- 1Innovare Biomarkers Laboratory, Medicine and Experimental Surgery Nucleus, University of Campinas, Campinas, Brazil
- 2Pediatric Department, University of Campinas, Campinas, Brazil
- 3Clinical Pathology Department, University of Campinas, Campinas, Brazil
Background: Cystic fibrosis (CF) is a disabling genetic disease with an increased prevalence in European heritage populations. Currently, the most used technique for collection of CF samples and diagnosis is provided through uncomfortable tests, with uncertain results, mostly based on chloride concentration in sweat. Since CF mutation induces many metabolic changes in patients, exploring these alterations might be an alternative to visualize potential biomarkers that could be used as interesting tools for further diagnostic upgrade, prioritizing simplicity, low cost, and quickness.
Methods: This contribution describes an accurate strategy to provide potential biomarkers related to CF, which may be understood as a potential tool for new diagnostic approaches and/or for monitoring disease evolution. Therefore, the present proposal consists of using skin imprints on silica plates as a way of sample collection, followed by direct-infusion high-resolution mass spectrometry and multivariate data analysis, intending to identify metabolic changes in skin composition of CF patients.
Results: Metabolomics analysis allowed identifying chemical markers that can be traced back to CF in patients’ skin imprints, differently from control subjects. Seven chemical markers from several molecular classes were elected, represented by bile acids, a glutaric acid derivative, thyrotropin-releasing hormone, an inflammatory mediator, a phosphatidic acid, and diacylglycerol isomers, all reflecting metabolic disturbances that occur due to of CF.
Conclusion: The comfortable method of sample collection combined with the identified set of biomarkers represent potential tools that open the range of possibilities to manage CF and follow the disease evolution. This exploratory approach points to new perspectives about the development of diagnostic assay using biomarkers and the management CF.
Introduction
Cystic fibrosis (CF) is a recessive genetic exocrine disorder that affects the transport of fluids and electrolytes through cell membrane, resulting in abnormal viscous secretion in multiple organs. Although this disease affects all racial and ethnic groups, Caucasians are the most affected; 1 in every 2,000–3,000 European newborns presents CF (1). Soon after this disease was characterized, in 1953 (2), CF was considered almost inevitably lethal, mainly at the first decade of life. However, improvements at diagnostic methods and clinical management have led to a considerable increase in life expectancy for CF patients over the years, as shown by recent statistical data indicating that the birth cohort of the year 2000 may present a survival median of 50 years (3). Although most CF patients are able to manage well disease, their quality of life is still affected by limitations such as alterations in overall physiological functioning (4), reduced lung function (5), and increase of gastrointestinal and pancreaticobiliary tracts malignancies (6).
All CF manifestations are caused by mutations affecting the cystic fibrosis transmembrane conductance regulator (CFTR). Although there are multiple mutations at CFTR gene associated with CF disorders, the most common corresponds to a deletion of a phenylalanine at position 508 on chromosome 7, called F508del mutation (7). Approximately 2,000 CFTR mutations are known to cause CF; some may be responsible for milder CF phenotypes, while others present more severe manifestations. These differences at CFTR gene mutation locus generates great heterogeneity, making clinical and even laboratory diagnosis difficult tasks (8).
The two most used tests for CF laboratory diagnosis are DNA analysis and sweat test; the first one is applied to recognize known mutations on CFTR gene; however, gene mutations not reported yet may not be diagnosed due to a limited number of gene alterations present in mutation panels, predefined for commercial laboratory tests. All molecular methods require expertise to perform and interpret and should be well established what are the limitations and mutations not identified (9). Furthermore, this test allows the analysis of the complete CFTR gene sequence; nonetheless, the available current genetic analyses are unable to detect mutations that occur out of coding regions, which renders the assessment of these regions even more difficult. The list of CFTR-causing mutations was limited to 23 mutations until recently, being expanded to 312, according to CFTR2 database.1 However, some of these CFTR mutations are also identified in non-CF patients, demonstrating unclear functional and clinical consequences. Ooi et al. (10, 11) showed that using the 122 CFTR mutations list for genotyping occurred an increase of 4.9% of CF diagnosis; meanwhile, 81.3% of patients tested remained with unclear diagnosis. This study also demonstrated the variation of genotype diagnosis by the patients phenotype (8, 10). Moreover, genotyping is an expensive and time-consuming process that is performed in specialized molecular and genetics laboratories, making it a test with limited access for patients (8).
In addition to DNA analysis, the most widely employed laboratory diagnostic test in CF diagnosis is the quantitative pilocarpine iontophoresis testing (QPIT), also known as sweat test (12). Although it is an uncomfortable method, QPIT is considered the gold-standard test, and consists in the determination of chloride concentration in sweat; in this test, the normal upper limit can be <30 mmol/L for infants or <40 mmol/L for older adults (13). For sweat collection, perspiration is induced on skin of the forearm through local administration of pilocarpine nitrate, followed by the application of a battery-operated electric current that stimulates rapid and increased sweating rate. After sweat collection, chloride levels are evaluated and concentrations above 60 mmol/L for non-infants and infants are considered positive for CF (12, 13).
Although QPIT is considered the method of choice for CF diagnosis, there is an obvious window of inaccurate diagnosis for chloride concentrations between 30 and 60 mmol/L, which classifies patients within this range as indeterminate cases, requiring further evaluation (13). Considering chloride quantification <30 mmol/L as “normal” and ≥30 mmol/L as “at risk” for CF, QPIT sensitivity and specificity are 100 and 92.8%, respectively (14). However, the current standard diagnostic method does not differentiate positive and negative patients for CF who present chloride concentration between 30 and 60 mmol/L (13). Besides the borderline window, the sweat test was designed based on the diagnostic of patients with multi-organ symptoms. However, patients presenting single-organ symptoms may have inconclusive sweat tests (10). In addition to the large borderline window and the inconclusive characteristic in some cases, the test procedures are liable of failure, mainly during steps such as sweat collection, weighting, dilution and elution, and even due biological variation, which hamper a more refined diagnosis. Furthermore, accurate sweat test results may be challenging taking into account the high frequency of technical errors and misinterpretation (13). There is a need of additional tests for the diagnosis of borderline patients, such as the use of nasal potential difference (NPD) testing for the measurement of bioelectric characteristic of nasal epithelium and extensive genotyping. At the same time, the lack of agreement between test results became a clinical concern (10). Therefore, the association of different testing parameters and the development of new techniques is needed to improve CF diagnosis, reduce the borderline window, and embrace the most cases of CF.
The metabolomics approach has been growing as a promising tool for monitoring biochemical pathways and their relationship with biological phenotypes in an integrated perspective (15). Metabolomics evaluation of biological samples allows researchers to identify biomarkers or sets of biomarkers that could be useful to differentiate health and disease profiles, which may lead to more accurate diagnostic tools (16). Previously, metabolomics had been employed for the analysis of CF using different biological samples, such as sweat (17), stool (18), bronchoalveolar lavage fluid (19), exhaled breath (20), and sputum (21). In this context, this contribution presents a concept study with an exploratory approach, intended to provide a set of chemical markers for CF, based on a non-invasive, non-sweat-stimulating collection method that relies on species that are present in the skin surface of CF patients with the F508del mutation, associated with high-resolution mass spectrometry. Ultimately, we aimed at providing guidance with molecules that improve the knowledge about CF metabolism and may work as alternative options for the development of new, more comprehensive potential diagnostic tools.
Materials and Methods
Patients’ Selection
Sixteen patients were selected in a retrospective fashion at CF Clinic of the Clinics Hospital at University of Campinas. Inclusion criteria were that patients presented at least one sweat test with chloride concentration greater than or equal to 60 mEq/L, and presence of homozygosis for the mutation F508del, characterized by genetic studies. Exclusion criteria were the presence of skin lesions on the patients’ back, local of sample collection, and/or another skin disease. After selection, these patients were submitted to sample collection during scheduled visits to the hospital. The control (CT) group was composed of healthy individuals at the same age and gender as the CF group, recruited from schools and universities. Sample collection was approved by the Research Ethics Committee of the School of Medical Sciences—University of Campinas/Brazil (Protocol number: 1.100.978) with written informed consent from all subjects and parental consent from incompetent patients, when applicable. All experiments were performed in accordance with relevant guidelines and regulations regarding samples of human origin (22).
Sample Collection and Preparation
Skin metabolites were collected from both CF and CT groups with plates of silica gel 60 G, suitable for thin-layer chromatography (Merck, Darmstadt, Germany). These plates were cut into small squares of 1 cm2 and were superposed on the individuals’ back for 1 min. Then, silica plates were extracted with 1,000 µL of methanol:water (1:1), and 1 µL ammonium hydroxide was added to each sample to facilitate ionization.
Mass Spectrometry
Samples were directly injected in an ESI-LTQ-XL Orbitrap Discovery instrument (Thermo Scientific, Bremen, Germany) with nominal resolution of 30,000 (FWHM) under the following conditions: flow rate of 10 µL/min, sheath gas at 10 AU, spray voltage of 5 kV, and capillary temperature of 280°C. Analyses were performed in triplicates, and all data were acquired in the negative ion mode at the mass range of 200–700 m/z.
Statistical Analysis and Biomarker Election
The discrimination of each group analyzed was performed using an Orthogonal Partial Least Squares Discriminant Analysis (O-PLS-DA) available in the online software MetaboAnalyst 3.02 (23). Characteristic ions from each group was considered using the VIP score list (Variable Importance in Projection) generated by the software.
The selected biomarkers were identified using online databases: METLIN (Scripps Center for Metabolomics, La Jolla, CA, USA), HMDB version 3.6 (Human Metabolome Database),3 Lipid MAPS online database (University of California, San Diego, CA, USA),4 and KEGG Pathway Database (Kyoto Encyclopedia of Genes and Genomes).5 These databases were chosen to identify the potential biomarkers elected by statistical analysis, and it was established that mass error between the exact mass (acquired) and theoretical mass should not be greater than 2 ppm.
Results
During the study, 16 CF patients were selected, with ages ranging from 5 to 19 years old (mean: 12 years; median: 13 years), with 56% males and 44% females. The sweat test presented an average concentration of chloride of 111 mEq/L (minimum: 76 mEq/L; maximum: 166 mEq/L). All patients presented deficits in lung function and 69% presented two or more different microorganism isolated from sputum within the past 12 months. Regarding further clinical conditions, 100% of patients present pancreatic insufficiency, 67% have evidence of liver disease, 44% make use of ursodeoxycholic acid, and 19% developed diabetes. Regarding the CT group, it was composed of 16 healthy individuals within the same gender and age range as the CF group and was submitted to sample collection at the same period as CF patients.
HRMS analysis on negative ion mode was performed, and Figure 1 shows representative mass spectra from each group. Information extracted from spectral data served as basis for the statistical analysis using O-PLS-DA, which compared the CT and CF groups to elect biomarkers for CF. O-PLS-DA plot (Figure 2) shows complete separation of CT and CF groups; from these analysis, one biomarker was selected for CT group and seven biomarkers for CF group, all presenting VIP scores above 2. Detailed information of the elected biomarkers for CT and CF groups can be seen in Table 1.
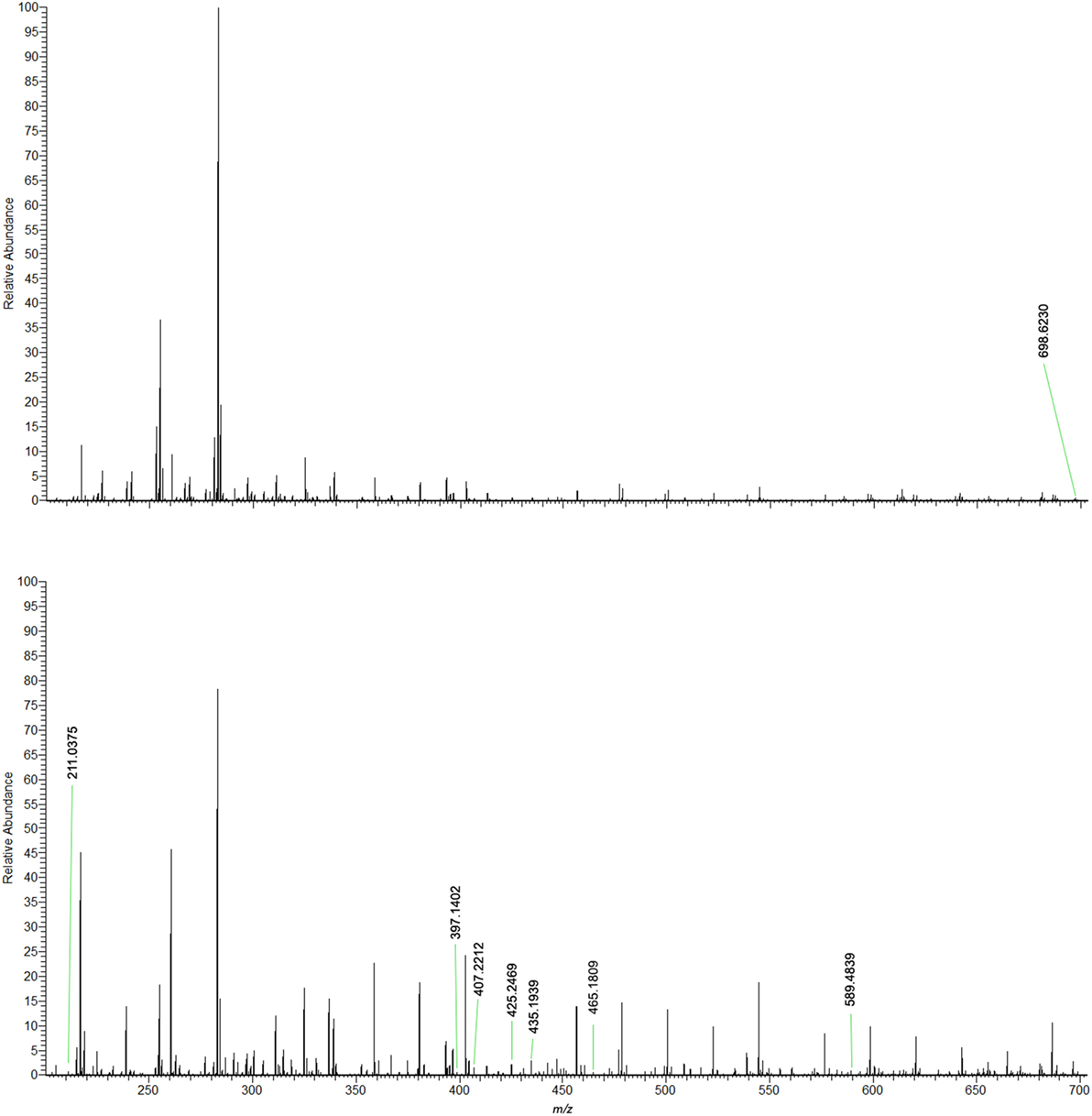
Figure 1. Representative mass spectra comparing the skin imprints of control individuals and FC patients. Negative ion mode.
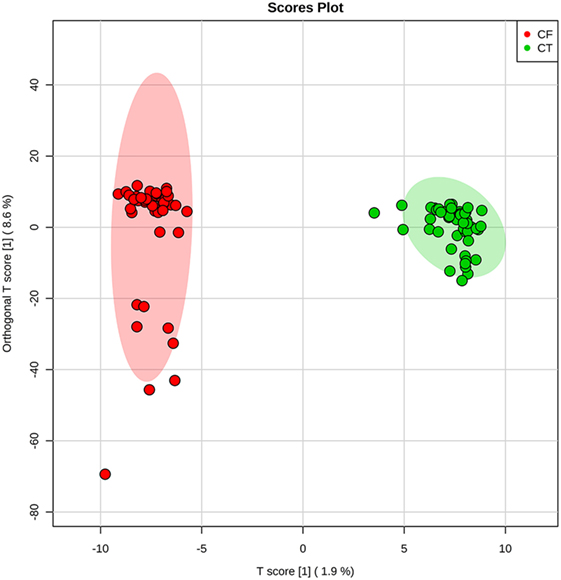
Figure 2. Two-dimensional plot provided by Orthogonal Partial Least Squares Discriminant Analysis. It is possible to observe a clear separation between control (CT) subjects and patients with cystic fibrosis (CF).
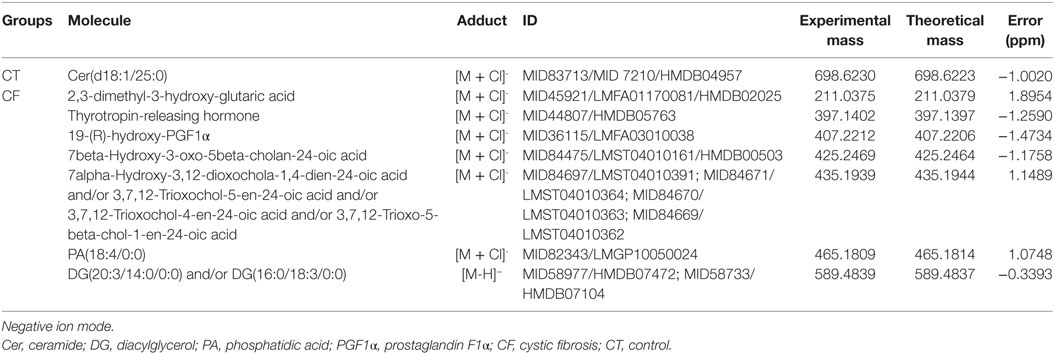
Table 1. Biomarkers selected by Orthogonal Partial Least Squares Discriminant Analysis, elucidated by HRMS.
Discussion
Although sweat test remains the gold-standard method for CF diagnosis, there are some points that can be improved. When using the gold-standard QPIT, there are lacks of sensitivity and specificity at the concentration range of 30–60 mmol/L of chloride in sweat. In addition, sweat collection may be time-consuming and uncomfortable for the patient, especially for babies and children. Due to frequent methodological errors, retesting is often required more than once to present confirmatory results, which may be stressful considering the patients are, for the most part, children (24). In addition to the use of QPIT for the diagnosis of CF, other efforts have been applied to access the expression and function of CFTR during the diagnosis and therapy. Methods based on in vivo measurements of NPD (10, 25), combined ion channel measurements (11, 26), rectal CFTR-mediated Cl− secretion (intestinal combined ion channel measurements) (26), and the evaluation of β-adrenergic secretory response by evaporometry (27) as CF disease parameters may be useful for the diagnosis of patients with questionable QPIT results, CF diagnosis, and to predict patients prognosis. All these methods when applied alone or combined reflect the pursuit for CF diagnosis clarification.
Intending to find chemical markers that could guide researchers through new methodologies, we have proposed a method based on mass spectrometry and metabolomics that presents a simple and quick workflow for sample collection, data acquiring, and processing. For that, silica plates were chosen for sample collection due to its useful particularities of adsorption and stabilization of molecules, such as those present in the skin surface, what seems advantageous and dispenses the need for additional apparatus for sample preserving (28). This relatively simple sample collection and preparation, coupled with direct-infusion mass spectrometry, provides a more straightforward analysis protocol for biomarkers monitoring. With less sample preparation steps, the errors associated to the methodology decrease and the method becomes more reproducible, which is in accordance with a primary requisite to standardize a method in biomarkers identification: reproducibility.
CT Group Biomarker
A healthy skin is extremely important for humans, as it provides protection against environment injuries and covers the underlying tissues. It is composed of different layers: stratum corneum, epidermis, dermis, and the fat layer. The outermost skin layer, stratum corneum, is composed of keratinocytes and lipids, specifically ceramides (50%), cholesterol (25%), and fatty acids (10–20%). These lipids provide fundamental limitation to water and electrolyte movement and act as a barrier against microorganisms’ invasion (29, 30). Consequently, epidermis presents unique structures, such as epidermal ceramides that are not found in any other cell types in the human body (30). For instance, this study has identified Cer (d18:1/25:0) (698 m/z) in the epidermis of CT subjects, an odd-numbered fatty acid carbon chain, uncommonly found in human cells in general, but present among epidermis’ lipids. About 30% of the total ceramide (Cer) present in the epidermis is represented by Cer containing odd-numbered fatty acids (30), which corroborates the election of Cer (d18:1/25:0) as a CT group marker. Therefore, this class of Cer stands out in this comparison, defining the CT group’s skin, as determined by O-PLS-DA.
CF Biomarkers
Distinct molecules were identified through the present method as CF biomarkers, and each one is associated with metabolic dysfunctions observed in this disease. In healthy subjects, CFTR channel gating is predominantly regulated by protein kinase A phosphorylation, which mediates gene expression, conformational changes, and protein trafficking from the endoplasmic reticulum (ER) to Golgi apparatus and cell surface (31). However, the phenylalanine deletion at position 508 (F508del) of the CFTR gene in CF reduces the rates of phosphorylation and may contribute to improper folding, defective trafficking, and slower rates of channel activation (31, 32).
The F508del-CFTR is produced and retained in the ER and undergoes ER-associated degradation through the proteasome pathway. Nevertheless, a limited number of misfolded proteins reach the Golgi apparatus for glycosylation and few of them reach cell surface (31). The export from ER depends on the interaction between the CFTR and the coat complex (COP) budding machinery (31, 33). Formation of COPII-coated vesicles depends on the hydrolysis of phosphatidylcholine to phosphatidic acid (PA) by phospholipase D (PLD) (33). The relationship between PA and CFTR trafficking remains unknown, but Hashimoto and coworkers (33) showed that PLD-mediated PA formation is required for CFTR transport from ER to Golgi. In CF, it may be assumed that the organism is trying to maintain a compensatory mechanism of PA synthesis to enhance the concentration of COPII and the transport of F508del-CFTR to maturation in the Golgi apparatus, aiming to recover the imbalance caused by inoperative CFTR mutated. Therefore, the election of PA (465 m/z) as a CF biomarker in the present study is coherent with the cellular physiological condition. Considering PA’s importance for different intracellular events, different studies have also demonstrated PA as a regulatory lipid, even in signal transduction (34, 35). Accordingly, intracellular PA must be tightly regulated, which occurs through turnover into diacylglycerol (DG). Among the present results, DG isomers (589 m/z) were also elected as biomarkers for CF patients, a plausible marker for CF, taking into account that the excess of PA available for ERAD translocation process must enter into turnover process.
In addition to PA and DG biomarkers, this study has also identified an inflammatory mediator as CF biomarker: 19-(R)-hydroxy-PGF1α (407 m/z). The lack of functional CFTR in CF results in intrinsic inflammation, the major cause of morbidity and mortality in patients (36, 37). CFTR mutation induces the expression of high levels of nuclear factor kappa-light-chain-enhancer of activated B cells, and phospholipase A2 (37, 38). In turn, these molecules induce the overproduction of inflammatory mediators, as well as cytokines and prostaglandins (38–40), which is in accordance with the presented results. Furthermore, the redox imbalance caused by F508del-CFTR altered ion transportation causes abnormal generation of reactive oxygen species, leading to exacerbation of oxidative stress, and development of inflammatory and degenerative lesions in target issues (41). Thereby, the increased inflammatory response and oxidative stress corroborate the election of hydroxylated prostaglandin F1-alpha as a CF biomarker.
Considering that mutant and misfolded proteins, as well as F508del-CFTR, are submitted to intense degradation through proteasome pathway, it is important to evaluate the products of amino acids catabolism, which will stand out from healthy cells metabolites. The conventional catabolism pathway of F508del-CFTR misfolded proteins occurs through glutaryl-CoA dehydrogenase and, alternatively, by the production of glutaric acid and its derivatives, which justifies the election of 2,3-dimethyl-3-hydroxy-glutaric acid (211 m/z) as a CF biomarker. Considering that this biomarker is typically overproduced on ketogenic patients (42, 43), it is important to ponder that around 18.5% of the CFTR protein is composed of lysine and leucine, two purely ketogenic amino acids (44). Thus, the identification of 2,3-dimethyl-3-hydroxy-glutaric acid on CF skin is plausible, since most of F508del-CFTR misfolded proteins are under intense degradation process in proteasomes.
Given that F508del-CFTR alters ionic transportation, many intracellular processes may be affected, including hypothalamic–pituitary–thyroid axis. Several studies have reported subclinical hypothyroidism on CF patients, and the absence of CFTR has been tested to elucidate its importance on synthesis of thyroid hormones (45). Within this context, Li and coauthors suggested that the increased Na+ absorption due to CFTR−/− may contribute to hypothyroidism on CF patients (46). Since CF patients may be affected by low levels of thyroid hormones, every cell responsible for its synthesis might be altered. In addition to the thyroid gland, skin cells are also responsible for the synthesis and metabolism of several hormones, including thyroid-stimulating hormone (TSH) (47). Cutaneous TSH expression is increased by thyrotropin-releasing hormone (TRH) and reduced by thyroid hormones (47, 48). Since CF population is reported to present subclinical hypothyroidism and iodine deficiency (49), it is expected that high levels of TRH (397 m/z) be found in skin samples of CF patients. Interestingly, TRH was elected as a CF marker by the proposed methods in our study; this brings to our attention that a more frequent follow-up regarding the levels of thyroid hormones is necessary, since these tests are not routinely performed.
Another physiological important alteration in CF patients is cystic fibrosis liver disease (CFLD), a serious complication responsible for 2–4% of total mortality. The diagnosis of CFLD lack specific and sensitive markers; however, the prevalence rate ranges between 27 and 35% in patients older than 18 years (50, 51). Steatosis, for instance, can affect between 23 and 67% of CF patients of any age, presenting bile alkalization and viscosity increases. These events, in turn, result in higher levels of free radicals and accumulation of hydrophilic bile acids, which may provide damage to hepatocytes and bile ducts (50). When in high concentration in the bloodstream, bile acids may be deposited on the skin, which corroborates the election of primary bile acids (425 and 435 m/z) as CF markers. Taking into account that 67% of patients selected for this study were suspected of CFLD, the identification of bile acids among CF skin surface molecules is coherent with the evaluated patients. Although 44% of CF individuals evaluated in this study were under treatment with ursodeoxycholic acid, a drug used to reduce bile viscosity, its effectiveness has not been confirmed yet, and thus bile acids cannot be ruled out as potential biomarkers.
As shown above, the chosen markers are not only involved with the ionic imbalance caused by CF in the sweat gland but also with the pathophysiology and progression of the disease; therefore, they may be considered relevant markers when monitored together for the F508del mutation. Since this contribution has evaluated only an F508del scenario, it is not possible to infer that the same set of biomarkers will be useful in identifying biomarkers in CF patients with any of the mutations, since the platform is sufficiently sensitive to detect small levels of altered components associated with physiological changes caused by other mutations. Nonetheless, this is a proof-of-concept study, and further investigation is required, including studies that gather a larger number of patients, subjects with borderline sweat test results, and more heterogeneous CF groups regarding mutations, for improved results that can encompass the largest possible number of probabilities.
Taking into account the results shown above, the method applied for sample collection showed simplicity and less probability of methodological errors, differently from the standard method currently used. In addition, the proposed methodology using mass spectrometry analysis combined with statistical markers election allowed identifying the above-prospected biomarkers. When observed in a CF pathological condition, these biomarkers gain context and evidence points of metabolic disturbances that might be carefully evaluated as potential tools for new diagnostic investments. Interestingly, this contribution has evidenced the identification of primary bile acids as biomarkers for CF, which demonstrates that the concept of this approach may be useful not only for prospecting molecules that render new guides for diagnosis of CF but may also be used for monitoring the progression of the disease. Therefore, our study was deemed successful in providing chemical markers for CF that show great potential to be employed not only for diagnostic purposes but also to work as a parameter that evaluates disease evolution. These are valuable information for CF management and improvement in patients’ quality of life.
Ethics Statement
This study was carried out in accordance with the recommendations of the Research Ethics Committee of the School of Medical Sciences—University of Campinas/Brazil (Protocol number: 1.100.978) with written informed consent from all subjects. All subjects gave written informed consent in accordance with the Declaration of Helsinki. The protocol was approved by the Research Ethics Committee of the School of Medical Sciences—University of Campinas/Brazil.
Author Contributions
CE, LD, CG, JR, and AR performed sample collection. CE and LD performed experiments and wrote the manuscript. EL, DO, CM, JD, CG, JR, AR, and CL performed data analysis and performed manuscript review. RC idealized all experiments and managed the research group.
Conflict of Interest Statement
The authors declare that the research was conducted in the absence of any commercial or financial relationships that could be construed as a potential conflict of interest.
Acknowledgments
We thank the Cystic Fibrosis Clinic Team—Clinical Hospital—University of Campinas for providing samples and support from the subjects involved in this research.
Funding
The authors thank the São Paulo Research Foundation (FAPESP) for the fellowships of CE (14/00302-0) and CFORM (16/17066-2), as well as for the grants to RC (11/50400-0 and 15/06809-1).
Abbreviations
Cer, ceramide; COP, coat complex; CT, control; CF, cystic fibrosis; CFLD, cystic fibrosis liver disease; CFTR, cystic fibrosis transmembrane conductance regulator; DG, diacylglycerol; ER, endoplasmic reticulum; NPD, nasal potential difference; O-PLS-DA, Orthogonal Partial Least Squares Discriminant Analysis; PA, phosphatidic acid; PC, phosphatidylcholine; PLA2, phospholipase A2; PLD, phospholipase D; PKA, protein kinase A; QPIT, quantitative pilocarpine iontophoresis testing; TSH, thyroid-stimulating hormone; TRH, thyrotropin-releasing hormone.
Footnotes
References
1. Salvatore D, Buzzetti R, Mastella G. Update of literature from cystic fibrosis registries 2012–2015. Part 6: Epidemiology, nutrition and complications. Pediatr Pulmonol (2017) 52(3):390–98. doi:10.1002/ppul.23611
2. di Sant’Agnese PA, Darling RC, Perera GA, Shea E. Sweat electrolyte disturbances associated with childhood pancreatic disease. Am J Med (1953) 15(6):777–84. doi:10.1016/0002-9343(53)90169-7
3. Dodge J, Lewis P, Stanton M, Wilsher J. Cystic fibrosis mortality and survival in the UK: 1947–2003. Eur Respir J (2007) 29(3):522–6. doi:10.1183/09031936.00099506
4. Simmonds NJ, Cullinan P, Hodson ME. Growing old with cystic fibrosis – the characteristics of long-term survivors of cystic fibrosis. Respir Med (2009) 103(4):629–35. doi:10.1016/j.rmed.2008.10.011
5. Horsley A, Siddiqui S. Putting lung function and physiology into perspective: cystic fibrosis in adults. Respirology (2015) 20(1):33–45. doi:10.1111/resp.12382
6. Ooi CY, Durie PR. Cystic fibrosis from the gastroenterologist’s perspective. Nat Rev Gastroenterol Hepatol (2016) 13(3):175–85. doi:10.1038/nrgastro.2015.226
7. Riordan JR. Identification of the cystic fibrosis gene: cloning and characterization of complementary DNA. Trends Genet (1989) 5:363. doi:10.1016/0168-9525(89)90155-8
8. Sosnay PR, Siklosi KR, Van Goor F, Kaniecki K, Yu H, Sharma N, et al. Defining the disease liability of variants in the cystic fibrosis transmembrane conductance regulator gene. Nat Genet (2013) 45(10):1160–7. doi:10.1038/ng.2745
9. Dequeker E, Stuhrmann M, Morris MA, Casals T, Castellani C, Claustres M, et al. Best practice guidelines for molecular genetic diagnosis of cystic fibrosis and CFTR-related disorders-updated European recommendations. Eur J Human Genet (2009) 17(1):51–65. doi:10.1038/ejhg.2008.136
10. Ooi CY, Dupuis A, Ellis L, Jarvi K, Martin S, Ray PN, et al. Does extensive genotyping and nasal potential difference testing clarify the diagnosis of cystic fibrosis among patients with single-organ manifestations of cystic fibrosis? Thorax (2014) 69(3):254–60. doi:10.1136/thoraxjnl-2013-203832
11. Ooi CY, Dupuis A, Gonska T, Ellis L, Ni A, Jarvi K, et al. Does integration of various ion channel measurements improve diagnostic performance in cystic fibrosis? Ann Am Thorac Soc (2014) 11(4):562–70. doi:10.1513/AnnalsATS.201311-412OC
12. Gibson LE, Cooke RE. A test for concentration of electrolytes in sweat in cystic fibrosis of the pancreas utilizing pilocarpine by iontophoresis. Pediatrics (1959) 23(3):545–9.
13. Collie JT, Massie RJ, Jones OA, LeGrys VA, Greaves RF. Sixty-five years since the New York heat wave: advances in sweat testing for cystic fibrosis. Pediatr Pulmonol (2014) 49(2):106–17. doi:10.1002/ppul.22945
14. Warwick W, Huang N, Waring W, Cherian A, Brown I, Stejskal-Lorenz E, et al. Evaluation of a cystic fibrosis screening system incorporating a miniature sweat stimulator and disposable chloride sensor. Clin Chem (1986) 32(5):850–3.
15. Medina S, Dominguez-Perles R, Gil J, Ferreres F, Gil-Izquierdo A. Metabolomics and the diagnosis of human diseases-a guide to the markers and pathophysiological pathways affected. Curr Med Chem (2014) 21(7):823–48. doi:10.2174/0929867320666131119124056
16. Gowda GN, Zhang S, Gu H, Asiago V, Shanaiah N, Raftery D. Metabolomics-based methods for early disease diagnostics. Expert Rev Mol Diagn (2008) 8(5):617–33. doi:10.1586/14737159.8.5.617
17. Macedo AN, Mathiaparanam S, Brick L, Keenan K, Gonska T, Pedder L, et al. The sweat metabolome of screen-positive cystic fibrosis infants: revealing mechanisms beyond impaired chloride transport. ACS Cent Sci (2017) 3(8):904–13. doi:10.1021/acscentsci.7b00299
18. Kaakoush NO, Pickford R, Jaffe A, Ooi CY. Is there a role for stool metabolomics in cystic fibrosis? Pediatr Int (2016) 58(8):808–11. doi:10.1111/ped.13063
19. Wolak JE, Esther CR Jr, O’Connell TM. Metabolomic analysis of bronchoalveolar lavage fluid from cystic fibrosis patients. Biomarkers (2009) 14(1):55–60. doi:10.1080/13547500802688194
20. Robroeks CM, van Berkel JJ, Dallinga JW, Jöbsis Q, Zimmermann LJ, Hendriks HJ, et al. Metabolomics of volatile organic compounds in cystic fibrosis patients and controls. Pediatr Res (2010) 68(1):75–80. doi:10.1203/00006450-201011001-00143
21. Yang J, Eiserich JP, Cross CE, Morrissey BM, Hammock BD. Metabolomic profiling of regulatory lipid mediators in sputum from adult cystic fibrosis patients. Free Radic Biol Med (2012) 53(1):160–71. doi:10.1016/j.freeradbiomed.2012.05.001
22. Clínicas BP. Documento de las Américas. IV Conferencia Panamericana para la armonización de la reglamentación farmacéutica. República Dominicana: Conferencia. Santo Domingo (2005).
23. Xia J, Sinelnikov IV, Han B, Wishart DS. MetaboAnalyst 3.0 – making metabolomics more meaningful. Nucleic Acids Res (2015) 43(W1):W251–7. doi:10.1093/nar/gkv380
24. Mattar ACV, Leone C, Rodrigues JC, Adde FV. Sweat conductivity: an accurate diagnostic test for cystic fibrosis? J Cyst Fibr (2014) 13(5):528–33. doi:10.1016/j.jcf.2014.01.002
25. Knowles M, Paradiso A, Boucher R. In vivo nasal potential difference: techniques and protocols for assessing efficacy of gene transfer in cystic fibrosis. Hum Gene Ther (1995) 6(4):445–55. doi:10.1089/hum.1995.6.4-445
26. Hirtz S, Gonska T, Seydewitz HH, Thomas J, Greiner P, Kuehr J, et al. CFTR Cl-channel function in native human colon correlates with the genotype and phenotype in cystic fibrosis. Gastroenterology (2004) 127(4):1085–95. doi:10.1053/j.gastro.2004.07.006
27. Quinton P, Molyneux L, Ip W, Dupuis A, Avolio J, Tullis E, et al. β-Adrenergic sweat secretion as a diagnostic test for cystic fibrosis. Am J Respir Crit Care Med (2012) 186(8):732–9. doi:10.1164/rccm.201205-0922OC
28. Lima EDO, Macedo CSD, Esteves CZ, Oliveira DND, Pessolani MCV, Nery JADC, et al. Skin imprinting in silica plates: a potential diagnostic methodology for leprosy using high-resolution mass spectrometry. Anal Chem (2015) 87(7):3585–92. doi:10.1021/acs.analchem.5b00097
29. Pappas A. Epidermal surface lipids. Dermatoendocrinol (2009) 1(2):72–6. doi:10.4161/derm.1.2.7811
30. Kihara A. Synthesis and degradation pathways, functions, and pathology of ceramides and epidermal acylceramides. Prog Lipid Res (2016) 63:50–69. doi:10.1016/j.plipres.2016.04.001
31. Chin S, Hung M, Bear CE. Current insights into the role of PKA phosphorylation in CFTR channel activity and the pharmacological rescue of cystic fibrosis disease-causing mutants. Cell Mol Life Sci (2017) 74(1):57–66. doi:10.1007/s00018-016-2388-6
32. McClure ML, Barnes S, Brodsky JL, Sorscher EJ. Trafficking and function of the cystic fibrosis transmembrane conductance regulator: a complex network of posttranslational modifications. Am J Physiol Lung Cell Mol Physiol (2016) 311(4):L719–33. doi:10.1152/ajplung.00431.2015
33. Hashimoto Y, Okiyoneda T, Harada K, Ueno K, Sugahara T, Yamashita A, et al. Phosphatidic acid metabolism regulates the intracellular trafficking and retrotranslocation of CFTR. Biochim Biophys Acta (2008) 1783(1):153–62. doi:10.1016/j.bbamcr.2007.08.011
34. Bi K, Roth MG, Ktistakis NT. Phosphatidic acid formation by phospholipase D is required for transport from the endoplasmic reticulum to the Golgi complex. Curr Biol (1997) 7(5):301–7. doi:10.1016/S0960-9822(06)00153-9
35. Brindley DN, English D, Pilquil C, Buri K, Ling Z-C. Lipid phosphate phosphatases regulate signal transduction through glycerolipids and sphingolipids. Biochim Biophys Acta (2002) 1582(1):33–44. doi:10.1016/S1388-1981(02)00135-X
36. Jacquot J, Tabary O, Le Rouzic P, Clement A. Airway epithelial cell inflammatory signalling in cystic fibrosis. Int J Biochem Cell Biol (2008) 40(9):1703–15. doi:10.1016/j.biocel.2008.02.002
37. Vij N, Mazur S, Zeitlin PL. CFTR is a negative regulator of NFκB mediated innate immune response. PLoS One (2009) 4(2):e4664. doi:10.1371/journal.pone.0004664
38. Medjane S, Raymond B, Wu Y, Touqui L. Impact of CFTR ΔF508 mutation on prostaglandin E 2 production and type IIA phospholipase A 2 expression by pulmonary epithelial cells. Am J Physiol Lung Cell Mol Physiol (2005) 289(5):L816–24. doi:10.1152/ajplung.00466.2004
39. Soltys J, Bonfield T, Chmiel J, Berger M. Functional IL-10 deficiency in the lung of cystic fibrosis (cftr−/−) and IL-10 knockout mice causes increased expression and function of B7 costimulatory molecules on alveolar macrophages. J Immunol (2002) 168(4):1903–10. doi:10.4049/jimmunol.168.4.1903
40. Verhaeghe C, Delbecque K, de Leval L, Oury C, Bours V. Early inflammation in the airways of a cystic fibrosis foetus. J Cyst Fibros (2007) 6(4):304–8. doi:10.1016/j.jcf.2006.12.001
41. Galli F, Battistoni A, Gambari R, Pompella A, Bragonzi A, Pilolli F, et al. Oxidative stress and antioxidant therapy in cystic fibrosis. Biochim Biophys Acta (2012) 1822(5):690–713. doi:10.1016/j.bbadis.2011.12.012
43. Daum RS, Scriver CR, Mamer OA, Delvin E, Lamm P, Goldman H. An inherited disorder of isoleucine catabolism causing accumulation of α-methylacetoacetate and α-methyl-β-hydroxybutyrate, and intermittent metabolic acidosis. Pediatr Res (1973) 7(3):149–60. doi:10.1203/00006450-197303000-00007
44. Sawers RG. Amino acid degradation. eLS. Chichester: John Wiley & Sons Ltd (2015). p. 1–11. doi:10.1002/9780470015902.a0001388.pub3
45. Luca F, Trimarchi F, Sferlazzas C, Benvenga S, Costante G, Mami C, et al. Thyroid function in children with cystic fibrosis. Eur J Pediatr (1982) 138(4):327–30. doi:10.1007/BF00442510
46. Li H, Ganta S, Fong P. Altered ion transport by thyroid epithelia from CFTR−/− pigs suggests mechanisms for hypothyroidism in cystic fibrosis. Exp Physiol (2010) 95(12):1132–44. doi:10.1113/expphysiol.2010.054700
47. Harvey S, Aramburo C, Sanders E. Extrapituitary production of anterior pituitary hormones: an overview. Endocrine (2012) 41(1):19–30. doi:10.1007/s12020-011-9557-z
48. Bodó E, Kany B, Gáspár E, Knüver J, Kromminga A, Ramot Y, et al. Thyroid-stimulating hormone, a novel, locally produced modulator of human epidermal functions, is regulated by thyrotropin-releasing hormone and thyroid hormones. Endocrinology (2010) 151(4):1633–42. doi:10.1210/en.2009-0306
49. Naehrlich L, Dörr H-G, Bagheri-Behrouzi A, Rauh M. Iodine deficiency and subclinical hypothyroidism are common in cystic fibrosis patients. J Trace Elem Med Biol (2013) 27(2):122–5. doi:10.1016/j.jtemb.2012.08.002
50. Kobelska-Dubiel N, Klincewicz B, Cichy W. Liver disease in cystic fibrosis. Prz Gastroenterol (2014) 9(3):136–41. doi:10.5114/pg.2014.43574
Keywords: cystic fibrosis, cystic fibrosis transmembrane conductance regulator, screening, skin, biomarkers, metabolomics
Citation: Esteves CZ, de Aguiar Dias L, de Oliveira Lima E, de Oliveira DN, Rodrigues Melo CFO, Delafiori J, Souza Gomez CC, Ribeiro JD, Ribeiro AF, Levy CE and Catharino RR (2018) Skin Biomarkers for Cystic Fibrosis: A Potential Non-Invasive Approach for Patient Screening. Front. Pediatr. 5:290. doi: 10.3389/fped.2017.00290
Received: 07 November 2017; Accepted: 18 December 2017;
Published: 10 January 2018
Edited by:
Musharraf Jelani, King Abdulaziz University, Saudi ArabiaReviewed by:
Chee Yee Ooi, University of New South Wales, AustraliaSaadullah Khan, Decision Research, United States
Copyright: © 2018 Esteves, de Aguiar Dias, de Oliveira Lima, de Oliveira, Rodrigues Melo, Delafiori, Souza Gomez, Ribeiro, Ribeiro, Levy and Catharino. This is an open-access article distributed under the terms of the Creative Commons Attribution License (CC BY). The use, distribution or reproduction in other forums is permitted, provided the original author(s) or licensor are credited and that the original publication in this journal is cited, in accordance with accepted academic practice. No use, distribution or reproduction is permitted which does not comply with these terms.
*Correspondence: Rodrigo Ramos Catharino, rrc@fcm.unicamp.br