- 1Divsion of Neonatology, Dr. von Hauner Children’s Hospital, Ludwig-Maximilians-University, Munich, Germany
- 2Institute for Epidemiology and Medical Biometry, University of Ulm, Ulm, Germany
- 3Divsion of Neonatology, University Hospital of Leipzig, Leipzig, Germany
Background: Ventilator-induced lung injury with subsequent bronchopulmonary dysplasia remains an important issue in the care of extremely low-birth-weight infants. Permissive hypercapnia has been proposed to reduce lung injury. Hypercapnia changes cerebral perfusion, but its influence on the peripheral microcirculation is unknown.
Methods: Data were collected from 12 infants, who were randomized to a permissive high PCO2 target group (HTG) or a control group (CG). Inclusion criteria were birth weight between 400 and 1,000 g, gestational age from 23 to 28 6/7 weeks, intubation during the first 24 h of life, and no malformations. The PCO2 target range was increased stepwise in both groups for weaning and was always 15 mmHg higher in the HTG than in the CG. Skin microvascular parameters were assessed non-invasively with sidestream dark field imaging on the inner side of the right arm every 24 h during the first week of life and on the 14th day of life.
Results: Infants in the HTG had significantly higher max. PCO2 exposure, which was associated with a significantly and progressively reduced functional vessel density (FVD, p < 0.01). Moreover, there were significant differences in the diameter distribution over time, with HTG subjects having fewer small vessels but more large vessels.
Conclusion: High PCO2 levels significantly impaired peripheral microcirculation in preterm infants, as shown by a decreased FVD, presumably secondary to peripheral vasoconstriction.
ISRCTN: 56143743.
Introduction
Most extremely low-birth-weight infants (<1,000 g) require respiratory assistance after birth, leading to bronchopulmonary dysplasia (BPD), a chronic form of lung disease (1, 2). Permissive hypercapnia is a strategy of tolerating higher PCO2 levels for the benefit of less aggressive ventilation and therefore less mechanical trauma to the lung (3–7). In randomized trials, ventilatory intensities were reduced but resulted in only marginal positive effects on clinical outcome (6, 8–11). Concerns about possible adverse effects such as intraventricular hemorrhage with consecutive developmental impairment, as suggested by retrospective data (12–15), have not been substantiated in randomized controlled trials (6, 8–11). We know that hypercapnia causes dilatation of cerebral arterioles with possibly increased risk of intracranial bleeding (16). On the level of the microcirculation, hypoxia and hypercapnia lead to an increase in perfusion in the cerebral cortex of the rat (17, 18).
In the past 10 years, we have learned that disturbances in the microcirculation have profound effects on outcome. In sepsis, for example, a persistent decrease in functional vessel density (FVD) is indicative of poor survival (19, 20). Changes in the microcirculation were also observed in many different settings such as subarachnoid hemorrhage, congenital heart disease, or myocardial ischemia (21–23). In the preterm infant, a persistent ductus arteriosus (PDA) significantly changes the microcirculation of the skin (24). In preterm hypotensive newborns, FVD in the periphery was significantly elevated in comparison to normotensive infants, suggesting a redistribution of microvascular flow as an underlying mechanism for hypotension (25).
Therefore, the microcirculation is an important, but underrecognized physiologic function with possible impact on important clinical outcome parameters. Furthermore, the microcirculation may be influenced by PCO2. We sought to measure the microcirculation in very preterm infants participating in a multicenter randomized controlled trial of different PCO2 target ranges (PHELBI) (10). The skin of the preterm infants is a specific and easily accessible organ to observe changes in the microcirculation. Our hypothesis was that the cutaneous microcirculation might be affected by higher PCO2 levels during permissive hypercapnia regarding FVD, diameter distribution, and quality of flow.
Materials and Methods
This study was part of the randomized, prospective multicenter hypercapnia study (PHELBI, ISRCTN 56143743) (10). Infants with gestational ages between 23 and 28 6/7 weeks and birth weights between 400 and 1,000 g, who were intubated during the first 24 h, were randomized within the next 12 h to either a high PCO2 target group (HTG) or a control group (CG) after written parental consent. Exclusion criteria were congenital malformations, chromosomal aberrations, and severe asphyxia [pH < 7.0 and heart rate (HR) < 100/min at 10 min of life]. All infants who participated in the PHELBI trial at our center within a predefined time frame of 18 months were included in this study.
During the first 14 days of life, the PCO2 target ranges were increased stepwise with the target range in the HTG always 15 mmHg higher than in the CG. PCO2 levels were kept between 55 and 65 mmHg in the HTG and 40 and 50 mmHg in the CG during the first 3 days, increased to 60–70 mmHg (HTG) and 45–55 mmHg (CG) on the fourth day, and increased to 65–75 mmHg (HTG) and 50–60 mmHg (CG) again on the seventh day until extubation or peak inspiratory pressure <12 cm H2O or day 14. At our center, the skin microcirculation of a subgroup of randomized infants was evaluated as single-center substudy. The institutional review board of the Medical Faculty of Ludwig Maximilians University of Munich approved the study protocol and the consent forms, and all parents granted written informed consent.
Sidestream dark field (SDF) imaging with the handheld video microscope “MicroScan” (Microvision Medical, Wallingford, Pennsylvania) with a five times magnification was used for the assessment of the microcirculation. Monochromatic green light (530 nm) emitted from LEDs, surrounding a light guide, is absorbed by hemoglobin in the erythrocytes and reflected by other tissues or structures. The result is a real-time video of perfused vessels to a depth of 3 mm, thus producing a functional video of the microcirculation. SDF imaging has been validated against conventional capillary microscopy in various studies (26–29). We obtained sequences of 15 s on three different areas on the right inner upper arm. The sublingual region usually preferred for SDF imaging in adults is not feasible in preterm infants. In previous studies, the upper arm has been shown to be easily accessible and to provide a good image quality (24, 25, 30, 31). To reduce artifacts by lanugo hair, we chose the inner side of the arm. Since a PDA influences the skin microcirculation, we consistently used only the right arm to avoid the effects of PDA as a confounder (24).
Measurements started on the first day of life and continued over the first week in 24-h intervals, and the last measurement was obtained on the fourteenth day of life. All measurements were done by one investigator (KS). All video loops were analyzed offline with the microvision analysis software, which offers a semiautomatic analysis. Vessels are automatically detected on the screen, but individual corrections for artifacts such as hair are possible. FVD, which represents total vessel length in millimeter per image area in square millimeter, as well as the distribution of vessel diameters is subsequently calculated. The latter results in the representation of small (<10 μm), medium (10–20 µm), and large (20–100 µm) vessels as a percentage of the total vessel length. Moreover, quality of flow was analyzed, classifying vessels of a quadrant into vessels with “no flow,” “intermittent flow,” “sluggish flow,” “continuous flow,” and “hyperdynamic flow.” Normal flow is defined as continuous flow and hyperdynamic flow as extremely fast flow. No flow and intermittent and sluggish flow stand for different stages of hypodynamic and thus pathological flow. Flow classification was undertaken on the third, fourth, sixth, seventh, and fourteenth day of life and expressed as percentage of vessels with the respective quality of flow. All video analyses were performed with the investigator blinded to patient, treatment, and time point.
Simple statistical analyses were performed with GraphPadPrism 5 (GraphPad Software Inc., La Jolla, CA, USA). For each time point, three video scans per arm were analyzed, and the mean value of a variable was used for statistical analysis. To compare both groups over time, we used linear mixed effects regression models to account for the dependencies of measurements within each infant (fitted with proc mixed, SAS software version 9.4, SAS Institute, Cary, NC, USA). Group comparisons for categorical variables were performed using the chi-square test or Fisher’s exact test as appropriate.
For unpaired comparisons between the two groups, the Mann–Whitney U test was used for non-parametric data. For paired comparisons, the Wilcoxon signed-rank test was used.
To consider the development of PCO2 exposure between both groups, a further linear mixed effects regression model was used. For other laboratory values (hematocrit, lactate, and CRP), we compared minimal and maximal values over the observation period with the Mann–Whitney U test. Because of the exploratory nature of our study, the results of the statistical tests should not be interpreted as confirmatory. An adjustment for multiple testing was not done. p < 0.05 was considered as statistically significant.
The PCO2 levels were monitored by blood gas analyses, using arterial blood if available, or capillary blood. CRP levels, hemoglobin levels, medication, and blood transfusions were extracted from the charts. Vital signs were recorded before the start of each microcirculation measurement.
Results
Clinical Variables
Twelve preterm infants were enrolled in our study (six from each randomization group, see Figure 1). Gender and birth weight distribution and other variables were similar (Table 1). One infant of the HTG group had to be excluded, as it was extubated on day 2 of life. Another infant of the HTG group died on day 4 of life. Microcirculatory and clinical values of this infant were included as available. Ventilation parameters such as mean airway pressure, positive end-expiratory pressure (PEEP), positive inspiratory pressure (PIP), high-frequency oscillation amplitude, and frequency tended to be lower in the HTG group, as expected, but not significantly. PCO2 values were significantly higher (Figure 2; Table 1). Incubator temperature and humidity, HR, and blood pressure did not differ. Other laboratory values, including hemoglobin, hematocrit, lactate, and CRP, did not differ significantly.
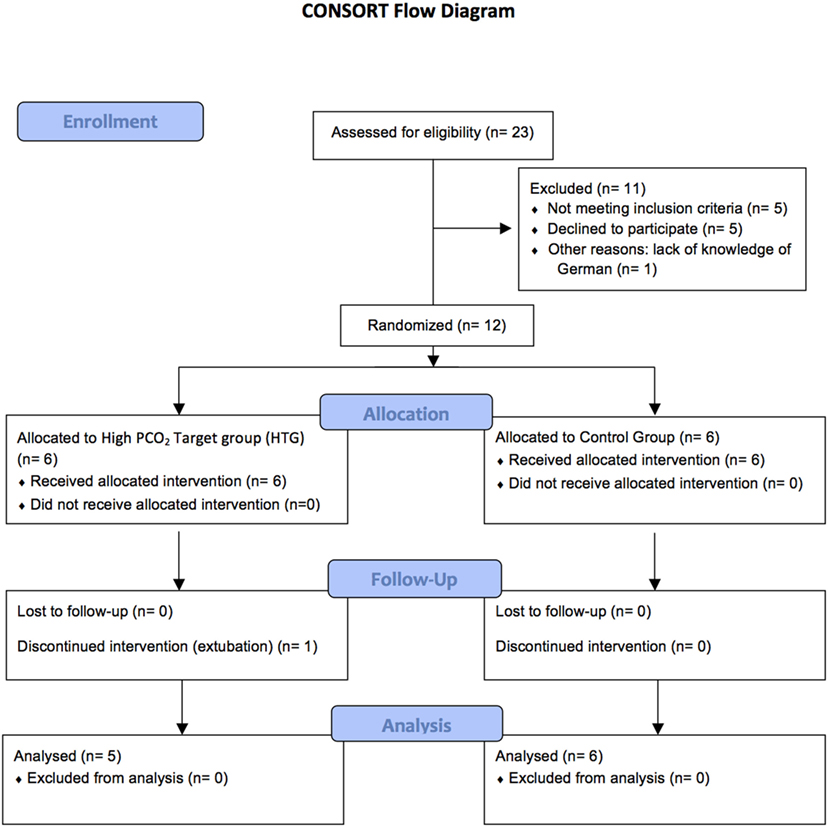
Figure 1. A total number of 12 patients could be included to the study as illustrated in the Consort flow diagram.
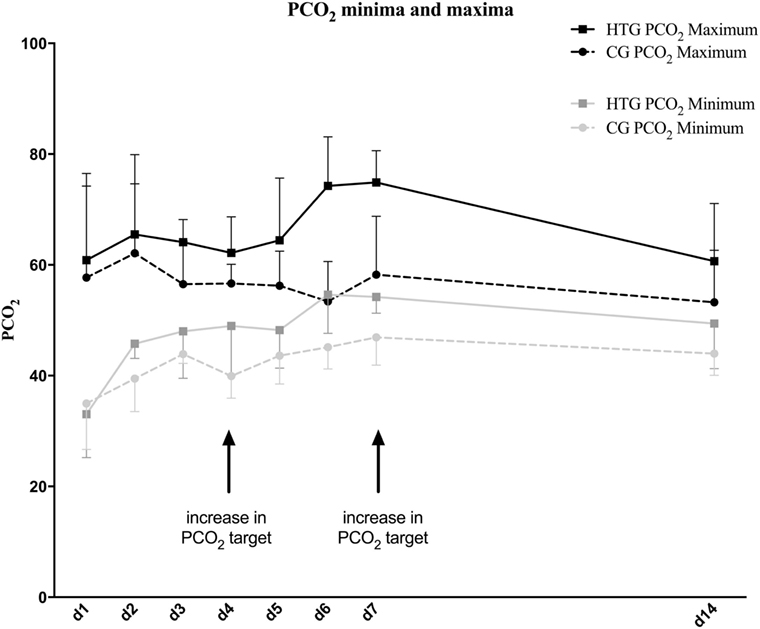
Figure 2. PCO2 minima and maxima in the high PCO2 target group (HTG) and control group (CG) over the course of 14 days after birth. Minimum and maximum values of all patients are presented as mean values with SDs. PCO2 target levels were stepwise increased on the fourth and seventh day of life. As intended, PCO2 levels were higher in the HTG.
Microcirculatory Variables
Images showed similar vasculature at baseline, but declining vessel density toward the end of the study in the HTG (Figure 3). Similar results were determined by the quantitative evaluation.
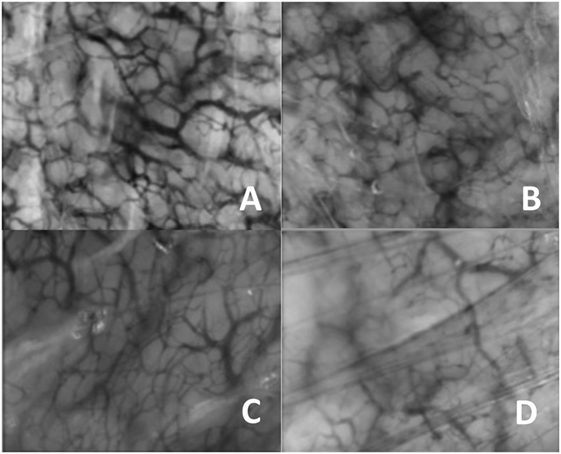
Figure 3. Typical image of the microcirculation. (A) Day 1 control group, (B) day 14 control group, (C) day 1 high PCO2 target group, and (D) day 14 high PCO2 target group.
Functional Vessel Density
Functional vessel density did not differ at baseline between HTG and CG, but decreased significantly over the first week in the HTG and remained lower until the end of the study (Figure 4), compared to CG. The comparison between groups and the time effect was significant (both p < 0.01). Vessel surface (VS) was also significantly lower in the HTG group over time (Figure 5, p < 0.01 for the between-group comparison and p < 0.01 for the time effect).
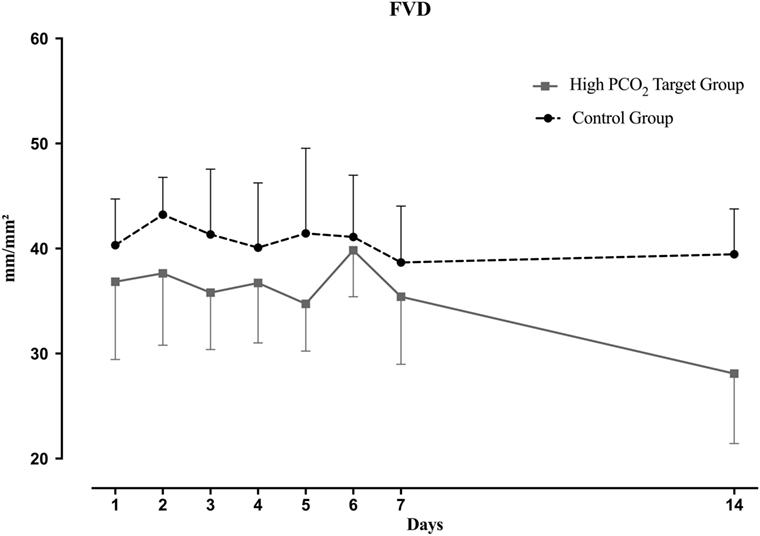
Figure 4. Functional vessel density (FVD) over the course of 14 days. Mean and SD are shown. Overall, FVD was significantly lower in the high PCO2 target group (p < 0.01 by a linear mixed effects regression model) and time dependent (p < 0.01).
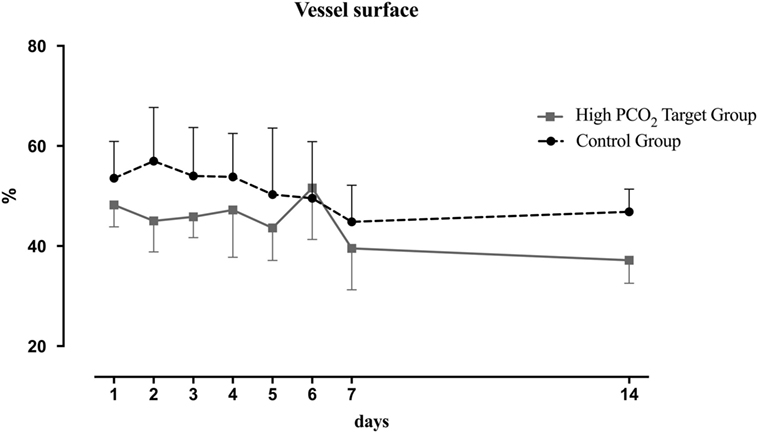
Figure 5. Vessel surface (VS) over the course of 14 days. Mean and SD are shown. Overall, VS was significantly lower in the high PCO2 target group (p < 0.01 for the between groups comparison, p < 0.01 for the time effect).
Diameter
Most detected vessels were small with diameters <10 µm and varied between 60 and 80% of vessel density with no significant difference between the groups at baseline. In the HTG group, the proportion of small vessels significantly decreased (Figure 6), and therefore, the proportion of medium and large vessels increased significantly over time (Figure 7). This resulted in a significant difference between groups in the vessel distribution (p < 0.01) with a significant time effect (p < 0.05).
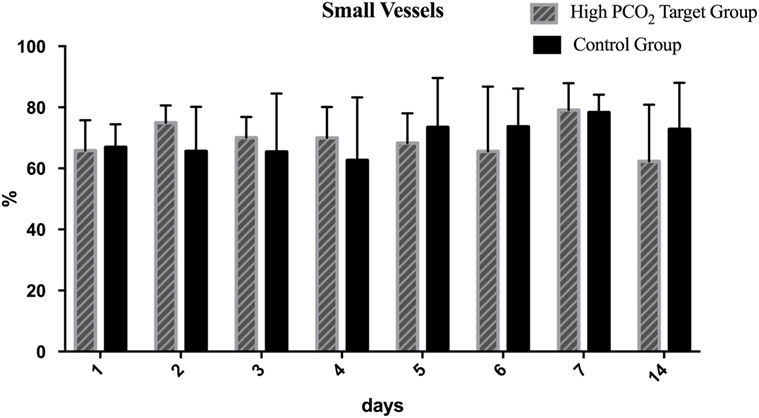
Figure 6. Distribution of small vessels over the course of 14 days. Mean and SD are shown. In the high PCO2 target group, the proportion of small vessels significantly decreased resulting in a significant difference between groups in the vessel distribution (p < 0.01).
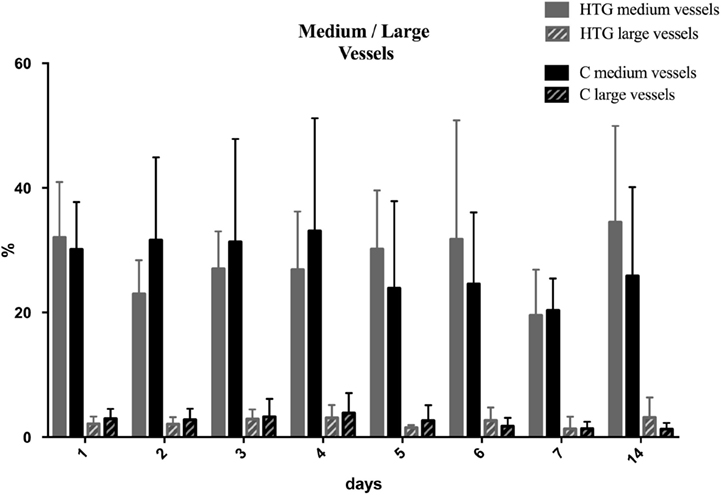
Figure 7. Distribution of medium and large vessels over the course of 14 days. Mean and SD are shown. In the high PCO2 target group (HTG), the proportion of medium and large vessels significantly increased over time (p < 0.05), resulting in a significant difference between groups in the vessel distribution (p < 0.01).
Flow
The flow in most vessels was continuous, and only a small fraction <6% had no flow in both groups. There were no significant differences between both groups over the time observed. The stepwise increases of PCO2 targets on days 4 and 7 were not consistently followed by temporary increases of flow to hyperdynamic levels in all patients (data not shown).
Discussion
This study demonstrates that 14 days of permissive hypercapnia with relatively high PCO2 targets as used in this study have a significant impact on the microcirculation of the skin, compared to the CG with only mildly elevated targets. We demonstrated a significant decrease in FVD, whereas FVD remained constant in the CG. Thus, hypercapnia significantly reduced skin perfusion. The decrease is more prominent in the second week of permissive hypercapnia. This suggests a long-term influence of higher PCO2 levels on skin capillaries. This is the first study to evaluate the influence of hypercapnia on the microcirculation of preterm infants over a longer time period (16–18, 32–43). Moreover, we demonstrate significant changes in the diameter distribution with a shift toward larger vessels in the HTG group. At the same time, other clinical parameters as well as ventilation parameters such as PIP and PEEP did not differ between both groups. Thus, the only difference between both groups relevant for our measurements can be seen in the partial pressure of arterial CO2.
Peripheral oxygenation is essential for preserving organ function (44). Macrocirculatory parameters are only a poor indicator for tissue oxygen delivery (19). SDF imaging provides a non-invasive technique to visualize peripheral vasoregulation by using a microscope at the bedside.
The reduction of FVD and VS may be the result of a PCO2-dependent redistribution of blood flow. Respiratory-induced changes in the partial pressure of arterial CO2 also play a major role in the regulation of cerebral blood flow (45). Hypercapnia leads to vasodilatation of cerebral arterioles, whereas hypocapnia leads to vasoconstriction and a subsequent decrease in cerebral arterial flow (46). Therefore, one would have expected a higher FVD in the HTG group, but the opposite effect was found. One possible explanation may be concurrent hypercapnia-induced vasodilatation in deeper areas of the body, including, but not limited, the brain, which may have drawn blood supply away from the skin, leading to a compensatory vasoconstriction and thus explaining the effects observed in this study.
Alternatively, high PCO2 may have a direct negative effect on skin perfusion. Both suggested mechanisms would lead to a redistribution of the blood volume toward larger vessels in the deeper areas.
Another possible explanation for a reduction in FVD and VS may be the so-called Bohr effect. Raised PCO2 levels and a low pH reduce the oxygen affinity of hemoglobin and facilitate oxygen unloading, thus enhancing oxygen diffusion from hemoglobin to the tissues (32, 34). Therefore, the lower FVD does not need to be a negative effect; it could also be a sign of better tissue oxygenation while reducing the need for perfused blood vessels.
A fourth possible explanation for the reduction in FVD may be vasodilation due to high PCO2 values, which could explain the larger proportion of medium and large vessels observed in our HTG group. As a compensatory mechanism, small vessels would constrict and would result in a reduced overall FVD and VS due to hypercapnia.
Therefore, the significant changes in diameter distribution seem to be another interesting finding in our study. After birth, both groups showed a similar distribution of vessel diameter. When analyzed on the fourteenth day, the fraction of small vessels had decreased in the HTG, while it increased in the CG. In return, medium and large vessels were more prominent in the HT group.
Komori et al. (36) described proportionate increasing diameters and velocity while increasing PCO2 up to levels of 80 mmHg in rabbits. Hudetz et al. (18) obtained similar results when studying the effects of hypercapnia in the capillaries of the rat cerebral cortex. The increasing diameters are consistent with our observation that the proportion of medium and large vessels increases with higher PCO2 levels. However, we cannot confirm the increased velocity, because even though the fraction of hyperdynamic flow appeared to increase with higher PCO2 targets, this did not reach statistical significance in our study. In sepsis models, similar microcirculatory changes indicated a poorer outcome (47, 48).
Due to the strict exclusion criteria and the very time-consuming methods and analyses, our study population was rather small and random errors cannot be ruled out. Another limitation might be that analyses of the flow were performed semiquantitatively.
The microcirculation is influenced by various factors, such as hematocrit and hemoglobin level, incubator temperature, blood transfusions, infections, PDA, and gender (16, 18, 24, 28, 31, 49–52). There were no significant differences in our groups concerning these possible confounders. Blood pressure and HR showed no significant or only individual influence on the microcirculation in previous studies (30, 53) and were similar in both groups. Catecholamine treatment has been shown to increase FVD in premature infants (25), which might be a possible confounder in our results. Catecholamine treatment appeared to occur more frequently in the CG than the HTG, although this has not been statistically significant. However, catecholamines were given only for a few days. Effects of catecholamine treatment on the microcirculation have been demonstrated only in the first 6 h after birth and not after discontinuation of the treatment. Therefore, we do not think that catecholamine treatment confounded our results, showing long-term reduction in FVD seen in the HTG.
Another possible limitation is the lack of measurements between the seventh and the fourteenth day. We had decided beforehand to concentrate on the immediate effects after elevation of PCO2 as well as the long-term effects under treatment with permissive hypercapnia and not to focus on single data points.
Vascular development is a key process in lung development, and impaired vascular development has been linked to the emergence of BPD. Due to our small patient group, we were unable to detect significant differences in clinical outcome. However, if we look at the missing benefit or even worsened pulmonary outcome of the patients of the HTG group in the PHELBI trial (10), we speculate that the pulmonary outcome may have been linked to the differences in microcirculation. If lung vessels respond in the same manner as skin vessels, the reduced vessel density after prolonged hypercapnia observed in this study may be one possible additional mechanism why permissive hypercapnia as performed in this study has not fulfilled the hope of reducing the incidence of BPD (10). Although flow was not significantly impaired in our study, which might be due to the small number of patients, a reduction in FVD results in a pronounced transformation of microvascular architecture, which could be an important pathophysiological mechanism in the genesis of BPD.
In conclusion, our study showed a significant decrease in FVD after long-term treatment with high PCO2 targets, possibly due to flow redistribution toward larger vessels in deeper areas. The microcirculation is a sensitive parameter that reacts to changes in multiple diseases or conditions. In our case, it provided a different perspective on the benefits and disadvantages reported under permissive hypercapnia (9) and should be further explored in larger studies.
Ethics Statement
This study was carried out in accordance with the recommendations of the institutional review board of the Ludwig Maximilians University with written informed consent from all subjects. All subjects gave written informed consent in accordance with the Declaration of Helsinki. The protocol was approved by the institutional review board of the Ludwig Maximilians University.
Author Contributions
AP-S, KS, ZM, OG, and UT conceived and planned the trial and the measurements. KS and ZM carried out the measurements. KS did the analysis of the microcirculatory videos and clinical data. AP-S, KS, ZM, OG, JD, and UT contributed to the interpretation of the results. JD, UT, and AP-S performed the statistical analyses. AP-S took the lead in writing the manuscript. All authors provided critical feedback and helped shape the investigations, analyses, and the manuscript.
Conflict of Interest Statement
The authors declare that the research was conducted in the absence of any commercial or financial relationships that could be construed as a potential conflict of interest.
Acknowledgments
The study was part of the dissertation of KS.
Funding
The main trial was funded by taxpayer funds through the DFG (Deutsche Forschungsgemeinschaft DFG TH626-5/1).
Abbreviations
A/C, assisted/controlled; AUC, area under the curve; BPD, bronchopulmonary dysplasia; ELBI, extremely low birth weight infant; SDF, sidestream dark field; FVD, functional vessel density; HTG, high PCO2 target group; HFO, high-frequency oscillation; HR, heart rate; IVH, intraventricular hemorrhage; MAP, mean airway pressure; CG, control group; OPS, orthogonal polarization spectral; PDA, persistent ductus arteriosus; PEEP, positive end-expiratory pressure; PIP, positive inspiratory pressure; SIMV, synchronized intermittent mandatory ventilation; VS, vessel surface.
References
1. Christou H, Brodsky D. Lung injury and bronchopulmonary dysplasia in newborn infants. J Intensive Care Med (2005) 20(2):76–87. doi:10.1177/0885066604273494
2. Suguihara C, Lessa AC. [Strategies to minimize lung injury in extremely low birth weight infants]. J Pediatr (Rio J) (2005) 81(1 Suppl):S69–78.
3. Hagen EW, Sadek-Badawi M, Carlton DP, Palta M. Permissive hypercapnia and risk for brain injury and developmental impairment. Pediatrics (2008) 122(3):e583–9. doi:10.1542/peds.2008-1016
4. Laffey JG, Honan D, Hopkins N, Hyvelin JM, Boylan JF, McLoughlin P. Hypercapnic acidosis attenuates endotoxin-induced acute lung injury. Am J Respir Crit Care Med (2004) 169(1):46–56. doi:10.1164/rccm.200205-394OC
5. Laffey JG, Jankov RP, Engelberts D, Tanswell AK, Post M, Lindsay T, et al. Effects of therapeutic hypercapnia on mesenteric ischemia-reperfusion injury. Am J Respir Crit Care Med (2003) 168(11):1383–90. doi:10.1164/rccm.2108078
6. Mariani G, Cifuentes J, Carlo WA. Randomized trial of permissive hypercapnia in preterm infants. Pediatrics (1999) 104(5 Pt 1):1082–8. doi:10.1542/peds.104.5.1082
7. Sinclair SE, Kregenow DA, Lamm WJ, Starr IR, Chi EY, Hlastala MP. Hypercapnic acidosis is protective in an in vivo model of ventilator-induced lung injury. Am J Respir Crit Care Med (2002) 166(3):403–8. doi:10.1164/rccm.200112-117OC
8. Stark AR. High-frequency oscillatory ventilation to prevent bronchopulmonary dysplasia – are we there yet? N Engl J Med (2002) 347(9):682–4. doi:10.1056/NEJMe020080
9. Thome UH, Carroll W, Wu TJ, Johnson RB, Roane C, Young D, et al. Outcome of extremely preterm infants randomized at birth to different PaCO2 targets during the first seven days of life. Biol Neonate (2006) 90(4):218–25. doi:10.1159/000092723
10. Thome UH, Genzel-Boroviczeny O, Bohnhorst B, Schmid M, Fuchs H, Rohde O, et al. Permissive hypercapnia in extremely low birthweight infants (PHELBI): a randomised controlled multicentre trial. Lancet Respir Med (2015) 3(7):534–43. doi:10.1016/S2213-2600(15)00204-0
11. Network SSGotEKSNNRCarlo WA, Finer NN, Walsh MC, Rich W, Gantz MG, et al. Target ranges of oxygen saturation in extremely preterm infants. N Engl J Med (2010) 362(21):1959–69. doi:10.1056/NEJMoa0911781
12. Fabres J, Carlo WA, Phillips V, Howard G, Ambalavanan N. Both extremes of arterial carbon dioxide pressure and the magnitude of fluctuations in arterial carbon dioxide pressure are associated with severe intraventricular hemorrhage in preterm infants. Pediatrics (2007) 119(2):299–305. doi:10.1542/peds.2006-2434
13. Kaiser JR, Gauss CH, Williams DK. The effects of hypercapnia on cerebral autoregulation in ventilated very low birth weight infants. Pediatr Res (2005) 58(5):931–5. doi:10.1203/01.pdr.0000182180.80645.0c
14. Kaiser JR, Tilford JM, Simpson PM, Salhab WA, Rosenfeld CR. Hospital survival of very-low-birth-weight neonates from 1977 to 2000. J Perinatol (2004) 24(6):343–50. doi:10.1038/sj.jp.7211113
15. Miller JD, Carlo WA. Safety and effectiveness of permissive hypercapnia in the preterm infant. Curr Opin Pediatr (2007) 19(2):142–4. doi:10.1097/MOP.0b013e3280895e12
16. Harkin CP, Schmeling WT, Kampine JP, Farber NE. The effects of hyper- and hypocarbia on intraparenchymal arterioles in rat brain slices. Neuroreport (1997) 8(8):1841–4. doi:10.1097/00001756-199705260-00010
17. Bereczki D, Wei L, Otsuka T, Hans FJ, Acuff V, Patlak C, et al. Hypercapnia slightly raises blood volume and sizably elevates flow velocity in brain microvessels. Am J Physiol (1993) 264(5 Pt 2):H1360–9.
18. Hudetz AG, Biswal BB, Feher G, Kampine JP. Effects of hypoxia and hypercapnia on capillary flow velocity in the rat cerebral cortex. Microvasc Res (1997) 54(1):35–42. doi:10.1006/mvre.1997.2023
19. De Backer D, Creteur J, Preiser JC, Dubois MJ, Vincent JL. Microvascular blood flow is altered in patients with sepsis. Am J Respir Crit Care Med (2002) 166(1):98–104. doi:10.1164/rccm.200109-016OC
20. Sakr Y, Dubois MJ, De Backer D, Creteur J, Vincent JL. Persistent microcirculatory alterations are associated with organ failure and death in patients with septic shock. Crit Care Med (2004) 32(9):1825–31. doi:10.1097/01.CCM.0000138558.16257.3F
21. Chierego M, Verdant C, De Backer D. Microcirculatory alterations in critically ill patients. Minerva Anestesiol (2006) 72(4):199–205.
22. Elbers PW, Ince C. Mechanisms of critical illness – classifying microcirculatory flow abnormalities in distributive shock. Crit Care (2006) 10(4):221. doi:10.1186/cc4568
23. Verdant C, De Backer D. How monitoring of the microcirculation may help us at the bedside. Curr Opin Crit Care (2005) 11(3):240–4. doi:10.1097/01.ccx.0000158849.94225.11
24. Hiedl S, Schwepcke A, Weber F, Genzel-Boroviczeny O. Microcirculation in preterm infants: profound effects of patent ductus arteriosus. J Pediatr (2010) 156(2):191–6. doi:10.1016/j.jpeds.2009.08.034
25. Schwepcke A, Weber FD, Mormanova Z, Cepissak B, Genzel-Boroviczeny O. Microcirculatory mechanisms in postnatal hypotension affecting premature infants. Pediatr Res (2013) 74(2):186–90. doi:10.1038/pr.2013.78
26. Goedhart PT, Khalilzada M, Bezemer R, Merza J, Ince C. Sidestream Dark Field (SDF) imaging: a novel stroboscopic LED ring-based imaging modality for clinical assessment of the microcirculation. Opt Express (2007) 15(23):15101–14. doi:10.1364/OE.15.015101
27. Groner W, Winkelman JW, Harris AG, Ince C, Bouma GJ, Messmer K, et al. Orthogonal polarization spectral imaging: a new method for study of the microcirculation. Nat Med (1999) 5(10):1209–12. doi:10.1038/13529
28. Harris AG, Sinitsina I, Messmer K. Validation of OPS imaging for microvascular measurements during isovolumic hemodilution and low hematocrits. Am J Physiol Heart Circ Physiol (2002) 282(4):H1502–9. doi:10.1152/ajpheart.00475.2001
29. Mathura KR, Vollebregt KC, Boer K, De Graaff JC, Ubbink DT, Ince C. Comparison of OPS imaging and conventional capillary microscopy to study the human microcirculation. J Appl Physiol (2001) 91(1):74–8. doi:10.1152/jappl.2001.91.1.74
30. Genzel-Boroviczeny O, Strotgen J, Harris AG, Messmer K, Christ F. Orthogonal polarization spectral imaging (OPS): a novel method to measure the microcirculation in term and preterm infants transcutaneously. Pediatr Res (2002) 51(3):386–91. doi:10.1203/00006450-200203000-00019
31. Weidlich K, Kroth J, Nussbaum C, Hiedl S, Bauer A, Christ F, et al. Changes in microcirculation as early markers for infection in preterm infants – an observational prospective study. Pediatr Res (2009) 66(4):461–5. doi:10.1203/PDR.0b013e3181b3b1f6
32. Cevese A. Coronary circulation: nitric oxide and hypercapnic acidosis. Cardiovasc Res (2005) 66(1):7–8. doi:10.1016/j.cardiores.2005.01.022
33. Fries M, Weil MH, Sun S, Huang L, Fang X, Cammarata G, et al. Increases in tissue Pco2 during circulatory shock reflect selective decreases in capillary blood flow. Crit Care Med (2006) 34(2):446–52. doi:10.1097/01.CCM.0000196205.23674.23
34. Hickling KG, Joyce C. Permissive hypercapnia in ARDS and its effect on tissue oxygenation. Acta Anaesthesiol Scand Suppl (1995) 107:201–8. doi:10.1111/j.1399-6576.1995.tb04359.x
35. Kluess HA, Buckwalter JB, Hamann JJ, Clifford PS. Acidosis attenuates P2X purinergic vasoconstriction in skeletal muscle arteries. Am J Physiol Heart Circ Physiol (2005) 288(1):H129–32. doi:10.1152/ajpheart.00574.2004
36. Komori M, Takada K, Tomizawa Y, Nishiyama K, Kawamata M, Ozaki M. Permissive range of hypercapnia for improved peripheral microcirculation and cardiac output in rabbits. Crit Care Med (2007) 35(9):2171–5. doi:10.1097/01.ccm.0000281445.77223.31
37. Malvin G. Microcirculatory effects of hypoxic and hypercapnic vasoconstriction in frog skin. Am J Physiol (1993) 264(2 Pt 2):R435–9.
38. Mazzoni MC, Cragoe EJ Jr, Arfors KE. Systemic blood acidosis in low-flow ischemia induces capillary luminal narrowing. Int J Microcirc Clin Exp (1994) 14(3):144–50. doi:10.1159/000178822
39. McGillivray-Anderson KM, Faber JE. Effect of acidosis on contraction of microvascular smooth muscle by alpha 1- and alpha 2-adrenoceptors. Implications for neural and metabolic regulation. Circ Res (1990) 66(6):1643–57. doi:10.1161/01.RES.66.6.1643
40. Nakanishi T, Gu H, Momma K. Effect of acidosis on contraction, intracellular pH and calcium in the rabbit mesenteric small artery. J Mol Cell Cardiol (1996) 28(8):1715–26. doi:10.1006/jmcc.1996.0161
41. Nakanishi T, Gu H, Momma K. Developmental changes in the effect of acidosis on contraction, intracellular pH, and calcium in the rabbit mesenteric small artery. Pediatr Res (1997) 42(6):750–7. doi:10.1203/00006450-199712000-00006
42. Okazaki K, Endou M, Okumura F. Involvement of barium-sensitive K+ channels in endothelium-dependent vasodilation produced by hypercapnia in rat mesenteric vascular beds. Br J Pharmacol (1998) 125(1):168–74. doi:10.1038/sj.bjp.0702048
43. Wang X, Wu J, Li L, Chen F, Wang R, Jiang C. Hypercapnic acidosis activates KATP channels in vascular smooth muscles. Circ Res (2003) 92(11):1225–32. doi:10.1161/01.RES.0000075601.95738.6D
44. De Backer D, Donadello K, Taccone FS, Ospina-Tascon G, Salgado D, Vincent JL. Microcirculatory alterations: potential mechanisms and implications for therapy. Ann Intensive Care (2011) 1(1):27. doi:10.1186/2110-5820-1-27
45. Brian JE Jr, Faraci FM, Heistad DD. Recent insights into the regulation of cerebral circulation. Clin Exp Pharmacol Physiol (1996) 23(6–7):449–57. doi:10.1111/j.1440-1681.1996.tb02760.x
46. Wasserman AJ, Patterson JL Jr. The cerebral vascular response to reduction in arterial carbon dioxide tension. J Clin Invest (1961) 40:1297–303. doi:10.1172/JCI104359
47. Buchele GL, Ospina-Tascon GA, De Backer D. How microcirculation data have changed my clinical practice. Curr Opin Crit Care (2007) 13(3):324–31. doi:10.1097/MCC.0b013e3280c1e5c5
48. De Backer D, Ospina-Tascon G, Salgado D, Favory R, Creteur J, Vincent JL. Monitoring the microcirculation in the critically ill patient: current methods and future approaches. Intensive Care Med (2010) 36(11):1813–25. doi:10.1007/s00134-010-2005-3
49. Perera P, Kurban AK, Ryan TJ. The development of the cutaneous microvascular system in the newborn. Br J Dermatol (1970) 82:86–91. doi:10.1111/j.1365-2133.1970.tb07993.x
50. Genzel-Boroviczeny O, Christ F, Glas V. Blood transfusion increases functional capillary density in the skin of anemic preterm infants. Pediatr Res (2004) 56(5):751–5. doi:10.1203/01.PDR.0000141982.38959.10
51. Genzel-Boroviczeny O, Seidl T, Rieger-Fackeldey E, Abicht J, Christ F. Impaired microvascular perfusion improves with increased incubator temperature in preterm infants. Pediatr Res (2007) 61(2):239–42. doi:10.1203/pdr.0b013e31802d77a2
52. Stark MJ, Clifton VL, Wright IM. Sex-specific differences in peripheral microvascular blood flow in preterm infants. Pediatr Res (2008) 63(4):415–9. doi:10.1203/01.pdr.0000304937.38669.63
Keywords: microcirculation, hypercapnia, extremely low birth weight infants, functional vessel density, skin perfusion
Citation: Puchwein-Schwepcke AF, Schottmayer K, Mormanová Z, Dreyhaupt J, Genzel-Boroviczeny O and Thome UH (2018) Permissive Hypercapnia Results in Decreased Functional Vessel Density in the Skin of Extremely Low Birth Weight Infants. Front. Pediatr. 6:52. doi: 10.3389/fped.2018.00052
Received: 23 December 2017; Accepted: 23 February 2018;
Published: 13 March 2018
Edited by:
Eugene Dempsey, University College Cork, IrelandReviewed by:
Gerhard Pichler, Medizinische Universität Graz, AustriaKai König, Medicum Wesemlin, Switzerland
Copyright: © 2018 Puchwein-Schwepcke, Schottmayer, Mormanová, Dreyhaupt, Genzel-Boroviczeny and Thome. This is an open-access article distributed under the terms of the Creative Commons Attribution License (CC BY). The use, distribution or reproduction in other forums is permitted, provided the original author(s) and the copyright owner are credited and that the original publication in this journal is cited, in accordance with accepted academic practice. No use, distribution or reproduction is permitted which does not comply with these terms.
*Correspondence: Alexandra Francoise Puchwein-Schwepcke, YWxleGFuZHJhLnNjaHdlcGNrZUBtZWQudW5pLW11ZW5jaGVuLmRl