- 1Children’s Medical Center, Taichung Veterans General Hospital, Taichung City, Taiwan
- 2Department of Medical Research, Taichung Veterans General Hospital, Taichung City, Taiwan
- 3School of Medicine, National Yang Ming Chiao Tung University, Taipei City, Taiwan
- 4Department of Food and Nutrition, Providence University, Taichung City, Taiwan
- 5School of Medicine, Chung Shan Medical University, Taichung City, Taiwan
- 6Department of Post-baccalaureate Medicine, College of Medicine, National Chung Hsing University, Taichung City, Taiwan
Introduction: Immune thrombocytopenia (ITP) is one of the most common hematologic disorders in children. However, its etiology is still unclear. Epidemiological studies have shown that air pollution is a plausible risk factor in stimulation of oxidative stress, induction of inflammation, and onset of autoimmune diseases. The objective of this article is to examine the effects of prenatal exposure to air pollution on the occurrence of immune thrombocytopenia (ITP) in children.
Materials and Methods: This is a nationwide, population-based, matched case-control study. Using data from Taiwan’s Maternal and Child Health Database (MCHD), we identified 427 children with ITP less than 6 years of age and age-matched controls without ITP between 2004 and 2016. Levels of prenatal exposure to air pollutants were obtained from 71 Environmental Protection Administration monitoring stations across Taiwan according to the maternal residence during pregnancy. Patients who had outpatient visits or admission with diagnosis of ITP and subsequently received first-line treatment of intravenous immunoglobulin or oral glucocorticoids were defined as incidence cases.
Results: Prenatal exposure to particulate matter <10 μm (PM10) in diameter and the pollutant standard index (PSI) increased the risk of childhood ITP. Conversely, carbon monoxide (CO) exposure during pregnancy was negatively associated with the development of ITP.
Conclusion: Certain prenatal air pollutant exposure may increase the incidence of ITP in children.
Introduction
Immune thrombocytopenia (ITP) is one of the most common hematologic disorders in children, which is characterized by isolated thrombocytopenia (platelet count <100,000/uL with normal hemoglobin level and white blood cell count) and presents with an increased bleeding risk. Children with ITP can present at any age, but it occurs mainly in young children; 50 percent of the patients were between one and 4 years of age and approximately 80 percent were younger than 8 years of age (1–3). The pathogenesis of ITP remains incompletely understood. It might be triggered by a viral infection or other immunologic triggers (4). Aberrant immune response to some environmental triggers in individuals with genetic susceptibility may also contribute to the pathogenesis of ITP (5).
Air pollution is reported to be associated with stimulation of oxidative stress, induction of inflammation and onset of autoimmune diseases (6, 7). Prenatal air pollution exposure, as an intrauterine toxin and a strong oxidant, is associated with poor pregnancy outcomes and long-term health effects in the offsprings (8). Epidemiological studies have also revealed prenatal exposure to air pollution as a risk factor for small for gestational age, preterm birth, impaired newborn lung, impaired immune function, brain developmental disorders, and cognitive disorders after birth (9–12). Children’s acquired immune disorders, such as allergic diseases and Kawasaki disease, have also been reported to be associated with prenatal air pollution exposure (13–16). However, data regarding prenatal exposure to air pollution and its possible association with ITP are lacking. This nationwide longitudinal study assessed the correlation between prenatal and early-life air pollution exposure to the development of ITP.
Materials and Methods
Study Design and Data Source
This is a nationwide, population-based, matched case-control study. The data source was Taiwan’s Maternal and Child Health Database (MCHD), which is governed by the Health and Welfare Data Science Center (HWDC) of the Ministry of Health and Welfare (MOHW). The MCHD consists of Taiwan’s birth registry, birth notifications, mortality registrations, and the National Health Insurance Research Database (NHIRD) (17). The National Health Insurance (NHI) program was launched in Taiwan in March 1995, and includes comprehensive medical claims data from 99% of Taiwanese population of nearly 24 million (18, 19). The National Health Insurance Research Database (NHIRD) comprises all-inclusive claims data on patient’s registration, residence, demographic characteristics, examinations, diagnoses, procedures, medical expenditure, surgeries, medication prescriptions, inpatient services, and outpatient services (20). By integrating these databases, we obtained a detailed linked profile of all births to mothers from the MCHD, and we extracted both inpatient and outpatient medical claims data of children and mothers from the NHIRD. The main data sources for analysis were the inpatient expenditures by admission (DD) and the ambulatory care expenditures by visit (CD) files. Diagnoses in the NHIRD are coded using the International Classification of Diseases, Ninth Revision, Clinical Modification (ICD-9-CM) format. To protect patients’ identity and validate the reliability of the databases, investigators are demanded to perform onsite analysis at HWDC via remote connection to MOHW servers (21). This study protocol was approved by the institutional review board of Taichung Veterans General Hospital (CE17178A-4). The review board waived the requirement for written informed consent as all patient data were anonymized prior to analysis.
Case Identification
The cohort included births from 2004 to 2010. Births with unclear information on residence, multiple delivery, brothers or sisters (only the first child during the study period in a family was included), or congenital anomaly (ICD-9 code 740 to 758) were excluded from the analysis. Children under 6 years old with a diagnosis of ITP (ICD-9 code 287.3 and ICD-10 code D69.3) who were treated with either intravenous immunoglobulin (IVIG, ATC code: J06BA02) or oral glucocorticoids, including dexamethasone (ATC code: H02AB02) or prednisolone (ATC code: H02AB06) between January 2004 to December 2016 were identified as cases (22). The control groups were matched to the ITP group by age and index month, and were randomly chosen at a control-to-case ratio of 4:1. The cohort was followed up until the end of 2016 to ensure that every child in this cohort was followed up for at least 6 years.
Assessment of Exposure to Air Pollutants
Concentrations of each air pollutant, including Sulfur dioxide (SO2), Ozone (O3), Carbon monoxide (CO), Carbon dioxide (CO2), particulate matter <10 μm in diameter (PM10), particulate matter <2.5 μm in diameter (PM2.5), Nitric Oxide (NO), Nitrogen dioxide (NO2), and Nitrogen Oxides (NOx), have been measured hourly by 76 Environmental Protection Administration monitoring stations across Taiwan since 1999 (23). Among them, 5 national park and background air quality monitoring stations were excluded from the analysis. We estimated the air pollutant levels at each residential location utilizing a spatio-temporal model established via a deep-learning approach (24). The Pollutant Standards Index (PSI), a type of air quality index, was determined by the highest value for the five pollutants (i.e., SO2, PM10, NO2, CO, and O3) after transforming their concentrations into a scale from 0 through 500 (25). By using the analysis of Cressman, we computed the PSI and the average concentrations of these air pollutants in 368 townships across Taiwan (26). Finally, we apportioned the prenatal air pollutant exposures in each township to the child according to their mother’s residential postal code during pregnancy.
Statistics
Data were expressed as either frequency and percentage or mean and standard deviation. Continuous variables were compared using the t-test, and categorical variables were compared using the Pearson’s chi-square test. We selected covariates based on data availability, expert knowledge, and the literature. The association between prenatal exposure to air pollution and risk of ITP was examined using a multiple logistic regression analysis after adjusting for age, gender, maternal age, mode of delivery, birth weight, maternal illness, and allergic disease. We also calculated the odds of ITP per interquartile range (IQR) change in PSI level to determine the dose-dependent effect. All data were analyzed using the SAS statistical package (version 9.4; SAS Institute, Cary, NC, United States). A p < 0.05 was considered statistically significant.
Results
Baseline Characteristics of Immune Thrombocytopenia and Age-Matched Controls
Of the 917359 children born in Taiwan from 2004 through 2010, 427 children under the age of 6 years old with ITP were identified as the ITP group. A total of 1,708 children (1:4) without ITP, matched by age and index date, were randomly selected as the control group (Figure 1). Patients in the ITP group and the those in non-ITP group had similar distributions of age, birth weight, maternal age, mode of delivery, gestational age, maternal comorbidity, and allergic disease. ITP occurred mostly at the age of 0–2 years, with a significant male predominance (60.9%) (Table 1).
Prenatal Exposure to Air Pollutants
When comparing air pollutant exposure between ITP and non-ITP groups, levels of prenatal exposure to PSI, and PM10 were significantly higher in patients with ITP than in the matched controls. On the other hand, exposure to SO2, CO2, O3, PM2.5, NOx, NO, and NO2 did not show any significant differences. Interestingly, exposure to CO was found to have an inverse association with the risk of ITP development (Table 2).
Multiple Logistic Regression Analysis of Factors Associated With Immune Thrombocytopenia
A multiple logistic regression model was applied to analyze the associations between exposure to each air pollutant (i.e., SO2, CO, CO2, O3, PM2.5, PM10, NO, NO2, and NOx) or PSI and ITP. After adjustment for potential confounders (age, gender, maternal age, mode of delivery, preterm, maternal comorbidity, and allergic disease), prenatal air pollutant exposure calculated by PSI was demonstrated to be associated with a significantly higher risk for developing ITP in early childhood. On the other hand, prenatal CO exposure showed a protective effect (Table 3).
Dose-Dependent Effect of Air Pollution on Risk of Immune Thrombocytopenia
Quartiles of the cumulative amounts of air pollutants were then calculated to analyze the dose- dependent effect of prenatal exposure on ITP. The odds ratios of ITP increased with increasing in cumulative amounts of pollutants. Linear trends could be observed for PSI and PM10 (Figure 2).
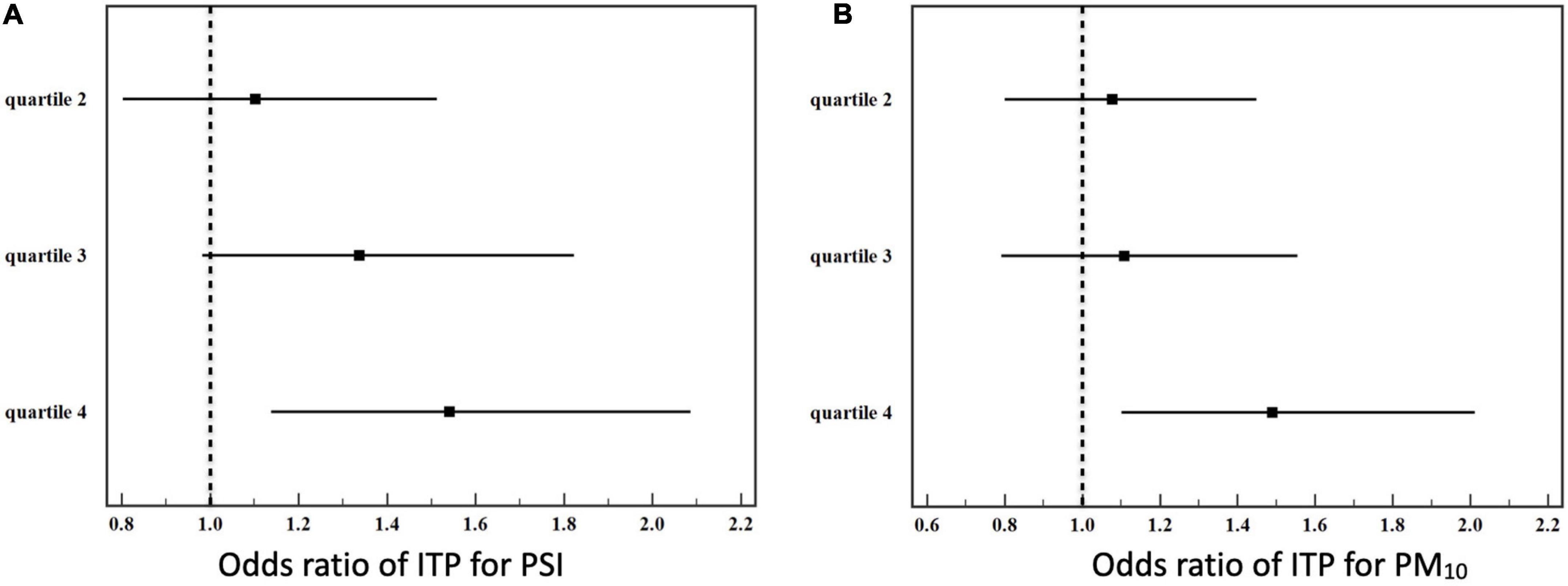
Figure 2. Quartiles of prenatal cumulative air pollutant exposure to the risk of ITP. (A) Pollutant standard index (PSI), (B) PM10.
Discussion
This study investigated the association between prenatal exposure to air pollution and the development of pediatric ITP. We observed prenatal exposure to certain types of air pollutants increased the risk of IPP in early childhood. The study is the first investigation assessing the effect of prenatal exposure to air pollutants on childhood ITP. Furthermore, this study is a nationwide, population-based cohort study.
Immune thrombocytopenia, an autoimmune disease, is characterized by bleeding episodes, purpura, and isolated thrombocytopenia resulted from antiplatelet autoantibodies (3). The predominant pathophysiological theory for ITP is an antibody-mediated destruction against targeted platelets leading to a markedly reduced platelet lifespan and defective megakaryopoiesis (3). Among ITP patients, abundant surface antigens on platelet membrane, such as glycoprotein (GP) Ib/IX, GP IIb/IIIa, GP Ia/IIa, and GP VI can be binded by autoantibodies (27). Both genetic and acquired factors contribute to the pathogenesis of ITP. However it hasn’t been completely understood. Among the environmental factors, the proinflammatory effects of air pollutants have been reported to be associated with the generation of autoimmunity and inflammation (28). As a strong oxidant and an intrauterine toxin, prenatal exposure to particulate matter was associated with long-term complications in the offspring, including impaired neonatal lung function, immune function disorders, and abnormal organogenesis (8). Ambient air pollution has been suspected to trigger autoimmune disease (29). Epidemiological studies have provided authentic evidence of the relationship between air pollution and subsequent occurrence of autoimmune diseases in human, such as diabetes mellitus type 1, juvenile idiopathic arthritis, systemic sclerosis, systemic lupus erythematosus (SLE), autoimmune myositis, and rheumatic arthritis (28, 30–33). Prenatal air pollutants exposure has also been reported to be associated with the development of Kawasaki disease, childhood asthma, and atopic dermatitis (13, 34). Those evidences support our hypothesis that prenatal exposure to air pollutants can prompt postnatal immune dysregulation, which eventually results in increased risk of pediatric ITP.
Laboratory studies also provide evidence to support that prenatal particulate matter exposure to be associated with intrauterine inflammation by revealing higher maternal C-reactive protein levels among exposure mothers (35, 36). In addition, animal models also reveal the link between antenatal inflammation and an alteration in subsequent postnatal immune response (37, 38). Moreover, prenatal exposure to air pollution has been reported to elevate fetal C-reactive protein levels and to influence lymphocyte immunophenotypes and interleukin-1b production (35, 39, 40). Furthermore, epigenetic studies have also shown that air pollution can alter prenatal and postnatal organ development (41, 42). Epigenetic contribution to autoimmune disease has also been reported (43).
Interestingly, a negative association of CO exposure during pregnancy with ITP in children was observed. CO is commonly identified as a poisonous gas because of its better affinity to hemoglobin than oxygen and formation of carboxyhemoglobin, leading to the tissue hypoxia due to impaired oxygen-carrying capacity in circulation (44). Nevertheless, many studies have revealed its cytoprotective properties and the anti-inflammatory effect of CO through regulating the balance between anti-inflammatory Th2 cells and pro-inflammatory Th1/Th17 (44). Given that CO serves as a potential therapeutic bioactive molecule, the therapeutic potential has been reported in experimental models, including ischemia-reperfusion injury, (45) hypoxia, (46) and autoimmune diseases (47). Indeed, these studies have recognized CO as a potential pharmaceutical agent for autoimmune diseases for its anti-apoptotic and anti-inflammatory effects.
There are some limitations in this study. First, it is possible that some pediatric patients with ITP did not seek medical advice as no symptoms or only mild symptoms were experienced, and cases of ITP might have been underestimated in the NHIRD. However, it is reasonable to assume that the majority of patients who did not seek medical help had less severe disease activity. Indeed, children with moderate to severe disease burden are of greater concern. Second, we apportioned township-level air pollution exposure to each case and did not calculate or measure individual exposure, including height of residence or proximity to major roads, which could have resulted in misclassification.
In summary, our results demonstrated that prenatal exposure to air pollution may be associated with the development of ITP in preschool-aged children. More research is needed to elucidate the causal relationships between maternal exposure to air pollutants and development of ITP in early childhood.
Data Availability Statement
The data analyzed in this study is subject to the following licenses/restrictions: To protect patients’ identity and validate the reliability of the databases, investigators are required to perform onsite analysis at HWDC via remote connection to MOHW servers. Requests to access these datasets should be directed to C-HL, ZXBpZEBtczM5LmhpbmV0Lm5ldC4=
Ethics Statement
The studies involving human participants were reviewed and approved by Institutional review board of Taichung Veterans General Hospital. Written informed consent from the participants’ legal guardian/next of kin was not required to participate in this study in accordance with the national legislation and the institutional requirements.
Author Contributions
H-CY wrote the manuscript. C-HL performed data anslysis. M-CL designed the research and review the final version of the manuscript. Y-CH helped in data analysis. Y-HL helped in the study design. All authors contributed to the article and approved the submitted version.
Funding
This study was supported by Taichung Veterans General Hospital Research Fund (Registration numbers TCVGH-NHRI11001, TCVGH-1107303C, and TCVGH-1107309D).
Conflict of Interest
The authors declare that the research was conducted in the absence of any commercial or financial relationships that could be construed as a potential conflict of interest.
Publisher’s Note
All claims expressed in this article are solely those of the authors and do not necessarily represent those of their affiliated organizations, or those of the publisher, the editors and the reviewers. Any product that may be evaluated in this article, or claim that may be made by its manufacturer, is not guaranteed or endorsed by the publisher.
References
1. Terrell DR, Beebe LA, Vesely SK, Neas BR, Segal JB, George JN. The incidence of immune thrombocytopenic purpura in children and adults: a critical review of published reports. Am J Hematol. (2010) 85:174–80. doi: 10.1002/ajh.21616
2. Zeller B, Rajantie J, Hedlund-Treutiger I, Tedgard U, Wesenberg F, Jonsson OG, et al. Childhood idiopathic thrombocytopenic purpura in the Nordic countries: epidemiology and predictors of chronic disease. Acta Paediatr. (2005) 94:178–84. doi: 10.1111/j.1651-2227.2005.tb01887.x
3. D’Orazio JA, Neely J, Farhoudi N. ITP in children: pathophysiology and current treatment approaches. J Pediatr Hematol Oncol. (2013) 35:1–13. doi: 10.1097/MPH.0b013e318271f457
4. Provan D, Arnold DM, Bussel JB, Chong BH, Cooper N, Gernsheimer T, et al. Updated international consensus report on the investigation and management of primary immune thrombocytopenia. Blood Adv. (2019) 3:3780–817. doi: 10.1182/bloodadvances.2019000812
5. Swinkels M, Rijkers M, Voorberg J, Vidarsson G, Leebeek FWG, Jansen AJG. Emerging concepts in immune thrombocytopenia. Front Immunol. (2018) 9:880. doi: 10.3389/fimmu.2018.00880
6. Sun G, Hazlewood G, Bernatsky S, Kaplan GG, Eksteen B, Barnabe C. Association between air pollution and the development of rheumatic disease: a systematic review. Int J Rheumatol. (2016) 2016:5356307. doi: 10.1155/2016/5356307
7. Wu W, Jin Y, Carlsten C. Inflammatory health effects of indoor and outdoor particulate matter. J Allergy Clin Immunol. (2018) 141:833–44. doi: 10.1016/j.jaci.2017.12.981
8. Chen H, Oliver BG, Pant A, Olivera A, Poronnik P, Pollock CA, et al. Particulate matter, an intrauterine toxin affecting foetal development and beyond. Antioxidants (Basel). (2021) 10:732. doi: 10.3390/antiox10050732
9. Wang B, Chen H, Chan YL, Wang G, Oliver BG. Why do intrauterine exposure to air pollution and cigarette smoke increase the risk of asthma? Front Cell Dev Biol. (2020) 8:38. doi: 10.3389/fcell.2020.00038
10. Li Z, Tang Y, Song X, Lazar L, Li Z, Zhao J. Impact of ambient PM2.5 on adverse birth outcome and potential molecular mechanism. Ecotoxicol Environ Saf. (2019) 169:248–54. doi: 10.1016/j.ecoenv.2018.10.109
11. Chen L, Bennett E, Wheeler AJ, Lyons AB, Woods GM, Johnston F, et al. Maternal exposure to particulate matter alters early post-natal lung function and immune cell development. Environ Res. (2018) 164:625–35. doi: 10.1016/j.envres.2018.03.029
12. Soto SF, Melo JO, Marchesi GD, Lopes KL, Veras MM, Oliveira IB, et al. Exposure to fine particulate matter in the air alters placental structure and the renin-angiotensin system. PLoS One. (2017) 12:e0183314. doi: 10.1371/journal.pone.0183314
13. Yorifuji T, Tsukahara H, Kashima S, Doi H. Intrauterine and early postnatal exposure to particulate air pollution and Kawasaki disease: a nationwide longitudinal survey in Japan. J Pediatr. (2018) 193:e2. doi: 10.1016/j.jpeds.2017.10.012
14. Hsu HH, Chiu YH, Coull BA, Kloog I, Schwartz J, Lee A, et al. Prenatal particulate air pollution and asthma onset in Urban children. Identifying sensitive windows and sex differences. Am J Respir Crit Care Med. (2015) 192:1052–9. doi: 10.1164/rccm.201504-0658OC
15. Chu HW, Lin CH, Lin MC, Hsu YC. Increased risk of Kawasaki disease in infants born of mothers with immune disorders. Front Pediatr. (2021) 9:659598. doi: 10.3389/fped.2021.659598
16. Chang CL, Lin MC, Lin CH, Ko TM. Maternal and perinatal factors associated with Kawasaki disease among offspring in Taiwan. JAMA Netw Open. (2021) 4:e213233. doi: 10.1001/jamanetworkopen.2021.3233
17. Wu TP, Liang FW, Huang YL, Chen LH, Lu TH. Maternal mortality in Taiwan: a nationwide data linkage study. PLoS One. (2015) 10:e0132547. doi: 10.1371/journal.pone.0132547
18. Ho Chan WS. Taiwan’s healthcare report 2010. EPMA J. (2010) 1:563–85. doi: 10.1007/s13167-010-0056-8
19. Lin MC, Lai MS. Pediatricians’ role in caring for preschool children in Taiwan under the national health insurance program. J Formos Med Assoc. (2009) 108:849–55. doi: 10.1016/s0929-6646(09)60416-2
20. Chen HH, Yong YM, Lin CH, Chen YH, Chen DY, Ying JC, et al. Air pollutants and development of interstitial lung disease in patients with connective tissue disease: a population-based case-control study in Taiwan. BMJ Open. (2020) 10:e041405. doi: 10.1136/bmjopen-2020-041405
21. Hsieh CY, Su CC, Shao SC, Sung SF, Lin SJ, Kao Yang YH, et al. Taiwan’s national health insurance research database: past and future. Clin Epidemiol. (2019) 11:349–58. doi: 10.2147/CLEP.S196293
22. Neunert C, Lim W, Crowther M, Cohen A, Solberg L Jr, Crowther MA, et al. The American society of hematology 2011 evidence-based practice guideline for immune thrombocytopenia. Blood. (2011) 117:4190–207. doi: 10.1182/blood-2010-08-302984
23. Environmental Protection Administration. Taiwan Air Quality Monitoring Net Work. (2021). Available online at: https://airtw.epa.gov.tw/ENG/EnvMonitoring/Central/Background_Intro.aspx (accessed July 14, 2021).
24. Qi Y, Li Q, Karimian H, Liu D. A hybrid model for spatiotemporal forecasting of PM2.5 based on graph convolutional neural network and long short-term memory. Sci Total Environ. (2019) 664:1–10. doi: 10.1016/j.scitotenv.2019.01.333
25. Mandal TK, Gorai AK. Air quality indices: a literature review. J Environ Sci Eng. (2014) 56:357–62.
26. Cressman GP. An operational objective analysis system. Mon Weather Rev. (1959) 87:367–74. doi: 10.1175/1520-049319590872.0.Co;2
27. Zufferey A, Kapur R, Semple JW. Pathogenesis and therapeutic mechanisms in immune thrombocytopenia (ITP). J Clin Med. (2017) 6:16. doi: 10.3390/jcm6020016
28. Tang KT, Tsuang BJ, Ku KC, Chen YH, Lin CH, Chen DY. Relationship between exposure to air pollutants and development of systemic autoimmune rheumatic diseases: a nationwide population-based case-control study. Ann Rheum Dis. (2019) 78:1288–91. doi: 10.1136/annrheumdis-2019-215230
29. Oikonen M, Laaksonen M, Laippala P, Oksaranta O, Lilius EM, Lindgren S, et al. Ambient air quality and occurrence of multiple sclerosis relapse. Neuroepidemiology. (2003) 22:95–9. doi: 10.1159/000067108
30. Hathout EH, Beeson WL, Ischander M, Rao R, Mace JW. Air pollution and type 1 diabetes in children. Pediatr Diabetes. (2006) 7:81–7. doi: 10.1111/j.1399-543X.2006.00150.x
31. Zeft AS, Prahalad S, Schneider R, Dedeoglu F, Weiss PF, Grom AA, et al. Systemic onset juvenile idiopathic arthritis and exposure to fine particulate air pollution. Clin Exp Rheumatol. (2016) 34:946–52.
32. Heydarpour P, Amini H, Khoshkish S, Seidkhani H, Sahraian MA, Yunesian M. Potential impact of air pollution on multiple sclerosis in Tehran, Iran. Neuroepidemiology. (2014) 43:233–8. doi: 10.1159/000368553
33. Orione MA, Silva CA, Sallum AM, Campos LM, Omori CH, Braga AL, et al. Risk factors for juvenile dermatomyositis: exposure to tobacco and air pollutants during pregnancy. Arthritis Care Res (Hoboken). (2014) 66:1571–5. doi: 10.1002/acr.22358
34. Lavigne E, Belair MA, Rodriguez Duque D, Do MT, Stieb DM, Hystad P, et al. Effect modification of perinatal exposure to air pollution and childhood asthma incidence. Eur Respir J. (2018) 51:1701884. doi: 10.1183/13993003.01884-2017
35. van den Hooven EH, de Kluizenaar Y, Pierik FH, Hofman A, van Ratingen SW, Zandveld PY, et al. Chronic air pollution exposure during pregnancy and maternal and fetal C-reactive protein levels: the generation R Study. Environ Health Perspect. (2012) 120:746–51. doi: 10.1289/ehp.1104345
36. Nachman RM, Mao G, Zhang X, Hong X, Chen Z, Soria CS, et al. Intrauterine inflammation and maternal exposure to ambient PM2.5 during preconception and specific periods of pregnancy: the Boston birth Cohort. Environ Health Perspect. (2016) 124:1608–15. doi: 10.1289/EHP243
37. Blumer N, Herz U, Wegmann M, Renz H. Prenatal lipopolysaccharide-exposure prevents allergic sensitization and airway inflammation, but not airway responsiveness in a murine model of experimental asthma. Clin Exp Allergy. (2005) 35:397–402. doi: 10.1111/j.1365-2222.2005.02184.x
38. Kunzmann S, Glogger K, Been JV, Kallapur SG, Nitsos I, Moss TJ, et al. Thymic changes after chorioamnionitis induced by intraamniotic lipopolysaccharide in fetal sheep. Am J Obstet Gynecol. (2010) 202: 476 e1–9. doi: 10.1016/j.ajog.2010.02.035
39. Latzin P, Frey U, Armann J, Kieninger E, Fuchs O, Roosli M, et al. Exposure to moderate air pollution during late pregnancy and cord blood cytokine secretion in healthy neonates. PLoS One. (2011) 6:e23130. doi: 10.1371/journal.pone.0023130
40. Hertz-Picciotto I, Herr CE, Yap PS, Dostal M, Shumway RH, Ashwood P, et al. Air pollution and lymphocyte phenotype proportions in cord blood. Environ Health Perspect. (2005) 113:1391–8. doi: 10.1289/ehp.7610
41. Ji H, Khurana Hershey GK. Genetic and epigenetic influence on the response to environmental particulate matter. J Allergy Clin Immunol. (2012) 129:33–41. doi: 10.1016/j.jaci.2011.11.008
42. Lockett GA, Huoman J, Holloway JW. Does allergy begin in utero? Pediatr Allergy Immunol. (2015) 26:394–402. doi: 10.1111/pai.12408
43. Surace AEA, Hedrich CM. The role of epigenetics in autoimmune/inflammatory disease. Front Immunol. (2019) 10:1525. doi: 10.3389/fimmu.2019.01525
44. Naito Y, Takagi T, Uchiyama K, Katada K, Yoshikawa T. Multiple targets of carbon monoxide gas in the intestinal inflammation. Arch Biochem Biophys. (2016) 595:147–52. doi: 10.1016/j.abb.2015.06.020
45. Zhang X, Shan P, Otterbein LE, Alam J, Flavell RA, Davis RJ, et al. Carbon monoxide inhibition of apoptosis during ischemia-reperfusion lung injury is dependent on the p38 mitogen-activated protein kinase pathway and involves caspase 3. J Biol Chem. (2003) 278:1248–58. doi: 10.1074/jbc.M208419200
46. Otterbein LE, Mantell LL, Choi AM. Carbon monoxide provides protection against hyperoxic lung injury. Am J Physiol. (1999) 276:L688–94. doi: 10.1152/ajplung.1999.276.4.L688
Keywords: immune thrombocytopenia (ITP), air pollution, prenatal exposure, children, pollutant standard index (PSI), PM10, PM10 (particulate matter)
Citation: Yen H-C, Lin C-H, Lin M-C, Hsu Y-C and Lin Y-H (2022) Prenatal Exposure to Air Pollution and Immune Thrombocytopenia: A Nationwide Population-Based Cohort Study. Front. Pediatr. 10:837101. doi: 10.3389/fped.2022.837101
Received: 16 December 2021; Accepted: 11 February 2022;
Published: 18 March 2022.
Edited by:
Marzia Duse, Sapienza University of Rome, ItalyReviewed by:
Tong Wang, Chinese Academy of Sciences (CAS), ChinaDrucilla Jane Roberts, Massachusetts General Hospital and Harvard Medical School, United States
Copyright © 2022 Yen, Lin, Lin, Hsu and Lin. This is an open-access article distributed under the terms of the Creative Commons Attribution License (CC BY). The use, distribution or reproduction in other forums is permitted, provided the original author(s) and the copyright owner(s) are credited and that the original publication in this journal is cited, in accordance with accepted academic practice. No use, distribution or reproduction is permitted which does not comply with these terms.
*Correspondence: Ming-Chih Lin, bWluZ2NsaW5AZ21haWwuY29t