- 1Department of Urology, Second Affiliated Hospital of Soochow University, Suzhou, China
- 2Department of Radiology, Second Affiliated Hospital of Soochow University, Suzhou, China
Trophinin-associated protein (TROAP) has been shown to be overexpressed and promotes tumor progression in some tumors. We performed this study to assess the biological and clinical significance of TROAP in prostate cancer. We downloaded TROAP mRNA expression data from TCGA and GEO databases. We analyzed expressions of TROAP and other genes in prostate cancer tumors at different stages and assessed Gleason scores. We used Celigo image, Transwell, and rescue assays, and flow cytometry detection to assess growth, apoptosis, proliferation, migration, and invasion of the prostate cancer cells. We identified and validated up- and down-stream genes in the TROAP pathway. The mRNA data suggested that TROAP expression was markedly upregulated in prostate cancer compared with its expression in normal tissues, especially in cancers with high stages and Gleason scores. Moreover, a high TROAP expression was associated with poor patient survival. Results of our in vitro assay showed that TROAP knockdown inhibited DU145 and PC3 cell proliferation and viability via cell apoptosis and S phase cycle arrest. The Transwell assay showed that TROAP knockdown inhibited cell migration and invasion, probably through MMP-9 and E-Cadherin modulation. Overexpression of TWIST partially abrogated the inhibitory effects of TROAP knockdown on prostate cancer cells. Our integrative mechanism dissection revealed that TROAP is in a pathway downstream of EZH2 and that it activates the TWIST/c-Myc pathway to regulate prostate cancer progression. In all, we identified TROAP as a driver of prostate cancer development and progression, providing a novel target for prostate cancer treatments.
Introduction
Prostate cancer is the most common malignant tumor in men and ranks as the leading cause of cancer-related mortality in most countries of the world (1, 2). Although multiple therapeutic strategies, including surgery, ADT, and chemotherapy, are initially highly effective, progression eventually occurs years later (3, 4). Clarifying the molecular mechanisms of prostate cancer progression is important to improve prostate cancer therapy.
TROAP, also called tastin, interacts with trophinin (5, 6). Previous spatial expression profile studies indicated that TROAP mRNA is highly expressed in some normal tissues and tumors (6–8). According to previous findings, a high TROAP expression predicts poor prognosis or tumor progression in ovarian and breast cancers and in hepatocellular carcinoma (8–10). TROAP has been shown to promote some tumor progressions, such as those of breast and colorectal cancers (9, 11). In prostate cancers, reduced TROAP expression can inhibit cancer cell proliferation and induce a cell cycle arrest (12). However, the mechanisms of TROAP in prostate cancer remain obscure. In this study, we first compared the expression levels of TROAP between prostate cancer tissues and paired normal tissues using data from TCGA and GEO databases. In addition, to explore the functions of TROAP in prostate cancer, we performed gene coexpression analyses with in vitro assays in prostate cancer cell lines.
Materials and Methods
Microarray Data Sources
We downloaded TROAP mRNA expression and clinical information of patients with prostate cancer from the TCGA database and the public GEO repository. We calculated P-values using the Student’s t-tests.
Cell Lines and Culture
The human prostate cancer cell lines DU145 and PC-3 were purchased from the Cell Bank of the Chinese Academy of Science (Shanghai, USA). All cells lines were incubated in DMEM (Hyclone Laboratories; GE Healthcare Life Sciences, Logan, UT, USA) with 10% fetal bovine serum (FBS) and cultured at 37 °C in a humidified atmosphere with 5% CO2. Antibiotic solutions of 100 U/ml penicillin G and 100 μg/ml streptomycin were added to the culture medium to prevent bacterial contamination.
RNA Extraction and qPCR
Total RNA was isolated from DU145 and PC-3 cells using the RNAiso Plus reagent (Takara Bio, Otsu, Japan). Next, 1-μg total RNA samples were isolated using a PrimeScript™ RT reagent kit (Takara Bio). qPCRs were performed using Premix Ex Taq™ II (Takara Bio) with a Roche Light Cycler 480 Real-Time PCR system and using β-actin as the internal control. The reverse reaction conditions were as follows: 95 °C for 1 min, 40 cycles of 95 °C for 5 s, 60 °C for 30 s, and 75 °C for 30 s. The primer sequences used were the following: TROAP (forward, 5′-GGTCAGGAGAAAAGGGGAGGAAG-3′; reverse, 5′-AGGCGTGCGTTTCTGAGAGC-3′); and β-actin (forward, 5′-GTGGACATCCGCAAAGAC-3′; reverse, 5′-AAAGGGTGTAACGCAACTA-3′). We used the 2-ΔΔCq method to calculate the relative TROAP expression levels.
Lentivirus Infection of Prostate Cancer Cells
We designed a TROAP-shRNA by connecting the shTROAP sequence to the pGreenPuro™ Cloning and Expression Lentivector (SBI System Biosciences, Palo Alto, CA, USA). The sequence of shTROAP was: 5′-AGAACCAAGATCCAAGGAGATCTCGAGATCTCCTTGGATCTTGGTTCT-3′. And, the control-shRNA was 5′-TTCTCCGAACGTGTCACGTCTCGAGACGTGACACGTTCGGAGAA-3′. Lentiviral particles were produced in 93T cells and harvested by ultra-centrifugation. For TROAP downregulation, we used the lentivirus containing shTROAP to infect DU145 and PC-3 cell lines.
Western Blot Analyses
We extracted whole cell proteins from prostate cancer cells after lysis in RIPA buffer (Beyotime, Haimen, China). Total protein samples were quantified using the Coomassie brilliant blue method. We loaded supernatant samples onto SDS-PAGE gels and separated the proteins before electro-transferring them onto PVDF membranes (Merck Millipore, Darmstadt, Germany). The membranes were further incubated with specific antibodies listed in the Supplementary Table 1. After tris buffer solution washes, we incubated specific protein bands with secondary antibody for 1 h and detected the signal using enhanced chemiluminescent assays.
Cell Growth Assays
We evaluated the effects of TROAP knockdown in prostate cancer cell growth using Celigo image cytometry. Lentivirus-infected DU145 and PC-3 cells were incubated at 37 °C in a 5% humidified atmosphere for 5 days. We used a Celigo Imaging Cytometer (Nexcelom Bioscience, Lawrence, MA, USA) to conduct cell count assays.
Cell Viability Assays
We used the 3-(4, 5-dimethyl-2-thiazolyl)-2, 5-diphenyl-2h-tetrazolium bromide (MTT) proliferation assay to assess cell viability. After 12-h cultures, lentivirus-infected DU145 and PC-3 cells were placed onto 96-well plates (2,000 cells/well) and incubated for 5 days. Next, MTT and an acidic isopropanol solution were added to every well for 1 h at 37°C. The absorbance at 595 nm was measured in a spectrophotometric plate reader (BioTek Instruments, Winooski, VT, USA).
Flow Cytometry Detection
We assessed the cell cycle and apoptosis rates of prostate cancer cells using flow cytometric analyses. All infected DU145 and PC-3 cells were inoculated with trypsinization. For the cell cycle analysis, after harvesting and washing them with phosphate-buffered saline (PBS) twice, we incubated the cells in the dark after adding the propidium iodide (PI) and then scanned them in a FACScan (BD Biosciences, Franklin Lakes, NJ, USA). We calculated and compared the percentages of prostate cancer cells in three cycle phases. For the cell apoptosis analyses, after washing the cells in PBS, we cultured apoptotic and necrotic cells for 30 min at 37°C after adding 5 μl of FITC-Annexin V and 10 μl of PI. We calculated stained cells and apoptosis rates using the FACScan (BD Biosciences).
Cell Invasion and Migration Assay
We used a Transwell assay to detect the invasion and migration abilities of DU145 and PC-3 cells 96 h after transfection. After washing the cells with the BPS, we seeded them into 24-well plates at a density of 1 × 105 cells/well. We added FBS as a chemoattractant to the lower chamber. After 24 h of incubation, we washed the invasive cells located on the bottom membrane surface using PBS, fixed them in 95% ethanol, and stained them with 0.1% crystal violet. We randomly selected three independent visual fields, and determined the number of stained cells under a light microscope (Lecia, Germany).
Statistical Analysis
We used Student t tests to assess associations between different variables with a continuous distribution and Pearson tests to analyze correlations between genes. We considered P-values < 0.05 as statistically significant. All statistical analyses were performed using the SPSS 24.0 software package and GraphPad Prism Software 6.0.
Results
TROAP Is Over-Expressed in Prostate Cancer Cells
To assess the expression levels of TROAP mRNA in prostate cancer and normal tissues, we downloaded RNA sequencing data from the TCGA and GEO databases. Base on the GEO datasets GSE32571, GSE70770, GSE60329 and GSE71016 (Figures 1A–D), the results showed that TROAP mRNA is overexpressed in prostate cancer tissues compared with which in normal tissues. The GSE6919 dataset showed similar results with TROAP mRNA being overexpressed in cancer tissues, but the overexpression was especially high in tissues with metastasis (Figure 1E). Our results also showed that cancers with high Gleason scores had high TROAP mRNA expression levels (GSE32571, Figure 1F; GSE70770, Figure 1G; GSE141551, Figure 1H). Results from the TCGA prostate cancer (PRAD) database showed similar results with TROAP mRNA being overexpressed in prostate cancer tissues compared with the expression in normal prostate tissues (Figure 1I). Data from PRAD also showed that expression of TROAP mRNA was high in prostate cancer with high Gleason scores (Figure 1J), high T stages (Figure 1K), high N stages (Figure 1L) and recurrent tumors (Figure 1M). We used information from the PRAD to perform disease free survival analyses and our results show that a high TROAP expression predicted a poor disease free survival rate (Figure 1N, we used the mean TROAP expression to set a cutoff value to divide patients into high and low TROAP groups). Our results suggest that TROAP is associated with tumorigenesis and development of prostate cancer.
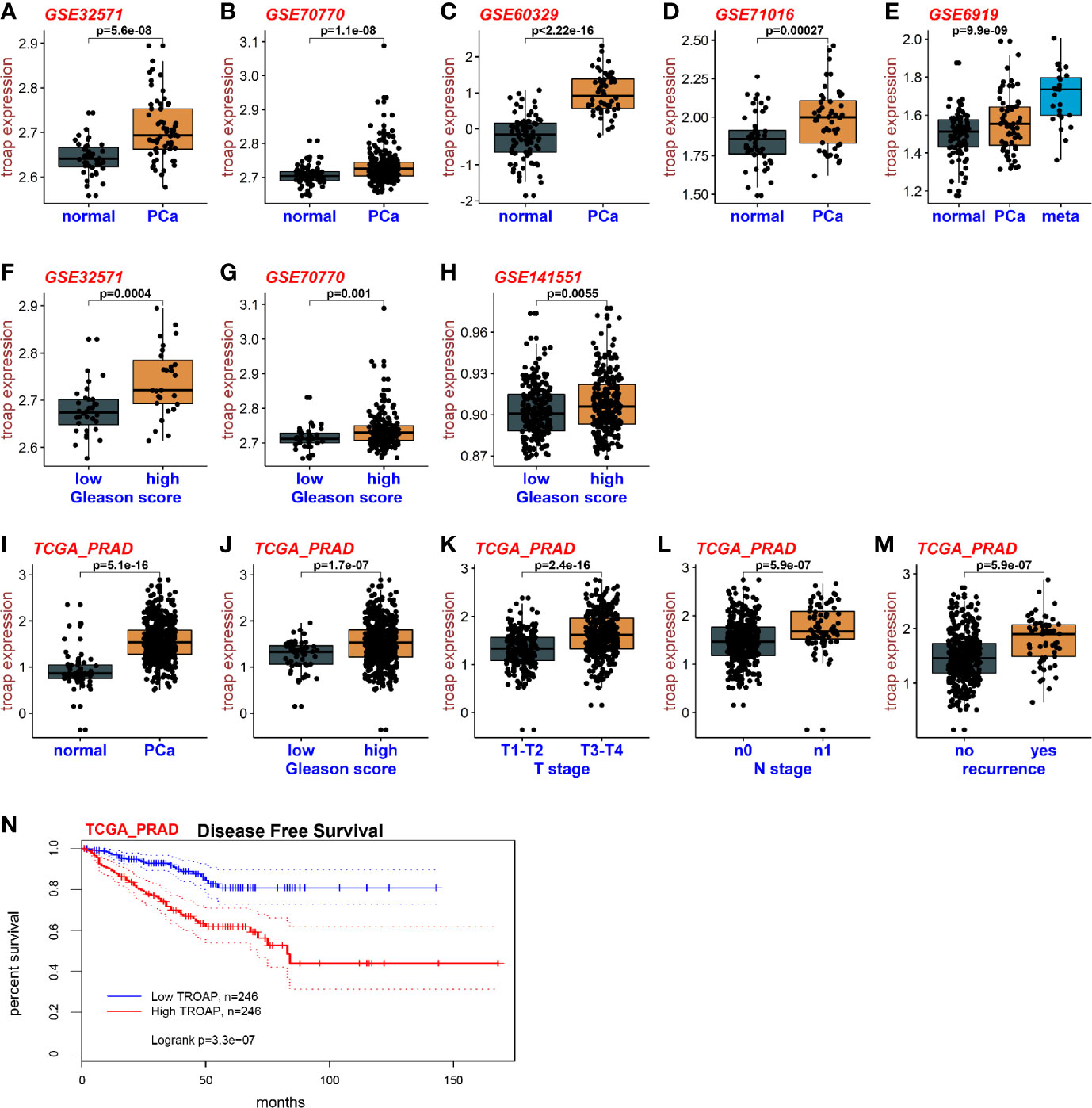
Figure 1 Expression of TROAP in prostate cancer. The expression of TROAP mRNA in prostate cancer tissues was higher than that in normal tissues in different GEO datasets (A–D). TROAP expression was high in prostate cancer with metastasis (E). TROAP expression was high in prostate cancer samples with high Gleason scores (F–H). Results from the PRAD show that TROAP was overexpressed in prostate cancer tissues (I). TROAP expressions were high in prostate cancer with high Gleason scores, T and N stages, and recurrent tumors (J–M). High TROAP expression predicts a poor disease-free survival (N).
TROAP Knockdown Inhibited Prostate Cancer Cell Proliferation and Viability
To investigate the effects of TROAP on DU145 and PC-3 cell proliferation and viability, we performed MTT assays and calculated cell numbers using a Celigo cell imaging analyzer. TROAP was knocked down by 55.71% (in DU145 cells) and 54.13% (in PC3 cells) in cells infected with shTROAP lentivirus, when compared to the expressions in the same cells infected with shCtrl lentivirus (Figures 2A, D, P < 0.01). In DU145 (Figures 2B, C) and PC3 (Figures 2E, F) cells, TROAP knockdown significantly inhibited cell proliferation on the 3rd, 4th and 5th days after infection. The MTT assay results also showed that TROAP knockdown inhibited cell viability by 35.43% on the 3rd day (P < 0.01), 33.82% on the 4th day (P < 0.01), and 45.09% on the 5th day (P < 0.001) in DU145 cells, and by 34.63% on the 3rd day (P < 0.01), 40.52% on the 4th day (P < 0.01), and 43.66% on the 5th (P < 0.001) in PC3 cells, compared with the viabilities of the shCtrl cells on at the same timepoints (Figures 2G, H).
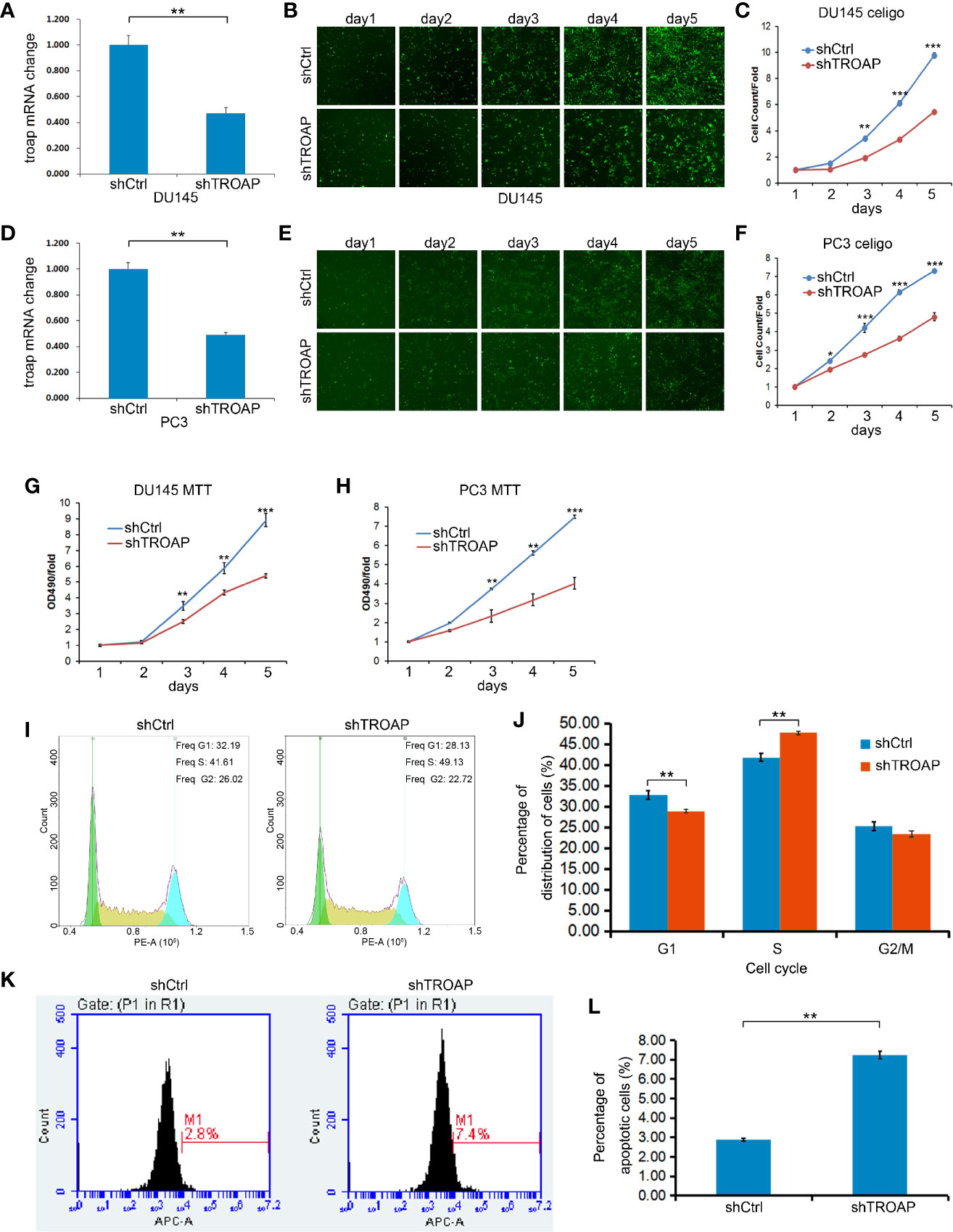
Figure 2 Infection with shTROAP lentivirus inhibited expression of TROAP in DU145 cells (A). TROAP knockdown suppressed DU145 cell proliferation (B, C). Similar results were observed in PC3 cells (D–F). The MTT assay results show that TROAP knockdown inhibited prostate cancer cell (DU145 and PC3) viability (G, H). TROAP knockdown induced S phase arrest of DU145 cells (I, J). TROAP knockdown induced DU145 cell apoptosis (K, L). **P < 0.01, ***P < 0.001.
TROAP Knockdown Induced Cell Cycle Arrest and Apoptosis
The role of TROAP in regulating the cell cycle and apoptosis was evaluated by flow cytometry. Based on our cell cycle analysis, we found that TROAP knockdown induced S phase arrest (Figures 2I, J, 47.72% in shTROAP vs 41.81% in shCtrl, P < 0.01). Further, the apoptosis analysis showed that TROAP knockdown promoted cell apoptosis (Figures 2K, L, 7.25% in shTROAP vs 2.88% in shCtrl, P < 0.01).
TROAP Knockdown Inhibited Migration and Invasion Abilities of Prostate Cancer Cells
Aberrant cell invasion and migration are frequently correlated with prostate cancer, and enhanced invasion and migration abilities are thought to be correlated with cancer metastases. Therefore, we assessed the biological role of TROAP in DU145 and PC-3 cells using Transwell assays. Our results showed that TROAP depletion significantly suppressed the migration of DU145 and PC-3 cells by 76.33% and 89.62%, respectively (Figures 3A, B, P < 0.01). Moreover, the Transwell invasion assay results showed that TROAP knockdown suppressed the invasion abilities of DU145 and PC-3 cells by 75.70% and 89.92%, respectively (Figures 3C, D, P < 0.01). Furthermore, we quantified the expression of EMT (epithelial-mesenchymal transition)-related proteins in PC-3 cells and found that TROAP knockdown inhibited the expression of MMP-9 and promoted the expression of E-Cadherin (Figure 3E).
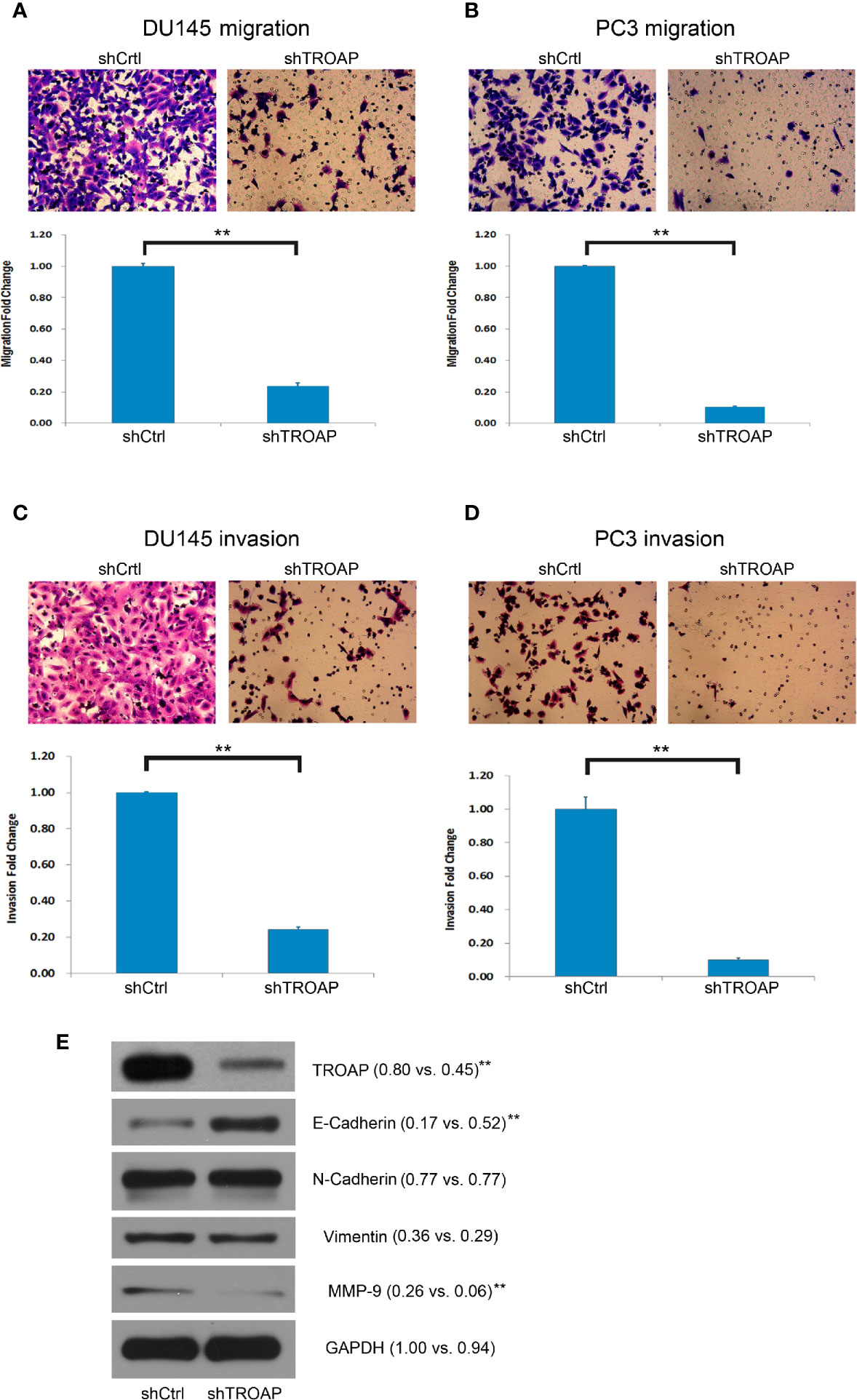
Figure 3 Cell invasion and migration assays in DU145 and PC-3 cells. Cell migration assay results indicate that TROAP depletion in DU145 and PC3 cells significantly suppressed the migration ability of the prostate cancer cells (A, B). The invasion assay results were similar with low migration of the cells (C, D). TROAP knockdown inhibited expression of MMP-9 and promoted expression of E-Cad (E). Data are presented as the mean ± standard deviation. **P < 0.01.
TROAP Silencing Inhibited the TWIST/c-Myc Pathway
To identify the potential downstream genes of TROAP we selected 20 genes associated with cancer cell cycles, EMT, or apoptosis and quantified their expression with qPCR in shTROAP and shCtrl prostate cancer cells. Our results showed that expression of TWIST was significantly down-regulated in the TROAP-silenced cells (Figure 4A, 36.84%, P < 0.001). A GSEA analysis of genes coexpressed with TROAP showed that c-Myc and E2F1 signals had a strong correlation with TROAP (Figure 4B). We inferred that TROAP might interact with c-Myc, E2F1, and TWIST in prostate cancer cells, but the association among the four genes was unclear.
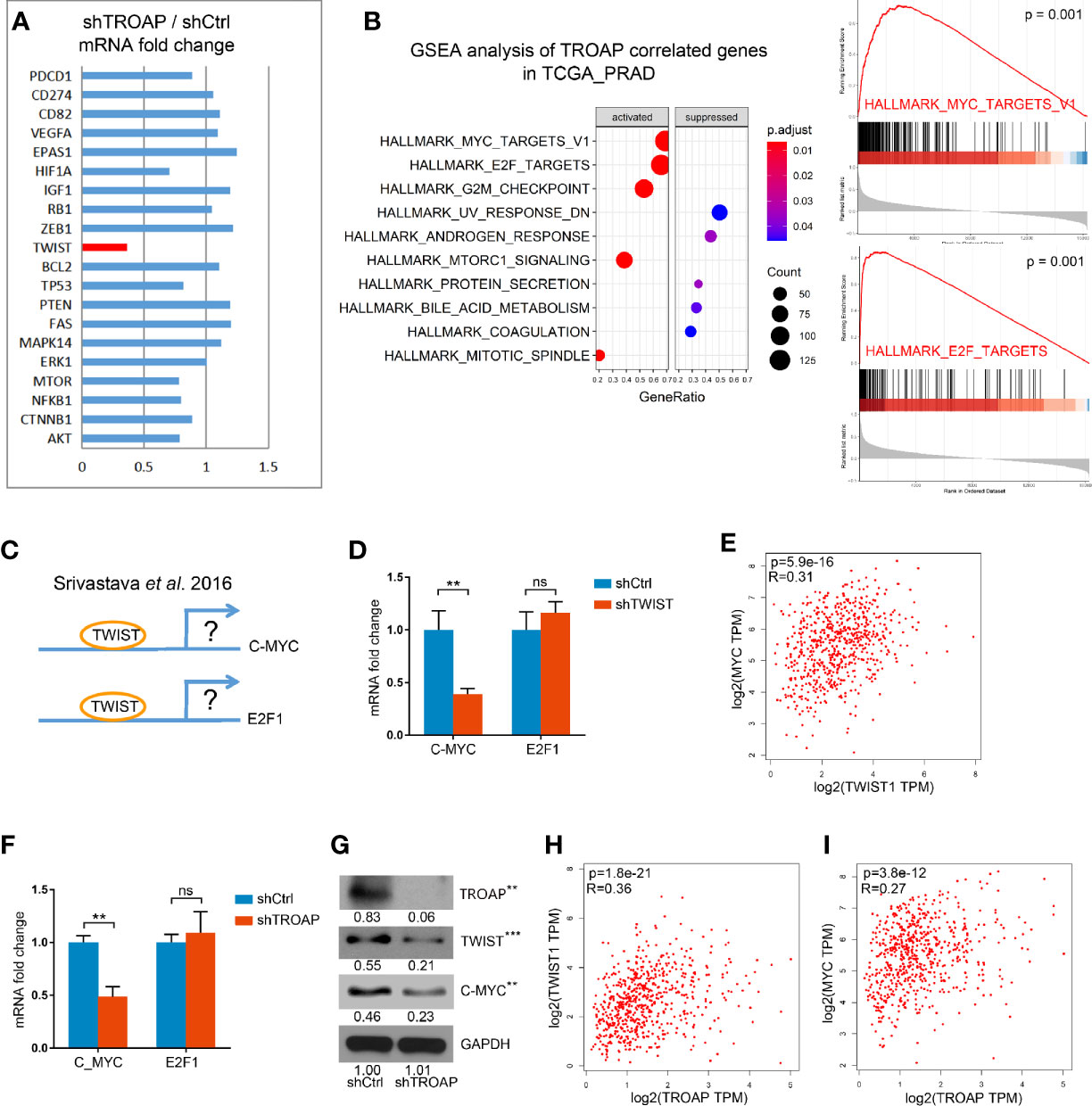
Figure 4 qPCR results show that TROAP knockdown inhibited TWIST expression (A). GSEA analysis of TROAP coexpressed genes showed that c-Myc and E2F1 signals were correlated with TROAP expression (B). TWIST could bound to the promoter regions of E2F1 and c-Myc to regulate E2F1 and c-Myc expression (C). TWIST knockdown could inhibit the expression of c-Myc and had no effect on expression of E2F1 (D). Analysis of the TCGA database showed a correlation between TWIST and c-Myc (E). TROAP knockdown suppressed TWIST and c-Myc expressions at the levels of mRNA and protein (F, G). TROAP expression was positively correlated with TWIST and c-Myc expressions (H, I). **P < 0.01, ***P < 0.001. ns means no significance.
A previous study showed that TWIST could bind to the promoter regions of E2F1 and c-Myc to regulate E2F1 and c-Myc expression (Figure 4C) (13). Thus, we quantified the expressions of E2F1 and c-Myc mRNAs in cells infected with shTWIST lentivirus (shTWIST lentivirus inhibited TWIST mRNA expression by 81.55%, not shown). These results indicate that knockdown of TWIST could inhibit the expression of c-Myc by 59.65% and that it had no effect on the expression of E2F1 (Figure 4D). Further verification in the TCGA database showed a strong correlation between TWIST and c-Myc (Figure 4E).
To explore the role of TROAP in the regulation of TWIST, E2F1, and c-Myc, we performed qPCRs and western blots. Our results showed that TROAP knockdown suppressed the expression of TWIST and c-Myc at the mRNA and protein levels (Figures 4F, G). Also, the coexpression analysis showed that TROAP expression was positively correlated with those of TWIST and c-Myc (Figures 4H, I). These results indicate that TROAP could regulate c-Myc expression via TWIST.
TWIST Overexpression Partially Abrogated the Inhibitory Effects of the TROAP Knockdown on Prostate Cancer Cells
Following the determination that TWIST is a downstream target of TROAP, we performed additional experiments to explore whether TWIST mediates the inhibitory effect of TROAP knockdown. We constructed a TWIST overexpression lentivirus (oeTWIST) and coinfected prostate cancer cells with oeTWIST and shTWIST lentiviruses, or with negative control; next, we performed cell proliferation, viability, and invasion assays. According to the cell numbers calculated with Celigo cell imaging analyzer, TWIST overexpression partially abrogated the inhibition of the cell proliferation induced by TROAP silencing (Figures 5A, B). Similarly, TWIST overexpression partially reversed the inhibitory effect on cell viability (Figure 5C, MTT) and cell invasion ability (Figures 5D, E, Transwell invasion assay). All these results demonstrate that TWIST upregulation partially abrogated the inhibition of the effect mediated by TROAP knockdown on prostate cancer cells, which further suggests that TWIST might be a major downstream target regulated by TROAP.
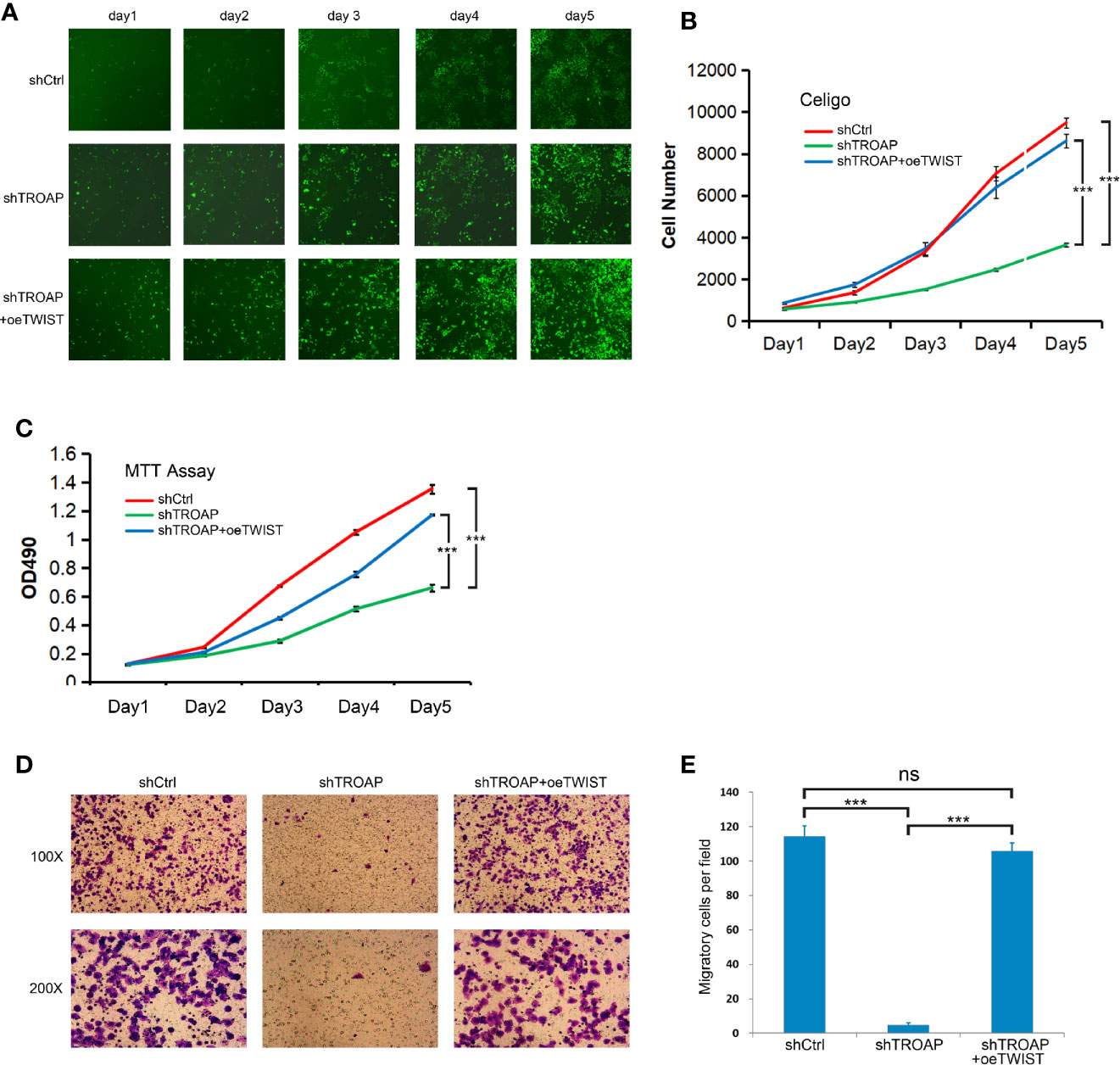
Figure 5 TWIST upregulation partially abrogated the inhibition mediated by TROAP knockdown on prostate cancer cells (A, B). Overexpression partially abrogated the inhibition of the cell proliferation induced by TROAP silencing. Overexpression of TWIST reversed the inhibitory effect on cell viability (C) and cell invasion (D, E). ***P < 0.001; ns, no significant.
TROAP and E2F1 Are Co-Regulated by EZH2
The interaction between E2F1 and TROAP was unclear. Our coexpression analysis showed a strong correlation between E2F1 and TROAP (Figure 6A). However, qPCR (Figure 6B) and microarray (Figures 6C, D) results showed that E2F1 knockdown had no effect on the expression of TROAP. Thus, E2F1 and TROAP cannot regulate each other directly (based on Figures 4F, 6B–D). In our previous study (14), we had noticed that E2F1 and TROAP were coexpressed with EZH2; therefore, we further explored the expressions of EZH2 and those two genes. Similarly to our previous results, we found a strong correlation between the expression of EZH2 and those of either TROAP or E2F1 (Figures 6E, F). To determine which of the three genes plays the key role in prostate cancer, we performed a principal component analysis (PCA) using the TCGA_PRAD and the results showed that EZH2 plays a more important driving role in prostate cancer (Figure 6G). Our qPCR (Figure 6H) and microarray (Figures 6I, J) results confirmed that EZH2 knockdown inhibited the expressions of E2F1 and TROAP. And knockdown of E2F1 could inhibit expression of EZH2 only in PC3 cells (Supplementary Figure 1). Thus, TROAP and E2F1 seem to be coregulated by EZH2 (Figure 6K).
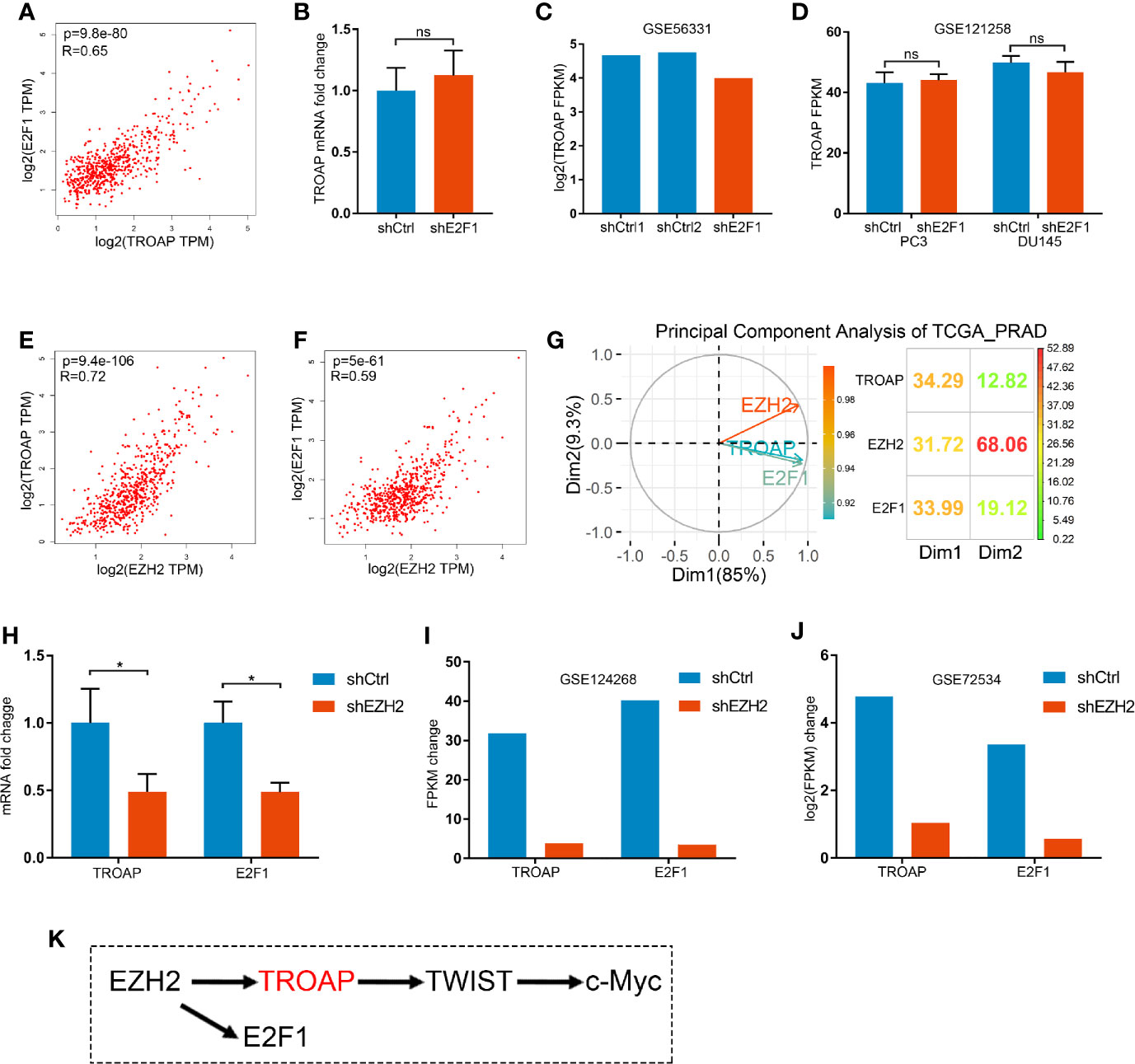
Figure 6 Correlation between the expressions of E2F1 and TROAP (A). Knockdown of E2F1 produced no effect on the expression of TROAP (B–D). Expression of EZH2 was correlated with TROAP and E2F1 expressions (E, F). Results of the principal component analysis (PCA) show that EZH2 was a driver in prostate cancer (G). qPCR (H) and microarray (I, J) results confirm that knockdown of EZH2 inhibited the expressions of E2F1 and TROAP. TROAP and E2F1 are regulated by EZH2, and TROAP induced the TWIST/c-Myc pathway to regulate the prostate cancer cell process (K). *P < 0.05; ns, no significant.
Discussion
TROAP is considered a critical cell adhesion molecule for the function of trophinin (14). TROAP plays important roles in cellular processes; it acts as a part of the adhesion complex and plays an essential role during early embryo implantation (5, 15). In addition, TROAP is thought to be essential for cell proliferation, because it was shown to be required for spindle assembly and centrosome integrity during mitosis (16). At the same time, TROAP was shown to be abnormally expressed in some tumor tissues and cell lines (17–19), including in prostate cancer (12), and it could regulate cancer cell biological processes. Nonetheless, little is known about the role of TROAP in prostate cancer development and progression.
In the present study, we analyzed TROAP expression in TCGA and GEO databases. The results showed that TROAP expression was obviously higher in prostate cancer than in normal prostate tissues, and that it was also higher in prostate cancers with higher Gleason scores, higher T or N stages, and in recurrent cancers than in other prostate cancers. We further investigated the potential biological functions of TROAP in vitro. Our results showed that TROAP knockdown inhibited cell proliferation and induced cell apoptosis by mediating cell cycle arrest on the S phase. We also observed that cell apoptosis rate rises not significantly (7.25% in shTROAP vs 2.88% in shCtrl). The inhibition of cell proliferation might be caused mainly by the cell cycle arrest on the S phase. The S phase of cell cycle allows cell DNA replication without accumulating genetic abnormalities. Some anti-tumor drugs, such as hinokitiol and xanthohumol, arrest cells in an S phase and inhibit cell growth (20, 21). In our study, TROAP showed a growth inhibitory effect by arresting cells in the S phase of the cycle.
TWIST is a transcription factor shown to mediate activation of EMT in multiple cancer cells (22, 23). Our results showed that TROAP knockdown could suppress prostate cancer cell migration and invasion by inhibiting TWIST and MMP-9 expressions. To further confirm the role of TROAP in the gene regulatory network in prostate cancer, we assessed gene coexpression by qPCR. Our results indicate that TROAP and E2F1 were co-regulated by EZH2 and that TROAP could regulate cell processes partially via the TWIST/c-Myc pathway. In addition, TWIST and c-Myc could both promote tumor development. However, the mechanisms by which TWIST interacts with c-Myc are differently described in different studies (13, 24). The c-Myc protein can bind and activate the TWIST1 promoter to promote the expression of TWIST1 (24). But, TWIST can also bind the promoter regions of c-Myc at the canonical E-box binding motif and directly regulate its transcription (13). During the development of different tumors, different pathways or transcription factors play different leading roles at different stages, and this may explain the changing regulatory roles between TWIST and c-Myc. In prostate cancer, TWIST knockdown could suppress the expression of c-Myc.
In this study, we used PCA to distinguish the main factor among EZH2, TROAP and E2F1 causing tumorigenesis and development of prostate cancer. PCA is a method in dimension reduction, with numerous applications in biology, chemistry, and many other disciplines (25, 26). It is an efficient method capable of identifying independent eigenmodes responsible for observed variations (26, 27). The results of our PCA showed that the three genes are important for prostate cancer, but that EZH2 probably plays a driving role.
In summary, we observed overexpression of TROAP in prostate cancer when compared with the expression in normal tissues. Our experiment results indicate that TROAP could promote prostate cancer development and progression, at least partially, via a TWIST/c-Myc pathway. Furthermore, our observations suggest that TROAP and E2F1 are co-regulated by EZH2. Taken together, these findings suggest novel prostate cancer treatment targets.
Data Availability Statement
The raw data supporting the conclusions of this article will be made available by the authors, without undue reservation.
Author Contributions
All authors contributed to data analysis, drafting, or revising the article. All authors contributed to the article and approved the submitted version.
Funding
This study was supported by grants for JZ from the National Natural Science Foundation of China (no. 81773221), the Natural Science Foundation of Jiangsu Province (no. BK20161222), the Suzhou Science and Technology Planed Projects (no. SS201857), and the Key Young Talents of Medicine in Jiangsu (no.QNRC2016875). Grants for LJ from CNNC Science Fund for Talented Young Scholars (2018-272-4).
Conflict of Interest
The authors declare that the research was conducted in the absence of any commercial or financial relationships that could be construed as a potential conflict of interest.
Supplementary Material
The Supplementary Material for this article can be found online at: https://www.frontiersin.org/articles/10.3389/fonc.2020.592239/full#supplementary-material
Supplementary Figure 1 | Knockdown of E2F1 inhibited expression of EZH1 in PC3 cells. In DU145 cells it is not obvious.
References
1. Posadas EM, Chi KN, de Wit R, de Jonge MJA, Attard G, Friedlander TW, et al. Pharmacokinetics, Safety, and Antitumor Effect of Apalutamide with Abiraterone Acetate plus Prednisone in Metastatic Castration-Resistant Prostate Cancer: Phase 1b Study. Clin Cancer Res (2020) 26:3517–24. doi: 10.1158/1078-0432.CCR-19-3402
2. Attard G, Parker C, Eeles RA, Schröder F, Tomlins SA, Tannock I, et al. Prostate cancer. Lancet (2016) 387:70–82. doi: 10.1016/S0140-6736(14)61947-4
3. Karantanos T, Evans CP, Tombal B, Thompson TC, Montironi R, Isaacs WB. Understanding the mechanisms of androgen deprivation resistance in prostate cancer at the molecular level. Eur Urol (2015) 67:470–9. doi: 10.1016/j.eururo.2014.09.049
4. Graham L, Schweizer MT. Targeting persistent androgen receptor signaling in castration-resistant prostate cancer. Med Oncol (2016) 33:44. doi: 10.1007/s12032-016-0759-3
5. Fukuda MN, Sugihara K. Trophinin in cell adhesion and signal transduction. Front Biosci (Elite Ed) (2012) 4:342–50. doi: 10.2741/e381
6. Fukuda MN, Sato T, Nakayama J, Klier G, Mikami M, Aoki D, et al. Trophinin and tastin, a novel cell adhesion molecule complex with potential involvement in embryo implantation. Genes Dev (1995) 9:1199–210. doi: 10.1101/gad.9.10.1199
7. Nadano D, Nakayama J, Matsuzawa S, Sato TA, Matsuda T, Fukuda MN. Human tastin, a proline-rich cytoplasmic protein, associates with the microtubular cytoskeleton. Biochem J (2002) 364:669–77. doi: 10.1042/bj20011836
8. Hu H, Xu L, Chen Y, Luo SJ, Wu YZ, Xu SH, et al. The Upregulation of Trophinin-Associated Protein (TROAP) Predicts a Poor Prognosis in Hepatocellular Carcinoma. J Cancer (2019) 10:957–67. doi: 10.7150/jca.26666
9. Li K, Zhang R, Wei M, Zhao L, Wang Y, Feng X, et al. TROAP Promotes Breast Cancer Proliferation and Metastasis. BioMed Res Int (2019) 2019:6140951. doi: 10.1155/2019/6140951
10. Godoy H, Mhawech-Fauceglia P, Beck A, Miliotto A, Miller A, Lele S, et al. Developmentally restricted differentiation antigens are targets for immunotherapy in epithelial ovarian carcinoma. Int J Gynecol Pathol (2013) 32:536–40. doi: 10.1097/PGP.0b013e318275a550
11. Harada O, Suga T, Suzuki T, Nakamoto K, Kobayashi M, Nomiyama T, et al. The role of trophinin, an adhesion molecule unique to human trophoblasts, in progression of colorectal cancer. Int J Cancer (2007) 121:1072–8. doi: 10.1002/ijc.22821
12. Ye J, Chu C, Chen M, Shi Z, Gan S, Qu F, et al. TROAP regulates prostate cancer progression via the WNT3/survivin signalling pathways. Oncol Rep (2019) 41:1169–79. doi: 10.3892/or.2018.6854
13. Srivastava J, Rho O, Youssef RM, DiGiovanni J. Twist1 regulates keratinocyte proliferation and skin tumor promotion. Mol Carcinog (2016) 55:941–52. doi: 10.1002/mc.22335
14. Zhu J, Jin L, Zhang A, Gao P, Dai G, Xu M, et al. Coexpression Analysis of the EZH2 Gene Using The Cancer Genome Atlas and Oncomine Databases Identifies Coexpressed Genes Involved in Biological Networks in Breast Cancer, Glioblastoma, and Prostate Cancer. Med Sci Monit (2020) 26:e922346. doi: 10.12659/MSM.922346
15. Armant DR. Life and death responses to trophinin-mediated adhesion during blastocyst implantation. Cell Cycle (2011) 10:574–5. doi: 10.4161/cc.10.4.14846
16. Yang S, Liu X, Yin Y, Fukuda MN, Zhou J. Tastin is required for bipolar spindle assembly and centrosome integrity during mitosis. FASEB J (2008) 22:1960–72. doi: 10.1096/fj.07-081463
17. Jiao Y, Li Y, Lu Z, Liu Y. High Trophinin-Associated Protein Expression Is an Independent Predictor of Poor Survival in Liver Cancer. Dig Dis Sci (2019) 64:137–43. doi: 10.1007/s10620-018-5315-x
18. Chen Z, Zhou Y, Luo R, Liu K, Chen Z. Trophinin-associated protein expression is an independent prognostic biomarker in lung adenocarcinoma. J Thorac Dis (2019) 11:2043–50. doi: 10.21037/jtd.2019.04.86
19. Lian Y, Fan W, Huang Y, Wang H, Wang J, Zhou L, et al. Downregulated Trophinin-Associated Protein Plays a Critical Role in Human Hepatocellular Carcinoma Through Upregulation of Tumor Cell Growth and Migration. Oncol Res (2018) 26:691–701. doi: 10.3727/096504017X15101398724809
20. Lee YS, Choi KM, Kim W, Jeon YS, Lee YM, Hong JT, et al. Hinokitiol inhibits cell growth through induction of S-phase arrest and apoptosis in human colon cancer cells and suppresses tumor growth in a mouse xenograft experiment. J Nat Prod (2013) 76:2195–202. doi: 10.1021/np4005135
21. Yong WK, Ho YF, Malek SN. Xanthohumol induces apoptosis and S phase cell cycle arrest in A549 non-small cell lung cancer cells. Pharmacogn Mag (2015) 11:S275–83. doi: 10.4103/0973-1296.166069
22. Castanon I, Baylies MK. A Twist in fate: evolutionary comparison of Twist structure and function. Gene (2002) 287:11–22. doi: 10.1016/S0378-1119(01)00893-9
23. Ansieau S, Bastid J, Doreau A, Morel AP, Bouchet BP, Thomas C, et al. Induction of EMT by twist proteins as a collateral effect of tumor-promoting inactivation of premature senescence. Cancer Cell (2008) 14:79–89. doi: 10.1016/j.ccr.2008.06.005
24. Selmi A, de Saint-Jean M, Jallas AC, Garin E, Hogarty MD, Bénard J, et al. TWIST1 is a direct transcriptional target of MYCN and MYC in neuroblastoma. Cancer Lett (2015) 357:412–8. doi: 10.1016/j.canlet.2014.11.056
25. Song Y, Westerhuis JA, Aben N, Michaut M, Wessels LFA, Smilde AK. Principal component analysis of binary genomics data. Brief Bioinform (2019) 20:317–29. doi: 10.1093/bib/bbx119
26. Jolliffe IT, Cadima J. Principal component analysis: a review and recent developments. Philos Trans A Math Phys Eng Sci (2016) 374:20150202. doi: 10.1098/rsta.2015.0202
Keywords: prostate cancer, EZH2, TWIST, c-Myc, trophinin associated protein
Citation: Jin L, Zhou Y, Chen G, Dai G, Fu K, Yang D and Zhu J (2021) EZH2-TROAP Pathway Promotes Prostate Cancer Progression Via TWIST Signals. Front. Oncol. 10:592239. doi: 10.3389/fonc.2020.592239
Received: 27 August 2020; Accepted: 31 December 2020;
Published: 22 February 2021.
Edited by:
César López-Camarillo, Universidad Autónoma de la Ciudad de México, MexicoReviewed by:
Gang Li, Houston Methodist Research Institute, United StatesUsha Nagarajan, Central University of Haryana (CUH), India
Copyright © 2021 Jin, Zhou, Chen, Dai, Fu, Yang and Zhu. This is an open-access article distributed under the terms of the Creative Commons Attribution License (CC BY). The use, distribution or reproduction in other forums is permitted, provided the original author(s) and the copyright owner(s) are credited and that the original publication in this journal is cited, in accordance with accepted academic practice. No use, distribution or reproduction is permitted which does not comply with these terms.
*Correspondence: Jin Zhu, amluenVyb2xAb3V0bG9vay5jb20=; Dongrong Yang, ZHJ5YW5nc3V6aG91QG91dGxvb2suY29t
†These authors have contributed equally to this work