- 1Oncovirology Program, Instituto Nacional de Câncer, Rio de Janeiro, Brazil
- 2Division of Infectious Diseases, Department of Medicine, Weill Cornell Medicine, New York, NY, United States
- 3Drug Development Department (DITEP), Gustave Roussy, Paris-Saclay University, Villejuif, France
- 4Department of Pathology and Laboratory Medicine, Weill Cornell Medicine, New York, NY, United States
- 5Center for Research in Infectious Diseases (CIENI), National Institute of Respiratory Diseases (INER), Mexico City, Mexico
In people living with HIV (PLWH), chronic inflammation can lead to cancer initiation and progression, besides driving a dysregulated and diminished immune responsiveness. HIV infection also leads to increased transcription of Human Endogenous Retroviruses (HERVs), which could increase an inflammatory environment and create a tumor growth suppressive environment with high expression of pro-inflammatory cytokines. In order to determine the impact of HIV infection to HERV expression on the breast cancer microenvironment, we sequenced total RNA from formalin-fixed paraffin-embedded (FFPE) breast cancer samples of women HIV-negative and HIV-positive for transcriptome and retrotranscriptome analyses. We performed RNA extraction from FFPE samples, library preparation and total RNA sequencing (RNA-seq). The RNA-seq analysis shows 185 differentially expressed genes: 181 host genes (178 upregulated and three downregulated) and four upregulated HERV transcripts in HIV-positive samples. We also explored the impact of HERV expression in its neighboring breast cancer development genes (BRCA1, CCND1, NBS1/NBN, RAD50, KRAS, PI3K/PIK3CA) and in long non-coding RNA expression (AC060780.1, also known as RP11-242D8.1). We found a significant positive association of HERV expression with RAD50 and with AC060780.1, which suggest a possible role of HERV in regulating breast cancer genes from PLWH with breast cancer. In addition, we found immune system, extracellular matrix organization and metabolic signaling genes upregulated in HIV-positive breast cancer. In conclusion, our findings provide evidence of transcriptional and retrotranscriptional changes in breast cancer from PLWH compared to non-HIV breast cancer, including dysregulation of HERVs, suggesting an indirect effect of the virus on the breast cancer microenvironment.
Introduction
The majority of mammary tumors in mice are caused by the mouse mammary tumor virus (MMTV) (1), and the suggestion that a retrovirus related to MMTV might be involved in breast cancer in humans is one of the longest running controversies in human retrovirology (2, 3). Early studies of the breast cancer cell line T47D showed retroviral particles which were responsive to estradiol (4–8). These were later identified as human endogenous retrovirus (HERV)-K envelope transcripts, and were found both in cell lines and in breast cancer tissues (9, 10). HERVs are “fossil” retrovirus sequences remnant in the human genome, which originated millions of years ago from retrovirus germline cell infections. They are transmitted vertically, but they do not show infective capabilities in humans (11–13). They comprise ~8% of the human genome (14).
In women with breast cancer, HERV-derived material has been found in peripheral blood (15, 16). The consequences of changes in HERV expression include translation of some HERV proteins and stimulation of anti-HERV immune responses (17–19). A chimeric antigen receptor (CAR) specific for HERV-K env protein was effective in reducing tumor burden in a mouse model (20). The corollary is that HERV-K expression may be related to oncogenesis, and that HERV-K Env proteins appear to play an important role in tumorigenesis and metastasis (21). A recent study showed that the endogenous retrovirus-derived long noncoding RNA (lncRNA) TROJAN promotes triple-negative breast cancer progression via ZMYND8 degradation (22). Thus, expression of HERVs in breast cancer may relate to pathogenesis, but also to generation of anti-HERV immunity with protective potential.
In HIV infection, we, and others, have found that HERV expression is changed in CD4+ cells (23–41), and that it is partially mediated by HIV Vif (28). Although no bystander specific HERV activation has been observed, interestingly, people living with HIV (PLWH) have higher levels of HERV-K RNA expression, which is negatively associated with the level of T cell activation (28, 31).
No study has yet addressed the impact of HIV mediated HERV transcription in breast cancer. Since breast cancer cell lines and tissues appear to express HERVs, and the local microenvironment of breast cancer contains potentially HIV infected-CD4+ cells, there is the possibility of direct or indirect interaction between HERVs dysregulated by HIV and HERVs dysregulated in breast cancer. We undertook this retrospective pilot analysis of HERV expression from archived formalin-fixed, paraffin-embedded (FFPE) tissue samples in women who had breast cancer with or without HIV infection.
Materials and Methods
Sample Collection
FFPE blocks of breast tissue from 10 women with invasive ductal carcinoma (IDCA) of the female mammary gland (four HIV-negative: six HIV-positive) registered from 2003 to 2014 at the Brazilian National Cancer Institute (INCA), were used in this study.
The study was approved by the local Institutional Review Board, as well as by the Brazilian National Ethics Committee. All subjects had signed informed consent forms in accordance with the Declaration of Helsinki.
RNA Extraction
FFPE tissue blocks were sectioned into 3 μm thick curls and placed in 2 mL Safe-Lock tubes (Eppendorf, cat. no. 022600044), with the first three 3 μm sections discarded. No more than ten sample curls were placed in each Eppendorf tube, ~30 μm worth of material, according to manufacturer's instructions of a maximum 40 μm processing limit. Samples were then deparaffinized in 320 μL Deparaffinization Solution (Qiagen, cat. no. 19093) at 56°C for 3 min, then allowed to cool at room temperature.
RNA extraction and purification from FFPE tissue sections were carried out using the RNeasy FFPE Kit (Qiagen, cat. no. 73504). The RNeasy MinElute spin columns were incubated for 5 min with RNase-free water before centrifugation. Samples were eluted in 20 μL RNase-free water. A DNase treatment was added using HL-dsDNase kit (ArticZymes, cat. no. 70800-201 250U), with additive MgCl2 (ThermoFisher Scientific, cat. no. AB0359) and DTT, Molecular Grade (Promega, cat. no. P117A). RNA samples were DNase-treated at room temperature for 15 min, followed by a 55°C 10-min incubation to complete enzyme activity. RNA was stored at −80°C.
RNA Quantification and Quality Assessment
RNA was assessed for quantity on a Qubit™ 2.0 Fluorometer using the RNA Broad Range Assay Kit (Invitrogen, cat. no. Q32855). RNA was assessed for quality and integrity on an Agilent Bioanalyzer 2100 unit using Agilent RNA 6000 Nano Series II Kit (Agilent Technologies, cat. no. 5067-1511).
Library Preparation and Quality Control
RNA samples were prepared with Ovation® Human FFPE RNA-Seq Library Systems (Nugen, cat. no. 0340, 0341). Clean-up steps, including magnetic bead purifications, were performed with freshly made 70% EtOH from 100% proof Absolute Ethanol (Fisher BioReagents™, cat. no. BP2818-500), nuclease-free water (Ambion®, cat. no. AM9939), and DynaMag™-96 Side magnetic plates (Invitrogen, cat. no. 12331D). As a rule, each library was amplified for 18 PCR cycles. Amplified library products were routinely checked for quantity and purity before pooling for Next Generation Sequencing. Final products were assessed on an Agilent Bioanalyzer 2100 unit using the High Sensitivity DNA Kit (Agilent Technologies, cat. no. 5067-4626). Final products were also assessed using KAPA Library Quantification Kit (Illumina) and/or ROX Low qPCR Mix (Roche, cat. no. 07960336001). If samples passed quality control inspection, each library prep was diluted at 4 nM using 10 mM Tris-HCl, 0.1% Tween-20, pH 8.5 (Teknova, cat. no. T7724). Libraries were pooled in 8 sample sets, and sequenced in a paired-end mode, Mid-Output platform on an Illumina NextSeq 2000 Sequencing System.
Bioinformatics
BCL2FastQ2 Conversion Software (version 2.20, Illumina Inc.) was used to demultiplex data and convert the sequencing files to reads in FASTQ format. Paired-end reads were trimmed for adaptors with Trimmomatic (42) and filtered by quality (phred ≥ 30) and length (≥ 35). Filtered paired-end reads were then aligned to the reference human genome (hg38) using Bowtie2 (43). The Bowtie2 output was used as input for Telescope software (44) to define and quantify retrotransposon elements (HERV and LINE-1) using annotations, previously described and available at https://github.com/mlbendall/telescope_annotation_db/tree/master/builds.
In addition, we mapped all reads to the human genome (hg38) with STAR (45). The output was then used for gene quantification using Htseq-count (46) with Gencode version 31. Telescope and Htseq-count table outputs were merged and genes or retrotransposon elements which were present in less than two samples were removed. The processed output was then used to calculate HERVs and genes differentially expressed genes in breast cancer from HIV-positive vs. HIV-negative patients using DESeq2, Wald-test (47).
Volcano plots were drawn with the Bioconductor EnhancedVolcano (https://github.com/kevinblighe/EnhancedVolcano). HERVs and host genes with adjusted p-value <0.05 and absolute(log2FoldChange) > 1.0 were considered differentially expressed genes (DEGs) and results were shown using pheatmap and ggplot R packages. HERV localization in the human genome was visualized with integrative genomics viewer (IGV) software (Broad Institute, Cambridge, MA) using Gencode version 31 and HERV annotations as previously described (41). We also analyzed nearby HERVs to genes associated with breast cancer development (Supplementary Table 1) (48–50). The HERV localization was defined and HERV and nearby gene expression results were shown in graphs using ggplot R packages.
Gene set enrichment analysis (GSEA) was performed with WebGestal (available at http://webgestalt.org/) using Reactome as functional pathway database (51). We also analyzed the differentially immune gene expression, including CD4 and CD8 (CD8A and CD8B) mRNA in the samples to check T-cell-derived material in transcriptome data. The graphs were constructed using the ggplot R and the adjusted p-value was calculated from DEseq2 analysis performed using the Wald-test.
All data generated in the study has been deposited in GEO under the accession number GSE149156.
Immunohistochemistry
Immunohistochemical (IHC) was done using a standard protocol for FFPE tissues. Breast tumor biopsies from six HIV-positive and four HIV-negative women were sectioned into 10 μm slices and then deparaffinized, rehydrated, and subjected to antigen retrieval with 10 mM sodium citrate buffer and heat for 10 min. Staining was done using antibodies for four differentially expressed genes PROM1/CD133 (CST D2V8Q XP® Rabbit mAb #64326, 1:400 dilution), LAMB3 (Abcam antibody ab97765, 1:400 dilution), SLC6A4 (ThermoFisher polyclonal antibody PA5-50624, 1:100 dilution), and MRPS12 (ThermoFisher polyclonal antibody 15225-1-AP, 1:50 dilution). Detection of the signal was done using SignalStain Boost Detection Reagent (Cell Signaling Technology) and brightfield images were taken using a Keyence BZ-X810 microscope.
Results
We analyzed RNA-seq data of breast tissue from women with IDCA HIV-positive and -negative breast cancer. Samples from both HIV-positive and -negative groups were predominantly of luminal subtype and of advanced stage at diagnosis (Table 1). The one case of triple-negative IDCA was not of medullary subtype, and there was no morphologically apparent increase in tumor-infiltrating lymphocytes in any of the samples. No patient received neo-adjuvant therapy before resection. It is important to note that, although gene expression data was available, IDCA subtyping was performed using immunohistochemical analysis (IHC) of estrogen receptor and progesterone receptor and a combination of IHC and in situ hybridization analysis, as necessary. The transcriptomic analyses showed no significant difference in expression of ESR1, ESR2, PGR, or ERBB2 by RNA-Seq analysis. We found a total of 181 DEGs when comparing both groups, HIV-positive and HIV-negative breast cancer [FDR <0.05, absolute(log2FoldChange) > 1.0; Figures 1A,B]. One hundred and seventy eight of those genes were upregulated and three were downregulated in HIV-positive breast cancer (Supplementary Table 2). PROM1, LAMB3, OSR1, and CAVIN1 were the most upregulated host genes in HIV-positive women with a long2foldchange of 20.8, 7.9, 7.5, and 7.1, respectively (Supplementary Table 2). In contrast, SLC5A11, SLC6A4 and MRPS12 were the most downregulated in HIV-positive breast cancer with a long2foldchange of −6.7, 6.5 and −4.7, respectively (Supplementary Table 2). To biologically validate these findings, we performed IHC assay to detect the expression of four proteins (PROM1, LAMB3, SLC6A4, and MRPS12) generated from those genes found to be differentially expressed in HIV-positive breast cancer samples (Figure 1C). PROM1 and LAMB3 showed lower protein levels in HIV-negative breast cancer tissues compared in those found in HIV-positive breast cancer tissues. Conversely, protein levels of Mrps12 and Slc6a4 were increased in HIV-negative tissues compared to HIV-positive tissues. Thus, these data corroborate with transcriptome results (Supplementary Table 2).
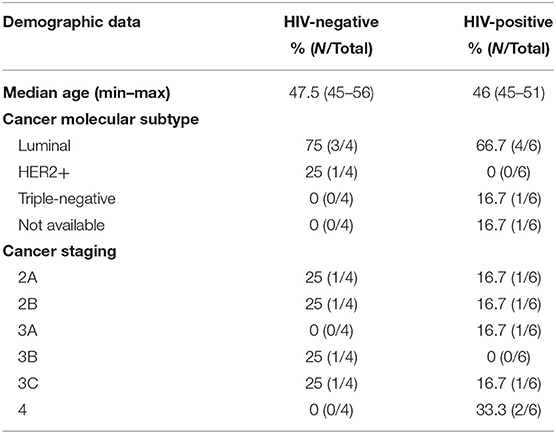
Table 1. Demographic and pathologic data of breast cancer samples from HIV-negative and HIV-positive women.
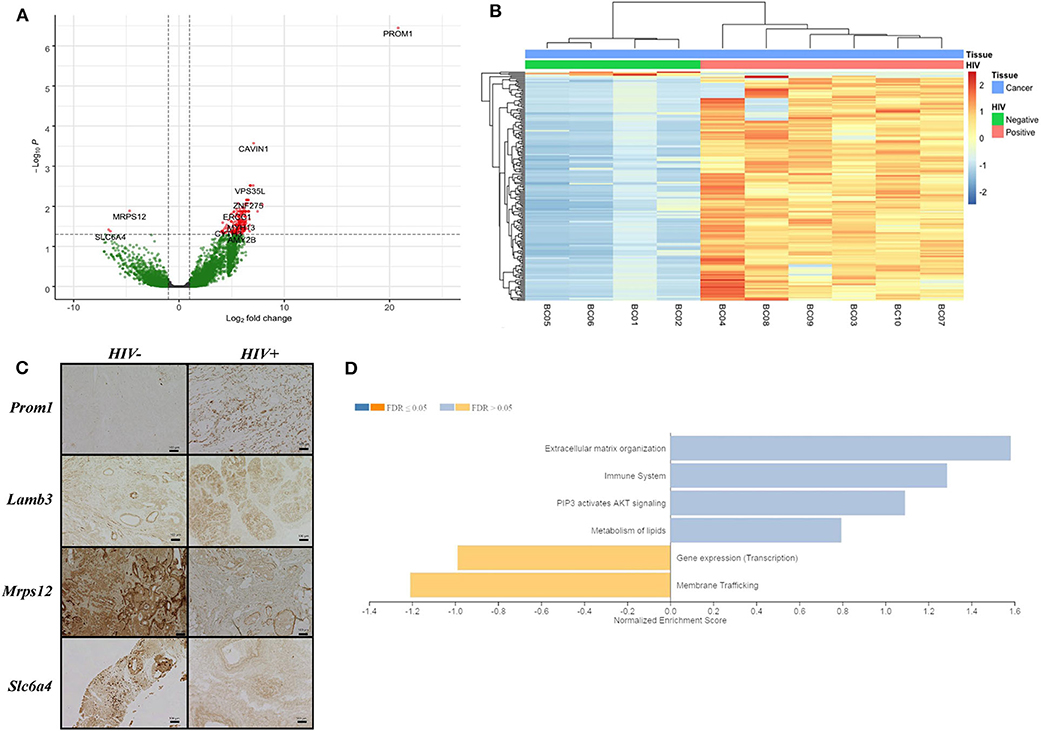
Figure 1. Differentially expressed genes in breast cancer from HIV patients. (A) Volcano plot shows differentially expressed HERV and host genes, up-regulated (right side) and down-regulated (left side) in breast cancer HIV-positive samples. Threshold lines to adjusted p-value <0.05 (x-axis) and log2FoldChange > 1.0 (y-axis) are shown. Green and red colors indicate significant and non-significant genes, respectively, found in the differential gene expression analysis. (B) Heatmap plot demonstrates 181 differentially expressed genes in the horizontal dendogram to breast cancer samples (labeled in blue) from HIV-positive (labeled in pink) and HIV-negative (labeled in green). Unsupervised clustering for rows and columns was performed using Euclidean distances and a complete linkage method. (C) Immunohistochemical analysis of four host proteins (PROM1, LAMB3, SLC6A4, and MRPS12) in HIV-negative and HIV-positive breast cancer tissues. (D) GSEA illustrates cellular pathway gene enrichment in HIV-positive samples. False discovery rate (FDR) shows up- and down-regulated pathways in light orange and blue, respectively, for q-value > 0.05, while q-value <0.05 is represented in dark orange and blue.
Furthermore, the results showed enrichment of extracellular matrix organization, immune system, PI3K/AKT signaling and lipid metabolism pathways, all upregulated in HIV-positive samples, but at non-significant levels (Figure 1D and Supplementary Table 3). Transcription machinery and membrane trafficking pathways were found to be downregulated in HIV-positive samples (Figure 1D and Supplementary Table 3).
Additionally, we quantified locus-specific HERV expression at 14,968 genomic loci using Telescope, a software developed for solving multimapping reads, and performed a combined differential expression analysis with all annotated genes. Four HERV transcripts (HERVL_22q13.31, HERVL40_2p23.3b, HUERSP1_15q22.31, LTR19_14q23.1) were significantly upregulated in HIV-positive samples (Figure 2). HERVL40_2p33.3b and LTR19_14q33.1 were present in the intronic region of intersectin (ITSN2) and Pecanex 4 (PCNX4) genes, respectively (Figures 3B,D and Supplementary Figures 1B,D), HERVL_22q13.31 localized in an alternative polyadenylation site of Fibulin 1 (FBLN1) gene and HUERSP1_15q22.31 is an intergenic HERV (Figures 3A,C and Supplementary Figures 1A,C). Despite none of these host genes was statistically differentially expressed, we analyzed their expression in association with their nearby HERV expression (Supplementary Figure 1). Interestingly, the HUERSP1_15q22.31 expression was associated with the upstream SMAD6 long non-coding RNA expression, lnc-SMAD6 (AC110048.2), in HIV-positive samples (R2 = 0.86, p-value = 0.029) (Supplementary Figure 1C). In the same way, the LTR19_14q23.1 and PCNX4 expression also showed a positive association in HIV-positive samples (R2 = 0.86, p-value = 0.027) (Supplementary Figure 1D). In addition, we also analyzed HERVs that were associated, by localization, with breast cancer development genes (BRCA1, CCND1, ATM, NBS1/NBN, RAD50, KRAS, PI3K/PIK3CA) (Supplementary Table 1). Although both HERV and genes in this analysis were not differentially expressed in HIV-positive samples, we found significant association between RAD50 and its intronic HERV, ERVLE_5q31.1d, expression (R2 = 0.82, p-value = 0.044) (Supplementary Figure 2A). Additionally, the last two exons of the BRCA1 long non-coding RNA, lnc-BRCA1 (AC060780.1), are contained in the HARLEQUIN_17q21.31 and therefore the expression of both elements, the lncRNA and the HERV are also correlated (R2 = 0.96, p-value = 0.0019) (Supplementary Figure 2D). These results suggest a possible role of HERVs in the regulation of at least some of the breast cancer development genes.
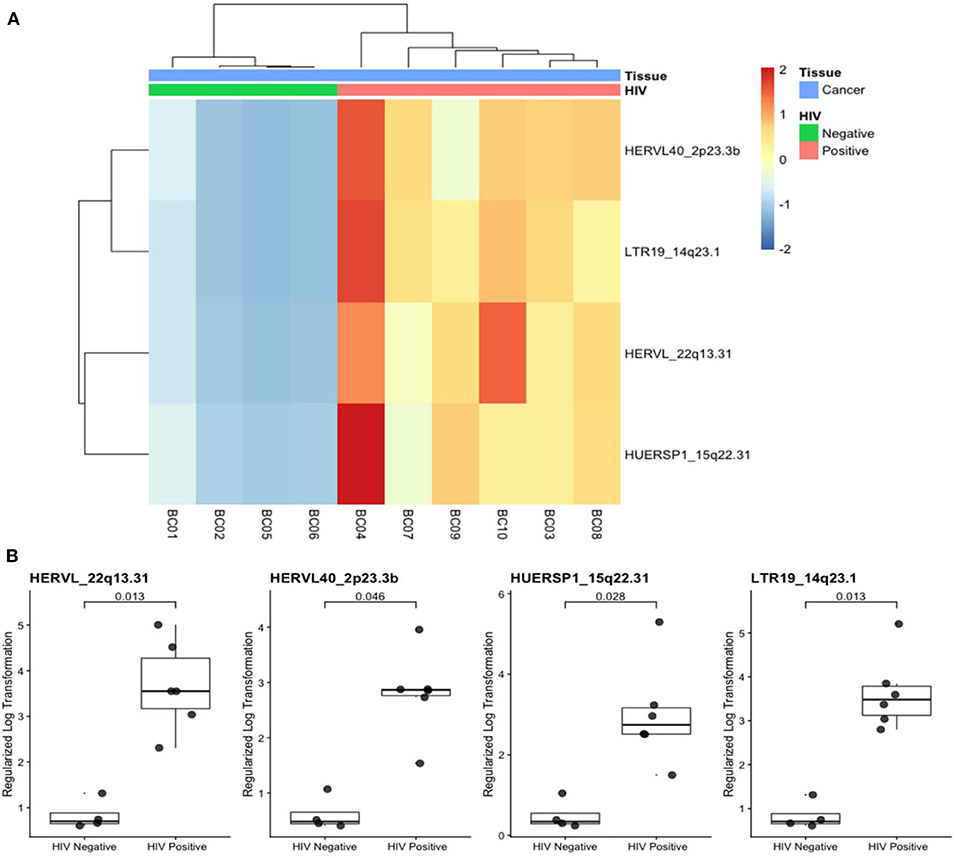
Figure 2. Differentially expressed HERV transcripts in breast cancer from HIV-positive patients. (A) Heatmap plot shows the four differentially expressed HERVs in horizontal dendogram to breast cancer samples (labeled in blue) from HIV-positive (pink) and HIV negative (green) are annotated. (B) Expression of the four HERV transcripts with adjusted p-values are shown.
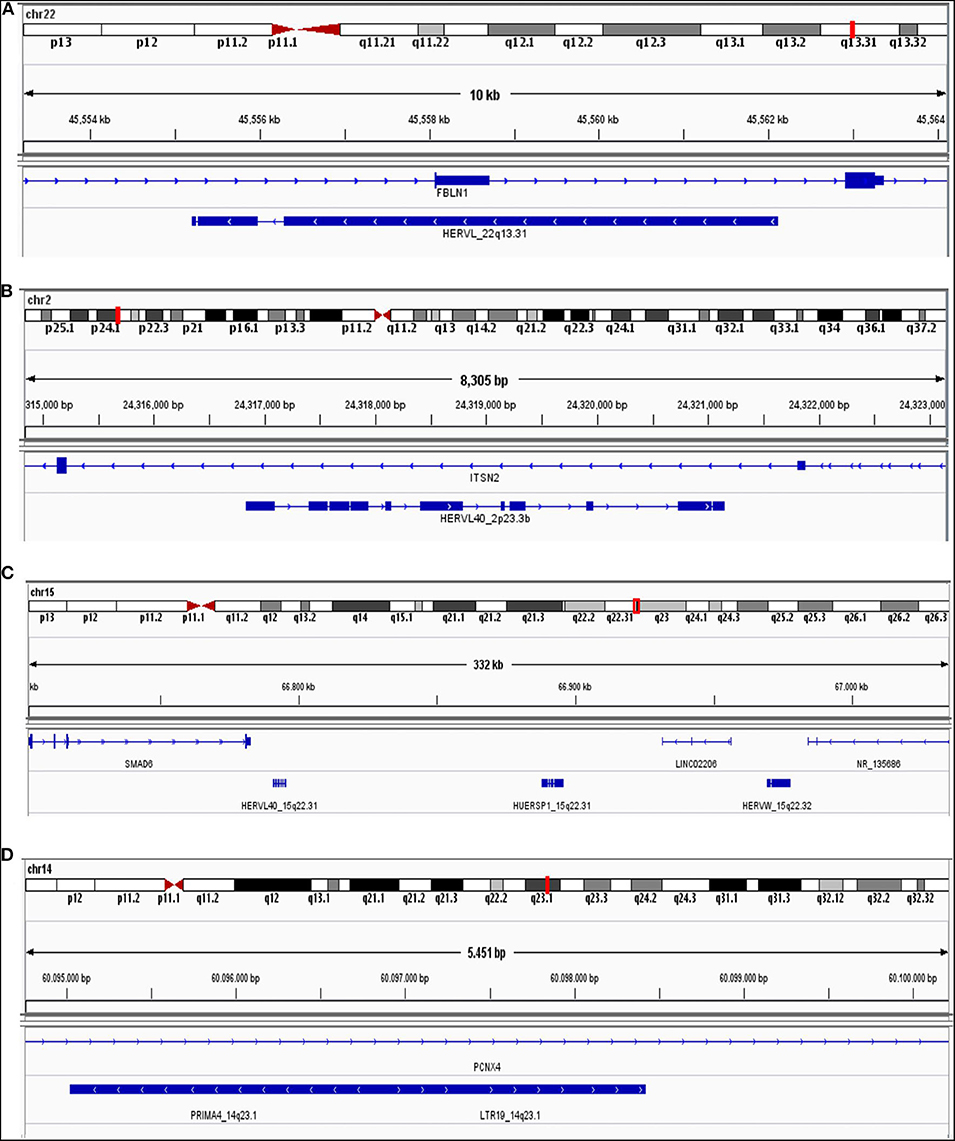
Figure 3. Genome localization from differentially expressed HERV genes. HERVL_22q13.31 (A), HERVL40_2p23.3b (B), HUERSP1_15q22.31 (C), and LTR19_14q33.1 (D) are shown in human genome (hg38). Chromosome number and region, beside exon (blue square) and intron (blue line) are illustrated.
We also analyzed CD4 and CD8 mRNA expression in the samples, as a proxy for the presence of T-cell-derived material in transcriptome data. The CD8 mRNA expression was higher in HIV-positive than in HIV-negative samples (Figure 4), but at non-significant levels. We further analyzed the differential expression of T-cell signature genes (Supplementary Figure 3), and we found the PIK3IP1 gene upregulated in HIV-positive samples. This pilot study highlighted the importance of additional studies to confirm the increase of tumor-infiltrating lymphocytes and their immune effects on the IDCA microenvironment in HIV-positive patients.
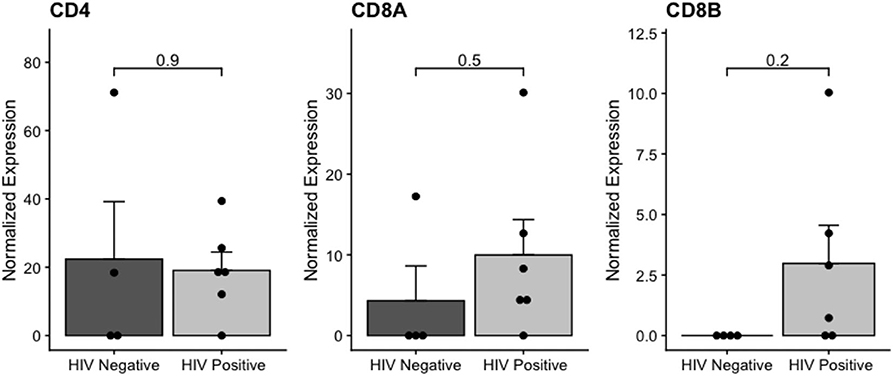
Figure 4. Normalized CD4 and CD8 expression in HIV-positive and HIV-negative from breast cancer samples. The adjusted p-value is shown (FDR).
Discussion
Breast carcinoma is a heterogeneous malignancy originating from the epithelium of the terminal ductal-lobular unit, and it is the most common malignancy in women worldwide (52). Interestingly, women living with HIV (WLWH) have an approximate 40% lower risk for developing this kind of cancer (SIR = 0.63, 95% CI = 0.58–0.68) and have a lower frequency of breast tumors larger than 5 cm (SIR = 0.65, CI 95% = 0.50–0.83) (53). However, the biological mechanism associated with the lower incidence of breast cancer in WLWH is unknown.
In this study, we have described the transcriptome and retrotranscriptome of breast cancer FFPE samples from HIV-positive and HIV-negative women with breast cancer. RNA extracted from FFPE samples may be partially degraded, and performing RNA sequencing from these samples is technically challenging (54–56). However, studies have shown that RNA-seq from FFPE tissues can be used as an alternative for frozen samples, and it can promote advances in clinical retrospective studies, especially in rare types of cancer. The rarity of breast cancer among WLWH poses a major hurdle to prospective analyses of fresh tumor samples, which yields higher-quality sequencing data.
Herein, we found 181 differentially expressed genes and HERVs between HIV-positive and HIV-negative women with breast cancer, with 178 genes upregulated in HIV-positive samples. These data agree with a recent study on HIV-related diffuse large B-cell lymphoma (57), in which digital gene expression profiling and array comparative genomic hybridization from FFPE samples were performed. Diffuse large B-cell lymphoma from HIV infected patients showed differential gene expression, and genes associated with cell cycle progression, DNA replication and DNA damage repair were significantly increased in HIV-positive compared to HIV-negative tumors (57). HIV infection is characterized by persistent inflammation and cell-signaling pathway dysregulation in immune cells (58). Together, these results show that HIV may regulate the host gene expression and impact cellular pathways in addition to inducing HERV expression.
The upregulation of HERV has been implicated in oncogenesis and metastases of breast cancer cells (21). Expression of the HERV-K protein np9 is increased in breast cancer (19) and is considered a viral oncogene due to its association to cellular signaling pathways such as WNT, ERK, Akt and Notch1 (59, 60). HERV and breast cancer associations are frequently based on the analysis of HERV-K expression (9, 16, 19, 61, 62). In this study, we did not find differential HERV-K expression profiles in breast cancer samples from HIV-positive women when compared to samples from HIV-negative women. However, expression of other HERV families have been described in various other types of cancer such as colorectal, stomach and others (63). Studies of HIV/HERV associations also mostly have relied on HERV-K analyses (28, 31, 32, 64).
Furthermore, one critical aspect of HIV pathogenesis is the suppression of the intracellular viral restriction factor APOBEC3 by the HIV protein Vif in CD4 T cells (65). Reduced APOBEC3 activity has been associated with activation of HERV in HIV infection (28, 66). Despite the defined associations between HERV and both HIV and breast cancer, very little is known about the impact of HERV expression in the context of breast cancer in WLHW.
In the current study, we used the Telescope program, a pipeline for locus specific HERV expression identification (44, 67). Telescope is able to identify HERV locus specific expression in RNA-seq data with higher accuracy than other methods (44). We found four overly expressed HERV genes in HIV-positive from breast cancer samples (HERVL_22q13.31, HERVL40_2p23.3b, HUERSP1_15q22.31, LTR19_14q23.1), two of them from HERV-L clades. Interesting, we previously reported HERV-L specific immunity in HIV-1 infection, characterizing a potential novel target for assessment of HIV pathogenesis (27). Moreover, HERV-L shows elevated numbers of somatic single-nucleotide variations in cancer (68), which may impact cancer progression. In addition, HERVs are able to induce carcinogenesis and cancer progression by regulating nearby host genes (12, 69, 70). Herein we reported positive association between expression of some HERVs and their neighbor genes. The HUERSP1_15q22.31 and LTR19_14q23.1, differential expressed in HIV-positive samples, were associated with the SMAD6 long non-coding RNA and PCNX4 expression, respectively. In addition, the analysis of HERVs and breast cancer oncogenes also showed a positive association in HIV-positive samples. The ERVLE_5q31.1d and HARLEQUIN_17q21.31 were associated, respectively, with RAD50 and BRCA1 long non-coding RNA expression. Mutation in RAD50 is associated with breast cancer, genome instability and poor survival (71, 72). Additionally, dysregulation of long non-coding RNA expression has been associated with tumorigenesis (73, 74). Together, these data suggest the association between HERV and host gene expression, including oncogenes, which may have a key role in carcinogenesis in breast tissues from HIV-positive persons. HERV expression has also been involved in the aggressiveness and plasticity of cancer cells, showing an important effect in the tumor microenvironment (75). We also found an upregulation of CAVIN1 (caveolae associated protein 1) in HIV-positive breast cancer samples. This protein, which is present in abundance at the cell surface is associated with caveolae formation, and it has been proposed as tumor suppressor protein (76, 77). In contrast, studies have shown proteins of the CAVIN family downregulated in breast cancer (77) from HIV-negative samples. These findings suggest that upregulated CAVIN1 in HIV infection might have an important role in HIV-positive breast cancer suppression. In addition, PROM1 (prominin 1) was found upregulated in HIV-positive breast cancer samples, a gene commonly overexpressed in ovarian, esophageal and liver cancer (78). Its expression is negatively associated with cancer prognosis (78, 79). However, its biological function and role are not well understood in breast cancer.
The breast cancer microenvironment is complex, and there are many interactions between different kind of cells that may impact breast cancer gene expression (80–82). Interestingly, we found a higher but non-significant CD8 mRNA expression in HIV-positive breast cancer compared to HIV-negative, suggesting a higher concentration of tumor-infiltrating lymphocytes in these samples. We also found immune pathway enrichment in HIV-positive breast cancer women according to GSEA. In addition, by analyzing immune T-cell signature genes, we showed PIK3IP1 upregulated in HIV-positive breast cancer samples. The PIK3P1 protein is a negative regulator of T-cell activation and of antitumor T-cell immunity (83, 84). Interestingly, tumor-infiltrating lymphocytes are able to induce an immune response, and their presence is often associated with a good prognosis across a spectrum of malignancies in HIV-negative patients (85, 86). Our findings may also indicate differential immune response regulation and lymphocyte recruitment in the HIV-related breast cancer microenvironment. Therefore, this pilot report highlights the importance of additional studies of HIV-positive breast cancer patients to evaluate HERV expression effects on breast cancer cells.
Based on our results, the impact of HIV infection in the context of breast cancer in WLWH might rely on a specific HERV expression profile different from the previously HERV-K and breast cancer association seen in HIV-negative women. This pilot study is the first to analyze the impact of HIV infection in breast cancer using transcriptome and retrotranscriptome data and it can serve as basis to further studies. Despite of limited samples number due the rarity of breast cancer among WLWH, our findings suggest that HIV infection might indirectly modulate host and HERV gene expressions in the breast cancer microenvironment. Future studies to better understand the interplay between HIV and HERVs in the breast cancer microenvironment are warranted.
Data Availability Statement
The datasets presented in this study can be found in online repositories. The names of the repository/repositories and accession number(s) can be found below: the NCBI Gene Expression Omnibus (GSE149156).
Ethics Statement
The studies involving human participants were reviewed and approved by the local Institutional Review Board, as well as by the Brazilian National Ethics Committee. The patients/participants provided their written informed consent to participate in this study.
Author Contributions
GC, GB, LI, SC, CO, GR-T, MS, DN, MB, FL, MdM, and RF: conceptualization. GC, GB, LI, PdC, JM, and RF: investigation. GC and DN: writing original draft preparation and writing review and editing. MS, DN, MB, FL, and MdM: supervision. DN: funding acquisition. All authors contributed to the article and approved the submitted version.
Funding
The work was supported in part by US National Institutes of Health grants: CA206488 (DN) and AI076059 (DN), and by the Brazilian Research Council grant #305765/2015-9 (MS). Additionally, this work was partially funded by the American Society of Tropical Medicine & Hygiene through the Benjamin H. Kean Travel Fellowship in Tropical Medicine (JM). GC is the recipient of a Ph.D. fellowship by the Brazilian National Cancer Institute (INCA). MM supported in part by the Department of Medicine, Fund for the Future program at Weill Cornell Medicine, sponsored by the Kellen Foundation.
Conflict of Interest
The authors declare that the research was conducted in the absence of any commercial or financial relationships that could be construed as a potential conflict of interest.
Supplementary Material
The Supplementary Material for this article can be found online at: https://www.frontiersin.org/articles/10.3389/fonc.2020.553983/full#supplementary-material
References
1. Bittner JJ. Some possible effects of nursing on the mammary gland tumor incidence in mice. Science (80-). (1936) 84:162. doi: 10.1126/science.84.2172.162
2. Lawson JS, Glenn WK. Evidence for a causal role by mouse mammary tumour-like virus in human breast cancer. npj Breast Cancer. (2019) 5:40. doi: 10.1038/s41523-019-0136-4
3. Lehrer S, Rheinstein PH. Mouse mammary tumor viral env sequences are not present in the human genome but are present in breast tumors and normal breast tissues. Virus Res. (2019) 266:43–7. doi: 10.1016/j.virusres.2019.03.011
4. Ono M, Kawakami M, Ushikubo H. Stimulation of expression of the human endogenous retrovirus genome by female steroid hormones in human breast cancer cell line T47D. J Virol. (1987) 61:2059–62. doi: 10.1128/JVI.61.6.2059-2062.1987
5. Seifarth W, Skladny H, Krieg-Schneider F, Reichert A, Hehlmann R, Leib-Mösch C. Retrovirus-like particles released from the human breast cancer cell line T47-D display type B- and C-related endogenous retroviral sequences. J Virol. (1995) 69:6408–16. doi: 10.1128/JVI.69.10.6408-6416.1995
6. Etkind PR, Lumb K, Du J, Racevskis J. Type 1 HERV-K genome is spliced into subgenomic transcripts in the human breast tumor cell line T47D. Virology. (1997) 234:304–8. doi: 10.1006/viro.1997.8670
7. Wang-Johanning F, Frost AR, Jian B, Epp L, Lu DW, Johanning GL. Quantitation of HERV-K env gene expression and splicing in human breast cancer. Oncogene. (2003) 22:1528–35. doi: 10.1038/sj.onc.1206241
8. Nguyen TD, Davis J, Eugenio RA, Liu Y. Female sex hormones activate human endogenous retrovirus type K through the OCT4 transcription factor in T47D breast cancer cells. AIDS Res Hum Retroviruses. (2019) 35:348–56. doi: 10.1089/aid.2018.0173
9. Wang-Johanning F, Frost AR, Johanning GL, Khazaeli MB, LoBuglio AF, Shaw DR, et al. Expression of human endogenous retrovirus k envelope transcripts in human breast cancer. Clin Cancer Res. (2001) 7:1553–60.
10. Frank O, Verbeke C, Schwarz N, Mayer J, Fabarius A, Hehlmann R, et al. Variable transcriptional activity of endogenous retroviruses in human breast cancer. J Virol. (2008) 82:1808–18. doi: 10.1128/JVI.02115-07
11. Hohn O, Hanke K, Bannert N. HERV-K(HML-2), the best preserved family of HERVs: endogenization, expression, and implications in health and disease. Front Oncol. (2013) 3:246. doi: 10.3389/fonc.2013.00246
12. Garcia-Montojo M, Doucet-O'Hare T, Henderson L, Nath A. Human endogenous retrovirus-K (HML-2): a comprehensive review. Crit Rev Microbiol. (2018) 44:715–38. doi: 10.1080/1040841X.2018.1501345
13. Grandi N, Tramontano E. Human endogenous retroviruses are ancient acquired elements still shaping innate immune responses. Front Immunol. (2018) 9:2039. doi: 10.3389/fimmu.2018.02039
14. Griffiths DJ. Endogenous retroviruses in the human genome sequence. Genome Biol. (2001) 2:1–5. doi: 10.1186/gb-2001-2-6-reviews1017
15. Rhyu D-W, Kang Y-J, Ock M-S, Eo J-W, Choi Y-H, Kim W-J, et al. Expression of human endogenous retrovirus env genes in the blood of breast cancer patients. Int J Mol Sci. (2014) 15:9173–83. doi: 10.3390/ijms15069173
16. Wang-Johanning F, Li M, Esteva FJ, Hess KR, Yin B, Rycaj K, et al. Human endogenous retrovirus type K antibodies and mRNA as serum biomarkers of early-stage breast cancer. Int J Cancer. (2014) 134:587–95. doi: 10.1002/ijc.28389
17. Wang-Johanning F, Radvanyi L, Rycaj K, Plummer JB, Yan P, Sastry KJ, et al. Human endogenous retrovirus K triggers an antigen-specific immune response in breast cancer patients. Cancer Res. (2008) 68:5869–77. doi: 10.1158/0008-5472.CAN-07-6838
18. Wang-Johanning F, Rycaj K, Plummer JB, Li M, Yin B, Frerich K, et al. Immunotherapeutic potential of anti-human endogenous retrovirus-K envelope protein antibodies in targeting breast tumors. J Natl Cancer Inst. (2012) 104:189–210. doi: 10.1093/jnci/djr540
19. Zhao J, Rycaj K, Geng S, Li M, Plummer JB, Yin B, et al. Expression of human endogenous retrovirus type K envelope protein is a novel candidate prognostic marker for human breast cancer. Genes Cancer. (2011) 2:914–22. doi: 10.1177/1947601911431841
20. Zhou F, Krishnamurthy J, Wei Y, Li M, Hunt K, Johanning GL, et al. Chimeric antigen receptor T cells targeting HERV-K inhibit breast cancer and its metastasis through downregulation of Ras. Oncoimmunology. (2015) 4:e1047582. doi: 10.1080/2162402X.2015.1047582
21. Zhou F, Li M, Wei Y, Lin K, Lu Y, Shen J, et al. Activation of HERV-K Env protein is essential for tumorigenesis and metastasis of breast cancer cells. Oncotarget. (2016) 7:84093–117. doi: 10.18632/oncotarget.11455
22. Jin X, Xu XE, Jiang YZ, Liu YR, Sun W, Guo YJ, et al. The endogenous retrovirus-derived long noncoding RNA TROJAN promotes triple-negative breast cancer progression via ZMYND8 degradation. Sci Adv. (2019) 5:1–15. doi: 10.1126/sciadv.aat9820
23. Contreras-Galindo R, González M, Almodovar-Camacho S, González-Ramírez S, Lorenzo E, Yamamura Y. A new Real-Time-RT-PCR for quantitation of human endogenous retroviruses type K (HERV-K) RNA load in plasma samples: Increased HERV-K RNA titers in HIV-1 patients with HAART non-suppressive regimens. J Virol Methods. (2006) 136:51–7. doi: 10.1016/j.jviromet.2006.03.029
24. Contreras-Galindo R, Kaplan MH, Markovitz DM, Lorenzo E, Yamamura Y. Detection of HERV-K(HML-2) viral RNA in plasma of HIV type 1-infected individuals. AIDS Res Hum Retroviruses. (2006) 22:979–84. doi: 10.1089/aid.2006.22.979
25. Contreras-Galindo R, López P, Vélez R, Yamamura Y. HIV-1 infection increases the expression of human endogenous retroviruses type K (HERV-K) in vitro. AIDS Res Hum Retroviruses. (2007) 23:116–22. doi: 10.1089/aid.2006.0117
26. Contreras-Galindo R, Kaplan MH, He S, Contreras-Galindo AC, Gonzalez-Hernandez MJ, Kappes F, et al. HIV infection reveals widespread expansion of novel centromeric human endogenous retroviruses. Genome Res. (2013) 23:1505–13. doi: 10.1101/gr.144303.112
27. Garrison KE, Jones RB, Meiklejohn DA, Anwar N, Ndhlovu LC, Chapman JM, et al. T cell responses to human endogenous retroviruses in HIV-1 infection. PLoS Pathog. (2007) 3:e165. doi: 10.1371/journal.ppat.0030165
28. Jones RB, Garrison KE, Mujib S, Mihajlovic V, Aidarus N, Hunter DV, et al. HERV-K-specific T cells eliminate diverse HIV-1/2 and SIV primary isolates. J Clin Invest. (2012) 122:4473–89. doi: 10.1172/JCI64560
29. Monde K, Contreras-Galindo R, Kaplan MH, Markovitz DM, Ono A. Human endogenous retrovirus K gag coassembles with HIV-1 gag and reduces the release efficiency and infectivity of HIV-1. J Virol. (2012) 86:11194–208. doi: 10.1128/JVI.00301-12
30. Monde K, Terasawa H, Nakano Y, Soheilian F, Nagashima K, Maeda Y, et al. Molecular mechanisms by which HERV-K Gag interferes with HIV-1 Gag assembly and particle infectivity. Retrovirology. (2017) 14:27. doi: 10.1186/s12977-017-0351-8
31. Ormsby CE, Sengupta D, Tandon R, Deeks SG, Martin JN, Jones RB, et al. Human endogenous retrovirus expression is inversely associated with chronic immune activation in HIV-1 infection. PLoS ONE. (2012) 7:e41021. doi: 10.1371/journal.pone.0041021
32. Gonzalez-Hernandez MJ, Swanson MD, Contreras-Galindo R, Cookinham S, King SR, Noel RJ, et al. Expression of Human endogenous retrovirus type K (HML-2) is activated by the tat protein of HIV-1. J Virol. (2012) 86:7790–805. doi: 10.1128/JVI.07215-11
33. Bhardwaj N, Maldarelli F, Mellors J, Coffin JM. HIV-1 infection leads to increased transcription of human endogenous retrovirus HERV-K (HML-2) proviruses in vivo but not to increased virion production. J Virol. (2014) 88:11108–20. doi: 10.1128/JVI.01623-14
34. Michaud H-A, de Mulder M, SenGupta D, Deeks SG, Martin JN, Pilcher CD, et al. Trans-activation, post-transcriptional maturation, and induction of antibodies to HERV-K (HML-2) envelope transmembrane protein in HIV-1 infection. Retrovirology. (2014) 11:10. doi: 10.1186/1742-4690-11-10
35. Michaud H-A, SenGupta D, de Mulder M, Deeks SG, Martin JN, Kobie JJ, et al. Cutting edge: an antibody recognizing ancestral endogenous virus glycoproteins mediates antibody-dependent cellular cytotoxicity on HIV-1–infected cells. J Immunol. (2014) 193:1544–8. doi: 10.4049/jimmunol.1302108
36. Vincendeau M, Göttesdorfer I, Schreml JMH, Wetie AGN, Mayer J, Greenwood AD, et al. Modulation of human endogenous retrovirus (HERV) transcription during persistent and de novo HIV-1 infection. Retrovirology. (2015) 12:27. doi: 10.1186/s12977-015-0156-6
37. Bowen LN, Tyagi R, Li W, Alfahad T, Smith B, Wright M, et al. HIV-associated motor neuron disease. Neurology. (2016) 87:1756–62. doi: 10.1212/WNL.0000000000003258
38. Terry SN, Manganaro L, Cuesta-Dominguez A, Brinzevich D, Simon V, Mulder LCF. Expression of HERV-K108 envelope interferes with HIV-1 production. Virology. (2017) 509:52–9. doi: 10.1016/j.virol.2017.06.004
39. de Mulder M, SenGupta D, Deeks SG, Martin JN, Pilcher CD, Hecht FM, et al. Anti-HERV-K (HML-2) capsid antibody responses in HIV elite controllers. Retrovirology. (2017) 14:41. doi: 10.1186/s12977-017-0365-2
40. Douville RN, Nath A. Human endogenous retrovirus-K and TDP-43 expression bridges ALS and HIV neuropathology. Front Microbiol. (2017) 8:1–8. doi: 10.3389/fmicb.2017.01986
41. Gray LR, Jackson RE, Jackson PEH, Bekiranov S, Rekosh D, Hammarskjöld M-L. HIV-1 Rev interacts with HERV-K RcREs present in the human genome and promotes export of unspliced HERV-K proviral RNA. Retrovirology. (2019) 16:40. doi: 10.1186/s12977-019-0505-y
42. Bolger AM, Lohse M, Usadel B. Trimmomatic: a flexible trimmer for Illumina sequence data. Bioinformatics. (2014) 30:2114–20. doi: 10.1093/bioinformatics/btu170
43. Langmead B, Salzberg SL. Fast gapped-read alignment with Bowtie 2. Nat Methods. (2012) 9:357–9. doi: 10.1038/nmeth.1923
44. Bendall ML, de Mulder M, Iñiguez LP, Lecanda-Sánchez A, Pérez-Losada M, Ostrowski MA, et al. Telescope: characterization of the retrotranscriptome by accurate estimation of transposable element expression. PLoS Comput Biol. (2019) 15:e1006453. doi: 10.1371/journal.pcbi.1006453
45. Dobin A, Davis CA, Schlesinger F, Drenkow J, Zaleski C, Jha S, et al. STAR: ultrafast universal RNA-seq aligner. Bioinformatics. (2013) 29:15–21. doi: 10.1093/bioinformatics/bts635
46. Anders S, Pyl PT, Huber W. HTSeq–a Python framework to work with high-throughput sequencing data. Bioinformatics. (2015) 31:166–9. doi: 10.1093/bioinformatics/btu638
47. Love MI, Huber W, Anders S. Moderated estimation of fold change and dispersion for RNA-seq data with DESeq2. Genome Biol. (2014) 15:1–21. doi: 10.1186/s13059-014-0550-8
48. Lee EYHP, Muller WJ. Oncogenes and tumor suppressor genes. Cold Spring Harb Perspect Biol. (2010) 2:a003236. doi: 10.1101/cshperspect.a003236
49. Mohammadizadeh F, Hani M, Ranaee M, Bagheri M. Role of cyclin D1 in breast carcinoma. J Res Med Sci. (2013) 18:1021–5.
50. Hwang K-T, Kim BH, Oh S, Park SY, Jung J, Kim J, et al. Prognostic role of KRAS mRNA expression in breast cancer. J Breast Cancer. (2019) 22:548. doi: 10.4048/jbc.2019.22.e55
51. Wang J, Vasaikar S, Shi Z, Greer M, Zhang B. WebGestalt 2017: a more comprehensive, powerful, flexible and interactive gene set enrichment analysis toolkit. Nucleic Acids Res. (2017) 45:W130–7. doi: 10.1093/nar/gkx356
52. Bray F, Ferlay J, Soerjomataram I, Siegel RL, Torre LA, Jemal A. Global cancer statistics 2018: GLOBOCAN estimates of incidence and mortality worldwide for 36 cancers in 185 countries. CA Cancer J Clin. (2018) 68:394–424. doi: 10.3322/caac.21492
53. Coghill AE, Engels EA, Schymura MJ, Mahale P, Shiels MS. Risk of breast, prostate, and colorectal cancer diagnoses among HIV-infected individuals in the United States. J Natl Cancer Inst. (2018) 110:959–66. doi: 10.1093/jnci/djy010
54. Masuda N, Ohnishi T, Kawamoto S, Monden M, Okubo K. Analysis of chemical modification of RNA from formalin-fixed samples and optimization of molecular biology applications for such samples. Nucleic Acids Res. (1999) 27:4436–43. doi: 10.1093/nar/27.22.4436
55. von Ahlfen S, Missel A, Bendrat K, Schlumpberger M. Determinants of RNA quality from FFPE samples. PLoS ONE. (2007) 2:e1261. doi: 10.1371/journal.pone.0001261
56. Bossel Ben-Moshe N, Gilad S, Perry G, Benjamin S, Balint-Lahat N, Pavlovsky A, et al. mRNA-seq whole transcriptome profiling of fresh frozen versus archived fixed tissues. BMC Genomics. (2018) 19:1–11. doi: 10.1186/s12864-018-4761-3
57. Maguire A, Chen X, Wisner L, Malasi S, Ramsower C, Kendrick S, et al. Enhanced DNA repair and genomic stability identify a novel HIV-related diffuse large B-cell lymphoma signature. Int J Cancer. (2019) 145:3078–88. doi: 10.1002/ijc.32381
58. Guo H, Gao J, Taxman DJ, Ting JPY, Su L. HIV-1 Infection induces interleukin-1β production via TLR8 protein-dependent and NLRP3 inflammasome mechanisms in human monocytes. J Biol Chem. (2014) 289:21716–26. doi: 10.1074/jbc.M114.566620
59. Armbruester V, Sauter M, Roemer K, Best B, Hahn S, Nty A, et al. Np9 protein of human endogenous retrovirus K interacts with ligand of numb protein X. J Virol. (2004) 78:10310–9. doi: 10.1128/JVI.78.19.10310-10319.2004
60. Chen T, Meng Z, Gan Y, Wang X, Xu F, Gu Y, et al. The viral oncogene Np9 acts as a critical molecular switch for co-activating β-catenin, ERK, Akt and Notch1 and promoting the growth of human leukemia stem/progenitor cells. Leukemia. (2013) 27:1469–78. doi: 10.1038/leu.2013.8
61. Johanning GL, Malouf GG, Zheng X, Esteva FJ, Weinstein JN, Wang-Johanning F, et al. Expression of human endogenous retrovirus-K is strongly associated with the basal-like breast cancer phenotype. Sci Rep. (2017) 7:41960. doi: 10.1038/srep41960
62. Tavakolian S, Goudarzi H, Faghihloo E. Evaluating the expression level of HERV-K env, np9, rec and gag in breast tissue. Infect Agent Cancer. (2019) 14:1–5. doi: 10.1186/s13027-019-0260-7
63. Zhang M, Liang JQ, Zheng S. Expressional activation and functional roles of human endogenous retroviruses in cancers. Rev Med Virol. (2019) 29:e2025. doi: 10.1002/rmv.2025
64. van der Kuyl AC. HIV infection and HERV expression: a review. Retrovirology. (2012) 9:6. doi: 10.1186/1742-4690-9-6
65. Goila-Gaur R, Strebel K. HIV-1 Vif, APOBEC, and intrinsic immunity. Retrovirology. (2008) 5:51. doi: 10.1186/1742-4690-5-51
66. Koito A, Ikeda T. Intrinsic immunity against retrotransposons by APOBEC cytidine deaminases. Front Microbiol. (2013) 4:1–9. doi: 10.3389/fmicb.2013.00028
67. Iñiguez LP, de Mulder Rougvie M, Stearrett N, Jones RB, Ormsby CE, Reyes-Terán G, et al. Transcriptomic analysis of human endogenous retroviruses in systemic lupus erythematosus. Proc Natl Acad Sci. (2019) 116:21350–1. doi: 10.1073/pnas.1907705116
68. Chang TC, Goud S, Torcivia-Rodriguez J, Hu Y, Pan Q, Kahsay R, et al. Investigation of somatic single nucleotide variations in human endogenous retrovirus elements and their potential association with cancer. PLoS ONE. (2019) 14:1–23. doi: 10.1371/journal.pone.0213770
69. Curty G, Marston JL, de Mulder Rougvie M, Leal FE, Nixon DF, Soares MA. Human endogenous retrovirus K in cancer: a potential biomarker and immunotherapeutic target. Viruses. (2020) 12:726. doi: 10.3390/v12070726
70. Salavatiha Z, Soleimani-Jelodar R, Jalilvand S. The role of endogenous retroviruses-K in human cancer. Rev Med Virol. (2020) e2142. doi: 10.1002/rmv.2142
71. Heikkinen K. RAD50 and NBS1 are breast cancer susceptibility genes associated with genomic instability. Carcinogenesis. (2005) 27:1593–9. doi: 10.1093/carcin/bgi360
72. Fan C, Zhang J, Ouyang T, Li J, Wang T, Fan Z, et al. RAD50 germline mutations are associated with poor survival in BRCA1/2 -negative breast cancer patients. Int J Cancer. (2018) 143:1935–42. doi: 10.1002/ijc.31579
73. Lo P-K, Zhang Y, Wolfson B, Gernapudi R, Yao Y, Duru N, et al. Dysregulation of the BRCA1/long non-coding RNA NEAT1 signaling axis contributes to breast tumorigenesis. Oncotarget. (2016) 7:65067–89. doi: 10.18632/oncotarget.11364
74. Slack FJ, Chinnaiyan AM. The role of non-coding RNAs in oncology. Cell. (2019) 179:1033–55. doi: 10.1016/j.cell.2019.10.017
75. Balestrieri E, Argaw-Denboba A, Gambacurta A, Cipriani C, Bei R, Serafino A, et al. Human endogenous retrovirus K in the crosstalk between cancer cells microenvironment and plasticity: a new perspective for combination therapy. Front Microbiol. (2018) 9:1448. doi: 10.3389/fmicb.2018.01448
76. Chiu W-T, Lee H-T, Huang F-J, Aldape KD, Yao J, Steeg PS, et al. Caveolin-1 upregulation mediates suppression of primary breast tumor growth and brain metastases by stat3 inhibition. Cancer Res. (2011) 71:4932–43. doi: 10.1158/0008-5472.CAN-10-4249
77. Bai L, Deng X, Li Q, Wang M, An W, Gao Z, et al. Down-regulation of the cavin family proteins in breast cancer. J Cell Biochem. (2012) 113:322–8. doi: 10.1002/jcb.23358
78. Saha SK, Islam SMR, Kwak K-S, Rahman MS, Cho S-G. PROM1 and PROM2 expression differentially modulates clinical prognosis of cancer: a multiomics analysis. Cancer Gene Ther. (2019) 27:147–67. doi: 10.1038/s41417-019-0109-7
79. Joseph C, Arshad M, Kurozomi S, Althobiti M, Miligy IM, Al-izzi S, et al. Overexpression of the cancer stem cell marker CD133 confers a poor prognosis in invasive breast cancer. Breast Cancer Res Treat. (2019) 174:387–99. doi: 10.1007/s10549-018-05085-9
80. Place AE, Jin Huh S, Polyak K. The microenvironment in breast cancer progression: biology and implications for treatment. Breast Cancer Res. (2011) 13:227. doi: 10.1186/bcr2912
81. Soysal SD, Tzankov A, Muenst SE. Role of the tumor microenvironment in breast cancer. Pathobiology. (2015) 82:142–52. doi: 10.1159/000430499
82. Lim B, Woodward WA, Wang X, Reuben JM, Ueno NT. Inflammatory breast cancer biology: the tumour microenvironment is key. Nat Rev Cancer. (2018) 18:485–99. doi: 10.1038/s41568-018-0010-y
83. DeFrances MC, Debelius DR, Cheng J, Kane LP. Inhibition of T-cell activation by PIK3IP1. Eur J Immunol. (2012) 42:2754–9. doi: 10.1002/eji.201141653
84. Chen Y, Wang J, Wang X, Li X, Song J, Fang J, et al. Pik3ip1 is a negative immune regulator that inhibits antitumor T cell Immunity. Clin Cancer Res. (2019) 25:6180–94. doi: 10.1158/1078-0432.CCR-18-4134
85. Mao Y, Qu Q, Chen X, Huang O, Wu J, Shen K. The prognostic value of tumor-infiltrating lymphocytes in breast cancer: a systematic review and meta-analysis. PLoS ONE. (2016) 11:e0152500. doi: 10.1371/journal.pone.0152500
Keywords: breast cancer, HIV, human endogenous retrovirus, retrotranscriptome, microenvironment, formalin-fixed paraffin-embedded, telescope software, breast cancer oncogenes
Citation: Curty G, Beckerle GA, Iñiguez LP, Furler RL, de Carvalho PS, Marston JL, Champiat S, Heymann JJ, Ormsby CE, Reyes-Terán G, Soares MA, Nixon DF, Bendall ML, Leal FE and de Mulder Rougvie M (2020) Human Endogenous Retrovirus Expression Is Upregulated in the Breast Cancer Microenvironment of HIV Infected Women: A Pilot Study. Front. Oncol. 10:553983. doi: 10.3389/fonc.2020.553983
Received: 29 May 2020; Accepted: 17 September 2020;
Published: 22 October 2020.
Edited by:
Zhenyu Jia, University of California, Riverside, United StatesReviewed by:
Jihoon Lee, The University of Texas Health Science Center at San Antonio, United StatesShao-Chun Wang, China Medical University, Taiwan
Jukun Song, Guizhou Provincial People's Hospital, China
Copyright © 2020 Curty, Beckerle, Iñiguez, Furler, de Carvalho, Marston, Champiat, Heymann, Ormsby, Reyes-Terán, Soares, Nixon, Bendall, Leal and de Mulder Rougvie. This is an open-access article distributed under the terms of the Creative Commons Attribution License (CC BY). The use, distribution or reproduction in other forums is permitted, provided the original author(s) and the copyright owner(s) are credited and that the original publication in this journal is cited, in accordance with accepted academic practice. No use, distribution or reproduction is permitted which does not comply with these terms.
*Correspondence: Douglas F. Nixon, ZG5peG9uQG1lZC5jb3JuZWxsLmVkdQ==; Gislaine Curty, Z2NmLnNjaWVuY2VAZ21haWwuY29t
†These authors share first authorship
‡These authors share last authorship