- 1Department of Pediatrics, Faculty of Medicine, Kagawa University, Kagawa, Japan
- 2Division of Analytical Technology, Department of Medical Technology, Kagawa Prefectural University of Health Sciences, Kagawa, Japan
- 3Maternal Perinatal Center, Faculty of Medicine, Kagawa University, Kagawa, Japan
Human fetal and neonatal bilirubin metabolism is centered on 4Z,15Z-bilirubin IXα (BR) due to the extremely low BR conjugating capacity of the liver. BR is a unique, highly lipophilic substance with physiological and toxic effects in the cell membranes of organs and body tissues. The fetus excretes BR through the placenta to the maternal circulation. After birth, BR is thought to act as an antioxidant against the increase in reactive oxygen species caused by the rapid increase in oxygen concentration during the adaptation process from in amniotic fluid to in air. However, bilirubin encephalopathy is a toxic effect of bilirubin. Due to the lipophilic nature of BR, it must be bound to a carrier to be distributed to various parts of the body by hydrophilic blood. This carrier of BR is human serum albumin (HSA). In humans, BR can be excreted efficiently after undergoing photochemical reactions upon high affinity binding to HSA. HSA also plays an important role in the prevention of bilirubin encephalopathy. This review focuses on the developmental and physiological role of bilirubin metabolism during the fetal and neonatal periods.
1. Introduction
4Z,15Z-Bilirubin IXα (BR) and human serum albumin (HSA) have unique chemical structures that underlie their physiological importance in humans. Nonetheless, their in vivo actions are not fully understood. In particular, bilirubin has been the focus of considerable attention because it causes bilirubin encephalopathy. In 1954, Bernhard et al. reported that bilirubin stabilizes and improves the absorption of vitamin A and linoleic acid, a polyunsaturated fatty acid, in the intestinal tract (1). The stabilizing effect of bilirubin on oxidizable substances was subsequently reported (2–5). The antioxidant properties of bilirubin have also gained attention in terms of its physiological effects in preterm and term infants who have weak protection against activated oxygen as well as in terms of why humans in particular develop neonatal jaundice (6, 7). BR binds with high affinity to HSA, which is responsible for the very low levels of free BR in the blood and its wide distribution in the body (8, 9). However, HSA is uniquely evolved compared with the serum albumin in non-human primates in terms of its photochemical reaction with BR (10–13). This review aims to elucidate the following basic issues, with a focus on physiological effects: (1) the chemical structure and metabolism of BR; (2) the oxidation products of BR; (3) the photochemical reaction of BR in phototherapy for neonatal hyperbilirubinemia; (4) fetal bilirubin metabolism; and (5) neonatal bilirubin metabolism.
2. Chemical structure and metabolism of 4Z,15Z-bilirubin IXα
BR is a tetrapyrrole with four imino groups, two carbonyl groups, and two propionic acid groups. Despite the presence of the many polar groups, BR is extremely insoluble in water. This is due to the intramolecular hydrogen bonding of these polar groups and the presence of hydrophobic groups such as methyl and vinyl groups on the surface of the BR molecule (14, 15). The cleavage of protoheme IX (heme B) at the α position is essential for the formation of the BR structure, and the substrate specificity of the cleavage enzyme, heme oxygenase, is vital. This specificity is reported to be due to the electrostatic interaction of the propionate groups of heme B with Lys-18, Lys-179, and Arg-183 of the heme oxygenase protein and hydrogen bonding with Tyr-134, which fixes the α–γ axis of heme B, resulting in a fixed binding direction between heme B and heme oxygenase (16, 17).
For BR to be distributed throughout the body, it needs a carrier in the hydrophilic bloodstream. Its hydrophobic physicochemical properties make it suitable for distribution in lipids, mainly in cell membranes. HSA serves this purpose. BR is thought to bind with high affinity to HSA at domain IIA with a binding constant on the order of 107 and to be carried and distributed to various organs and tissues where it exerts antioxidant effects (18–20). The amino acid pocket of HSA is a salt-type right-hand chirality enantiomer with some of the BR hydrogen bonds broken and hydroxyl groups displayed, and it is ionically bound with high affinity to Lys-190 and hydrophobic bonding to hydrophobic amino acids (21, 22). The binding of BR to HSA allows for unique photochemical reactions due to the asymmetric structure of the dipyrrole facing its methylene bridge and the conformation of the bound BR (see Photochemistry of 4Z,15Z-bilirubin IXα in phototherapy for neonatal hyperbilirubinemia).
The antioxidant effect of BR involves both scavenging and quenching activity. In the former pathway, BR combines with reactive oxygen species (ROS) to form oxidation products, and in the latter pathway, BR is oxidized by electron transfer to produce biliverdin, which can be metabolized back to BR by biliverdin reductase, a widely distributed enzyme in the body. However, it is difficult to assess the in vivo antioxidant activity of this cycle (23).
3. Oxidation products of 4Z,15Z-bilirubin IXα
To validate the evidence indicating that BR acts as an antioxidant, it is necessary to demonstrate both a decrease in BR and an increase in BR oxidation products resulting from the scavenging and quenching of ROS. Therefore, the identification of BR and its oxidation products is important. The oxidation products of BR are shown in Figure 1 and comprise mainly tetrapyrroles, tripyrroles, dipyrroles, and monopyrroles and their oxidized metabolites (27–30). In the past, it was thought that BR was itself a photosensitizer and that this oxidative pathway was the primary route of BR metabolism in phototherapy for neonatal hyperbilirubinemia (32). However, it was clarified that the photochemical reactions of BR are not type II photochemical reaction in which energy is transferred from the photoexcited state of BR to triplet oxygen to generate singlet oxygen that oxidizes BR (33, 34). Elucidation of the photochemical reactions of BR revealed that the main reactions are geometric isomerization and subsequent structural isomerization, while the aforementioned tetrapyrrole oxidation products remain intact (see Photochemistry of 4Z,15Z-bilirubin IXα in phototherapy for neonatal hyperbilirubinemia).
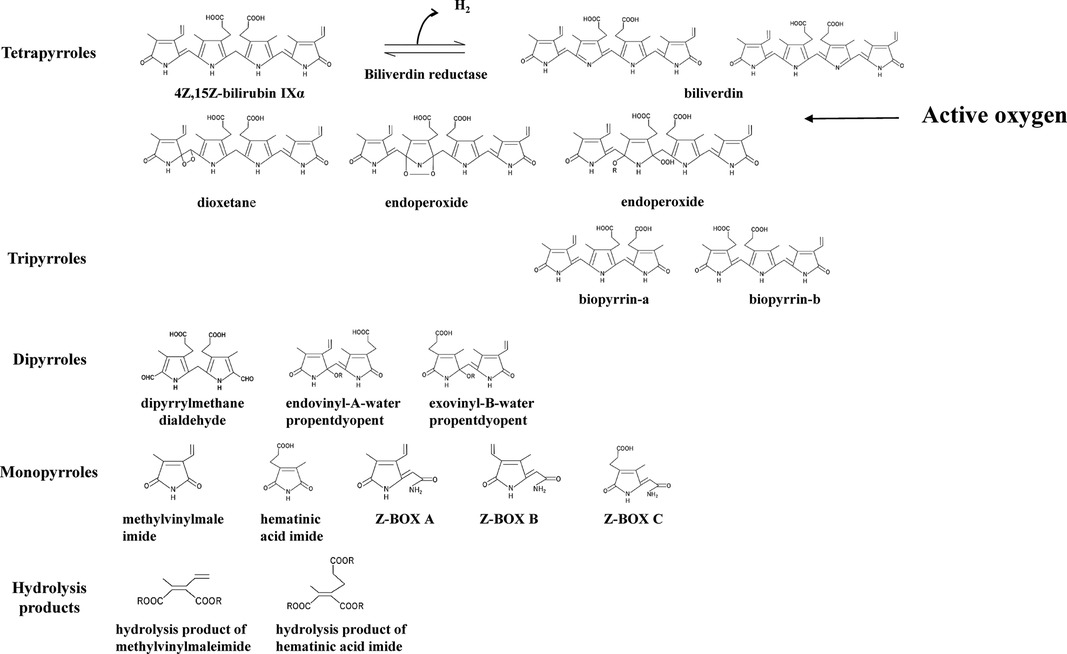
Figure 1. 4Z,15Z-bilirubin IXα and bilirubin oxidation products. The bilirubin oxidation products formed from 4Z,15Z-bilirubin IXα are divided into tetrapyrroles, tripyrroles, dipyrroles, monopyrroles, and hydrolysis products with their corresponding structures. Bilirubin oxidation products produced by quenching activity: biliverdin. Bilirubin oxidation products produced by scavenging activity: oxidizing substances other than biliverdin. The abbreviations and the articles in which they are listed: biliverdin (23–26); biopyrrin-a and biopyrrin-b (27); exovinyl-B-water propentdyopent, endovinyl-B-water propentdyopent, and hematinic acid imide (28); Z-BOX, Z-bilirubin oxidant product; Z-BOX A and Z-BOX B (29); Z-BOX C (30); hydrolysis product of methylvinylmaleimide and hematinic acid imide (28); and exovinyl-B-water propentdyopent & endovinyl-B-water propentdyopent [propentdyopents determined by pentdyopent reaction (23, 31)].
Turning to the BR oxidation products, biliverdin produced by quenching activity (electron transfer only) and the bilirubin oxidation products produced by scavenging activity (reaction with ROS) have been detected in vivo. The following products have been identified: biliverdin (24–26), biotripyrrin-a (27), biotripyrrin-b (27), exovinyl-B-water propentdyopent (28), endovinyl-B-water propentdyopent (28), hematinic acid imide (28), Z-BOX A (29), Z-BOX B (29), Z-BOX C (30), and a hydrolysis product of methylvinylmaleimide and hematic acid imide (28).
4. Photochemistry of 4Z,15Z-bilirubin IXα in phototherapy for neonatal hyperbilirubinemia
The major photochemical reaction of BR occurring during phototherapy is shown in Figure 2 (35–38). BR bound with high affinity to domain IIA of HSA undergoes specific photochemical reactions, which is explained in the section on physiological aspects in this review. The major product of the geometric isomerization reaction is 4Z,15E-bilirubin IXα, while the major product of the structural isomerization reaction is 4E,15Z-cyclobilirubin IXα (Z-lumirubin) (39). 4Z,15E-Bilirubin IXα moves to domain IB, where HSA binding is weak (40), and the levels of its free form increase. This form is excreted from the liver into the bile and then may reconvert to BR in the digestive tract to be retained in the body through the enterohepatic circulation. BR excreted into the intestinal tract is thought to play a role in antioxidant activity. In blood, the amount of BR bound to domain IIA of HSA is reduced and toxic free BR can bind again at the domain II site, resulting in a decrease in free BR (10, 41–43).
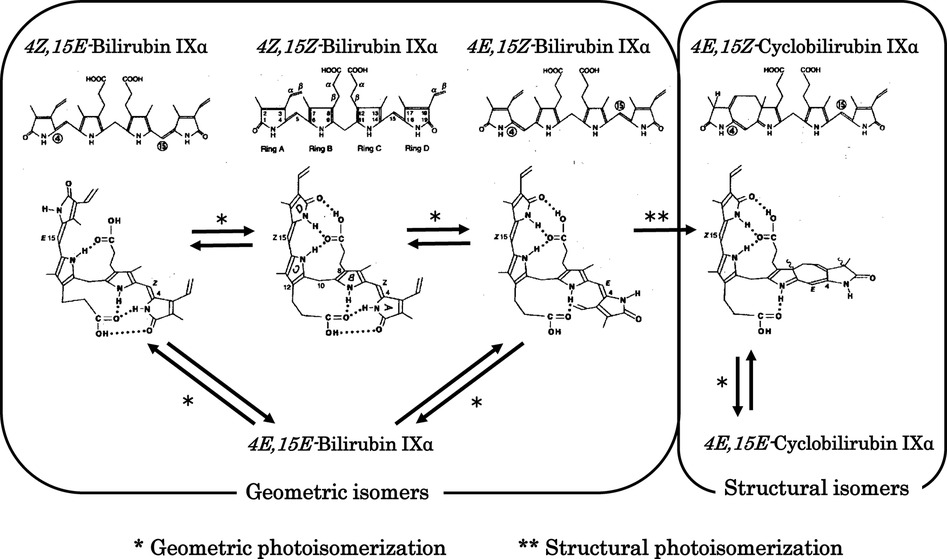
Figure 2. Photochemical changes in 4Z,15Z-bilirubin IXα. Photochemical changes in 4Z,15Z-bilirubin IXα in bilirubin–human serum albumin are predominantly to 4Z,15E-bilirubin IXα and cyclobilirubin IXα (lumirubin). Storage route of bilirubin: 4Z,15E-Bilirubin IXα is excreted from the liver into the bile and then may reconvert to 4Z,15Z-bilirubin IXα in the digestive tract to be retained in the body through the enterohepatic circulation. Elimination route of bilirubin: 4E,15Z-Cyclobilirubin IXα (Z-lumirubin) and 4E,15E-cyclobilirubin IXα (E-lumirubin) are the most important pathways affecting the clinical efficacy of phototherapy because they polymerize into a dark brown substance that causes bronze baby syndrome, an adverse reaction to phototherapy, and is ultimately excreted from the body.
4E,15Z-Cyclobilirubin IXα is the most important compound formed during phototherapy because it polymerizes into a dark brown substance that causes bronze baby syndrome, an adverse reaction to phototherapy, and is ultimately excreted from the body (44, 45). In particular, the rate of formation of 4E,15Z-cyclobilirubin IXα via 4E,15Z-bilirubin IXα from BR bound to HSA is much higher than for other albumins in non-human primates (10–13, 46–50). Phototherapy LED light centered at turquoise and green wavelengths produces more cyclobilirubin and less 4Z,15E-bilirubin (50, 51). The asymmetric structure of the dipyrroles centered on the methylene bridge of BR is important for effectively achieving both geometric and structural isomerization reactions. In contrast, it is difficult to achieve these reactions for the symmetric 4Z,15Z-bilirubin IIIα and XIIIα (52). In solution with various acid catalysts, BR can undergo rearrangement to a mixture bilirubin IIIα, BR and XIIIα through structural isomerization (intermolecular dipyyrole exchange) (53). This isomerization reaction has caused many erroneous results in vitro (54), but plays no important role in bilirubin photoisomerization in vivo (55).
In the bilirubin oxidation pathway, the total excretion of BR oxidation products during phototherapy is very low (28). Before phototherapy, hematinic acid imide and hydrolysis products of methylvinylmaleimide and hematinic acid imide are detected by high-performance liquid chromatography. They increase during phototherapy, and exovinyl-B-water propentdyopent and endovinyl-B-water propentdyopent are present as well (28). We speculate that BR is oxidized and acts as an antioxidant even before phototherapy. According to a previous report (28), the increase in these BR oxidation products during phototherapy is considered to reflect the BR antioxidant activity. This increase indirectly indicates an increase in ROS generated endogenously by photosensitizers such as riboflavin, which is highly photosensitizing in the blue wavelength region (56, 57). Irradiation of a BR–HSA complex solution containing riboflavin with blue-white light induces a greater increase in BR oxidation products compared with green fluorescent light (58). The wavelength characteristics of the phototherapy light source are important for clinical efficacy and green light also has sufficient clinical benefits (51, 59–62). However, the photosensitizing effect of endogenous substances as an adverse reaction is also important, and green light is thought to lessen the severity of this adverse reaction compared with conventional blue light (58).
5. Fetal bilirubin metabolism
During the fetal period, bilirubin-producing enzymes and bilirubin-conjugating enzymes are developmentally specific. The substrate specificity of the heme oxygenase that produces biliverdin is important (63, 64). From 14 to 15 weeks of gestation, bilirubin IXβ, which cannot form intramolecular hydrogen bonds, is the major component; it is excreted in bile and urine in an intact form without conjugation (65, 66). Bilirubin IXα is present at 16–17 weeks of gestation, but bilirubin IXβ predominates until 20 weeks of gestation (65). Bilirubin IXδ, bilirubin IXγ, and biliverdin also have physicochemical properties similar to those of bilirubin IXβ (66, 67), but the physiological significance of bilirubin IXβ during development is unknown.
The development of BR conjugation leads to the presence of xylose, glucose, and unidentified monoconjugated bilirubin at around 20 weeks of gestation, with monoglucuronosyl bilirubin appearing only at 20–23 weeks in bile. Bilirubin monoconjugated to glucose or xylose predominates up to 30 weeks, while monoglucuronosyl bilirubin becomes the predominant form at term (65). Bilirubin UDP-glucuronosyltransferase activity in the fetal human liver is 1/1,000 of that in adults until 30 weeks of gestation, after which it increases to 1/100 of that at term (68). Thus, hepatic bilirubin UDP-glucuronosyltransferase activity is extremely low during the fetal period. An overview of fetal bilirubin metabolism is shown in Figure 3 (69). Bilirubin IXβ and conjugated bilirubin are substances that accumulate in the fetal intestine and amniotic fluid, whereas bilirubin IXα is excreted by the mother after crossing the placenta. In this regard, extremely low hepatic bilirubin UDP-glucuronosyltransferase activity and placental function are important for the physiology of fetal developmental. In contrast, the physiological effects of the bilirubin IXβ that accumulates in the fetal intestine and amniotic fluid have not been elucidated, although a possibility is that it has a stabilizing effect on easily oxidized substances (1–4). Abnormalities in fetal bilirubin metabolism include fetal hemolytic disease due to Rh-incompatible hemolytic disease on the fetal side. If placental function is normal, fetal hyperbilirubinemia is not a concern because the fetus excretes BR through the placenta to the maternal circulation, although fetal hydrops due to severe anemia is a problem (70). Maternal Dubin-Johnson syndrome has a high rate of miscarriage (71). In Gunn rats, maternal hyperbilirubinemia has been reported to decrease pregnancy rates (72). In humans, maternal hyperbilirubinemia increases preterm birth rates (73), but the prognosis for the infants is better in late pregnancy (74).
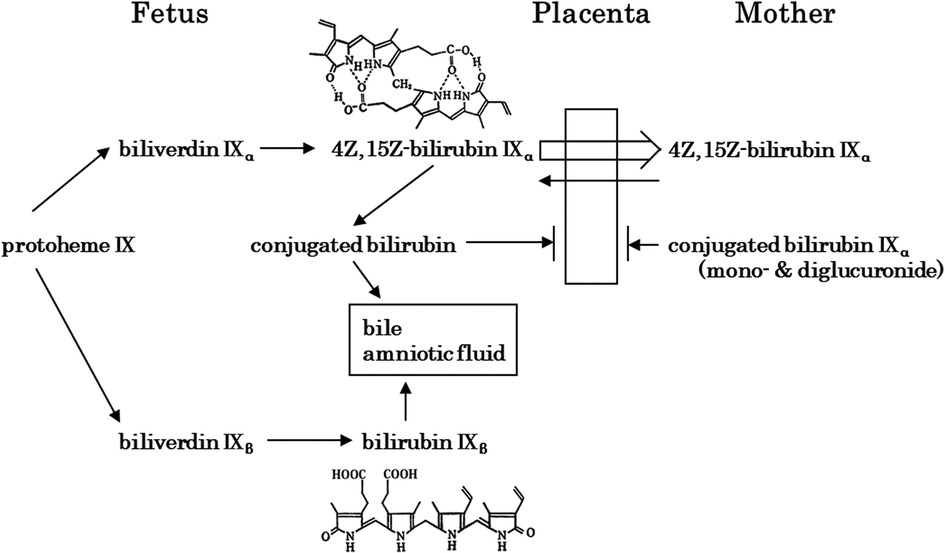
Figure 3. Fetal bilirubin metabolism [modified in part from itoh (65)]. Bilirubin IXβ, which is produced in early fetal life, is hydrophilic and is excreted via the gastrointestinal tract and amniotic fluid. In contrast, 4Z,15Z-bilirubin IXα, which is produced later in life, is hydrophobic and crosses the placenta for excretion by the mother. At the fetal stage, the extremely low activity of 4Z,15Z-bilirubin IXα-conjugating enzymes is important.
6. Bilirubin metabolism in neonates
After birth, maternal excretion of bilirubin from the placenta no longer occurs, and the neonate’s own ability to metabolize and excrete bilirubin is required. In that process, physiological neonatal jaundice can develop, which involves the production, excretion, and enterohepatic circulation of BR. Bilirubin production in neonates is reported to be 8.5 mg/kg/day, about twice that in adults (75). This is due to the shorter erythrocyte lifespan of about 52 days in neonates (76) versus about 120 days in adults (77, 78). Placental transfusion of red blood cells to infants occurs at birth, which acts as a source of BR. Approximately 20 ml/kg of blood flows to the infant from the placenta by the time the umbilical cord pulsation stops (79). The BR load is calculated by assuming that the baby weighs 3 kg and that the hemoglobin (Hb) concentration of the cord blood is 15 g/dL. The following is a summary of the formula (Figure 4).
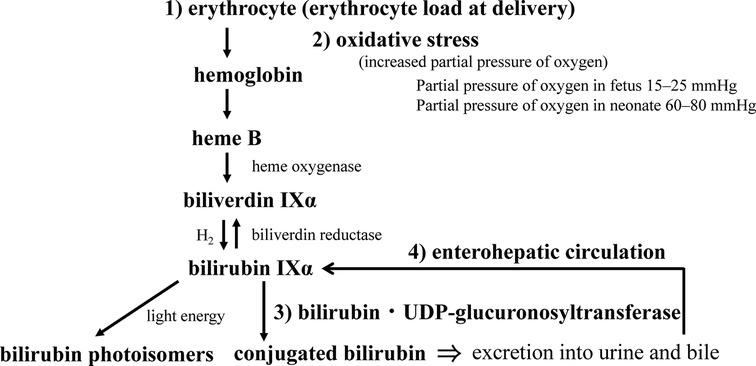
Figure 4. Neonatal bilirubin metabolism. The perinatal metabolism of bilirubin is summarized in terms of (1) bilirubin load, (2) its response to oxidative stress at birth, (3) the development of conjugating enzymes, and (4) the significance of the enterohepatic circulation (see text for details).
This load can be adjusted by the time of cord clamping at delivery. Early cord clamping has been reported to decrease the effectiveness of phototherapy in a comparative study of Japanese term infants (80), but other studies have reported different results (81–83). The American College of Obstetricians and Gynecologists has suggested that many newborns—not only preterm infants but also term infants—would benefit greatly from a cord clamping delay of 30–60 s or more (84). However, this recommendation requires consideration of the impact of race on neonatal jaundice. Peak serum bilirubin levels of physiological jaundice are more than twice as high in Asians as in Caucasians, and many Asian newborns develop neonatal hyperbilirubinemia (85–87). If the bilirubin levels requiring treatment are the same, the number of patients treated for the same bilirubin load will be higher in the Asian population. This is also why the hour-specific nomogram of transcutaneous bilirubin concentration, which is used to identify treatment reference subjects, should also be generated for each race (88). In other words, applying the hour-specific nomogram of the transcutaneous bilirubin concentration created for Caucasians to Asians would increase the number of neonates eligible for blood sampling.
Erythrocyte lifespan is believed to be shortened in neonates due to differences between the membranes of neonatal and adult erythrocytes. The relationship between fetal hemoglobin and erythrocyte lifespan has been examined with respect to subunit interface strength. Erythrocytes with a weaker interface strength have a shorter lifespan. The dimer interface strength of fetal hemoglobin is weaker than that of adult hemoglobin, representing maturation from weaker to stronger monomer-monomer subunit contacts (89). Neonatal erythrocyte membranes have been reported to exhibit mechanical fragility (90). We compared ROS production in response to oxidative stress between cord blood and maternal erythrocytes in vitro. The production of ROS was significantly higher in cord blood erythrocytes than in maternal erythrocytes (91). The low vitamin E content (92, 93) and high lipid composition with high levels of active hydrogen in neonatal erythrocyte membranes is believed to be the cause. However, the reason for the significant positive correlation of ROS production between the mother and neonate is unknown. Regarding racial differences in neonatal bilirubin production, one study found significantly higher levels of serum carboxyhemoglobin in Japanese neonates than in Caucasian neonates (94). Thus, bilirubin is thought to exert some physiological effects due to its increased production in the neonatal period, causing neonatal jaundice.
An important aspect of the metabolic pathway for bilirubin excretion is the bilirubin conjugating capacity of the liver. The capacity in the liver of term neonates is about 1% of that of adults, with a rapid increase in activity at birth that lasts until 90 postnatal days, after which it plateaus until adulthood (Figure 5A) (95). It has also been demonstrated that the activity increases after birth in preterm infants (Figure 5B) (68). Many studies have reported the effects of genetic polymorphisms in UGT1A1 on neonatal jaundice, including breast milk jaundice and preterm jaundice, with UGT1A1 TATA-box polymorphism (96), UGT1A1*6 (97–100), and UGT1A1*28 (101) as the reported jaundice enhancers. As an excretion route other than conjugation, photoequilibrium between BR and 4Z,15E-bilirubin IXα is established in bright environments, and 4Z,15E-bilirubin IXα is excreted into the bile without conjugation. However, 4E,15Z-cyclobilirubin IXα is produced at low amounts, depending on the amount of ambient light energy (26, 39, 102). Conjugated bilirubin and photoisomers of bilirubin excreted in the bile can be reconverted to BR in the gastrointestinal tract and are reabsorbed in the intestines, where they enter the enterohepatic circulation. Conjugated bilirubin is metabolized back to BR by the underdeveloped intestinal microbiome and high β-glucuronidase activity in the intestinal tract and breast milk (103). The free 4Z,15E-bilirubin IXα is easily reconverted to the BR in a process that requires energy, such as body temperature (37). Thus, the neonatal period is designed to store BR in vivo.
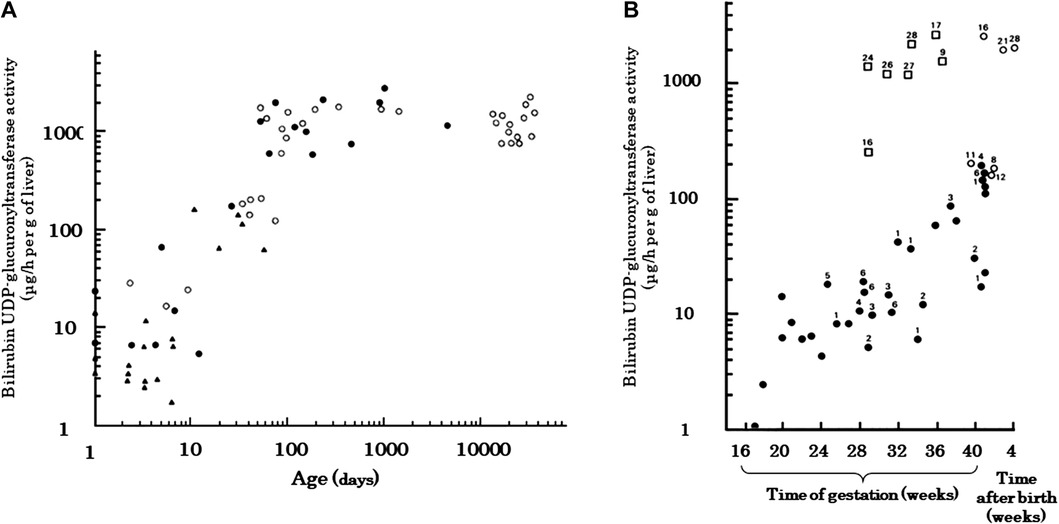
Figure 5. Developmental change in human hepatic bilirubin UDP-glucuronosyltransferase activity [adapted from onishi et al. (95) and Kawade and Onishi (68)]. (A) Measurements from newborn to adult. Both the ordinate and the abscissa are plotted on logarithmic scales. ●, autopsied cases; ○, upper abdominal laparotomy; ▴, preterm neonates. (B) Relationship between enzyme activity and survival. Preterm delivery, irrespective of gestational age, evokes an early increase in transferase activities, equal in rate to the normal postnatal increase. The number shown beside the symbols represent the age (days) at which death occurred. Transferase activities are also shown for preterm (□) and term (○) infants who lived more than 8 days after birth. ●, Transferase activities for fetuses and preterm and term infants who died within 7 days of birth.
The enterohepatic circulation of this bilirubin is believed to play an important role in breast milk jaundice, which is considered physiological. Reported causes of breast milk jaundice include inhibitors of hepatic UGT1A1 in breast milk (pregnane-3α, 20β-diol, non-esterified fatty acid), increased enterohepatic circulation (β-glucuronidase, epidermal growth factor), genetic polymorphisms (UGT1A1 mutations such as UGT1A1*6 and UGT1A1*28), dehydration, starvation, IL-1β, and α-fetoprotein (97–101, 104–106). Of these, the elevation of the enterohepatic circulation is reported to be due to the presence of β-glucuronidase (103) and epidermal growth factor (107) in breast milk, and the influence of inhibitors of intestinal UGT1A1 activity in breast milk has also been identified recently. In neonatal hUGTI mice, UGT1A1 metabolizes bilirubin in the gastrointestinal tract to prevent the accumulation of bilirubin. Intestinal UGT1A1 is under control by IKK/NF-кB signaling. Breast milk can inhibit the NF-кB mediated transcription of UGT1A1 (108).
When considering the antioxidant role of bilirubin in neonates, it is necessary to consider the extent to which bilirubin acts relative to many low-molecular-weight antioxidants. In terms of antioxidant concentrations, neonates have higher levels of bilirubin, uric acid, and vitamin C, but lower levels of vitamin E, carotenoids, and vitamin A compared with adults, and bilirubin is often present at the highest concentration among these antioxidants. However, albumin, haptoglobin, and hemopexin, which are proteins with antioxidant properties, are at low levels, while transferrin is high in response to Fe2+, which tends to increase in the neonatal period (109). Serum bilirubin concentrations have been studied in relation to the reduction in antioxidant capacity and oxidants (Table 1) and in relation to diseases caused by ROS damage. The relationship between serum bilirubin and total antioxidant status appears to be affected by low concentrations of bilirubin, and the status is remarkably robust at high concentrations of bilirubin. However, conflicting results have been obtained for total oxidant status, which is expected to decrease with elevation of bilirubin due to its antioxidant effect, which increases with bilirubin concentration (110, 111). Neonatal diseases that develop due to ROS damage, including retinopathy of prematurity, intraventricular hemorrhage, necrotizing enterocolitis, chronic lung disease (bronchopulmonary dysplasia), and sepsis or severe fungal infection, were evaluated via a comparison of bilirubin levels with those of controls. The results did not provide sufficient evidence that bilirubin prevents the development of these diseases through its antioxidant effect (112, 113). When considering the antioxidant effects of bilirubin, it is necessary to examine the relationship between the decrease in bilirubin and the increase in BR oxidation products. In basic in vitro studies of phototherapy light sources for neonatal hyperbilirubinemia, the reduction in BR levels with light irradiation was thought to be mainly due to photochemical changes (Figure 2) and also due to a small amount of cyclobilirubin polymerization and BR oxidation products. Under conditions with minimal cyclobilirubin polymerization, the amount of BR oxidation products is [amount of BR reduced−amount of total BR photoisomer]. Under these conditions, a comparison of the phototherapy light source with blue-white and green fluorescent light demonstrated that there are fewer BR oxidation products with green light under the addition of flavin mononucleotide (58). In addition, methylxanthine derivatives exhibited enhanced production of BR oxidation products under blue-white fluorescent light irradiation in the presence of flavin mononucleotide (114). The Umu test, which measures DNA mutagenicity, was also significantly higher under blue-white fluorescent light compared with green fluorescent light (115).
Daylight irradiation is reported to cause DNA damage in vitro, which was attributed not to bilirubin but to ROS generated by endogenous substances (116, 117). In vivo effects include those seen in a famous animal study where Gunn rats were treated with riboflavin-5-phosphate and exposed to blue light, which caused adverse reactions such as blistering and bleeding (56). These results are also thought to be due to ROS produced by the photosensitizing effect of riboflavin irradiated with light at sensitive wavelengths.
Regarding breast milk jaundice, vitamin A and unsaturated fatty acids, which were first noted for their antioxidant effects on bilirubin, may act as stabilizers in the intestinal tract (1–5). In our previous study, there was a significant positive correlation between serum BR and urinary propentdyopent reactive substances (31). Breast milk jaundice may also have some physiological effect due to the antioxidant effects of bilirubin. We hope that further progress in the identification of BR oxidation products will clarify the role of bilirubin in diseases caused by ROS toxicity in the neonatal period.
7. Conclusion
Bilirubin metabolism in the fetus and neonate is centered on BR. The physiological significance of neonatal jaundice, including breast milk jaundice, which occurs only in humans and rhesus monkeys, needs to be studied in terms of antioxidant effects. Phototherapy for neonatal hyperbilirubinemia requires the development of safe and effective light sources that do not increase BR oxidation products, and it is also necessary to determine a safe threshold for the bilirubin level that does not exert bilirubin toxicity but still has antioxidant effects.
Author contributions
SI contributed to the conception and design of this review and drafted the manuscript. HO, YK, and TI prepared the figures and table. KK, SN, and TK performed English editing and literature review. TK critically reviewed the manuscript. All authors contributed to the article and approved the submitted version.
Funding
This study was financially supported by JSPS KAKENHI Grants-in-Aid (Nos. 19K08253, 19K08349, 20H00102, 21K02709, 22K02408, and 22K07822), and the research grant from the Ministry the Health, Labour and Welfare of Japan (No. 21FC1001).
Acknowledgments
This review includes many results made possible by S. Onishi and N. Kawade, who have passed away, and our co-workers of Nagoya City University and Kagawa University. We thank them and are grateful to the scientists, biochemists, and pediatricians who have published their contributions.
Conflict of interest
SI received an honorarium from Konica Minolta, Inc. who supervised the use of the hour-specific nomogram JM-105. The remaining authors declare that the research was conducted in the absence of any commercial or financial relationships that could be construed as a potential conflict of interest.
Publisher's note
All claims expressed in this article are solely those of the authors and do not necessarily represent those of their affiliated organizations, or those of the publisher, the editors and the reviewers. Any product that may be evaluated in this article, or claim that may be made by its manufacturer, is not guaranteed or endorsed by the publisher.
References
1. Bernhard K, Ritzel G, Steiner KU. On a biological significance of bile pigments: bilirubin and biliverdin as antioxidants for vitamin A and essential fatty acids. Helv Chim Acta. (1954) 37:306–13. doi: 10.1002/hlca.19540370139
2. Beer H, Bernhard K. The effect of bilirubin and vitamin E on the oxidation of unsaturated fatty acids by ultraviolet irradiation. Chimia. (1959) 13:291–2.
3. Kaufman HP, Garloff H. Pro- and antioxidants in lipid reach II: on naturally occurring antioxidants, I. A report. Fette Seifen Anstrichmittel. (1961) 63:334–44. doi: 10.1002/lipi.19610630406
4. Onishi S, Yamakawa T, Ogawa J. Photochemical and photobiological studies on light-treated newborn infant. XIII International congress of pediatrics, vol. 1, perinatology (1971). p. 373–9
5. Stocker R, Peterhans E. Synergistic interaction between vitamin E and the bile pigments bilirubin and biliverdin. Biochim Biophys Acta. (1989) 1002:238–44. doi: 10.1016/0005-2760(89)90293-2
6. Onishi S, Itoh S, Isobe K. Chapter 4: Physiology of bilirubin metabolism. In: Balistreri WF, Ohi R, Todani T, Tsuchida Y, editors. Hepatobiliary, splenic and pancreatic disease in children: Medical and surgical management. Tokyo: Elisevier (1997). p. 37–70.
7. McDonagh AF. Is bilirubin good for you. Clin Perinatol. (1990) 17:359–69. doi: 10.1016/S0095-5108(18)30572-4
8. Brodersen R. Bilirubin; solubility and interaction with albumin and phospholipid. J Biol Chem. (1979) 254:2364–9. doi: 10.1016/S0021-9258(17)30230-2
9. Weisiger RA, Ostrow JD, Koehler RK, Webster CC, Mukerjee P, Pascolo L, et al. Affinity of human serum albumin for bilirubin varies with albumin concentration and buffer composition: results of a novel ultrafiltration method. J Biol Chem. (2001) 276:29953–60. doi: 10.1074/jbc.M104628200
10. Iwase T, Kusaka T, Itoh S. (EZ)-cyclobilirubin formation from bilirubin in complex with serum albumin derived from various species. J Photochem Photobiol B. (2010) 98:138–43. doi: 10.1016/j.jphotobiol.2009.11.012
11. Okada H, Itoh S, Nii K, Sugino M, Fuke N, Koyano K, et al. Bilirubin photoisomers in rhesus monkey serum. J Photochem Photobiol B. (2018) 185:50–4. doi: 10.1016/j.jphotobiol.2018.05.019
12. Nii K, Okada H, Itoh S, Kusaka T. Characteristics of bilirubin photochemical changes under green light-emitting diodes in humans compared with animal species. Sci Rep. (2021) 11:6391. doi: 10.1038/s41598-021-85632-5
13. Okada H, Itoh S, Fuke N, Koyano K, Suzuki T, Yasuda S, et al. Species differences in bilirubin photoisomers formation by blue fluorescent lamp. International conference on CME (Complex medical and engineering) 2020, takamatsu city in Japan (2020).
14. Bonnett R, Davis JE, Hursthouse MB. Structure of bilirubin. Nature. (1976) 262:326–8. doi: 10.1038/262326a0
15. Bonnett R, Davis JE, Hursthouse MB. The structure of bilirubin. Prc R Soc London B Biol Sci. (1978) 202:249–68. doi: 10.1098/rspb.1978.0066
16. Zhou H, Migita CT, Sato M, Sun D, Zhang X, Ikeda-Saito M, et al. Participation of carboxylate amino acid side chain in regiospecific oxidation of heme by heme oxygenase. J Am Chem Soc. (2000) 122:8311–2. doi: 10.1021/ja0002868
17. Wang J, Lad L, Poulos TL, Ortiz de Montellano PR. Regiospecificity determinants of human heme oxygenase: differential NADPH- and ascorbate-dependent heme cleavage by the R183E mutant. J Biol Chem. (2005) 280:2840–6. doi: 10.1074/jbc.M409526200
18. Jacobsen J, Brodersen R. The effect of pH on albumin-bilirubin affinity. Birth Defects Orig Artic Ser. (1976) 12:175–8.8170
19. Sir Ranjini A, Das P, Balaram P. Binding constant measurement by hyper-Rayleigh scattering: bilirubin-human serum albumin binding as a case study. J Phys Chem B. (2005) 109:5950–3. doi: 10.1021/jp047268n
20. Moosavi-Movahedi Z, Safarian S, Zahedi M, Sadeghi M, Saboury AA, Chamani J, et al. Calorimetric and binding dissections of HSA upon interaction with bilirubin. Protein J. (2006) 25:193–201. doi: 10.1007/s10930-006-9002-y
21. Mugnoli A, Manitto P, Monti D. Structure of di-isopropylammonium bilirubinate. Nature. (1978) 273:568–9. doi: 10.1038/273568a0
22. Lightner DA, Reisinger M, Landen GL. On the structure of albumin-bound bilirubin: selective binding of intramoleculary hydrogen-bonded conformational enantiomers. J Biol Chem. (1986) 261:6034–8. doi: 10.1016/S0021-9258(17)38489-2
23. Kunikata T, Itoh S, Ozaki T, Kondo M, Isobe K, Onishi S. Formation of propentdyopnets and biliverdin, oxidized metabolites of bilirubin, in infants receiving oxygen therapy. Pediatr Int. (2000) 42:331–6. doi: 10.1046/j.1442-200x.2000.01246.x
24. Knobloch E, Mandys F, Hodr R, Hujer R, Mader R. Study of the mechanism of the photoisomerization and photooxidation of bilirubin using a model for the phototherapy of hyperbilirubinemia. J Chromatogr. (1991) 566:89–99. doi: 10.1016/0378-4347(91)80113-Q
25. Nishida T, Itoh S, Onishi S, Isobe K, Terushita M, Ishi Y, et al. Dyamic changes in the plasma biliverdin level during neonatal jaundice phototherapy. Photomed Photobiol. (1991) 13:85–8.
26. Itoh S, Kondo M, Imai T, Kusaka T, Isobe K, Onishi S. Relationships between serum (ZZ)-bilirubin, its subfractions and biliverdin concentrations in infants at 1-month check-ups. Ann Clin Biochem. (2001) 38:323–8. doi: 10.1258/0004563011900821
27. Yamaguchi T, Shioji I, Sugimoto A, Komoda Y, Nakajima H. Chemical structure of a new family of bile pigments from human urine. J Biochem. (1994) 116:298–303. doi: 10.1093/oxfordjournals.jbchem.a124523
28. Lightner DA, Linnane WP, Alfors CE. Bilirubin photooxidation products in the urine of jaundice neonates receiving phototherapy. Pediatr Res. (1984) 18:696–700. doi: 10.1203/00006450-198408000-00003
29. Wurster WL, Pyne-Geithman GL, Peat IR, Clark JF. Bilirubin oxidation products (BOXes): synthesis, stability and chemical characteristics. Acta Neurochir Suppl. (2008) 104:43–50. doi: 10.1007/978-3-211-75718-5_8
30. Ritter M, Neupane S, Seidel RA, Steinbeck C, Pohnert G. In vivo and in vitro identification of Z-BOX C: a new bilirubin oxidation end product. Org Biomol Chem. (2018) 16:3553–5. doi: 10.1039/C8OB00164B
31. Itoh S, Ohtaki Y, Hayashida Y, Okada H, Kusaka T, Sugihara S, et al. Propentdyopent in the urine of infant with prolonged non-obstructive jaundice at one-month check-up, well-baby clinic for one-month old babies. J Jpn Pediatr Soc. (1995) 99:112–6.
32. Ostrow JD. Chapter 27: Photochemical and biochemical basis of the treatment of neonatal jaundice in progress in liver diseases. In: Popper H, Schaffner F, editors. Vol 4. New York: Grune & Stratton (1972). p. 447–62.
33. Land EJ. The triplet excited state of bilirubin. Photochem Photobiol. (1976) 24:475–7. doi: 10.1111/j.1751-1097.1976.tb06857.x
34. Sloper RW, Truscott TG. The quantum yield for bilirubin photoismerization. Photochem Photobiol. (1982) 35:743–5. doi: 10.1111/j.1751-1097.1982.tb02640.x
35. Onishi S, Itoh S, Kawade N, Isobe K, Sugiyama S. The separation of configurational isomers of bilirubin by high pressure liquid chromatography and mechanism of jaundice phototherapy. Biochem Biophys Res Commun. (1979) 90:890–6. doi: 10.1016/0006-291X(79)91911-9
36. Isobe K, Onishi S. Kinetics of the photochemical interconversion among geometric photoisomers of bilirubin. Biochem J. (1981) 193:1029–31. doi: 10.1042/bj1931029
37. Isobe K, Itoh S, Onishi S, Yamakawa T, Ogino T, Yokoyama T. Kinetic study of photochemical and thermal conversion of bilirubin IXα and its photoproducts. Biochem J. (1983) 209:695–700. doi: 10.1042/bj2090695
38. Onishi S, Miura I, Isobe K, Itoh S, Ogino T, Yokoyama T, et al. Structure and thermal interconversion of cyclobilirubin IXα. Biochem J. (1984) 218:667–76. doi: 10.1042/bj2180667
39. Onishi S, Isobe K, Itoh S, Manabe M, Sasaki K, Fukuzaki R, et al. Metabolism of bilirubin and its photoisomers in newborn infants during phototherapy. J Biochem. (1986) 100:789–95. doi: 10.1093/oxfordjournals.jbchem.a121772
40. Zunszain PA, Ghuman J, McDonagh AF, Curry S. Crystallographic analysis of human serum albumin complexed with 4Z,15E-bilirubin IXα. J Mol Biol. (2008) 381:394–406. doi: 10.1016/j.jmb.2008.06.016
41. Itoh S, Yamakawa T, Onishi S, Isobe K, Manabe M, Sasaki K. The effect of bilirubin photoisomers on unbound-bilirubin concentration estimated by the peroxidase method. Biochem J. (1986) 239:417–21. doi: 10.1042/bj2390417
42. McDonagh AF, Vreman HJ, Wong RJ, Stevenson DK. Photoisomers: obfuscating factors in clinical peroxidase measurements of unbound bilirubin? Pediatrics. (2009) 123:67–76. doi: 10.1542/peds.2008-0492
43. Okada H, Kusaka T, Koyano K, Koyano K, Kunikata J, Iwase T, et al. Influence of bilirubin photoisomers on unbound bilirubin measurement in clinical settings. Ann Clin Biochem. (2012) 49:595–9. doi: 10.1258/acb.2012.012024
44. Onishi S, Itoh S, Isobe K, Togari H, Kitoh H, Nishimura Y. Mechanism of development of bronze baby syndrome in neonates treated with phototherapy. Pediatrics. (1982) 69:273–6. doi: 10.1542/peds.69.3.273
45. Itoh S, Okada H, Kuboi T, Kusaka T. Phototherapy for neonatal hyperbilirubinemia. Pediatr Int. (2017) 59:959–66. doi: 10.1111/ped.13332
46. McDonagh AF, Palma LA, Trull FR, Lightner DA. Phototherapy for neonatal jaundice: configurational isomers of bilirubin. J Am Chem Soc. (1982) 104:6865–7. doi: 10.1021/ja00388a103
47. McDonagh AF, Palma LA, Lightner DA. Phototherapy for neonatal jaundice: stereospecific and regioselective photoisomerization of bilirubin bound to human serum albumin and NMR characterization of intra-molecular cyclized photoproducts. J Am Chem Soc. (1982) 104:6867–9. doi: 10.1021/ja00388a104
48. Itoh S, Onishi S. Kinetic study of the photochemical changes of (ZZ)-bilirubin IXα bound human serum albumin: demonstration of (EZ)-bilirubin IXα as an intermediate in photochemical changes from (ZZ)-bilirubin IXα to (EZ)-cyclobilirubin IXα. Biochem J. (1985) 226:251–8. doi: 10.1042/bj2260251
49. Onishi S, Itoh S, Yamakawa T, Isobe K, Manabe M, Toyota S, et al. Comparison of kinetic study of the photochemical changes of (ZZ)-bilirubin IXα bound to human serum albumin with that bound to rat serum albumin. Biochem J. (1985) 230:561–7. doi: 10.1042/bj2300561
50. Onishi S, Itoh S, Isobe K. Wavelength-dependence of the relative rate constants for the main geometric and structural photoisomerization of bilirubin IXα bound to human serum albumin: demonstration of green light at 510 nm as the most effective wavelength in photochemical changes from (ZZ)-bilirubin IXα to (EZ)-cyclobilirubin IXα via (EZ)-bilirubin IXα. Biochem J. (1986) 236:23–9. doi: 10.1042/bj2360023
51. Itoh S, Onishi S, Isobe K, Manabe M, Yamakawa T. Wavelength dependence of the geometric and structural potoisomerization of bilirubin bound to human serum albumin. Biol Neonate. (1987) 51:10–7. doi: 10.1159/000242625
52. Yokoyama T, Ogino T, Onishi S, Isobe K, Itoh S, Yamakawa T. Significance of the endo-vinyl group of bilirubin in photochemical reactions. Biochem J. (1984) 220:377–83. doi: 10.1042/bj2200377
53. McDonagh AF, Assisi F. The ready isomerization of bilirubin IX-α in aqueous solution. Biochem J. (1972) 129:797–800. doi: 10.1042/bj1290797
54. Blanckaert N, Gollan J, Schmid R. Mechanism of bilirubin diglucuronides formation in intact rat: bilirubin diglucuronide formation in vivo. J Clin Invest. (1980) 65:1332–42. doi: 10.1172/JCI109797
55. McDonagh AF. Thermal and photochemical reactions of bilirubin IXα. Ann NY Acad Sci. (1975) 244:533–69. doi: 10.1111/j.1749-6632.1975.tb41554.x
56. Ballowitz L, Bunjamin A, Hanefeld F, Lietz L, Stüttgen G, Wirjadi D. Effects of riboflavin on rats under phototherapy. Pediatr Res. (1979) 13:1307–15. doi: 10.1203/00006450-197912000-00001
57. Knoch E, Hodr R. Metabolism of bilirubin and riboflavin in the course of phototherapy for hyperbilirubinaemia in the newborn. Czech Med. (1989) 12:134–44.2509172
58. Ando M, Nishida T, Kondo M, Ochi M, Onishi K, Isobe K, et al. Pathophysiological significance of neonatal jaundice in defense system against active oxygen in the prenatal period comparison of bilirubin photochemical reactions in presence of flavin mononucleotide by blue-white light with those by green light. Photomed Photobiol. (1989) 11:115–7.
59. Greenberg JW, Malhotra V, Ennever JF. Wavelength dependence of the quantum yield for the structural isomerization of bilirubin. Photochem Photobiol. (1987) 46:453–6. doi: 10.1111/j.1751-1097.1987.tb04794.x
60. Vreman HJ, Kourula S, Jašprová J, Ludvíková L, Klán P, Muchová L, et al. The effect of light wavelength on in vitro bilirubin photodegradation and photoisomer production. Pediatr Res. (2019) 85:865–73. doi: 10.1038/s41390-019-0310-2
61. Kuboi T, Kusaka T, Okada H, Arioka M, Nii K, Takahashi M, et al. Green light-emitting diode phototherapy for neonatal hyperbilirubinemia: randomized controlled trial. Pediatr Int. (2019) 61:465–70. doi: 10.1111/ped.13821
62. Ebbesen F, Rodrigo-Domingo M, Moeller AM, Vreman HJ, Donneborg ML. Effect of blue LED photothrapy at 478 nm versus 459 nm in hyperbilirubinemic neonates: a randomized study. Pediatr Res. (2021) 89:598–603. doi: 10.1038/s41390-020-0911-9
63. Hayaishi O, Nozaki M. Nature and mechanisms of oxygenases. Science. (1969) 164:389–96. doi: 10.1126/science.164.3878.389
64. Tenhunen R, Marver HS, Schimid R. Microsomal heme oxygenase: characterization of the enzyme. J Biol Chem. (1969) 244:6388–94. doi: 10.1016/S0021-9258(18)63477-5
65. Blumenthal SG, Stucker T, Rasmussen RD, Ikeda RM, Ruebner BH, Bergstrom DE, et al. Changes in bilirubins in human prenatal development. Biochem J. (1980) 186:693–700. doi: 10.1042/bj1860693
66. Blanckaert N, Fevery J, Heirwegh KP, Compernolle F. Characterization of the major diazo-positive pigments in bile of homozygous gunn rats. Biochem J. (1977) 164:237–49. doi: 10.1042/bj1640237
67. McDongh AF, Palma LA, Schmid R. Reduction of biliverdin and placental transfer of bilirubin and biliverdin in the pregnant pig. Biochem J. (1981) 194:273–82. doi: 10.1042/bj1940273
68. Kawade N, Onishi S. The prenatal and postnatal development of UDP-glucuronyltransferase activity towards bilirubin and the effect of premature birth on this activity in the human liver. Biochem J. (1981) 196:257–60. doi: 10.1042/bj1960257
70. Lobato G, Soncini CS. Relationship between obstetric history and rh(D) alloimmunization severity. Arch Gynecol Obstet. (2008) 277:245–8. doi: 10.1007/s00404-007-0446-x
71. Zoglio DI, Cardilli E. The Dubin-Johnson syndrome and pregnancy. Ostet Gynecol. (1973) 42:560–3. doi: 10.1097/00006250-197310000-00013
72. Davis DR, Yeary RA, Lee K. Improved embryonic survival in the jaundiced female rat fed activated charcoal. Pediatr Pharmacol. (1983) 3:79–85.
73. Friedlaender P, Osler M. Icterus and pregnancy. Am J Obstet Gynecol. (1967) 97:894–900. doi: 10.1016/0002-9378(67)90513-3
74. Waffarn F, Carlisle S, Pena I, Hodgman JE, Bonham D. Fetal exposure to maternal hyperbilirubinemia; neonatal course and outcome. Am J Dis Child. (1982) 136:416–7. doi: 10.1001/archpedi.1982.03970410034007
75. Maisels MJ, Pathak A, Nelson NM, Nathan DG, Smith CA. Endogenous production of carbon monoxide in normal and erythroblastotic newborn infants. J Clin Invest. (1971) 50:1–8. doi: 10.1172/JCI106463
76. Kuruvilla DJ, Widness JA, Nalbant D, Schmidt RL, Mock DM, Veng-Pedersen P. A method to evaluate fetal erythropoiesis from postnatal survival of fetal RBCs. AAPS J. (2015) 17:1246–54. doi: 10.1208/s12248-015-9784-y
77. Mock DM, Lankford GL, Widness JA, Burmeister LF, Kahn D, Strauss RG. Measurement of red cell survival using biotin-labeled red cells: validation against 51Cr-labeled red cells. Transfusion. (1999) 39:156–62. doi: 10.1046/j.1537-2995.1999.39299154729.x
78. Mock DM, Matthews NI, Zhu S, Strauss RG, Schmidt RL, Nalbant D, et al. Red blood cell (RBC) survival determined in humans using RBCs labeled at multiple biotin densities. Transfusion. (2011) 51:1047–57. doi: 10.1111/j.1537-2995.2010.02926.x
79. Yao AC, Lind J. Placental transfusion. Am J Dis Child. (1974) 127:128–41. doi: 10.1001/archpedi.1974.02110200130021
80. Itoh S, Matunaga T, Hara T, Hayakawa J, Isobe K, Onishi S. Relationship between plasma bilirubin levels in the neonatal period and the time of umbilical cord cramping. Syusankiigaku. (1978) 8:974–8.
81. Cernadas JMC, Carroli G, Pllegrini L, Otaño L, Ferreira M, Ricci C, et al. The effect of timing of cord clamping on neonatal venous hematocrit values and clinical outcome at term: a randomized, controlled trial. Pediatrics. (2006) 117:e779–786. doi: 10.1542/peds.2005-1156
82. Shinohara E, Kataoka Y. Prevalance and risk factors for hyperbilirubinemia among newborns from a row-risk birth setting usin delayed cord clamping in Japan. Jpn J Nur Sci. (2021) 18:e 12372. doi: 10.1111/jjns.12372
83. Qian Y, Lu Q, Shao H, Ying X, Huang W, Hua Y. Timing of umbilical cord clamping and neonatal jaundice in singleton term pregnancy. Early Hum Dev. (2020) 142:104948. doi: 10.1016/j.earlhumdev.2019.104948
84. American College of Obstetricians and Gynecologists’ Committee on Obstetric Practice. Delayed umbilical cord clamping after birth: ACOG committee opinion, number 814. Obstet Gynecol. (2020) 136:e100–6. doi: 10.1097/AOG.0000000000004167
85. Horiguchi T, Bauer C. Ethnic differences in neonatal jaundice: comparison of Japanese and Caucasian newborn infants. Am J Obstet Gynecol. (1975) 121:71–4. doi: 10.1016/0002-9378(75)90978-3
86. Friedman L, Lewis PJ, Clifton P, Bulpitt CJ. Factors influencing the incidence of neonatal jaundice. Brit Med J. (1978) 13:1235–7. doi: 10.1136/bmj.1.6122.1235
87. Setia S, Villaveces A, Dhillon P. Neonatal jaundice in Asian, white, and mixed race infants. Arch Pediatr Adolec Med. (2002) 156:276–9. doi: 10.1001/archpedi.156.3.276
88. Kuboi T, Kusaka T, Kawada K, Koyano K, Nakamura S, Okubo K, et al. Hour-specific nomogram for transcutaneous bilirubin in Japanese neonates. Pediatr Int. (2013) 55:608–11. doi: 10.1111/ped.12149
89. Manning LR, Russell JE, Padovan JC, Chait BT, Popowicz A, Manning RS, et al. Human embryonic, fetal, and adult hemoglobins have different subunit interface strengths. Correlation with lifespan in the red cell. Protein Sci. (2007) 16:1641–58. doi: 10.1110/ps.072891007
90. Bhöler T, Leo A, Standler A, Linderkamp O. Mechanical fragility of erythrocyte membrane in neonates and adults. Pediatr Res. (1992) 32:92–6. doi: 10.1203/00006450-199207000-00018
91. Kondo M, Itoh S, Kusaka T, Imai T, Isobe K, Onishi S. The ability of neonatal and maternal erythrocytes to produce reactive oxygen species in response to oxidative stress. Early Hum Dev. (2002) 66:81–8. doi: 10.1016/S0378-3782(01)00234-1
92. Ciccoli L, Hayek Y, Berti D, Bracci R. Fatty acid pattern of the erythrocyte lipids and plasma vitamin E in the first days of life. Biol Neonate. (1981) 40:187–95. doi: 10.1159/000241489
93. Jain SK. The neonatal erythrocyte and its oxidative susceptibility. Semin Hematol. (1989) 26:286–300.2479105
94. Fischer AF, Nakamura H, Uetani Y, Vreman HJ, Stevenson DK. Comparison of bilirubin production in Japanese and Caucasian infants. J Pediatr Gastroenterol Nutr. (1988) 7:27–9. doi: 10.1097/00005176-198801000-00007
95. Onishi S, Kawade N, Itoh S, Isobe K, Sugiyama S. Postnatal development of uridine diphosphate glucuronyltransferase activity towards bilirubin and 2-aminophenolo in human liver. Biochem J. (1979) 184:705–7. doi: 10.1042/bj1840705
96. Burchell B, Hume R. Molecular genetic basis of Gilbert's syndrome. J Gastroenterol Hepatol. (1999) 13:960–6. doi: 10.1046/j.1440-1746.1999.01984.x
97. Akaba K, Kimura T, Sasaki A, Tanabe S, Ikegami T, Hashimoto M, et al. Neonatal hyperbilirubinemia and mutation of the bilirubin uridine diphosphate-glucuronosyltransferase gene: a common missense mutation among Japanese, Koreans and Chinese. Biochem Mol Biol Int. (1998) 46:21–6. doi: 10.1080/15216549800203512
98. Maruo Y, Nishizawa K, Sato H, Doida Y, Shimada M. Association of neonatal hyperbilirubinemia with bilirubin UDP-glucuronosyltransferase polymorphism. Pediatrics. (1999) 103:1224–7. doi: 10.1542/peds.103.6.1224
99. Maruo Y, Nishizawa K, Sato H, Sawa H, Shimada M. Prolonged unconjugated hyperbilirubinemia associated with breast milk and mutations of the bilirubin uridine diphosphate- glucuronosyltransferase gene. Pediatrics. (2000) 106:E59. doi: 10.1542/peds.106.5.e59
100. Yanagi T, Nakahara S, Maruo Y. Bilirubin uridine diphosphate-glucuronosyltransferase polymorphism as a risk factor for prolonged hyperbilirubinemia in Japanese preterm infants. J Pediatr. (2017) 190:159–62. doi: 10.1016/j.jpeds.2017.07.014
101. Liu W, Chang LW, Xie M, Li WB, Rong ZH, Wu L, et al. Correlation between UGT1A1 polymorphism and neonatal hyperbilirubinemia of neonates in Wuhan. J Huazhong Univ Sci Technolog Med Sci. (2017) 37:740–3. doi: 10.1007/s11596-017-1797-6
102. Yasuda S, Itoh S, Imai T, Isobe K, Onishi S. Cyclobilirubin formation by in vitro photoirradiation with neonatal phototherapy light. Pediatr Int. (2001) 43:270–5. doi: 10.1046/j.1442-200x.2001.01398.x
103. Gourley GR, Arend RA. Beta-glucuronidase and hyperbilirubinaemia in breast-fed and formula-fed babies. Lancet. (1986) 1:644–6. doi: 10.1016/S0140-6736(86)91724-1
104. Newman AJ, Gross S. Hyperbilirubinemia in breast-fed infants. Pediatrics. (1963) 32:955–1001. doi: 10.1542/peds.32.6.995
105. Arias IM, Gartner LM, Seifter S, Furman M. Prolonged neonatal unconjugated hyperbilirubinemia associated with breast feeding and a steroid, pregnane-3(alpha), 20(beta)-diol, in maternal milk that inhibits glucuronide formation in vitro. J Clin Invest. (1964) 43:2037–47. doi: 10.1172/JCI105078
106. Lawrence M, Gartner LM, Lee KS, Mosconi D. Effect of milk feeding on intestinal bilirubin absorption in the rat. J Pediatr. (1983) 103:464–71. doi: 10.1016/S0022-3476(83)80429-6
107. Kumral A, Ozkan H, Duman N, Yesilirmak DC, Islekel H, Ozalp Y. Breast milk jaundice correlates with high levels of epidermal growth factor. Pediatr Res. (2009) 66:218–21. doi: 10.1203/PDR.0b013e3181ac4a30
108. Fujiwara R, Maruo Y, Chen S, Tukey RH. Role of extrahepatic UDP-glucuronosyltransferase 1A1: advances in understanding breast milk-induce neonatal hyperbilirubinemia. Toxicol Appl Pharmacol. (2015) 289:124–32. doi: 10.1016/j.taap.2015.08.018
109. Onishi S, Itoh S, Isobe K, Imai T, Ando M, Ochi M, et al. Pathophysiologic significance of phase I reaction and phase II reaction in generation and elimination of active oxygen in the perinatal period. Photochem Photobiol. (1989) 11:51–69.
110. Doğan M, Peker E, Kirimi E, Sal E, Akbayram S, Erel O, et al. Evaluation of oxidant and antioxidant status in infants with hyperbilirubinemia and kernicterus. Hum Exp Toxicol. (2011) 30:1751–60. doi: 10.1177/0960327111401638
111. Bulut O, Erek A, Duruyen S. Effects of hyperbilirubinemia on markers of genotoxicity and total oxidant and antioxidant status in newborns. Drug Chem Toxicol. (2022) 45:451–5. doi: 10.1080/01480545.2019.1710182
112. Fereshtehnejad SM, Mir KPB, Mir APB, Mohagheghi P. Evaluation of the possible antioxidant role of bilirubin protecting from free radical related illnesses in neonates. Acta Med Iran. (2012) 50:153–63.22418983
113. Faichère JC, Meier-Gibbons FE, Koerner F, Bossi E. Retinopathy of prematurity and bilirubin: no clinical evidence for a beneficial role of bilirubin as a physiological anti-oxidant. Eur J Pediatr. (1994) 153:358–62. doi: 10.1007/BF01956419
114. Itoh S, Isobe K, Onishi S, Imai T, Kondo M, Hashimoto M, et al. The effects of methylxanthine derivative and related substances on the acceleration or inhibition of photooxidation of bilirubin using the photosensitizer of vitamin B2. Photomed Photobiol. (1991) 13:131–3.
115. Ando M, Isobe K, Itoh S, Onishi S. Pathophysiological significance of neonatal jaundice in defense system against active oxygen in the neonatal period: a comparison of bilirubin photochemical reactions of blue-white and green light in the presence of flavin mononucleotide. J Jpn Soc Premature Newborn Med. (1990) 2:96–103.
116. Speck WT, Rosenkranz HS. Phototherapy for neonatal hyperbilirubinemia: a potential environmental health hazard to newborn infants. Environ Mutagen. (1979) 1:321–36. doi: 10.1002/em.2860010404
Keywords: bilirubin photoisomers, breast milk jaundice, human serum albumin, neonatal jaundice, physiological effects, reactive oxygen species, 4Z,15Z-bilirubin IXα, oxidation products
Citation: Itoh S, Okada H, Koyano K, Nakamura S, Konishi Y, Iwase T and Kusaka T (2023) Fetal and neonatal bilirubin metabolism. Front. Pediatr. 10:1002408. doi: 10.3389/fped.2022.1002408
Received: 25 July 2022; Accepted: 30 December 2022;
Published: 7 February 2023.
Edited by:
Claudio Tiribelli, Italian Liver Foundation ONLUS, Italy© 2023 Itoh, Okada, Koyano, Nakamura, Konishi, Iwase and Kusaka. This is an open-access article distributed under the terms of the Creative Commons Attribution License (CC BY). The use, distribution or reproduction in other forums is permitted, provided the original author(s) and the copyright owner(s) are credited and that the original publication in this journal is cited, in accordance with accepted academic practice. No use, distribution or reproduction is permitted which does not comply with these terms.
*Correspondence: Takashi Kusaka a3VzYWthLnRha2FzaGlAa2FnYXdhLXUuYWMuanA=
Specialty Section: This article was submitted to Neonatology, a section of the journal Frontiers in Pediatrics