- 1Center for Pediatrics, Department of Neonatology, Faculty of Medicine, Medical Center - University of Freiburg, Freiburg, Germany
- 2Institute of Virology, Faculty of Medicine, Medical Center - University of Freiburg, Freiburg, Germany
- 3Institute for Infection Prevention and Hospital Epidemiology, Faculty of Medicine, Medical Center - University of Freiburg, Freiburg, Germany
- 4Department of Obstetrics and Gynecology, Faculty of Medicine, Medical Center - University of Freiburg, Freiburg, Germany
- 5Institute of Medical Microbiology and Hygiene, Faculty of Medicine, Medical Center - University of Freiburg, Freiburg, Germany
Background: Human milk (HM) for preterm infants will often be pasteurized for cytomegalovirus (CMV) inactivation and reduction of its bacterial count. High-temperature short-time (HTST) treatment compared to standard Holder pasteurization (HoP) reduces the impact of heat treatment on bioactive HM proteins while effectively inactivating CMV. No data are available for the efficacy of bacterial count reduction using HTST treatments that are available for clinical use.
Objective: To test the antiviral and antibacterial efficacy of HTST treatment protocols in HM using a modified HTST treatment device compared to standard HoP.
Methods: Holder pasteurized 95 mL HM samples were inoculated with Staphylococcus aureus (ATCC 6538), Enterococcus faecalis (ATCC 29212), Pseudomonas aeruginosa (ATCC 27853), Serratia marcescens (Smarc 00697), two different strains of Klebsiella pneumoniae (ATCC 700603 and Kpn 01605) or spiked with 2 × 105 50% tissue culture infective dose of CMV (AD169) and subsequently subjected to HoP (62.5°C/30 min) or HTST treatment (62°C/5 s, 62°C/15 s, 72°C/5 s, 72°C/15 s, 87°C/2 s, and 87°C/5 s). Bacterial count was determined after treated HM was cultured for 24 h. CMV infectivity was determined by the number of specific CMV immediate early antigen stained nuclei after inoculating human fibroblasts with appropriately prepared HM samples.
Results: Holder pasteurized samples revealed no growth after 24 h incubation. Viable bacterial cultures were retrieved from all tested strains after HTST treatment with the default HTST protocol (62°C/5 s) that is available for clinical use. Using other time-temperature combinations, growth rates of S. aureus, E. faecalis, P. aeruginosa, K. pneumoniae, K. pneumonia, and S. marcescens were depending on treatment time, treatment temperature, bacterial genera and strain. Only after treatment temperatures above 72°C no bacterial growth was observed. CMV was inactivated by any tested time-temperature combination.
Conclusions: HTST treatment inactivates CMV in 95 mL HM samples but is less effective than HoP in bacterial count reduction at a time-temperature combination of 62°C/5 s. For a reliable bacterial count reduction HTST treatment at 87°C was required in this study.
Introduction
Human milk (HM) is naturally colonized with various microbiota but its content may increase and diversify during HM handling routines in a neonatal intensive care setting (1). HM may also serve as a vector for cytomegalovirus (CMV) which is frequently reactivated in the mammary gland of the CMV seropositive lactating mother and transmitted via HM (2). HM acquired bacterial sepsis and postnatal CMV infection has been observed in preterm infants, displaying various degrees of illness from clinically unapparent infection to septicaemia and death (3). Hence, screening of maternal CMV serostatus and bacterial HM content is performed in many neonatal units (4–7). In case of suspected CMV shedding, HM for preterm infants may either be freeze-thawed or pasteurized for CMV inactivation, temporarily withheld or even discarded within some neonatal units (7). Similar strategies are pursed for bacterial HM content that is exceeding certain threshold levels or includes certain bacterial genera or species (6). Holder pasteurization (HoP, 62.5°C/30 min) which has shown to eliminate most life forms of HM microbiota will be applied by most neonatal units (6–8). However, HoP has an adverse effect on bioactive HM content and may adversely influence the immunocompetence of the preterm infant (9). In contrast, high-temperature short-time treatment (HTST, e.g., 62°C/5 s) preserves bioactive HM proteins compared to Holder pasteurization while effectively inactivating CMV (10). However, data to support the use of HTST treatment to reduce the bacterial HM content are limited and mostly generated by experimental pasteurizers that are not available for practical clinical use (10–13). The aim of this study was to test the antimicrobial efficacy of a HTST treatment system that is available for clinical use compared to standard HoP in artificially inoculated HM.
Materials and Methods
Human Milk Sampling
We obtained HM samples from three mothers whose infants were treated in a German tertiary neonatal care unit, written informed consent was obtained from the donating mothers. Two of the donors were CMV seronegative as documented in their prenatal care documentation, one was CMV seropositive. Their expressed HM exceeded their infant's enteral nutrition requirements and was stored frozen in our institutional milk bank. Donors did not receive any antibiotics for at least 4 weeks prior expressing their HM.
Human Milk Preparation for Bacterial Inoculation
HM samples from the CMV seropositive donor, frozen at −22°C, were thawed overnight at 4°C and Holder pasteurized for 30 min holding time in a water bath at a plateau temperature of 62 ± 0.5°C using a LABU Muttermilchpasteur 40 (Labu Buchrucker, Ottensheim, Austria) to eliminate any potentially colonizing HM bacteria. Immediately after the heat treatment, samples of 95 mL were prepared, and microbial cultures were performed as detailed below.
Bacteria for artificial inoculation of HM were cultured on blood agar at 36 ± 0.5°C overnight (Thermo Fisher, Waltham, MA). Thereafter colonies were suspended in 0.9% sodium chloride and enumerated using the optical density method with a turbidity meter at 620 nm (Dade Behring, Sacramento, CA). Concentration of the bacterial suspension was 1 × 108 cfu/mL of which 95 μl was added to a 95 mL HM sample resulting in an inoculation dose of 1 × 105 cfu/mL. Seventy-six HM samples of 95 mL were subsequently inoculated with either Staphylococcus aureus (ATCC 6538, n = 16), Enterococcus faecalis (ATCC 29212, n = 16), Pseudomonas aeruginosa (ATCC 27853, n = 12), Klebsiella pneumoniae (ATCC 700603, n = 11), Serratia marcescens (Smarc 00697, n = 12) or Klebsiella pneumoniae (Kpn 01605, n = 9). Those aliquots were then subjected to heat treatment immediately after inoculation.
The selection of tested bacterial genera and species was based on culture results from the routine HM surveillance screening of our institutional milk bank or based on clinical isolates (Smarc 00697, Kpn 01605) obtained at our neonatal unit.
Human Milk Preparation for Viral Inoculation
HM samples of the two CMV seronegative donors, frozen at −22°C, were thawed overnight at 4°C. All samples were pooled, and one unpasteurized aliquot of the pooled samples was analyzed by CMV-PCR (RealStar, altona Diagnostics, Hamburg, Germany) to confirm that donors did not excrete CMV via HM and one further unpasteurized aliquot of the CMV seropositive donor was accordingly analyzed. Sixteen aliquots of 95 mL milk were prepared and spiked with 2 × 105 50% tissue infective doses per mL/HM of cell-free culture supernatant of CMV laboratory strain AD169. An unpasteurized CMV spiked HM aliquot and an unpasteurized sample of the CMV seropositive donor served as positive reference samples for the CMV cultures. One aliquot of the seronegative donors that was not inoculated with CMV served as an unpasteurized negative reference sample for the CMV cultures. Positive and negative control samples were equally subjected to milk processing for viral analysis (Figure 1).
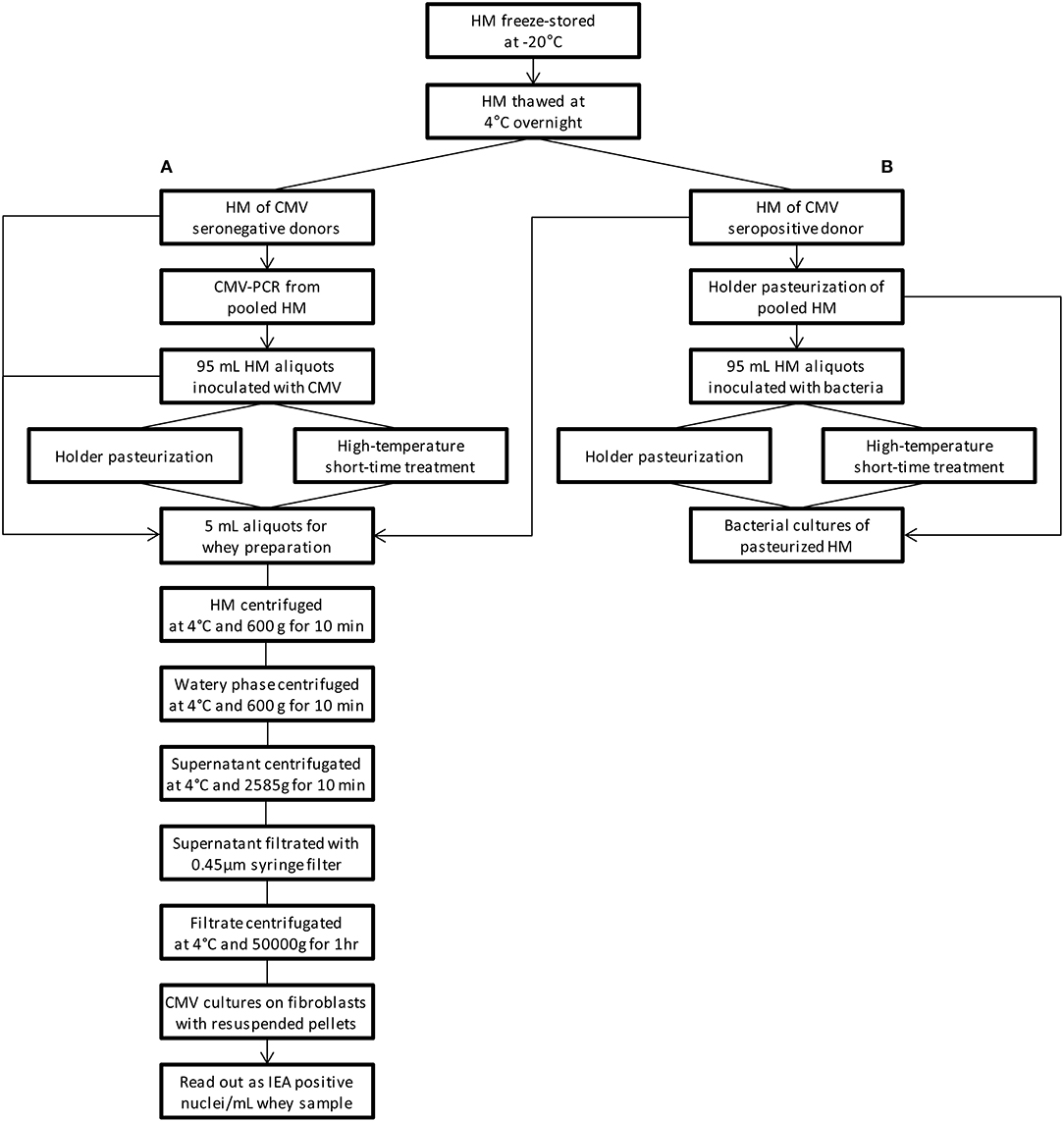
Figure 1. Flowchart of the study procedure. (A) viral studies, (B) bacterial studies. PCR-Studies of CMV the seropositive donor are not depicted. HM human milk, CMV cytomegalovirus, IEA immediate early antigen.
High-Temperature Short-Time Treatment
High-temperature short-time treatment was performed using a Virex II (Lauf, Tübingen, Germany) as described in detail elsewhere (13). For this study this machine was modified to allow heat treatment not only at the usually non-adjustable default setting (62°C/5 s) but also at various adjustable temperatures (62, 72, and 87°C) and time (2, 5, and 15 s) combinations. To increase the applicability of HTST treatment in a human milk bank setting, the bulk volume was increased from the default volume (50 mL) to 95 mL.
The 95 mL HM samples that were inoculated with bacteria or spiked with CMV were successively placed in a rotating glass flask. The resulting thin milk layer was heated by hot air to a plateau temperature of either 62°C for a holding time of 2 s (CMV only), 5 s and 15 s or heated to 72°C for 5 and 15 s or to 87°C for 2 and 5 s (CMV not tested at 87°C), respectively (Table 1). Afterwards, the glass flask was rapidly cooled with water at 14°C.
Each HTST treatment for a tested time-temperature combination and for a given bacterial species as well as the CMV spiked samples was repeated two fold. Time-temperature curves of every HTST cycle were measured with a sample-frequency of 10 Hz and digitally recorded on an external hard drive. The device was cleaned according to the manufacturer's specification in between the treatments to avoid cross-contamination. Immediately after completion of the heat treatment two agar discs were inoculated with 100 μL pasteurized HM of each sample. The viral samples were stored at 4°C until further processing.
Holder Pasteurization
Three of each 95 mL HM samples that were incubated with different bacterial strains or spiked with CMV as detailed above were subjected to Holder pasteurization at 62.5 ± 0.5°C/30 min using the clinitherm Pasteur 40 (Barkey, Leopoldshöhe, Germany). Immediately after completion of the pasteurization process 100 μL of the HM samples were inoculated onto agar disks, the CMV spiked samples were stored at 4°C until further processing.
Human Milk Processing for Viral Analysis
From each of the three Holder pasteurized CMV spiked samples, 12 HTST pasteurized CMV samples, the unpasteurized CMV spiked samples and the donors' unpasteurized positive and negative reference samples, two 5 mL aliquots (n = 36) were prepared using a Falcon test tube (BD, New Jersey, NJ). To separate the milk fractions aliquots were centrifuged at 4°C and 600 g for 10 min (Centrifuge 5810 R, Eppendorf, Hamburg, Germany). The resulting aqueous layer was centrifuged at 4°C and 600 g for 10 min and the subsequently resulting whey supernatant was further centrifuged at 4°C and 2585 g for 10 min. The resulting whey supernatant was filtrated with the Arodisc Syringe Filter 0,45 μm with Supor Membrane (Pall, NY, NY) and then subjected to ultracentrifugation 4°C and 50,000 g for 60 min (Optima MAX XP, Beckmann Coulter, Brea, CA). The resulting pellet was resuspended in 250 μl tissue culture medium and used to quantify CMV infectivity.
Detection of CMV Infectivity
Human foreskin fibroblasts were seeded into 24 well plates (Falcon, BD, New Jersey, NJ) and were maintained in minimal essential medium until further use (Gibco MEM supplemented with 5% fetal calf serum, Thermo Fischer). The medium was removed before inoculation and 100 μL of processed HM specimen was added to each well. Inoculated plates were centrifuged at 4°C and 900 g for 50 min (Centrifuge 5810 R, Eppendorf, Hamburg, Germany).
After centrifugation virus inoculum was removed, 1 mL of minimal essential medium was added to each well and the cultures were incubated at 37°C and 5% carbon dioxide for 18 h. Afterwards, monolayers were washed twice in phosphate-buffered saline and fixed with ice-cold acetone for 20 min. Cell monolayers were then incubated with an anti-CMV immediate early antigen (IEA) monoclonal antibody (bioMérieux, Lyon, France) and, after washing, with a fluorescein isothiocyanate-labeled goat anti-mouse immunoglobulin G conjugate (Agilent, Santa Clara, CA). Read-out was the number of CMV specific IEA stained nuclei/mL whey. Duplicates for each sample were analyzed.
Bacterial Count Analysis
We inoculated Columbia blood agar with 5% sheep blood (Thermo Fisher) with 100 μL of the pasteurized whole milk samples. Duplicate plates for each sample were inoculated and counted in all instances. The agar were incubated for 24 h at 36 ± 0.5°C and 5% carbon dioxide in a Heracell incubator (Thermo Fisher) before colony-forming units per milliliter (cfu/mL) were determined with a lower limit of detection of < 10 cfu/mL.
Data Analysis and Statistics
Bacterial HM concentration was determined as cfu/mL; antibacterial efficacy is reported as raw values and log reduction rates. Bacterial counts were transformed to log10 values for statistical analysis. A one-sample t-test was employed to compare the reduction in the HTST treatment with the complete reduction observed in the HoP treatment. Statistical analyses were performed using GraphPad Prism (V5.02, GraphPad, San Diego, CA). A p-value < 0.05 was considered significant.
Results
A flowchart summarizing the study procedure is given in Figure 1. Characteristic time-temperature curves of the HTST pasteurization process at of 62 and 72°C/15 s are shown in Figure 2.
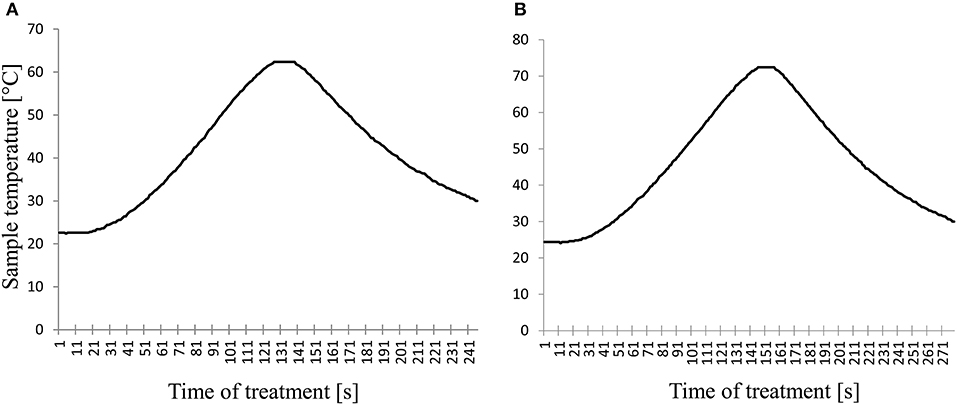
Figure 2. Characteristic time-temperature curves of the high-temperature short-time pasteurization processes. (A) time-temperature combination of 62°C/15 s; (B) time-temperature combination of 72°C/15 s.
No bacterial growth could be detected in any of the pooled and Holder pasteurized HM samples before experimental bacterial inoculation.
Bacterial concentrations in the inoculated 95 mL HM samples after culturing for 24 h were 7.9 × 104 cfu/mL for S. aureus, 1.16 × 105 cfu/mL for E. faecalis, 1 × 105 cfu/mL for P. aeruginosa, 1.04 × 105 cfu/mL for S. marcescens, 3.6 × 104 cfu/mL for K. pneumoniae (Kpn01605), and 5.7 × 104 cfu/mL for K. pneumoniae (ATCC 700603). Again, following HoP no bacterial growth for any of the tested bacterial species or strains could be detected (Table 1).
Viable cultures were retrieved from all tested strains after HTST pasteurization with the default HTST protocol of 62°C/5 s of S. aureus, E. faecalis, P. aeruginosa (p < 0.0001), and S. marcescens (p = 0.002). In general, growth rates after HTST pasteurization compared to HoP depended on treatment time, treatment temperature, bacterial genera, species and strain (Table 1). Positive bacterial cultures could be retrieved for any tested plateau temperature of < 87°C for the cultures incubated with S. aureus and E. faecalis albeit differences compared to HoP were not consistently statically significant (Table 1). The samples incubated with P. aeruginosa, K. pneumoniae and S. marcescens remained without detectable growth after pasteurization of at least 72°C/5 s. The two tested strains of Klebsiella did exhibit differing heat susceptibility (p = 0.029). All positive cultures revealed monomicrobial growth consisting of the respective incubated strain.
Number of CMV copies obtained by PCR from the CMV seropositive donors' milk was 7,000 IE/mL and mean (SD) number of IEA positive cells in the positive control sample from the CMV seropositive donor was 20 ± 0/mL. No CMV copies were obtained by PCR and no IEA positive cells could be detected in the negative control samples from the CMV seronegative donor. After experimental CMV spiking a mean (SD) of 32 ± 5.6 IEA positive cells/mL were detected before pasteurization. CMV was inactivated by HoP and HTST pasteurization at all tested time-temperature combinations, no IEA positive cells were found.
Discussion
This HTST treatment system was not as effective in bacterial count reduction as standard HoP at a time-temperature combination of 62°C/5 s. Colony counts of E. faecalis and P. aeruginosa were still present in abundance and counts of S. aureus and S. marcescens were reduced by more than two orders of magnitude but were still cultivable after exposure to 62°C/5 s. Only after an being exposed to 87°C all culture remained without growth.
Antibacterial efficacy of different types of HTST pasteurizers have been tested before at temperatures above 70°C and the choice of plateau temperatures within our study was modeled on these observations. Bacterial counts of naturally colonizing HM treated at 72 and 87°C for 1, 3, and 15 s was reduced by 83% to more than 99% (cfu/mL), respectively (12). Heating HM to 71°C/5.75 s, 71°C/9 s or 71°C/18.5 s in a continuous flow pasteurizer revealed more than 5 log reduction in HM artificially inoculated with S. aureus or E. coli, respectively (11). These observations are in line with our results, while temperatures as low as 62°C for bacterial count reduction in HM were not tested before.
The tested microbiota exhibited variable susceptibility to heat treatment, even between strains (8). This may explain the divergent results from previous studies where HTST treatment at 62°C/5 s of naturally colonized human milk was nearly as effective as HoP (10). Furthermore, this may result in less predictable pasteurization results if bacterial count reduction is pursued.
HTST treatment is currently applied in some neonatal units for CMV inactivation and bacterial count reduction while applying widely differing cut off values for HM microbiota content indicating pasteurization (4–7). Neonatologists that are intending to reduce bacterial HM content need to be aware of the potential limitation of HTST treatment systems in regard of their antibacterial efficacy compared to HoP. However, bacterial HM count reduction to decrease morbidity in preterm infants–namely from late-onset-sepsis and necrotising enterocolitis–remains debated because sound data to prove a beneficial influence of bacterial count reduction by pasteurization are missing (14–16).
Furthermore, any kind of heat treatment indiscriminately degrades cellular and non-cellular content of HM to a variable degree (17). Affected are both naturally colonizing HM bacteria and biologically active components that are involved in gut maturation and digestion, in shaping the infants immune system as well as its intestinal microbiome (18).
HTST has been shown to increase the retention rate of immunoglobulin A (10), lactoferrin (12, 19), and growth factors (20) amongst other proteins (21) compared to HoP. Therefore, HTST treatment as opposed to HoP of milk for preterm infants may be beneficial to improve neonatal outcome but clinical data are lacking so far. Nevertheless, HM should be subjected to the lowest energy intake possible to achieve a pursued aim, i.e., reducing bacterial count, inactivating virus or both.
CMV inactivation in HM for preterm infants is performed in many neonatal units to prevent HM acquired postnatal CMV infection (5–7). The original purpose of this tested HTST system with a non-adjustable default setting of 62°C/5 s is to inactivate CMV (13, 20). Viral inactivation is facilitated by rapidly heating a thin layer of HM within a rotating glass flask. We increased the default bulk of HM from 50 to 95 mL to advance the feasibility of HTST pasteurization in a human milk bank setting. Because of the resulting increase in thickness of the resulting fluid layer CMV inactivation had to be retested under this condition resulting in an effective CMV inactivation in 95 mL batches.
The apparent desire by some neonatologists to control bacterial content of HM should be considered when developing HM pasteurizers (22). Temperature-time combinations and modes of operation should be tested for its antibacterial efficacy (21) and guided by official regulations concerning pasteurization requirements (23). However, if exclusively CMV inactivation is pursued, a much lower energy intake may be used to treat HM and those requirements may not fit to the current definition of pasteurization of non-human milk (24).
Our study has some limitations. HTST pasteurizers utilized in previous studies were experimental prototypes with different modes of action (continuous flow vs. bulk pasteurization) and different modes of heating (plate heater exchanger vs. heating thermostat), this must be considered when comparing our findings to previous observations. We were not able to test all time-temperature combinations for every bacterium due to limited HM supply. Genera, species and strains other than those tested in our study may be present in HM. Heat susceptibility of those microbiota may be different than the ones tested, and the choice of different strains might have influenced our results. Within the study we exclusively tested HM inoculated with single strains of bacteria, but clinical HM isolates may contain multiple strains. We can only speculate if inoculation with multiple strains could have influenced our results. As different cultural agars exhibit varying recovery rates of heat-processed bacteria the choice of agar might have influenced our results (25). We chose to apply those agars used in the routine HM screening program of our neonatal unit. We did not test HM samples for the presence of bacterial growth inhibitors. Furthermore, different HM concentrations of antimicrobial proteins e.g., lactoferrin or immunoglobulins between donors could have influenced our results. However, there was no history of recent antibiotic treatment of donors and since HM was pooled results for both modes of pasteurization would have been equally affected.
Conclusions
This HTST treatment procedure inactivates CMV in 95 mL HM samples but is not as effective as standard HoP in bacterial count reduction at a time-temperature combination of 62°C/5 s.
Ethics Statement
This study was approved by the ethics committee of the Albert-Ludwigs-University of Freiburg, Germany (No. 184/15).
Author Contributions
DK conceived and designed the study, participated in the acquisition of the HM samples, performed the pasteurization process, analyzed the data, and wrote the first draft of the manuscript. MS designed the study, performed the pasteurization process, and contributed to drafting the manuscript. VF designed the study, performed the viral assays, and contributed to data analysis and interpretation. DJ designed the study, supervised the bacterial studies, and contributed to data analysis. MK contributed to acquisition of the HM samples and drafting of the manuscript. AW contributed to the study design and reviewed the manuscript. HF and RH contributed to the study design and drafting of the manuscript. All authors reviewed the manuscript.
Conflict of Interest Statement
The authors declare that the research was conducted in the absence of any commercial or financial relationships that could be construed as a potential conflict of interest.
Acknowledgments
The authors are indebted to Sabine Weber and Doris Scheibert for their assistance in performing the bacterial cultures and to Ariane Kaiser for her assistance in performing the viral assays.
Abbreviations
ATCC, American type culture collection; HM, human milk; cfu, colony-forming unit; CMV, Cytomegalovirus; HoP, Holder pasteurization; HTST, High-temperature short-time; PCR, polymerase chain reaction
References
1. Fitzstevens JL, Smith KC, Hagadorn JI, Caimano MJ, Matson AP, Brownell EA. Systematic review of the human milk microbiota. Nutr Clin Pract. (2017) 32:354–64. doi: 10.1177/0884533616670150
2. Hamprecht K, Maschmann J, Vochem M, Dietz K, Speer CP, Jahn G. Epidemiology of transmission of cytomegalovirus from mother to preterm infant by breastfeeding. Lancet (2001) 357:513–8. doi: 10.1016/S0140-6736(00)04043-5
3. Lanzieri TM, Dollard SC, Josephson CD, Schmid DS, Bialek SR. Breast milk-acquired cytomegalovirus infection and disease in VLBW and premature infants. Pediatrics (2013) 131:e1937–45. doi: 10.1542/peds.2013-0076
4. Omarsdottir S, Casper C, Akerman A, Polberger S, Vanpée M. Breastmilk handling routines for preterm infants in Sweden: a national cross-sectional study. Breastfeed Med. (2008) 3:165–70. doi: 10.1089/bfm.2007.0033
5. Cossey V, Johansson A-B, de Halleux V, Vanhole C. The use of human milk in the neonatal intensive care unit: practices in Belgium and Luxembourg. Breastfeed Med. (2012) 7:302–6. doi: 10.1089/bfm.2011.0112
6. Klotz D, Jansen S, Gebauer C, Fuchs H. Handling of breast milk by neonatal units: Large differences in current practices and beliefs. Front Pediatr. (2018) 6:235 doi: 10.3389/fped.2018.00235
7. Buxmann H, Falk M, Goelz R, Hamprecht K, Poets CF, Schloesser RL. Feeding of very low birth weight infants born to HCMV-seropositive mothers in Germany, Austria and Switzerland. Acta Paediatr. (2010) 99:1819–23. doi: 10.1111/j.1651-2227.2010.01954.x
8. Scientific Evaluation of Pasteurisation for Pathogen Reduction in Milk and Milk Products Scientific Evaluation . Available online at: https://www.foodstandards.gov.au/code/proposals/documents/Scientific%20Evaluation.pdf (Accessed November 16, 2017)
9. Ewaschuk JB, Unger S, O'Connor DL, Stone D, Harvey S, Clandinin MT, et al. Effect of pasteurization on selected immune components of donated human breast milk. J Perinatol. (2011) 31:593–8. doi: 10.1038/jp.2010.209
10. Klotz D, Joellenbeck M, Winkler K, Kunze M, Huzly D, Hentschel R. High temperature short time pasteurisation of human breast milk is efficient in retaining protein and reducing the bacterial count. Acta Paediatr. (2017) 106:763–7. doi: 10.1111/apa.13768
11. Dhar J, Fichtali J, Skura B j, Nakai S, Davidson A. Pasteurization efficiency of a HTST system for human milk. J Food Sci. (1996) 61:569–73. doi: 10.1111/j.1365-2621.1996.tb13160.x
12. Goldblum RM, Dill CW, Albrecht TB, Alford ES, Garza C, Goldman AS. Rapid high-temperature treatment of human milk. J Pediatr. (1984) 104:380–5.
13. Hamprecht K, Maschmann J, Müller D, Dietz K, Besenthal I, Goelz R, et al. Cytomegalovirus (CMV) inactivation in breast milk: reassessment of pasteurization and freeze-thawing. Pediatr Res. (2004) 56:529–35. doi: 10.1203/01.PDR.0000139483.35087.BE
14. Schanler RJ, Lau C, Hurst NM, Smith EO. Randomized trial of donor human milk versus preterm formula as substitutes for mothers' own milk in the feeding of extremely premature infants. Pediatrics (2005) 116:400–6. doi: 10.1542/peds.2004-1974
15. Cossey V, Vanhole C, Eerdekens A, Rayyan M, Fieuws S, Schuermans A. Pasteurization of mother's own milk for preterm infants does not reduce the incidence of late-onset sepsis. Neonatology (2013) 103:170–6. doi: 10.1159/000345419
16. Stock K, Griesmaier E, Brunner B, Neubauer V, Kiechl-Kohlendorfer U, Trawöger R. Pasteurization of breastmilk decreases the rate of postnatally acquired cytomegalovirus infections, but shows a nonsignificant trend to an increased rate of necrotizing enterocolitis in very preterm infants–a preliminary study. Breastfeed Med. (2015) 10:113–117. doi: 10.1089/bfm.2014.0108
17. Peila C, Moro GE, Bertino E, Cavallarin L, Giribaldi M, Giuliani F, et al. The effect of holder pasteurization on nutrients and biologically-active components in donor human milk: a review. Nutrients (2016) 8:477. doi: 10.3390/nu8080477
18. Le Doare K, Holder B, Bassett A, Pannaraj PS. Mother's Milk: A purposeful contribution to the development of the infant microbiota and immunity. Front Immunol. (2018) 9:361. doi: 10.3389/fimmu.2018.00361
19. Baro C, Giribaldi M, Arslanoglu S, Giuffrida MG, Dellavalle G, Conti A, et al. Effect of two pasteurization methods on the protein content of human milk. Front Biosci. (2011) 3:818–29. doi: 10.2741/289
20. Goelz R, Hihn E, Hamprecht K, Dietz K, Jahn G, Poets C, Elmlinger M. Effects of different CMV-heat-inactivation-methods on growth factors in human breast milk. Pediatr Res. (2009) 65:458–61. doi: 10.1203/PDR.0b013e3181991f18
21. Peila C, Emmerik NE, Giribaldi M, Stahl B, Ruitenberg JE, van Elburg RM, et al. Human milk processing: a systematic review of innovative techniques to ensure the safety and quality of donor milk. J Pediatr Gastroenterol Nutr. (2017) 64:353–61. doi: 10.1097/MPG.0000000000001435
22. Giribaldi M, Coscia A, Peila C, Antoniazzi S, Lamberti C, Ortoffi M, et al. Pasteurization of human milk by a benchtop high-temperature short-time device. Innov Food Sci Emerg Technol. (2016) 36:228–33. doi: 10.1016/j.ifset.2016.07.004
23. Commission Regulation (EU) No 605/2010 of 2 July 2010 Laying Down Animal and Public Health and Veterinary Certification Conditions for the Introduction into the European Union of Raw Milk and Dairy Products Intended for Human Consumption. Available online at: https://eur-lex.europa.eu/LexUriServ/LexUriServ.do?uri=OJ:L:2010:175:0001:0024:EN:PDF (Accessed October 12, 2018)
24. Food Agriculture Organization of the United Nations ed,. Code of Hygienic Practice for Milk Milk Products in Milk Milk Products. Rome: Food Agriculture Organization of the United Nations (2004). Available online at: http://www.fao.org/fao-who-codexalimentarius/sh-proxy/en/?lnk=1&url=https%253A%252F%252Fworkspace.fao.org%252Fsites%252Fcodex%252FStandards%252FCAC%2BRCP%2B57-2004%252FCXP_057e.pdf (Accessed October 12, 2018)
Keywords: bacteria, human milk, cytomegalovirus, holder pasteurization, high-temperature short-time, HTST treatment, preterm
Citation: Klotz D, Schreiner M, Falcone V, Jonas D, Kunze M, Weber A, Fuchs H and Hentschel R (2018) High-Temperature Short-Time Treatment of Human Milk for Bacterial Count Reduction. Front. Pediatr. 6:359. doi: 10.3389/fped.2018.00359
Received: 31 August 2018; Accepted: 05 November 2018;
Published: 27 November 2018.
Edited by:
Guido Eugenio Moro, Associazione Italiana delle Banche del Latte Umano Donato (AIBLUD), ItalyReviewed by:
David Lembo, Università degli Studi di Torino, ItalyJoseph M. Bliss, Women & Infants Hospital of Rhode Island, United States
Copyright © 2018 Klotz, Schreiner, Falcone, Jonas, Kunze, Weber, Fuchs and Hentschel. This is an open-access article distributed under the terms of the Creative Commons Attribution License (CC BY). The use, distribution or reproduction in other forums is permitted, provided the original author(s) and the copyright owner(s) are credited and that the original publication in this journal is cited, in accordance with accepted academic practice. No use, distribution or reproduction is permitted which does not comply with these terms.
*Correspondence: Daniel Klotz, ZGFuaWVsLmtsb3R6QHVuaWtsaW5pay1mcmVpYnVyZy5kZQ==