- 1Department of Gastrointestinal Gland Surgery, The First Affiliated Hospital of Guangxi Medical University, Nanning, China
- 2Clinical Research Lab, Guangxi Key Laboratory of Enhanced Recovery After Surgery for Gastrointestinal Cancer, Nanning, China
- 3Department of Gastrointestinal, Hernia and Enterofistula Surgery, The People’s Hospital of Guangxi Zhuang Autonomous Region, Nanning, China
Introduction: MicroRNAs (miRNAs) are small noncoding RNA molecules that regulate genetic expression and are also vital for tumor initiation and development. MiR-29b-3p was found to be involved in regulating various biological processes of tumors, including tumor cell proliferation, metastasis, and apoptosis inhibition; however, the biofunction and molecule-level mechanisms of miR-29b-3p inpapillary thyroid carcinoma (PTC) remain unclear.
Methods: The expression of miR-29b-3p in PTC samples was tested via qRT-PCR. Cellular proliferation was analyzed by CCK-8 and EdU assays, and cellular migratory and invasive abilities were assessed utilizing wound-healing and Transwell assays. In addition, protein expressions of COL1A1, COL5A1, E-cadherin, N-cadherin, Snail, and Vimentin were identified via Western blot (WB) assay. Bioinformatics, qRT-PCR, WB, and dual luciferase reporter assays were completed to identify whether miR-29b-3p targeted COL1A1 and COL5A1. In addition, our team explored the treatment effects of miR-29b-3p on a murine heterograft model.
Results: Our findings revealed that miR-29b-3p proved much more regulated downward in PTC tissue specimens than in adjacent non-cancerous tissues. Meanwhile, decreased expression of miR-29b-3p was strongly related to the TNM stage of PTC patients (p<0.001), while overexpression of miR-29b-3p in PTC cells suppressed cellular migration, invasion, proliferation, and EMT. Conversely, silencing miR-29b-3p yielded the opposite effect. COL1A1 and COL5A1 were affirmed as the target of miR-29b-3p. Additionally, the COL1A1 and COL5A1 were highly expressed in PTC tumor samples than in contrast to neighboring healthy samples. Functional assays revealed that overexpression of COL1A1 or COL5A1 reversed the suppressive role of miR-29b-3p in migration, invasion, and EMT of PTC cells. Finally, miR-29b-3p agomir treatment dramatically inhibited Xenograft tumor growth in the animal model.
Conclusions: These findings document that miR-29b-3p inhibited PTC cells invasion and metastasis by targeting COL1A1 and COL5A1; this study also sparks new ideas for risk assessment and miRNA replacement therapy in PTC.
Introduction
Papillary thyroid carcinoma (PTC) is the most common primary malignancy of the endocrine system, with the incidence increasing year by year worldwide (1, 2). Its prevalence among female individuals across the world is 10.2 per 100,000 individuals, which constitutes threefold that of male individuals; however, PTC patients demonstrate excellent long-term prognosis (3, 4). About 30%–65% of PTC cases feature performed lymph node metastasis, and <10% of PTC cases have shown distant metastasis in the bones and lungs (5). Although surgical resection remains the most effective treatment for PTC, and active surveillance is suggested for select low-risk PTC patients, according to the 2015 ATA guidelines, a reluctance to offer it continues due to lack of robust evidence or protocols (6). On the other hand, the clinical outcomes of PTC patients attended by high invasion and proliferation remain poor (7). Approximately 5%–10% of PTC sufferers encounter relapse in 5 years of primary treatment, which reduces patient survival (8). For that reason, it remains vital to expose the potential causal link between PTC development and etiopathogenesis, which might deliver us additional PTC regimens.
MiRNAs are endogenetic single-stranded non-coding RNA molecules that bind to protein-coding genes by means of 3′-untranslated region (3′-UTR) of target messenger RNA mediating post-transcriptional gene expression (9, 10). An ever-growing number of proofs demonstrate that miRNAs participate in the biological processes of cancer (11, 12). Studies have revealed that miR-29b-3p is involved in the initiation and progress of various cancers, including pulmonary carcinoma, prostate cancer, cholangiocarcinoma, pancreatic cancer, and bladder cancer (13–17). Dysregulation of miR-29b-3p expression has been implicated in cell promotion, migration, and invasion in these tumors. Nevertheless, the effects of miR-29b-3p on thyroid carcinoma remain elusive.
Extra-cellular matrix (ECM) is closely correlated with tumor progression (18). Collagens, the main protein component of ECM, exhibit a variety of effects, demonstrating proliferative, migratory, and differentiative cellular activities in vivo (19). In turn, miR-29b-3p is a major regulator of the collagen family, which has been studied in a plurality of basic studies in the context of a range of diseases (20–22). Nevertheless, no studies regarding the regulation of collagen by miR-29b-3p in PTC are found in the literature.
The present research demonstrated that miR-29b-3p was low expressed in PTC samples, which was negatively associated with the proliferative and metastatic abilities of PTC cells. Furthermore, we confirmed that COL1A1 and COL5A1 are two immediate targets of miR-29b-3p and participated in the effects of miR-29b-3p on the biological capacity of PTC cells.
Finally, we contended that miR-29b-3p may serve as an underlying target for PTC treatment and exert pivotal effects on progression.
Materials and Methods
Bioinformatics Analysis
MicroRNA expression data retrieved from the Gene Expression Omnibus (GEO) database were collected to compare miR-29b-3p expression between human PTC samples and their adjacent normal, benign thyroid tissues. The GEO database (GSE15740) was adopted in order to analyze the expression of genes. Subsequently, differential analysis was carried out on differentially expressed genes with R language in the “limma” R package of Bioconductor, by screening criteria, viz., |logFC| > 1.8 and p-value < 0.05. Furthermore, heatmaps were generated in R, with the “ggplots” R package.
mRNA expression (mRNA SeqV2) data of human PTC were extracted from The Cancer Genome Atlas (TCGA) database. Furthermore, we predicted the downstream target gene of miR-29b-3p through the online prediction software miRmap, microTissue, miRanda, PicTar, and TargetScan to determine the downstream target gene of miR-29b-3p. Thereafter, a protein–protein interaction (PPI) net was established via the STRING (https://string-db.org/) database. Gene Ontology (GO) and Kyoto Encyclopedia of Genes and Genomes (KEGG) assays were conducted employing the GO database (http://geneontology.org) and the KEGG database (http://www.genome.jp/kegg/), respectively.
Patients and Tissue Samples
From January 2020 to November 2020, fresh tissue samples (PTC tumor and neighboring healthy samples) were harvested from PTC sufferers from The First Affiliated Hospital of GuangXi Medical University, none of whom had received any adjuvant treatment before surgery, with respect to which, it bears noting that the present research was accepted by the Ethical Board of The First Affiliated Hospital of GuangXi Medical University (Nanning, China). A total of 48 pairs of tissue samples were collected; the specimens were maintained in liquid nitrogen for long-term preservation and stored at −80°C for short-term assays.
Cell Culture and Transfection
Human PTC (K1, TPC-1, KTC-1, and B-CPAP) and immortalized thyroid lineage cells (Nthyori3-1) purchased from the Type Culture Collection of the Chinese Academy of Sciences (Shanghai, China) were cultivated in Roswell Park Memorial Institute (RPMI) intermediary 1640 basic (RPMI 1640) (Thermo Fisher Scientific, MA, USA) and DMEM (Gibco, CA, USA) mixed with 100 mg/L streptomycins, 100 kU/L penicillin, and 10% fetal bovine serum (FBS) (HyClone, Logan, UT, USA) and kept in a 37°C incubator at 5% carbon dioxide.
The mimics/inhibitors of miR-29b-3p (miR-29b-3p mimic/miR-29b-3p inhibitor) and negative control (NC mimic/NC inhibitor) were purchased from Ribobio (RiboBio, Guangzhou, China). The small interfering RNAs of COL1A1 (si-COL1A1), COL5A1 (si-COL5A1), and negative control (NC) (GenePharma, Shanghai, China) were adopted to transfect the PTC cells with Lipofectamine 8000 reagent (Invitrogen, CA, USA). Overexpression of COL5A1 and COL1A1 was achieved by transfecting pcDNA 3.1 vector-cloned with gene sequences of COL5A1 and COL1A1 into PTC cells with Lipofectamine 3000 reagent (Thermo Fisher Scientific, MA, USA). Subsequently, these transfected PTC cells were cultivated for 24–48h before all the experiments herein were conducted.
qRT-PCR
This study adopted a Fastpure Cell/Tissue Overall RNA Separation tool V2 (Vazyme, Nanjing, China) to extract all the RNAs and a reverse transcriptase tool (Vazyme, Nanjing, China) to prepare the cDNAs of COL5A1 and COL1A1 and miR-29b-3p; qRT-PCR was completed via the real-time fluorescent quantitation PCR equipment (ABI7500, America) with the SYBR qPCR Master Mix tool kit (Vazyme, Nanjing, China). The relative miR-29b-3p, COL1A1, and COL5A1 were identified via applying the 2−ΔΔCt formula, where U6 and glyceraldehyde 3-phosphate dehydrogenase (GAPDH) were regarded as the internal references. The sequences of the entire primers herein are shown in Table 1.
Western Blot
Initially, a radio-immunoprecipitation assay (RIPA) lysis buffering solution (Beyotime, Shanghai, China) was applied to abstract proteins. Following the separation of proteins by sodium salt-polyacrylamide gel electrophoresis, target proteins were electrophoretically moved to nitrocellulose films, blocked via 5% milk-TBST for 2 h under room temperature. Subsequently, they were washed three times in TBST buffering solution and cultivated with the first anti-substances, viz., COL5A1, COL1A1, E-cadherin, N-cadherin, snail, GADPH rabbit polyclonal antibody (Proteintech, USA), vimentin, and vinculin mouse polyclonal antibody (Proteintech, USA) at 4°C overnight. Subsequently, the films were washed three times in TBST again, each for 10 min. Goat anti-rabbit IgG H&L (HRP) and goat anti-mouse IgG H&L (HRP) (Abcam, USA) were added then for cultivation. Afterwards, the membranes were cleaned in TBST as per the steps mentioned above to analyze relative protein expression with Image-Pro Plus software 6.0.
Wound-Healing Assay
When the confluency of cells reached 80%–90%, a 200-μl pipet was employed to scratch along the central axis of the culture dish, which was then washed twice with PBS. Subsequently, the cells were cultivated in an intermediary without serum. Furthermore, wound-healing pictures were harvested under a microscope at 0 and 24 h after the wound occurrence. We measured the migration rate with NIH ImageJ software.
Transwell Assay
Transwell assay included migration and invasion assays. The migrating cells (4 × 105) were inoculated into a 24-transwell chamber with an 8-µm Matrigel-coated aperture film, and the invading cells (5 × 105) were uniformly inoculated in a 24-well plate containing matrix glue. The upper chamber contained RPMI 1640 intermediary without serum, whereas the lower chamber was filled with RPMI 1640 medium mixed with 10% FBS. Subsequent to the incubation under 37°C for 24 h, the cells on the superficial region of the film were discarded via a cotton swab, and those on the lower surface of the membrane were dyed with gentian violet dye after fixation with 75% alcohol. Later, the cells were imaged via a microscopic device in order to obtain the images for analysis with NIH ImageJ software.
CCK-8 Assay
Cells in the log-growth stage (200 µl) were seeded in a 96-well plate at a concentration of 1×105 cells per well and incubated for 24 h. Thereafter, 10 µl of CCK8 reagent was supplemented to all wells, followed by cultivation in a dark incubator for 120 min. Thereafter, the optical density (OD) results of this mixture (cells + reagent) were identified via ELISA with a wavelength of 450 nm. The proliferation rate of cells was determined by comparing the OD values of the mixture after inoculation for 6, 24, 48, 72, and 96 h.
EdU Assay
An EdU assay kit (RiboBio, Guangzhou, China) was adopted for carrying out the EdU assay. Initially, 2 ml of cells were plated into a 96-well dish with a concentration of 4 × 104 cells/well, cultivated with EdU reaction fluid (50 µM) for 2 h, followed by permeabilization with 0.3% TritonX-100 (Jiayan Biotech, Guangzhou, China) for 10 min, and fixation in 4% paraformaldehyde (Leagene, Beijing, China) for 0.5 h. Subsequently, 3% bovine serum albumin (BSA) (Solarbio, Beijing, China) was applied to wash the cells three times, each for 5 min, after which the cells were treated with an Apollo reaction mixture for 0.5 h, before being cleaned three times in 3% BSA, each for 5 min. Subsequently, the nuclei of cells were dyed in DAPI (Solarbio, Beijing, China) for 10 min and examined under the microscope.
Immunofluorescence
After being cultured, the cells were subjected to fixation in 4% paraformaldehyde (Leagene, China) for 15 min and permeabilization in 0.3% Triton (Jiayan Biotech, China) and finally blocked with 3% BSA (Solarbio, China) for 30 min. Cells were afterwards cultivated with primary antibodies, viz., E-cadherin rabbit polyclonal anti-substance 1:100 and vimentin mouse polyclonal antibody 1:200 under 4°C overnight. Subsequent to cleaning, the cells were cultivated with anti-mouse IgG fluorescence-conjugated secondary anti-substance, anti-rabbit IgG fluorescence-conjugated secondary anti-substance (goat anti-mouse IgG H&L, 1:2,000), and goat antirabbit IgG H&L, 1:2,000 (Proteintech, USA) under room temperature for 60 min. Then, the nuclei of cells were stained via DAPI (1 µg/ml) (Solarbio, China), which were observed under a fluorescent microscope (DM6 B; Leica Microsystems) at 63× magnification, to analyze the captured images with Fiji software.
Animal Experiments
All the animal assays were accepted by the GuangXi Medical University Ethical Board on Animal Research. Nude mice with a BAL/c background (6-week-old females with a bodyweight of 16–18 g) from Junke Bioengineering Co., Ltd. (Nanjing, China) were raised and monitored according to requirements for a specific pathogen-free (SPF) status. For the cancer-growth assays, 1 × 106 K1 cells were introduced into BALB/c nude mice via injection. The tumorous volume was observed and measured every 3 days throughout the experimental period with Vernier calipers. One week later, tumorous volume was computed via the equation: volume = 0.5 × length × width2.
Approximately 21 days later, when the cancers reached a mean volume of 100 mm3, the animals were stochastically separated into two groups (five mice/group) and were delivered an intratumoral injection containing 20 mg/kg miR-29b-3p agomir or control agomir (Guangzhou, China) every 3 days for 12 days (four injections in total), which was repeated four times. After 33 days, the tumors were collected from the sacrificed mice and weighed; the growth of these xenografts was evaluated after the tumors were removed, weighed, and photographed, with tissues fixed with 4% paraformaldehyde and paraffin-embedded for immunohistochemistry (IHC) analyses.
Immunohistochemistry
Immunohistochemistry of mouse tumors was performed according to the following standard procedures: tumor tissue embedding, specimen sectioning, dewaxing, rehydration, and antigen recovery. After serum occlusion, sections were incubated with COL1A1 or COL5A1 antibody (1:100, desaturated in PBS with 1% standard goat serum) under 4°C for a single night and afterwards were incubated with second anti-substances under RT for 60 min. The staining procedure was performed following the DAB detection kit protocol with DAB (DAB-0031; Fuzhou Maixin Biotech Company, Fuzhou, China).
Dual-Luciferase Reporter Assay
In order to examine the straight binding of miR-29b-3p to target genes, COL1A1 and COL5A1, a luciferase reporter assay was completed as previously described. Reporter vectors, namely, Luc-COL1A1 and Luc-COL5A1, or mutation vectors with miR-29b-3p-binding spot mutations, were cotransfected with miR-29b-3p mimetic substances (RiboBio, China) into HEK-293 T cells. Firefly luciferase and Renilla fluorescein enzyme activity were identified following transfection for 48 h with the Dual-Luciferase Reporter Assay System as per the supplier’s specification. Moreover, the miR-29b-3p promotor-reporter construct with wild type was introduced into HEK-293 T cells via transfection, and the pTK-Club vector was considered the control, all of which were treated with hypoxic or normoxic conditions for 48 h.
Quantification and Statistical Analysis
Statistical analyses and the construction of graphs were conducted with statistical packages, namely, GraphPad Prism 5 (USA) and SPSS 21.0 (IBM, USA). The data collected in the experiments are described as x ± SD. A t-test was used for cell testing of intergroup contrast, and Mann–Whitney U-test was utilized for clinical data analysis. A Kruskal–Wallis test was applied when comparing three or more groups. p < 0.05 indicates a remarkable diversity.
Results
Downregulation of miR-29b-3p Is Associated With Disease Development in Human PTC
The expression of miR-29b-3p was much more downregulated in PTC tissues than adjacent normal tissues in GEO databases (GSE15740) (filtered by p < 0.05, Figures 1A, B). This result was validated in our 48 pairs of tissue samples by qRT-PCR (Figure 1C). Specifically, miR-29b-3p expression was downregulated in 62.5% of samples, while no significant difference was demonstrated in 27.08% of samples and was upregulated in 10.42% of samples (Figure 1D). Subsequently, we investigated the association of miR-29b-3p with clinicopathological features, which demonstrated an obvious decrease in miR-29b-3p expression in N1 vs. N0 PTCs, in T3/T4 vs. T1/T2 PTCs, in TNM stage II/III vs. I PTCs, and in age ≥55 years vs. <55 years PTCs (Figures 1E–H; Table 2).
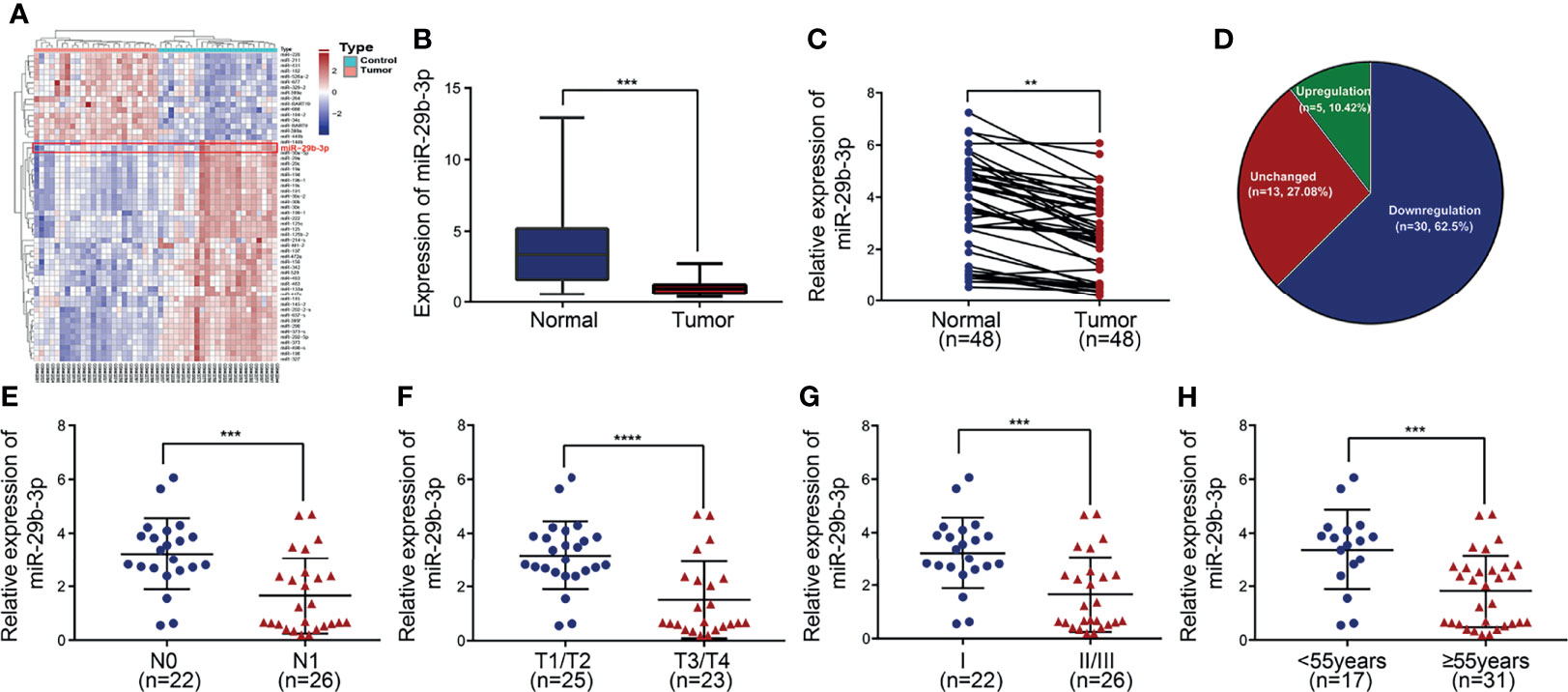
Figure 1 Downregulation of miR-29b-3p is associated with disease progression in PTC. (A) Heatmap of differentially expressed genes in GEO dataset coloring the samples groups. (B) Expression level of miR-29b-3p in PTC based on data retrieved from the GEO database and the ArrayExpress database. (C, D) The expression of miR-29b-3p was evaluated by qRT-PCR in 48 paired human PTC tissues and their matched adjacent non-tumor thyroid tissues. MiR-29b-3p expression was significantly downregulated in PTC tumor tissues compared with the corresponding non-tumor thyroid tissues (U6 was used as an internal control). (E–H) Relationships between miR-29b-3p expression and TMN stage of PTC. In the figure, **p < 0.01, ***p < 0.001, ****p < 0.0001.
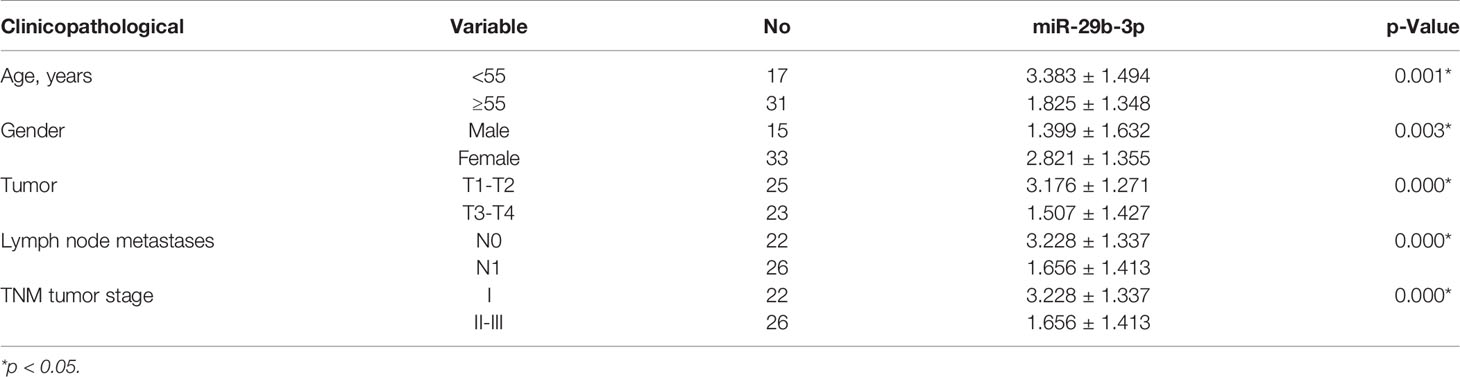
Table 2 The relationship between the relative expression of miR-29b-3p and clinicopathological characteristics in thyroid cancer patients.
miR-29b-3p Inhibits PTC Cell Proliferation and Metastasis In Vitro
To understand the mechanism involved in the downregulation of miR-29b-3p in PTC, its expression levels of miR-29b-3p were examined, employing qRT-PCR in the normal thyroid cell line (Nthyori3-1) and 4 PTC cell lines (TPC-1, KTC-1, K1, and B-CPAP). Among these PTC cell lines, the K1 and the B-CPAP cells showed the lowest expression of miR-29b-3p. Thus, these two cell lines were therefore selected for the follow-up experiments (Figure 2A).
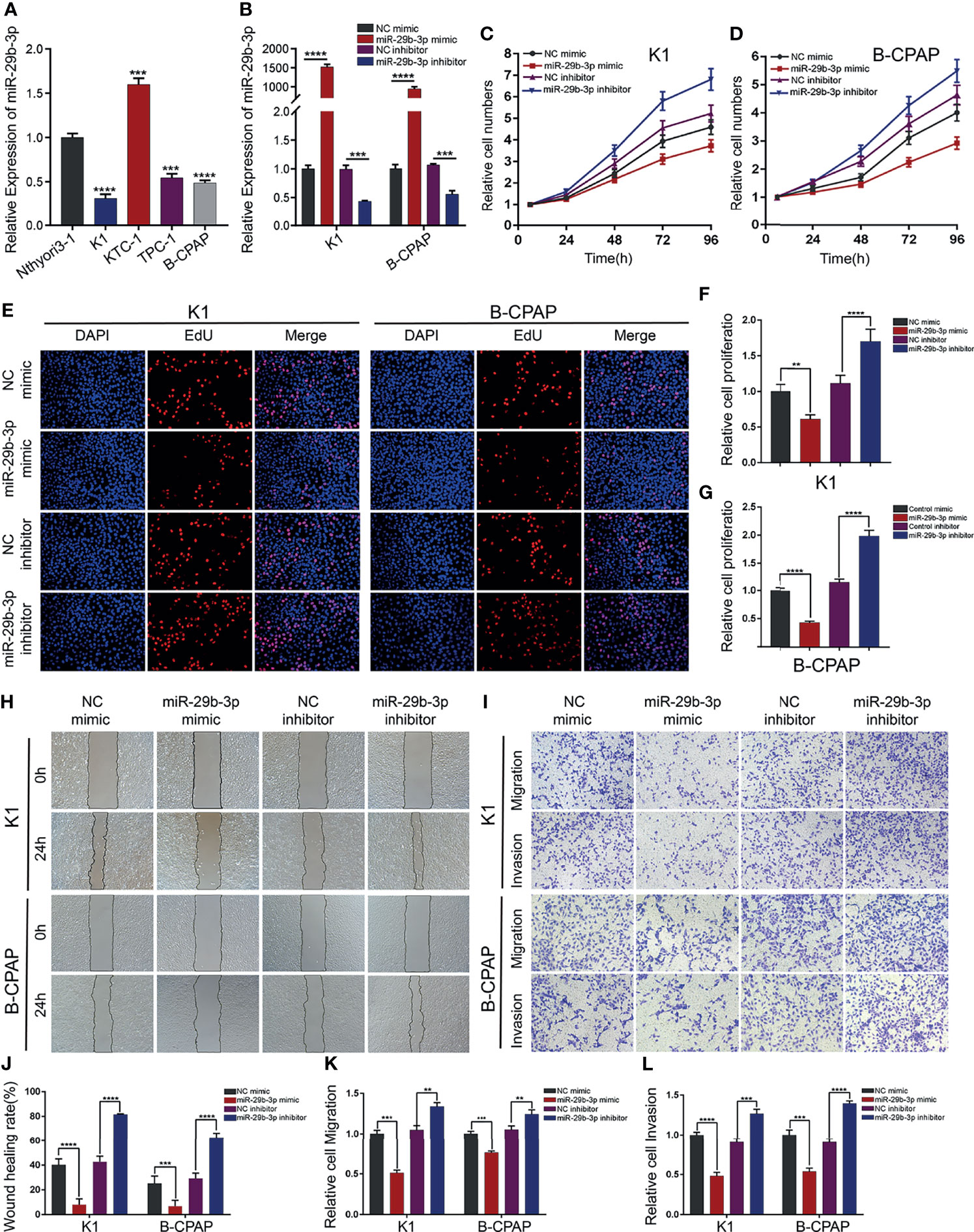
Figure 2 miR-29b-3p inhibits PTC cell migration and invasion in vitro. (A) Expression of miR-29b-3p was verified by qRT-PCR in PTC cell lines (K1, KTC-1, TPC-1, and B-CPAP) and normal thyroid cell lines (Nthyori3–1); two lowly expressing (K1 and B-CPAP) cell lines were selected for further study. (B) The knockdown and overexpression efficiencies of miR-29b-3p was verified by qRT-PCR after transfection with miR-29b-3p mimic/inhibitor or NC mimic/inhibitor. Cell proliferation ability of PTC cells was determined by CCK-8 assays (C, D) and EdU assays (E–G) after miR-29b-3p overexpression or knockdown. The effects of miR-29b-3p overexpression and knockdown in PTC cells on cell migration and invasion were analyzed by wound healing assays (H) and Transwell assays (I). (J, L) Results of the Transwell assays and wound healing assays. In the figure,**p < 0.01, ***p < 0.001,****p < 0.0001.
To explore the function of miR-29b-3p in PTC cells, K1 and B-CPAP cells were transfected with miR-29b-3p mimic or miR-29b-3p inhibitor, NC mimic and NC inhibitor were transfected as respective controls; and transfection efficiency was evaluated by qRT-PCR. It was observed that the expression level of miR-29b-3p significantly increased in PTC cells after transfection with miR-29b-3p mimic as compared to that in cells transfected with NC mimic. In addition, the expression of miR-29b-3p significantly decreased after transfection of the cells with miR-29b-3p inhibitor (Figure 2B).
CCK-8 and EdU assays revealed that, when transfected with miR-29b-3p mimic, the proliferation ability of PTC cells was reduced significantly in comparison to those transfected with NC mimic. In turn, the downregulated expression of miR-29b-3p contributed to the proliferation of PTC cells (Figures 2C–E; Supplementary Table S2). Accordingly, the results were quantified and are presented in Figures 2F–G (all p-values <0.01).
Furthermore, the PTC cells’ migration and invasive ability were assessed using Transwell and wound-healing assays (48 h after transfection for K1 and the B-CPAP cells). The results suggested that overexpression of miR-29b-3p inhibited proliferation and migration abilities, while an opposite effect occurred in the miR-29b-3p-knockdown cells; the results are presented in Figures 2H–I. The quantification of these results is shown in Figures 2J–L and Supplementary Tables S3 and S4 (**p < 0.01 and *** p < 0.001; **** p < 0.0001).
miR-29b-3p Directly Targets COL1A1 and COL5A1
Using online software, we predicted potential targets of miR-29b-3p, and 17 genes were found to be potential targets (Figures 3A, B). GO function annotations and KEGG pathway analysis were carried out for the target genes. The results revealed that the potential target genes are involved in the regulation of ECM adhesion dynamics (Figure 3C). The PPI network data, with the confidence score set at 0.4, revealed that the hub genes bearing higher degrees comprised COL1A1, COL2A1, COL5A1, COL7A1, COL11A, and COL9A1 (Figure 3D). Based on the above results, we further validated the relationship between hub genes and miR-29b-3p. Specifically, we performed qRT-PCR to detect the expression of hub genes at mRNA levels in PTC cells transfected with miR-29b-3p mimic or inhibitor. Both COL1A1 and COL5A1 showed significant inverse correlations with miR-29b-3p expression in PTC cells (Figures 3E, F). Western blot analysis results showed that miR-29b-3p overexpression obviously suppressed the protein expression of COL1A1 and COL5A1 in PTC cells (Figures 3G, H; Supplementary Table S5).
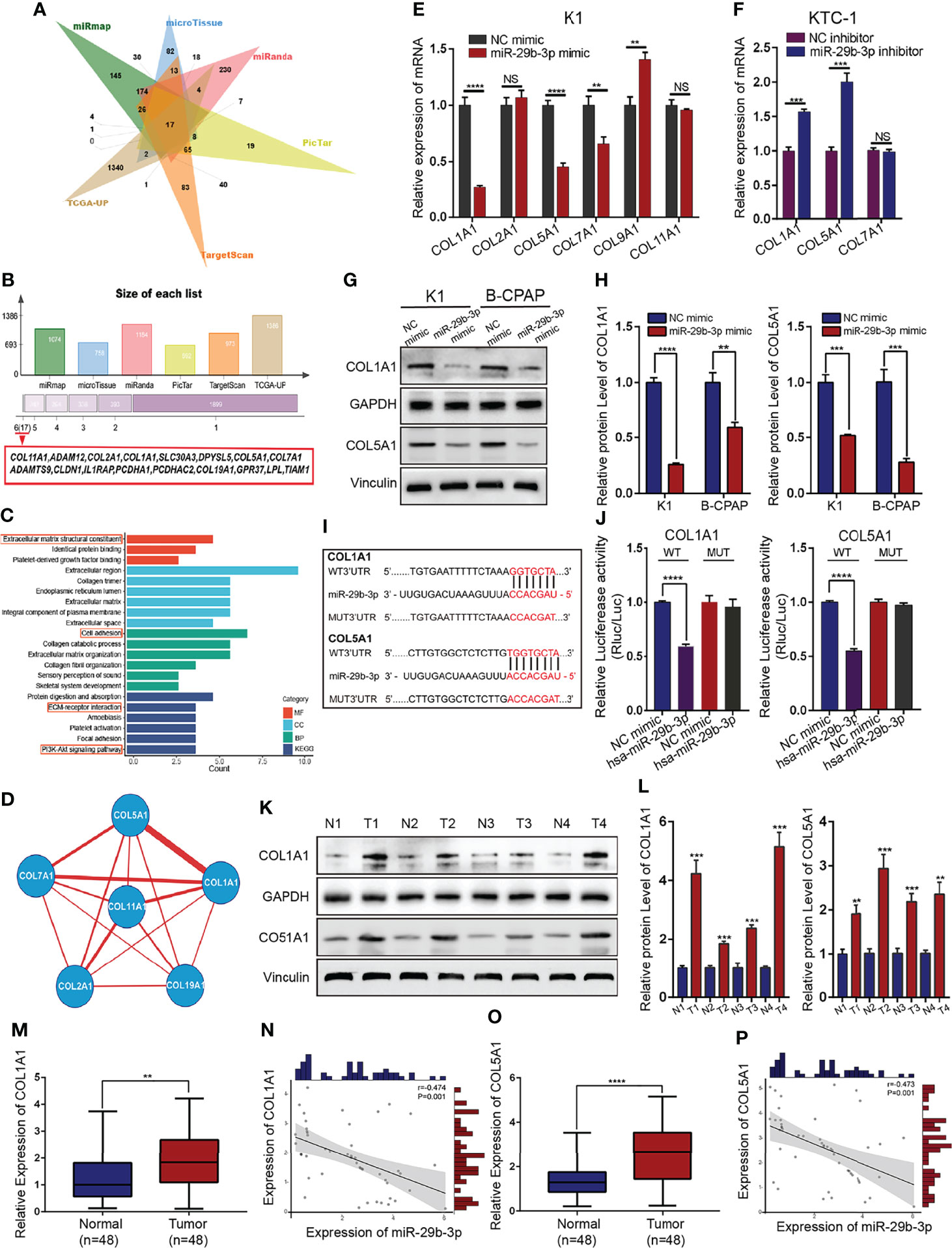
Figure 3 COL1A1 and COL5A1 as direct targets of miR-29b-3p in PTC tissues. (A, B) The bioinformatic predictions of miR-29b-3p candidate target genes and Venn diagram depicting the overlap of target genes predicted by five miRNA databases (miRmap, microTissue, miRanda, PicTar, and TargetScan) and TCGA datasets. (C) GO and KEGG enrichment analysis of candidate miR-29b-3p target genes. (D) The miR-target gene regulatory network constructed using Cytoscape. (E, F) Fold change in mRNA expression of six candidate target genes upon transfection of miR-29b-3p mimic/inhibitor and validation of COL1A1 and COL5A1 as the direct target genes of miR-29b-3p by qRT-PCR. (G, H) Western blot analysis of COL1A1 and COL5A1 expression after transfection with miR-29b-3p mimics. (I, J) The 3-UTRs of COL1A1 and COL5A1 as potential binding sites for miR-29b-3p, determined by the luciferase assays. (K, L) Western blots showing the relative protein expression levels of COL1A1 and COL5A1 in PTC tissues and adjacent non-cancerous normal tissues from four patients. (M–P) Relative expression levels of COL1A1 and COL5A1 in 48 paired human PTC tissues and adjacent non-tumor thyroid tissues, as evaluated by qRT-PCR. Both COL1A1 and COL5A1 mRNA highly expressed in PTC tissues and negatively correlated with miR-29b-3p expression. In the figure, **p < 0.01, ***p < 0.001, ****p < 0.0001; NS, not significant.
To test whether COL1A1 and COL5A1 have a transcriptional regulatory relationship, we separately overexpressed COL1A1 and COL5A1 in order to determine whether the overexpression of COL1A1 and COL5A1 would bear an influence on COL5A1 and COL1A1 expression in PTC cells, and accordingly, qRT-PCR analysis results demonstrated no transcriptional regulatory relationship between COL1A1 and COL5A1.
Hence, a dual‐luciferase reporter assay was employed for further verification of the targeted relationship between miR-29b-3p and COL1A1/COL5A1. For this investigation, 3′-UTRs of these two genes were initially cloned downstream of firefly luciferase. Thereafter, the luciferase reporters were co-transfected into HEK-293 T cells with miR-29B-3p mimics. We observed that overexpression of miR-29b-3p significantly reduced luciferase activity in vectors containing COL1A1 and COL5A1 3′-UTR. Moreover, a mutation in the seed regions within 3′-UTRs of either COL1A1 or COL5A1 blocked the inhibition of these genes by miR-29b-3p (Figures 3I, J; Supplementary Table S6).
The mRNA and protein expression levels of COL1A1 and COL5A1 in PTC tissues and adjacent normal tissues were determined by qRT-PCR and WB analysis. We determined that COL1A1 and COL5A1 were upregulated in PTC tissues and inversely correlated with miR-29b-3p expression (Figures 3K–P; Supplementary Table S1). From these results, it could be inferred that miR-29b-3p downregulated COL1A1 and COL5A1 by means of directly targeting their 3′-UTRs.
Overexpression of COL1A1 or COL5A1 Partially Blocked the Inhibitive Effects of miR-29b-3p on Cell Invasion
To investigate whether the proliferation and migration suppressive effect of miR-29b-3p on PTC cells was mediated by repression of COL1A1 or COL5A1, K1 and B-CPAP cells were transfected with COL1A1 or COL5A1 overexpression plasmid, while the control group was transfected with empty vector plasmids. The overexpression efficiency of constructed plasmids was validated by qRT-PCR (Figures 4A, B) and Western blot (Figures 4C–E; Supplementary Table S7). Afterwards, PTC cells were transfected with NC mimic, miR-29b-3p mimic, miR-29b-3p mimic + Vector, miR-29b-3p mimic + OE- COL1A1, or miR-29b-3p mimic + OE- COL5A1, respectively. Furthermore, the invasion assay indicated that overexpression of miR-29b-3p inhibits invasion and migration of PTC cells. However, the co-transfection of miR-29b-3p mimic and OE-COL1A1 or OE-COL5A1 partially restored the ability of cells to invade (Figures 4F, G; Supplementary Tables S8, S9). Experiments were repeated independently, and the results were quantified (Figures 4H–J). Nevertheless, this result was not confirmed in cell proliferation experiments. Based on the above analysis, we hypothesized that COL1A1 and COL5A1 might be mainly involved in the proliferation and invasion of PTC cells.
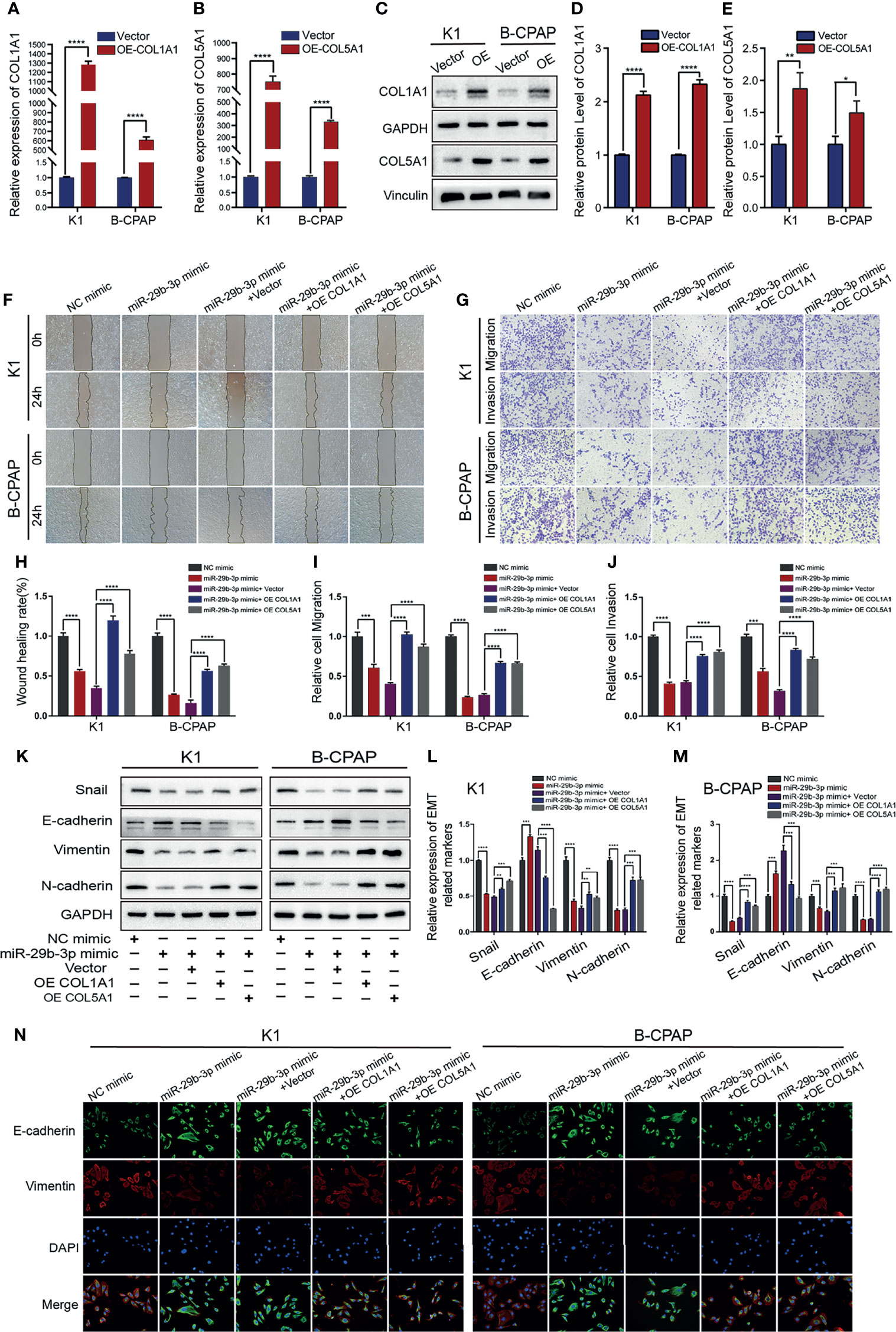
Figure 4 Overexpression of COL1A1 or COL5A1 partially blocks the suppressive effects of miR-29b-3p on invasion in PTC cells. (A, B) qRT-PCR and (C) Western blot were performed to assess the transfection efficiency of COL1A1 and COL5A1 overexpression plasmids. (D, E) Quantification of COL1A1 and COL5A1 levels in Western blot normalized over GAPDH and Vinculin. The wound healing assays (F) and Transwell assays (G) demonstrated that the overexpression of COL1A1 and COL5A1 could efficiently alleviate the miR-29b-3p mimic-induced inhibition of the migration and invasion activities of K1 and B-CPAP cells. (H–J) Quantified results of the wound-healing assays and Transwell assays. Inhibition of miR-29b-3p mimic-induced EMT by overexpressed COL1A1 or COL5A1; the EMT-related proteins were investigated by (K) Western blot and (N) immunofluorescence. (L, M) The EMT-related proteins expression for each group was analyzed by Image J software. In the figure, *p < 0.05, **p < 0.01, ***p < 0.001, ****p < 0.0001.
The tumor invasion and metastatic potential were enhanced by the epithelial–mesenchymal transition (EMT). We examined whether miR-29b-3p, COL1A1, and COL5A1 promote EMT; in indicated cells after the same transfection, Western blot detected EMT‐related proteins including E-cadherin, Snail, Vimentin, and N-cadherin. After treatment with the miR-29b-3p mimic, the results indicated that the expression of E-cadherin was upregulated in the K1 and B-CPAP cells. In contrast, the expressions of Snail, Vimentin, and N-cadherin were downregulated. These results established that miR-29b-3p inhibited the EMT process. However, the results were reversed after transfection of the K1 and B-CPAP cells with a plasmid containing either of the genes-COL1A1 or COL5A1 (Figures 4K–M; Supplementary Table S10). Additionally, the immunofluorescence method was used to obtain the same results as WB (Figure 4N). Collectively, these results demonstrated that miR-29b-3p regulated cell migration, invasion, and EMT in PTC cells by targeting COL1A1 and COL5A1.
Downregulating COL1A1 or COL5A1 Expression Can Inhibit Migration, Invasion, and EMT in PTC Cells
To further verify the involvement of COL1A1 and COL5A1 in migration and invasion of PTC cells, siRNA knockdown was performed. The efficiency of siRNA knockdown was verified by qRT-PCR and Western blot. According to our results, si-COL1A1–2 and si-COL5A1–1 demonstrated the most significant knockdown efficiency, which in turn showed a capacity to reduce the expression of COL1A1 and COL5A1 mRNA and protein by 60%–80% (Figures 5A–C; Supplementary Table S7) and so was used in the subsequent experiments. Western blot quantification are shown in Figures 5D, E. Wound healing and Transwell assays confirmed that silencing of COL1A1 or COL5A1 markedly inhibited the invasive capacity of K1 and B-CPAP (Figures 5F, G). The statistical analyses of the results mentioned above are represented in Figures 5H–J and Supplementary Tables S11 and S12. In addition, the expression of EMT-related proteins was detected by Western blot. After the analysis, it was observed that after transfection with si-COL1A1–2 or si-COL5A1–1, the protein expression of E-cadherin was upregulated, whereas the protein expressions of Snail, Vimentin, and N-cadherin were significantly downregulated (Figure 5K). These data were also confirmed through immunofluorescence analysis (Figure 5L). Quantification of WB analysis is shown in Figures 5M, N and Supplementary Table S13.
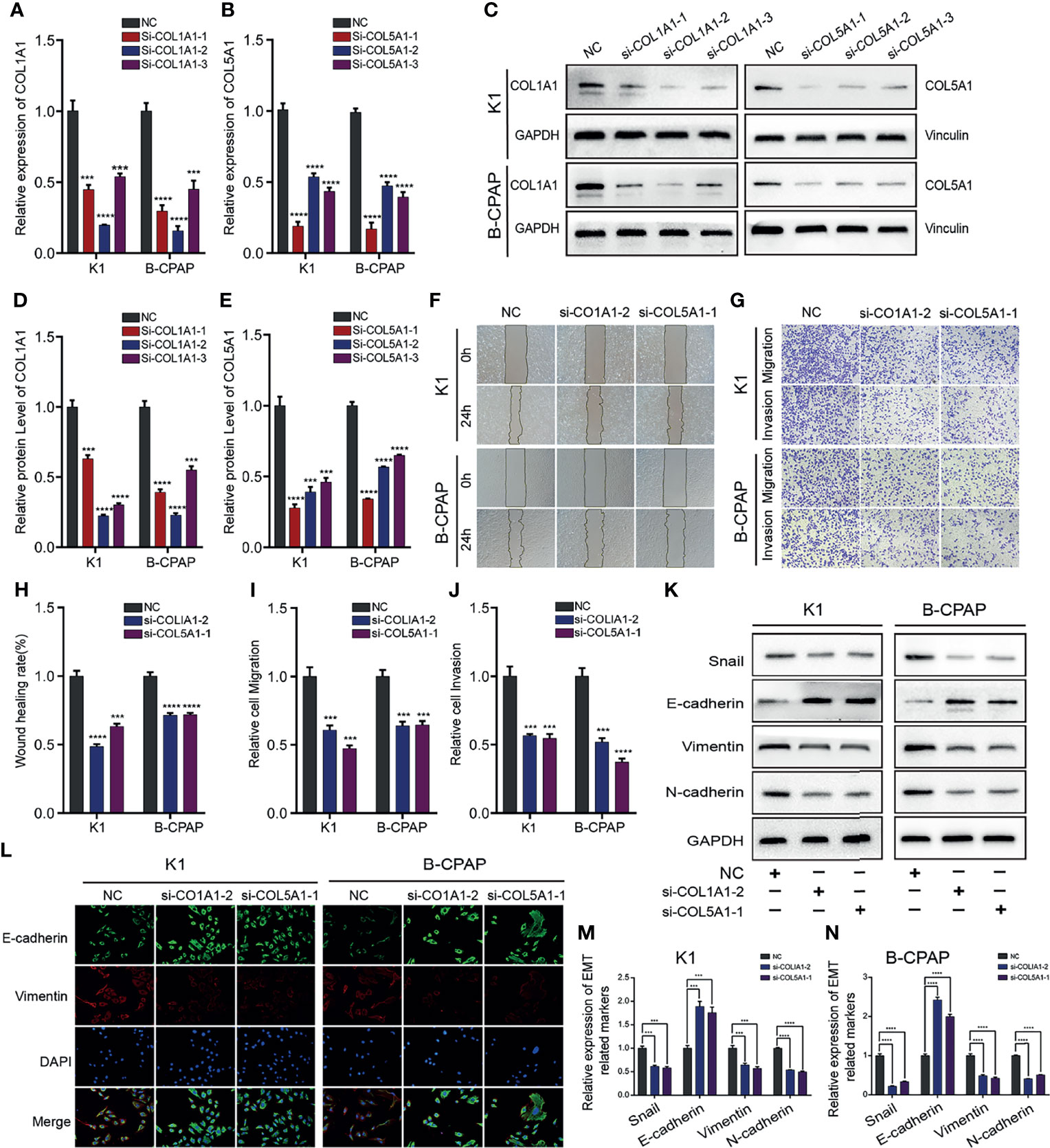
Figure 5 Downregulating COL1A1 or COL5A1 expression can inhibit migration, invasion, and EMT in PTC cells. The knockdown efficiency of COL1A1 and COL5A1 were analyzed by (A, B) qRT-PCR and (C–E) and Western blotting. Additionally, (F) wound healing and (G) Transwell assays were conducted to evaluate PTC cells migration and invasion, which proved that both si-COL1A1–2 and si-COL5A1–1 significantly impaired migration and invasion ability in K1 and B-CPAP cells. (H–J) The statistical results of wound healing assays and Transwell assays. (K) Western blot and (L) immunofluorescence analyses were performed to determine the expression of EMT-related proteins detection in PTC cells transfected with si-COL1A1–2 and si-COL5A1–1. (M, N) Western blotting assay showing the protein expression levels of EMT-related in PTC cells. In the figure, ***p < 0.001, ****p < 0.0001.
miR-29b-3p Inhibits PTC Cell Growth and Decreases the Expression of COL1A1 and COL5A1 In Vivo
A tumor formation assay was performed in nude mice to confirm the effects of miR-29b-3p on the tumorigenesis of PTC. We successfully established a subcutaneously implanted tumor model of PTC in nude mice by subcutaneous injection of K1 cells. After 3 weeks’ growth, miR-29b-3p agomir or control agomir were intratumorally injected every 3 days for 12 days, and tumor growth was monitored (Figure 6A). According to the curves of tumor growth, an injection of the miR-29b-3p agomir significantly inhibited tumor growth compared to the control group (Figure 6B; Supplementary Table S14). As shown in Figures 6C, D, 33 days after tumor xenografts, tumors were excised from the nude mice and weighed, and tumors in the miR-29b-3p agomir group were lighter than the control agomir group. Furthermore, immunohistochemical analysis of COL1A1 and COL5A1 expression was performed using the xenograft tumors from miR-29b-3p agomir or control agomir-treated mice. It was revealed that the protein expression levels of COL1A1 and COL5A1 were significantly decreased in the miR-29b-3p agomir group as compared with that of the control agomir group (Figure 6E). The results of our in vivo experiments established a negative correlation between miR-29b-3p and COL1A1 and between miR-29b-3p and COL5A1.
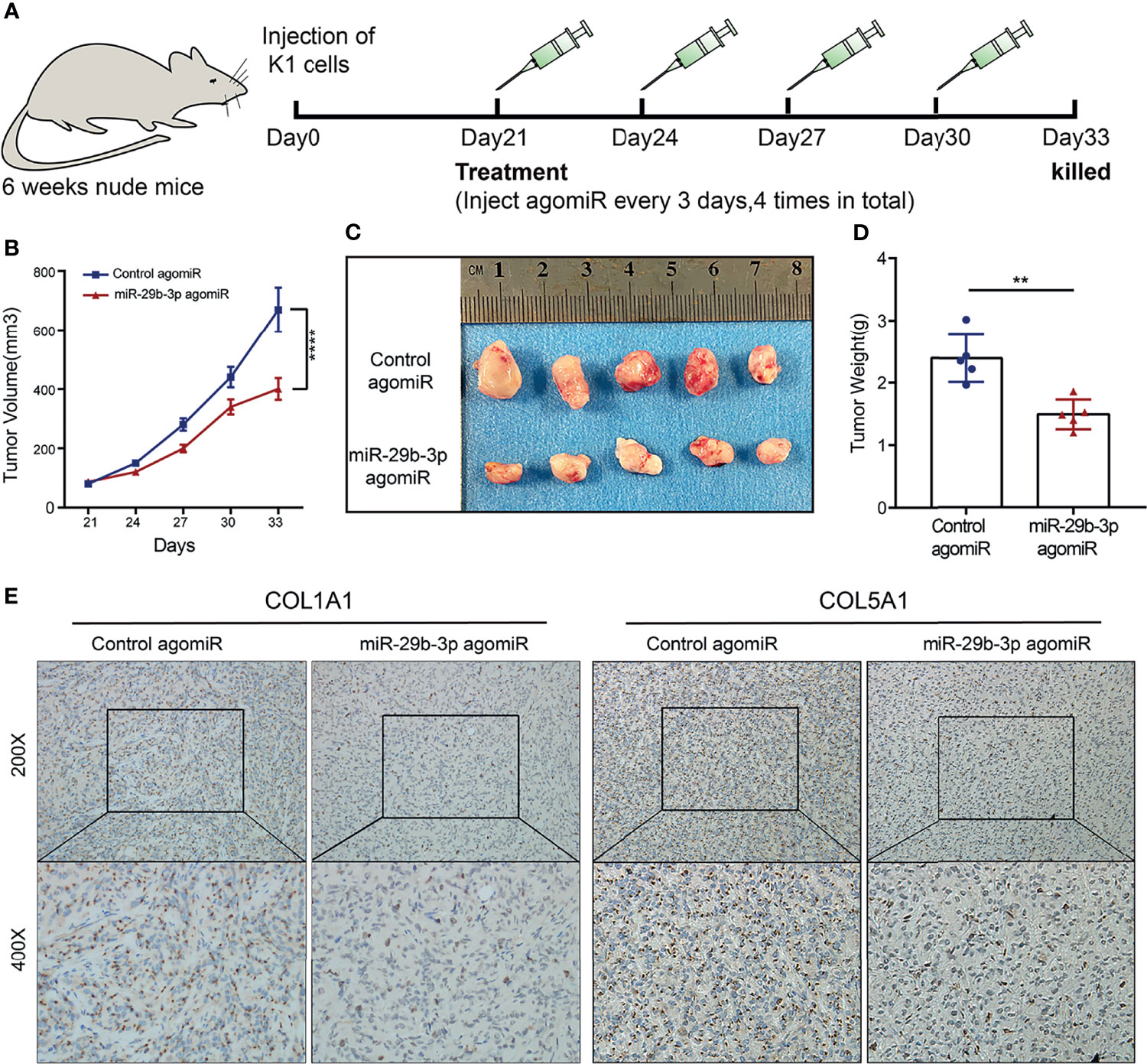
Figure 6 miR-29b-3p inhibits PTC cell growth and decreased COL1A1 and COL5A1 expression in vivo. (A) Schematic diagram of the in vivo experimental process. (B) Tumor growth curves of control agomiR (n = 5) and miR-29b-3p agomiR (n = 5) in nude mice. (C) Gross pathology of tumors at post-transplanted day 33. (D) Statistical analysis of the mean tumor weight of tumor xenografts from nude mice after 33 days of treatment. (E) Immunohistochemistry analysis of protein expression levels of COL1A1 and COL5A1 in tumor xenografts treated with miR-29b-3p agomiR and control agomiR. In the figure, **p < 0.01, ****p < 0.0001.
Discussion
It is critical to explore molecular biomarkers of invasion and proliferation for prognosis and for carrying out personalized treatment regimens of tumor (23–27). MiRNA is a component of the gene regulation network, which regulates 30% human genes at the post-transcription level and participates in the occurrence of a range of diseases (28). MiRNA dysregulation has been identified as a hallmark of human malignancies (10, 29) and may be correlated with clinicopathological characteristics and disease activity of cancer (30–32). Several studies have demonstrated that miRNAs play an important role in the metastasis and progression of thyroid cancer (33–39). In the present study, we showed for the first time that miR-29b-3p expression was downregulated in PTC tissues as compared to normal tissues and was significantly negatively correlated in terms of tumor size, local lymph node metastasis, and TNM stage in patients with PTC.
To further explore the biological function of miR-29b-3p in PTC, we conducted experiments in vivo and in vitro. Further functional analysis revealed that the overexpression of miR-29b-3p could prominently constrain cellular proliferative, migratory, and invasive abilities of PTC cells. Furthermore, in vivo experiments showed that miR-29b-3p could inhibit in vivo growth of PTC cells in nude mice. This result is also found in other tumors in the study. For instance, miR-29b-3p functioned as an anti-cancer agent in glioblastoma tumors (40). Zhao et al. suggested that miR-29b-3p might inhibit pancreatic ductal adenocarcinoma cell migration, invasion, and endothelial dysfunction tube formation through inhibiting the expression of VGEFA, a well-known pro-cancerous gene (41). Subsequent studies supported that miR-29b-3p could suppress the growth of multiple myeloma and ovarian cancer cells (42, 43) along with the migratory and invasive abilities of liver carcinoma and esophageal cancer cells (44, 45) and the progression of bladder cancer by inhibiting the proliferative, migratory, and invasive abilities of oncocytes (46). These discoveries indicate the common essential function of miR-29b-3p in tumor metastasis and proliferation. The anticancer properties of miR-29b-3p have attracted growing interest, which has prompted us to further explore the downstream target of miR-29b-3p.
MiRNAs regulate gene expression by combining with the mRNA 3′-UTR, leading to target mRNA translation inhibition or cleavage. To explore the biological functions of miR-29b-3p, we found, by online bioinformatic tools, that six collagen genes were the potential target genes of miR-29b-3p. Collagens are extracellular macromolecules that are major structural proteins of the ECM. ECM is a major component of the cell microenvironment and provides support for surrounding cells (47). It plays an essential role in regulating cell proliferation, migration, morphology, function, and development (48). ECM remodeling is a critical biological process for the adhesion, motility, and migration of tumor cells (49, 50). Studies have suggested that ECM participates in tumorigenesis through cellular migration, growth, and EMT (51, 52). It is noteworthy that collagen-containing fibers can promote the migratory ability of oncocytes, and their proliferation and migration can be fostered by increasing the collagen density (53). Therefore, increasing evidence confirms that collagen is an essential regulatory protein that controls tumor infiltration, angiogenesis, invasion, and migration (54–57). Moreover, collagens have also been demonstrated to be involved in the initiation and progression of thyroid cancer (58–60).
In our study, the data from qRT-PCR, WB, and dual luciferase reporter assay solidly confirmed that miR-29b-3p directly targeted COL1A1 and COL5A1 3′‐UTR and thus caused degradation of type I and V collagens, which could explain the downregulation of miR-29b-3p in clinical tumor tissues. Our findings showed that COL1A1 and COL5A1 are highly expressed at both mRNA and protein levels in PTC tissues. Type I collagen is an important constituent of ECM and is the most abundant member of the collagen family. It has also been confirmed that COL1A1 can hasten the malignant phenotype and progression of various malignancies, such as gastric cancer, prostate carcinoma, mammary carcinoma, pancreatic ductal carcinoma, liver carcinoma, and lung carcinoma (61–67). A substantial number of studies have verified that COL5A1 is an essential factor for the metastasis of cells in gastric cancer (68), which may also be a new prognostic factor with respect to lung adenocarcinoma, breast cancer, tongue squamous cell carcinoma, and other tumors (69–71). High expression of COL5A1 can also accelerate the growth and progression of renal cell carcinoma (72). The functional analysis unveiled that both COL1A1 and COL5A1 facilitated the migratory and invasive abilities of PTC cells, and their overexpression could reverse the inhibitory roles of miR-29b-3p in the malignant behavior of PTC cells. We have further demonstrated that COL1A1 and COL5A1 trigger the EMT process and therefore participate in the maintenance of the aggressive phenotype and migratory phenotype of PTC cells. Nevertheless, in contrast to the controls, the overexpression of COL1A1 or COL5A1 did not markedly change the proliferative ability of PTC cells. Our study is the first to prove that both COL1A1 and COL5A1 enhance the invasion, migration, and EMT of PTC cells. Nevertheless, more evidence is essential to explain the pathways of these target genes participating in the migratory and invasive activities of PTC cells.
The above indicates that miR-29b-3p participates in the development of PTC by the remodeling of the ECM, which provides a new theoretical basis for future studies to PTC tumor microenvironment and its progression. However, several deficiencies exist in this study, as we observed a consistent relationship between the COL1A1 and COL5A1, while no regulatory relationship between them at the transcriptional level was shown. These results reflected that COL1A1 and COL5A1 were mainly regulated at the protein–protein level, not at the transcriptional level. However, due to the limitations in experimental conditions, the relationship of protein–protein interaction could not be investigated in this study. In addition, the ideal tumor metastatic models of PTC were not successfully established through injecting K1or B-CPAP cells into the abdomen or tail vein in this study.
In conclusion, we identified that miR-29b-3p is significantly downregulated in PTC, being correlated with cell proliferation, migration, and invasion. The mechanistic investigation revealed that miR-29b-3p directly targeted COL1A1 and COL5A1, thereby suppressing ECM remodeling, which is crucial for tumor invasion and metastasis. This study also generates new ideas for risk assessment and miRNA replacement therapy in PTC.
Data Availability Statement
The datasets presented in this study can be found in online repositories. The names of the repository/repositories and accession number(s) can be found in the article/Supplementary Material.
Ethics Statement
The studies involving human participants were reviewed and approved by the Bioethics Committee of First Affiliated Hospital of Guangxi Medical University [No. 2015(KY-E-018)]. The patients/participants provided their written informed consent to participate in this study. The animal study was reviewed and approved by the Animal Ethics Committee of Guangxi Medical University (No. 202010015).
Author Contributions
CW, YW, and JC contributed to the study conception and design. Material preparation, data collection, and analysis were performed by WH, ZY, SZ, JW, ZF, KZ, and SL. The first draft of the manuscript was written by CW. JC contributed to checking of this manuscript. All authors contributed to the article and approved the submitted version.
Funding
This work was supported by the National Natural Science Foundation of China (grant no. 82060430), the Guangxi Science and Technology Project (grant no. AD19245196), the Guangxi Key Research and Development Project (grant no. AB18126058), and the Guangxi Scientific Research and Technology Development Project (grant no. 1598011-4).
Conflict of Interest
The authors declare that the research was conducted in the absence of any commercial or financial relationships that could be construed as a potential conflict of interest.
Publisher’s Note
All claims expressed in this article are solely those of the authors and do not necessarily represent those of their affiliated organizations, or those of the publisher, the editors and the reviewers. Any product that may be evaluated in this article, or claim that may be made by its manufacturer, is not guaranteed or endorsed by the publisher.
Acknowledgments
We thank TCGA database and GEO database for providing the original study data of PTC patients.
Supplementary Material
The Supplementary Material for this article can be found online at: https://www.frontiersin.org/articles/10.3389/fonc.2022.837581/full#supplementary-material
Abbreviation
miRNAs, microRNAs; PTC, papillary thyroid carcinoma; qRT-PCR, quantitative reverse transcription polymerase chain reaction; EMT, epithelial–mesenchymal transition; ECM, extracellular matrix; DMEM, Dulbecco’s modified Eagle’s medium; FBS, fetal bovine serum; CCK-8, Cell Counting Kit-8; TBST, Tris-buffered saline with Tween 20; DAPI, 2-(4-Amidinophenyl)-6-indolecarbamidine dihydrochloride; IHC, immunohistochemistry; EdU, 5-ethynyl-2′-deoxyuridine; BSA, bovine serum albumin; WB, Western blot.
References
1. Siegel R, Miller K, Fuchs H, Jemal A. Cancer Statistics, 2021. CA: Cancer J Clin (2021) 71:7–33. doi: 10.3322/caac.21654
2. Mao Y, Xing M. Recent Incidences and Differential Trends of Thyroid Cancer in the USA. Endocr Relat Cancer (2016) 23:313–22. doi: 10.1530/erc-15-0445
3. LiVolsi V. Papillary Thyroid Carcinoma: An Update. Mod Pathol (2011) 24:S1–9. doi: 10.1038/modpathol.2010.129
4. Na K, Choi H. Immune Landscape of Papillary Thyroid Cancer and Immunotherapeutic Implications. Endocr Relat Cancer (2018) 25:523–31. doi: 10.1530/erc-17-0532
5. Hartl D, Travagli J. The Updated American Thyroid Association Guidelines for Management of Thyroid Nodules and Differentiated Thyroid Cancer: A Surgical Perspective. Thyroid (2009) 19:1149–51. doi: 10.1089/thy.2009.1600
6. Roman B, Brito J, Saucke M, Lohia S, Jensen C, Zaborek N, et al. National Survey of Endocrinol Ogists and Surgeons Regarding Active Surveillance for Low-Risk Papillary Thyroid Cancer. Endocr Pract (2021) 27:1–7. doi: 10.1016/j.eprac.2020.11.003
7. Lee S, Roh J, Gong G, Cho K, Choi S, Nam S, et al. Risk Factors for Recurrence After Treatment of N1b Papillary Thyroid Carcinoma. Ann Surg (2019) 269:966–71. doi: 10.1097/sla.0000000000002710
8. Haugen B, Alexander E, Bible K, Doherty G, Mandel S, Nikiforov Y, et al. 2015 American Thyroid Association Management Guidelines for Adult Patients With Thyroid Nodules and Differentiated Thyroid Cancer: The American Thyroid Association Guidelines Task Force on Thyroid Nodules and Differentiated Thyroid Cancer. Thyroid (2016) 26:1–133. doi: 10.1089/thy.2015.0020
9. Chen K, Rajewsky N. The Evolution of Gene Regulation by Transcription Factors and microRNAs. Nat Rev Genet (2007) 8:93–103. doi: 10.1038/nrg1990
10. Iorio M, Croce C. Causes and Consequences of microRNA Dysregulation. Cancer J (Sudbury Mass) (2012) 18:215–22. doi: 10.1097/PPO.0b013e318250c001
11. Rupaimoole R, Slack F. MicroRNA Therapeutics: Towards a New Era for the Management of Cancer and Other Diseases. Nat Rev Drug Discov (2017) 16:203–22. doi: 10.1038/nrd.2016.246
12. Kasinski A, Slack F. Epigenetics and Genetics. MicroRNAs En Route to the Clinic: Progress in Validating and Targeting microRNAs for Cancer Therapy. Nat Rev Cancer (2011) 11:849–64. doi: 10.1038/nrc3166
13. Hao C, Xu C, Zhao X, Luo J, Wang G, Zhao L, et al. Up-Regulation of VANGL1 by IGF2BPs and miR-29b-3p Attenuates the Detrimental Effect of Irradiation on Lung Adenocarcinoma. J Exp Clin Cancer Res CR (2020) 39:256. doi: 10.1186/s13046-020-01772-y
14. Worst T, Previti C, Nitschke K, Diessl N, Gross J, Hoffmann L, et al. miR-10a-5p and miR-29b-3p as Extracellular Vesicle-Associated Prostate Cancer Detection Markers. Cancers (2019) 12:43. doi: 10.3390/cancers12010043
15. Hozaka Y, Seki N, Tanaka T, Asai S, Moriya S, Idichi T, et al. miR-29-3pmolecular Pathogenesis and Regulation of the -Family: Involvement of and in Intra-Hepatic Cholangiocarcinoma. Cancers (2021) 13:2804. doi: 10.3390/cancers13112804
16. Sun Y, Wang P, Yang W, Shan Y, Zhang Q, Wu H. The Role of lncRNA MSC-AS1/miR-29b-3p Axis-Mediated CDK14 Modulation in Pancreatic Cancer Proliferation and Gemcitabine-Induced Apoptosis. Cancer Biol Ther (2019) 20:729–39. doi: 10.1080/15384047.2018.1529121
17. Lv M, Zhong Z, Huang M, Tian Q, Jiang R, Chen J. lncRNA H19 Regulates Epithelial-Mesenchymal Transition and Metastasis of Bladder Cancer by miR-29b-3p as Competing Endogenous RNA. Biochim Biophys Acta Mol Cell Res (2017) 1864:1887–99. doi: 10.1016/j.bbamcr.2017.08.001
18. Karamanos N, Piperigkou Z, Passi A, Götte M, Rousselle P, Vlodavsky I. Extracellular Matrix-Based Cancer Targeting. Trends Mol Med (2021) 10:1000–13. doi: 10.1016/j.molmed.2021.07.009
19. Arseni L, Lombardi A, Orioli D. From Structure to Phenotype: Impact of Collagen Alterations on Human Health. Int J Mol Sci (2018) 19:1407. doi: 10.3390/ijms19051407
20. Tao R, Fan X, Yu H, Ai G, Zhang H, Kong H, et al. MicroRNA-29b-3p Prevents Schistosoma Japonicum-Induced Liver Fibrosis by Targeting COL1A1 and COL3A1. J Cell Biochem (2018) 119:3199–209. doi: 10.1002/jcb.26475
21. Zhao B, Song X, Guan H. CircACAP2 Promotes Breast Cancer Proliferation and Metastasis by Targeting miR-29a/B-3p-COL5A1 Axis. Life Sci (2020) 244:117179. doi: 10.1016/j.lfs.2019.117179
22. Cao W, Feng Y. LncRNA XIST Promotes Extracellular Matrix Synthesis, Proliferation and Migration by Targeting miR-29b-3p/COL1A1 in Human Skin Fibroblasts After Thermal Injury. Biol Res (2019) 52:52. doi: 10.1186/s40659-019-0260-5
23. Marcu L. Imaging Biomarkers of Tumour Proliferation and Invasion for Personalised Lung Cancer Therapy. J Pers Med (2020) 10:222. doi: 10.3390/jpm10040222
24. Jiang C, Li X, Zhao H, Liu H. Long Non-Coding RNAs: Potential New Biomarkers for Predicting Tumor Invasion and Metastasis. Mol Cancer (2016) 15:62. doi: 10.1186/s12943-016-0545-z
25. Awasthee N, Rai V, Chava S, Nallasamy P, Kunnumakkara A, Bishayee A, et al. Targeting Iκappab Kinases for Cancer Therapy. Semin Cancer Biol (2019) 56:12–24. doi: 10.1016/j.semcancer.2018.02.007
26. Sharma G, Okada Y, Von Hoff D, Goel A. Non-Coding RNA Biomarkers in Pancreatic Ductal Adenocarcinoma. Semin Cancer Biol (2020) 75:153–68. doi: 10.1016/j.semcancer.2020.10.001
27. Yu L, Xu J, Liu J, Zhang H, Sun C, Wang Q, et al. The Novel Chromatin Architectural Regulator SND1 Promotes Glioma Proliferation and Invasion and Predicts the Prognosis of Patients. Neuro-Oncology (2019) 21:742–54. doi: 10.1093/neuonc/noz038
28. Tonevitsky A, Maltseva D, Abbasi A, Samatov T, Sakharov D, Shkurnikov M, et al. Dynamically Regulated miRNA-mRNA Networks Revealed by Exercise. BMC Physiol (2013) 13:9. doi: 10.1186/1472-6793-13-9
29. Croce C. Causes and Consequences of microRNA Dysregulation in Cancer. Nat Rev Genet (2009) 10:704–14. doi: 10.1038/nrg2634
30. Cui X, Liu Y, Sun W, Ding J, Bo X, Wang H. Comprehensive Analysis of miRNA-Gene Regulatory Network With Clinical Significance in Human Cancers. Sci China Life Sci (2020) 63:1201–12. doi: 10.1007/s11427-019-9667-0
31. Fortis S, Vaxevanis C, Mahaira L, Sofopoulos M, Sotiriadou N, Dinou A, et al. Serum miRNA-Based Distinct Clusters Define Three Groups of Breast Cancer Patients With Different Clinicopathological and Immune Characteristics. Cancer Immunol Immunother CII (2019) 68:57–70. doi: 10.1007/s00262-018-2252-7
32. Chen F, Li X, Fu D, Huang J, Yang S. Clinical Potential of miRNA-221 as a Novel Prognostic Biomarker for Hepatocellular Carcinoma. Cancer Biomarkers Section A Dis Markers (2017) 18:209–14. doi: 10.3233/cbm-161671
33. Lee J, Gundara J, Glover A, Serpell J, Sidhu S. MicroRNA Expression Profiles in the Management of Papillary Thyroid Cancer. Oncol (2014) 19:1141–7. doi: 10.1634/theoncologist.2014-0135
34. Aragon Han P, Weng C, Khawaja H, Nagarajan N, Schneider E, Umbricht C, et al. MicroRNA Expression and Association With Clinicopathologic Features in Papillary Thyroid Cancer: A Systematic Review. Thyroid (2015) 25:1322–9. doi: 10.1089/thy.2015.0193
35. Cahill S, Smyth P, Denning K, Flavin R, Li J, Potratz A, et al. Effect of BRAFV600E Mutation on Transcription and Post-Transcriptional Regulation in a Papillary Thyroid Carcinoma Model. Mol Cancer (2007) 6:21. doi: 10.1186/1476-4598-6-21
36. Jazdzewski K, Boguslawska J, Jendrzejewski J, Liyanarachchi S, Pachucki J, Wardyn K, et al. Thyroid Hormone Receptor Beta (THRB) Is a Major Target Gene for microRNAs Deregulated in Papillary Thyroid Carcinoma (PTC). J Clin Endocrinol Metab (2011) 96:E546–53. doi: 10.1210/jc.2010-1594
37. Laukiene R, Jakubkevicius V, Ambrozaityte L, Cimbalistiene L, Utkus A. Dysregulation of microRNAs as the Risk Factor of Lymph Node Metastasis in Papillary Thyroid Carcinoma: Systematic Review. Endokrynol Pol (2021) 72:145–52. doi: 10.5603/EP.a2021.0010
38. Knyazeva M, Korobkina E, Karizky A, Sorokin M, Buzdin A, Vorobyev S, et al. Reciprocal Dysregulation of MiR-146b and MiR-451 Contributes in Malignant Phenotype of Follicular Thyroid Tumor. Int J Mol Sci (2020) 21:5950. doi: 10.3390/ijms21175950
39. Pishkari S, Paryan M, Hashemi M, Baldini E, Mohammadi-Yeganeh S. The Role of microRNAs in Different Types of Thyroid Carcinoma: A Comprehensive Analysis to Find New miRNA Supplementary Therapies. J Endocrinol Invest (2018) 41:269–83. doi: 10.1007/s40618-017-0735-6
40. Shin J, Shim H, Hwang T, Kim H, Kang S, Dho Y, et al. Restoration of miR-29b Exerts Anti-Cancer Effects on Glioblastoma. Cancer Cell Int (2017) 17:104. doi: 10.1186/s12935-017-0476-9
41. Zhao X, Liu Y, Li Z, Zheng S, Wang Z, Li W, et al. Linc00511 Acts as a Competing Endogenous RNA to Regulate VEGFA Expression Through Sponging hsa-miR-29b-3p in Pancreatic Ductal Adenocarcinoma. J Cell Mol Med (2018) 22:655–67. doi: 10.1111/jcmm.13351
42. Liu D, Wang J, Liu M. Long Noncoding RNA TUG1 Promotes Proliferation and Inhibits Apoptosis in Multiple Myeloma by Inhibiting miR-29b-3p. Biosci Rep (2019) 39:2489. doi: 10.1042/bsr20182489
43. Yang X, Xin N, Qu H, Wei L, Han Z. Long Noncoding RNA TUG1 Facilitates Cell Ovarian Cancer Progression Through Targeting MiR-29b-3p/MDM2 Axis. Anat Rec (Hoboken NJ 2007) (2020) 303:3024–34. doi: 10.1002/ar.24367
44. Zhou Y, Li K, Dai T, Wang H, Hua Z, Bian W, et al. Long non-Coding RNA HCP5 Functions as a Sponge of miR-29b-3p and Promotes Cell Growth and Metastasis in Hepatocellular Carcinoma Through Upregulating DNMT3A. Aging (2021) 13:16267–86. doi: 10.18632/aging.203155
45. Zhao W, Huang Z, Liu H, Wang C. LncRNA GIHCG Promotes the Development of Esophageal Cancer by Modulating miR-29b-3p/ANO1 Axis. OncoTargets Ther (2020) 13:13387–400. doi: 10.2147/ott.S282348
46. Zhao C, Li Y, Hu X, Wang R, He W, Wang L, et al. LncRNA HCP5 Promotes Cell Invasion and Migration by Sponging miR-29b-3p in Human Bladder Cancer. OncoTargets Ther (2020) 13:11827–38. doi: 10.2147/ott.S249770
47. Huang G, Li F, Zhao X, Ma Y, Li Y, Lin M, et al. Functional and Biomimetic Materials for Engineering of the Three-Dimensional Cell Microenvironment. Chem Rev (2017) 117:12764–850. doi: 10.1021/acs.chemrev.7b00094
48. Chen P, Miao Y, Zhang F, Huang J, Chen Y, Fan Z, et al. Nanoscale Microenvironment Engineering Based on Layer-by-Layer Self-Assembly to Regulate Hair Follicle Stem Cell Fate for Regenerative Medicine. Theranostics (2020) 10:11673–89. doi: 10.7150/thno.48723
49. Marques-Magalhães Â, Cruz T, Costa Â, Estêvão D, Rios E, Canão P, et al. Decellularized Colorectal Cancer Matrices as Bioactive Scaffolds for Studying Tumor-Stroma Interactions. Cancers (2022) 14:359. doi: 10.3390/cancers14020359
50. Kang J, La Manna F, Bonollo F, Sampson N, Alberts I, Mingels C, et al. Tumor Microenvironment Mechanisms and Bone Metastatic Disease Progression of Prostate Cancer. Cancer Lett (2022) 530:156–69. doi: 10.1016/j.canlet.2022.01.015
51. Ros M, Sala M, Saltel F. Linking Matrix Rigidity With EMT and Cancer Invasion. Dev Cell (2020) 54:293–95. doi: 10.1016/j.devcel.2020.06.032
52. Jiang J, Wang K, Chen Y, Chen H, Nice E, Huang C. Redox Regulation in Tumor Cell Epithelial-Mesenchymal Transition: Molecular Basis and Therapeutic Strategy. Signal Transduct Target Ther (2017) 2:17036. doi: 10.1038/sigtrans.2017.36
53. Nissen N, Karsdal M, Willumsen N. Collagens and Cancer Associated Fibroblasts in the Reactive Stroma and Its Relation to Cancer Biology. J Exp Clin Cancer Res CR (2019) 38:115. doi: 10.1186/s13046-019-1110-6
54. Graf F, Horn P, Ho A, Boutros M, Maercker C. The Extracellular Matrix Proteins Type I Collagen, Type III Collagen, Fibronectin, and Laminin 421 Stimulate Migration of Cancer Cells. FASEB J (2021) 35:e21692. doi: 10.1096/fj.202002558RR
55. Peng D, Ungewiss C, Tong P, Byers L, Wang J, Canales J, et al. ZEB1 Induces LOXL2-Mediated Collagen Stabilization and Deposition in the Extracellular Matrix to Drive Lung Cancer Invasion and Metastasis. Oncogene (2017) 36:1925–38. doi: 10.1038/onc.2016.358
56. Ray A, Provenzano P. Aligned Forces: Origins and Mechanisms of Cancer Dissemination Guided by Extracellular Matrix Architecture. Curr Opin Cell Biol (2021) 72:63–71. doi: 10.1016/j.ceb.2021.05.004
57. Kim M, Ha S, Wu M, Zogg H, Ronkon C, Lee M, et al. Extracellular Matrix Biomarkers in Colorectal Cancer. Int J Mol Sci (2021) 22:9185. doi: 10.3390/ijms22179185
58. Geng H, Guo M, Xu W, Zang X, Wu T, Teng F, et al. SHCBP1 Promotes Papillary Thyroid Carcinoma Carcinogenesis and Progression Through Promoting Formation of Integrin and Collagen and Maintaining Cell Stemness. Front Endocrinol (2020) 11:613879. doi: 10.3389/fendo.2020.613879
59. Yoshida T, Suganuma N, Sato S, Toda S, Nakayama H, Masudo K, et al. Membrane Type 1 Matrix Metalloproteinase Regulates Anaplastic Thyroid Carcinoma Cell Growth and Invasion Into the Collagen Matrix. Biochem Biophys Res Commun (2020) 529:1195–200. doi: 10.1016/j.bbrc.2020.06.043
60. Parascandolo A, Rappa F, Cappello F, Kim J, Cantu D, Chen H, et al. Extracellular Superoxide Dismutase Expression in Papillary Thyroid Cancer Mesenchymal Stem/Stromal Cells Modulates Cancer Cell Growth and Migration. Sci Rep (2017) 7:41416. doi: 10.1038/srep41416
61. Shi Y, Duan Z, Zhang X, Zhang X, Wang G, Li F. Down-Regulation of the Let-7i Facilitates Gastric Cancer Invasion and Metastasis by Targeting COL1A1. Protein Cell (2019) 10:143–48. doi: 10.1007/s13238-018-0550-7
62. Yu L, Sui B, Fan W, Lei L, Zhou L, Yang L, et al. Exosomes Derived From Osteogenic Tumor Activate Osteoclast Differentiation and Concurrently Inhibit Osteogenesis by Transferring COL1A1-Targeting miRNA-92a-1-5p. J Extracell Vesicles (2021) 10:e12056. doi: 10.1002/jev2.12056
63. Rennhack J, To B, Swiatnicki M, Dulak C, Ogrodzinski M, Zhang Y, et al. Integrated Analyses of Murine Breast Cancer Models Reveal Critical Parallels With Human Disease. Nat Commun (2019) 10:3261. doi: 10.1038/s41467-019-11236-3
64. Tian C, Huang Y, Clauser K, Rickelt S, Lau A, Carr S, et al. Suppression of Pancreatic Ductal Adenocarcinoma Growth and Metastasis by Fibrillar Collagens Produced Selectively by Tumor Cells. Nat Commun (2021) 12:2328. doi: 10.1038/s41467-021-22490-9
65. Chakravarthy D, Muñoz A, Su A, Hwang R, Keppler B, Chan D, et al. Palmatine Suppresses Glutamine-Mediated Interaction Between Pancreatic Cancer and Stellate Cells Through Simultaneous Inhibition of Survivin and COL1A1. Cancer Lett (2018) 419:103–15. doi: 10.1016/j.canlet.2018.01.057
66. Ma H, Chang H, Bamodu O, Yadav V, Huang T, Wu A, et al. Collagen 1a1 (COL1A1) Is a Reliable Biomarker and Putative Therapeutic Target for Hepatocellular Carcinogenesis and Metastasis. Cancers (2019) 11:786. doi: 10.3390/cancers11060786
67. Geng Q, Shen Z, Li L, Zhao J. COL1A1 Is a Prognostic Biomarker and Correlated With Immune Infiltrates in Lung Cancer. PeerJ (2021) 9:e11145. doi: 10.7717/peerj.11145
68. Zhang Y, Jing Y, Wang Y, Tang J, Zhu X, Jin W, et al. NAT10 Promotes Gastric Cancer Metastasis via N4-Acetylated COL5A1. Signal Transduct Target Ther (2021) 6:173. doi: 10.1038/s41392-021-00489-4
69. Liu W, Wei H, Gao Z, Chen G, Liu Y, Gao X, et al. COL5A1 May Contribute the Metastasis of Lung Adenocarcinoma. Gene (2018) 665:57–66. doi: 10.1016/j.gene.2018.04.066
70. Wu M, Sun Q, Mo C, Pang J, Hou J, Pang L, et al. Prospective Molecular Mechanism of COL5A1 in Breast Cancer Based on a Microarray, RNA Sequencing and Immunohistochemistry. Oncol Rep (2019) 42:151–75. doi: 10.3892/or.2019.7147
71. Chen H, Tseng Y, Shu C, Weng T, Liou H, Yen L, et al. Differential Clinical Significance of COL5A1 and COL5A2 in Tongue Squamous Cell Carcinoma. J Oral Pathol Med (2019) 48:468–76. doi: 10.1111/jop.12861
Keywords: microRNAs, miR-29b-3p, papillary thyroid carcinoma, downregulation, COL1A1, COL5A1, gene expression
Citation: Wang C, Wang Y, Fu Z, Huang W, Yu Z, Wang J, Zheng K, Zhang S, Li S and Chen J (2022) MiR-29b-3p Inhibits Migration and Invasion of Papillary Thyroid Carcinoma by Downregulating COL1A1 and COL5A1. Front. Oncol. 12:837581. doi: 10.3389/fonc.2022.837581
Received: 16 December 2021; Accepted: 16 March 2022;
Published: 22 April 2022.
Edited by:
Carlos Pérez-Plasencia, National Autonomous University of Mexico, MexicoReviewed by:
Demitrios Vynios, University of Patras, GreeceJossimar Coronel Hernandez, National Institute of Cancerology (INCAN), Mexico
Copyright © 2022 Wang, Wang, Fu, Huang, Yu, Wang, Zheng, Zhang, Li and Chen. This is an open-access article distributed under the terms of the Creative Commons Attribution License (CC BY). The use, distribution or reproduction in other forums is permitted, provided the original author(s) and the copyright owner(s) are credited and that the original publication in this journal is cited, in accordance with accepted academic practice. No use, distribution or reproduction is permitted which does not comply with these terms.
*Correspondence: Junqiang Chen, Y2hlbmp1bnFpYW5nQGd4bXUuZWR1LmNu
†These authors have contributed equally to this work and share first authorship