- 1Department of Pediatrics, David Geffen School of Medicine, University of California, Los Angeles, Los Angeles, CA, United States
- 2Lundquist Institute for Biomedical Innovation at Harbor-UCLA Medical Center, Torrance, CA, United States
- 3Department of Medicine, David Geffen School of Medicine, University of California, Los Angeles, Los Angeles, CA, United States
After shifting away from invasive mechanical ventilation and intratracheal instillation of surfactant toward non-invasive ventilation with nasal CPAP and less invasive surfactant administration in order to prevent bronchopulmonary dysplasia in preterm infants with respiratory distress syndrome, fully non-invasive surfactant nebulization is the next Holy Grail in neonatology. Here we review the characteristics of animal-derived (clinical) and new advanced synthetic lung surfactants and improvements in nebulization technology required to secure optimal lung deposition and effectivity of non-invasive lung surfactant administration. Studies in surfactant-deficient animals and preterm infants have demonstrated the safety and potential of non-invasive surfactant administration, but also provide new directions for the development of synthetic lung surfactant destined for aerosol delivery, implementation of breath-actuated nebulization and optimization of nasal CPAP, nebulizer circuit and nasal interface. Surfactant nebulization may offer a truly non-invasive option for surfactant delivery to preterm infants in the near future.
Introduction
Early respiratory failure in very preterm infants (respiratory distress syndrome, RDS) is caused by lung immaturity and not yet started production of lung surfactant (1). Mammalian lung surfactant, a mixture of phospholipids and four surfactant proteins, is produced by alveolar type 2 cells and consists of the essential phospholipids dipalmitoyl phosphatidylcholine (DPPC) and phosphatidylglycerol and the surfactant proteins SP-A, SP-B, SP-C, and SP-D. DPPC contributes to the formation of a rigid interfacial film that reduces surface tension in the alveoli to low values during dynamic compression, whereas fluid phospholipids and neutral lipids significantly improve film spreading. SP-A and SP-D play important roles in native immunity, whereas SP-B and SP-C enhance the absorption of phospholipids into the air-water interface and optimize surface tension reduction. SP-B is a crucial protein component of lung surfactant and its deficiency due to genetic causes is lethal in both animals and humans (2). SP-C deficiency can cause childhood interstitial lung disease with variable disease severity in infancy (3).
After studies in preterm animals in the 1980s demonstrated a rapid improvement of oxygenation and lung compliance after intratracheal bolus instillation of an animal-derived lung surfactant, its general clinical use, in combination with technological advances in infant ventilators and perinatal care, has dramatically improved survival of very preterm infants (4). Limited availability, high production costs, and scarcity of animal sources have led to the design and development of synthetic lung surfactant formulations, consisting of SP-B and/or SP-C peptide mimics in a phospholipid mixture (5). Similarly, respiratory care of very preterm infants with RDS has been shifting away from intubation and mechanical ventilation to nasal continuous positive airway pressure (nCPAP) (6, 7) to avoid invasive mechanical ventilation lung injury and induction of bronchopulmonary dysplasia, a major pulmonary complication among extreme preterm infants that is often accompanied by neurodevelopmental disabilities. Although surfactant can be instilled intratracheally during spontaneous breathing on nCPAP by inserting a thin catheter in the trachea and thus does not require intubation, this so-called less invasive surfactant administration (LISA) requires advanced technical skills to be able to pass a catheter through the vocal cords (8). Aerosol delivery of surfactant would therefore complement non-invasive ventilatory management of preterm infants with RDS. Technical advances in non-invasive aerosol delivery of surfactant are an interplay of nebulizer technology, appropriate dry powder or liquid animal-derived or synthetic lung surfactant, an optimum delivery route, and lung dose.
Whereas surfactant replacement therapy has become standard of care for surfactant deficiency in preterm infants, it can also enhance the treatment of surfactant dysfunction disorders in infants and children with acute respiratory distress syndrome (ARDS). Meconium aspiration syndrome is an example of surfactant dysfunction in (near) term infants (9), but ARDS can also occur in infants and children who have experienced trauma, pneumonia, aspiration, or immune compromise (10). Here, we review current developments in the surfactant field that are important for treatment of RDS in preterm infants.
Surfactant
Currently available surfactant can be divided into clinical and synthetic lung surfactant preparations. Clinical surfactants are derived from bovine (Survanta®, Infasurf®, Alveofact®) or porcine (Curosurf®) lungs and consist of phospholipids and small quantities (~1 weight%) of the hydrophobic proteins SP-B and SP-C. SP-A and SP-D are hydrophilic proteins and are lost during extraction of the lavage material or minced animal lung tissue. Clinical surfactants were preceded by the first generation of synthetic lung surfactants, i.e., ALEC® (11), a mixture of DPPC and egg phosphatidylglycerol (PG), and Exosurf® (12), a mixture of DPPC with hexadecanol and tyloxapol. These pure phospholipid-based preparations lacking SP-B and SP-C were quickly overshadowed by the introduction of clinical surfactants with a rapid and more consistent improvement in lung compliance after intratracheal administration. The second generation of synthetic lung surfactants contained SP-B and/or SP-C peptide mimics, such as the SP-B analog KL4 [Surfaxin® (13) and Aerosurf® (14)] and recombinant hSP-C [Venticute® (15)], but their efficacy was no match with the animal-derived surfactants and demonstrated the complex 3-D structure of native SP-B and the propensity of SP-C for amyloid formation of its transmembrane region. Advances in the design, development, and production of SP-B and SP-C peptide mimics and phospholipid mixtures subsequently led to the creation of new surfactant formulations with surface activity equivalent to the clinical surfactants.
Human SP-B is a hydrophobic homodimer of two 79 amino-acid polypeptide chains that are linked by disulfide bonds. Each monomer has a molecular weight of 8.7 kDa and consists of 5 α-helices stabilized by three intramolecular disulfide bonds and the two monomers are connected with one interchain disulfide. The N- and C-terminal peptides of SP-B enhance adsorption and surface spreading of phospholipids in a similar way as native SP-B (16) and these characteristics were used to design and develop the SP-B peptide mimics Mini-B, Super Mini-B and B-YL (5). Mini-B is a 34 amino acid peptide that incorporates the N-terminal (residues 8–25) and C-terminal (residues 63–78) α-helices of native SP-B, joined with a customized turn and cross-linked with two adjoining disulfide bonds to form an α-helix hairpin (17). Super Mini-B and B-YL contain the hydrophobic N-terminal insertion sequence of SP-B (residues 1–7) and are 41 amino-acid peptides (18, 19). B-YL has no disulfide bonds after replacing the cysteine residues of Super Mini-B with tyrosine and is less sensitive to oxidation by replacing the methionine residues with leucine. These SP-B peptide mimics are highly stable in phospholipid mixtures and exert excellent surface activity in vitro and in animal models of surfactant deficiency (20, 21).
Human SP-C is an extremely hydrophobic 35 amino-acid protein with a molecular weight of 4.2 kDa. It consists of an α-helix (residues 9–34) and two palmitoyl chains bound to cysteines in positions 4 and 5 via labile thioester bonds. Several large multicenter studies using recombinant SP-C (rhSP-C) without palmitoyl chains (Venticute) demonstrated that palmitoylation of SP-C is essential for optimal surface activity. Johansson et al. (22) solved this problem by replacing the palmitoylated cysteines with serine and preserving the transmembrane helical domain by substituting leucines for valines (rSP-C33Leu). SP-C33Leu has shown to have good in vitro and in vivo surface activity, especially in combination with a SP-B peptide mimic (23). Stabilization of the α-helix of SP-C with intrapeptide salt-bridges (“ion locks”) (24) and use of helical poly-N-substituted glycines (peptoids) (25) have also created stable SP-C mimics with elevated in vitro and in vivo surface activity.
Availability of these highly functional SP-B and SP-C mimics has led to the development of third generation synthetic lung surfactants when mixed in surfactant phospholipids. CHF5633 (Chiesi Farmaceutici, Parma, Italy) is a liquid combination of 0.2% Mini-B and 1.5% SP-C33 in a 1:1 DPPC:POPG mixture (23) and has recently shown clinical efficacy and safety in preterm infants with neonatal RDS (26–28) if administered intratracheally. Minisurf® is a liquid synthetic surfactant based on 3% Super Mini-B in a 5:3:2 DPPC:POPC:POPG (mole:mole) mixture (Molecular Express Inc., Rancho Dominguez, CA 90220) and is in a preclinical stage. Both CHF5633 and Minisurf have demonstrated in vitro and in vivo surface activity similar to animal-derived clinical surfactants. Aerosurf (Surfaxin for inhalation) is a combination of KL4 surfactant and aerosolization technologies from Windtree Therapeutics (Warrington, PA 18976). The lyophilized KL4 surfactant needs to be reconstituted with sterile water prior to non-invasive aerosol delivery with the Aerosurf Delivery System (ADS), which uses novel heated capillary-based aerosol generator (CAG) technology (29). Aerosurf is currently being tested in clinical studies.
The challenge of pulmonary drug delivery is to achieve a high lung deposition of inhaled aerosols. The fate of inhaled particles is determined by their physicochemical properties, deposition site in the airways and biological defenses, such as mucociliary transport and the presence of resident airway macrophages. Solid state drugs have improved chemical stability and this is true for lung surfactant too. Whereas most clinical and synthetic lung surfactant formulations are liquids and consist of SP-B and/or SP-C (peptide mimics) in a phospholipid mixture, dry powder surfactant undergoes spray-drying steps aimed at controlling particle size, density, shape, charge, solid state, and hygroscopicity in order to manipulate agglomeration and powder blending (30). Formulation strategies may include the addition of hygroscopic excipients, such as sugars (e.g., lactose, trehalose, and mannitol) and sodium chloride, and/or dispersion enhancers, such as l-leucine, to alter particle-particle interaction forces and improve the deagglomeration of the fine particles (31, 32). These newer micron-sized dry powder synthetic lung surfactant formulations, such as B-YL:Trehalose surfactant (21, 31), are highly surface active, possess the required aerosolization characteristics, and are biophysically stable. In combination with an appropriate nebulizer, both liquid and dry powder clinical and synthetic surfactant formulations have great potential to resolve surfactant deficiency in vivo.
Nebulization
Aerosol delivery of surfactant has a 30-year history (33), but early studies in preterm infants with clinical surfactant in the 1990s with Alveofact (34), Exosurf (35), and Curosurf (36) did not meet expectations. The gradual shift to non-invasive ventilation of very preterm infants (37) and LISA via a thin intratracheal catheter (8) sparked enthusiasm for the pursuit of fully non-invasive surfactant treatment on two fronts, nebulization devices and appropriate (synthetic) lung surfactant formulations.
Very preterm infants are obligate nose breathers and have a low functional residual capacity, a high and variable respiratory rate, low tidal volumes and small airways (38). In healthy infants, nasal airway resistance accounts for nearly half of the respiratory resistance and turbulent flow in the upper airways is a major causative factor for drug loss through impaction (39). These characteristics pose a major challenge to aerosol delivery of surfactant because they are associated with a low lung deposition of inhaled drugs. However, lung deposition is also affected by the type of non-invasive ventilation (NIV) used, the choice of aerosol generator and its configuration within the ventilation circuit, the choice of surfactant composition and its aerosol particle size distribution, and, last but not least, the interface between the delivery system and the preterm infant. Attention to all these factors is necessary to optimize aerosol delivery of lung surfactant and its clinical efficacy (38).
Non-invasive Ventilation
Current standards of respiratory care for very preterm infants with RDS aim at reducing bronchopulmonary dysplasia through early use of nCPAP and, if necessary, less invasive surfactant administration (40). Other modes of NIV, i.e., nasal intermittent positive pressure ventilation (NIPPV), biphasic positive airway pressure (BiPAP), and high-flow nasal cannula (HFNC) are less often used than nCPAP. CPAP failure in very preterm infants is associated with a higher risk of poor outcome and is an indication for intubation and invasive mechanical ventilation (41). Widespread use of nCPAP has led to the development of a multitude of shapes and sizes of face masks and nasal prongs for preterm and term infants. Differences in their geometrics can influence airflow characteristics, especially airway resistance, and ultimately affect the lung surfactant dosage necessary to elucidate a clinical effect (42). Air leakage continues to require outmost attention, but is not only limited to face masks and nasal prongs, but may also concern air leakage through the mouth.
Nebulizers
Nebulizers are one of the oldest clinically used aerosol generating systems and form a mist of micronized fine particles of a solid or liquid active pharmaceutical ingredients (API) that can be inhaled into the lungs (43). Particle size is expressed as mass median aerodynamic diameter (MMAD) and lung surfactant particles should be within a 1 to 5 μm range (44).
Pressurized metered-dose inhalers (pMDIs) and jet, ultrasonic, and vibrating mesh nebulizers are the most commonly used aerosol delivery devices in infants (45). A pMDI produces in a short time period an aerosol cloud that moves at high speed and requires active inhalation. pMDIs are therefore more often used in children with respiratory conditions such as asthma and cystic fibrosis and who can coordinate actuation and inhalation. In vitro and in vivo studies have shown that vibrating-membrane nebulizers (VMNs) outperform jet and ultrasonic nebulizers in aerosol delivery of liquid surfactant formulations to ventilated newborn infants. Tiemersma et al. (45) compared two prototypes of a VMN, a jet nebulizer and a pressurized metered-dose inhaler (pMDI) with a holding chamber in an upper airway (nose-throat) model of a 32-week preterm infant (1,750 g). Lung dose was 18.0–20.6, 1.5, and 6.8%, respectively. This has led to a shift away from jet nebulizers to VMNs in animal and clinical studies (Tables 1, 2). Synchronization of aerosol delivery and spontaneous respiration can further enhance lung dose as recently shown in ventilated, surfactant-deficient juvenile rabbits and in a porcine ARDS model using liquid bovine surfactant and a novel breath-actuated small particle VMN with photo-defined aperture plate (PDAP) technology (Aerogen Pharma, San Mateo, CA) placed between the Y- connector of the ventilator circuit and the endotracheal tube (63, 64). Synchronization of surfactant delivery was managed by a flow sensor in the inspiratory line of the ventilator and minimized surfactant loss during expiration. Estimation of the lung dose in vitro suggested an efficiency of ~65%. Developments in this field are going fast and Wiegandt et al. (65) recently produced a nasal prong with an integrated miniaturized aerosol valve that allows breath-triggered drug release directly inside the nasal prong with a short response time.
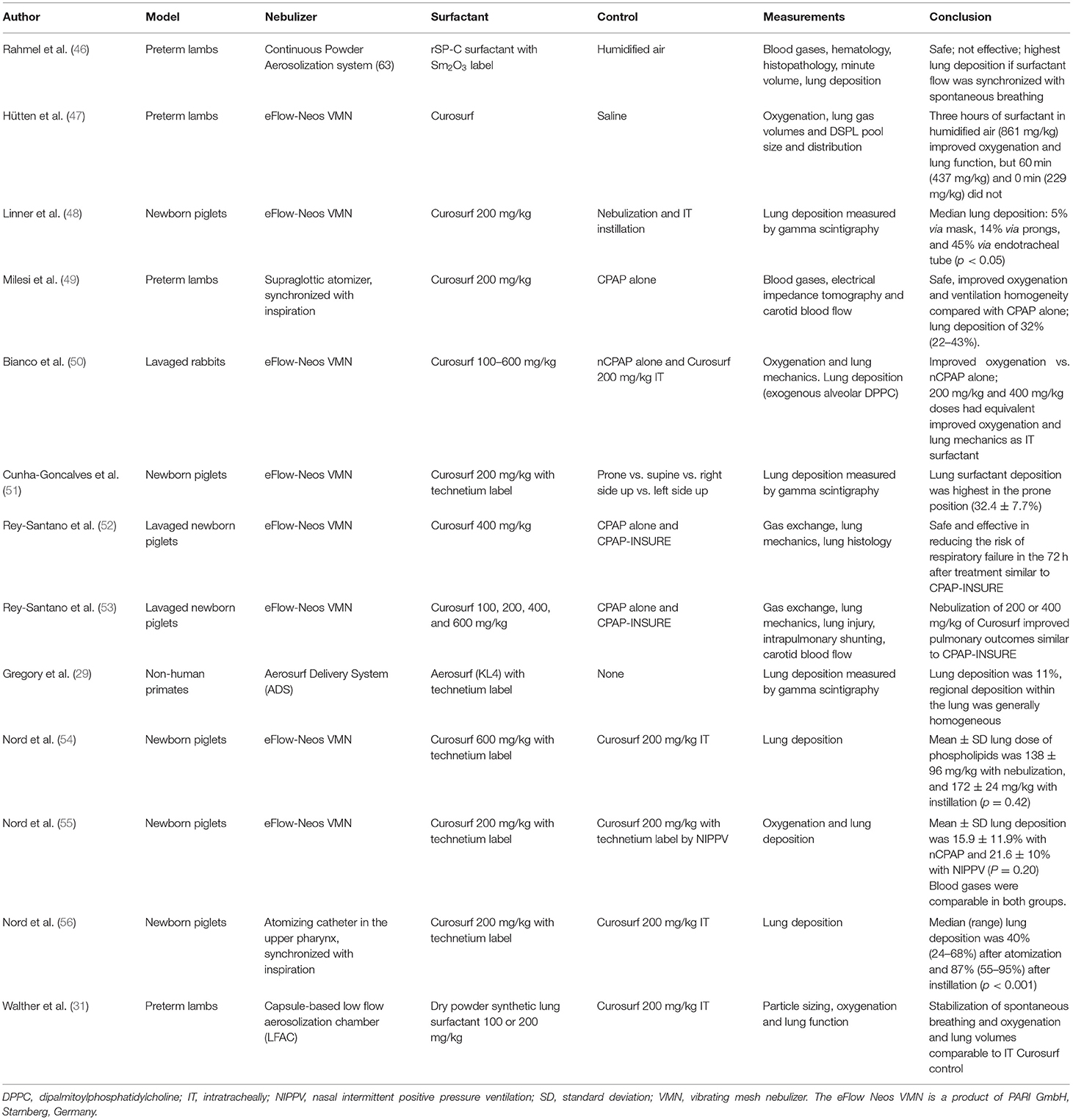
Table 1. Summary of aerosolized surfactants and nebulizers utilized in studies in animals supported with nasal CPAP (nCPAP) ventilation.
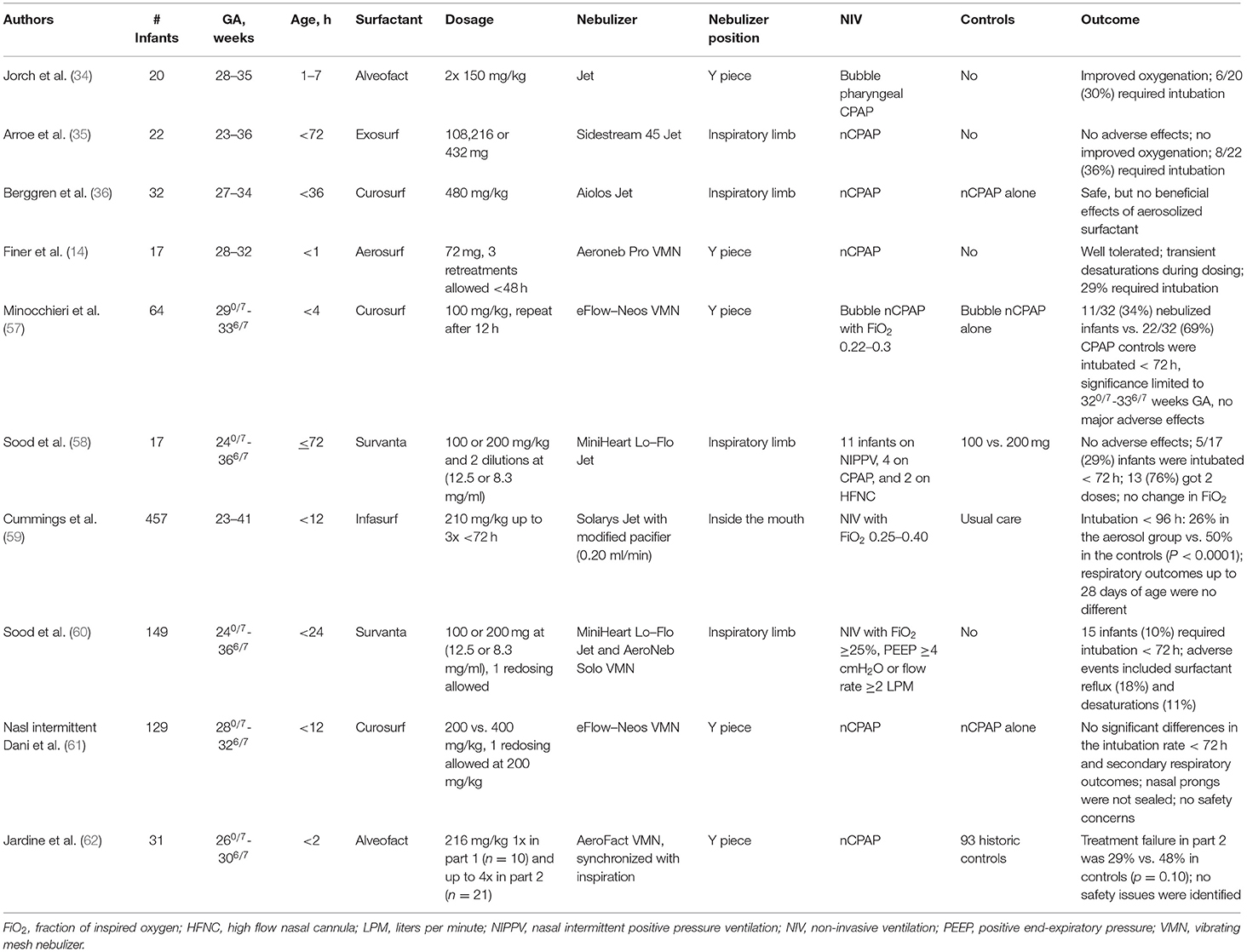
Table 2. Summary of aerosolized surfactants and nebulizers in clinical studies of preterm infants supported with nasal CPAP (nCPAP).
Aerosol delivery of dry powder surfactant can be achieved by generating an aerosol using the inspiratory air flow from the ventilator or by separately producing an aerosol and inserting it into the inspiratory air flow (66). Lung dose from dry powder inhalers is affected by humidity when ventilatory circuits are driven with warm and humified gas and this is why dry powder surfactant is formulated with hygroscopic excipients and/or a dispersion enhancer. We recently reported the feasibility of nasal delivery of dry powder synthetic lung surfactant with B-YL peptide as SP-B mimic and trehalose as excipient to preterm lambs with RDS who were supported with bubble nCPAP. A cylindrical low-flow aerosol chamber (LFAC) device (31) was placed below the Y of the nCPAP circuit and accommodated a perforated capsule with surfactant powder that was delivered at low flows (4–10 L/min) (31). The dry powder surfactant particles tended to aggregate upon delivery due to humidification of the oxygen respiratory gases in the circuit. Half of the lambs required a second dose of ~100 mg/kg of surfactant to reach oxygenation and lung gas volumes similar to the positive Curosurf controls.
Beyond nebulizer technology, location of the nebulizer in the ventilatory circuit and the characteristics of the CPAP interface (prongs or facemask) are important for its efficacy. Placing the nebulizer between the Y-piece and the CPAP interface achieves far higher lung doses than placement in the inspiratory limb way before the Y-piece. Depositional loss of nasally inhaled aerosols in infants has been investigated in vitro in a preterm nose-throat replica (67). Prong type (internal or external), configuration (single and dual), and insertion depth all affect lung delivery efficiency. Dual prongs at an insertion depth of 2 mm are probably best with upper airway losses of 15–20% (44). Various studies describe ingenious modifications of the nasal interface to improve aerosol delivery during nCPAP support, but these have not been tested in vivo yet (68).
Findings from Animal and Clinical Studies
Animal Studies
Table 1 shows an overview of animal studies in which non-invasive ventilatory support was provided with nCPAP and various clinical and synthetic surfactants were delivered by aerosolization (46–56, 69). Nebulization of surfactant was safe and major adverse effects were limited to reflux of surfactant and temporary desaturation during the procedure. Effectivity, measured by improvement in oxygenation and lung compliance, varied considerably and correlated with lung deposition which varied from 11 to about 40%. Doubling of the clinically used dosage of Curosurf (400 instead of 200 mg/kg) was well tolerated and possibly more effective, but tripling of the dosage (600 mg/kg) did not provide better results (50, 53, 54). Most animal studies opted for the use of VMN nebulizers, demonstrating the move away from jet nebulizers in newborns. Synchronizing surfactant delivery with inspiration led to higher lung deposition (49, 56) and therefore deserves further exploration. Older synthetic surfactants (29, 46) were less effective than clinical or newer synthetic lung surfactant (31).
Clinical Studies
Early clinical studies with jet ventilators published in the 1990s indicated that surfactant nebulization was safe, except for surfactant reflux and desaturations, but clinical results did not meet expectations [Table 2, (34–36)]. The pilot study by Finer et al. (14) with a prototype VMN nebulizer has rekindled clinical studies on non-invasive surfactant administration in preterm infants supported with nCPAP (Table 2). Up so far, six published clinical studies have investigated whether non-invasive aerosol delivery of animal-derived (clinical) surfactant can prevent the need for early intubation for invasive mechanical ventilation and/or intratracheal surfactant instillation (57–62). Three studies showed a significant reduction in nCPAP failure (57, 59, 62), two studies (58, 60) did not recruit controls but their results pointed in the same direction, and one study (61) did not show any effectivity, possibly because of air leakage around the nasal prongs. The most recent published study (62) used a VMN nebulizer with breath-actuation of surfactant delivery and suggests that a double dose of clinical surfactant given at the right time of the respiratory cycle may be the way forward in non-invasive surfactant administration in preterm infants with RDS who are supported with nCPAP ventilation. Although this study is relatively small, its positive results warrant larger studies exploring this technology.
Both animal and clinical studies show the important role of currently available clinical surfactant preparations in aerosol delivery of surfactant therapy in infants. Newer dry powder and liquid synthetic surfactant formulations are catching up with clinical surfactants and may provide a more cost-effective therapeutic option than animal-derived (clinical) surfactants in the near future.
Conclusion
Non-invasive surfactant delivery is currently the Holy Grail in neonatology, but its search is challenged by technical problems to optimize lung deposition of nebulized surfactant. The currently available evidence suggests that non-invasive nebulization of clinical and advanced synthetic lung surfactant with breath-actuated VMN technology and an appropriate nasal interface is a promising way forward and has great potential to become part of future non-invasive respiratory care for preterm and term infants. Ongoing research on nebulizer technology and its nasal interface should focus on optimization of the delivery route and lung dose of highly active lung surfactant synthetic formulations.
Author Contributions
FW wrote and AW revised the manuscript. All authors contributed to the article and approved the submitted version.
Funding
This work was supported by the Bill & Melinda Gates Foundation (INV-001227 and INV-040846). Under the grant conditions of the Foundation, a Creative Commons Attribution 4.0 Generic License has already been assigned to the author accepted manuscript version that might arise from this submission. The funders had no role in study design, data collection and analysis, decision to publish, or preparation of the manuscript.
Conflict of Interest
The authors declare that the research was conducted in the absence of any commercial or financial relationships that could be construed as a potential conflict of interest.
Publisher's Note
All claims expressed in this article are solely those of the authors and do not necessarily represent those of their affiliated organizations, or those of the publisher, the editors and the reviewers. Any product that may be evaluated in this article, or claim that may be made by its manufacturer, is not guaranteed or endorsed by the publisher.
Abbreviations
ARDS, Acute respiratory distress syndrome; CPAP, Continuous positive airway pressure; DPPC, Dipalmitoylphosphatidylcholine; FiO2, Fraction of inspired oxygen; HFNC, High flow nasal cannula; INSURE, Intubation, surfactant extubation; IT, Intratracheally; LFAC, Low flow aerosolization chamber; LISA, Less invasive surfactant administration; LPM, Liters per minute; nCPAP, Nasal CPAP; NIPPV, Nasal intermittent positive pressure ventilation; NIV, Noninvasive ventilation; PEEP, Positive end-expiratory pressure; PG, Phosphatidylglycerol; pMDI, Pressurized metered-dose inhalers; POPC, 1-palmitoyl-2-oleoyl-sn-glycero-3-phosphocholine; POPG, 1-palmitoyl 2-oleyl phosphatidylglycerol; RDS, Respiratory distress syndrome; rhSP-C, Recombinant human SP-C; SD, Standard deviation; SP, Surfactant protein; VMN, Vibrating mesh nebulizer.
References
1. Sardesai S, Biniwale M, Wertheimer F, Garingo A, Ramanathan R. Evolution of surfactant therapy for respiratory distress syndrome: past, present, and future. Pediatr Res. (2017) 81:240–8. doi: 10.1038/pr.2016.203
2. Nogee LM, Wert SE, Proffit SA, Hull WM, Whitsett JA. Allelic heterogeneity in hereditary surfactant protein B (SP-B) deficiency. Am J Respir Crit Care Med. (2000) 161:973–81. doi: 10.1164/ajrccm.161.3.9903153
3. Griese M, Lorenz E, Hengst M, Schams A, Wesselak T, Rauch D, et al. Surfactant proteins in pediatric interstitial lung disease. Pediatr Res. (2016) 79:34–41. doi: 10.1038/pr.2015.173
4. Stoll BJ, Hansen NI, Bell EF, Walsh MC, Carlo WA, Shankaran S, et al. Trends in care practices, morbidity, and mortality of extremely preterm neonates, 1993–2012. JAMA. (2015) 314:1039–51. doi: 10.1001/jama.2015.10244
5. Walther FJ, Gordon LM, Waring AJ. Advances in synthetic lung surfactant protein technology. Expert Rev Respir Med. (2019) 13:499–501. doi: 10.1080/17476348.2019.1589372
6. Wiingreen R, Greisen G, Ebbesen F, Petersen JP, Zachariassen G, Henriksen TB, et al. Surfactant need by gestation for very preterm babies initiated on early nasal CPAP: a Danish observational multicentre study of 6,628 infants born 2000–2013. Neonatology. (2017) 111:331–6. doi: 10.1159/000451021
7. Hatch LD 3rd, Clark RH, Carlo WA, Stark AR, Ely EW, Patrick SW. Changes in use of respiratory support for preterm infants in the US, 2008–2018. JAMA Pediatr. (2021) 175:1017–24. doi: 10.1001/jamapediatrics.2021.1921
8. Herting E, Härtel C, Göpel W. Less invasive surfactant administration (LISA): chances and limitations. Arch Dis Child Fetal Neonatal Ed. (2019) 104:F655–9. doi: 10.1136/archdischild-2018-316557
9. Findlay RD, Taeusch HW, Walther FJ. Surfactant replacement therapy for meconium aspiration syndrome. Pediatrics. (1996) 97:48–52. doi: 10.1542/peds.97.1.48
10. Cornfield DN. Acute respiratory distress syndrome in children: physiology and management. Curr Opin Pediatr. (2013) 25:338–43. doi: 10.1097/MOP.0b013e328360bbe7
11. Morley CJ, Greenough A, Miller NG, Bangham AD, Pool J, Wood S, et al. Randomized trial of artificial surfactant (ALEC) given at birth to babies from 23 to 34 weeks gestation. Early Hum Dev. (1988) 17:41–54. doi: 10.1016/S0378-3782(88)80056-2
12. Tooley WH, Clements JA, Muramatsu K, Brown CL, Schlueter MA. Lung function in prematurely delivered rabbits treated with a synthetic surfactant. Am Rev Respir Dis. (1987) 136:651–6. doi: 10.1164/ajrccm/136.3.651
13. Moya FR, Gadzinowski J, Bancalari E, Salinas V, Kopelman B, Bancalari A, et al. A multicenter, randomized, masked, comparison trial of lucinactant, colfosceril palmitate, and beractant for the prevention of respiratory distress syndrome among very preterm infants. Pediatrics. (2005) 115:1018–29. doi: 10.1542/peds.2004-2183
14. Finer NN, Merritt TA, Bernstein G, Job L, Mazela J, Segal R. An open label, pilot study of Aerosurf® combined with nCPAP to prevent RDS in preterm neonates. J Aerosol Med Pulm Drug Deliv. (2010) 23:303–9. doi: 10.1089/jamp.2009.0758
15. Spragg RG, Lewis JF, Walmrath HD, Johannigman J, Bellingan G, Laterre PF, et al. Effect of recombinant surfactant protein C-based surfactant on the acute respiratory distress syndrome. N Engl J Med. (2004) 351:884–92. doi: 10.1056/NEJMoa033181
16. Walther FJ, Gordon LM, Waring AJ. Design of surfactant protein B peptide mimics based on the saposin fold for synthetic lung surfactants. Biomed Hub. (2016) 1:1–21. doi: 10.1159/000451076
17. Waring AJ, Walther FJ, Gordon LM, Hernandez-Juviel JM, Hong T, Sherman MA, et al. The role of charged amphipathic helices in the structure and function of surfactant protein B. J Pept Res. (2005) 66:364–74. doi: 10.1111/j.1399-3011.2005.00300.x
18. Walther FJ, Waring AJ, Hernandez-Juviel JM, Gordon LM, Wang Z, Jung CL, et al. Critical structural and functional roles for the N-terminal insertion sequence in surfactant protein B analogs. PLoS ONE. (2010) 5:e8672. doi: 10.1371/journal.pone.0008672
19. Walther FJ, Gupta M, Gordon LM, Waring AJ. A sulfur-free peptide mimic of surfactant protein B (B-YL) exhibits high in vitro and in vivo surface activities. Gates Open Res. (2018) 2:13. doi: 10.12688/gatesopenres.12799.2
20. Waring AJ, Gupta M, Gordon LM, Fujii G, Walther FJ. Stability of an amphipathic helix-hairpin surfactant peptide in liposomes. Biochim Biophys Acta. (2016) 1858:3113–9. doi: 10.1016/j.bbamem.2016.09.014
21. Walther FJ, Sharma S, Gordon LM, Waring AJ. Structural and functional stability of the sulfur-free surfactant protein B peptide mimic B-YL in synthetic surfactant lipids. BMC Pulm Med. (2021) 21:330. doi: 10.1186/s12890-021-01695-0
22. Johansson J, Some M, Linderholm BM, Almlén A, Curstedt T, Robertson B, et al. Synthetic surfactant based on a poly-Leu SP-C analog and phospholipids: effects on tidal volumes and lung gas volumes in ventilated immature newborn rabbits. J Appl Physiol. (2003) 95:2055–63. doi: 10.1152/japplphysiol.00153.2003
23. Ricci F, Murgia X, Razzetti R, Pelizzi N, Salomone F. In vitro and in vivo comparison between poractant alfa and the new generation synthetic surfactant CHF5633. Pediatr Res. (2017) 81:369–75. doi: 10.1038/pr.2016.231
24. Walther FJ, Waring AJ, Hernández-Juviel JM, Ruchala P, Wang Z, Notter RH, et al. Surfactant protein C peptides with salt-bridges (“ion-locks”) promote high surfactant activities by mimicking the α-helix and membrane topography of the native protein. PeerJ. (2014) 2:e485. doi: 10.7717/peerj.485
25. Brown NJ, Lin JS, Barron AE. Helical side chain chemistry of a peptoid-based SP-C analogue: Balancing structural rigidity and biomimicry. Biopolymers. (2019) 110:e23277. doi: 10.1002/bip.23277
26. Sweet DG, Turner MA, Stranák Z, Plavka R, Clarke P, Stenson BJ, et al. A first-in-human clinical study of a new SP-B and SP-C enriched synthetic surfactant (CHF5633) in preterm babies with respiratory distress syndrome. Arch Dis Child Fetal Neonatal Ed. (2017) 102:F497–503. doi: 10.1136/archdischild-2017-312722
27. Sweet DG, Turner M, Stranák Z, Plavka R, Clarke P, Stenson B, et al. A first-in-human clinical study of a new SP-B and SP-C enriched synthetic surfactant (CHF5633) in preterm babies with respiratory distress syndrome: two-year outcomes. J Matern Fetal Neonatal Med. (2020) 20:1–5. doi: 10.1080/14767058.2020.1863363
28. Ramanathan R, Biniwale M, Sekar K, Hanna N, Golombek S, Bhatia J, et al. Synthetic surfactant CHF5633 compared with poractant alfa in the treatment of neonatal respiratory distress syndrome: a multicenter, double-blind, randomized, controlled clinical trial. J Pediatr. (2020) 225:90–6.e1. doi: 10.1016/j.jpeds.2020.06.024
29. Gregory TJ, Irshad H, Chand R, Kuehl PJ. Deposition of aerosolized lucinactant in nonhuman primates. J Aerosol Med Pulm Drug Deliv. (2020) 33:21–33. doi: 10.1089/jamp.2018.1505
30. Chow AHL, Tong HHY, Chattopadhyay P, Shekunov BY. Particle engineering for pulmonary drug delivery. Pharm Res. (2007) 24:411–37. doi: 10.1007/s11095-006-9174-3
31. Walther FJ, Waring AJ, Otieno M, DiBlasi RM. Efficacy, dose-response, and aerosol delivery of dry powder synthetic lung surfactant treatment in surfactant-deficient rabbits and premature lambs. Respir Res. (2022) 23:78. doi: 10.1186/s12931-022-02007-8
32. Boc S, Momin MAM, Farkas DR, Longest W, Hindle M. Development and characterization of excipient enhanced growth (EEG) surfactant powder formulations for treating neonatal respiratory distress syndrome. AAPS PharmSciTech. (2021) 22:136. doi: 10.1208/s12249-021-02001-1
33. Lewis JF, Ikegami M, Jobe AH, Tabor B. Aerosolized surfactant treatment of preterm lambs. J Appl Physiol. (1991) 70:869–76. doi: 10.1152/jappl.1991.70.2.869
34. Jorch G, Hartl H, Roth B, Kribs A, Gortner L, Schaible T, et al. Surfactant aerosol treatment of respiratory distress syndrome in spontaneously breathing premature infants. Pediatr Pulmonol. (1997) 24:222–4. doi: 10.1002/(SICI)1099-0496(199709)24:3<222::AID-PPUL9>3.0.CO;2-O
35. Arroe M, Pedersen-Bjergaard L, Albertsen P. Inhalation of aerosolized surfactant (Exosurf®) to neonates treated with nasal continuous positive airway pressure. Prenat Neonat Med. (1998) 3:346–52.
36. Berggren E, Liljedahl M, Winbladh B, Andreasson B, Curstedt T, Robertson B, et al. Pilot study of nebulized surfactant therapy for neonatal respiratory distress syndrome. Acta Paediatr. (2000) 89:460–4. doi: 10.1111/j.1651-2227.2000.tb00084.x
37. Bancalari E, Claure N. Non-invasive ventilation of the preterm infant. Early Hum Dev. (2008) 84:815–9. doi: 10.1016/j.earlhumdev.2008.09.010
38. Bianco F, Salomone F, Milesi I, Murgia X, Bonelli S, Pasini E, et al. Aerosol drug delivery to spontaneously-breathing preterm neonates: lessons learned. Respir Res. (2021) 22:71. doi: 10.1186/s12931-020-01585-9
39. DiBlasi RM. Clinical controversies in aerosol therapy for infants and children. Respir Care. (2015) 60: 894–914. doi: 10.4187/respcare.04137
40. Cummings JJ, Polin RA. Committee on fetus and newborn, american academy of pediatrics. Non-invasive respiratory support. Pediatrics. (2016) 138:e20161576. doi: 10.1542/peds.2015-3758
41. Dargaville PA, Gerber A, Johansson S, De Paoli AG, Kamlin CO, Orsini F, et al. Incidence and outcome of CPAP failure in preterm infants. Pediatrics. (2016) 138:e20153985. doi: 10.1542/peds.2015-3985
42. Bianco F, Pasini E, Nutini M, Murgia X, Stoeckl C, Schlun M, et al. Extended pharmacopeial characterization of surfactant aerosols generated by a customized eFlow Neos nebulizer delivered through neonatal nasal prongs. Pharmaceutics. (2020) 12:319. doi: 10.3390/pharmaceutics12040319
43. Matuszak M, Ochowiak M, Włodarczak S, Krupińska A, Doligalski M. State-of-the-art review of the application and development of various methods of aerosol therapy. Int J Pharm. (2022) 614:121432. doi: 10.1016/j.ijpharm.2021.121432
44. Bass K, Momin MAM, Howe C, Aladwani G, Strickler S, Kolanjiyil AV, et al. Characterizing the effects of nasal prong interfaces on aerosol deposition in a preterm infant nasal model. AAPS PharmSciTech. (2022) 23:114. doi: 10.1208/s12249-022-02259-z
45. Tiemersma S, Minocchieri S, van Lingen RA, Nelle M, Devadason SG. Vibrating membrane devices deliver aerosols more efficient than standard devices: a study in a neonatal upper airway model. J Aerosol Med Pulm Drug Deliv. (2013) 26:280–6. doi: 10.1089/jamp.2012.0993
46. Rahmel DK, Pohlmann G, Iwatschenko P, Volland J, Liebisch S, Kock H, et al. The non-intubated, spontaneously breathing, continuous positive airway pressure (CPAP) ventilated pre-term lamb: a unique animal model. Reprod Toxicol. (2012) 34:204–15. doi: 10.1016/j.reprotox.2012.05.089
47. Hütten MC, Kuypers E, Ophelders DR, Nikiforou M, Jellema RK, Niemarkt HJ, et al. Nebulization of Poractant alfa via a vibrating membrane nebulizer in spontaneously breathing preterm lambs with binasal continuous positive pressure ventilation. Pediatr Res. (2015) 78:664–9. doi: 10.1038/pr.2015.165
48. Linner R. Perez-de-Sa V, Cunha-Goncalves D. Lung deposition of nebulized surfactant in newborn piglets. Neonatology. (2015) 107:277–82. doi: 10.1159/000369955
49. Milesi I, Tingay DG, Lavizzari A, Bianco F, Zannin E, Tagliabue P, et al. Supraglottic atomization of surfactant in spontaneously breathing lambs receiving continuous positive airway pressure. Pediatr Crit Care Med. (2017) 18:e428–34. doi: 10.1097/PCC.0000000000001267
50. Bianco F, Ricci F, Catozzi C, Murgia X, Schlun M, Bucholski A, et al. From bench to bedside: in vitro and in vivo evaluation of a neonate-focused nebulized surfactant delivery strategy. Respir Res. (2019) 20:134. doi: 10.1186/s12931-019-1096-9
51. Cunha-Goncalves D, Nord A, Bianco F, Salomone F, Ricci F, Schlun M, et al. Impact of body position on lung deposition of nebulized surfactant in newborn piglets on nasal continuous positive airway pressure. Neonatology. (2020) 117:467–73. doi: 10.1159/000508349
52. Rey-Santano C, Mielgo VE, Gomez-Solaetxe MA, Bianco F, Salomone F, Loureiro B. Nebulized Poractant Alfa reduces the risk of respiratory failure at 72 hours in spontaneously breathing surfactant-deficient newborn piglets. Crit Care Med. (2020) 48:e523–31. doi: 10.1097/CCM.0000000000004318
53. Rey-Santano C, Mielgo V, Gomez-Solaetxe MA, Ricci F, Bianco F, Salomone F, et al. Dose-response study on surfactant nebulization therapy during nasal continuous positive airway pressure ventilation in spontaneously breathing surfactant-deficient newborn piglets. Pediatr Crit Care Med. (2020) 21:e456–66. doi: 10.1097/PCC.0000000000002313
54. Nord A, Bianco F, Salomone F, Ricci F, Schlun M, Linner R, et al. Nebulization of high-dose poractant alfa in newborn piglets on nasal continuous positive airway pressure yields therapeutic lung doses of phospholipids. Am J Perinatol. (2020). doi: 10.1055/s-0040-1721392. [Epub ahead of print].
55. Nord A, Linner R, Salomone F, Bianco F, Ricci F, Murgia X, et al. Lung deposition of nebulized surfactant in newborn piglets: nasal CPAP vs. nasal IPPV. Pediatr Pulmonol. (2020) 55:514–20. doi: 10.1002/ppul.24603
56. Nord A, Linner R, Milesi I, Zannin E, di Castri M, Bianco F, et al. A novel delivery system for supraglottic atomization allows increased lung deposition rates of pulmonary surfactant in newborn piglets. Pediatr Res. (2020) 87:1019–24. doi: 10.1038/s41390-019-0696-x
57. Minocchieri S, Berry CA, Pillow JJ, CureNeb Study Team. Nebulised surfactant to reduce severity of respiratory distress: a blinded, parallel, randomised controlled trial. Arch Dis Child Fetal Neonatal Ed. (2019) 104:F313–9. doi: 10.1136/archdischild-2018-315051
58. Sood BG, Cortez J, Kolli M, Sharma A, Delaney-Black V, Chen X. Aerosolized surfactant in neonatal respiratory distress syndrome: phase I study. Early Hum Dev. (2019) 134:19–25. doi: 10.1016/j.earlhumdev.2019.05.005
59. Cummings JJ, Gerday E, Minton S, Katheria A, Albert G, Flores-Torres J, et al. Aerosolized calfactant for newborns with respiratory distress: a randomized trial. Pediatrics. (2020) 146:e20193967. doi: 10.1542/peds.2019-3967
60. Sood BG, Thomas R, Delaney-Black V, Xin Y, Sharma A, Chen X. Aerosolized beractant in neonatal respiratory distress syndrome: a randomized fixed-dose parallel-arm phase II trial. Pulm Pharmacol Ther. (2021) 66:101986. doi: 10.1016/j.pupt.2020.101986
61. Dani C, Talosi G, Piccinno A, Ginocchio VM, Balla G, Lavizzari A, et al. A randomized, controlled trial to investigate the efficacy of nebulized poractant alfa in premature babies with respiratory distress syndrome. J Pediatr. (2022). doi: 10.1016/j.jpeds.2022.02.054. [Epub ahead of print].
62. Jardine L, Lui K, Liley HG, Schindler T, Fink J, Asselin J, et al. Trial of aerosolised surfactant for preterm infants with respiratory distress syndrome. Arch Dis Child Fetal Neonatal Ed. (2022) 107:51–5. doi: 10.1136/archdischild-2021-321645
63. DiBlasi RM, Kajimoto M, Poli JA, Deutsch G, Pfeiffer J, Zimmerman J, et al. Breath-synchronized nebulized surfactant in a porcine model of acute respiratory distress syndrome. Crit Care Explor. (2021) 3:e0338. doi: 10.1097/CCE.0000000000000338
64. DiBlasi RM, Micheletti KJ, Zimmerman JD, Poli JA, Fink JB, Kajimoto M. Physiologic effects of instilled and aerosolized surfactant using a breath-synchronized nebulizer on surfactant-deficient rabbits. Pharmaceutics. (2021) 13:1580. doi: 10.3390/pharmaceutics13101580
65. Wiegandt FC, Froriep UP, Müller F, Doll T, Dietzel A, Pohlmann G. Breath-triggered drug release system for preterm neonates. Pharmaceutics. (2021) 13:657. doi: 10.3390/pharmaceutics13050657
66. Dhand R. How should aerosols be delivered during invasive mechanical ventilation? Respir Care. (2017) 62:1343–67. doi: 10.4187/respcare.05803
67. Minocchieri S, Burren JM, Bachmann MA, Stern G, Wildhaber J, Buob S, et al. Development of the premature infant nose throat-model (PrINT-Model): an upper airway replica of a premature neonate for the study of aerosol delivery. Pediatr Res. (2008) 64:141–6. doi: 10.1203/PDR.0b013e318175dcfa
68. Howe C, Momin MAM, Bass K, Aladwani G, Bonasera S, Hindle M, et al. In vitro analysis of nasal interface options for high-efficiency aerosol administration to preterm infants. J Aerosol Med Pulm Drug Deliv. (2022). doi: 10.1089/jamp.2021.0057. [Epub ahead of print].
69. Pohlmann G, Iwatschenko P, Koch W, Windt H, Rast M, de Abreu MG, et al. A novel continuous powder aerosolizer (CPA) for inhalative administration of highly concentrated recombinant surfactant protein-C (rSP-C) surfactant to preterm neonates. J Aerosol Med Pulm Drug Deliv. (2013) 26:370–9. doi: 10.1089/jamp.2012.0996
Keywords: surfactant, nasal CPAP, non-invasive ventilation, aerosol delivery, nebulization, neonatal respiratory distress syndrome, vibrating mesh nebulizers
Citation: Walther FJ and Waring AJ (2022) Aerosol Delivery of Lung Surfactant and Nasal CPAP in the Treatment of Neonatal Respiratory Distress Syndrome. Front. Pediatr. 10:923010. doi: 10.3389/fped.2022.923010
Received: 18 April 2022; Accepted: 24 May 2022;
Published: 15 June 2022.
Edited by:
Martin Post, University of Toronto, CanadaReviewed by:
Haifeng Zong, Southern Medical University, ChinaLi Wang, University-Town Hospital of Chongqing Medical University, China
Copyright © 2022 Walther and Waring. This is an open-access article distributed under the terms of the Creative Commons Attribution License (CC BY). The use, distribution or reproduction in other forums is permitted, provided the original author(s) and the copyright owner(s) are credited and that the original publication in this journal is cited, in accordance with accepted academic practice. No use, distribution or reproduction is permitted which does not comply with these terms.
*Correspondence: Frans J. Walther, Zmp3YWx0aGVyQHVjbGEuZWR1