- 1Department of Epidemiology, Fielding School of Public Health, University of California at Los Angeles (UCLA), Los Angeles, CA, United States
- 2Center for Human Nutrition, Department of Medicine, UCLA David Geffen School of Medicine, University of California at Los Angeles (UCLA), Los Angeles, CA, United States
- 3Department of Biostatistics, Fielding School of Public Health, University of California at Los Angeles (UCLA), Los Angeles, CA, United States
- 4Translational Sciences Section, School of Nursing, University of California at Los Angeles (UCLA), Los Angeles, CA, United States
- 5Jonsson Comprehensive Cancer Center, University of California at Los Angeles (UCLA), Los Angeles, CA, United States
Background: A decreased level of serum adiponectin is associated with obesity and an increased risk of breast cancer among postmenopausal women. Yet, the interplay between genetic variants associated with adiponectin phenotype, obesity, and breast cancer risk is unclear in African American (AA) women.
Methods: We examined 32 single-nucleotide polymorphisms (SNPs) previously identified in genome-wide association and replication studies of serum adiponectin levels using data from 7,991 AA postmenopausal women in the Women’s Health Initiative SNP Health Association Resource.
Results: Stratifying by obesity status, we identified 18 adiponectin-related SNPs that were associated with breast cancer risk. Among women with BMI ≥ 30 kg/m2, the minor TT genotype of FER rs10447248 had an elevated breast cancer risk. Interaction was observed between obesity and the CT genotype of ADIPOQ rs6773957 on the additive scale for breast cancer risk (relative excess risk due to interaction, 0.62; 95% CI, 0.32–0.92). The joint effect of BMI ≥ 30 kg/m2 and the TC genotype of OR8S1 rs11168618 was larger than the sum of the independent effects on breast cancer risk.
Conclusions: We demonstrated that obesity plays a significant role as an effect modifier in an increased effect of the SNPs on breast cancer risk using one of the most extensive data on postmenopausal AA women.
Impact: The results suggest the potential use of adiponectin genetic variants as obesity-associated biomarkers for informing AA women who are at greater risk for breast cancer and also for promoting behavioral interventions, such as weight control, to those with risk genotypes.
Introduction
Obesity, defined as body mass index (BMI) of 30.0 kg/m2 or greater, is a well-established risk factor for postmenopausal breast cancer risk (1, 2). It contributes about 10% of all postmenopausal breast cancer incidents in the United States (3). Obesity disproportionately affects African American (AA) women, where AA women have notably the highest prevalence of obesity and experience the continuing rise (4–6). This trend may reflect increased postmenopausal breast cancer incidence observed among AA women, whereas it has been stable for White women (2, 7–9). During 1999 through 2013, breast cancer incidence among women aged 50 to 59 years decreased slower among AA women (−0.1% per year) compared with White women (−1.7% per year). Furthermore, rates of breast cancer incidence among individuals aged 60 to 79 years increased for AA women, whereas the rates decreased for White women (8). The continuing trend of increased obesity in AA than White women explain the existing difference in breast cancer incidence and may results in widening the racial gap. Notwithstanding the strong epidemiologic evidence that differs considerably by race, biological mechanisms underlying the racial differences in the obesity and postmenopausal breast cancer is yet to be fully elucidated.
Adiponectin is a protein hormone that is secreted by adipose tissue playing a key role in regulating the metabolism of glucose and lipid, adipocyte inflammation, and cell proliferation (10, 11). Adiponectin levels are inversely associated with obesity (12, 13). In obesity, adiponectin resistance is increased with reduced expression of adiponectin receptors (ADIPOR1 and ADIPOR2) in breast cancer cells (14, 15). Consequently, hypoadiponectinemia may predispose to breast cancer development by inhibiting cell apoptosis and enhancing cell proliferation through blocking several downstream signaling pathways, including AMP-activated protein kinase (AMPK) and mitogen-activated protein kinase (MAPK) signaling pathways (15, 16). Observational studies showed that adiponectin levels were lower in women with postmenopausal breast cancer compared to healthy women (17–19). Moreover, lower concentrations of adiponectin were found in AA postmenopausal women compared with White postmenopausal women (20). As such, adiponectin is emerging as a crucial adipokine in breast cancer development in women with obesity, and potentially explains the difference in the breast cancer incidence between AA and White women.
Only a few studies have identified genetic variants (i.e., single nucleotide polymorphisms [SNPs]) that were associated with functional and structural regulation of adiponectin and their association with breast cancer risk; but the findings were inconsistent and conducted mostly in population with European or Asian ancestry (21–25). In particular, rs1501299 in the adiponectin gene (ADIPOQ) was associated with an increased risk of breast cancer in some studies (21, 23), but not in others (22). No consensus could be reached for ADIPOQ rs2241766, where a positive, a negative, and no associations with breast cancer risk have been reported across different studies (21–24). Of these studies, one study was conducted in AA women reporting that only ADIPOQ rs1501299 was associated with increased breast cancer incidence (23). A pressing need remains to consider SNPs in other genes that were found to be associated with adiponectin levels including CDH13 (26), FER (27), and ARL15 (28) as the existing studies solely examined SNPs in adiponectin and its receptor genes. Investigating SNPs in the ADIPOQ, ADIPOR1, ADIPOR2, and other genes, and further examining the role of obesity in the association between the adiponectin-related SNPs and breast cancer risk could further shed light on the gene-obesity interrelated molecular pathway of adiponectin in breast cancer development.
The purpose of this study was to examine the effects of candidate SNPs that were previously confirmed by genome-wide association and independent replication studies of serum adiponectin levels on breast cancer risk among AA postmenopausal women, who are vulnerable to both high incidence of obesity and breast cancer risk, using a large prospective cohort study from the Women’s Health Initiative (WHI) (23, 26, 27, 29–35). We hypothesized that the effects of candidate SNPs on breast cancer risk differs by obesity status, and therefore, investigated adiponectin-related SNPs that interact with obesity for their associations with breast cancer risk (Supplementary Figure 1).
Methods
Study Population
The study included postmenopausal women aged 50 to 79 years enrolled in the WHI Clinical Trial and Observational Study that was conducted from 1993 to 2005. The details of its study design and method are described elsewhere (36, 37). Briefly, the WHI was designed to identify risk factors for major causes of morbidity and mortality and to develop prevention strategies for chronic diseases among postmenopausal women. Women were eligible for the WHI study if they were aged 50 to 79 years at the study enrollment; postmenopausal; and likely to reside in the same area for at least 3 years. Genome-wide genotype data have been collected on a subset of participants after obtaining additional consent for genetic studies. We included postmenopausal women enrolled in the WHI SNP Health Association Resources (SHARe) providing the molecular and genetic data of AA and Hispanic women (38).
For the purpose of our study, subjects must meet the following inclusion criteria to be included in the study analysis: the subjects (i) were AA postmenopausal women aged 50 to 79 years; (ii) without a diagnosis of cancer at the time of study enrollment (except non-melanoma skin cancer); and (iii) reported at least one of four physical measurements (i.e., height, weight, waist, and hip). Assuming that those who ended participation early are more likely to have incomplete outcome information leading to potential follow-up bias, the study excluded those who had been followed up for less than 1 year. In addition, individuals who had developed invasive breast cancer within the 1-year follow-up period were excluded to avoid the potential effects of reverse causation between obesity and invasive breast cancer risk.
Of 50,256 participants, a total of 8,380 identified their race or ethnicity as AA. We excluded 372 subjects who reported a diagnosis of any type of cancer at the time of enrollment, and two subjects who were missing information on all four physical measurements. Further, we excluded 15 participants who had developed invasive breast cancer within the 1-year follow-up period. There was no withdrawal or cessation of participation within the 1-year follow-up period. After applying the eligibility criteria, a total of 7,991 subjects were included in the analysis. Of 7,991 participants, 402 (5.0%) of the eligible women, greater than the breast cancer incidence of AA postmenopausal women in the US (9), developed invasive breast cancer (Figure 1).
Breast Cancer Outcome
Self-reported invasive breast cancer cases were verified by adjudication of medical records in all participants of all phases of the WHI studies (39). As a result of the comprehensive outcome-assessment procedure, we did not have missing outcomes. Given that each type of breast cancer has distinct etiologies and prognoses for patients, the current study only included participants with primary invasive breast cancer. The participants were followed up from the date of enrollment to invasive breast cancer diagnosis, death, or end of follow-up.
Obesity Status: Body Mass Index, Waist-to-Hip Ratio, and Waist Circumference
We used three indices measuring body fat based on anthropometric measurements: BMI, waist-to-hip ratio (WHR), and waist circumference (WC). Each index was considered as a potential effect modifier to estimate its effect on the association between adiponectin-related SNPs and breast cancer among AA women. Also, these indices were each separately considered as a confounder of the relationship. Trained research personnel measured anthropometric measurements as continuous variables at the baseline (40). We used internationally recommended cutoff points for assessing adiposity-related risk (41, 42). BMI was categorized to define overall obesity with the following scale: underweight (< 18.5 kg/m2), normal (18.5–24.9 kg/m2), overweight (25–29.9 kg/m2), and obesity (≥ 30 kg/m2) (41). WHR used a cutoff of 0.85 (42), and WC used a cutoff of 88 cm in women to define abdominal obesity (41).
Adiponectin-Related SNPs
We conducted a candidate SNP approach, focusing on variants previously identified in GWA and replication studies of serum adiponectin levels (23, 26, 27, 29–35). Using the annotation file from the Affymetrix Genome-Wide Human SNP Array 6.0, we identified a total of 32 candidate SNPs (23, 26, 27, 29–35) and extracted them from the WHI SHARe dataset using the PLINK 1.9 software (Supplementary Table 1). Quality control was performed to exclude SNPs with a call rate less than 90%, a minor allele frequency less than 1%, and a Hardy-Weinberg equilibrium among AA women using a p-value cutoff of (38). We identified 3 SNPs in ADIPOQ (rs3774261, rs6444174, and rs6773957) that were in high linkage disequilibrium at a pairwise r2 threshold of 0.80. Of the 32 candidate SNPs, 8 SNPs were located within or nearby ADIPOQ or adiponectin receptor genes (23, 29). The other 24 SNPs, which may support the function of transcriptional control structures or indirectly regulate adiponectin expression, were found within non-adiponectin–specific or uncharacterized genes (27, 29, 32, 33, 35, 43).
Statistical Analysis
Baseline characteristics were compared across breast cancer status using a chi-square test for categorical variables and a t-test for continuous variables. We estimated hazard ratio (HR) and its 95% confidence intervals (CIs) for an effect of each SNP in predicting breast cancer development using a Cox proportional hazards regression model. Prior to fitting the model, the proportional hazard assumption was verified using the Schoenfeld residuals.
For each SNP, two sets of adjusted models were used, with the first adjusting for only age at baseline (Model 1) and the second adjusting for all covariates (Model 2). Covariates included in the analysis as potential confounding factors were measured at the baseline: age at baseline (year), family income (<$34,999, $35,000–$100,000, and ≥$100,000), employment status (yes vs. no), depressive symptom (depression scale ranging from 0 to 1 with a higher score indicating greater depressive severity), smoking status (ever smoke vs. no), age at menopause (year), number of pregnancies (never pregnant, 1 pregnancy, 2–4 pregnancies, and ≥5 pregnancies), exogenous estrogen use ever (yes vs. no), exogenous estrogen and progesterone use ever (yes vs. no), diabetic status (yes vs. no), dietary alcohol per day (gram), dietary total fat (gram), and physical activity (metabolic equivalent of task [MET] hours per week). We performed a complete case analysis excluding study participants with missing data in covariates. All 7,991 participants had data on age at baseline for fitting model 1, and a total of 6,121 participants (77%) were eligible for fitting model 2.
We compared crude and adjusted HRs to assess the effect of obesity as a confounding factor on the association between adiponectin-related SNPs and breast cancer risk. BMI, WHR, and WC are highly correlated, and thus, each index was entered individually in the regression models. A change greater than or equal to 10% indicates the presence of a confounding effect (44). For interaction analysis, two strategies were employed to assess the role of obesity on the relationship between adiponectin-related SNPs and breast cancer risk: (i) stratified analysis and (ii) analysis of the joint effects. The stratified analysis evaluates effect modification by comparing strata-specific HRs to one another and to the crude estimates. The analyses were performed separately for each index of obesity. Next, we calculated the relative excess risk due to interaction (RERI) to assess the joint effects of obesity and adiponectin-related SNPs on breast cancer risk on the additive scale with its 95% CIs obtained by the delta method (45). RERI equals 0 in the absence of additive interaction. Any departure from 0 indicates the presence of additive interaction. All statistical tests considered two-tailed p values less than 0.05 to be indicative of statistical significance. To account for the correction of multiple comparisons, we additionally applied the Benjamini and Hochberg procedure and controlled the false discovery rate at q-value of 0.05 in each adiponectin-related SNP (46). The R3.6.0 (dplyr, survival, epiR, and msm packages) was used.
Results
Baseline Characteristics
Of 7,991 subjects, 402 (5.0%) reported developing breast cancer (Table 1). The overall mean age at the baseline was 60.9 years (SD, 6.8 years) with a mean follow-up year of 14.5 years (SD, 3.15 years). The mean BMI was 31.0 kg/m2 (SD, 6.3 kg/m2), the mean WHR was 0.82 (SD, 0.07), and the mean WC was 91.3 cm (SD, 13.3 cm). Characteristics of participants were generally balanced between those with and without breast cancer.
The Association Between Adiponectin-Related SNPs and Breast Cancer Risk
Among 32 adiponectin-related SNPs (Supplementary Table 2), three candidate SNPs were observed to have potential association between genotype and breast cancer risk (Table 2). Without adjusting for obesity, the heterozygous TC genotype of OR8S1 rs11168618 (effect allele/reference allele: T/C) was correlated with a lower risk of breast cancer compared to the major CC genotype (HR, 0.65; 95% CI, 0.48–0.88) in model 1. The heterozygous TC genotype of EIF4A2 rs266719 (T/C) decreased breast cancer risk compared with the major CC genotype in model 2 (HR, 0.65; 95% CI, 0.44–0.95). The heterozygous CA genotype of KCNK9 rs2468677 (C/A) had increased breast cancer risk compared with the major AA genotype; however, it was found to be statistically significant only in model 2 with an additional adjustment for BMI (HR, 1.35; 95% CI, 1.00–1.80). After adjustments for multiple testing, those SNPs did not reach the significance level. Further, the assessment of confounding by BMI, WHR, and WC on the SNP-breast cancer relationship revealed that a confounding effect is unlikely to be a concern.
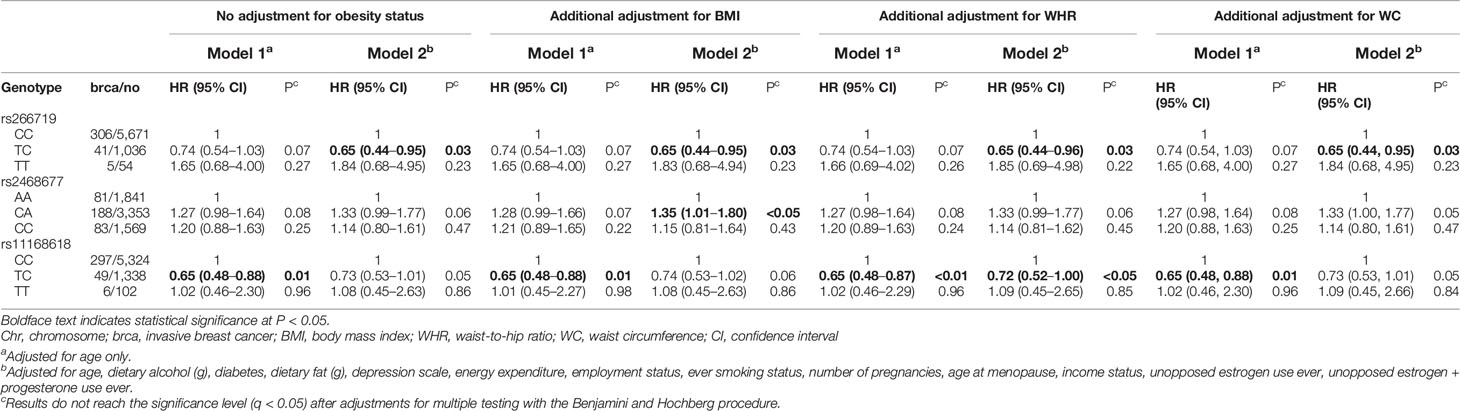
Table 2 Associations of adiponectin-related SNPs and postmenopausal invasive breast cancer risk with or without adjusting for BMI, WHR, and WC.
BMI, WHR, and WC as Effect Modifiers of the Association Between Adiponectin-Related SNPs and Breast Cancer Risk
Tables 3–5 present analysis stratified by BMI (under/normal weight, overweight, and obesity), WHR (<0.85 vs. ≥0.85), and WC (<88 cm vs. ≥88 cm), respectively. The effects of obesity status on the relationship between several SNPs and breast cancer differed between strata. In model 1, the heterozygous TC genotype in OR8S1 rs11168618 (T/C) was inversely associated with breast cancer risk among individuals with under/normal weight, overweight, WHR <0.85, and WC <88 cm. However, the significance was no longer observed in model 2. There was also a possible interaction of BMI ≥30 kg/m2 with the heterozygous TC genotype in OR8S1 rs11168618 (T/C) (RERI, 0.58; 95% CI, 0.35–0.80).
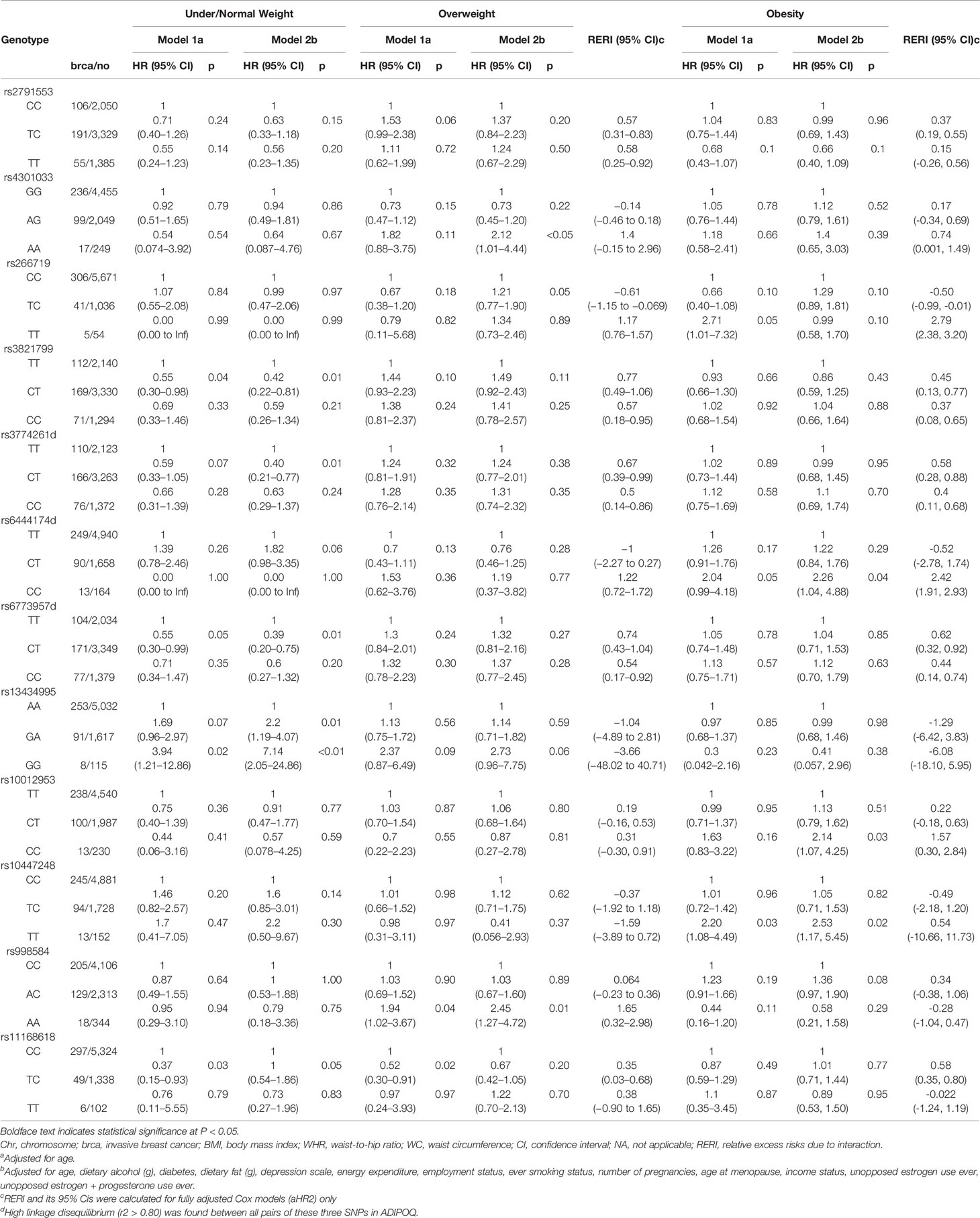
Table 3 Association between adiponectin-related SNPs and postmenopausal invasive breast cancer risk, by BMI status.
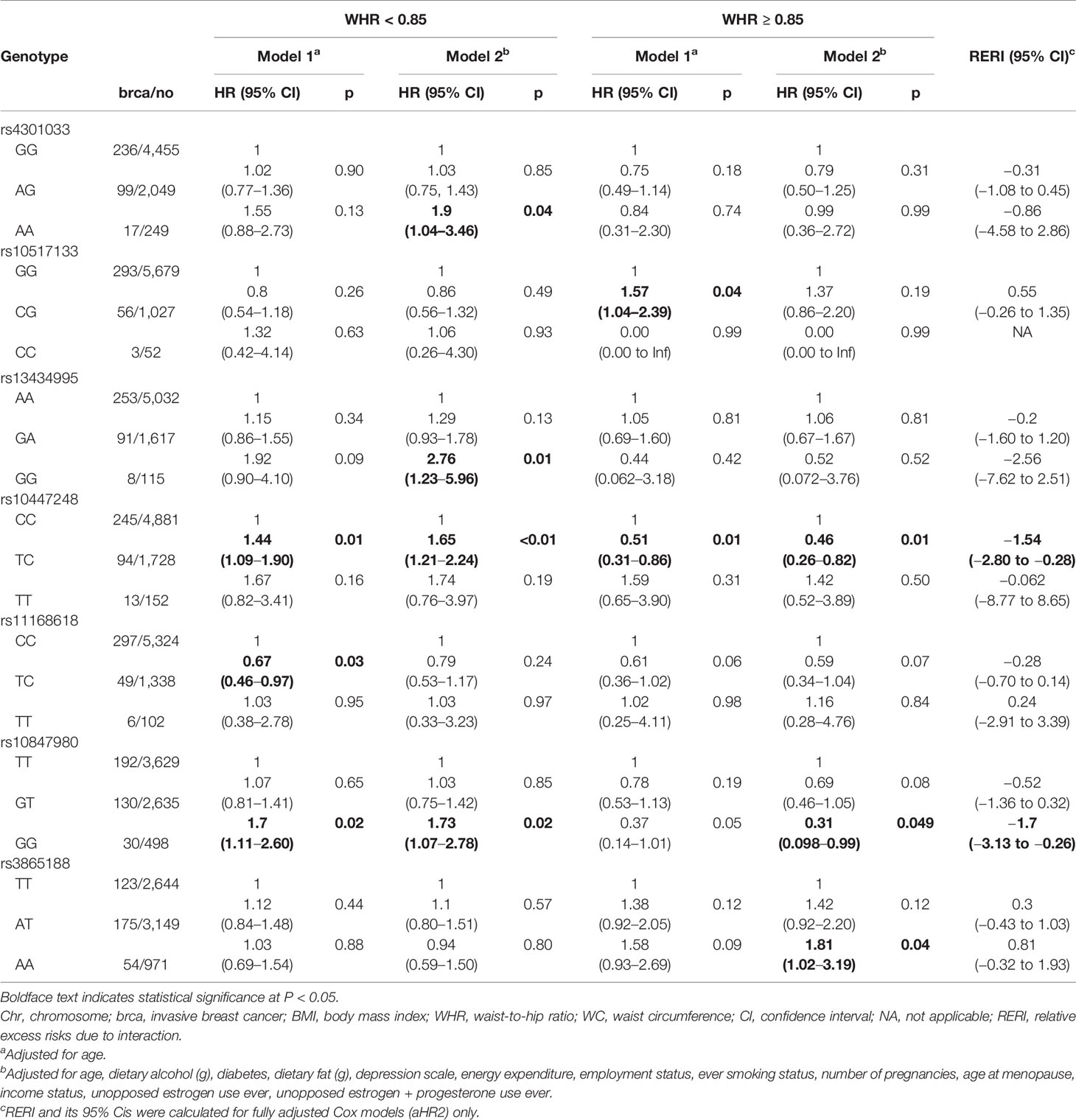
Table 4 Association between adiponectin-related SNPs and postmenopausal invasive breast cancer risk, by WHR status.
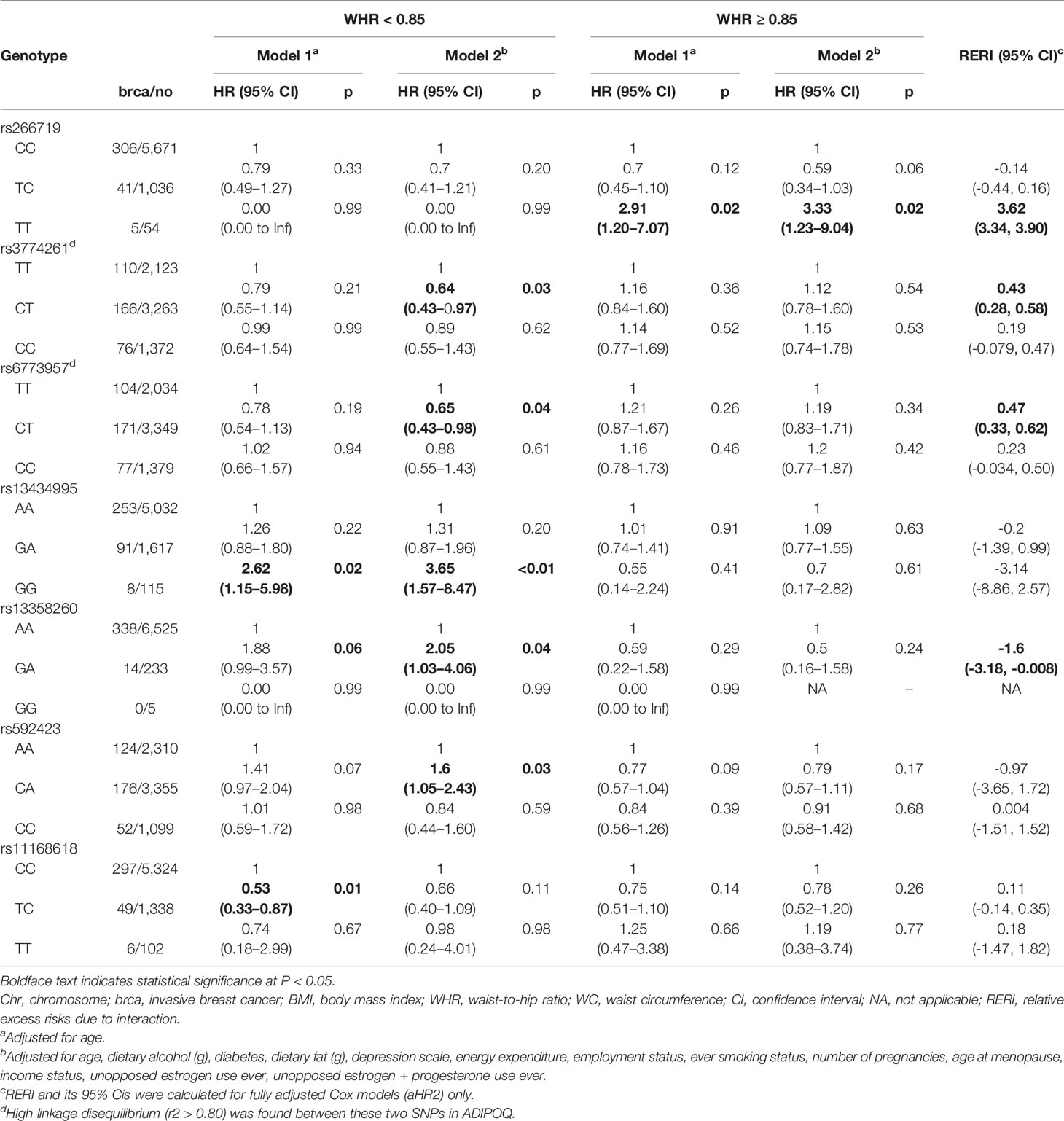
Table 5 Association between adiponectin-related SNPs and postmenopausal invasive breast cancer risk, by WC status.
In relation to the ADIPOQ gene, the heterozygous CT genotype in rs6773957 (C/T) was negatively associated with breast cancer risk among individuals with under/normal weight by roughly 60% in model 2. An interaction between BMI ≥30 kg/m2 and the heterozygous CT genotype was observed with a RERI of 0.62 with 95% CI of 0.32 to 0.92. Among those with WC <88 cm, the heterozygous CT genotype in rs6773957 (C/T) appeared to have a lower risk of breast cancer compared with the major CC genotype (HR, 0.65; 95% CI, 0.43–0.98). WC ≥88 cm showed an RERI of 0.47 with 95% CI of 0.33 to 0.62, suggesting super-additivity for the interaction between ADIPOQ rs6773957 (C/T) and WC. In addition, whereas effect alleles in ADIPOR1 rs2232853 (T/C) were associated with an increased risk of breast cancer among White women (21), its association with breast cancer risk was not found among AA women.
For FER rs10447248 (T/C), women with BMI ≥30 kg/m2 and the minor TT genotype had increased breast cancer risk in comparison to those with major CC genotype by approximately 2-fold in both model 1 (HR, 2.20; 95% CI, 1.08–4.49) and model 2 (HR, 2.53; 95% CI, 1.17–5.45). When we stratified the analysis by WHR status, different patterns were observed for the association between FER rs10447248 (T/C) and breast cancer risk. Carriers of the heterozygous TC genotype had an elevated risk of breast cancer among WHR <0.85 compared with those with the major CC genotype (HR, 1.44; 95% CI, 1.09–1.90), whereas the reduced risk among WHR >0.85 (HR, 0.51; 95% CI, 0.31–0.86) in model 1.
Discussion
Low circulating levels of adiponectin have been observed in obese individuals and women with postmenopausal breast cancer (12, 13, 17–19). Yet, the genetic mechanisms underlying the association between adiponectin and obesity in breast cancer risk have not been fully elucidated. Our study evaluated the association between genetic variants involved in regulating adiponectin circulating levels and breast cancer risk by obesity status among postmenopausal AA women. We found that heterozygotes of OR8S1 rs11168618 (T/C) and EIF4A2 rs266719 (T/C) were negatively associated with breast cancer risk, whereas the heterozygote of KCNK9 rs2468677 (C/A) had an elevated risk. The crude estimates of breast cancer risk did not differ from the adjusted estimates, thus confounding by obesity is unlikely. The findings suggest that low circulating levels of adiponectin may serve as a risk factor for breast cancer, independent of obesity. Indeed, in vivo and in vitro studies have demonstrated a direct effect of adiponectin on breast cancer development with and without obesity environment (47). Increased levels of adiponectin attenuated cell proliferation in several breast cancer cell lines, including MCF-7 (48, 49), T47D (48, 50–52), SKBR3 (48), MDA-MB-231 (50, 51), and MCF-10A (53). Furthermore, transgenic mice with adiponectin injection reduced mammary tumorigenesis (50), whereas mice with reduced adiponectin expression led to earlier tumor onset and accelerated tumor growth compared to those with normal expression (54).
The evidence on the association of SNPs in ADIPOQ and ADIPOR1 with breast cancer risk has been inconsistent (21–25). A previous study found that ADIPOQ rs17366568 influenced adiponectin plasma levels in non-Hispanic White women but not in AA women (55). In addition, women who carried effect alleles in ADIPOR1 rs2232853 (T/C) were associated with increased risk of breast cancer in a case-control study that consists of predominantly White women aged 20 to 87 years (21). However, we did not find a significant correlation between this SNP and breast cancer risk among AA women aged 50 to 79 years. These results in part explain the existing racial variations between AA and White women in breast cancer incidence and adiponectin levels (2, 7–9, 20). The findings also support that different adiponectin-related genetic factors may contribute to the increased risk of breast cancer by race. Understanding racial differences in adiponectin-related SNPs by accounting for their associations with adiponectin levels and breast cancer risk is an important area for future research.
We also observed that SNPs in non-adiponectin-specific genes were associated with breast cancer risk, and these associations were modified by obesity. Individuals with the minor TT genotype of FER rs10447248 (T/C) and having BMI ≥30 kg/m2 had an elevated risk of postmenopausal breast cancer. FER tyrosine kinase increases NF-κβ activation and signals interleukin-6 (IL-6) to regulate STAT3 phosphorylation (56, 57), which may explain its relationship with breast cancer risk through adiponectin and obesity. A decline in adiponectin secretion leads to overexpression of pro-inflammatory cytokines, including IL-6 and TNF-α, in an obese individual as a consequence of excess inflammatory response (58). The induction of TNF-α activates NF-κβ, which promotes breast cancer development (59, 60). IL-6 activates the Janus kinase-signal transducer and activator of transcription signaling pathway inducing the STAT3 dimer (58, 61, 62). This STAT3 dimer stimulates the transcription of genes strongly associated with the promotion of tumor growth and immunosuppression. This suggests that FER rs10447248 may predispose breast cancer by inducing NF-κβ and IL-6 to trigger downstream signaling pathways.
OR8S1 is an olfactory receptor (OR) that belongs to G protein-coupled receptors influencing tumorigenesis (63). An OR is most abundant in not only olfactory sensory neurons in an olfactory epithelium but is also found in tissues throughout the body. In the current study, the effect of OR8S1 rs11168618 (T/C), which decreases adiponectin levels (33), was inversely associated with breast cancer risk. It has been reported that an activated OR 544 (Olfr544) increased adiponectin secretion in 3T3-L1 mouse adipocytes (64). In relation to breast cancer, OR2B6 and OR2W3 were ectopically expressed in breast cancer cell lines and breast cancer tissues making them potential biomarkers (65, 66). An activation of ORs in cancer cells promotes apoptosis and inhibits cell proliferation by inducing AMPK or MAPK signaling pathways (65, 67). Given the limited existing evidence on the role of ORs with adiponectin or different types of cancer, we can only speculate that activation of ORs in adipose tissue has an indirect effect on lowering breast cancer risk through increasing adiponectin levels. To our knowledge, only the present study has evaluated OR8S1 rs11168618 and breast cancer risk.
It is important to note the potential limitations that the study has. Although breast cancer outcome and anthropometric measurements were prospectively measured with strict ascertainment procedure, other variables included in the regression models were mainly obtained from the self-reported questionnaire at the time of enrollment, leading to recall bias. However, there was no difference in distributions of baseline characteristics among cohorts. Missing data were also unavoidable in this study. In particular, information on the family history of breast cancer had a low response rate of 37.4% reducing statistical efficiency of the estimates. Thus, we decided not to include family history of breast cancer to obtain sufficient power while sustaining a potential confounding effect. Low circulating adiponectin levels may contribute to a more aggressive phenotype of breast cancer, ER-negative breast cancer risk compared to ER-positive breast cancer risk (68). Also, reduced breast cancer risk was observed in women with increased high-molecular weight adiponectin levels and lower BMI (14). Nevertheless, we could not further analyze the data by molecular subtypes of breast cancer or by adiponectin isomers due to the small sample sizes. Lastly, the study was limited to postmenopausal AA women harming the generalizability of our results. Despite these drawbacks, we used one of the most extensive data on postmenopausal AA women and conducted the genetic association study of adiponectin concerning postmenopausal breast cancer risk. In many cases, genetic data of a minority racial or ethnic group are not readily available nor have a sufficient sample size to obtain a comfortable statistical efficiency of the estimates. In addition to finding the associations between candidate SNPs in adiponectin genes and breast cancer risk, the study considered other loci in non–adiponectin-specific genes Associated with regulating adiponectin expression. In doing so, we were able to identify genetic variants of circulating adiponectin levels that were not directly considered in previous studies but may predispose to breast cancer development.
In summary, our study evaluated the association between previously identified adiponectin-related SNPs and primary invasive breast cancer risk among AA postmenopausal women. We detected that several adiponectin-related SNPs interacted with obesity, altering the risk of postmenopausal breast cancer. As obese women have an approximately 30% increased risk in developing breast cancer compared with those with normal weight (69), weight management is recommended as breast cancer prevention strategies (70). In light of the evidence, such an intervention would be particularly beneficial to AA postmenopausal women who carry the risk alleles of the adiponectin-related SNPs. Also, the identified SNPs could be used as clinical and genetic predictors of breast cancer in conjunction with obesity for AA postmenopausal women. Future studies are warranted to incorporate genetic variants of other cytokines from adipocytes (e.g., leptin) to unravel the complexity of the underlying mechanisms between obesity and breast cancer risk among AA women. Also, comparing the effects of adiponectin-related SNPs across different racial/ethnic groups can contribute to better understanding of the racial disparity in breast cancer risk. Nonetheless, our findings may assist in reducing the persistent racial gap in breast cancer incidence between AA and White women by examining the role of obesity and adiponectin in postmenopausal breast cancer etiology that may differ by these racial groups.
Data Availability Statement
The data that support the findings of this study are available in accordance with policies developed by the NHLBI and WHI in order to protect sensitive participant information and approved by the Fred Hutchinson Cancer Research Center, which currently serves as the IRB of record for the WHI. Data requests may be made by emailing aGVscGRlc2tAV0hJLm9yZw==.
Ethics Statement
The studies involving human participants were reviewed and approved by the institutional review boards of each participating clinical center of the WHI and the University of California, Los Angeles. The patients/participants provided their written informed consent to participate in this study.
Author Contributions
GN, Z-FZ, and SJ designed the study. GN and SJ performed the genomic data QC. GN performed the statistical analysis. Z-FZ, JR, HZ, and SJ participated in the study coordination and interpreted the data. SJ supervised the genomic data QC and data analysis and interpretation. SJ secured funding for this project. All authors contributed to the article and approved the submitted version.
Funding
This study was supported by the National Institute of Nursing Research of the National Institutes of Health under Award Number K01NR017852. GN is supported by the T32 Training Grant in Cancer Epidemiology (T32CA009142) at University of California, Los Angeles. Part of the data for this project was provided by the WHI program, which is funded by the National Heart, Lung, and Blood Institute, the National Institutes of Health, and the U.S. Department of Health and Human Services through contracts HHSN268201100046C, HHSN268201100001C, HHSN268201100002C, HHSN268201100003C, HHSN268201100004C, and HHSN271201100004C.
Conflict of Interest
The authors declare that the research was conducted in the absence of any commercial or financial relationships that could be construed as a potential conflict of interest.
Acknowledgments
The datasets used for the analyses described in this manuscript were obtained from dbGaP at http://www.ncbi.nlm.nih.gov/sites/entrez?db=gap through dbGaP accession (phs000200.v11.p3).
Program Office: National Heart, Lung, and Blood Institute, Bethesda, MD: Jacques Rossouw, Shari Ludlam, Dale Burwen, Joan McGowan, Leslie Ford, and Nancy Geller.
Clinical Coordinating Center: Fred Hutchinson Cancer Research Center, Seattle, WA: Garnet Anderson, Ross Prentice, Andrea LaCroix, and Charles Kooperberg.
Investigators and Academic Centers: Brigham and Women’s Hospital, Harvard Medical School, Boston, MA: JoAnn E. Manson; MedStar Health Research Institute/Howard University, Washington, DC: Barbara V. Howard; Stanford Prevention Research Center, Stanford, CA: Marcia L. Stefanick; The Ohio State University, Columbus, OH: Rebecca Jackson; University of Arizona, Tucson/Phoenix, AZ: Cynthia A. Thomson; University at Buffalo, Buffalo, NY: Jean Wactawski-Wende; University of Florida, Gainesville/Jacksonville, FL: Marian Limacher; University of Iowa, Iowa City/Davenport, IA: Robert Wallace; University of Pittsburgh, Pittsburgh, PA: Lewis Kuller; Wake Forest University School of Medicine, Winston-Salem, NC: Sally Shumaker.
Supplementary Material
The Supplementary Material for this article can be found online at: https://www.frontiersin.org/articles/10.3389/fonc.2021.698198/full#supplementary-material
References
1. World Health Organization. Global Cancer Observatory . Available at: https://gco.iarc.fr/.
2. Bandera EV, Maskarinec G, Romieu I, John EM. Racial and Ethnic Disparities in the Impact of Obesity on Breast Cancer Risk and Survival: A Global Perspective. Adv Nutr (2015) 6(6):803–19. doi: 10.3945/an.115.009647
3. Polednak AP. Estimating the Number of U.S. Incident Cancers Attributable to Obesity and the Impact on Temporal Trends in Incidence Rates for Obesity-Related Cancers. Cancer Detect Prev (2008) 32(3):190–9. doi: 10.1016/j.cdp.2008.08.004
4. Hales CM, Carroll MD, Fryar CD, Ogden CL. Prevalence of Obesity Among Adults: United States, 2017-2018. Hyattsville, MD: National Center for Health Statistics (2020).
5. Flegal KM, Kruszon-Moran D, Carroll MD, Fryar CD, Ogden CL. Trends in Obesity Among Adults in the United States, 2005 to 2014. JAMA (2016) 315(21):2284–91. doi: 10.1001/jama.2016.6458
6. Hales CM, Carroll MD, Fryar CD, Ogden CL. Prevalence of Obesity Among Adults and Youth: United States, 2015-2016. Hyattsville, MD: National Center for Health Statistics (2017).
7. Baquet CR, Mishra SI, Commiskey P, Ellison GL, Deshields M. Breast Cancer Epidemiology in Blacks and Whites: Disparities in Incidence, Mortality, Survival Rates and Histology. J Natl Med Assoc (2008) 100(5):480–89. doi: 10.1016/S0027-9684(15)31294-3
8. Richardson LC, Henley SJ, Miller JW, Massetti G, Thomas CC. Patterns and Trends in Age-Specific Black-White Differences in Breast Cancer Incidence and Mortality - United States, 1999-2014. MMWR Morb Mortal Wkly Rep (2016) 65(40):1093–98. doi: 10.15585/mmwr.mm6540a1
9. Howlander N, Noone AM, Krapcho M, Miller D, Brest A, Yu M, et al. SEER Cancer Statistics Review, 1975-2016. Bethesda, MD: National Cancer Institute (2019). Available at: https://seer.cancer.gov/csr/1975_2016/.
10. Nagaraju GP, Rajitha B, Aliya S, Kotipatruni RP, Madanraj AS, Hammond A, et al. The Role of Adiponectin in Obesity-Associated Female-Specific Carcinogenesis. Cytokine Growth Factor Rev (2016) 31:37–48. doi: 10.1016/j.cytogfr.2016.03.014
11. Parida S, Siddharth S, Sharma D. Adiponectin, Obesity, and Cancer: Clash of the Bigwigs in Health and Disease. Int J Mol Sci (2019) 20(10):2519. doi: 10.3390/ijms20102519
12. Silha JV, Krsek M, Skrha JV, Sucharda P, Nyomba BL, Murphy LJ. Plasma Resistin, Adiponectin and Leptin Levels in Lean and Obese Subjects: Correlations With Insulin Resistance. Eur J Endocrinol (2003) 149(4):331–5. doi: 10.1530/eje.0.1490331
13. Matsubara M, Maruoka S, Katayose S. Inverse Relationship Between Plasma Adiponectin and Leptin Concentrations in Normal-Weight and Obese Women. Eur J Endocrinol (2002) 147(2):173–80. doi: 10.1530/eje.0.1470173
14. Engin A. Obesity-Associated Breast Cancer: Analysis of Risk Factors. Adv Exp Med Biol (2017) 960:571–606. doi: 10.1007/978-3-319-48382-5_25
15. Zimta AA, Tigu AB, Muntean M, Cenariu D, Slaby O, Berindan-Neagoe I. Molecular Links Between Central Obesity and Breast Cancer. Int J Mol Sci (2019) 20(21):5364. doi: 10.3390/ijms20215364
16. Di Zazzo E, Polito R, Bartollino S, Nigro E, Porcile C, Bianco A, et al. Adiponectin as Link Factor Between Adipose Tissue and Cancer. Int J Mol Sci (2019) 20(4):839. doi: 10.3390/ijms20040839
17. Gu L, Cao C, Fu J, Li Q, Li DH, Chen MY. Serum Adiponectin in Breast Cancer: A Meta-Analysis. Medicine (Baltimore) (2018) 97(29):e11433. doi: 10.1097/MD.0000000000011433
18. Yu Z, Tang S, Ma H, Duan H, Zeng Y. Association of Serum Adiponectin With Breast Cancer: A Meta-Analysis of 27 Case-Control Studies. Medicine (Baltimore) (2019) 98(6):e14359. doi: 10.1097/MD.0000000000014359
19. Pena-Cano MI, Saucedo R, Morales-Avila E, Valencia J, Zavala-Moha JA, Lopez A. Deregulated Micrornas and Adiponectin in Postmenopausal Women With Breast Cancer. Gynecol Obstet Invest (2019) 84(4):369–77. doi: 10.1159/000496340
20. Cohen SS, Gammon MD, Signorello LB, North KE, Lange EM, Fowke JH, et al. Serum Adiponectin in Relation to Body Mass Index and Other Correlates in Black and White Women. Ann Epidemiol (2011) 21(2):86–94. doi: 10.1016/j.annepidem.2010.10.011
21. Kaklamani VG, Sadim M, Hsi A, Offit K, Oddoux C, Ostrer H, et al. Variants of the Adiponectin and Adiponectin Receptor 1 Genes and Breast Cancer Risk. Cancer Res (2008) 68(9):3178–84. doi: 10.1158/0008-5472.CAN-08-0533
22. Nyante SJ, Gammon MD, Kaufman JS, Bensen JT, Lin DY, Barnholtz-Sloan JS, et al. Common Genetic Variation in Adiponectin, Leptin, and Leptin Receptor and Association With Breast Cancer Subtypes. Breast Cancer Res Treat (2011) 129(2):593–606. doi: 10.1007/s10549-011-1517-z
23. Kaklamani VG, Hoffmann TJ, Thornton TA, Hayes G, Chlebowski R, Van Horn L, et al. Adiponectin Pathway Polymorphisms and Risk of Breast Cancer in African Americans and Hispanics in the Women's Health Initiative. Breast Cancer Res Treat (2013) 139(2):461–8. doi: 10.1007/s10549-013-2546-6
24. Pasha HF, Mohamed RH, Toam MM, Yehia AM. Genetic and Epigenetic Modifications of Adiponectin Gene: Potential Association With Breast Cancer Risk. J Gene Med (2019) 21(10):e3120. doi: 10.1002/jgm.3120
25. Teras LR, Goodman M, Patel AV, Bouzyk M, Tang W, Diver WR, et al. No Association Between Polymorphisms in LEP, LEPR, ADIPOQ, ADIPOR1, or ADIPOR2 and Postmenopausal Breast Cancer Risk. Cancer Epidemiol Biomarkers Prev (2009) 18(9):2553–7. doi: 10.1158/1055-9965.EPI-09-0542
26. Morisaki H, Yamanaka I, Iwai N, Miyamoto Y, Kokubo Y, Okamura T, et al. CDH13 Gene Coding T-Cadherin Influences Variations in Plasma Adiponectin Levels in the Japanese Population. Hum Mutat (2012) 33(2):402–10. doi: 10.1002/humu.21652
27. Qi L, Menzaghi C, Salvemini L, De Bonis C, Trischitta V, Hu FB. Novel Locus Fer is Associated With Serum HMW Adiponectin Levels. Diabetes (2011) 60(8):2197–201. doi: 10.2337/db10-1645
28. Richards JB, Waterworth D, O'Rahilly S, Hivert MF, Loos RJ, Perry JR, et al. A Genome-Wide Association Study Reveals Variants in ARL15 That Influence Adiponectin Levels. PLoS Genet (2009) 5(12):e1000768. doi: 10.1371/journal.pgen.1000768
29. Ling H, Waterworth DM, Stirnadel HA, Pollin TI, Barter PJ, Kesaniemi YA, et al. Genome-Wide Linkage and Association Analyses to Identify Genes Influencing Adiponectin Levels: The GEMS Study. Obesity (Silver Spring) (2009) 17(4):737–44. doi: 10.1038/oby.2008.625
30. Jee SH, Sull JW, Lee JE, Shin C, Park J, Kimm H, et al. Adiponectin Concentrations: A Genome-Wide Association Study. Am J Hum Genet (2010) 87(4):545–52. doi: 10.1016/j.ajhg.2010.09.004
31. Wu Y, Li Y, Lange EM, Croteau-Chonka DC, Kuzawa CW, McDade TW, et al. Genome-Wide Association Study for Adiponectin Levels in Filipino Women Identifies CDH13 and a Novel Uncommon Haplotype at KNG1-ADIPOQ. Hum Mol Genet (2010) 19(24):4955–64. doi: 10.1093/hmg/ddq423
32. Comuzzie AG, Cole SA, Laston SL, Voruganti VS, Haack K, Gibbs RA, et al. Novel Genetic Loci Identified for the Pathophysiology of Childhood Obesity in the Hispanic Population. PLoS One (2012) 7(12):e51954. doi: 10.1371/journal.pone.0051954
33. Wu Y, Gao H, Li H, Tabara Y, Nakatochi M, Chiu YF, et al. A Meta-Analysis of Genome-Wide Association Studies for Adiponectin Levels in East Asians Identifies a Novel Locus Near WDR11-Fgfr2. Hum Mol Genet (2014) 23(4):1108–19. doi: 10.1093/hmg/ddt488
34. Heid IM, Henneman P, Hicks A, Coassin S, Winkler T, Aulchenko YS, et al. Clear Detection of ADIPOQ Locus as the Major Gene for Plasma Adiponectin: Results of Genome-Wide Association Analyses Including 4659 European Individuals. Atherosclerosis (2010) 208(2):412–20. doi: 10.1016/j.atherosclerosis.2009.11.035
35. Dastani Z, Hivert MF, Timpson N, Perry JR, Yuan X, Scott RA, et al. Novel Loci for Adiponectin Levels and Their Influence on Type 2 Diabetes and Metabolic Traits: A Multi-Ethnic Meta-Analysis of 45,891 Individuals. PLoS Genet (2012) 8(3):e1002607. doi: 10.1371/journal.pgen.1002607
36. Women’s Health Initative. Design of the Women's Health Initiative Clinical Trial and Observational Study. The Women's Health Initiative Study Group. Control Clin Trials (1998) 19(1):61–109. doi: 10.1016/S0197-2456(97)00078-0
37. Women’s Health Intiative. “Volume 1, Section 1: Protocol for Clinical Trial and Observational Study Components”. In: WHI (1993 - 2005): Study Manual. Seattle, WA: WHI Clinical Coordinating Center, Fred Hutchinson Cancer Research Center. (2003).
38. NCBI Databse of Genotypes and Phenotypes (dbGaP). Women's Health Initiative - SNP Health Association Resource (WHI-SHARe). A Sub-Study of Women's Health Initiative Web Site. Available at: https://www.ncbi.nlm.nih.gov/projects/gap/cgi-bin/study.cgi?study_id=phs000386.v8.p3.
39. Women’s Health Intiative. Volume 8, Section 4: Outcome Classifications: Cancer Outcomes. In: . WHI (1993 - 2005): Study Manual. Seattle, WA: WHI Clinical Coordinating Center, Fred Hutchinson Cancer Research Center (1999).
40. Women’s Health Intiative. “Volume 2, Section 9: Clinical Measurements”. In: WHI (1993 - 2005): Study Manual (2003).
41. Centers for Disease Control and Prevention. Healthy Weight – Assessing Your Weight . Available at: https://www.cdc.gov/healthyweight/assessing/index.html.
42. World Health Organization. Waist Circumference and Waist-Hip Ratio: Report of a Who Expert Consultation (2008). Available at: http://apps.who.int/iris/bitstream/handle/10665/44583/9789241501491_eng.pdf;jsessionid=34C2160F7DCA2A5DBCBD0E975177C2D1?sequence=1.
43. Aslibekyan S, An P, Frazier-Wood AC, Kabagambe EK, Irvin MR, Straka RJ, et al. Preliminary Evidence of Genetic Determinants of Adiponectin Response to Fenofibrate in the Genetics of Lipid Lowering Drugs and Diet Network. Nutr Metab Cardiovasc Dis (2013) 23(10):987–94. doi: 10.1016/j.numecd.2012.07.010
44. Budtz-Jorgensen E, Keiding N, Grandjean P, Weihe P. Confounder Selection in Environmental Epidemiology: Assessment of Health Effects of Prenatal Mercury Exposure. Ann Epidemiol (2007) 17(1):27–35. doi: 10.1016/j.annepidem.2006.05.007
45. Knol MJ, VanderWeele TJ. Recommendations for Presenting Analyses of Effect Modification and Interaction. Int J Epidemiol (2012) 41(2):514–20. doi: 10.1093/ije/dyr218
46. Dudoit S, Shaffer JP, Boldrick JC. Multiple Hypothesis Testing in Microarray Experiments. Stat Sci (2003) 18(1):71–103. doi: 10.1214/ss/1056397487
47. Jardé T, Perrier S, Vasson MP, Caldefie-Chézet F. Molecular Mechanisms of Leptin and Adiponectin in Breast Cancer. Eur J Cancer (2011) 47(1):33–43. doi: 10.1016/j.ejca.2010.09.005
48. Grossmann ME, Nkhata KJ, Mizuno NK, Ray A, Cleary MP. Effects of Adiponectin on Breast Cancer Cell Growth and Signaling. Br J Cancer (2008) 98(2):370–9. doi: 10.1038/sj.bjc.6604166
49. Jardé T, Caldefie-Chézet F, Goncalves-Mendes N, Mishellany F, Buechler C, Penault-Llorca F, et al. Involvement of Adiponectin and Leptin in Breast Cancer: Clinical and In Vitro Studies. Endocr Relat Cancer (2009) 16(4):1197–210. doi: 10.1677/ERC-09-0043
50. Wang Y, Lam JB, Lam KS, Liu J, Lam MC, Hoo RL, et al. Adiponectin Modulates the Glycogen Synthase Kinase-3ß/ß-Catenin Signaling Pathway and Attenuates Mammary Tumorigenesis of MDA-MB-231 Cells in Nude Mice. Cancer Res (2006) 66(23):11462–70. doi: 10.1158/0008-5472.CAN-06-1969
51. Nakayama S, Miyoshi Y, Ishihara H, Noguchi S. Growth-Inhibitory Effect of Adiponectin via Adiponectin Receptor 1 on Human Breast Cancer Cells Through Inhibition of S-Phase Entry Without Inducing Apoptosis. Breast Cancer Res Treat (2008) 112(3):405–10. doi: 10.1007/s10549-007-9874-3
52. KöRner A, Pazaitou-Panayiotou K, Kelesidis T, Kelesidis I, Williams CJ, Kaprara A, et al. Total and High-Molecular-Weight Adiponectin in Breast Cancer: In Vitro and In Vivo Studies. J Clin Endocrinol Metab (2007) 92(3):1041–48. doi: 10.1210/jc.2006-1858
53. Treeck O, Lattrich C, Juhasz-Boess I, Buchholz S, Pfeiler G, Ortmann O. Adiponectin Differentially Affects Gene Expression in Human Mammary Epithelial and Breast Cancer Cells. Br J Cancer (2008) 99(8):1246–50. doi: 10.1038/sj.bjc.6604692
54. Lam JB, Chow KH, Xu A, Lam KS, Liu J, Wong NS, et al. Adiponectin Haploinsufficiency Promotes Mammary Tumor Development in MMTV-PyVT Mice by Modulation of Phosphatase and Tensin Homolog Activities. PLoS One (2009) 4(3):e4968. doi: 10.1371/journal.pone.0004968
55. Cohen SS, Gammon MD, North KE, Millikan RC, Lange EM, Williams SM, et al. ADIPOQ, ADIPOR1, and ADIPOR2 Polymorphisms in Relation to Serum Adiponectin Levels and Bmi in Black and White Women. Obes (Silver Spring) (2011) 19(10):2053–62. doi: 10.1038/oby.2010.346
56. Guo C, Stark GR. Fer Tyrosine Kinase (FER) Overexpression Mediates Resistance to Quinacrine Through EGF-Dependent Activation of NF-κb. Proc Natl Acad Sci U S A (2011) 108(19):7968–73. doi: 10.1073/pnas.1105369108
57. Zoubeidi A, Rocha J, Zouanat FZ, Hamel L, Scarlata E, Aprikian AG, et al. The Fer Tyrosine Kinase Cooperates With Interleukin-6 to Activate Signal Transducer and Activator of Transcription 3 and Promote Human Prostate Cancer Cell Growth. Mol Cancer Res (2009) 7(1):142–55. doi: 10.1158/1541-7786.MCR-08-0117
58. Wang S, Sun Y. The IL-6/JAK/STAT3 Pathway: Potential Therapeutic Strategies in Treating Colorectal Cancer (Review). Int J Oncol (2014) 44(4):1032–40. doi: 10.3892/ijo.2014.2259
59. Wang W, Nag SA, Zhang R. Targeting the Nfκb Signaling Pathways for Breast Cancer Prevention and Therapy. Curr Med Chem (2015) 22(2):264–89. doi: 10.2174/0929867321666141106124315
60. Biswas DK, Shi Q, Baily S, Strickland I, Ghosh S, Pardee AB, et al. Nf-κb Activation in Human Breast Cancer Specimens and its Role in Cell Proliferation and Apoptosis. Proc Natl Acad Sci U S A (2004) 101(27):10137–42. doi: 10.1073/pnas.0403621101
61. Johnson DE, O'Keefe RA, Grandis JR. Targeting the IL-6/JAK/STAT3 Signalling Axis in Cancer. Nat Rev Clin Oncol (2018) 15(4):234–48. doi: 10.1038/nrclinonc.2018.8
62. Sánchez-Jiménez F, Pérez-Pérez A, de la Cruz-Merino L, Sánchez-Margalet V. Obesity and Breast Cancer: Role of Leptin. Front Oncol (2019) 9:596. doi: 10.3389/fonc.2019.00596
63. Bar-Shavit R, Maoz M, Kancharla A, Nag JK, Agranovich D, Grisaru-Granovsky S, et al. G Protein-Coupled Receptors in Cancer. Int J Mol Sci (2016) 17(8):1320. doi: 10.3390/ijms17081320
64. Wu C, Hwang SH, Jia Y, Choi J, Kim YJ, Choi D, et al. Olfactory Receptor 544 Reduces Adiposity by Steering Fuel Preference Toward Fats. J Clin Invest (2017) 127(11):4118–23. doi: 10.1172/JCI89344
65. Weber L, Maßberg D, Becker C, Altmüller J, Ubrig B, Bonatz G, et al. Olfactory Receptors as Biomarkers in Human Breast Carcinoma Tissues. Front Oncol (2018) 8:33. doi: 10.3389/fonc.2018.00033
66. Masjedi S, Zwiebel LJ, Giorgio TD. Olfactory Receptor Gene Abundance in Invasive Breast Carcinoma. Sci Rep (2019) 9(1):13736. doi: 10.1038/s41598-019-50085-4
67. Neuhaus EM, Zhang W, Gelis L, Deng Y, Noldus J, Hatt H. Activation of an Olfactory Receptor Inhibits Proliferation of Prostate Cancer Cells. J Biol Chem (2009) 284(24):16218–25. doi: 10.1074/jbc.M109.012096
68. Miyoshi Y, Funahashi T, Kihara S, Taguchi T, Tamaki Y, Matsuzawa Y, et al. Association of Serum Adiponectin Levels With Breast Cancer Risk. Clin Cancer Res (2003) 9(15):5699–704.
69. Picon-Ruiz M, Morata-Tarifa C, Valle-Goffin JJ, Friedman ER, Slingerland JM. Obesity and Adverse Breast Cancer Risk and Outcome: Mechanistic Insights and Strategies for Intervention. CA Cancer J Clin (2017) 67(5):378–97. doi: 10.3322/caac.21405
Keywords: obesity, adiponectin, postmenopausal breast cancer, African American women, single nucleotide polymorphism
Citation: Nam GE, Zhang Z-F, Rao J, Zhou H and Jung SY (2021) Interactions Between Adiponectin-Pathway Polymorphisms and Obesity on Postmenopausal Breast Cancer Risk Among African American Women: The WHI SHARe Study. Front. Oncol. 11:698198. doi: 10.3389/fonc.2021.698198
Received: 21 April 2021; Accepted: 02 July 2021;
Published: 21 July 2021.
Edited by:
Farnam Mohebi, University of California, Berkeley, United StatesCopyright © 2021 Nam, Zhang, Rao, Zhou and Jung. This is an open-access article distributed under the terms of the Creative Commons Attribution License (CC BY). The use, distribution or reproduction in other forums is permitted, provided the original author(s) and the copyright owner(s) are credited and that the original publication in this journal is cited, in accordance with accepted academic practice. No use, distribution or reproduction is permitted which does not comply with these terms.
*Correspondence: Su Yon Jung, c2p1bmdAc29ubmV0LnVjbGEuZWR1