found that cfDNA concentration was significantly correlated with PFS and was an independent risk factor for patients, suggesting that the plasma cfDNA level can predict the response and prognosis regardless of the mutational status, which was also supported by previous reports (37–39).
Many studies support the viewpoint that bTMB can be used as a biomarker to predict clinical efficacy in anti-PD-1/PD-L1 immunotherapy. Patients with high bTMB have a better ORR and PFS than patients with low bTMB (1, 40). However, a retrospective analysis of the POPLAR and OAK studies found that bTMB may not effectively distinguish the benefit in OS of patients receiving immunotherapy at second-line or higher (1, 41). Giles et al. (42) recently also found worse PFS and OS in NSCLC patients with higher bTMB in first-line immunotherapy. This suggests us that evidence is still needed to support the effectiveness of bTMB as a biomarker in immunotherapy, especially in multiline combined therapy. We provided such evidence in this article and found that the level of bTMB cannot effectively distinguish the benefit population in second-line and multi-line anti-angiogenic/ICI combined therapy. We speculate that one of the possible reasons is that the combined therapy improved the patients’ response, and weakened the stratification of bTMB on the patients’ response and prognosis observed in single-agent based immunotherapy.
It has been previously reported that PD-L1 expression can effectively stratify the therapeutic effect of ICIs, and the PD-L1 expression has been approved by the FDA as a companion diagnostic marker for anti-PD-1/PD-L1 treatment. Patients with high PD-L1 expression are more likely to benefit from immunotherapy (43). However, the PEMBRO-RT study found that PFS and OS in patients with PD-L1 high expression were shorter in the second-line immunotherapy combined with radiotherapy for lung cancer (23), also suggesting that the efficacy of biomarkers in multiline combined therapy involving PD1/PD-L1 blockade may be different from previous observations with immunotherapy alone. Ideally, the PD-L1 expression status of all patients with recurrent or resistant tumors should be reexamined, and multiline therapeutic strategies should be established based on the information. However, practically, many patients were reluctant to receive a second biopsy with both PD-L1 test and NGS test, because they believe that immunotherapy combined with other therapies was possibly the only option for them when the disease progressed, and therefore there was no need to repeat the tests anymore. As a result, the reexamination rate in these patients was very low. We were facing the similar situation in this study. Therefore, we tried to link the PD-L1 expression from primary tumor with the response of 2nd–6th lines therapy to see if we can identify any correlation, while no significant correlation was found, as shown in Supplementary Figure S1. Another concern is that due to the better response in patients with combined therapy, the effect of stratification by PD-L1 may not be significant anymore, and patients with negative PD-L1 expression may also benefit from the therapy, which was reflected in some recent studies (15–17).
Previous reports have shown that tumors were not effectively controlled and hyperprogression finally developed after immunotherapy in some patients (44). Further clinical studies have shown that these patients may carry genetic alterations, such as EGFR amplification, MDM2/MDM4 amplification, and chromosome 11 band 13-related gene amplification (FGF4, FGF19) (45). In this study, we prospectively enrolled two patients, who carried EGFR amplification and FGF4 amplification, respectively. They quickly developed PD with PFS of 1.9 and 2.2 months, respectively, far lower than those who did not carry hyperprogression-relevant gene mutations. Our observation suggests that the clinical response of patients with hyperprogression-relevant gene mutations was also poor in multiline anti-agiogenic/ICI combined therapy. Similarly, evidence also showed that NSCLC patients with TKI-related driver gene mutations often had poor ORR and PFS in anti-PD-1/PD-L1 treatment, in which the evidence for EGFR mutations and ALK fusions were strong, while other TKI-related driver gene mutations were not confirmed (46, 47). In this study, we compared patients with or without TKI-related driver gene mutations and found no significant differences in PFS between the two groups, suggesting that not all TKI-related driver gene mutations affect anti-angiogenic/ICI combined therapy. Interestingly, we found that two patients with ALK mutations (not fusions) with PFS of 12 and 1.4 months, respectively. The latter had a shorter PFS possibly due to a MIKI67 mutation, since MIKI67 mutations were an independent factor of poor prognosis. Our observation suggests that ALK non-fusion mutations may not affect the response of the combined therapy.
There were some limitations of this study. Firstly, heterogeneity of therapy was a weakness of the study, especially when therapeutic lines from 2nd to 6th were all involved. This is why we stratified the patients into 2nd line and 3rd–6th lines in this study, at least the therapy for 2nd line patients was homogeneous. By comparing these two populations, we found that the PFS of 2nd line treatment might be better than that of 3rd—6th treatment (Supplementary Table S2), which was one of main findings in the study. Secondly, two control cohorts, including one Camrelizumab and one Apatinib cohort, should be included to compare with the Camrelizumab and Apatinib combined cohort. However, it is difficult in reality to recruit patients using Camrelizumab or Apatinib alone, since both doctors and patients are aware that patients with combined therapy may potentially respond better than those treated with a single drug, therefore, challenges exist in both practical therapy and ethics if single drug group is recruited in parallel. However, some previous patients using the single drug may be involved as the control cohort. Thirdly, it was unfortunate in this study that we were unable to obtain any tissue samples from patients at advanced therapy stages, and therefore the test for tissue TMB was not possible. This was mainly because patients normally receive the tissue sampling and test when they are first diagnosed (before any therapy), and tissue sampling at later treatment is performed only when specific genetic alterations related to disease progression are highly suspected. In future study, tissue TMB should be obtained whenever possible, since difference might exist between tissue and blood TMB in therapy guidance. Fourthly, a relatively small cohort was one limitation of the study. For example, Figure 2 showed all mutations with significant stratification of survival. Due to the limited number of patients with MIKI67 or CREBBP mutants, the conclusion was not solid, although significant stratification was observed. The results need further validation with larger cohort in future.
Conclusion
In summary, we found that the combination of anti-angiogenic with PD-1 blockade therapy significantly enhanced the ORR and PFS of NSCLC patients in second-line or multiline treatment. ctDNA concentration, MIKI67 mutation, and HPD-relevant gene mutations were independent risk factors for PFS. The blood ctDNA mutations can potentially identify the benefit population and predict the patients’ response and prognosis. In addition, ctDNA detection at series time points can effectively monitor disease relief or progression. Our study provided valuable evidence for treatment strategy selection in the second-line and multiline anti-angiogenic/ICI combined treatment of advanced NSCLC. There were also limitations in this study, mainly due to the limited number of patients included, while more patients should be enrolled in the future to confirm the conclusion of this study.
Data Availability Statement
The datasets presented in this study can be found in online repositories. The names of the repository/repositories and accession number(s) can be found below: Genome Sequence Archive (https://bigd.big.ac.cn/gsa-human/browse/HRA000239).
Ethics Statement
The studies involving human participants were reviewed and approved by The First Affiliated Hospital of Zhejing University. The patients/participants provided their written informed consent to participate in this study. Written informed consent was obtained from the individual(s) for the publication of any potentially identifiable images or data included in this article.
Author Contributions
YW and LS designed the study and proofread the manuscript. YC and XL provided the samples and collected the clinical information and diagnostic information. YC, XL, and LS performed the statistics and wrote the manuscript. GL, SC, and MX performed the sequencing experiments and analyzed the sequencing data. LS submitted the manuscript. All authors contributed to the article and approved the submitted version.
Funding
This study was supported by the Special Funds for Strategic Emerging Industry Development of Shenzhen (grant number 20170922151538732) and the Science and Technology Project of Shenzhen (grant number JSGG20180703164202084). This study was also supported by the financial grants from Zhejiang Provincial Natural Science Foundation of China (LY16H160006) and Hangzhou Science and Technology Bureau (20160533 B72). All funders did not participate in the study design, study implementation, data collection, data analysis, data interpretation, and manuscript writing of the study.
Conflict of Interest
XL, GL, SC, MX, and LS are employed by the company HaploX Biotechnology, Co., Ltd.
The remaining authors declare that the research was conducted in the absence of any commercial or financial relationships that could be construed as a potential conflict of interest.
Supplementary Material
The Supplementary Material for this article can be found online at: https://www.frontiersin.org/articles/10.3389/fonc.2020.01706/full#supplementary-material
FIGURE S1 | Correlation of PD-L1 expression level with other markers or patient response or survival. Results of correlation were shown for PD-L1 expression with PFS (A), bTMB (B), ctDNA concentration (C), response (D). The influence of PD-L1 expression (negative or positive) on survival was shown in panel (E), and the influence of PD-L1 expression (<50% or ≥50%)was shown in panel (F). PD-L1 expression level of >1% was considered positive, and PD-L1 expression level of ≥50% was considered high expression. In 15 patients with available PD-L1 expression data, 11 had expression level of >1% and 4 had expression level of ≥50% (Supplementary Table S3).
Footnotes
References
1. Zhijie W, Jianchun D, Shangli C, Miao H, Hua D, Jun Z, et al. Assessment of blood tumor mutational burden as a potential biomarker for immunotherapy in patients with non-small cell lung cancer with use of a next-generation sequencing cancer gene panel. JAMA Oncol. (2019) 5:696–702. doi: 10.1001/jamaoncol.2018.7098
2. Fan Y, Mao W. Immune checkpoint inhibitors in lung cancer: current status and future directions. Chin Clin Oncol. (2017) 6:17. doi: 10.21037/cco.2017.02.05
3. Zhang D, Huang J, Zhang C, Guan Y, Guo Q. [Progress on PD-1/PD-L1 checkpoint inhibitors in lung cancer]. Zhongguo Fei Ai Za Zhi. (2019) 22:369–79. doi: 10.3779/j.issn.1009-3419.2019.06.07
4. Sanmamed MF, Chen LA. Paradigm shift in cancer immunotherapy: from enhancement to normalization. Cell. (2019) 176:677. doi: 10.1016/j.cell.2019.01.008
5. Zhang Y, Chen L. Classification of advanced human cancers based on tumor immunity in the microenvironment (TIME) for cancer immunotherapy. JAMA Oncol. (2016) 2:1403–4. doi: 10.1001/jamaoncol.2016.2450
6. Brahmer J, Reckamp KL, Baas P, Crinò L, Eberhardt WE, Poddubskaya E, et al. Nivolumab versus docetaxel in advanced squamous-cell non-small-cell lung cancer. N Engl J Med. (2015) 373:123–35. doi: 10.1056/NEJMoa1504627
7. Horn L, Spigel DR, Vokes EE, Holgado E, Ready N, Steins M, et al. Nivolumab versus docetaxel in previously treated patients with advanced non-small-cell lung cancer: two-year outcomes from two randomized, open-label, phase III trials (CheckMate 017 and CheckMate 057). J Clin Oncol. (2017) 35:3924–33. doi: 10.1200/JCO.2017.74.3062
8. Dhillon S, Syed YY. Atezolizumab first-line combination therapy: a review in metastatic nonsquamous NSCLC. Target Oncol. (2019) 14:759–68. doi: 10.1007/s11523-019-00686-w
9. Socinski MA, Jotte RM, Cappuzzo F, Orlandi F, Stroyakovskiy D, Nogami N, et al. Atezolizumab for first-line treatment of metastatic nonsquamous NSCLC. N Engl J Med. (2018) 378:2288–301. doi: 10.1056/NEJMoa1716948
10. Lynch TJ, Bondarenko I, Luft A, Serwatowski P, Barlesi F, Chacko R, et al. Ipilimumab in combination with paclitaxel and carboplatin as first-line treatment in stage IIIB/IV non-small-cell lung cancer: results from a randomized, double-blind, multicenter phase II study. J Clin Oncol. (2012) 30:2046–54. doi: 10.1200/JCO.2011.38.4032
11. Gandhi L, Rodríguez-Abreu D, Gadgeel S, Esteban E, Felip E, De Angelis F, et al. Pembrolizumab plus chemotherapy in metastatic non-small-cell lung cancer. N Engl J Med. (2018) 378:2078–92. doi: 10.1056/NEJMoa1801005
12. Langer CJ, Gadgeel SM, Borghaei H, Papadimitrakopoulou VA, Patnaik A, Powell SF, et al. Carboplatin and pemetrexed with or without pembrolizumab for advanced, non-squamous non-small-cell lung cancer: a randomised, phase 2 cohort of the open-label KEYNOTE-021 study. Lancet Oncol. (2016) 17:1497–508. doi: 10.1016/S1470-2045(16)30498-3
13. Rittmeyer A, Barlesi F, Waterkamp D, Park K, Ciardiello F, von Pawel J, et al. Atezolizumab versus docetaxel in patients with previously treated non-small-cell lung cancer (OAK): a phase 3, open-label, multicentre randomised controlled trial. Lancet. (2017) 389:255–65. doi: 10.1016/S0140-6736(16)32517-X
14. Garon EB, Rizvi NA, Hui R, Leighl N, Balmanoukian AS, Eder JP, et al. Pembrolizumab for the treatment of non-small-cell lung cancer. N Engl J Med. (2015) 372:2018–28. doi: 10.1056/NEJMoa1501824
15. Zhang F, Huang D, Li T, Zhang S, Wang J, Zhang Y, et al. Anti-PD-1 therapy plus chemotherapy and/or bevacizumab as second line or later treatment for patients with advanced non-small cell lung cancer. J Cancer. (2020) 11:741–9. doi: 10.7150/jca.37966
16. Liang H, Wang M. Prospect of immunotherapy combined with anti-angiogenic agents in patients with advanced non-small cell lung cancer. Cancer Manag Res. (2019) 11:7707–19. doi: 10.2147/cmar.s212238
17. Rossi A. New options for combination therapy for advanced non-squamous NSCLC. Expert Rev Respir Med. (2019) 13:1095–107. doi: 10.1080/17476348.2019.1667233
18. Hellmann MD, Paz-Ares L, Bernabe Caro R, Zurawski B, Kim SW, Carcereny Costa E, et al. Nivolumab plus ipilimumab in advanced non-small-cell lung cancer. N Engl J Med. (2019) 381:2020–31. doi: 10.1056/NEJMoa1910231
19. Spitzer MH, Carmi Y, Reticker-Flynn NE, Kwek SS, Madhireddy D, Martins MM, et al. Systemic immunity is required for effective cancer immunotherapy. Cell. (2017) 168:487–502.e15.
20. Sade-Feldman M, Yizhak K, Bjorgaard SL, Ray JP, de Boer CG, Jenkins RW, et al. Defining T cell states associated with response to checkpoint immunotherapy in melanoma. Cell. (2018) 175:998–1013.e20. doi: 10.1016/j.cell.2018.10.038
21. Yost KE, Satpathy AT, Wells DK, Qi YY, Wang CL, Kageyama R, et al. Clonal replacement of tumor-specific T cells following PD-1 blockade. Nat Med. (2019) 25:1251–9. doi: 10.1038/s41591-019-0522-3
22. Paz-Ares L, Luft A, Vicente D, Tafreshi A, Gümüş M, Mazières J, et al. Pembrolizumab plus chemotherapy for squamous non-small-cell lung cancer. N Engl J Med. (2018) 379:2040–51. doi: 10.1056/NEJMoa1810865
23. Theelen WSME, Peulen HMU, Lalezari F, van der Noort V, de Vries JF, Aerts JGJV, et al. Effect of pembrolizumab after stereotactic body radiotherapy vs pembrolizumab alone on tumor response in patients with advanced non-small cell lung cancer: results of the PEMBRO-RT Phase 2 randomized clinical trial. JAMA Oncol. (2019) 5:1276–82. doi: 10.1001/jamaoncol.2019.1478
24. Gadgeel SM, Stevenson JP, Langer CJ, Gandhi L, Borghaei H, Patnaik A, et al. Pembrolizumab and platinum-based chemotherapy as first-line therapy for advanced non-small-cell lung cancer: phase 1 cohorts from the KEYNOTE-021 study. Lung Cancer. (2018) 125:273–81. doi: 10.1016/j.lungcan.2018.08.019
25. Borghaei H, Langer CJ, Gadgeel S, Papadimitrakopoulou VA, Patnaik A, Powell SF, et al. 24-Month overall survival from KEYNOTE-021 Cohort G: pemetrexed and carboplatin with or without pembrolizumab as first-line therapy for advanced nonsquamous non-small cell lung cancer. J Thorac Oncol. (2019) 14:124–9. doi: 10.1016/j.jtho.2018.08.004
26. Lacal PM, Graziani G. Therapeutic implication of vascular endothelial growth factor receptor-1 (VEGFR-1) targeting in cancer cells and tumor microenvironment by competitive and non-competitive inhibitors. Pharmacol Res. (2018) 136:97–107. doi: 10.1016/j.phrs.2018.08.023
27. Goldberg SB, Narayan A, Kole AJ, Decker RH, Teysir J, Carriero NJ, et al. Early assessment of lung cancer immunotherapy response via circulating tumor DNA. Clin Cancer Res. (2018) 24:1872–80. doi: 10.1158/1078-0432.CCR-17-1341
28. Raja R, Kuziora M, Brohawn PZ, Higgs BW, Gupta A, Dennis PA, et al. Early reduction in ctDNA predicts survival in patients with lung and bladder cancer treated with durvalumab. Clin Cancer Res. (2018) 24:6212–22. doi: 10.1158/1078-0432.CCR-18-0386
29. Cremolini C, Rossini D, Dell’Aquila E, Lonardi S, Conca E, Del Re M, et al. Rechallenge for patients with RAS and BRAF wild-type metastatic colorectal cancer with acquired resistance to first-line cetuximab and irinotecan: a phase 2 single-arm clinical trial. JAMA Oncol. (2019) 5:343–50. doi: 10.1001/jamaoncol.2018.5080
30. Barault L, Amatu A, Siravegna G, Ponzetti A, Moran S, Cassingena A, et al. Discovery of methylated circulating DNA biomarkers for comprehensive non-invasive monitoring of treatment response in metastatic colorectal cancer. Gut. (2018) 67:1995–2005. doi: 10.1136/gutjnl-2016-313372
31. Rossi G, Mu Z, Rademaker AW, Austin LK, Strickland KS, Costa RLB, et al. Cell-Free DNA and circulating tumor cells: comprehensive liquid biopsy analysis in advanced breast cancer. Clin Cancer Res. (2018) 24:560–8. doi: 10.1158/1078-0432.CCR-17-2092
32. Hrebien S, Citi V, Garcia-Murillas I, Cutts R, Fenwick K, Kozarewa I, et al. Early ctDNA dynamics as a surrogate for progression-free survival in advanced breast cancer in the BEECH trial. Ann Oncol. (2019) 30:945–52. doi: 10.1093/annonc/mdz085
33. Lim HY, Merle P, Weiss KH, Yau T, Ross P, Mazzaferro V, et al. Phase II studies with refametinib or refametinib plus sorafenib in patients with RAS-mutated hepatocellular carcinoma. Clin Cancer Res. (2018) 24:4650–61. doi: 10.1158/1078-0432.CCR-17-3588
34. Kim ST, Cristescu R, Bass AJ, Kim KM, Odegaard JI, Kim K, et al. Comprehensive molecular characterization of clinical responses to PD-1 inhibition in metastatic gastric cancer. Nat Med. (2018) 24:1449–58. doi: 10.1038/s41591-018-0101-z
35. Wang Y, Zhao C, Chang L, Jia R, Liu R, Zhang Y, et al. Circulating tumor DNA analyses predict progressive disease and indicate trastuzumab-resistant mechanism in advanced gastric cancer. EBioMedicine. (2019) 43:261–9. doi: 10.1016/j.ebiom.2019.04.003
36. Abbosh C, Birkbak NJ, Wilson GA, Jamal-Hanjani M, Constantin T, Salari R, et al. Phylogenetic ctDNA analysis depicts early-stage lung cancer evolution. Nature. (2017) 545:446–51. doi: 10.1038/nature22364
37. Tissot C, Toffart AC, Villar S, Souquet PJ, Merle P, Moro-Sibilot D, et al. Circulating free DNA concentration is an independent prognostic biomarker in lung cancer. Eur Respir J. (2015) 46:1773–80. doi: 10.1183/13993003.00676-2015
38. Ai B, Liu H, Huang Y, Peng P. Circulating cell-free DNA as a prognostic and predictive biomarker in non-small cell lung cancer. Oncotarget. (2016) 7:44583–95. doi: 10.18632/oncotarget.10069
39. Chen KZ, Lou F, Yang F, Zhang JB, Ye H, Chen W, et al. Circulating Tumor DNA Detection in Early-Stage Non-Small Cell Lung Cancer Patients by Targeted Sequencing. Sci Rep. (2016) 6:31985. doi: 10.1038/srep31985
40. Chen YT, Seeruttun SR, Wu XY, Wang ZX. Maximum somatic allele frequency in combination with blood-based tumor mutational burden to predict the efficacy of atezolizumab in advanced non-small cell lung cancer: a pooled analysis of the randomized POPLAR and OAK studies. Front Oncol. (2019) 9:1432. doi: 10.3389/fonc.2019.01432
41. Gandara DR, Paul SM, Kowanetz M, Schleifman E, Zou W, Li Y, et al. Blood-based tumor mutational burden as a predictor of clinical benefit in non-small-cell lung cancer patients treated with atezolizumab. Nat Med. (2018) 24:1441–8. doi: 10.1038/s41591-018-0134-3
42. Chae YK, Davis AA, Agte S, Pan A, Simon NI, Iams WT, et al. Clinical Implications of circulating tumor DNA tumor mutational burden (ctDNA TMB) in non-small cell lung cancer. Oncologist. (2019) 24:820–8. doi: 10.1634/theoncologist.2018-0433
43. Brahmer JR, Rodríguez-Abreu D, Robinson AG, Hui R, Csõszi T, Fülöp A, et al. Health-related quality-of-life results for pembrolizumab versus chemotherapy in advanced, PD-L1-positive NSCLC (KEYNOTE-024): a multicentre, international, randomised, open-label phase 3 trial. Lancet Oncol. (2017) 18:1600–9. doi: 10.1016/S1470-2045(17)30690-3
44. Togasaki K, Sukawa Y, Kanai T, Takaishi H. Clinical efficacy of immune checkpoint inhibitors in the treatment of unresectable advanced or recurrent gastric cancer: an evidence-based review of therapies. Onco Targets Ther. (2018) 11:8239–50. doi: 10.2147/OTT.S152514
45. Kim JY, Lee KH, Kang J, Borcoman E, Saada-Bouzid E, Kronbichler A, et al. Hyperprogressive disease during anti-PD-1 (PDCD1) / PD-L1 (CD274) therapy: a systematic review and meta-analysis. Cancers (Basel). (2019) 11:699. doi: 10.3390/cancers11111699
46. Mazieres J, Drilon A, Lusque A, Mhanna L, Cortot AB, Mezquita L, et al. Immune checkpoint inhibitors for patients with advanced lung cancer and oncogenic driver alterations: results from the IMMUNOTARGET registry. Ann Oncol. (2019) 30:1321–8. doi: 10.1093/annonc/mdz167
Keywords: NSCLC, immunotherapy, PD-1, angiogenic, ctDNA, TMB, PFS, Apatinib
Citation: Chen Y, Li X, Liu G, Chen S, Xu M, Song L and Wang Y (2020) ctDNA Concentration, MIKI67 Mutations and Hyper-Progressive Disease Related Gene Mutations Are Prognostic Markers for Camrelizumab and Apatinib Combined Multiline Treatment in Advanced NSCLC. Front. Oncol. 10:1706. doi: 10.3389/fonc.2020.01706
Received: 22 March 2020; Accepted: 30 July 2020;
Published: 04 September 2020.
Edited by:
Said Dermime, National Center for Cancer Care and Research, QatarReviewed by:
Cristina Maccalli, Sidra Medicine, QatarMona Omar Mohsen, University of Oxford, United Kingdom
Copyright © 2020 Chen, Li, Liu, Chen, Xu, Song and Wang. This is an open-access article distributed under the terms of the Creative Commons Attribution License (CC BY). The use, distribution or reproduction in other forums is permitted, provided the original author(s) and the copyright owner(s) are credited and that the original publication in this journal is cited, in accordance with accepted academic practice. No use, distribution or reproduction is permitted which does not comply with these terms.
*Correspondence: Lele Song, c29uZ2xlbGVAc2luYS5jb20=; Yina Wang, MTUwMzA5OUB6anUuZWR1LmNu
†These authors have contributed equally to this work