- 1Department of Neonatology, Leiden University Medical Center, Leiden, Netherlands
- 2Center Proteomics Metabolomics, Leiden University Medical Center, Leiden, Netherlands
- 3The Ritchie Centre, Hudson Institute for Medical Research, Melbourne, VIC, Australia
- 4Department of Obstetrics and Gynaecology, Monash University, Melbourne, VIC, Australia
- 5Department of Neonatology, Emma Children's Hospital, Amsterdam UMC, University of Amsterdam, Amsterdam, Netherlands
Background: Infants are currently stabilized at birth with initial low FiO2 which increases the risk of hypoxia and suppression of breathing in the first minutes after birth. We hypothesized that initiating stabilization at birth with a high O2 concentration, followed by titration, would improve breathing effort when compared to a low O2 concentration, followed by titration.
Methods: In a bi-center randomized controlled trial, infants <30 weeks gestation were stabilized at birth with an initial O2 concentration of 30 or 100%, followed by oxygen titration. Primary outcome was minute volume of spontaneous breathing. We also assessed tidal volumes, mean inspiratory flow rate (MIFR) and respiratory rate with a respiratory function monitor in the first 5 min after birth, and evaluated the duration of mask ventilation in the first 10 min after birth. Pulse oximetry was used to measure heart rate and SpO2 values in the first 10 min. Hypoxemia was defined as SpO2 < 25th percentile and hyperoxemia as SpO2 >95%. 8-iso-prostaglandin F2α (8iPGF2α) was measured to assess oxidative stress in cord blood and 1 and 24 h after birth.
Results: Fifty-two infants were randomized and recordings were obtained in 44 infants (100% O2-group: n = 20, 30% O2-group: n = 24). Minute volumes were significantly higher in the 100% O2-group (146.34 ± 112.68 mL/kg/min) compared to the 30% O2-group (74.43 ± 52.19 mL/kg/min), p = 0.014. Tidal volumes and MIFR were significantly higher in the 100% O2-group, while the duration of mask ventilation given was significantly shorter. Oxygenation in the first 5 min after birth was significantly higher in infants in the 100% O2-group [85 (64–93)%] compared to the 30% O2-group [58 (46–67)%], p < 0.001. The duration of hypoxemia was significantly shorter in the 100% O2-group, while the duration of hyperoxemia was not different between groups. There was no difference in oxidative stress marker 8iPGF2α between the groups.
Conclusion: Initiating stabilization of preterm infants at birth with 100% O2 led to higher breathing effort, improved oxygenation, and a shorter duration of mask ventilation as compared to 30% O2, without increasing the risk for hyperoxia or oxidative stress.
Clinical Trial Registration: This study was registered in www.trialregister.nl, with registration number NTR6878.
Introduction
In the fetus, spontaneous breathing movements enable small volumes of liquid to move in and out of the airways. During apnea, the glottis is predominantly closed, restraining the efflux of lung liquid which is essential for normal lung growth and development by maintaining the lung in a distended state (1–3). After birth, the requirement for breathing movements changes to ventilation of the gas exchange regions of the lung, which necessitates that the liquid filling the lungs is replaced with air. However, after birth the glottis continues to function as it does in the fetus. It closes during apnea, is predominantly closed and opens only briefly during spontaneous breaths when the breathing pattern is unstable, but is predominantly open if the newborn has a stable breathing pattern (4–6).
While most preterm infants need respiratory support at birth because of their low muscle strength and structural and biochemical immaturity of the lung, support is mainly provided non-invasively instead of invasively to reduce the risk of lung and brain injury. However, successful application of non-invasive respiratory support requires an open upper airway in order for air to enter the trachea and aerate the lung. A closed glottis during apnea hampers the effectiveness of non-invasively applied positive pressure ventilation. This has been demonstrated by both animal (4) and human studies, with the latter showing that a non-invasively applied sustained inflation in preterm infants at birth was ineffective unless the infant was spontaneously breathing (5). Interventions aimed at improving effectiveness of non-invasive ventilation should therefore focus on stimulation of spontaneous breathing in order to open the glottis and enable success of non-invasive respiratory support.
One of the factors influencing breathing activity is oxygenation. Hypoxia is a potent inhibitor of fetal breathing movements before birth and this inhibitory effect persists for days-weeks after birth (7). As hypoxia is a likely common mechanism for apnea at birth, hypoxia should be avoided in order to enhance spontaneous breathing and opening of the glottis so that non-invasive respiratory support can be more effective. This, in turn, might reduce the need for intubation and mechanical ventilation, thereby reducing the risk of lung and brain injury.
At birth, oxygenation is largely determined by the surface area available for gas exchange within the lung, the gas diffusion distance and the partial pressure gradient for oxygen between alveoli and adjacent capillaries. It has now become clear that, during stabilization of very and extremely preterm infants in the delivery room, to compensate for inadequate lung aeration a higher fraction of inspired oxygen (FiO2) is required because the surface area for gas exchange is suboptimal. However, the 2015 versions of the international resuscitation guidelines recommend that resuscitation of very and extremely preterm infants should commence with a low FiO2 in order to reduce the risk of hyperoxia (8–10). This is because hyperoxia is associated with increased production of oxygen free radicals leading to tissue damage in infants with an immature antioxidant capacity (11). This initial low FiO2 should then be titrated based on the 25th percentile of the oxygen saturation target ranges defined by Dawson et al. (12). The downside of this approach is an increased risk of hypoxia with a potential negative effect on breathing effort. It has been shown that persisting hypoxemia (SpO2 < 80%) at 5 min after birth is associated with a higher risk of mortality and the development of intraventricular hemorrhage (13). This might be caused by hypoxia-induced respiratory depression, leading to a higher risk of intubation and mechanical ventilation. Although multiple trials have assessed the effect of initiating stabilization at birth with high vs. low initial FiO2 levels on clinical outcomes, to date, breathing effort has not been evaluated with regard to the initial FiO2 used at birth.
We aimed to evaluate the effect of reducing hypoxemia on breathing effort of preterm infants at birth by using an initial high vs. low FiO2 (1.0 vs. 0.3). We hypothesized that initiating resuscitation of preterm infants at birth with a high FiO2 will increase breathing effort.
Methods
A single-blinded randomized controlled trial was conducted at the Leiden University Medical Centre (LUMC) and the Amsterdam University Medical Centre (Amsterdam UMC), both located in the Netherlands. Preterm infants with a gestational age between 240/7 and 296/7 weeks were included. Infants with congenital abnormalities or conditions that might have an adverse effect on breathing effort or ventilation were excluded. Infants were randomized for resuscitation with an initial FiO2 of 0.3 (30% O2-group) or 1.0 (100% O2-group) using Castor EDC (Amsterdam, the Netherlands), an electronic data capture system. Allocation was stratified by gestational age (240/7-266/7 weeks and 270/7-296/7 weeks), using variable block sizes (4–6).
After the initial FiO2 setting, FiO2 was titrated following SpO2 target ranges recommended by our local guidelines on stabilization after birth, which are based on the target ranges described by Dawson et al. (12). When SpO2 was below the 25th percentile of these reference ranges, FiO2 was titrated up to 0.5 and subsequently to 1.0 when the initial FiO2 setting was 0.3. In case of an initial FiO2 setting of 1.0, FiO2 was maintained at 1.0. When SpO2 exceeded 90%, FiO2 was titrated down to 0.5 and subsequently to 0.3 and 0.21 when the initial FiO2 setting was 1.0. If SpO2 exceeded 90% after initiation with FiO2 0.3, FiO2 was titrated down to 0.21 directly. SpO2 values were consistently monitored, and in case SpO2 values were outside the range, FiO2 was titrated every 30 s. Other procedures used in this study are described thoroughly in the published study protocol (14). At birth, cord clamping was performed after 30 s if the infants were apneic and after 60 s if the infants were breathing. In both groups, respiratory support was provided using a NeopuffTM infant T-piece resuscitator (Fisher & Paykel Healthcare, Auckland, New Zealand) or a Giraffe Star System (Anandic Medical Systems, Feuerthalen, Switzerland). Respiratory function monitoring (RFM) data were recorded during stabilization in the first 5 min after birth to assess respiratory effort, and RFM data were collected of the first 10 min after birth to assess duration of positive pressure ventilation and oxygenation. Blinding of data analysis was performed to increase validity of outcomes, without interfering with clinical practice by blinding the oxygen blender or changing the caregiver responsible for oxygen titration.
The RFM was used to measure inflation pressures, flow and tidal volumes. This was used to calculate minute volume, mean inspiratory flow rate (MIFR), and the total ventilation time. Gas flow in and out of the facemask or endotracheal tube was measured using a small variable resistance anemometer, which also measured airway pressure (Varflex Flow Transducer, Vyaire Medical, Yorba Linda, CA,USA). The flow signal was integrated to provide inspired and expired tidal volumes in real time, with the difference in volume providing the degree of leak from either the facemask or endotracheal tube. SpO2 and heart rate were measured using either a Masimo SET pulse oximeter (Masimo Radical, Masimo Corporation, Irvine, California, USA) or NellcorTM pulse oximeter (Covidien, Dublin, Ireland); the probe was placed around the right wrist or hand of the infant. Data on oxygenation and heart rates were extracted using a Philips Intellivue MP30 monitor (Philips, Eindhoven, the Netherlands). The FiO2 was measured using a portable oxygen analyzer AX300-I (Teledyne Analytical Instruments, CA, USA). The signals were digitized at 200 Hz using the NewLifeBox-R physiological recording system (Advanced Life Diagnostics, Weener, Germany) and all signals were recorded by the NewLifeBox Neo-RSD computer system (Advanced Life Diagnostics, Weener, Germany) supported by Polybench physiological software (Applied Biosignals, Weener, Germany). Pulmochart software (Applied Biosignals, Weener, Germany) was used to analyze primary and secondary outcomes.
The primary study parameter was breathing effort, expressed as average minute volume normalized for body weight in the first 5 min after birth. Area under the curve (AUC) of minute volume was calculated using the minute data of the first 5 min after birth. Other tidal breathing parameters that were assessed, were: respiratory rate, tidal volumes and MIFR in the first 5 min after birth as well as the duration of ventilation given via facemask in the first 10 min after birth. The incidence of breaths with tidal volumes >4 and >8 mL/kg were compared between the groups. We assessed Apgar scores at 1 and 5 min after birth. In addition, oxygenation of the infants was assessed by measuring SpO2 values in the first 10 min and determining the amount of time that infants were considered to be hypoxemic [SpO2 < 25th percentile of the target ranges defined by Dawson et al. (12)] or hyperoxemic (SpO2 > 95%). Average FiO2 given at specific time points after birth was assessed as well as the total exposure to O2 by measuring the AUC of FiO2 vs. time curve, calculated using values collected every 0.5 s.
The level of oxidative stress was assessed by measuring the level of 8-iso-prostaglandin F2α (8iPGF2α) at birth by using cord blood, and 1 and 24 h after birth using blood samples of the infant. A detailed description of sampling and the rationale for this marker are presented in the study protocol (14). The samples were analyzed using the same method as described previously by Mas et al. (15). Validation of the analysis has been described in the Supplementary Material. The demographic data collected were: gestational age, birth weight, gender, antenatal use of corticosteroids, mode of delivery, complications during pregnancy (pregnancy-induced hypertension (PIH), preterm prelabor rupture of membranes (PPROM), intra-uterine growth restriction (IUGR), proven intra-uterine infection [in this study defined as positive blood culture in infant <72 h after birth)] and maternal medication use. Short-term clinical outcomes were noted: intraventricular hemorrhage ≥ grade III, intubation during resuscitation or within 24 h after birth and death before hospital discharge.
In a recent study, the average minute volume of preterm infants over the first 100 breaths was 150 ± 70 mL/kg/min (16). The study of van Vonderen et al. (17) shows an 80% relative increase in respiratory drive when FiO2 was switched from 0.21 to 1.0, in the first minute after FiO2 was increased (from 134 to 240 mL/kg/min). However, since we investigate a FiO2 of 0.3 instead of 0.21 in the lower oxygen arm, we expected half of the effect (40% relative increase) in respiratory drive than reported by van Vonderen et al. (17). For this, a sample size of 44 infants would be needed (α of 0.05 and power (1–β) of 0.8, 2-sided). Anticipating a 10% fail of recording of physiological measurements, and an additional risk of withdrawal or decline of consent after the infant was included, 52 infants were recruited.
Statistical analysis was performed with SPSS software version 23.0 (IBM, Chicago, Illinois; 2012). Although allocation was stratified by gestational age, outcomes are analyzed and presented for both treatment groups as a whole, because of the relatively small sample size. Differences in parameters of breathing effort in the first 5 min after birth between the study groups were tested with a Student's t-test if the data were found to be parametric, or with Mann-Whitney U test when the data were non-parametric. The interaction of minute volume, tidal volumes and MIFR in the first 5 min after birth with time were analyzed with a linear mixed model, in which both study group (treatment) and time were taken in consideration. Other study parameters and basic characteristics were explored to test for differences between the study groups. Demographical data and data on clinical outcomes are shown for all infants who were randomized, including infants for whom the primary or secondary outcomes could not be assessed. In addition, these data are also shown separately for the group of infants in whom primary and secondary outcomes could be assessed. Data are presented as mean ± SD for parametric data, median (IQR) for non-parametric data and n (%) for categorical data. P < 0.05 were considered significant.
Results
A total of 109 eligible infants were born in the LUMC and Amsterdam UMC during the study enrolment period between January 2018 and March 2019. Fifty-seven infants could not be included in this study because antenatal parental consent was refused, it was inappropriate to approach parents for considering enrolment in the study, the study was conflicting with other trials or because of technical issues with the RFM. Therefore, a total of 52 infants were randomized for initiation of resuscitation with either 100 or 30% O2. Six of these infants were excluded from analysis for outcomes on breathing effort and oxygenation due to failure to record physiological parameters with the RFM and pulse oximetry. One infant was excluded from the complete analysis because no respiratory support was needed and one infant because deferred consent was not obtained (Figure 1). In the 100% O2-group, 11 infants were recruited by prospective consent and nine infants by deferred consent, and in the 30% O2-group 11 infants were recruited prospectively and 13 infants with deferred consent.
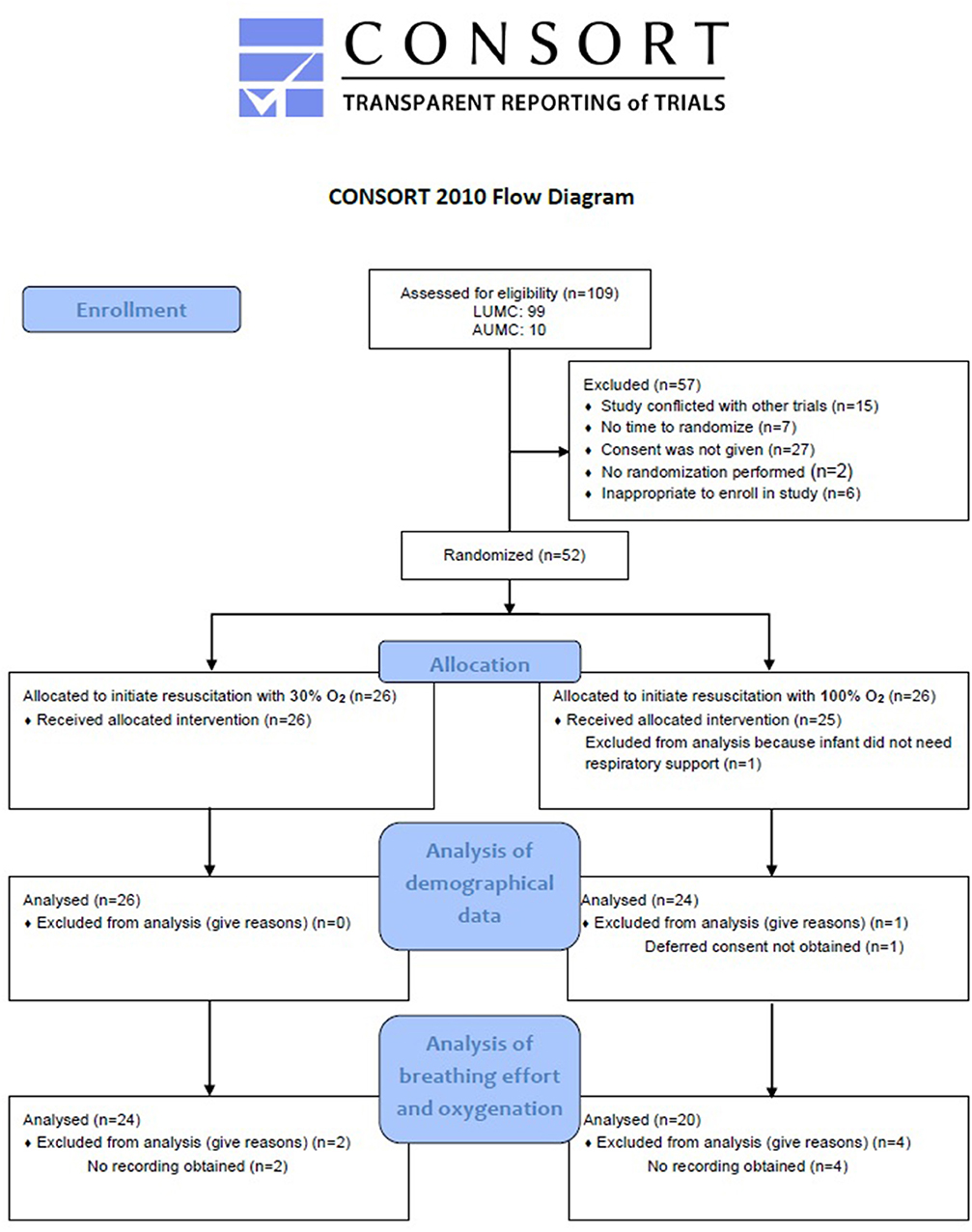
Figure 1. Flow diagram of allocation and analysis. LUMC, Leiden University Medical Centre; AUMC, Amsterdam University Medical Centre.
There were no significant differences between the 100% and the 30% O2-group with regard to gestational age, gender, birth weight, percentage of infants that received a full course of antenatal steroids or mode of delivery in both the total group and the group where physiological parameters were recorded (Tables 1, 2). Neither were there any differences regarding maternal medication use or complications during pregnancy that could have had an effect on respiration of the infant at birth (Tables 1, 2).
The average FiO2 received in the first 5 min after birth was significantly higher in the 100% O2-group compared to the 30% O2-group (FiO2 0.91 (0.67–0.98) vs. FiO2 0.48 (0.33–0.53), p < 0.001), as was the average FiO2 in the first 10 min after birth (FiO2 0.69 (0.41–0.88) vs. FiO2 0.45 (0.33–0.64), p = 0.020). Over the first 5 min after birth, total exposure to O2 (measured as AUC of FiO2 vs. time) was significantly higher in the 100% O2-group (AUC0−5 559.2 (346.6–745.2)%·min vs. 346.3 (260.9–450.9)%·min, p = 0.002, but when assessed over the first 10 min after birth it was not different between groups (AUC0−10 1011.3 (784.0–1191.7)%·min vs. 844.1 (741.0–1006.7)%·min, p = 0.178). This was because FiO2 was rapidly reduced in the 100% O2-group, whereas at the same time FiO2 levels in the 30% O2-group were increasing (Figure 2A).
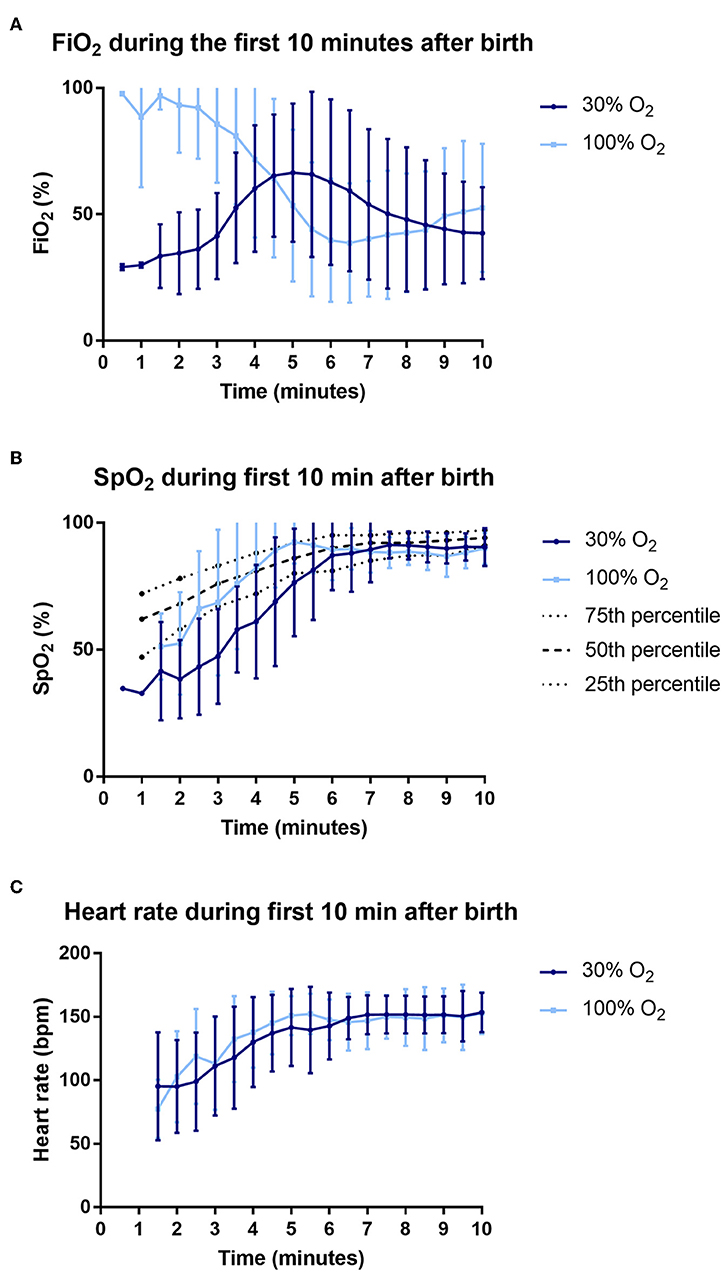
Figure 2. Data on fraction of inspired oxygen (FiO2) (A), oxygen saturations (SpO2) (B) and heart rate (C) during the first 10 min after birth.
Breathing Effort
The minute volume averaged over the first 5 min after birth was significantly higher in the 100% O2-group compared to the 30% O2-group (146.34 ± 112.68 mL/kg/min vs. 74.43 ± 52.19 mL/kg/min, p = 0.014; Figure 3). Similarly, the cumulative minute volume measured over the first 5 min after birth (AUC of MV vs. time) was significantly higher in infants whose resuscitation commenced with 100% O2 (498.18 ± 390.10 min.mL/kg) compared with 30% (244.63 ± 168.42 min.mL/kg, p = 0.014; Figure 3).
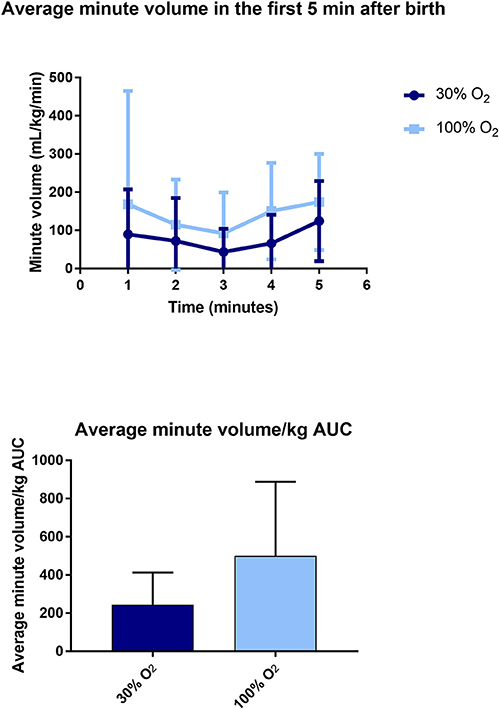
Figure 3. Data on average minute volume (upper) and area under the curve (AUC) of minute volume (lower) in the first 5 min after birth.
The average tidal volume and MIFR in the first 5 min after birth were significantly higher in the 100% O2-group (Table 3, Figure 4). When respiratory rates are measured at specific time points after birth, there was a trend toward a higher respiratory rate in the 100% O2-group, although this difference did not reach statistical significance (Table 3, Figure 4). Similarly, when the cumulative respiratory rate is measured over the first 5 min (AUC of respiratory rate vs. time) respiratory rates tended to be higher in the 100% O2-group (88.78 ± 58.54 vs. 69.83 ± 43.70 breaths, p = 0.226). Although there was a trend toward a higher incidence of breaths with a tidal volume >4 mL/kg (49 ± 25% vs. 35 ± 24%, p = 0.056), the incidence of recruitment breaths (>8 mL/kg) did not differ between study groups (Table 3).
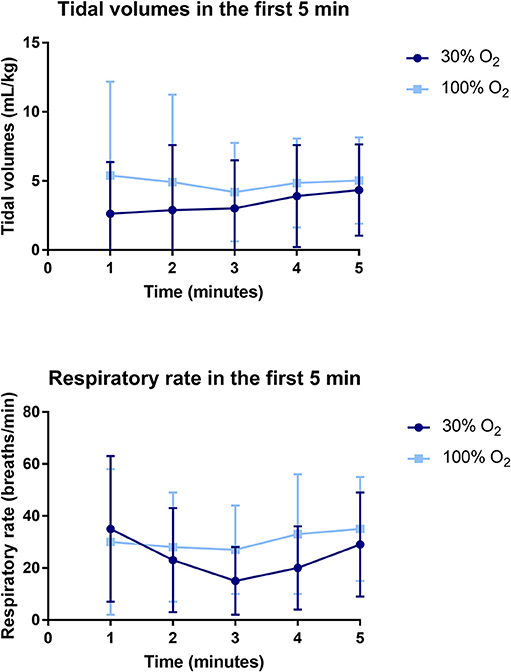
Figure 4. Data on tidal volumes (upper) and respiratory rate (lower) in the first 5 min after birth.
The duration of mask ventilation was significantly shorter in the 100% O2-group [23.6 (0.0–122.2) s vs. 108.3 (46.4–205.1) s, p = 0.021]. While Apgar scores at 1 min after birth were not significantly different between the study groups [6 (3–8) vs. 5 (3–7), p = 0.149], infants in the 100% O2-group showed significantly higher Apgar score at 5 min after birth [8 (8–9) vs. 8 (7–8), p = 0.028], as a result of higher scores on breathing and tone.
Oxygenation
The average oxygen saturation in the first 5 min after birth was significantly higher in infants in the 100% O2-group [SpO2 85 (64–93)% vs. 58 (46–67)%, p < 0.001]. In addition, there was a trend toward a higher heart rate in the 100% O2-group (Table 4, Figure 2C). Infants in the 100% O2-group had SpO2 values between 90 and 95% for a significantly higher percentage of time in the first 5 min after birth, while at 5–10 min after birth this was not significantly different between the groups (Table 4, Figure 2B). A SpO2 of 80% was reached significantly earlier in the 100% O2-group (time 178 ± 70 s after birth vs. 261 ± 80 s after birth, p = 0.001). In addition, the duration of hypoxemia [SpO2 < 25th percentile of reference ranges described by Dawson et al. (12)] was significantly shorter in the 100% O2-group (Table 4). However, the duration of hyperoxemia (SpO2 > 95%) did not significantly differ between the study groups.
Oxidative Stress
Cord blood from 32/44 (73%) infants could be obtained to analyze the level of 8iPGF2α at baseline. At 1 h after birth, blood samples of a total of 27/44 (61%) infants were obtained and analyzed, and at 24 h after birth, again blood samples of 25/44 (57%) infants were obtained and analyzed. The level of 8iPGF2α did not differ between the study groups over time, neither did the level of 8iPGF2α differ at the specific time points analyzed (Figure 5).
Short-Term Clinical Outcomes
Only two infants of the total study population needed intubation in the delivery room, both infants were randomized to the 30% O2-group. There were no differences between the study groups with regard to intubation, incidence of IVH ≥ grade III or death before hospital discharge (Table 5).
Discussion
In this bi-center, single blinded, randomized controlled trial, the effect of initiating resuscitation with an FiO2 of either 1.0 or 0.3 on breathing effort of preterm infants was evaluated. We demonstrated significantly higher breathing effort in preterm infants who were stabilized at birth with an initial FiO2 of 1.0 as compared to infants initiating with an FiO2 of 0.3. Similarly, oxygenation was significantly higher over the first 5 min after birth, with a shorter duration of hypoxemia without an increase in hyperoxemia or oxidative stress. While total O2 exposure (AUC of FiO2 vs. time) was increased over the first 5 min, it was less during the second 5 min in the 100% O2-group, resulting in no difference between groups after 10 min. The improved oxygenation and breathing effort were also reflected by a shorter duration of mask ventilation in the 100% O2-group. These results indicate that initiating stabilization at birth with a high FiO2 followed by careful titration to avoid hyperoxia, is a better option for stimulating breathing and decreasing the need for positive pressure ventilation in very and extremely preterm infants.
The effectiveness of non-invasive ventilation is hampered by a closed glottis during apnea, preventing air from entering the lung. While non-invasive respiratory support is universally adopted as the primary mode of respiratory support for preterm infants at birth, closure of the glottis between breaths underscores the importance of stimulating spontaneous breathing to enhance the success of this approach. Evaluating the effect of interventions used in the delivery room on breathing effort of preterm infants is therefore important to guide clinicians in prioritizing interventions to increase the effectiveness of non-invasive respiratory support. Although multiple randomized trials have been performed comparing the effect of a high vs. low initial FiO2 during stabilization of preterm infants at birth, none of these trials have evaluated breathing effort (18–23). The effect of oxygenation on breathing effort has so far only been demonstrated in an observational study where an increase in breathing effort was observed after switching FiO2 from 0.21 to 1.0 (17). However, we recently demonstrated the effect of the use of a FiO2 of 1.0 at birth on breathing effort in a spontaneously breathing preterm rabbit model (24). When compared to stabilization with FiO2 0.21, an FiO2 of 1.0 led to a more stable breathing pattern with a lower variability in inter-breath interval, higher respiratory rate and less apnea. We have now observed similar results in preterm infants, despite the fact that oxygen was titrated down instead of using a continuous FiO2 of 1.0, as used in preterm rabbits. This indicates that the initiating stabilization at birth with a high FiO2 reduces the hypoxia-induced respiratory depression, after which a reduction in FiO2 does not impact breathing effort.
The effect of other interventions used to increase breathing effort in the delivery room has been described previously (25, 26). Application of a regimented protocol of repetitive tactile stimulation to preterm infants at birth resulted in clinically relevant (although not statistically significant) increase of breathing effort, as compared to stimulation at the discretion of the caregiver; the result was not significant due to an increase in stimulation in the control group compared with historical levels of stimulation (25). In addition, infants who have been administered caffeine in the delivery room showed a significantly higher breathing effort (26). However, the magnitude of the difference in minute volume we found between the 100 and 30% O2-groups in the current study is much greater than the effect of any other treatment previously investigated in preterm infants at birth. This indicates that oxygen might be a major contributor to improving breathing effort at birth.
Infants in the 100% O2-group had significantly higher oxygenation levels (higher SpO2 values) and shorter periods of hypoxemia than infants in the 30% O2-group. Apart from the initial FiO2, our intent was to manage all infants with the same respiratory support strategy, but the 100% O2-group required less intermittent positive pressure ventilation than the 30% O2-group. As such, we consider that the higher initial partial pressure gradient for oxygen is likely to be the major contributor to the improved oxygenation in the 100% O2-group. While the total O2 exposure (AUC of FiO2 vs. time) was increased over the first 5 min after birth, it was not different between groups when measured over the first 10 min after birth. This is because the FiO2 was reduced between 2 and 6 min after birth in the 100% O2 group, whereas over the same time period, the FiO2 increased in the 30% O2 group (Figure 2). The finding that saturations were increasing while the FiO2 levels were decreasing in the 100% O2 group (between 2 and 6 min after birth) indicates that the O2 exchange capacity of the lung markedly increased over this time. This finding supports our contention that higher partial pressure gradients for oxygen (i.e., higher FiO2 levels) should be used initially, when the lung is partially aerated and the surface area for gas exchange is low, and that the FiO2 can be rapidly titrated down as the lung aerates and the potential for oxygen exchange increases. Indeed, as lung aeration and the increase in gas exchange surface area occurs in an exponential manner, presumably the decrease in FiO2 can follow a similar function.
Previous trials comparing the use of high vs. low initial oxygen concentrations for supporting preterm infants at birth show conflicting results. Some trials have found that initiating resuscitation with a low FiO2 (<0.3) results in significantly lower SpO2 values compared to a high FiO2 (>0.9) (18, 20, 23), whereas other trials detected no significant differences in oxygenation (19, 21, 22). However, different FiO2 titration protocols (both increasing and decreasing) between studies are likely to be a major confounding factor. FiO2 titration steps have ranged between 0.1 and 0.79, with step durations ranging between 10 s to every 5 min. There is also much heterogeneity in timepoints at which outcomes were assessed, with measurement start times ranging from at birth to 2 min after birth, whereas measurement end times ranging from 5 to 30 min after birth. Probably just as important is the prevention of hyperoxia and the tissue damage caused by increased oxygen free radicals. In our study, we found no difference in the duration of hyperoxemia or in the level of oxidative stress (assessed by measuring 8iPGF2α levels) between infants initially receiving either 30% or 100% O2. However, a previous study found that oxidative stress markers (advanced oxidative protein products AOPP and 8iPGF2α) were increased at 2 and/or 12 h after birth in infants initially receiving a high (FiO2 1.0) vs. low (FiO2 0.21) FiO2 (To2RPIDO trial) (27). Again, a difference in titration steps and timepoints of assessing the outcomes could explain the difference between these results and the results of our trial. The current study was not powered to detect differences in the level of oxidative stress, and in addition, blood samples could not be obtained and analyzed from every included infant. However, the results are from a large random sample and are likely representative for the total cohort.
Improving oxygenation in the first 5 min after birth has gained increased attention, as it has become clear that persistent hypoxemia at >5 min after birth is associated with an increased risk of intraventricular hemorrhage and mortality (13). As hypoxia inhibits breathing both in the fetus as well as after birth, persistent hypoxia likely reduces the efficacy of non-invasive ventilation resulting in an increased need for intubation and mechanical ventilation (7). Our trial was not designed to detect significant differences in clinical outcomes. However, it should be noted that IVH and death before hospital discharge occurred non-significantly more often in the 30% O2-group [IVH 0/24 (0%) vs. 4/26 (15%), p = 0.111; death before hospital discharge 2/24 (8%) vs. 5/26 (19%), p = 0.420].
The recent systematic review including trials on high vs. low FiO2 during resuscitation of preterm infants at birth showed no difference in clinical outcomes, including mortality, neurodevelopmental impairment and other key preterm morbidities (14). However, the level of evidence of included studies was assessed to be very low. In addition, the randomized trials included in this meta-analysis were highly variable with regard to the gestational age of included infants (<28– <35), FiO2 levels used for high (ranging from 0.6 to 1.0) and low (0.21–0.5) FiO2 study groups and SpO2 targets. In our study, we aimed to provide knowledge on one of the factors that could contribute to improve breathing effort at birth. It was shown that oxygen is a major contributing factor, in addition to other factors that have previously been shown to increase breathing effort, such as tactile stimulation and caffeine administration in the delivery room (25, 26). Incorporating these factors into the resuscitation guidelines on delivery room management may increase our effectiveness of supporting preterm infants non-invasively, leading to less intubation and mechanical ventilation and better clinical outcomes. We have demonstrated that oxygenation is a major determinant to enhance respiratory effort, but careful titration, which is mindful of the exponentially increasing potential of the lung to exchange oxygen, is required to prevent hyperoxia. It is time to rethink our current strategy of supporting preterm infants at birth, during which we allow them to be hypoxemic in the first minutes after birth. As long as we do not have found the best way to aerate the lung, we would need a higher oxygen concentration at birth to compensate for partial lung aeration at birth, in order to improve oxygenation and stimulate spontaneous breathing. Again, this has to be followed by careful titration of oxygen to reduce the risk for hyperoxia.
Conclusion
In this randomized controlled trial, we have demonstrated significantly higher breathing effort when resuscitation was initiated with an O2 concentration of 100% compared to 30% in very and extremely preterm infants. In addition, infants in the 100% O2-group were significantly better oxygenated with a shorter duration of hypoxemia without increasing the duration of SpO2 >95%, the total oxygen exposure (as measured by area under the curve of FiO2 received in the first 10 min after birth) or the level of 8-iso-prostaglandin F2α, measured at 1 and 24 h after birth. The results suggest that oxygenation might be an important determinant in stimulating breathing and decreasing the need for positive pressure ventilation in preterm infants at birth.
Data Availability Statement
The datasets generated for this study are available on request to the corresponding author.
Ethics Statement
The ethical committees of the LUMC and Amsterdam UMC approved the study protocol. Informed parental consent was obtained antenatally when possible. In case of an emergency situation (e.g., mother in full labor or when immediate delivery was necessary) or when obtaining antenatal consent was inappropriate (e.g., if the condition of the mother did not allow for proper consideration on participation), consent was asked retrospectively.
Author Contributions
JD, EL, MG, SH, and AP made substantial contributions to conception and design of the study. JD, TM, EL, EM, JH, RL AK, and AP recruited and included infants in the study and obtained data. Data was analyzed and interpreted by JD, TM, MG, AP, and SH. The first version of the manuscript was drafted by JD, AP, and SH. All authors are acknowledged for their critical revision of the manuscript and approval of the final version.
Funding
AP was recipient of an NWO innovational research incentives scheme (VIDI 91716428).
Conflict of Interest
The authors declare that the research was conducted in the absence of any commercial or financial relationships that could be construed as a potential conflict of interest.
Supplementary Material
The Supplementary Material for this article can be found online at: https://www.frontiersin.org/articles/10.3389/fped.2019.00504/full#supplementary-material
References
1. Hooper SB, Te Pas AB, Kitchen MJ. Respiratory transition in the newborn: a three-phase process. Arch Dis Child Fetal Neonatal Ed. (2016) 101:F266–71. doi: 10.1136/archdischild-2013-305704
2. Harding R, Hooper SB, Dickson KA. A mechanism leading to reduced lung expansion and lung hypoplasia in fetal sheep during oligohydramnios. Am J Obstet Gynecol. (1990) 163(6 Pt 1):1904–13. doi: 10.1016/0002-9378(90)90772-Y
3. Harding R, Bocking AD, Sigger JN. Upper airway resistances in fetal sheep: the influence of breathing activity. J Appl Physiol (1985). (1986) 60:160–5. doi: 10.1152/jappl.1986.60.1.160
4. Crawshaw JR, Kitchen MJ, Binder-Heschl C, Thio M, Wallace MJ, Kerr LT, et al. Laryngeal closure impedes non-invasive ventilation at birth. Arch Dis Child Fetal Neonatal Ed. (2018) 103:F112–9. doi: 10.1136/archdischild-2017-312681
5. van Vonderen JJ, Hooper SB, Hummler HD, Lopriore E, te Pas AB. Effects of a sustained inflation in preterm infants at birth. J Pediatr. (2014) 165:903–8.e1. doi: 10.1016/j.jpeds.2014.06.007
6. van Vonderen JJ, Hooper SB, Krabbe VB, Siew ML, Te Pas AB. Monitoring tidal volumes in preterm infants at birth: mask versus endotracheal ventilation. Arch Dis Child Fetal Neonatal Ed. (2015) 100:F43–6. doi: 10.1136/archdischild-2014-306614
7. Davey MG, Moss TJ, McCrabb GJ, Harding R. Prematurity alters hypoxic and hypercapnic ventilatory responses in developing lambs. Respir Physiol. (1996) 105:57–67. doi: 10.1016/0034-5687(96)00038-2
8. Perlman JM, Wyllie J, Kattwinkel J, Wyckoff MH, Aziz K, Guinsburg R, et al. Part 7: neonatal resuscitation: 2015 International consensus on cardiopulmonary resuscitation and emergency cardiovascular care science with treatment recommendations. Circulation. (2015) 132(16 Suppl. 1):S204–41. doi: 10.1161/CIR.0000000000000276
9. Wyckoff MH, Aziz K, Escobedo MB, Kapadia VS, Kattwinkel J, Perlman JM, et al. Part 13: neonatal resuscitation: 2015 American Heart Association guidelines update for cardiopulmonary resuscitation and emergency cardiovascular care. Pediatrics. (2015) 136(Suppl. 2):S196–218. doi: 10.1542/peds.2015-3373G
10. Wyllie J, Perlman JM, Kattwinkel J, Wyckoff MH, Aziz K, Guinsburg R, et al. Part 7: neonatal resuscitation: 2015 International consensus on cardiopulmonary resuscitation and emergency cardiovascular care science with treatment recommendations. Resuscitation. (2015) 95:e169–201. doi: 10.1016/j.resuscitation.2015.07.045
11. Vento M, Aguar M, Escobar J, Arduini A, Escrig R, Brugada M, et al. Antenatal steroids and antioxidant enzyme activity in preterm infants: influence of gender and timing. Antioxid Redox Signal. (2009) 11:2945–55. doi: 10.1089/ars.2009.2671
12. Dawson JA, Kamlin CO, Vento M, Wong C, Cole TJ, Donath SM, et al. Defining the reference range for oxygen saturation for infants after birth. Pediatrics. (2010) 125:e1340–7. doi: 10.1542/peds.2009-1510
13. Oei JL, Finer NN, Saugstad OD, Wright IM, Rabi Y, Tarnow-Mordi W, et al. Outcomes of oxygen saturation targeting during delivery room stabilisation of preterm infants. Arch Dis Child Fetal Neonatal Ed. (2018) 103:F446–54. doi: 10.1136/archdischild-2016-312366
14. Dekker J, Hooper SB, Giera M, McGillick EV, Hutten GJ, Onland W, et al. High vs. low initial oxygen to improve the breathing effort of preterm infants at birth: study protocol for a randomized controlled trial. Front Pediatr. (2019) 7:179. doi: 10.3389/fped.2019.00179
15. Mas E, Croft KD, Zahra P, Barden A, Mori TA. Resolvins D1, D2, and other mediators of self-limited resolution of inflammation in human blood following n-3 fatty acid supplementation. Clin Chem. (2012) 58:1476–84. doi: 10.1373/clinchem.2012.190199
16. Mian Q, Cheung PY, O'Reilly M, Pichler G, van Os S, Kushniruk K, et al. Spontaneously breathing preterm infants change in tidal volume to improve lung aeration immediately after birth. J Pediatr. (2015) 167:274–8.e1. doi: 10.1016/j.jpeds.2015.04.047
17. van Vonderen JJ, Narayen NE, Walther FJ, Siew ML, Davis PG, Hooper SB, et al. The administration of 100% oxygen and respiratory drive in very preterm infants at birth. PLoS ONE. (2013) 8:e76898. doi: 10.1371/journal.pone.0076898
18. Wang CL, Anderson C, Leone TA, Rich W, Govindaswami B, Finer NN. Resuscitation of preterm neonates by using room air or 100% oxygen. Pediatrics. (2008) 121:1083–9. doi: 10.1542/peds.2007-1460
19. Vento M, Moro M, Escrig R, Arruza L, Villar G, Izquierdo I, et al. Preterm resuscitation with low oxygen causes less oxidative stress, inflammation, and chronic lung disease. Pediatrics. (2009) 124:e439–49. doi: 10.1542/peds.2009-0434
20. Rabi Y, Singhal N, Nettel-Aguirre A. Room-air versus oxygen administration for resuscitation of preterm infants: the ROAR study. Pediatrics. (2011) 128:e374–81. doi: 10.1542/peds.2010-3130
21. Armanian AM, Badiee Z. Resuscitation of preterm newborns with low concentration oxygen versus high concentration oxygen. J Res Pharm Pract. (2012) 1:25–9. doi: 10.4103/2279-042X.99674
22. Kapadia VS, Chalak LF, Sparks JE, Allen JR, Savani RC, Wyckoff MH. Resuscitation of preterm neonates with limited versus high oxygen strategy. Pediatrics. (2013) 132:e1488–96. doi: 10.1542/peds.2013-0978
23. Oei JL, Saugstad OD, Lui K, Wright IM, Smyth JP, Craven P, et al. Targeted oxygen in the resuscitation of preterm infants, a randomized clinical trial. Pediatrics. (2017) 139:e20161452. doi: 10.1542/peds.2016-1452
24. Dekker J, Hooper SB, Croughan MK, Crossley KJ, Wallace MJ, McGillick EV, et al. (2019). Frontiers in Pediatrics. Increasing respiratory effort with 100% oxygen during resuscitation of preterm rabbits at birth. Front. Pediatr. 7:427. doi: 10.3389/fped.2019.00427
25. Dekker J, Hooper SB, Martherus T, Cramer SJE, van Geloven N, Te Pas AB. Repetitive versus standard tactile stimulation of preterm infants at birth—A randomized controlled trial. Resuscitation. (2018) 127:37–43. doi: 10.1016/j.resuscitation.2018.03.030
26. Dekker J, Hooper SB, van Vonderen JJ, Witlox R, Lopriore E, Te Pas AB. Caffeine to improve breathing effort of preterm infants at birth: a randomized controlled trial. Pediatr Res. (2017) 82:290–6. doi: 10.1038/pr.2017.45
Keywords: preterm infant, respiratory effort, breathing, oxygen, resuscitation
Citation: Dekker J, Martherus T, Lopriore E, Giera M, McGillick EV, Hutten J, van Leuteren RW, van Kaam AH, Hooper SB and te Pas AB (2019) The Effect of Initial High vs. Low FiO2 on Breathing Effort in Preterm Infants at Birth: A Randomized Controlled Trial. Front. Pediatr. 7:504. doi: 10.3389/fped.2019.00504
Received: 03 September 2019; Accepted: 21 November 2019;
Published: 12 December 2019.
Edited by:
Maximo Vento, University and Polytechnic Hospital of La Fe, SpainReviewed by:
Anne Lee Solevåg, Akershus University Hospital, NorwayJu Lee Oei, University of New South Wales, Australia
Copyright © 2019 Dekker, Martherus, Lopriore, Giera, McGillick, Hutten, van Leuteren, van Kaam, Hooper and te Pas. This is an open-access article distributed under the terms of the Creative Commons Attribution License (CC BY). The use, distribution or reproduction in other forums is permitted, provided the original author(s) and the copyright owner(s) are credited and that the original publication in this journal is cited, in accordance with accepted academic practice. No use, distribution or reproduction is permitted which does not comply with these terms.
*Correspondence: Janneke Dekker, amFubmVrZS5kZWtrZXJAaHVkc29uLm9yZy5hdQ==