- 1Department of Women and Children's Health, School of Life Course Sciences, Faculty of Life Sciences and Medicine, King's College London, London, United Kingdom
- 2Asthma UK Centre for Allergic Mechanisms in Asthma, King's College London, London, United Kingdom
- 3National Institute for Health Research (NIHR) Biomedical Research Centre at Guy's and St Thomas' National Health Service (NHS) Foundation Trust and King's College London, London, United Kingdom
Optimisation of respiratory support of infants with congenital diaphragmatic hernia (CDH) is critical. Infants with CDH often have severe lung hypoplasia and abnormal development of their pulmonary vasculature, leading to ventilation perfusion mismatch. It is vital that lung protective ventilation strategies are employed during both initial stabilisation and post-surgical repair to avoid ventilator induced lung damage and oxygen toxicity to prevent further impairment to an already diminished gas-exchanging environment. There is a lack of robust evidence for the routine use of surfactant therapy during initial resuscitation of infants with CDH and thus administration cannot be recommended outside clinical trials. Additionally, inhaled nitric oxide has been shown to have no benefit in reducing the mortality rates of infants with CDH. Other therapeutic agents which beneficially act on pulmonary hypertension are currently being assessed in infants with CDH in randomised multicentre trials. The role of novel ventilatory modalities such as closed loop automated oxygen control, liquid ventilation and heliox therapy may offer promise for infants with CDH, but the benefits need to be determined in appropriately designed clinical trials.
Introduction
The developmental disruption to the lungs and pulmonary vasculature of newborn infants with congenital diaphragmatic hernia (CDH) poses challenges during adaptation to postnatal life. The function of the lungs in providing essential gas exchange of oxygen and carbon dioxide at the alveolar-capillary membrane can be greatly diminished in infants presenting with this congenital abnormality (1). Importantly, appropriate, and timely intervention by clinicians is necessary to provide what can often be life-saving treatment, but may further adversely affect gas exchange. Ventilatory strategies and modalities are continuously being developed, with advances in technology contributing to such techniques. This review aims to provide clinicians with an outline of the recent evidence based ventilatory options available from birth and the initial stabilisation, through to surgery and post-operative management. It will also provide an insight into future developments.
Ventilatory Management During Resuscitation
Guidelines from the European Congenital Diaphragmatic Hernia (CDH EURO) Consortium and the American Academy of Pediatrics and American Heart Association (AAP/AHA) recommend routine intubation at birth of all infants with CDH where the prenatal diagnosis is known, with avoidance of bag and mask ventilation and the resultant inflation of herniated bowel contents (2). Peak inspiratory pressures of <25 cmH2O are recommended to avoid ventilator induced lung injury (VILI) to both lungs (2).
Respiratory function monitoring can measure the response to initial resuscitation and the results utilised to predict subsequent survival. Expiratory tidal volumes (p = 0.009) and lung compliance (p = 0.03) measured during the first minute of recorded resuscitation have been shown to be lower in non-survivors. An expiratory tidal volume of >3.8 ml/kg and a lung compliance of >0.12 ml/cmH20/kg was reported to be predictive of survival with 85% sensitivity and 90% specificity. Furthermore, tidal volumes of spontaneous breaths measured during the first 10 min after intubation have been described to be lower in those in those who either died prior to discharge or in those who developed chronic lung disease compared to survival without chronic lung disease (2.0 vs. 4.3 ml/kg; p = 0.004) (3). The achievement of higher maximal pre-ductal oxygen saturations (100 vs. 93%; p = 0.037) prior to transfer to neonatal intensive care have also been associated with greater survival in infants with a diagnosis of CDH (4). Such results are reflective of the degree of pulmonary hypoplasia in non-survivors. Additionally, respiratory function monitoring can be utilised to calculate the anatomical dead space in those with congenital malformations affecting the lungs. A larger anatomical dead space has been reported in those infants with CDH who survived to discharge [2.9 (2.8–3.3) ml/kg] compared to those who died [2.2 (2.1–2.7) ml/kg; p = 0.003] and can be used to predict survival [area under the curve (AUC) = 0.90] (5).
Dynamic lung compliance is low at birth and has been shown to be adversely affected by administration of a neuromuscular blocking agent. Indeed, the median lung compliance in a cohort of 15 infants with antenatally diagnosed CDH reduced from 0.22 to 0.16 ml.cmH2O−1.kg−1 (p < 0.001) immediately post administration of pancuronium bromide (6) (Figure 1). Hence, neuromuscular blocking agents should not be routinely administered during resuscitation (Figure 1). The respiratory function monitor used for pulmonary assessment within this study did not, however, measure oesophageal pressure
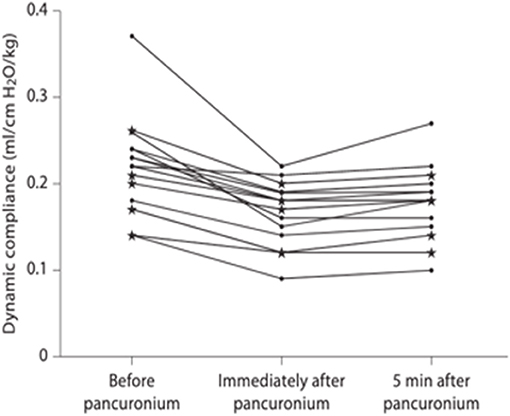
Figure 1. Dynamic compliance immediately before, immediately after and 5 min after pancuronium bromide administration [taken from (6)].
During resuscitation, physiological based cord clamping in animal models has been shown to be beneficial as dilatation of the pulmonary vasculature occurs following lung aeration, and thus pulmonary blood flow increases and oxygenation is improved (7). The feasibility of intact cord resuscitation in infants with CDH has recently been assessed in pilot studies. One prospective observational study (n = 40) reported no increase in neonatal adverse events whilst initiating resuscitation prior to cord clamping (8). A single-arm safety study (n = 20) determined the feasibility of intubation and ventilation prior to cord clamping and subsequently reported no significant difference in oxygenation indices or need for subsequent vasoactive therapy compared to those CDH infants undergoing immediate umbilical cord clamping (9). Recruitment is in progress into a multicentre international randomised trial determining the impact of physiological based cord clamping on clinically relevant outcomes in infants with CDH (10).
Where adequate antenatal development of the lung is expected, spontaneous breathing at birth can be considered. A recent, retrospective cohort study of 18 infants with mild CDH found a spontaneous breathing approach at delivery to be both safe and feasible (11). Prospective randomised trials are needed to appropriately evaluate this approach.
Initial Ventilatory Management Pre-surgery
Determining the optimal initial mode of ventilation in infants with CDH was assessed in a randomised trial (the VICI-trial) (12). Lung volume recruitment strategies utilised by high frequency oscillatory ventilation (HFOV) were not found to be superior to conventional mechanical ventilation (CMV) in reducing the combined outcome of death or bronchopulmonary dysplasia (BPD) [OR 0.62 (95% CI 0.25–1.55), p = 0.31]. With regards to secondary outcomes, however, the median (IQR) duration of mechanical ventilation was lower in the CMV group [10 (6–18) days] than the HFOV group [13 (8–23) days, p = 0.03]. Given the underlying lung pathology in CDH is that of pulmonary hypoplasia (a non-recruitable lung disease) this likely explains the inferiority of HFOV compared to CMV. Of those randomised to CMV, 42.9% required treatment with inhaled nitric oxide, compared to 56.2% of those on HFOV (p = 0.045). Additionally, a greater duration of vasoactive medication was needed in the HFOV [8 (4.3–19) days] compared to the CMV group [6 (3.3–11.8) days, p = 0.02]. The VICI trial also showed conventional ventilatory support to be beneficial in reducing the requirement for extracorporeal membrane oxygenation support (ECMO) (26 vs. 51%, p = 0.007) and is thus suggested as first line choice of ventilatory support in infants with CDH (2, 13, 14). Such benefits of CMV seen with regards to the secondary outcomes may however be reflective of the higher starting mean airway pressure (MAP) in the HFOV group (initial MAP 13–17 cmH20). A recent, multicentre cohort study of 328 infants also demonstrated no significant differences between HFOV or CMV as initial mode of ventilation when reporting on the outcome of mortality [OR 0.98 (95% CI 0.57–1.67)] or BPD [OR 1.66 (95% CI 0.50–5.49)] (15). That study, however, is limited by its retrospective nature, although propensity score matching was performed to reduce potential confounding. Furthermore, no significant difference was reported between mode of respiratory support (HFOV or CMV) at the time of surgical repair when considering oxygen dependency or death at 28 days (16). Use of high positive end-expiratory pressure (PEEP) may cause lung injury by over-inflation of the ipsilateral lung during HFOV and the ensuing pulmonary inflammatory response (12, 17). Nevertheless, use of HFOV may be indicated as rescue therapy following failure of initial conventional ventilatory strategies (18). Failure of conventional ventilation in CDH infants is considered when peak inspiratory pressures higher than 28 cmH2O are required to maintain oxygen saturations within target range and the partial pressure of carbon dioxide (pCO2) between 50 and 70 mmHg (2) (Figure 2).
Lung Protective Ventilatory Strategies
Pressure-Controlled Ventilation and Permissive Hypercapnia
Pressure controlled ventilation and permissive hypercapnia are strategies employed to avoid damage to the lung contralateral to the herniation. Indeed, a reduction in mortality was demonstrated in infants with CDH when pCO2 levels as high as 70 mmHg were permitted (42.9 vs. 14.3%; p = 0.002) (19). That study, however, only reported outcomes at a single institution before and after the introduction of permissive hypercapnia as a therapeutic strategy over a 16-year period. The results may, therefore, have been influenced by other significant changes and advances in management during that time. To avoid ventilatory induced lung damage, low ventilatory pressures are advised by the CDH EURO Consortium, with peak inspiratory pressures of <25 cmH2O recommended for use if possible, however that upper limit is not evidence based (2, 20).
Volume-Targeted Ventilation
The tidal volumes required to maintain effective minute ventilation and clearance of carbon dioxide in infants with CDH have been reported to be similar to that of control infants both pre (4.7 vs. 4.9 ml/kg; p = 0.49) and post (4.5 vs. 4.9 ml/kg; p = 0.14) operatively, however, in that retrospective cohort study, episodes in which tidal volumes corresponded with hypercapnic episodes were excluded. The most appropriate tidal volume targets in infants with CDH remains unanswered. Indeed, too low tidal volumes will result in an increase in the dead space to tidal volume ratio, yet use of too high tidal volumes may over-distend the already fewer alveoli existing in hypoplastic CDH lungs (20). No randomised trials have yet been performed to determine the optimal tidal volumes targets in infants with pulmonary hypoplasia secondary to congenital diaphragmatic hernia (21).
Intra-operative Ventilation
A pilot, randomised controlled trial aimed to determine the effect of open or thorascopic repair on intraoperative acidosis and hypercapnia. The thorascopic approach was associated with higher levels of intraoperative partial pressure of carbon dioxide (pCO2) (83 vs. 61 mmHg; p = 0.036) and prolonged acidosis compared to open CDH repair (14). The insufflation of CO2 during thorascopic repair was thought to adversely impact upon intra-operative ventilation; all the infants were supported by conventional ventilation. Subsequent potential countermeasures to hypercapnia during thorascopic repair, such as intrapulmonary percussive ventilation and pausing the insufflation of CO2 during surgery, have resulted in non-significant differences in the maximal pCO2 levels during thorascopic [55.9 (38–192) mmHg] and laparoscopic repair [54.1 (41–72) mmHg] (p = 0.60) (22). An observational study reported fewer infants with CDH to have hypercapnic (>60 mmHg) or hypoxic (SpO2 < 90%) episodes during thorascopic assisted repair than open repair, with a shorter duration of post-operative mechanical ventilation in the former group (p < 0.05) (23), but there was no reported significant difference in the overall post-operative survival rates between either choice of surgical repair.
Retrospective cohort analysis of infants who underwent thorascopic repair reported an increase in intraoperative pCO2, with subsequent acidosis regardless of whether conventional or high frequency ventilation was used. Intra-operative pCO2 was not significantly different when HFOV or CMV were chosen as the ventilatory modality during surgery; however, the infants receiving HFOV during thorascopic repair exhibited less marked respiratory acidosis compared to their pre-surgery values than infants supported by conventional ventilation (24). These results suggest that utilisation of HFOV during thorascopic repair may prevent deterioration of respiratory acidosis to a larger degree than conventional ventilation.
Ventilatory Management Post-surgical Repair
Respiratory compliance has been reported to decrease in infants with CDH following surgical repair (25). Furthermore, weight corrected respiratory system compliance (Crs) measured following surgery, in those infants with left sided CDH, has been shown to be negatively correlated with the need for prolonged post-operative mechanical ventilation (p = 0.006) (26). Positive end-expiratory pressure (pEEP) levels following surgical repair can affect respiratory system compliance and resistance in those with mild-moderate CDH and persistent pulmonary hypertension. In a randomised 1-h crossover trial, lung compliance increased by 30% when PEEP levels of 2 cmH2O were applied in comparison to use of 5 cmH2O of PEEP (p = 0.0001) (27). The improvement in oxygenation that occurred at the lower level of PEEP of 2 cmH2O, suggests higher PEEP levels are associated with over-distension of aerated “open” alveoli primarily within the ipsilateral lung (27). That study, however, was performed after surgical repair of the defect and so it remains to be answered whether low PEEP levels are beneficial pre-repair of the defect, given that the presence of viscera within the chest cavity may indeed prevent over-distension of the ipsilateral lung. Furthermore, high PEEP levels immediately after birth have been shown in an animal model to be beneficial in establishing functional residual capacity (28).
Prone positioning of mechanically ventilated infants post-operative repair of CDH has been associated with an improvement in oxygenation (PaO2/FiO2 ratio) (p = 0.032) and a reduction in the alveolar-arterial oxygen difference (p = 0.043) (29). Limitations of that study, however, were that measures of oxygenation and respiratory function were only measured for 30 min in each position and were not related to the adverse longer-term pulmonary outcomes suffered by infants with CDH. Post-surgical ventilation with tidal volumes of <5 ml/kg have been associated with an increase in the work of breathing in infants with CDH (p = 0.001) (30). That study, however, only included seven infants and future randomised, adequately powered studies are necessary to determine optimal tidal volume levels pre and post repair. Furthermore, the impact of such ventilatory strategies in relation to long-term pulmonary outcomes needs to be ascertained.
Additional Therapies
Supplementary Oxygen
There is a lack of randomised control trials determining the optimal fraction of supplemental oxygen during resuscitation in infants with CDH. In view of such paucity of robust data it is speculated that a starting fraction of the inspired oxygen (FiO2) of <1.0 during the initial resuscitation of newborn infants with CDH may be beneficial, with subsequent titration of the FiO2 to maintain preductal peripheral oxygen saturations (SpO2) between 80 and 95% (2). This starting FiO2 comes as a consequence of increasing concern related to the unfavourable effects of oxidative stress (31). Reducing free radical formation by lowering the levels of the inspired oxygen may subsequently reduce pulmonary vasoconstriction and the associated adverse consequences (32–34). Furthermore, animal models of persistent pulmonary hypertension of the newborn (PPHN) have shown that resuscitation with high levels of inspired oxygen (FiO2 1.0 vs. 0.5) can also impair the later pulmonary vasodilator response to inhaled nitric oxygen (iNO) which may be required as rescue therapy for respiratory failure in infants with CDH (35, 36). A lower starting FiO2 (0.5) during the resuscitation of infants with CDH has been shown to have no adverse effects upon survival (adjusted p = 0.142) or need for ECMO (adjusted p = 0.159) than starting resuscitation at a higher FiO2 (1.0) (37). If the SpO2, however, remained low and there was a subsequent need to increase the FiO2 to 1.0, then this was associated with a trend in reduced survival rates and postnatal ECMO requirement, but the worse outcomes were no longer significant after controlling for a lower gestational age at birth, liver position and lung-head ratio (LHR) (37). One limitation of those results is the retrospective cohort nature of the study. Future trials that randomise newborn infants with CDH to different starting levels of FiO2 during resuscitation are needed to provide clinicians with evidence based FiO2 targets and the later relationship to postnatal outcomes.
Knowledge of the partial pressure of arterial oxygen (PaO2) values are necessary to guide oxygenation indices and determine criteria for ECMO, with continuous monitoring of SpO2 utilised to guide optimal ventilatory strategies. The aim stated by consensus guidelines, is to achieve preductal oxygen saturations of between 80 and 95% 2 h after birth, with post ductal saturations above 70% (2). Provision of supplemental oxygen titrated to such SpO2 levels does, however, need careful monitoring (38, 39). Animal models of the CDH ventilatory responses during the first 2 h after birth have recently demonstrated unintentional cerebral hyperoxia to occur when cerebral blood flow is unmonitored, thought to be related to the rapid increases in carotid blood flow (38). Cerebral oxygenation, monitored by near-infrared spectroscopy, has been reported to be reduced during surgical repair of infants with severe CDH, regardless of conventional or high frequency modes of ventilatory support (p = 0.0001) (39). Those infants receiving HFOV however exhibited a prolonged reduction in cerebral oxygenation and required a longer duration of time to recover to normal values than those in the conventional group (p = 0.003) (39). The study, however, was not appropriately powered to determine the effects of mode of ventilation on cerebral oxygenation during surgery. Furthermore, the relationship of such findings with longer term neurodevelopmental outcomes were not reported.
Use of Inhaled Nitric Oxide
Inhaled nitric oxide (iNO) use in newborn infants with CDH has been reported in 68 (97.1%) participating centres in the Congenital Diaphragmatic Hernia Study Group registry (40). Of 2,174 infants diagnosed by echocardiography with pulmonary hypertension (PH) 74.2% received iNO therapy, however 36.4% of infants without PH were also treated with iNO. Propensity score analysis revealed iNO use to be associated with a 15% higher absolute mortality rate (average treatment effect on the treated: 0.15; 95% CI 0.10–0.20) (40). The use of iNO therapy for CDH infants with hypoxemic respiratory failure, unresponsive to conventional therapy, has also not been found to decrease the need for ECMO or reduce the primary outcome of death before discharge (41). Furthermore, only 16% of infants in that study (41) fully responded to iNO therapy at a dosage of 20 parts per million (ppm), as determined by an improvement of oxygenation indices. Inhaled nitric oxide is, however, often the first line drug of choice for pulmonary hypertension in newborn infants with CDH and forms a standard of care for the CDH Euro Consortium group (2). Nevertheless, as iNO has not been shown to be beneficial in reducing mortality rates, it is not routinely recommended by the American Pediatric Surgical Association (APSA) for the treatment of pulmonary hypertension in infants with CDH (13). Further trials are underway to determine if other therapeutic agents which act to reduce PH may offer more promise in infants with CDH. Currently, an international multicentre, randomised controlled trial is in the recruitment phase to assess whether intravenous sildenafil may be superior to iNO in reducing mortality in newborns with CDH (42). Additional trials are also setting out to determine the beneficial effects of inotropic agents, such as milrinone, in treating pulmonary hypertension caused by right and left ventricular dysfunction in CDH. Such therapy may improve left ventricular diastolic and systolic function, reduce afterload and subsequently lead to improved oxygenation in those with CDH. A randomised pilot trial is being conducted to determine the safety and feasibility of undertaking a larger multicentre trial (43).
Surfactant Therapy
Infants with CDH often exhibit reduced lung compliance (44). The use, therefore, of postnatal surfactant has been considered in infants with CDH. Use of postnatal surfactant administered to term infants (>37 weeks' gestational age, n = 522) was reported to have no beneficial impact upon survival, nor did such treatment reduce the incidence of chronic lung disease or the need for extra-corporeal membrane oxygenation (ECMO) (45). Furthermore, retrospective data from the CDH registry reported that administration of surfactant to preterm infants (<37 weeks' gestation, n = 424) was associated with a greater risk of death before discharge [odds ratio (OR) 2.17, 95% CI: 1.5–3.2; p < 0.01] (46). Additionally, surfactant replacement given to infants >35 weeks of gestation whilst on ECMO was reported to have no beneficial effects on the outcomes of survival to discharge (OR 1.0, 95% CI 0.67–1.62; p = 0.87) or requirement for supplemental home oxygen (OR 1.04, 95% CI 0.6–1.8; p = 0.90) (47). Surfactant concentrations in human foetuses with CDH have been reported to be similar to those of age matched controls, moreover the maturation and storage of surfactant appears not adversely impacted by this congenital pulmonary abnormality (48). Routine surfactant administration to infants with CDH is therefore not currently recommended in the EURO consensus guidance (2). Randomised clinical trials (RCTs) for surfactant therapy in preterm infants with CDH are warranted. If postnatal surfactant is given it should be noted that the standardised dosage regimens for weight are likely to be inaccurate due to the degree of pulmonary hypoplasia (48).
Novel Ventilatory Modalities
Neurally Adjusted Ventilatory Assist
Neurally adjusted ventilatory assist (NAVA) may confer protection to hypoplastic lungs. During NAVA, ventilatory support is delivered in response to diaphragmatic electrical activity. The utility of NAVA in newborn infants with structural diaphragmatic abnormalities has not been widely studied and since this ventilatory mode is dependent upon detection of neural diaphragmatic signals certain challenges may arise. In one study, after primary repair, infants with CDH placed on invasive NAVA were shown to have no differences in peak or resting electrical activity of the diaphragm during respiration compared to control infants with no underlying diaphragmatic abnormality, nor did they require higher levels of NAVA support (49). A recent case control study (n = 16) found no significant difference in the NAVA level (p = 0.286) used post-surgical repair in infants with CDH compared to those without CDH of similar age and weight at the time of study. Furthermore, NAVA use in those with diaphragmatic hernia was associated with a reduction in ventilatory requirements and the need for sedative therapy (49). Two retrospective feasibility studies performed in infants with CDH following surgical repair showed that post-operative weaning of ventilation with both invasive (n = 10) and non-invasive (n = 7) NAVA to be successful (50, 51). Additionally, the short-term outcomes of infants with CDH who have been placed on invasive NAVA post-surgery were assessed and NAVA was associated with a decrease in mean airway pressure (p < 0.001) and respiratory severity score (p < 0.001) at 72 h post initiation (p < 0.001) (49).
Closed Loop Automated Oxygen Control
Closed loop automated oxygen control systems have yet to be trialled in infants with CDH, but recent developments have shown promising results in optimising ventilatory support in other pulmonary conditions (52–56). Whether such a modality has a role in infants with congenital diaphragmatic anomalies has yet to be determined.
Heliox Therapy
Utilising heliox as an adjunctive therapy to the ventilation of infants with CDH was shown in one retrospective cohort study to be beneficial in reducing levels of hypercapnia (68 vs. 49 mmHg; p < 0.001) and levels of maximal ventilatory support required from high frequency oscillatory ventilation, and thus may be one such therapy to improve gas exchange in those with lung hypoplasia (57). Prospective randomised trials are required to ascertain the benefit of such therapy on short- and long-term pulmonary outcomes.
Liquid Ventilation
Lung growth in utero occurs as pulmonary fluid secretion provides a continuous distending pressure to the airways (58). Fluid filled lungs, combined with foetal breathing movements, underlie the mechanisms behind antenatal lung growth (59). Liquid at room temperature and less viscous that water, perfluorocarbons may thus provide some benefit when conventional ventilatory strategies remain challenging during postnatal life (60). By providing constant distending pressure, liquid ventilation may improve lung mechanics in a similar fashion to that of an increased PEEP. Results from animal models of severe respiratory failure have indeed shown partial liquid ventilation to be beneficial with regard to gas exchange and reducing pulmonary shunting (88 vs. 31%; p < 0.001) during ECMO (61). A randomised trial to assess the feasibility of partial liquid ventilation (perfluorocarbon-induced lung growth) in newborn infants with CDH (n = 13) confirmed the short-term safety of performance of this novel technique during ECMO (62). Larger randomised trials would be required to ascertain the long-term safety and benefits of liquid ventilation as a postnatal strategy in infants born with congenital diaphragmatic hernia, however as obtaining regulatory approval may prove challenging such therapy may be of doubtful clinical relevance.
Long Term Lung Function
Pulmonary morbidity and long-term lung function of infants with CDH is now of more significance as survival rates have increased (63). Follow up of 28 children with repaired left sided CDH (mean age 6.2 years) revealed 25% had abnormal pulmonary function (p < 0.01). Furthermore, those with abnormal pulmonary function had lower total lung volume on structural evaluation of chest tomography (826.5 ± 133.6 mls) than those with normal overall lung function (1,244.5 ± 407.9 mls; p < 0.05) (64). Longitudinal analysis of ventilation-perfusion (V/Q) mismatching has been recently reported in CDH survivors (65). In those with severe disease ipsilateral V/Q mismatch worsenend over time likely due to the progressive reduction in pulmonary perfusion (p = 0.012). Such perfusion deficits may be related to abnormal lung function and V/Q studies may thus be an important addition to exercise testing in order to identify and monitor those most at risk of worse outcomes.
Conclusion
This review has highlighted respiratory management techniques which could be utilised in resusciation and during the pre and post operative management stages in infants with CDH. Respiratory function monitoring is a useful tool to monitor pulmonary mechanics and the results may be utilised to predict subsequent survival. Conventional ventilatory modes are recommenced as initial respiratory support given the primary lung pathology of pulmonary hypoplasia. Further research and more randomised trials are, however, needed to provide clinicians with evidence based, optimal respiratory management strategies which have been shown to improve the long term pulmonary outcomes of infants with congenital diaphgramatic hernia.
Author Contributions
AG and EW designed the review and contributed to its production. Both authors contributed to the article and approved the submitted version.
Funding
EW was supported by a grant from the Charles Wolfson Charitable Trust and a non-conditional educational grant from SLE. This research was supported by the National Institute for Health Research (NIHR) Biomedical Research Centre at Guy's and St Thomas' NHS Foundation Trust and King's College London.
Author Disclaimer
The views expressed are those of the authors and not necessarily those of the NHS, the NIHR or the Department of Health.
Conflict of Interest
The authors declare that the research was conducted in the absence of any commercial or financial relationships that could be construed as a potential conflict of interest.
Publisher's Note
All claims expressed in this article are solely those of the authors and do not necessarily represent those of their affiliated organizations, or those of the publisher, the editors and the reviewers. Any product that may be evaluated in this article, or claim that may be made by its manufacturer, is not guaranteed or endorsed by the publisher.
References
1. Ting A, Glick PL, Wilcox DT, Holm BA, Gil J, DiMaio M. Alveolar vascularization of the lung in a lamb model of congenital diaphragmatic hernia. Am J Respir Crit Care Med. (1998) 157:31–4. doi: 10.1164/ajrccm.157.1.9703034
2. Snoek KG, Reiss IK, Greenough A, Capolupo I, Urlesberger B, Wessel L, et al. Standardized postnatal management of infants with congenital diaphragmatic hernia in Europe: The CDH EURO Consortium Consensus - 2015 Update. Neonatology. (2016) 110:66–74. doi: 10.1159/000444210
3. Mank A, Carrasco Carrasco C, Thio M, Clotet J, Pauws SC, DeKoninck P, et al. Tidal volumes at birth as predictor for adverse outcome in congenital diaphragmatic hernia. Arch Dis Child. (2020) 105:248–52. doi: 10.1136/archdischild-2018-316504
4. O'Rourke-Potocki A, Ali K, Murthy V, Milner A, Greenough A. Resuscitation of infants with congenital diaphragmatic hernia. Arch Dis Child Fetal Neonatal Ed. (2017) 102:F320–3. doi: 10.1136/archdischild-2016-311432
5. Williams EE, Dassios T, Murthy V, Greenough A. Anatomical deadspace during resuscitation of infants with congenital diaphragmatic hernia. Early Hum Dev. (2020) 149:105150. doi: 10.1016/j.earlhumdev.2020.105150
6. Murthy V, D'Costa W, Nicolaides K, Davenport M, Fox G, Milner AD, et al. Neuromuscular blockade and lung function during resuscitation of infants with congenital diaphragmatic hernia. Neonatology. (2013) 103:112–7. doi: 10.1159/000342332
7. Polglase GR, Blank DA, Barton SK, Miller SL, Stojanovska V, Kluckow M, et al. Physiologically based cord clamping stabilises cardiac output and reduces cerebrovascular injury in asphyxiated near-term lambs. Arch Dis Child Fetal Neonatal Ed. (2018) 103:F530–8. doi: 10.1136/archdischild-2017-313657
8. Lefebvre C, Rakza T, Weslinck N, Vaast P, Houfflin-Debarge V, Mur S, et al. Feasibility and safety of intact cord resuscitation in newborn infants with congenital diaphragmatic hernia (CDH). Resuscitation. (2017) 120:20–5. doi: 10.1016/j.resuscitation.2017.08.233
9. Foglia EE, Ades A, Hedrick HL, Rintoul N, Munson DA, Moldenhauer J, et al. Initiating resuscitation before umbilical cord clamping in infants with congenital diaphragmatic hernia: a pilot feasibility trial. Arch Dis Child Fetal Neonatal Ed. (2020) 105:322–6. doi: 10.1136/archdischild-2019-317477
10. Available, online at: https://clinicaltrials.gov/ct2/show/NCT04373902.
11. Cochius-den Otter SC, Horn-Oudshoorn EJ, Allegaert K, DeKoninck PL, Peters NC, Cohen-Overbeek TE, et al. Routine intubation in newborns with congenital diaphragmatic hernia. Pediatrics. (2020) 146:e20201258. doi: 10.1542/peds.2020-1258
12. Snoek KG, Capolupo I, Van Rosmalen J, de Jongste vanden Hout L, Vijfhuize S, Greenough A, et al. Conventional mechanical ventilation versus high-frequency oscillatory ventilation for congenital diaphragmatic hernia. Ann Surg. (2016) 263:867–74. doi: 10.1097/SLA.0000000000001533
13. Puligandla PS, Grabowski J, Austin M, Hedrick H, Renaud E, Arnold M, et al. Management of congenital diaphragmatic hernia: a systematic review from the APSA outcomes and evidence based practice committee. J PediatrSurg. (2015) 50:1958–70. doi: 10.1016/j.jpedsurg.2015.09.010
14. Bishay M, Giacomello L, Retrosi G, Thyoka M, Garriboli M, Brierley J, et al. Hypercapnia and acidosis during open and thoracoscopic repair of congenital diaphragmatic hernia and esophageal atresia: results of a pilot randomized controlled trial. Ann Surg. (2013) 258:895–900. doi: 10.1097/SLA.0b013e31828fab55
15. Fuyuki M, Usui N, Taguchi T, Hayakawa M, Masumoto K, Kanamori Y, et al. Prognosis of conventional vs. high-frequency ventilation for congenital diaphragmatic hernia: a retrospective cohort study. J Perinatol. (2021) 41:814–23. doi: 10.1038/s41372-020-00833-6
16. Derraugh G, Levesque M, Schantz D, Sesha M, Minski J, Baier J, et al. High-frequency vs. conventional ventilation at the time of CDH repair is not associated with higher mortality and oxygen dependency: a retrospective cohort study. Pediatr Surg Int. (2020) 36:1275–80. doi: 10.1007/s00383-020-04740-x
17. Dreyfuss D, Saumon G. Role of tidal volume, FRC, and end-inspiratory volume in the development of pulmonary edema following mechanical ventilation. Am Rev Respir Dis. (1993) 148:1194–203. doi: 10.1164/ajrccm/148.5.1194
18. Attar MA, Dechert RE, Donn SM. Rescue high frequency ventilation for congenital diaphragmatic hernia. J Neonat Perinat Med. (2019) 12:173–8. doi: 10.3233/NPM-1813
19. Guidry CA, Hranjec T, Rodgers BM, Kane B, McGahren ED. Permissive hypercapnia in the management of congenital diaphragmatic hernia: our institutional experience. J Am Coll Surg. (2012) 214:640–7. doi: 10.1016/j.jamcollsurg.2011.12.036
20. Keszler M. Mechanical ventilation strategies. Semin Fetal Neonat Med. (2017) 22:267–74. doi: 10.1016/j.siny.2017.06.003
21. Duncan KV, Polites S, Krishnaswami S, Scottoline BP. Congenital diaphragmatic hernia management: a systematic review and care pathway description including volume-targeted ventilation. Adv Neonat Care. (2021) 21:E138–43. doi: 10.1097/ANC.0000000000000863
22. Inoue M, Uchida K, Otake K, Nagano Y, Mori K, Hashimoto K, et al. Thoracoscopic repair of congenital diaphragmatic hernia with countermeasures against reported complications for safe outcomes comparable to laparotomy. Surg Endosc. (2016) 30:1014–9. doi: 10.1007/s00464-015-4287-6
23. Qin J, Ren Y, Ma D. A comparative study of thoracoscopic and open surgery of congenital diaphragmatic hernia in neonates. J Cardiothorac Surg. (2019) 14:118. doi: 10.1186/s13019-019-0938-3
24. Okazaki T, Okawada M, Ishii J, Koga H, Miyano G, Doi T, et al. Intraoperative ventilation during thoracoscopic repair of neonatal congenital diaphragmatic hernia. Pediatr Surg Int. (2017) 33:1097–101. doi: 10.1007/s00383-017-4143-y
25. Sakai H, Tamura M, Hosokawa Y, Bryan AC, Barker GA, Bohn DJ. Effect of surgical repair on respiratory mechanics in congenital diaphragmatic hernia. J Pediatr. (1987) 111:432–8. doi: 10.1016/S0022-3476(87)80475-4
26. Takeuchi M, Kinouchi K, Fukumitsu K, Imura K, Kitamura S. Respiratory system compliance and postoperative ventilator dependence in neonates with left-sided congenital diaphragmatic hernia. J Anesth. (2001) 15:139–44. doi: 10.1007/s005400170015
27. Guevorkian D, Mur S, Cavatorta E, Pognon L, Rakza T, Storme L. Lower distending pressure improves respiratory mechanics in congenital diaphragmatic hernia complicated by persistent pulmonary hypertension. J Pediatr. (2018) 200:38–43. doi: 10.1016/j.jpeds.2018.04.027
28. te Pas AB, Siew M, Wallace MJ, Kitchen MJ, Fouras A, Lewis RA, et al. Establishing functional residual capacity at birth: the effect of sustained inflation and positive end-expiratory pressure in a preterm rabbit model. Pediatr Res. (2009) 65:537–41. doi: 10.1203/PDR.0b013e31819da21b
29. Wu Q, Liu J, Liu Y, Jiang Y. Management and experience of postural placement in postoperative mechanical ventilation of newborns. Ann Palliat Med. (2020) 9:1997–2002. doi: 10.21037/apm-20-1003
30. Hunt K, Dassios T, Ali K, Greenough A. Work of breathing at different levels of volume-targeted ventilation in infants with congenital diaphragmatic hernia. Eur Respir J. (2018) 52:PA4663. doi: 10.1183/13993003.congress-2018.PA4663
31. Saugstad OD, Sejersted Y, Solberg R, Wollen EJ, Bjoras M. Oxygenation of the newborn: a molecular approach. Neonatology. (2012) 101:315–25. doi: 10.1159/000337345
32. Lakshminrusimha S, Swartz DD, Gugino SF, Ma CX, Wynn KA, Ryan RM, et al. Oxygen concentration and pulmonary hemodynamics in newborn lambs with pulmonary hypertension. Pediatr Res. (2009) 66:539–44. doi: 10.1203/PDR.0b013e3181bab0c7
33. Victor VM, Rocha M, Esplugues JV, De la Fuente M. Role of free radicals in sepsis: antioxidant therapy. Curr Pharm Des. (2005) 11:3141–58. doi: 10.2174/1381612054864894
34. Thébaud B, Goss KN, Laughon M, Whitsett JA, Abman SH, Steinhorn RH, et al. Bronchopulmonary dysplasia. Nat Rev Dis Primers. (2019) 5:78. doi: 10.1038/s41572-019-0127-7
35. Wedgwood S, Steinhorn RH, Lakshminrusimha S. Optimal oxygenation and role of free radicals in PPHN. Free Radic Biol Med. (2019) 142:97–106. doi: 10.1016/j.freeradbiomed.2019.04.001
36. Paneth N. The evidence mounts against use of pure oxygen in newborn resuscitation. J Pediatr. (2005) 147:4–6. doi: 10.1016/j.jpeds.2005.04.058
37. Riley JS, Antiel RM, Rintoul NE, Ades AM, Waqar LN, Lin N, et al. Reduced oxygen concentration for the resuscitation of infants with congenital diaphragmatic hernia. J Perinatol. (2018) 38:834–43. doi: 10.1038/s41372-017-0031-5
38. Kashyap AJ, Crossley KJ, DeKoninck PL, Rodgers KA, Thio M, Skinner SM, et al. Neonatal cardiopulmonary transition in an ovine model of congenital diaphragmatic hernia. Arch Dis Child Fetal Neonatal Ed. (2019) 104:F617–23. doi: 10.1136/archdischild-2018-316045
39. Conforti A, Giliberti P, Landolfo F, Valfre L, Columbo C, Mondi V, et al. Effects of ventilation modalities on near-infrared spectroscopy in surgically corrected CDH infants. J Pediatr Surg. (2016) 51:349–53. doi: 10.1016/j.jpedsurg.2015.07.021
40. Putnam LR, Tsao K, Morini F, Lally PA, Miller CC, Lally KP, et al. Evaluation of variability in inhaled nitric oxide use and pulmonary hypertension in patients with congenital diaphragmatic hernia. JAMA Pediatr. (2016) 170:1188–94. doi: 10.1001/jamapediatrics.2016.2023
41. Group NINOS. Inhaled nitric oxide and hypoxic respiratory failure in infants with congenital diaphragmatic hernia. Pediatrics. (1997) 99:838–45. doi: 10.1542/peds.99.6.838
42. Cochius-den Otter S, Schaible T, Greenough A, van Heijst A, Patel N, Allegaert K, et al. The CoDiNOS trial protocol: an international randomised controlled trial of intravenous sildenafil versus inhaled nitric oxide for the treatment of pulmonary hypertension in neonates with congenital diaphragmatic hernia. BMJ Open. (2019) 9:e032122. doi: 10.1136/bmjopen-2019-032122
43. Lakshminrusimha S, Keszler M, Kirpalani H, Van Meurs K, Chess P, Ambalavanan N, et al. Milrinone in congenital diaphragmatic hernia - a randomized pilot trial: study protocol, review of literature and survey of current practices. Matern Health Neonatol Perinatol. (2017) 3:27. doi: 10.1186/s40748-017-0066-9
44. Davey M. Surfactant levels in congenital diaphragmatic hernia. PLoS Med. (2007) 4:e243. doi: 10.1371/journal.pmed.0040243
45. Van Meurs K the Congenital Diaphragmatic Hernia Study Group. Is surfactant therapy beneficial in the treatment of the term newborn infant with congenital diaphragmatic hernia? J Pediatr. (2004) 145:312–6. doi: 10.1016/j.jpeds.2004.04.056
46. Lally KP, Lally PA, Langham MR, Hirschl R, Moya FR, Tibboel D, et al. Surfactant does not improve survival rate in preterm infants with congenital diaphragmatic hernia. J Pediatr Surg. (2004) 39:829–33. doi: 10.1016/j.jpedsurg.2004.02.011
47. Colby CE, Lally KP, Hintz SR, Lally PA, Tibboel D, Moya FR, et al. Surfactant replacement therapy on ECMO does not improve outcome in neonates with congenital diaphragmatic hernia. J Pediatr Surg. (2004) 39:1632–7. doi: 10.1016/j.jpedsurg.2004.07.005
48. Boucherat O, Benachi A, Chailley-Heu B, Franco-Montoya ML, Elie C, Martinovic J, et al. Surfactant maturation is not delayed in human fetuses with diaphragmatic hernia. PLoS Med. (2007) 4:e237. doi: 10.1371/journal.pmed.0040237
49. Kurland Y, Gurung K, Pallotto EK, Manimtim W, Feldman K, Staggs VS, et al. Neurally adjusted ventilatory assist in neonates with congenital diaphragmatic hernia. J Perinatol. (2021) 41:1910–5. doi: 10.1038/s41372-021-01098-3
50. Amin R, Arca MJ. Feasibility of non-invasive neurally adjusted ventilator assist after congenital diaphragmatic hernia repair. J Pediatr Surg. (2019) 54:434–8. doi: 10.1016/j.jpedsurg.2018.05.011
51. Meinen RD, Alali YI, Al-Subu A, Wilhelm M, Wraight CL, McAdams RM, et al. Neurally-adjusted ventilatory assist can facilitate extubation in neonates with congenital diaphragmatic hernia. Respir Care. (2021) 66:41–9. doi: 10.4187/respcare.07681
52. Sturrock S, Ambulkar H, Williams EE, Sweeney S, Bednarczuk NF, Dassios T, et al. A randomised crossover trial of closed loop automated oxygen control in preterm, ventilated infants. Acta Paediatr. (2021) 110:833–7. doi: 10.1111/apa.15585
53. Hallenberger A, Poets CF, Horn W, Seyfang A, Urschitz MS. Closed-loop automatic oxygen control (CLAC) in preterm infants: a randomized controlled trial. Pediatrics. (2014) 133:e379–85. doi: 10.1542/peds.2013-1834
54. Gajdos M, Waitz M, Mendler MR, Braun W, Hummler H. Effects of a new device for automated closed loop control of inspired oxygen concentration on fluctuations of arterial and different regional organ tissue oxygen saturations in preterm infants. Arch Dis Child Fetal Neonatal Ed. (2019) 104:F360–5. doi: 10.1136/archdischild-2018-314769
55. Salverda H, Oldenburger N, Rijken M, Pauws S, Dargaville P, Te Pas A. The effect of automated oxygen control on clinical outcomes in preterm infants: a pre-and post-implementation cohort study. Eur J Pediatr. (2021) 180:2107–13. doi: 10.1007/s00431-021-03982-8
56. van den Heuvel M, van Zanten HA, Bachman TE, Te Pas AB, van Kaam AH, Onland W. Optimal target range of closed-loop inspired oxygen support in preterm infants: a randomized cross-over study. J Pediatr. (2018) 197:36–41. doi: 10.1016/j.jpeds.2018.01.077
57. Wise AC, Boutin MA, Knodel EM, Proudfoot JA, Lane BP, Evans ML, et al. Heliox adjunct therapy for neonates with congenital diaphragmatic hernia. Respir Care. (2018) 63:1147–53. doi: 10.4187/respcare.06079
58. Scarpelli EM, Condorelli S, Cosmi EV, Taylor FA. Lamb fetal pulmonary fluid. i. validation and significance of method for determination of volume and volume change. Pediatr Res. (1975) 9:190–5. doi: 10.1203/00006450-197504000-00010
59. Joe P, Wallen LD, Chapin CJ, Lee CH, Allen L, Han VK, et al. Effects of mechanical factors on growth and maturation of the lung in fetal sheep. Am J Physiol Lung Cell Mol Physiol. (1997) 272:L95–105. doi: 10.1152/ajplung.1997.272.1.L95
60. Garcia A, Stolar CJ. Congenital diaphragmatic hernia and protective ventilation strategies in pediatric surgery. Surg Clin North Am. (2012) 92:659–68. doi: 10.1016/j.suc.2012.03.003
61. Hirschl RB, Parent A, Tooley R, Shaffer T, Wolfson M, Bartlett RH. Lung management with perfluorocarbon liquid ventilation improves pulmonary function and gas exchange during extracorporeal membrane oxygenation (ECMO). Artif Cells Blood Substit Immobil Biotechnol. (1994) 22:1389–96. doi: 10.3109/10731199409138842
62. Hirschl RB, Philip WF, Glick L, Greenspan J, Smith K, Thompson A, et al. A prospective, randomized pilot trial of perfluorocarbon-induced lung growth in newborns with congenital diaphragmatic hernia. J Pediatr Surg. (2003) 38:283–9. doi: 10.1053/jpsu.2003.50095
63. American American Academy of Pediatrics Section on Surgery American American Academy of Pediatrics Committee on Fetus and Newborn, Lally KP, Engle W. Postdischarge follow-up of infants with congenital diaphragmatic hernia. Pediatrics. (2008) 121:627–32. doi: 10.1542/peds.2007-3282
64. Koh JY, Jung E, Goo HW, Kim SC, Kim DY, Namgoong JM, et al. Functional and structural evaluation in the lungs of children with repaired congenital diaphragmatic hernia. BMC Pediatr. (2021) 21:120. doi: 10.1186/s12887-021-02586-3
Keywords: mechanical ventilation, pressure controlled ventilation, volume controlled ventilation, high frequency oscillation, surfactant, inhaled nitric oxide
Citation: Williams E and Greenough A (2021) Respiratory Support of Infants With Congenital Diaphragmatic Hernia. Front. Pediatr. 9:808317. doi: 10.3389/fped.2021.808317
Received: 03 November 2021; Accepted: 01 December 2021;
Published: 24 December 2021.
Edited by:
Gianluca Lista, Ospedale dei Bambini Vittore Buzzi, ItalyReviewed by:
Manuel Sanchez Luna, Complutense University of Madrid, SpainUlrich Herbert Thome, Leipzig University, Germany
Martin Keszler, Women & Infants Hospital of Rhode Island, United States
Copyright © 2021 Williams and Greenough. This is an open-access article distributed under the terms of the Creative Commons Attribution License (CC BY). The use, distribution or reproduction in other forums is permitted, provided the original author(s) and the copyright owner(s) are credited and that the original publication in this journal is cited, in accordance with accepted academic practice. No use, distribution or reproduction is permitted which does not comply with these terms.
*Correspondence: Anne Greenough, YW5uZS5ncmVlbm91Z2hAa2NsLmFjLnVr