- 1Paediatric Critical Care Research Group, Queensland Children's Hospital, The University of Queensland, Brisbane, QLD, Australia
- 2School of Medicine, The University of Queensland, Brisbane, QLD, Australia
- 3Mater Research Institute, The University of Queensland, Brisbane, QLD, Australia
- 4Critical Care Research Group, Adult Intensive Care Service, The Prince Charles Hospital, Brisbane, QLD, Australia
- 5Paediatric Research in Emergency Departments International Collaborative (PREDICT), Parkville, VIC, Australia
- 6Royal Children's Hospital, Emergency Department, Melbourne, VIC, Australia
- 7Murdoch Children's Research Institute, Melbourne, VIC, Australia
- 8Department of Paediatrics, Faculty of Medicine, Dentistry and Health Sciences, University of Melbourne, Melbourne, VIC, Australia
- 9Department of Medicine, School of Clinical Sciences, Monash University, Clayton, VIC, Australia
- 10Monash Medical Centre, Emergency Department, Melbourne, VIC, Australia
- 11College of Medicine and Dentistry, James Cook University, Townsville, QLD, Australia
- 12The Townsville Hospital, Emergency Department, Townsville, QLD, Australia
- 13KidzFirst Middlemore Hospital, Auckland, New Zealand
- 14University of Auckland, Auckland, New Zealand
- 15Department of Paediatrics, Gold Coast University Hospital, Southport, QLD, Australia
- 16School of Medicine, Griffith University, Gold Coast, QLD, Australia
- 17Faculty of Health Sciences and Medicine, Bond University, Gold Coast, QLD, Australia
- 18Paediatric Department, Ipswich General Hospital, Ipswich, QLD, Australia
- 19Paediatric Department, Caboolture Hospital, Caboolture, QLD, Australia
- 20Paediatric Department, Redcliffe Hospital, Redcliffe, QLD, Australia
- 21Children's Emergency Department, Starship Children's Hospital, Auckland, New Zealand
- 22Liggins Institute, University of Auckland, Auckland, New Zealand
Background: Bronchiolitis is the most common reason for hospital admission in infants, with one third requiring oxygen therapy due to hypoxemia. It is unknown what proportion of hypoxemic infants with bronchiolitis can be managed with nasal high-flow in room air and their resulting outcomes.
Objectives and Settings: To assess the effect of nasal high-flow in room air in a subgroup of infants with bronchiolitis allocated to high-flow therapy in a recent multicenter randomized controlled trial.
Patients and Interventions: Infants allocated to the high-flow arm of the trial were initially treated with room air high-flow if saturations were ≥85%. Subsequently, if oxygen saturations did not increase to ≥92%, oxygen was added and FiO2 was titrated to increase the oxygen saturations. In this planned sub-study, infants treated during their entire hospital stay with high-flow room air only were compared to infants receiving either standard-oxygen or high-flow with oxygen. Baseline characteristics, hospital length of stay and length of oxygen therapy were compared.
Findings: In the per protocol analysis 64 (10%) of 630 infants commenced on high-flow room air remained in room air only during the entire stay in hospital. These infants on high-flow room air were on average older and presented with moderate hypoxemia at presentation to hospital. Their length of respiratory support and length of stay was also significantly shorter. No pre-enrolment factors could be identified in a multivariable analysis.
Conclusions: In a small sub-group of hypoxemic infants with bronchiolitis hypoxemia can be reversed with the application of high-flow in room air only.
Trial registration: ACTRN12615001305516
Introduction
The application of oxygen for hypoxemia represents one of the most common medical and nursing interventions globally (1). Traditionally, oxygen was considered a safe intervention, but an increasing body of evidence demonstrates potential for harm in acute care settings including perinatal adaptation (2, 3), cardiac arrest (4), and acute stroke (5). Adverse effects of oxygen may relate to toxicity of free oxygen radicals, and adaptive mechanisms in health and disease (6–8). As a result, the application of oxygen as a drug is undergoing more scrutiny. Furthermore, access to oxygen in remote facilities and in low income countries can be problematic (9, 10).
The oxygen requirement in acute respiratory diseases leading to hypoxemia depends on several pathophysiological factors such as ventilation inhomogeneity, atelectasis, or consolidated lung parenchyma, an increased intra pulmonary shunt fraction and decreased alveolar-capillary membrane diffusion capacity (11). Traditionally, the first approach to improve hypoxemia consisted in increasing inspired oxygen fraction to treat the symptom whilst simultaneously searching for the cause of the hypoxemia. If this approach is ineffective then non-invasive, mainly continuous positive airway pressure (CPAP) or invasive ventilation strategies are commonly used in an incremental fashion, though both are highly resource and skill dependent (12). In contrast to oxygen alone, alveolar recruitment by provision of CPAP is likely to reverse or improve many conditions that lead to hypoxemia in children, whereas restricted oxygen diffusion due to thickened alveolar-capillary membrane is less commonly a feature early in the disease process. The application of CPAP however can be challenging, requires highly trained nursing and medical staff, is not always tolerated by patients, and therefore has been in the past restricted to intensive care unit (ICU) settings.
Nasal high-flow therapy, as a new positive airway pressure modality has been rapidly adopted in ICU, and subsequently in emergency departments (EDs) and general wards because of its ease of use (13–15). Physiological and clinical studies demonstrate a CPAP effect at high-flow rates that exceed peak inspiratory flow (16–18).
We recently published the findings of the Pediatric Acute Respiratory Intervention (PARIS) randomized controlled trial (RCT), which randomized 1,472 hypoxemic infants with bronchiolitis to either nasal high-flow therapy or standard-oxygen therapy with the option to use rescue high-flow if infants failed standard-oxygen therapy (19). Nested within this RCT we addressed the pre-planned question whether high-flow therapy can normalize oxygenation without any increase in the FiO2 provided (20). We hypothesized that high-flow room air is sufficient to restore adequate oxygenation in a subset of infants with bronchiolitis presenting with hypoxemia.
Methods
The protocol, methods, and results of the RCT have been described previously (19, 20). Briefly, 1,472 infants, <12 months of age, admitted to 17 hospitals in Australia and New Zealand with bronchiolitis and an oxygen requirement were randomized in an open-label study design to treatment with standard subnasal oxygen or nasal high-flow (20). The primary outcome for the trial was treatment failure, defined by the presence of ≥3 of a possible 4 abnormal physiological parameters, and escalation of care. The trial confirmed that treatment failure and escalation of care occurred in fewer patients randomized to high-flow compared with standard-oxygen (p < 0.001). The trial was undertaken between October 2013 and August 2016, and the relevant human research ethics committee at each participating site approved the study (HREC/13/QRCH/93). Informed written consent was obtained by parent or guardian.
The primary objective of this pre-specified sub-study was to test if the oxygen requirement can be reversed in infants with bronchiolitis, by the provision of room air high-flow alone. For this purpose, the study protocol required that all infants, who were allocated to the high-flow arm, and with enrolment oxygen saturation between 85 and≤92/94%, to be initially commenced on high-flow room air (FiO2 0.21) at 2 L/kg/min via age-appropriate Optiflow JuniorTM cannula and Airvo2TM high-flow system (Fisher & Paykel Healthcare; Auckland, New Zealand). If high-flow room air had failed to achieve target saturations of ≥92% in participating tertiary children's hospitals, and ≥94% in secondary hospitals (saturation targets were set by individual institution's standard current practice and not specified centrally by the trial investigators) after 10 min the FiO2 of the high-flow system was increased to achieve the target saturation. High-flow room air was stopped (i.e., flow rate reduced from 2 to 0 L/kg/min without gradual reduction) as soon as clinicians saw clinical improvement and participant's oxygen saturation was maintained at ≥92/94% for at least 4 h. For those infants receiving nasal high-flow oxygen, the FiO2 was titrated to keep saturations between a 92/94 and 98% target, and once on high-flow room air for 4 h and maintaining target saturations, high-flow therapy was stopped. If infants subsequently developed a further oxygen requirement, according to their institution's definition, high-flow was instigated using the same procedures as at initiation of high-flow at randomization. Infants allocated to standard-oxygen therapy received 100% subnasal oxygen up to a maximum of 2 L/min to maintain oxygen saturations within the same targets as the high-flow arm. All other bronchiolitis specific therapies were at the discretion of the treating clinician.
Oxygen Therapy Groups (Figure 1)
We defined four oxygen therapy groups for this sub-study. Infants allocated to standard-oxygen remained on subnasal standard-oxygen (standard-oxygen group) unless they required escalation of care, which in most of these infants was achieved by using high-flow with oxygen. Infants allocated to high-flow with saturations <85% at randomization received immediate high-flow with oxygen (high-flow with immediate oxygen group). The threshold of 85% was chosen in consensus with the participating hospitals. Infants allocated to high-flow presenting with saturations between 85 and≤92/94% were started on high-flow room air. Infants without improved oxygenation (saturation≤92/94%) after 10 min on high-flow room air were switched to high-flow with oxygen (high-flow with oxygen group) whereas infants who improved their saturations to ≥92/94% remained on high-flow room air (high-flow room air group).
Study Outcomes
The primary outcomes were: first a proof of concept to demonstrate whether a subgroup of hypoxemic infants with bronchiolitis can be treated with high-flow room air only while maintaining their target saturations; and secondly to determine the proportion of hypoxemic infants with bronchiolitis that respond to high-flow in room air. Secondary outcomes included differences in basic demographics, risk factors such as prematurity and pre-existing disease, virus detected, severity of disease at randomization, length of hospital stay, and length of high-flow therapy. The physiological response of heart and respiratory rate to high-flow room air was analyzed for the first 3 days of admission. In one of the participating hospitals all data on the change of respiratory and heart rate, and saturation of all infants enrolled were also obtained relating to the first hour of oxygen therapy post start of the intervention (Table 2).
The sub-group of infants receiving high-flow room air only were compared to the three oxygen groups; infants on standard oxygen, infants on high-flow with immediate oxygen, and infants high-flow with oxygen.
Statistical Analysis
The sample size of 1,400 infants for the RCT was based on a 50% reduction in failure rate of oxygen therapy at 90% power and type I error of 0.05. Allocation to treatment arm was as the per protocol analysis. Continuous data are presented as mean and standard deviation (SD) or median and interquartile range (IQR) dependent on variable distribution; categorical data are presented using number and percentage. Comparisons between the treatment groups were made using ANOVA, Kruskal–Wallis or Fisher's exact tests; following a significant p-value (<0.05) post-hoc comparisons were undertaken comparing the in-room air group to the other treatment groups (adjustment using Bonferroni's correction). Further analysis was undertaken comparing high-flow in room air vs. a combined group of standard-oxygen and high-flow with oxygen. Bivariable analysis was initially undertaken and variables with a p <0.25 remained in the multivariable model. Comparison of infants in the standard oxygen group presenting with pre-enrolment saturations <85% to infants with saturations between 85 and≤92/94% were undertaken using t-tests, Mann–Whitney U-test and Fisher's exact test. Analyses were conducted in StataIC version 14.1 (StataCorp LP, College Station, Texas).
Results
In the original RCT, 1,472 infants with bronchiolitis were randomized and subsequently provided written consent; in the per protocol analysis 710 received standard-oxygen and 746 received high-flow of which 52 (7%) commenced on high-flow with immediate oxygen, 630 (70%) commenced high-flow room air with a later increase of FiO2 and 64 (9%) received high-flow room air (Figure 1). Table 1 shows the baseline characteristics of the infants in high-flow room air compared to infants on high-flow with immediate oxygen, high-flow with oxygen and infants treated with standard-oxygen therapy. There were a number of statistical differences in several of the pre-enrolment factors between the three study groups (Table 1): the distribution of age at enrolment was significantly different between the treatment groups with the proportion of infants <3 months of age lowest in the high-flow room air (17.2%), the body weight was highest in the high-flow room air group, followed by the standard-oxygen group and then high-flow in oxygen. Infants on high-flow with immediate oxygen received more frequently antibiotics and sedation. Infants in the high-flow room air group showed higher oxygen saturation pre-enrolment than infants in the other groups (Table 2). In the cohort of infants (n=334) in which physiological parameters were measured within the first hour of treatment, infants on standard-oxygen showed the highest saturations. The changes in saturation, heart and respiratory rate in infants on high-flow room air are shown for the first 72 h in Figures 2, 3.
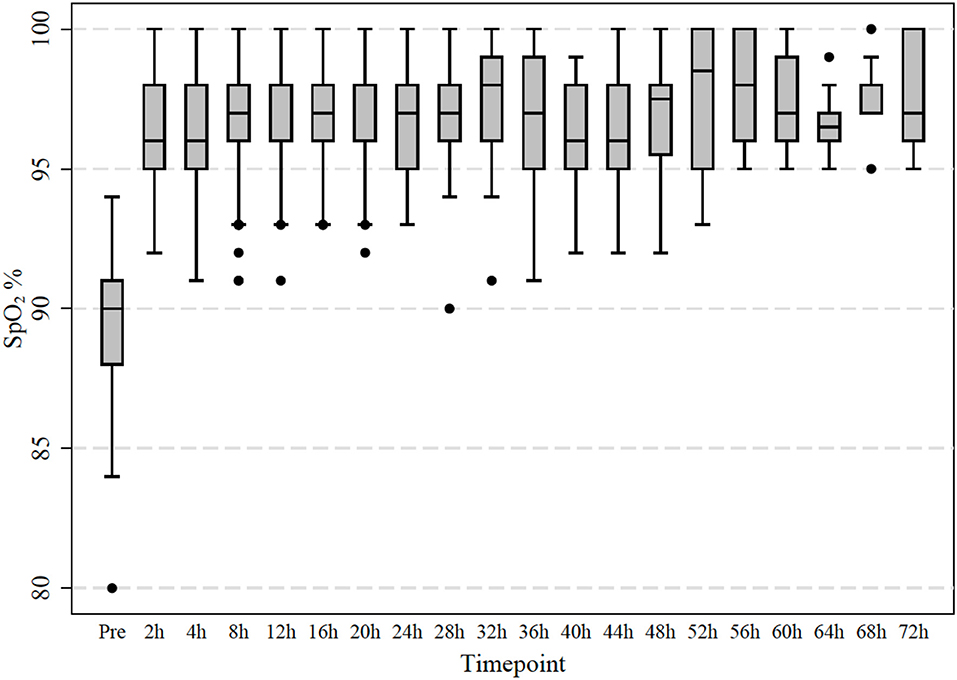
Figure 2. Measured transcutaneous saturation of oxygen levels in 64 infants treated with room air high-flow. Observations were taken initially after 2 h and then every 4 h.
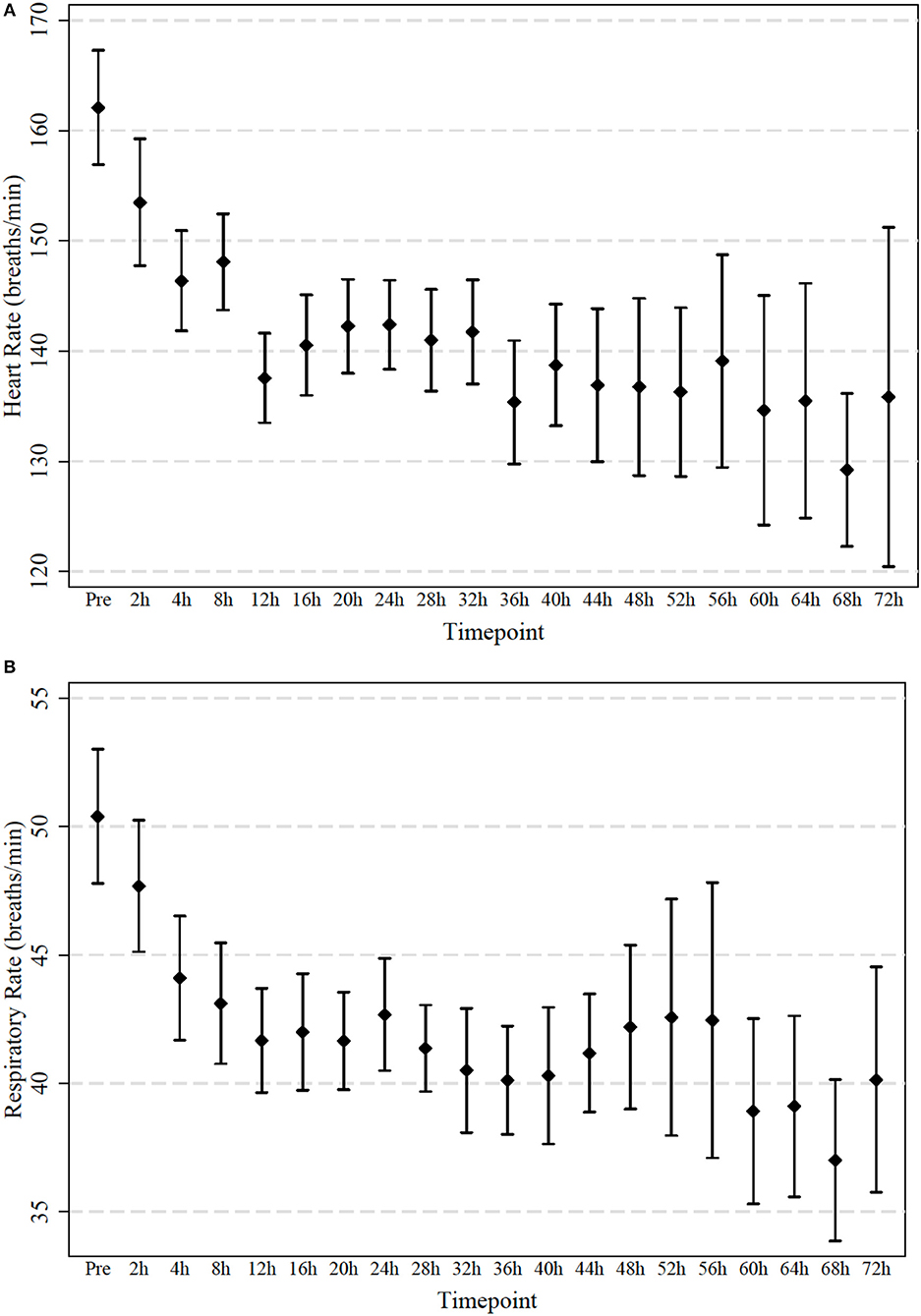
Figure 3. (A,B) Measured heart and respiratory rate in 64 infants treated with high-flow in room air. Observations were taken initially after 2 h and then every 4 h. Mean and 95% confidence interval are indicated.
The length of hospital stay was shortest in infants in the high-flow room air group (Table 2). None of the tested pre-enrolment factors such as age, prematurity, RSV, time of onset of disease to presentation or heart nor respiratory rate were predictive for a success of high-flow room air therapy (Table 3).
None of the infants on high-flow room air required intensive care admission and no adverse events were observed in either the high-flow room air group or those who commenced treatment initially in room air.
For completeness, infants in the standard-oxygen group presenting with pre-enrolment saturations <85% were compared to infants with saturations between 85 and≤92/94% and the comparison is shown in Table 4. Similarly, to the high-flow group, infants in the standard-oxygen arm presenting with pre-enrolment saturations of <85% were younger and had lower body weight. The infants in the standard-oxygen group and presenting with saturations <85% received similarly to the patients in the high-flow with immediate oxygen group, significantly more antibiotics and sedation.
Discussion
The World Health Organization recommended in 2012 that effective oxygen delivery systems should be a universal standard of care and should be made more widely available (21). At the same time, an increasing body of evidence suggests potential for harm related to oxygen application in various settings including perinatal adaptation, cardiac arrest, and intensive care (2–5). Furthermore, in adults receiving mechanical ventilation it has been shown that a large number of patients were left on unnecessarily high inspired oxygen fraction, which can contribute to lung disease (22). Bronchiolitis represents the most common disease leading to non-elective admission of infants and oxygen remains the mainstay of therapy for this patient group (23). Our data demonstrates that in a small subgroup of hypoxemic infants with bronchiolitis the oxygen requirement can be safely treated with high-flow in room air for their entire hospital stay. Infants responding to high-flow room air not only showed a significant improvement in their saturations but also a reduction in heart and respiratory rates indicating effective respiratory support (Figures 2, 3). The reversal of the hypoxemia was likely due to the positive airway pressure effect generated by nasal high-flow therapy, which improved the underlying pathophysiology such as ventilation maldistribution and loss of lung volume (atelectasis). Previous physiological studies have shown that high-flow rates at 2 L/kg/min generate a positive airway pressure in the range of 4–6 cm H2O, which can restore the patient's functional residual capacity and reduce ventilation inhomogeneity (24, 25).
The current recommendations for first line treatment of hypoxemia is the provision of oxygen (26). If supplemental oxygen is ineffective, then as a second line treatment some form of positive pressure ventilation either non-invasive or invasive ventilation should be used. The study design and outcomes in the high-flow arm suggests it is safe to initially institute high-flow in room air and to subsequently supplement oxygen in those who need it, except in infants with severe hypoxemia on presentation (saturation <85%). The results of this prospective trial indicate that a small proportion of infants (10%) will respond to high-flow room air alone, and those requiring oxygen can be safely titrated to the appropriate dose. Albeit a small proportion of infants in our study cohort responded to high-flow room air, these findings provide as a first interesting physiological proof of concept that in these infants oxygenation can be improved by providing positive airway pressure only.
A multivariate analysis did not demonstrate any predictive factors for responders of this approach. Infants who required high-flow room air only had a significantly shorter stay in hospital, most likely as a result of a milder form of the respiratory disease.
The results of our study serve as a proof of concept that may inform future clinical trials particularly in less well-resourced settings where access to oxygen is limited. This could prove beneficial for regions where the mortality of infants with chest infections and an oxygen requirement is 8% (African FEAST trial) (27, 28). A recent study in Bangladesh compared standard-oxygen, bubble CPAP, and high-flow in children with chest infections aged up to 5 years. The median saturation at presentation in this study was 86 % (IQR 82–88) with only 14% of enrolled children having a saturation of <85% (29). The current COAST trial being performed in Uganda and Kenya in children presenting with a chest infection and an oxygen requirement is currently investigating if high-flow in room air can reverse hypoxemia and reduce mortality (28).
One of the shifts in paradigm in this study was that oxygen therapy was started from “low”—in room air—and titrated up to reach the desired SpO2 threshold >92/94% whereas traditionally oxygen therapy was introduced from “high” to achieve maximal saturations and then titrated down. It is likely that the titrate up strategy will mean that saturation levels will be maintained closer to the lower acceptable levels than in the titrate down strategy. Hyperoxia is commonly observed in mechanically ventilated patients and most often related to the reluctance of clinicians to reduce the inspired oxygen fraction (22). In the small cohort of infants in whom physiological parameters were also measured within the first hour of treatment, the oxygen saturations were highest in the standard-oxygen group. In the high-flow group the clinicians were instructed to start with low FiO2 (room air) and only increase if required, whereas in the standard-oxygen group no instructions were given.
Limitations
The medical records in the participating sites did not allow measuring the fidelity if clinicians strictly adhered to the proposed high-flow room air approach, hence the proportion of patients who would have benefitted from the high-flow room air approach may have been greater. Our study protocol used a saturation target between 92 and 98% (in some centers 94–98%). WHO is recommending a lower threshold of 90% to initiate oxygen therapy. Therefore, using a lower treatment threshold for hypoxemia would have likely increased the proportion of successful treatments with high-flow in room air. A recent study in bronchiolitis in the UK showed that keeping a threshold of 90% compared to 94% did not impact on safety and efficacy (30).
Conclusion
A small proportion of infants with bronchiolitis and hypoxemia who were admitted to hospital did respond to nasal high-flow therapy using room air only. The clinical improvement of the high-flow therapy could be demonstrated immediately with decreased heart and respiratory rate. Nasal high-flow room air therapy may be a valuable option to treat hypoxemia in bronchiolitis in settings with limited access to oxygen.
Evidence Before this Study
A comprehensive literature search using MEDLINE, Cochrane, CINAHL, and EMBASE demonstrated that high-flow therapy has clinical benefit over standard oxygen therapy used in infants with bronchiolitis in general ward settings with two large randomized controlled trials showing a reduced need to escalate therapy. No study could be identified to investigate the role of high-flow in room air and its clinical effect.
Added Value of this Study
The study findings are a proof of concept. The results may instigate new trials using high-flow in room air in settings with limited access to oxygen supplies.
Implications of All the Available Evidence
This study presents as a first a novel concept to improve hypoxemia in infants with bronchiolitis. The approach is to first apply positive airway pressure support followed by increasing the oxygen fraction if required. Titration of oxygen from low to normal saturation as a mean to limit possible oxygen toxicity.
Data Availability Statement
All datasets generated for this study are included in the manuscript/supplementary files.
Ethics Statement
This study was carried out in accordance with the recommendations of Children's Health Queensland Ethics Committee and with written informed consent from all subjects. All subjects gave written informed consent in accordance with the Declaration of Helsinki. The protocol was approved by Children's Health Queensland Ethics Committee (HREC/13/QRCH/93).
Author Contributions
All authors listed have made a substantial, direct and intellectual contribution to the work, and approved it for publication.
Funding
The study was funded by a project grant from the National Health and Medical Research Council (NHMRC, GNT1081736), Canberra, Australia, and Queensland Emergency Medical Research Fund (QEMRF), Brisbane, Australia. The high-flow equipment and consumables for all study sites were provided free of charge by Fisher & Paykel Healthcare (Auckland, New Zealand).
Conflict of Interest
The authors declare that the research was conducted in the absence of any commercial or financial relationships that could be construed as a potential conflict of interest.
References
1. World-Health-Organization (ed.). Recommendations for Management of Common Childhood Conditions : Evidence for Technical Update of Pocket Book Recommendations : Newborn Conditions, Dysentery, Pneumonia, Oxygen Use and Delivery, Common Causes of Fever, Severe Acute Malnutrition and Supportive Care. Geneva: World Health Organization (2012).
2. Davis PG, Tan A, O'Donnell CP, Schulze A. Resuscitation of newborn infants with 100% oxygen or air: a systematic review and meta-analysis. Lancet. (2004) 364:1329–33. doi: 10.1016/S0140-6736(04)17189-4
3. Munkeby BH, Børke WB, Bjørnland K, Sikkeland LI, Borge GI, Halvorsen, et al. Resuscitation with 100% O2 increases cerebral injury in hypoxemic piglets. Pediatr Res. (2004) 56:783–90. doi: 10.1203/01.PDR.0000141988.89820.E3
4. Cabello JB, Burls A, Emparanza JI, Bayliss S, Quinn T. Oxygen therapy for acute myocardial infarction. Cochrane Database Syst Rev. (2013) 8:CD007160. doi: 10.1002/14651858.CD007160.pub3
5. Ronning OM, Guldvog B. Should stroke victims routinely receive supplemental oxygen? A quasi-randomized controlled trial. Stroke. (1999) 30:2033–7.
7. Davis WB, Rennard SI, Bitterman PB, Crystal RG. Pulmonary oxygen toxicity. Early reversible changes in human alveolar structures induced by hyperoxia. N Engl J Med. (1983) 309:878–83. doi: 10.1056/NEJM198310133091502
8. Davis WB, Rennard SI, Bitterman PB, Gadek JE, Sun XH, Wewers M, et al. Pulmonary oxygen toxicity. Bronchoalveolar lavage demonstration of early parameters of alveolitis. Chest. (1983) 83(5 Suppl.):35S. doi: 10.1378/chest.83.5.35sb
9. Duke T, Subhi R, Peel D, Frey B. Pulse oximetry: technology to reduce child mortality in developing countries. Ann Trop Paediatr. (2009) 29:165–75. doi: 10.1179/027249309X12467994190011
10. Duke T, Wandi F, Jonathan M, Matai S, Kaupa M, Saavu M, et al. Improved oxygen systems for childhood pneumonia: a multihospital effectiveness study in Papua New Guinea. Lancet. (2008) 372:1328–33. doi: 10.1016/S0140-6736(08)61164-2
11. Milic-Emili J. Applied Physiology in Respiratory Mechanics. Topics in Anaesthesia and Critical Care. Milan; New York, NY: Springer-Verlag (1998). p. 233.
12. Marohn K, Panisello JM. Noninvasive ventilation in pediatric intensive care. Curr Opin Pediatr. (2013) 25:290–6. doi: 10.1097/MOP.0b013e328360dbdf
13. Dysart K, Miller TL, Wolfson MR, Shaffer TH. Research in high flow therapy: mechanisms of action. Respir Med. (2009) 103:1400–5. doi: 10.1016/j.rmed.2009.04.007
14. Franklin D, Schibler A. Nasal high flow therapy in infants and children. Pediatr Respirol Crit Care (2018) 2:2–6. doi: 10.4103/prcm.prcm_22_17
15. Schlapbach LJ, Schaefer J, Brady AM, Mayfield S, Schibler A. High-flow nasal cannula (HFNC) support in interhospital transport of critically ill children. Intensive Care Med. (2014) 40:592–9. doi: 10.1007/s00134-014-3226-7
16. Hough JL, Pham TM, Schibler A. Physiologic effect of high-flow nasal cannula in infants with bronchiolitis. Pediatr Crit Care Med. (2014) 15:e214–9. doi: 10.1097/PCC.0000000000000112
17. Pham TM, O'Malley L, Mayfield S, Martin S, Schibler A. The effect of high flow nasal cannula therapy on the work of breathing in infants with bronchiolitis. Pediatr Pulmonol. (2014) 50:713–20. doi: 10.1002/ppul.2306
18. Rubin S, Ghuman A, Deakers T, Khemani R, Ross P, Newth CJ. Effort of breathing in children receiving high-flow nasal cannula. Pediatr Crit Care Med. (2014) 15:1–6. doi: 10.1097/PCC.0000000000000011
19. Franklin D, Babl FE, Schlapbach LJ, Oakley E, Craig S, Neutze J, et al. A randomized trial of high-flow oxygen therapy in infants with bronchiolitis. N Engl J Med. (2018) 378:1121–31. doi: 10.1056/NEJMoa1714855
20. Franklin D, Dalziel S, Schlapbach LJ, Babl FE, Oakley E, Craig SS, et al. Early high flow nasal cannula therapy in bronchiolitis, a prospective randomised control trial (protocol): a Paediatric Acute Respiratory Intervention Study (PARIS). BMC Pediatr. (2015) 15:183. doi: 10.1186/s12887-015-0501-x
21. World Health Organization. Recommendations for Management of Common Childhood Conditions: Evidence for Technical Update of Pocket Book Recommendations: Newborn Conditions, Dysentery, Pneumonia, Oxygen Use and Delivery, Common Causes of Fever, Severe Acute Malnutrition and Supportive Care. Geneva: World Health Organization (2012).
22. Suzuki S, Eastwood GM, Peck L, Glassford NJ, Bellomo R. Current oxygen management in mechanically ventilated patients: a prospective observational cohort study. J Crit Care. (2013) 28:647–54. doi: 10.1016/j.jcrc.2013.03.010
23. Schlapbach LJ, Straney L, Gelbart B, Alexander J, Franklin D, Beca J, et al. Burden of disease and change in practice in critically ill infants with bronchiolitis. Eur Respir J. (2017) 49:1601648. doi: 10.1183/13993003.01648-2016
24. Pham TM, O'Malley L, Mayfield S, Martin S, Schibler A. The effect of high flow nasal cannula therapy on the work of breathing in infants with bronchiolitis. Pediatr Pulmonol. (2015) 50:713–20. doi: 10.1002/ppul.23060
25. Schibler A, Henning R. Positive end-expiratory pressure and ventilation inhomogeneity in mechanically ventilated children. Pediatr Crit Care Med. (2002) 3:124–8.
26. Jevon P. Resuscitation in hospital: Resuscitation Council (UK) recommendations. Nurs Stand. (2002) 16:41–4.
27. Maitland K, Kiguli S, Opoka RO, Engoru C, Olupot-Olupot P, Akech SO, et al. Mortality after fluid bolus in African children with severe infection. N Engl J Med. (2011) 364:2483–95. doi: 10.1056/NEJMoa1101549
28. Maitland K, Kiguli S, Opoka RO, Olupot-Olupot P, Engoru C, Njuguna P, et al. Children's Oxygen Administration Strategies Trial (COAST): A randomised controlled trial of high flow versus oxygen versus control in African children with severe pneumonia. Wellcome Open Res. (2017) 2:100. doi: 10.12688/wellcomeopenres.12747.1
29. Chisti MJ, Salam MA, Smith JH, Ahmed T, Pietroni MAC, Shahunja KM, et al. Bubble continuous positive airway pressure for children with severe pneumonia and hypoxaemia in Bangladesh: an open, randomised controlled trial. Lancet. (2015) 386:1057–65. doi: 10.1016/S0140-6736(15)60249-5
Keywords: oxygen therapy, room air, bronchiolitis, respiratory illness, nasal high flow therapy
Citation: Franklin D, Babl FE, Gibbons K, Pham TMT, Hasan N, Schlapbach LJ, Oakley E, Craig S, Furyk J, Neutze J, Moloney S, Gavranich J, Shirkhedkar P, Kapoor V, Grew S, Fraser JF, Dalziel S, Schibler A and PARIS and PREDICT (2019) Nasal High Flow in Room Air for Hypoxemic Bronchiolitis Infants. Front. Pediatr. 7:426. doi: 10.3389/fped.2019.00426
Received: 02 May 2019; Accepted: 04 October 2019;
Published: 25 October 2019.
Edited by:
Martin Chalumeau, Université Paris Descartes, FranceReviewed by:
Christophe Marguet, Centre Hospitalier Universitaire (CHU) de Rouen, FranceVincent Gajdos, Assistance Publique Hopitaux De Paris (AP-HP), France
Copyright © 2019 Franklin, Babl, Gibbons, Pham, Hasan, Schlapbach, Oakley, Craig, Furyk, Neutze, Moloney, Gavranich, Shirkhedkar, Kapoor, Grew, Fraser, Dalziel, Schibler and PARIS and PREDICT. This is an open-access article distributed under the terms of the Creative Commons Attribution License (CC BY). The use, distribution or reproduction in other forums is permitted, provided the original author(s) and the copyright owner(s) are credited and that the original publication in this journal is cited, in accordance with accepted academic practice. No use, distribution or reproduction is permitted which does not comply with these terms.
*Correspondence: Donna Franklin, ZC5mcmFua2xpbjJAdXEuZWR1LmF1