- 1Department of Radiation Oncology, Institut Claudius Regaud/Institut Universitaire du Cancer de Toulouse-Oncopole, Toulouse, France
- 2Department of Biostatistics, Institut Claudius Regaud/Institut Universitaire du Cancer de Toulouse-Oncopole, Toulouse, France
- 3Department of Thoracic Oncology, Centre Hospitalier Universitaire de Toulouse, Hôpital Larrey, Toulouse, France
- 4Department of Pulmonology, Centre Hospitalier Intercommunal des Vallées de l’Ariège (CHIVA), Saint-Jean-de-Verges, France
- 5Department of Nuclear Medicine, Institut Claudius Regaud/Institut Universitaire du Cancer de Toulouse-Oncopole, Toulouse, France
- 6Université de Toulouse III Paul Sabatier, Toulouse, France
- 7Institut National de la Santé et de la Recherche Médicale U1037, Centre de Recherche contre le Cancer de Toulouse, Toulouse, France
Background: The optimal modalities of radiotherapy when combining concurrent chemoradiation (CCRT) and immunotherapy (IO) for locally advanced non-small cell lung cancer (LA-NSCLC) remain to be determined. The aim of this study was to investigate the impact of radiation on different immune structures and immune cells in patients treated with CCRT followed by durvalumab.
Material and methods: Clinicopathologic data, pre- and post-treatment blood counts, and dosimetric data were collected in patients treated with CCRT and durvalumab consolidation for LA-NSCLC. Patients were divided into two groups according to the inclusion (NILN-R+) or not (NILN-R−) of at least one non-involved tumor-draining lymph node (NITDLN) in the clinical target volume (CTV). Progression-free survival (PFS) and overall survival (OS) were estimated by the Kaplan–Meier method.
Results: Fifty patients were included with a median follow-up of 23.2 months (95% CI 18.3–35.2). Two-year PFS and 2-year OS were 52.2% (95% CI 35.8–66.3) and 66.2% (95% CI 46.5–80.1), respectively. In univariable analysis, NILN-R+ (hazard ratio (HR) 2.60, p = 0.028), estimated dose of radiation to immune cells (EDRIC) >6.3 Gy (HR 3.19, p = 0.049), and lymphopenia ≤ 500/mm3 at IO initiation (HR 2.69, p = 0.021) were correlated with poorer PFS; lymphopenia ≤ 500/mm3 was also associated with poorer OS (HR 3.46, p = 0.024). In multivariable analysis, NILN-R+ was the strongest factor associated with PFS (HR 3.15, p = 0.017).
Conclusion: The inclusion of at least one NITDLN station within the CTV was an independent factor for poorer PFS in the context of CCRT and durvalumab for LA-NSCLC. The optimal sparing of immune structures might help in achieving better synergy between radiotherapy and immunotherapy in this indication.
Introduction
The most important improvement in patients with unresectable stage III non-small cell lung cancer (NSCLC) was recently obtained by the addition of consolidation immunotherapy (durvalumab) to concurrent chemoradiation (CCRT), which now constitutes the standard of care (1, 2). However, the optimal radiation therapy regimen in the context of immunotherapy remains to be determined.
One of the immunosuppressive effects of radiotherapy is the direct depletion of circulating lymphocytes or progenitors in lymphoid organs (3–5). Before the era of immunotherapy (IO), several studies highlighted the detrimental impact of lymphopenia on patients treated by CCRT for unresectable LA-NSCLC (5–8). Several models have been proposed to estimate the dose delivered to circulating immune cells. Jin et al. developed a three-step model to calculate the estimated dose of radiation to immune cells (EDRIC) during thoracic radiotherapy, assuming the following: a) the dose to circulating immune cells including rapidly circulating ones in the heart, lung, and blood vessels and slowly circulating ones in the lymphatic system and blood reservoirs (a portion of veins/capillaries) is a surrogate for the EDRIC; b) at each fraction, the radiation dose is uniformly delivered to all cells for rapidly circulating ones and only to those in the irradiated volume for slowly circulating cells. In this model, the blood dose relating to the contribution of a given organ is approximated by its mean organ dose, the percentage of cardiac output, the percentage of blood volume it receives, the time for one blood circulation, the irradiation time, and the number of fractions. Second, the equivalent uniform dose (EUD) is determined from a blood dose/volume histogram (percentage of blood volume irradiated at a given dose). Third, the EDRIC is the sum of the EUDs of each organ. In summary, the EDRIC can be approximated as a function of the mean heart dose, the mean lung dose, the mean body dose, and the number of fractions (9). In a secondary analysis of the RTOG 0617 trial, they showed that a higher EDRIC was significantly associated with poorer outcomes (10). The model was adjusted and externally validated in a retrospective cohort of stage III NSCLC following definitive CCRT (11). In addition, EDRIC was negatively associated with lymphocyte and neutrophil counts. More recently, the impact of lymphopenia (12–14) and EDRIC (15) on outcomes has been suggested in the context of durvalumab consolidation.
As the tumor-draining lymph nodes (TDLNs) are the main sites of lymphocyte priming, their sparing in the context of radiotherapy and IO should be addressed. In the context of high dose per fraction, preclinical models have established the deleterious effect of TDLN irradiation on the radiation-induced anti-tumor immune response, whether or not it is associated with immune checkpoint inhibitors (16–18). However, the impact of radiation dose on non-involved tumor-draining lymph nodes (NITDLNs) as well as other “immune” organs at risk (iOARs), such as bone marrow, spleen, and immune cells in the context of conventionally fractionated CCRT and durvalumab consolidation for stage III NSCLC, remains to be established.
Therefore, the aim of this study was to assess the impact of radiation on different immune structures including NITDLNs and iOARs with regard to clinical outcomes in a cohort of patients treated by CCRT followed by durvalumab.
Materials and methods
Study population
Between January 2015 and March 2022, patients with unresectable LA-NSCLC who underwent platinum-based CCRT followed by durvalumab consolidation were retrospectively analyzed through the electronic database of a Comprehensive Cancer Center (xxx). Inclusion criteria were as follows: 1) histologically documented NSCLC; 2) imaging evaluation including at least computed tomography (CT) of the chest, abdomen, and pelvis and/or F-18 fluorodeoxyglucose positron emission tomography/computed tomography (18F FDG-PET/CT) and brain CT or magnetic resonance imaging (MRI); 3) diagnosis of unresectable locally advanced disease; 4) treatment with platinum-based CCRT (at least two cycles concurrent with radiotherapy) and initiation of durvalumab consolidation therapy (10 mg/kg every 2 weeks or 1,500 mg every 4 weeks) if no disease progression after CCRT; 5) complete blood counts accessible at baseline and follow-up. Patients who underwent sequential chemoradiotherapy were excluded.
This study was approved by the institutional review boards of our institution. Patients received a letter detailing the aim of the study and the use of data collection and could refuse inclusion at any time, but informed consent was not necessary because of the retrospective nature of the study.
Data collection
Demographics
Patient characteristics such as age, WHO performance status, clinical staging (TNM), histology, PD-L1 expression, and mutational status were collected. Standardized uptake value (SUV) max and SUV peak were evaluated from 18F FDG-PET/CT of eligible patients by a single physician.
Radiotherapy data
Treatment was delivered by intensity-modulated radiation therapy (IMRT) with volumetric modulated arc therapy (VMAT), and motion was managed by using 4D-CT and motion-adapted gross tumor volume (GTV) in all patients. Target volumes for the whole cohort were based on the European guidelines (19–21). The lung window setting on planning CT scan was used to delineate the GTV of the primary tumor. Depending on the histology, a 5–8-mm expansion was made and edited accounting for the surrounding anatomy to create the clinical target volume (CTV) of the primary tumor. Lymph nodes were included in target volumes in the event of enlarged and/or FDG-avid nodes (21). Nodal CTV was defined as the whole lymph node station(s) of the involved node(s). A 5-mm margin was applied around the CTV to create the planning target volume (PTV) in all patients. Patients were treated with curative intent radiotherapy, most commonly to 66 Gy in 2-Gy fractions, prescribed on the median of the PTV.
NITDLNs were retrospectively segmented in eligible patients by a single physician. Lymph node stations were defined according to the Japan Lung Cancer Society atlas (22). TDLNs were defined according to the topography of the primary tumor (23–25): stations 10/11R, 7, 4R, and 2R for right upper lobe tumors; stations 10/11R, 8, 7, 4R, and 2R for middle lobe or right lower lobe tumors; stations 10/11L, 7, 2L, 4L, 5, and 6 for left upper lobe tumors; stations 10/11L, 7, 2L, 4L, 5, 6, and 8 for left lower lobe tumors.
Patients were divided into two groups: 1) patients with at least one NITDLN station included in the CTV (radiation to non-involved lymph node (NILN-R+)) and 2) patients with no NITDLN station included in the CTV (NILN-R−).
In addition, we retrospectively delineated the thoracic vertebrae from T1 to T12 and the spleen on planning CT scans.
All dosimetric parameters were extracted from dose–volume histograms available in our planning system (Eclipse®, Varian, Palo Alto, CA, USA).
Biological data
White blood cell (WBC) count, absolute lymphocyte count (ALC), absolute neutrophil count (ANC), and neutrophil-to-lymphocyte ratio (NLR) were collected from complete blood count before treatment, at the end of CCRT and the initiation of consolidative immunotherapy (durvalumab). ALC nadir was also reported. Lymphopenia was graded according to the Common Terminology Criteria for Adverse Events (CTCAE v5.0). A cutoff of 500/mm3 was chosen as clinically relevant in this cohort.
The lymphocyte variation rate (LVR) from baseline to the end of CCRT was calculated according to the following equation:
Calculation of EDRIC
EDRIC was calculated by using dosimetric data including mean heart dose (MHD), mean lung dose (MLD), mean body dose (MBD), and the number of fractions as reported by Ladbury et al. (11):
With the use of data from Jin et al. and Ladbury et al. (10, 11), the 6.3-Gy cutoff was used to split the cohort into two groups.
Outcomes and follow-up
Overall survival (OS) was defined as the time from initiation of durvalumab to death or the last follow-up (censored data). Progression-free survival (PFS) was defined as the time from initiation of durvalumab to progression or death. Patients still alive and without recurrence were censored at the last follow-up. Time to local recurrence (TLR) was defined as the time from initiation of durvalumab to local recurrence. Patients who did not experience local recurrence as the first event were censored at the date of the first event (distant recurrence or death) or the last follow-up.
Controlled disease after CCRT was confirmed by CT in all patients. During consolidation IO, patients were monitored by full-body CT scan and clinical examination every 3 months. At the end of durvalumab or after confirmation of the first disease progression, follow-up was at the discretion of the treating oncologist.
Tumor response was evaluated according to the Response Evaluation Criteria in Solid Tumors (RECIST), version 1.1 (26).
Statistical analysis
Data were summarized by frequency and percentage for qualitative variables and by median and range for continuous variables. Groups were compared by using the chi-square or Fisher’s exact test for qualitative variables and the Kruskal–Wallis test for continuous variables. Correlations between continuous variables were calculated with Spearman’s coefficient.
All survival times were estimated by the Kaplan–Meier method with 95% confidence intervals (CIs). In univariable analyses, p-values were calculated by using the Cox proportional hazards model for continuous variables and the log-rank test for qualitative variables, and hazard ratios (HRs) with 95% confidence intervals were estimated with the Cox proportional hazards model for each variable. Cox proportional hazards model was also used to perform multivariable analyses. HRs with 95% confidence intervals were estimated for each variable.
All statistical tests were two-sided, and p-values<0.05 were considered significant. No adjustment was made for multiple comparisons. Statistical analyses were conducted by using Stata® version 16.
Results
Patient characteristics
In total, 50 patients were included. The baseline and treatment characteristics of the population are summarized in Table 1. Of the patients, 52% (n = 26/50) had adenocarcinoma histology, and 72% (n = 36) had stage IIIA or IIIB disease. PD-L1 expression was ≥1% in 37 patients. Most patients were treated with carboplatin-based concurrent chemoradiotherapy (58%, n = 29), and the median number of cycles of chemotherapy was 4 (range, 3–6). All patients were treated with IMRT and image-guided radiation therapy (IGRT). The median dose to the PTV was 66 Gy (55–66 Gy) with a median dose per fraction of 2 Gy (2–2.75 Gy). Eleven patients (22%) had NILN-R+. The median number of irradiated non-involved station(s) was 1 (1–3), and the most common stations targeted were 7, 4, and 2. Forty-two patients (84%) had a partial response at the end of CCRT. All patients received durvalumab as consolidative radiotherapy, and it was started at a median time of 34 days from the end of CCRT.
Dosimetric data
In the whole cohort, the median total PTV was 326 cm3 (114.1–1,284 cm3); mean heart, lung PTV, and body dose were 9.4 Gy (0.8–17.9), 12.2 Gy (5.6–19.2), and 7.1 Gy (2.7–12), respectively. Median EDRIC was 7.6 Gy (2.8–11.6). Of the patients, 72% (36/50) had EDRIC > 6.3 Gy. Forty-two patients (84%) had NITDLNs available for dosimetric analysis because eight patients in the NILN-R− group had all the TDLNs involved. The median mean dose to NITDLNs was 40.4 Gy (25.8–64.3) in the NILN-R+ group vs. 23.2 Gy (3.1–58.9) in the NILN-R− group (p = 0.002).
Dosimetric data are summarized in Table 2.
Biological data
Median ALC at baseline, at the end of CCRT, and IO initiation was 1,715/mm3 (628–3,060), 495/mm3 (130–1,500), and 705/mm3 (175–1,960), respectively. Median NLR at baseline, at the end of CCRT, and IO initiation was 2.75 (0.9–10.5), 5.46 (2–24.6), and 4.28 (1.4–16.2), respectively. Eleven patients (22%) experienced lymphopenia ≤ 500/mm3 at IO initiation. Median nadir lymphopenia was 480/mm3 (130–1,215). Twenty-seven (54%) patients experienced grade 3 or 4 (G3/4) lymphopenia. The median LVR was −71.7% (−84.6%; −26.7%). No clinical/dosimetric difference was found between patients with ALC ≤ 500/mm3 and patients with ALC > 500 mm3 at IO initiation. Patients with ALC ≤ 500/mm3 at IO initiation had significantly lower ALC at baseline and the end of CCRT when compared to patients with ALC >500 mm3, but both groups had a similar LVR (Supplementary Table 1). Similarly, no clinical/dosimetric difference was found between patients with grade 1/2 lymphopenia at nadir and patients with grade 3/4 lymphopenia.
Spearman’s correlation was weak between the LVR and any clinical/dosimetric relevant variables (Supplementary Table 2). There was no association between the LVR and the number of concurrent chemotherapy cures (LVR of −71.7% (−84.6; −26.7) versus –71.7% (−84.3; −42.2) for 1–2 versus 3 cures, respectively) nor between the LVR and the total prescribed dose (LVR of –71.7% (−84.3; −29.5) versus −71.7% (−84.6; −26.7) for dose< 66 Gy versus dose of 66 Gy, respectively).
Survival outcomes
The median follow-up time was 23.2 months (95% CI 18.3–35.2 months).
Fourteen patients (28%) had died at the cutoff date for analysis. The median OS was not reached, and the 2-year OS was 66.2% (95% CI 46.5–80.1). In univariable analysis, previous history of neoplasia (HR 4.44, 95% CI 1.27–15.5, p = 0.011) and lymphopenia ≤ 500/mm3 at IO initiation (HR 3.46, 95% CI 1.10–10.87, p = 0.024) were significantly associated with poor OS. In multivariable analysis, the results were similar, but the associations were not significant (Table 3).
At the time of analysis, 24 patients experienced disease progression or death. The median PFS was 31.4 months (95% CI 14.0–not reached). NILN-R+ (HR 2.60, 95% CI 1.08–6.27, p = 0.028), G3/4 lymphopenia at nadir (HR 2.73, 95% CI 1.09–6.82, p = 0.026), lymphopenia ≤ 500/mm3 at IO initiation (HR 2.69, 95% CI 1.12–6.46, p = 0.021), and EDRIC > 6.3 Gy (HR 3.19, 95% CI 0.94–10.82, p = 0.049) were associated with worse PFS in univariable analysis (Figure 1). In the univariable Cox regression model for continuous variables, tumor SUVmax (HR 1.04, 95% CI 1.00–1.08, p = 0.030), ALC at IO initiation (HR 0.85, 95% CI 0.72–1.00, p = 0.047), and nadir ALC (HR 0.79, 95% CI 0.63–0.99, p = 0.038) were significantly associated with PFS. The multivariable analysis, including NILN-R+, lymphopenia ≤ 500/mm3 at IO initiation, EDRIC > 6.3 Gy, and SUVmax, revealed that NILN-R+ was the strongest factor associated with PFS (HR 3.15, 95% CI 1.23–8.10, p = 0.017). SUVmax was still a prognostic factor (p = 0.038), and there was a trend toward worse PFS with EDRIC > 6.3 Gy (HR 3.03, 95% CI 0.83–11.00, p = 0.093) (Table 4).
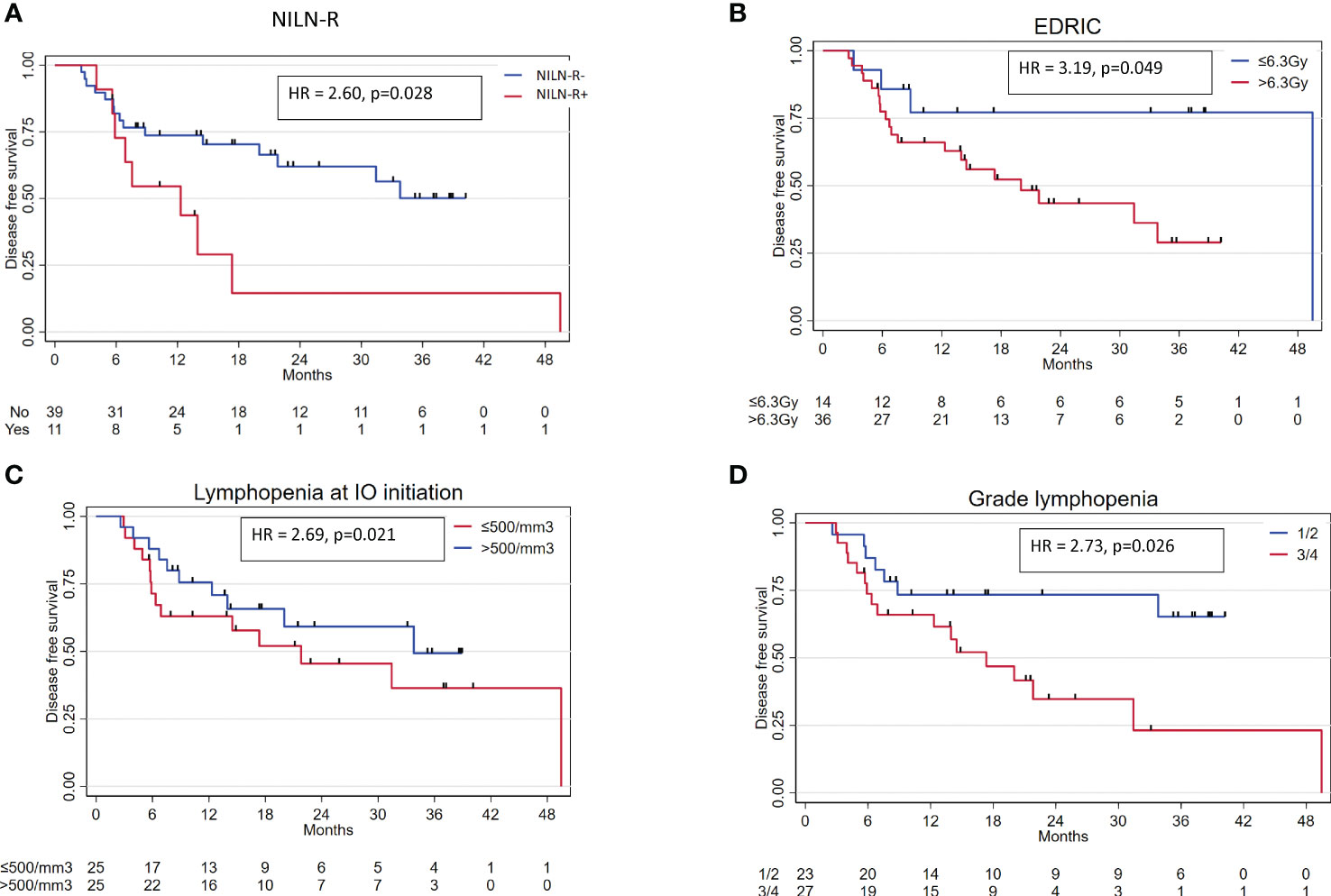
Figure 1 Main variables associated with PFS in univariable analysis. (A) NILN-R. (B) EDRIC. (C) Lymphopenia at IO initiation. (D) Grade of lymphopenia at nadir. NILN-R+, inclusion of at least one non-involved tumor-draining lymph node; IO, immunotherapy; EDRIC, estimated dose to immune cells; PFS, progression-free survival.
The association between PD-L1 status and OS or PFS was not tested owing to the small number of PD-L1-negative patients (n = 6).
Toxicity
At the end of CCRT, 96% had experienced at least one adverse event of any cause and grade, and 20% (10/50) had grade 3 toxicity. The most common grade 3 adverse event was hematologic toxicity (90%, 9/10), and one patient experienced grade 3 esophagitis. Immune-related adverse events (iRAEs) occurred in 66% (33/50) of patients. No grade 4 or 5 iRAE was reported. Five patients (15.2%) experienced grade 3 iRAE: three cases of musculoskeletal toxicity and two cases of skin toxicity (rash). No grade 3 pneumonitis adverse event occurred in the cohort.
Discussion
In this retrospective study, we evaluated the impact of radiation on the immune system, in the context of CCRT followed by durvalumab for stage III NSCLC. One of the main findings is the negative effect of the prophylactic radiation of at least one NITDLN station(s) (NILN-R+). To our knowledge, this is the first study to demonstrate the deleterious impact of radiation on NITDLNs on outcomes in the era of durvalumab after CCRT in NSCLC. Indeed, radiation to NITDLNs was an independent factor for worse PFS in our cohort.
Before the era of durvalumab, involved-field radiotherapy (IFRT) in patients treated with conformational 3D radiotherapy (3D-CRT) was shown to be non-inferior to prophylactic irradiation of all NITDLN stations, known as “elective nodal irradiation” (ENI), in terms of loco-regional recurrence (27–30). In a recent randomized trial, Nestle et al. compared two target volume delineation strategies: one strategy based upon 18F FDG-PET/CT only versus another combining 18F FDG-PET/CT and CT data plus ENI. This trial was the first to show the non-inferiority of reducing target volumes and avoiding ENI based on modern molecular imaging staging (31). Moreover, the risk of locoregional progression was lower in the 18F FDG-PET/CT-based target group (14% vs. 29% at 1 year, HR 0.57; per protocol analysis).
However, it was shown that incidental dose to NITDLNs is high when using IFRT with the 3D-CRT technique, as most of the uninvolved nodal stations receive more than 40 Gy (32). Since the implementation of IMRT, there has been no formal comparison of ENI vs. IFRT. In the study by Nestle et al., as many as 50% of patients were treated with IMRT (31). In our cohort of IMRT-only treatment, a subgroup of patients had at least one NITDLN station included in the CTV (e.g., in the event of NITDLNs between two involved nodal stations). Most of them had only one station included (64%), and the most common stations targeted were stations 2, 4, and 7. Whether or not this strategy is safe in the context of IMRT and consolidation IO remained to be established; herein, we showed that NILN-R+ was associated with worse outcomes.
These findings are in line with a disturbance of the anti-tumor immune response due to prophylactic nodal irradiation. Several preclinical data from TDLN irradiation support these findings. First, some studies highlighted the key role of TDLNs in the anti-tumor immune response (33, 34). Indeed, Dammeijer et al. demonstrated that in the context of immune checkpoint inhibitors, TDLNs contribute to the anti-tumor effects by generating progenitor-exhausted T cells that seed the tumor (33). Furthermore, they showed that PD-1/PD-L1 interactions in TDLN, but not in the tumor, correlate with prognosis in melanoma patients. Marciscano et al. underlined the fact that irradiation of TDLNs restrained the adaptive immune response when stereotactic radiation and immunotherapy were associated (16). A decrease in tumor-infiltrating immune cell density such as CD8+ T cells and attenuation of chemokines associated with T-cell chemoattraction could explain this phenomenon. Similarly, Buchwald et al. found a proliferation of tumor-specific CD8+ T cells in TDLNs following tumor radiotherapy without treatment of lymph nodes (17). More recently, Darragh et al. showed that ENI to a dose of 8 Gy × 3 could disrupt the local and systemic anti-tumor response following combined primary head and neck tumor radiation (3 × 8 Gy) and immunotherapy (anti-CD25) mainly through a decrease in tumor antigen-specific T-cell priming in TDLNs and consequently decrease in circulating antigen-specific T cells (both CD4+ and CD8+) and infiltration into the tumor microenvironment (18).
All this evidence suggests that ENI is probably not the optimal strategy when combining radiotherapy and IO. While the PACIFIC trial in NSCLC is the only phase III trial to have shown a benefit of the adjunction of immune checkpoint inhibitors (ICIs) to chemoradiotherapy for locally advanced disease, the JAVELIN trial and the PEMBRORAD trial in locally advanced head and neck cancer assessing the adjunction of avelumab and pembrolizumab to chemoradiotherapy, respectively, failed to demonstrate any improvement in outcome (35, 36), nor did the KEYNOTE-412 with pembrolizumab in head and neck cancer (NCT03040999) and the CALLA trial with durvalumab in cervical cancer (NCT03830866), according to recent unpublished data (37, 38). One of the key differences between the PACIFIC trial and the other negative trials is the absence of extended ENI in the former, while it was systematically used in the latter. Therefore, the sparing of uninvolved TDLNs during the planning of radiotherapy in the context of immunotherapy could be a promising approach to optimize such a therapeutic association, along with other approaches such as margin reduction, hypofractionation, or alternative radiotherapy techniques including FLASH radiotherapy (39).
We also performed an exploratory analysis to establish a dose cutoff that should not be exceeded in NITDLN. In univariable analysis, no dose cutoff to NITDLNs was correlated with outcomes, possibly due to the lack of power in our study. Interestingly, we found that the median (incidental) dose to NITDLNs in the NILN-R− group was 23.2 Gy. This dose obtained with IMRT is lower than the incidental dose delivered to NITDLNs (approximately 40 Gy) with 3D-CRT (32). This finding underlines the fact that IMRT can achieve better TDLN radiation dose-sparing in order to obtain a stronger synergic effect when combined with IO.
Moreover, our analysis of a modern homogeneous cohort treated with CCRT and consolidation IO seems to confirm the benefit of dose reduction to circulating immune cells as suggested by Ladbury et al. in the pre-immunotherapy era (11). At the 6.3-Gy cutoff, EDRIC was a prognostic factor for PFS (≤6.3 vs. >6.3 Gy, p = 0.049) in univariable analysis. In multivariable analysis, there was only a trend for significance (EDRIC > 6.3 Gy: HR 3.03, p = 0.093). In addition, a recent retrospective study found similar results in a cohort of 100 patients with locally advanced NSCLC treated with IO consolidation (15). Nevertheless, McCall et al. used the equation developed by Jin et al. by considering uniform body volume between patients (10, 15), while we used the model developed by Ladbury et al. with the incorporation of MBD instead of integral total dose divided by 62 × 103. The exploration of ALC at three different times was necessary to better appreciate our EDRIC data. We found that ALC ≤ 500/mm3 at durvalumab initiation was a poor prognostic factor for PFS in univariable analysis (HR 2.69, 95% CI 1.12–6.46, p = 0.021). Nadir ALC was also an important prognostic factor, as patients who did not experience G3/4 lymphopenia had better PFS. These results are consistent with the findings from Friedes et al. (14). Nonetheless, except for age, baseline ALC, and ALC at the end of CCRT, no clinical/dosimetric data were associated with lymphopenia ≤ 500/mm3 at IO initiation in our cohort, and no clinical/dosimetric correlation could be established with the LVR. Especially, neither NILN-R+ nor EDRIC was associated with lymphopenia in this cohort, perhaps because we did not consider the dose to large vessels in the model. Indeed, Cho et al. found a correlation between dose to large vessels and lymphopenia (13). Moreover, the monitoring of tumor-specific subpopulations of lymphocytes could not be assessed. For these reasons, we cannot rule out that the impact of radiation to NITDLNs on the outcome is correlated with circulating tumor-specific lymphocytes via a decrease in tumor antigen-specific T-cell priming.
We also explored the impact of radiation dose on other iOARs on outcomes. Dose to thoracic vertebrae was not associated with worse outcomes or lymphopenia. Doses to the spleen were very low in our cohort, so no correlation could be established.
This study has some limitations mainly due to its retrospective nature and its small cohort size. Multiple comparisons have been performed, which can inflate the alpha risk and the likelihood of type I error. However, due to the exploratory nature of our study, no adjustments were made for multiple comparisons, and all p-values and confidence intervals were shown to allow readers to interpret the results themselves according to the number of tests performed. Therefore, our results are exploratory and need to be confirmed in a larger cohort. However, notably, the single-center design guaranteed homogeneity in radiotherapy techniques and follow-up.
Conclusion
In conclusion, we found that prophylactic irradiation of at least one NITDLN was a strong independent factor for worse PFS in patients treated with consolidation immunotherapy following CCRT for locally advanced NSCLC. Moreover, we confirmed the impact of lymphopenia and irradiation of immune cells (EDRIC) on outcomes in this population. These findings lend weight to the idea that modern radiotherapy techniques should spare host immune structures and especially NITDLNs when combining radiotherapy and immunotherapy for locally advanced disease.
Data availability statement
The raw data supporting the conclusions of this article will be made available by the authors, without undue reservation.
Ethics statement
The studies involving human participants were reviewed and approved by Institutional review boards of Toulouse Cancer Institute. Written informed consent for participation was not required for this study in accordance with the national legislation and the institutional requirements.
Author contributions
Conceptualization, JK and CP; methodology, JK and CP; formal analysis, CP, LC, AL, and JK; investigation, all; data curation, CP; writing—original draft, CP and JK; writing—review and editing, all; supervision, JK. All authors contributed to the article and approved the submitted version.
Conflict of interest
The authors declare that the research was conducted in the absence of any commercial or financial relationships that could be construed as a potential conflict of interest.
Publisher’s note
All claims expressed in this article are solely those of the authors and do not necessarily represent those of their affiliated organizations, or those of the publisher, the editors and the reviewers. Any product that may be evaluated in this article, or claim that may be made by its manufacturer, is not guaranteed or endorsed by the publisher.
Supplementary material
The Supplementary Material for this article can be found online at: https://www.frontiersin.org/articles/10.3389/fonc.2023.1186479/full#supplementary-material
References
1. Antonia SJ, Villegas A, Daniel D, Vicente D, Murakami S, Hui R, et al. Overall survival with durvalumab after chemoradiotherapy in stage III NSCLC. N Engl J Med (2018) 379:2342–50. doi: 10.1056/NEJMoa1809697
2. Spigel DR, Faivre-Finn C, Gray JE, Vicente D, Planchard D, Paz-Ares L, et al. Five-year survival outcomes from the PACIFIC trial: durvalumab after chemoradiotherapy in stage III non-small-cell lung cancer. J Clin Oncol (2022). doi: 10.1200/JCO.21.01308
3. Trowell OA. The sensitivity of lymphocytes to ionising radiation. J Pathol Bacteriol (1952) 64:687–704. doi: 10.1002/path.1700640403
4. Chadha AS, Liu G, Chen HC, Das P, Minsky BD, Mahmood U, et al. Does unintentional splenic radiation predict outcomes after pancreatic cancer radiation therapy? Int J Radiat Oncol Biol Phys (2017) 97:323–32. doi: 10.1016/J.IJROBP.2016.10.046
5. Abravan A, Faivre-Finn C, Kennedy J, McWilliam A, van Herk M. Radiotherapy-related lymphopenia affects overall survival in patients with lung cancer. J Thorac Oncol (2020) 15:1624–35. doi: 10.1016/J.JTHO.2020.06.008
6. Damen PJJ, Kroese TE, van Hillegersberg R, Schuit E, Peters M, Verhoeff JJC, et al. The influence of severe radiation-induced lymphopenia on overall survival in solid tumors: a systematic review and meta-analysis. Int J Radiat Oncol Biol Phys (2021) 111:936–48. doi: 10.1016/J.IJROBP.2021.07.1695
7. Campian JL, Ye X, Brock M, Grossman SA. Treatment-related lymphopenia in patients with stage III non-small-cell lung cancer. Cancer Invest (2013) 31:183–8. doi: 10.3109/07357907.2013.767342
8. Tang C, Liao Z, Gomez D, Levy L, Zhuang Y, Gebremichael RA, et al. Lymphopenia association with gross tumor volume and lung V5 and its effects on non-small cell lung cancer patient outcomes. Int J Radiat Oncol Biol Phys (2014) 89:1084–91. doi: 10.1016/J.IJROBP.2014.04.025
9. Jin JY, Hu C, Xiao Y, Zhang H, Ellsworth S, Schild SE, et al. Higher radiation dose to immune system is correlated with poorer survival in patients with stage III non–small cell lung cancer: a secondary study of a phase 3 cooperative group trial (NRG oncology RTOG 0617). Int J Radiat Oncol (2017) 99:S151–2. doi: 10.1016/j.ijrobp.2017.06.351
10. Jin JY, Hu C, Xiao Y, Zhang H, Paulus R, Ellsworth SG, et al. Higher radiation dose to the immune cells correlates with worse tumor control and overall survival in patients with stage III NSCLC: a secondary analysis of RTOG0617. Cancers (Basel) (2021) 13. doi: 10.3390/CANCERS13246193
11. Ladbury CJ, Rusthoven CG, Camidge DR, Kavanagh BD, Nath SK. Impact of radiation dose to the host immune system on tumor control and survival for stage III non-small cell lung cancer treated with definitive radiation therapy. Int J Radiat Oncol Biol Phys (2019) 105:346–55. doi: 10.1016/J.IJROBP.2019.05.064
12. Thor M, Shepherd AF, Preeshagul I, Offin M, Gelblum DY, Wu AJ, et al. Pre-treatment immune status predicts disease control in NSCLCs treated with chemoradiation and durvalumab. Radiother Oncol (2022) 167:158–64. doi: 10.1016/J.RADONC.2021.12.016
13. Cho Y, Kim Y, Chamseddine I, Lee WH, Kim HR, Lee IJ, et al. Lymphocyte dynamics during and after chemo-radiation correlate to dose and outcome in stage III NSCLC patients undergoing maintenance immunotherapy. Radiother Oncol (2022) 168:1–7. doi: 10.1016/J.RADONC.2022.01.007
14. Friedes C, Chakrabarti T, Olson S, Prichett L, Brahmer JR, Forde PJ, et al. Association of severe lymphopenia and disease progression in unresectable locally advanced non-small cell lung cancer treated with definitive chemoradiation and immunotherapy. Lung Cancer (2021) 154:36–43. doi: 10.1016/J.LUNGCAN.2021.01.022
15. McCall NS, McGinnis HS, Janopaul-Naylor JR, Kesarwala AH, Tian S, Stokes WA, et al. Impact of radiation dose to the immune cells in unresectable or stage III non-small cell lung cancer in the durvalumab era. Radiother Oncol (2022) 174:133–40. doi: 10.1016/J.RADONC.2022.07.015
16. Marciscano AE, Ghasemzadeh A, Nirschl TR, Theodros D, Kochel CM, Francica BJ, et al. Elective nodal irradiation attenuates the combinatorial efficacy of stereotactic radiation therapy and immunotherapy. Clin Cancer Res (2018) 24:5058–71. doi: 10.1158/1078-0432.CCR-17-3427
17. Buchwald ZS, Nasti TH, Lee J, Eberhardt CS, Wieland A, Im SJ, et al. Tumor-draining lymph node is important for a robust abscopal effect stimulated by radiotherapy. J Immunother Cancer (2020) 8. doi: 10.1136/jitc-2020-000867
18. Darragh LB, Gadwa J, Pham TT, Van Court B, Neupert B, Olimpo NA, et al. Elective nodal irradiation mitigates local and systemic immunity generated by combination radiation and immunotherapy in head and neck tumors. Nat Commun (2022) 13:7015. doi: 10.1038/S41467-022-34676-W
19. De Ruysscher D, Faivre-Finn C, Moeller D, Nestle U, Hurkmans CW, Le Péchoux C, et al. European Organization for research and treatment of cancer (EORTC) recommendations for planning and delivery of high-dose, high precision radiotherapy for lung cancer. Radiother Oncol (2017) 124:1–10. doi: 10.1016/J.RADONC.2017.06.003
20. Nestle U, Le Pechoux C, De Ruysscher D. Evolving target volume concepts in locally advanced non-small cell lung cancer. Transl Lung Cancer Res (2021) 10:1999–2010. doi: 10.21037/TLCR-20-805
21. Nestle U, De Ruysscher D, Ricardi U, Geets X, Belderbos J, Pöttgen C, et al. ESTRO ACROP guidelines for target volume definition in the treatment of locally advanced non-small cell lung cancer. Radiother Oncol (2018). doi: 10.1016/j.radonc.2018.02.023
22. Itazawa T, Tamaki Y, Komiyama T, Nishimura Y, Nakayama Y, Ito H, et al. The Japan lung cancer society-Japanese society for radiation oncology consensus-based computed tomographic atlas for defining regional lymph node stations in radiotherapy for lung cancer. J Radiat Res (2017) 58:86–105. doi: 10.1093/JRR/RRW076
23. Ichinose Y, Kato H, Koike T, Tsuchiya R, Fujisawa T, Shimizu N, et al. Completely resected stage IIIA non-small cell lung cancer: the significance of primary tumor location and N2 station. J Thorac Cardiovasc Surg (2001) 122:803–8. doi: 10.1067/MTC.2001.116473
24. Kim AW. Lymph node drainage patterns and micrometastasis in lung cancer. Semin Thorac Cardiovasc Surg (2009) 21:298–308. doi: 10.1053/J.SEMTCVS.2009.11.001
25. Watanabe Y, Shimizu J, Tsubota M, Iwa T. Mediastinal spread of metastatic lymph nodes in bronchogenic carcinoma. mediastinal nodal metastases in lung cancer. Chest (1990) 97:1059–65. doi: 10.1378/CHEST.97.5.1059
26. Eisenhauer EA, Therasse P, Bogaerts J, Schwartz LH, Sargent D, Ford R, et al. New response evaluation criteria in solid tumours: revised RECIST guideline (version 1.1). Eur J Cancer (2009) 45:228–47. doi: 10.1016/j.ejca.2008.10.026
27. Chen M, Bao Y, Ma HL, Hu X, Wang J, Wang Y, et al. Involved-field radiotherapy versus elective nodal irradiation in combination with concurrent chemotherapy for locally advanced non-small cell lung cancer: a prospective randomized study. BioMed Res Int (2013) 2013. doi: 10.1155/2013/371819
28. Yuan S, Sun X, Li M, Yu J, Ren R, Yu Y, et al. A randomized study of involved-field irradiation versus elective nodal irradiation in combination with concurrent chemotherapy for inoperable stage III nonsmall cell lung cancer. Am J Clin Oncol (2007) 30:239–44. doi: 10.1097/01.COC.0000256691.27796.24
29. Yang K, Cao F, Wang J, Liu L, Zhang T, Wu G. Improved local control without elective nodal radiotherapy in patients with unresectable NSCLC treated by 3D-CRT. Front Med China (2007) 1:381–5. doi: 10.1007/S11684-007-0074-7
30. Li R, Yu L, Lin S, Wang L, Dong X, Yu L, et al. Involved field radiotherapy (IFRT) versus elective nodal irradiation (ENI) for locally advanced non-small cell lung cancer: a meta-analysis of incidence of elective nodal failure (ENF). Radiat Oncol (2016) 11. doi: 10.1186/S13014-016-0698-3
31. Nestle U, Schimek-Jasch T, Kremp S, Schaefer-Schuler A, Mix M, Küsters A, et al. Imaging-based target volume reduction in chemoradiotherapy for locally advanced non-small-cell lung cancer (PET-plan): a multicentre, open-label, randomised, controlled trial. Lancet Oncol (2020) 21:581–92. doi: 10.1016/S1470-2045(20)30013-9
32. Kimura T, Togami T, Nishiyama Y, Ohkawa M, Takashima H. Impact of incidental irradiation on clinically uninvolved nodal regions in patients with advanced non-small-cell lung cancer treated with involved-field radiation therapy: does incidental irradiation contribute to the low incidence of elective nodal failure? Int J Radiat Oncol Biol Phys (2010) 77:337–43. doi: 10.1016/J.IJROBP.2009.05.039
33. Dammeijer F, van Gulijk M, Mulder EE, Lukkes M, Klaase L, van den Bosch T, et al. The PD-1/PD-L1-Checkpoint restrains T cell immunity in tumor-draining lymph nodes. Cancer Cell (2020) 38:685–700.e8. doi: 10.1016/J.CCELL.2020.09.001
34. Fransen MF, Schoonderwoerd M, Knopf P, Camps MG, Hawinkels LJ, Kneilling M, et al. Tumor-draining lymph nodes are pivotal in PD-1/PD-L1 checkpoint therapy. JCI Insight (2018) 3. doi: 10.1172/JCI.INSIGHT.124507
35. Lee NY, Ferris RL, Psyrri A, Haddad RI, Tahara M, Bourhis J, et al. Avelumab plus standard-of-care chemoradiotherapy versus chemoradiotherapy alone in patients with locally advanced squamous cell carcinoma of the head and neck: a randomised, double-blind, placebo-controlled, multicentre, phase 3 trial. Lancet Oncol (2021) 22:450–62. doi: 10.1016/S1470-2045(20)30737-3
36. Tao Y, Biau J, Sun XS, Sire C, Martin L, Alfonsi M, et al. Pembrolizumab versus cetuximab, concurrent with radiotherapy in patients with locally advanced squamous cell carcinoma of head and neck unfit for cisplatin (GORTEC 2015-01 PembroRad): a multicenter, randomized, phase 2 trial. Ann Oncol (2022). doi: 10.1016/J.ANNONC.2022.10.006
37. Update on CALLA phase III trial of concurrent use of imfinzi and chemoradiotherapy in locally advanced cervical cancer . Available at: https://www.astrazeneca.com/media-centre/press-releases/2022/update-on-calla-phase-iii-trial-for-imfinzi.html (Accessed July 31, 2022).
38. Primary results of the phase III KEYNOTE-412 study: pembrolizumab (pembro) with chemoradiation therapy (CRT) vs placebo plus CRT for locally advanc.| OncologyPRO . Available at: https://oncologypro.esmo.org/meeting-resources/esmo-congress/primary-results-of-the-phase-iii-keynote-412-study-pembrolizumab-pembro-with-chemoradiation-therapy-crt-vs-placebo-plus-crt-for-locally-advanc (Accessed December 12, 2022).
Keywords: radiotherapy, immunotherapy, non-small cell lung cancer (NSCLC), tumor-draining lymph nodes (TDLN), EDRIC, lymphopenia, elective node irradiation
Citation: Pasquier C, Chaltiel L, Massabeau C, Rabeau A, Lebas L, Lusque A, Texier J-S, Moyal EC-J, Mazières J and Khalifa J (2023) Impact of radiation on host immune system in patients treated with chemoradiotherapy and durvalumab consolidation for unresectable locally advanced non-small cell lung cancer. Front. Oncol. 13:1186479. doi: 10.3389/fonc.2023.1186479
Received: 14 March 2023; Accepted: 30 May 2023;
Published: 16 June 2023.
Edited by:
David Azria, Université de Montpellier, FranceReviewed by:
Fernanda Herrera, Centre Hospitalier Universitaire Vaudois (CHUV), SwitzerlandPervin Hurmuz, Hacettepe University, Türkiye
Copyright © 2023 Pasquier, Chaltiel, Massabeau, Rabeau, Lebas, Lusque, Texier, Moyal, Mazières and Khalifa. This is an open-access article distributed under the terms of the Creative Commons Attribution License (CC BY). The use, distribution or reproduction in other forums is permitted, provided the original author(s) and the copyright owner(s) are credited and that the original publication in this journal is cited, in accordance with accepted academic practice. No use, distribution or reproduction is permitted which does not comply with these terms.
*Correspondence: Jonathan Khalifa, a2hhbGlmYS5qb25hdGhhbkBpdWN0LW9uY29wb2xlLmZy