- Department of Pediatrics, The First Affiliated Hospital, Sun Yat-sen University, Guangzhou, China
Background: Pediatric oncology patients with acute respiratory distress syndrome (ARDS) secondary to pneumonia are at high risk of mortality. Our aim was to describe the epidemiology of ARDS in this clinical population and to identify the association between the oxygenation status at 24 h after diagnosis and the 30-day mortality rates, stratified by the severity of ARDS.
Methods: This was a retrospective cohort study of 82 pediatric oncology patients, with a median age of 4 years, admitted to our pediatric intensive care unit with a diagnosis of ARDS between 2013 and 2021. Demographic and clinical factors were compared between the survivor (n = 52) and non-survivor (n = 30) groups. Univariate and multivariate Cox proportional hazards regression models were used to determine the association between the oxygenation status at 24 h after diagnosis and the 30-day mortality rates.
Results: The mean airway pressure at ARDS diagnosis, PaO2/FiO2 (P/F) ratio, oxygenation index (OI) value, peak inspiratory pressure, and lactate level at 24 h after ARDS diagnosis, as well as complications (i.e., septicemia and more than two extrapulmonary organ failures) and adjunctive continuous renal replacement therapy, were significant mortality risk factors. After adjusting for other covariates, the oxygenation status P/F ratio (Hazard ratio [HR] = 0.98, 95% confidence interval [CI] = 0.96–1.00, P = 0.043) and OI value (HR = 1.12, 95% CI = 1.02–1.23, P = 0.016) at 24 h remained independent mortality risk factors. According to the Kaplan–Meier survival curve, a low P/F ratio (≤ 150) and high OI (>10) were associated with a higher risk of 30-day mortality (50.9 and 52.9%, respectively; both P < 0.05)
Conclusion: The P/F ratio and OI value measured at 24 h after ARDS diagnosis can provide a better stratification of patients according to ARDS disease severity to predict the 30-day mortality risk.
Introduction
Acute respiratory distress syndrome (ARDS) is a type of acute-onset hypoxemic respiratory failure that is characterized by the rapid onset of widespread inflammation in the lungs caused by sepsis, pneumonia, trauma, coronavirus disease 2019, and other conditions (1). The prevalence of ARDS in the pediatric intensive care unit (PICU) has been reported to be 3% and is associated with a high mortality rate, ranging between 11 and 61% (2–4). While the management of pediatric ARDS has greatly improved, ARDS among oncological patients in the PICU remains a high-risk factor for mortality. These critically ill pediatric patients frequently require intubation and invasive mechanical ventilation, which are specific risk factors for in-hospital mortality (mortality rates of ~60%) (5, 6).
For a more precise assessment and treatment, one approach to reduce the heterogeneity is to sub-classify patients into those having pulmonary and extrapulmonary ARDS. Pulmonary ARDS accounts for >50% of all ARDS cases (2), while it accounts for 60–80% of all cancer patients (7–9). Pulmonary ARDS has been associated with significantly higher rates of lung epithelial injury, alveolar collapse, fibrin deposition, alveolar wall edema, and lower lung compliance (10, 11). Though some ARDS may be elements of both pulmonary and extra pulmonary injury, there are some different therapeutic management for the two specific subpopulations of patients (12).
In this study, we aimed to describe the epidemiology of pulmonary ARDS in this clinical population and to identify the association between the oxygenation status at 24 h after ARDS diagnosis and the 30-day mortality, stratified by the severity of ARDS. This information will contribute to the development of targeted treatment strategies to improve outcomes of pulmonary ARDS in pediatric oncology.
Materials and Methods
Statement of Ethics
Our study protocol was approved by Institutional Review Board of the First Affiliated Hospital of Sun Yat-sen University (number 2021/420, July 1, 2021).
Study Design and Cohort
This was a retrospective cohort study conducted in the eight-bed multidisciplinary PICU of the First Affiliated Hospital of Sun Yat-sen University, between January 2013 and January 2021. In total, 118 oncological children were admitted to the ICU, who had acute respiratory failure with pulmonary opacities, as shown by radiographic or chest computed tomography examination. Among them, the mortality rate of 14 patients with extrapulmonary ARDS was 64.3%. Five patients died within 24 h and two received withdrawal treatment within 48 h after admission. To reduce the heterogeneity, patients with extra pulmonary ARDS were excluded. In total, 82 patients with pulmonary ARDS were included (Figure 1). All patients met the 2015 PALICC meeting criteria and the Berlin criteria for ARDS diagnosis (1, 13). All patients received non-invasive ventilation or invasive mechanical ventilation for >24 h. Patients who died in hospital within 24 h after admission were not included.
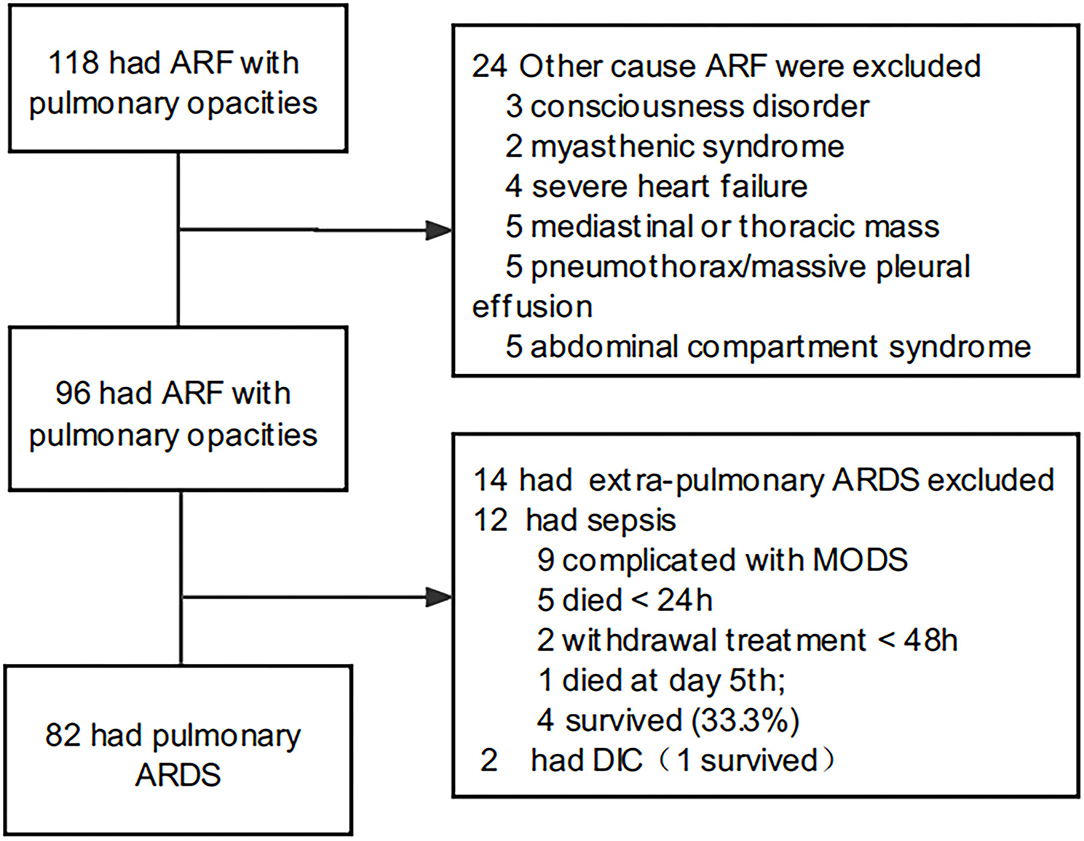
Figure 1. Flow chart concerning ARDS inclusion. ARF, acute respiratory failure; ARDS, acute respiratory distress syndrome.
Data Collection
The following data were extracted from patients' electronic medical records for analysis: demographics, clinical data, laboratory tests results, and ventilator parameters [peak inspiratory pressure (PIP), end-expiratory positive pressure (PEEP), mean airway pressure (MAP) and tidal volume]. Laboratory test results included blood gas analysis, routine blood examination, C-reactive protein (CRP) level, procalcitonin level, activated partial thromboplastin time, total bilirubin level, and lactate dehydrogenase level.
The Pediatric Critical Illness Score (PCIS) and the Pediatric Risk of Mortality (PRISM) III score were calculated at the time of admission to the PICU. Severe leukopenia was defined as an absolute white blood count <1,000 cells/mL. The oxygenation index (OI) and the PaO2 /FiO2 (P/F) ratio were used as markers of the severity of hypoxemic respiratory failure, calculated as follows: OI = (FiO2 × MAP × 100)/PaO2; and P/F = PaO2 /FiO2.
Statistical Analysis
Continuous variables are presented as means ± standard deviations (SDs) or medians and interquartile ranges (IQRs), as appropriate for the data distribution. Categorical data are reported as counts and percentages. Comparisons were made between the survivor and non-survivor groups at 30 days post-admission to the PICU for ARDS.
Baseline characteristics, shown in Table 1, were compared between the two groups using the unpaired two-tailed Student's t-test or the Mann–Whitney U test for continuous variables, the unadjusted chi-squared or Fisher's exact test for categorical variables, and the rank-sum test for ranked data. Univariate and multivariate Cox proportional hazard regression models were used to investigate factors associated with the 30-day survival outcome (survivor or non-survivor). The confounder model was adjusted for the P/F (24 h) ratio or the OI (24 h) index, with the following covariates included: age, sex, MAP (0 h), PIP (24 h), lactate (24 h), septicemia, number of extrapulmonary organ failure (≥2), and CRRT. We selected these confounders based on their associations with the outcome of interest (30-day mortality) or a change in the effect estimate >10% on univariate regression analysis. Then, we examined the association between oxygenation status (OI and P/F ratio) at 24 h and 30-day mortality (continuous analysis) and for the non-survivor group specification (dichotomized analysis).
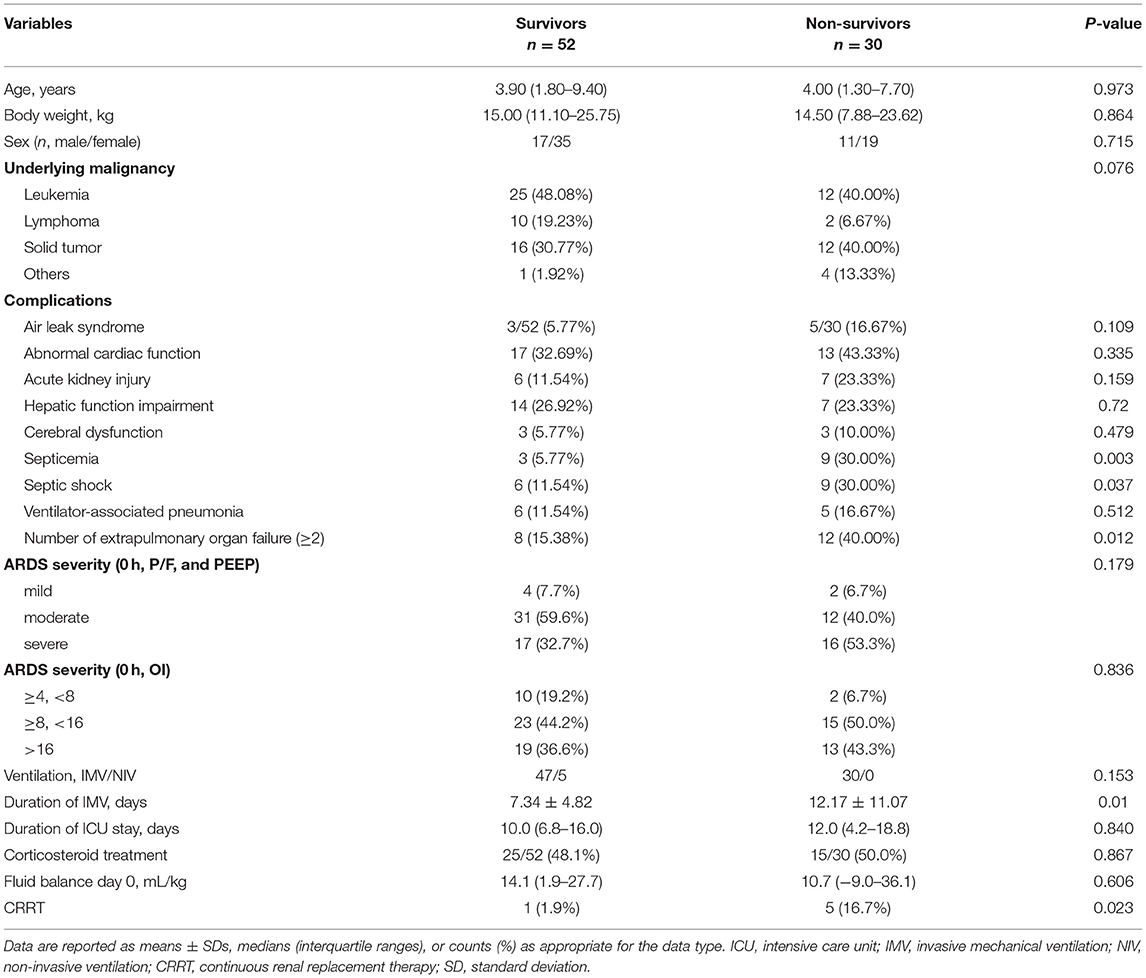
Table 1. Comparison of patients' clinical characteristics between the survivor and non-survivor groups.
All analyses were performed using Empower(R) (Empower Retirement LLC, Greenwood village, CO, USA) and R (R Foundation for Statistical Computing, Vienna, Austria). Statistical significance was defined as a two-sided P-value < 0.05.
Results
Clinical Characteristics of the Study Group
The median age was 4 (IQR, 1.5–9.2) years, with a 1:2 male (n = 28) to female (n = 54) ratio. The most common underlying oncological diagnoses were leukemia (37/82 of patients, 45.1%) and solid tumors (28/82 of patients, 34.1%). The median duration of pre-existing oncological disease was 3 (IQR, 1–8) months. The 30-day non-survival rate was 36.6% (30/82 of patients). Demographic and clinical variables are reported in Table 1. There were no significant differences between the 30-day survivor (n = 52) and non-survivor (n = 30) groups concerning age, sex, pre-existing comorbid condition, corticosteroid treatment, and fluid balance on day 0 (admission to the PICU). Compared to the survivor group, patients in the non-survivor group had a significantly higher incidence rate of complications, including septicemia, septic shock, and need for adjunctive CRRT therapy (all P < 0.05). In this study, six patients received CRRT treatment, four had acute kidney injury, one had cardiac dysfunction, and another one had severe sepsis. In addition, 40% of the patients in the non-survivor group complicated with more than two extrapulmonary organ failures, higher than that in the survivor group (15.38%).
The mortality rate differed based on the criteria used for ARDS diagnosis. However, no significance was found among ARDS severities evaluated at admission between the survivor and non-survivor groups.
Laboratory Results
Differences in laboratory results between the survivor and non-survivor group are reported in Table 2. Although 21 patients in the whole group had severe leukopenia, there were no significant between-group differences with regard to laboratory findings. The association between the critical illness scores (PCIS and PRISM III), the oxygenation status, and ventilator parameters for the survivor and non-survivor group is reported in Table 3. There were no significant differences of the PCIS and PRISM III scores between the two groups. At the time of ARDS diagnosis, the median OI and P/F ratio values were 15.62 ± 6.50 and 124.71 ± 44.69, respectively, with no difference between the survivor and non-survivor groups. However, at 24 h after diagnosis, the OI values were significantly higher, while the P/F ratio was lower in the non-survivor (27.51 ± 16.6 and 92.51 ± 45.75, respectively) than in the survivor (13.17 ± 7.02 and 160.86 ± 61.85, respectively) group (P < 0.001). MAP (0 h), PIP (24 h) and Lac (24 h) were also higher in the non-survivor than in the survivor group (P < 0.05).
Association Between Oxygenation (OI and P/F ratio) at 24 h and 30-Day Mortality
Clinical characteristics and laboratory results were used as independent variables in univariate and multivariate Cox regression models to evaluate the association between the oxygenation status at 24 h and 30-day mortality (Table 4). Both the 24-h P/F ratio and OI were significantly associated with 30-day mortality (Figure 2). After adjusting for other covariates, a low P/F ratio and a high OI at 24 h were associated with a higher 30-day mortality risk (both P < 0.05). We further analyzed the risk for 30-day mortality as a function of dichotomized 24 h P/F ratio and OI values for the whole patients, as follows: P/F >150 and ≤ 150; and OI ≥10 and <10). The 30-day mortality rates for severe ARDS reclassified at 24 h were as follows: 50.9% (27/53) for patients with an OI ≥10 and 52.94% (27/51) for patients with a P/F ratio ≤ 150. The 30-day mortality rates in the non-severe group were 10.3% (OI <10) and 9.7% (P/F >150). There was no difference in the duration of mechanical ventilation and ICU stay between the two groups (Table 5). The Kaplan–Meier survival curve analysis confirmed a higher survival rate among patients with a higher oxygenation status at 24 h: OI <10; P = 0.047; and P/F >150 (P = 0.026; Figure 3).
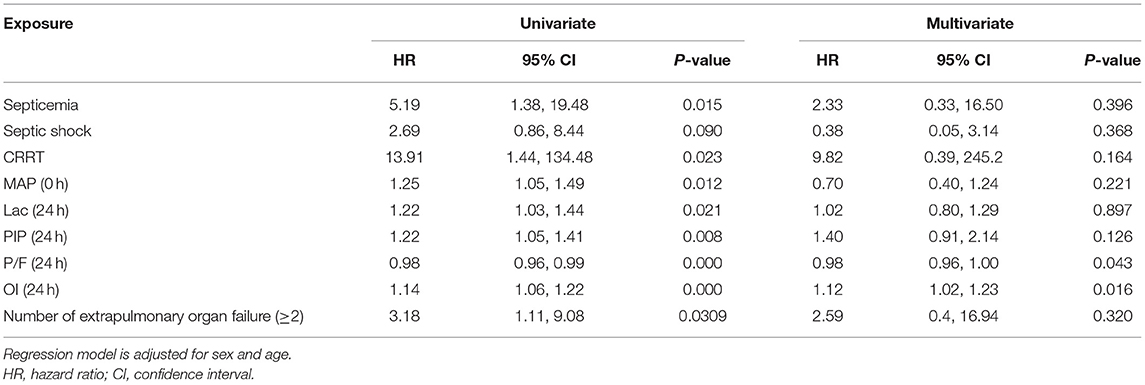
Table 4. Univariate and multivariate Cox regression analyses results of independent variables associated with a 30-day mortality.
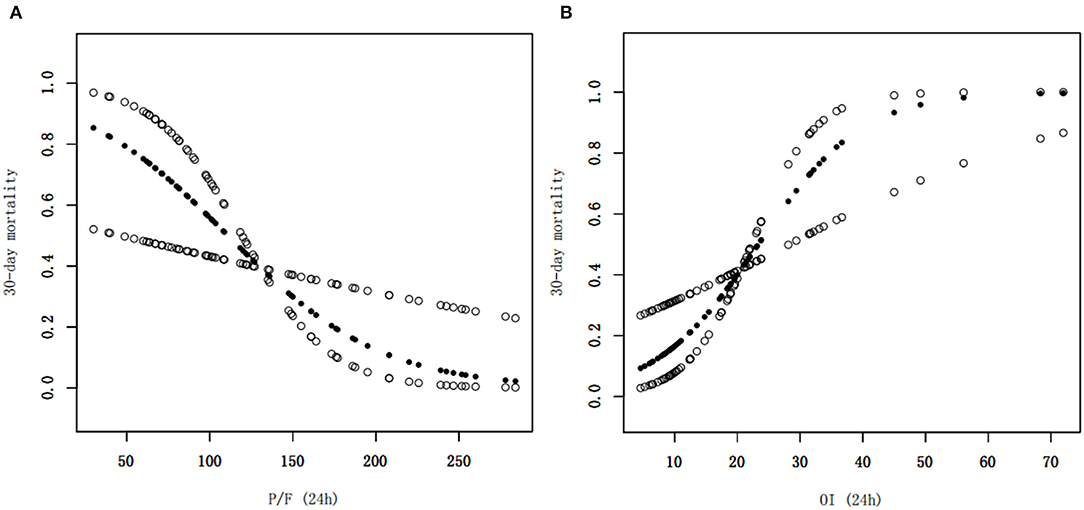
Figure 2. Smoothed regression curves showed that a low P/F ratio and a high OI at 24 h were associated with a higher 30-day mortality risk. The model is adjusted for sex, age, septicemia, MAP (0 h), PIP (24 h), and Lac (24 h), CRRT, and number of extrapulmonary organ failure (≥2). P/F, PaO2/FiO2; OI, oxygenation index, MAP, mean airway pressure; PIP, peak inspiratory pressure, Lac, lactate, CRRT, continuous renal replacement therapy.
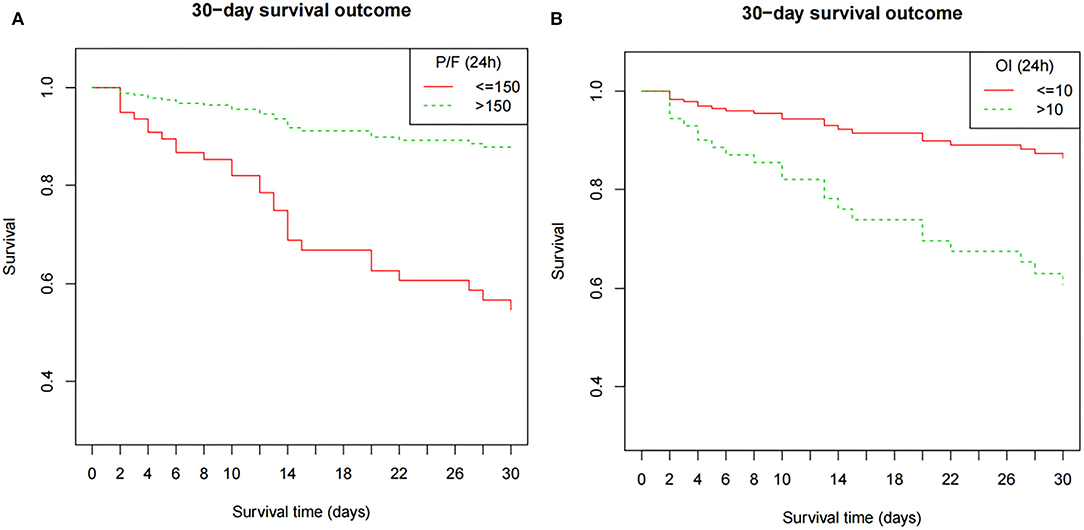
Figure 3. The Kaplan–Meier survival curve as a function of the oxygenation status at 24 h, using dichotomized indices: OI ≥10 and <10; P/F ratio >150 and ≤ 150). The survival results significantly differed between the groups (OI, P = 0.047; P/F ratio, P = 0.026). The model is adjusted for age, septicemia, CRRT, PIP (24 h), and Lac (24 h). P/F, PaO2/FiO2; OI, oxygenation index; MAP, mean airway pressure; PIP, peak inspiratory pressure; Lac, lactate; CRRT, continuous renal replacement therapy.
Discussion
Our study identified a lower oxygenation status at 24 h after ARDS diagnosis as a significant risk factor for 30-day mortality among pediatric oncological patients with pulmonary ARDS. These are important findings considering that acute respiratory failure is a common event in immunosuppressed patients with cancer. Approximately 15% of cancer patients develop acute respiratory failure requiring ICU admission (14). Many studies have demonstrated that oncological diseases are both pre-existing and risk factors for ARDS-related mortality (15, 16). Among adult patients with ARDS, the mortality rate was higher among those with (55.2%) than among those without cancer (24.3%), while it was reported to be 63% in patients with neutropenic cancer at 28 days (9). Chima et al. reported that among pediatric stem cell transplant recipients, only 39% (34/88) of those who required intubation and mechanical ventilation survived in their last PICU admission (17). The total mortality rate in our population was 36.6%. The 30-day mortality rates for severe ARDS reclassified at 24 h were as follows: 50.9% for patients with an OI ≥10 and 52.94% for patients with a P/F ratio ≤ 150, which were slightly lower than those reported in previous studies (7, 8, 18). There are some reasons for it. No patient with graft-vs.-host disease and long-time refractory neutropenia in our study underwent pediatric hematopoietic cell transplantation. Moreover, our cohort comprised only pulmonary cases, associated with less severe comorbidities, such as multiple organ dysfunction syndrome (MODS) and septic shock. However, the incidence of extrapulmonary organ failure (≥2) was higher in non-survivors. Sepsis-related ARDS is commonly associated with higher disease severity and mortality rates compared to non-sepsis-related ARDS. The proportions of sepsis-related ARDS combined with septic shock and MODS were >50 and >80%, respectively (8, 19, 20). In addition, most ARDS reports were focused on adults, especially elderly patients, who had more complications, such as chronic obstructive pulmonary disease, diabetes, chronic cardiac failure, and acute kidney injury (2, 21).
Although all patients in our study had pre-existing comorbid oncological diseases, a uniform treatment strategy was not possible as ARDS is a heterogenous syndrome. The stratification of the severity of ARDS is particularly important for treatment. However, our findings indicate that the oxygenation status (P/F ratio or OI) measured at the time of ARDS diagnosis failed to identify subgroups of patients with a distinct degree of lung injury. It is possible that children with ARDS may not have similar degrees of lung injury or prognosis, although they have a similar oxygenation status at baseline. In addition, some ARDS patients may have improved lung function within the initial 24 h of routine therapy, such as sedation and analgesia, liquid management, antibiotics, and selection of appropriate ventilator parameters. In contrast, oxygenation markers, namely the P/F ratio or OI, measured at 24 h after diagnosis, provides a more precise risk stratification for pediatric oncological patients with ARDS, independent of the patient's septicemia, PIP, and lactate levels. In our study, when ARDS severity was defined by an OI ≥10 or P/F ratio <150, the 30-day mortality rates (50.9 and 52.9%, respectively) were approximately 5-fold higher than those for the non-severe ARDS group (OI <10 or P/F ratio ≤ 150; mortality rates, 10.3 and 9.7%, respectively). More recent studies have reported comparable PICU mortality rates of 55.6 and 58% among children admitted with immunocompromised or oncologic disease requiring mechanical ventilation (18, 22).
Based on our findings, the P/F ratio measured at 24 h after ARDS diagnosis can provide a more precise classification of patients according to ARDS severity. This likely reflects that lung function can improve within the initial 24 h of usual care in some patients with ARDS. Thus, re-stratification of ARDS severity at 24 h after diagnosis would allow identification of patients with non-severe ARDS who would not require more invasive and aggressive therapies. In addition, this re-stratification at 24 h would enable personalized targeted interventions for patients with severe ARDS after 24 h of usual care, including the use of neuromuscular blocking agent (23), corticosteroid therapy (24), prone position ventilation (25), recruitment maneuvers (26), selection of the best ventilatory pattern, and inhaled nitric oxide treatment (27) or extracorporeal membrane oxygenation (28). The proposed re-stratification at 24 h is consistent with autopsy findings reported by Arnaud et al. that the proportion of diffuse alveolar damage depended on the severity of ARDS, being more frequent with moderate and severe ARDS, and that the presence of lung lesions markedly increased at ≥24 h after diagnosis (29).
Although previous studies have demonstrated the inadequacy of initial oxygenation in children and adults with ARDS, relative to oxygenation at 24 h after diagnosis (30–33), our work is the first to focus on a pediatric oncological population with ARDS secondary to severe pneumonia. The association between the oxygenation status at 24 h and mortality was confirmed based on the Berlin and PALICC criteria. However, we did not compare the responsiveness of the OI and P/F ratio for re-stratification of ARDS severity at 24 h after diagnosis. Nevertheless, the distribution of the patients identified relative to the mortality status was similar for the two markers of oxygenation status.
According to lung protection strategies during ventilation, high plateau pressures and tidal volumes should be limited. Panico et al. identified that a higher PIP on day 1 of ARDS was independently associated with mortality in pediatric patients with ARDS (34). Although MAP at ARDS diagnosis and the PIP at 24 h in our study was higher in the non-survivor than in the survivor group, we did not identify a significant correlation between the two factors and the 30-day mortality in the multivariate Cox regression model. This might reflect our use of a relatively balanced PEEP. This result is consistent with those reported by Yehya and Thomas (30) where oxygenation, rather than pressure variables, was the primary metric associated with mortality.
Previous studies have reported higher PRISM III scores and neutropenia as independent predictors of overall mortality among cancer patients with ARDS (18, 20). However, this was not a finding in our study. This may reflect the fact that only patients with ARDS secondary to severe pneumonia were included in our study, rather than other causes, such as sepsis and trauma. Thus, pulmonary ARDS patients had similar PRISM III scores. While our study cohort included 26 patients with severe leukopenia at the time of ARDS diagnosis, leucopenia was not identified as a mortality risk factor in our analysis. It has been suggested that a delay in recovery of neutrophil levels rather than an absolute low level of neutrophils may be associated with poor survival among pediatric oncological patients with acute respiratory failure (35). This variable should be considered in future studies focusing on mortality in this clinical population.
The limitations of our study need to be acknowledged. First, only pediatric oncological patients were included and, therefore, our study cohort was not representative of the entire pediatric population with ARDS. Second, as this was a retrospective study with a limited sample size from a single center, causation cannot be attributed. Therefore, further research is needed.
In summary, pediatric ARDS frequently occurs in oncological children and is associated with a high mortality rate. The oxygenation status at 24 h after diagnosis, classified using the OI and P/F ratio, may provide a better stratification of patients according to ARDS disease severity to predict the 30-day mortality risk. Disease stratification at 24 h could better inform the need for a more aggressive treatment and, in our opinion, should be used for trial enrollment and risk stratification.
Data Availability Statement
The original contributions presented in the study are included in the article/supplementary material, further inquiries can be directed to the corresponding author/s.
Ethics Statement
The studies involving human participants were reviewed and approved by Institutional Review Board of the First Affiliated Hospital of Sun Yat-sen University. Written informed consent to participate in this study was provided by the participants' legal guardian/next of kin. Written informed consent was obtained from the minor(s)' legal guardian/next of kin for the publication of any potentially identifiable images or data included in this article.
Author Contributions
XJ and YL designed the study and reviewed and revised the manuscript. XH and LX carried out the initial analyses and drafted the initial manuscript. YP, HH, CC, and WT coordinated and supervised the data collection. All authors contributed to the article and approved the submitted version.
Conflict of Interest
The authors declare that the research was conducted in the absence of any commercial or financial relationships that could be construed as a potential conflict of interest.
Publisher's Note
All claims expressed in this article are solely those of the authors and do not necessarily represent those of their affiliated organizations, or those of the publisher, the editors and the reviewers. Any product that may be evaluated in this article, or claim that may be made by its manufacturer, is not guaranteed or endorsed by the publisher.
Acknowledgments
The authors thank all participants for their support.
References
1. Ranieri VM, Rubenfeld GD, Thompson BT, Ferguson ND, Caldwell E, Fan E, et al. Acute respiratory distress syndrome: the Berlin definition. JAMA. (2012) 307:2526–33. doi: 10.1001/jama.2012.5669
2. Bellani G, Laffey JG, Pham T, Fan E, Brochard L, Esteban A, et al. Epidemiology, patterns of care, and mortality for patients with acute respiratory distress syndrome in intensive care units in 50 countries. JAMA. (2016) 315: 788-800. doi: 10.1001/jama.2016.0291
3. Wong JJ, Jit M, Sultana R, Mok YH, Yeo JG, Koh J, et al. Mortality in pediatric acute respiratory distress syndrome: a systematic review and meta-analysis. J Intensive Care Med. (2019) 34: 563–71. doi: 10.1177/0885066617705109
4. Khemani RG, Smith L, Lopez-Fernandez YM, Kwok J, Morzov R, Klein MJ, et al. Paediatric acute respiratory distress syndrome incidence and epidemiology (PARDIE): an international, observational study. Lancet Respir Med. (2019)7:115–28. doi: 10.1016/S2213-2600(18)30344-8
5. Wong J, Lee SW, Tan HL, Ma YJ, Sultana R, Mok YH, Lee JH. Lung-Protective mechanical ventilation strategies in pediatric acute respiratory distress syndrome. Pediatr Crit Care Med. (2020) 21:720–8. doi: 10.1097/PCC.0000000000002324
6. Rawal G, Yadav S, Kumar R. Acute respiratory distress syndrome: an update and review. J Transl Int Med. (2018) 6:74–7. doi: 10.1515/jtim-2016-0012
7. Lagier D, Platon L, Chow-Chine L, Sannini A, Bisbal M, Brun JP, et al. Severity of acute respiratory distress syndrome in haematology patients: long-term impact and early predictive factors. Anaesthesia. (2016) 71:1081–90. doi: 10.1111/anae.13542
8. Seong GM, Lee Y, Hong SB, Lim CM, Koh Y, Huh JW. Prognosis of acute respiratory distress syndrome in patients with hematological malignancies. J Intensive Care Med. (2020) 35:364–70. doi: 10.1177/0885066617753566
9. Soubani AO, Shehada E, Chen W, Smith D. The outcome of cancer patients with acute respiratory distress syndrome. J Crit Care. (2014) 29:183. doi: 10.1016/j.jcrc.2013.10.011
10. Albaiceta GM, Taboada F, Parra D, Blanco A, Escudero D, Otero J. Differences in the deflation limb of the pressure-volume curves in acute respiratory distress syndrome from pulmonary and extrapulmonary origin. Intensive Care Med. (2003) 29:1943–9. doi: 10.1007/s00134-003-1965-y
11. Hoelz C, Negri EM, Lichtenfels AJ, Concecao GM, Barbas CS, Saldiva PH, et al. Morphometric differences in pulmonary lesions in primary and secondary ARDS. A preliminary study in autopsies. Pathol Res Pract. (2001) 197:521–30. doi: 10.1078/0344-0338-00122
12. Spragg RG, Lewis JF, Walmrath HD, Johannigman J, Bellingan G, Laterre PF, et al. Effect of recombinant surfactant protein C-based surfactant on the acute respiratory distress syndrome. N Engl J Med. (2004) 351:884–92. doi: 10.1056/NEJMoa033181
13. Pediatric acute respiratory distress syndrome: consensus recommendations from the pediatric acute lung injury consensus conference. Pediatr Crit Care Med. (2015) 16:428–39. doi: 10.1097/PCC.0000000000000350
14. Azoulay E, Schlemmer B. Diagnostic strategy in cancer patients with acute respiratory failure. Intensive Care Med. (2006) 32:808–22. doi: 10.1007/s00134-006-0129-2
15. Zimmerman JJ, Akhtar SR, Caldwell E, Rubenfeld GD. Incidence and outcomes of pediatric acute lung injury. Pediatrics. (2009) 124:87–95. doi: 10.1542/peds.2007-2462
16. Flori H, Dahmer MK, Sapru A, Quasney MW. Comorbidities and assessment of severity of pediatric acute respiratory distress syndrome: proceedings from the pediatric acute lung injury consensus conference. Pediatr Crit Care Med. (2015)16:S41–50. doi: 10.1097/PCC.0000000000000430
17. Chima RS, Daniels RC, Kim MO, Li D, Wheeler DS, Davies SM, et al. Improved outcomes for stem cell transplant recipients requiring pediatric intensive care. Pediatr Crit Care Med. (2012) 13:e336–42. doi: 10.1097/PCC.0b013e318253c945
18. Zhang Q, Hu WT, Yin F, Qian H, Wang Y, Li BR, et al. The clinical characteristics of ARDS in children with hematological neoplasms. Front Pediatr. (2021) 9:696594. doi: 10.3389/fped.2021.696594
19. Mokart D, van Craenenbroeck T, Lambert J, Textoris J, Brun JP, Sannini A, et al. Prognosis of acute respiratory distress syndrome in neutropenic cancer patients. Eur Respir J. (2012) 40:169–76. doi: 10.1183/09031936.00150611
20. Prasertsan P, Anuntaseree W, Ruangnapa K, Saelim K, Geater A. Severity and mortality predictors of pediatric acute respiratory distress syndrome according to the pediatric acute lung injury consensus conference definition. Pediatr Crit Care Med. (2019) 20: e464-e472. doi: 10.1097/PCC.0000000000002055
21. Mauri T, Foti G, Fornari C, Grasselli G, Pinciroli R, Lovisari F, et al. Sigh in patients with acute hypoxemic respiratory failure and ARDS: the protection pilot randomized clinical trial. Chest. (2021) 159:1426–36. doi: 10.1016/j.chest.2020.10.079
22. Galassi MS, Arduini RG, Araujo OR, Sousa R, Petrilli AS, Silva D. Alveolar recruitment maneuvers for children with cancer and acute respiratory distress syndrome: a feasibility study. Rev Paul Pediatr. (2021) 39:e2019275. doi: 10.1590/1984-0462/2021/39/2019275
23. Alhazzani W, Belley-Cote E, Moller MH, Angus DC, Papazian L, Arabi YM, et al. Neuromuscular blockade in patients with ARDS: a rapid practice guideline. Intensive Care Med. (2020) 46:1977–86. doi: 10.1007/s00134-020-06227-8
24. Annane D, Pastores SM, Rochwerg B, Arlt W, Balk RA, Beishuizen A, et al. Guidelines for the diagnosis and management of critical illness-related corticosteroid insufficiency (CIRCI) in critically ill patients (Part I): society of critical care medicine (SCCM) and European society of intensive care medicine (ESICM) 2017. Intensive Care Med. (2017) 43:1751–63. doi: 10.1007/s00134-017-4919-5
25. Lupton-Smith A, Argent A, Rimensberger P, Frerichs I, Morrow B. Prone positioning improves ventilation homogeneity in children with acute respiratory distress syndrome. Pediatr Crit Care Med. (2017) 18:e229–34. doi: 10.1097/PCC.0000000000001145
26. Kung SC, Hung YL, Chen WL, Wang CM, Chang HC, Liu WL. Effects of stepwise lung recruitment maneuvers in patients with early acute respiratory distress syndrome: a prospective, randomized, controlled trial. J Clin Med. (2019) 8:20231. doi: 10.3390/jcm8020231
27. Yu B, Ichinose F, Bloch DB, Zapol WM. Inhaled nitric oxide. Br J Pharmacol. (2019) 176:246–55. doi: 10.1111/bph.14512
28. Lin JC. Extracorporeal membrane oxygenation for severe pediatric respiratory failure. Respir Care. (2017) 62:732–50. doi: 10.4187/respcare.05338
29. Thille AW, Esteban A, Fernandez-Segoviano P, Rodriguez JM, Aramburu JA, Penuelas O, et al. Comparison of the Berlin definition for acute respiratory distress syndrome with autopsy. Am J Respir Crit Care Med. (2013) 187:761–7. doi: 10.1164/rccm.201211-1981OC
30. Yehya N, Thomas NJ. Disassociating lung mechanics and oxygenation in pediatric acute respiratory distress syndrome. Crit Care Med. (2017) 45:1232–9. doi: 10.1097/CCM.0000000000002406
31. Yehya N, Servaes S, Thomas NJ. Characterizing degree of lung injury in pediatric acute respiratory distress syndrome. Crit Care Med. (2015) 43:937–46. doi: 10.1097/CCM.0000000000000867
32. Parvathaneni K, Belani S, Leung D, Newth CJ, Khemani RG. Evaluating the performance of the pediatric acute lung injury consensus conference definition of acute respiratory distress syndrome. Pediatr Crit Care Med. (2017) 18:17–25. doi: 10.1097/PCC.0000000000000945
33. Villar J, Blanco J, Del CR, Andaluz-Ojeda D, Diaz-Dominguez FJ, Muriel A, et al. Assessment of PaO(2)/FiO(2) for stratification of patients with moderate and severe acute respiratory distress syndrome. BMJ Open. (2015) 5:e006812. doi: 10.1136/bmjopen-2014-006812
34. Panico FF, Troster EJ, Oliveira CS, Faria A, Lucena M, Joao PR, et al. Risk factors for mortality and outcomes in pediatric acute lung injury/acute respiratory distress syndrome. Pediatr Crit Care Med. (2015) 16:e194–200. doi: 10.1097/PCC.0000000000000490
Keywords: ARDS, oxygenation status, oxygenation index, P/F ratio, risk factors
Citation: Huang X, Xu L, Pei Y, Huang H, Chen C, Tang W, Jiang X and Li Y (2022) The Association Between Oxygenation Status at 24 h After Diagnosis of Pulmonary Acute Respiratory Distress Syndrome and the 30-Day Mortality among Pediatric Oncological Patients. Front. Pediatr. 10:805264. doi: 10.3389/fped.2022.805264
Received: 30 October 2021; Accepted: 21 April 2022;
Published: 11 May 2022.
Edited by:
Demet Demirkol, Istanbul University, TurkeyReviewed by:
Guoping Lu, Fudan University, ChinaPhuc Huu Phan, Vietnam National Hospital of Pediatrics, Vietnam
Copyright © 2022 Huang, Xu, Pei, Huang, Chen, Tang, Jiang and Li. This is an open-access article distributed under the terms of the Creative Commons Attribution License (CC BY). The use, distribution or reproduction in other forums is permitted, provided the original author(s) and the copyright owner(s) are credited and that the original publication in this journal is cited, in accordance with accepted academic practice. No use, distribution or reproduction is permitted which does not comply with these terms.
*Correspondence: Xiaoyun Jiang, anhpYW95QG1haWwuc3lzdS5lZHUuY24=; Yijuan Li, bGl5aWp1YW5AbWFpbC5zeXN1LmVkdS5jbg==
†These authors have contributed equally to this work