- 1Department of Child Health, College of Medicine, King Khalid University, Abha, Saudi Arabia
- 2Department of Microbiology and Clinical Parasitology, College of Medicine, King Khalid University, Abha, Saudi Arabia
Meningitis is an inflammation of the brain and spinal cord meninges caused by infectious and non-infectious agents. Infectious agents causing meningitis include viruses, bacteria, and fungi. Viral meningitis (VM), also termed aseptic meningitis, is caused by some viruses, such as enteroviruses (EVs), herpesviruses, influenza viruses, and arboviruses. However, EVs represent the primary cause of VM. The clinical symptoms of this neurological disorder may rapidly be observed after the onset of the disease, or take prolonged time to develop. The primary clinical manifestations of VM include common flu-like symptoms of headache, photophobia, fever, nuchal rigidity, myalgia, and fatigue. The severity of these symptoms depends on the patient's age; they are more severe among infants and children. The course of infection of VM varies between asymptomatic, mild, critically ill, and fatal disease. Morbidities and mortalities of VM are dependent on the early recognition and treatment of the disease. There were no significant distinctions in the clinical phenotypes and symptoms between VM and meningitis due to other causative agents. To date, the pathophysiological mechanisms of VM are unclear. In this scientific communication, a descriptive review was performed to give an overview of pediatric viral meningitis (PVM). PVM may occasionally result in severe neurological consequences such as mental retardation and death. Clinical examinations, including Kernig's, Brudzinski's, and nuchal rigidity signs, were attempted to determine the clinical course of PVM with various success rates revealed. Some epidemiological correlates of PVM were adequately reviewed and presented in this report. They were seen depending mainly on the causative virus. The abnormal cytological and biochemical features of PVM were also discussed and showed potentials to distinguish PVM from pediatric bacterial meningitis (PBM). The pathological, developmental, behavioral, and neuropsychological complications of PVM were also presented. All the previously utilized techniques for the etiological diagnosis of PVM which include virology, serology, biochemistry, and radiology, were presented and discussed to determine their efficiencies and limitations. Finally, molecular testing, mainly PCR, was introduced and showed 100% sensitivity rates.
Introduction
Meningitis is a neuropathological disorder due to an inflammation of the meninges that enclose the brain and spinal cord (1). This condition is caused by infectious and non-infectious agents. Non-infectious causes of meningitis include some systemic diseases, drugs, and neoplasms (2). Infectious agents that cause meningitis include viruses, bacteria, and fungi. However, viruses are the most common causative agents of meningitis. Viral meningitis (VM), also called aseptic meningitis, is considered a slow and mild viral disease and regarded as a typical model for chronic nervous system diseases (1, 3). VM is the primary type of aseptic meningitis that commonly affects children. Several viruses were incriminated in the causation of aseptic VM, like enteroviruses, herpesviruses, orthomyxoviruses, arboviruses, and coronaviruses (3, 4). Enteroviruses (EVs) are the most common viruses that cause meningitis among pediatric patients (4, 5). Non-polio EVs were responsible for the highest incidence rates of pediatric VM (PVM) (6, 7). The severe acute respiratory syndrome-coronavirus-2 (SARS-CoV-2), which is responsible for the ongoing COVID-19 pandemic, was also recognized to cause minor cases of PVM (8). It was also reported that the highest incidences, morbidities, mortalities, and disease infectivity rates of PVM were seen among children between 5 and 10 years old (7, 9). Although no seasonal preference for VM was documented but most commonly observed to occur in summer and autumn (1, 7). The epidemiological correlates of PVM were well-described and reviewed in many parts of the world, particularly in the Middle East (ME) region (10–15). These studies indicated that PVM was most commonly occurring among children and young adults (10). They also confirmed that Echovirus 7 (E-7), E-9, E-11, and E-30 constitute the significant viral agents causing PVM (11, 12). Cases of PVM among newborns and infants have also been reported in several studies (7, 11, 14).
The clinical symptoms of PVM were confirmed to be almost the same in their phenotypes and presentations in all cases of the disease, irrespective of the viral etiology (16). Several risk factors associated with PVM, such as age and immunosuppression, were also investigated and confirmed to affect the disease occurrence and severity (1, 6, 7). Brudzinski's signs, Kernig's signs, or nuchal rigidity were employed as physical examinations by clinicians and considered of significant diagnostic values for all etiological types of meningitis including VM (17, 18). Lumbar puncture (LP) for pleocytosis determination or viral detection in the CSF was recommended to confirm the disease (18). Non-specific signs including fever, chills, abdominal pain, nausea, headache, fast breathing, loss of appetite, neck stiffness, and sensitivity to bright light were totally or partially observed among PVM patients (4, 19). Some neurological manifestations like misconception and blurt vision have also been observed in some PVM cases (20). Complications due to PVM are rare to occur, and spontaneous resolution of the disease is not uncommon (21). Clinical signs, disease history, and physical examinations constitute the potential diagnostic tools for PVM. Biochemistry and cytology of cerebrospinal fluid (CSF) were commonly determined and employed as differential diagnostic tests between VM and BM (2). Serological assays for detecting the viral antigens were also used and proved practical and efficient to diagnose VM (4). Polymerase chain reaction (PCR) is the gold standard test for detection of the viral nucleic acid in the CSF of the PVM patients, with the highest sensitivity rates attained (22–24). PCR was also employed to detect the viral nucleic acids during PVM cases in other clinical specimens like blood, throat and nasal swabs, and stools with high sensitivity and specificity levels obtained (25). Other molecular approaches like next-generation sequencing (NGS) were also recently employed to detect viruses in some clinical specimens of PVM (26). Following the systematic search in the literature, we found some publications addressing meningitis due to different etiologies. We also saw plenty of case reports highlighting the various clinical aspects of the disease among children. However, we did not come across a single review article explicitly discussing the PVM as a compiled presentation. Therefore, we would like to address the issue and provide a comprehensive descriptive presentation of the etiology, clinical phenotypes, epidemiology, laboratory abnormalities, complications, and diagnostic challenges of PVM. These elements of PVM are compared to those of PBM and summarized in Table 1.
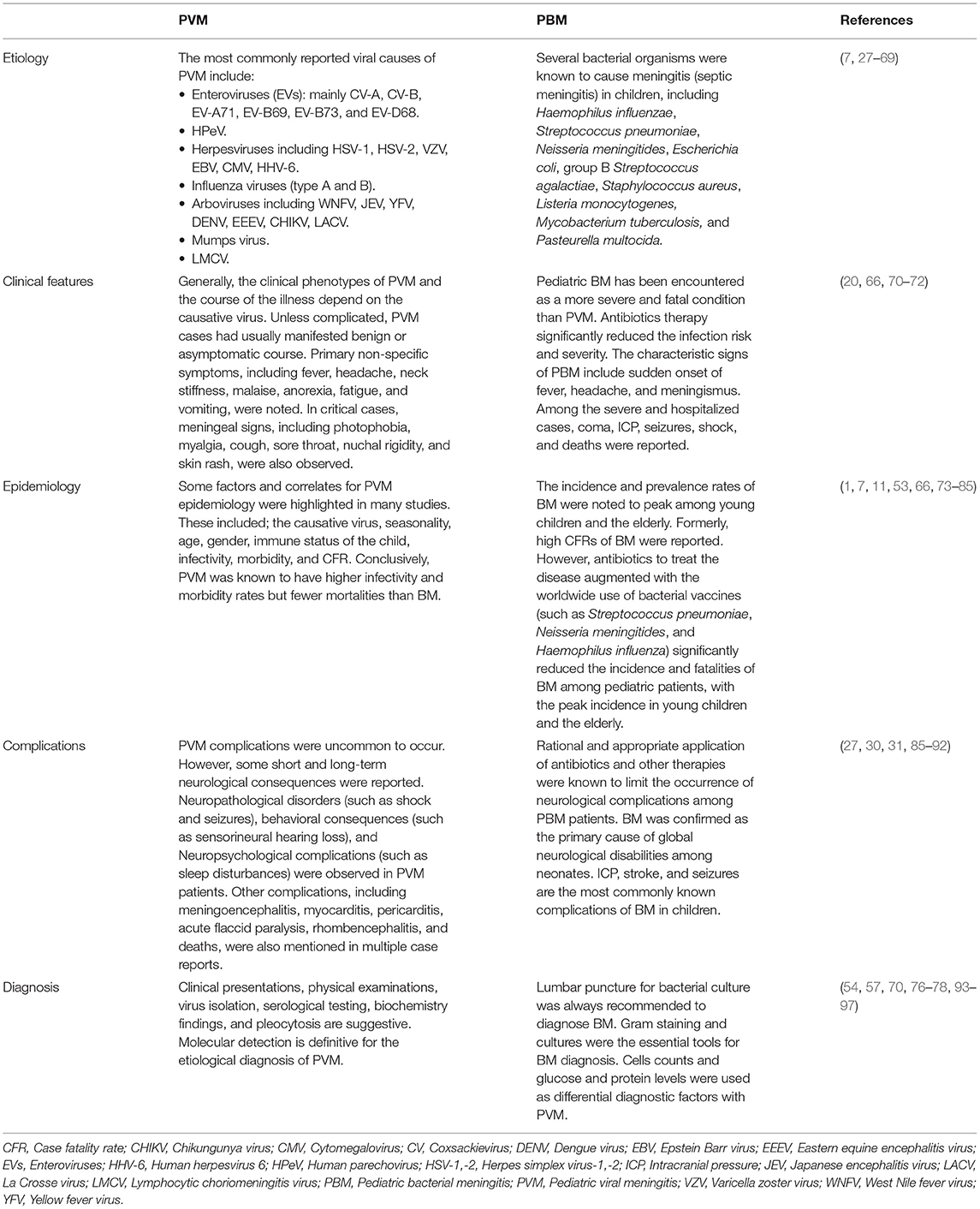
Table 1. Distinctive etiological, clinical, epidemiological, complications, and diagnostic elements of pediatric viral and bacterial meningitis: Comparative presentation.
Methods
A comprehensive systematic electronic search in the leading bibliographic platforms for the different scientific elements of PVM was made in this study. The data obtained were collected, registered, and declared in this study regardless of the geographical and seasonal considerations. The search was accomplished through the PubMed, MEDLINE, and Google Scholar databases. The keywords used to search for the information were meningitis, virus, pediatric, children, infants, epidemiology, symptoms, laboratory, complications, and diagnosis. The articles considered are those published from the ancient times till the year 2022. Inclusion criteria included articles with confirmed positive cases of PVM. The articles published in non-peer-reviewed journals were excluded. Publications related to all age groups of pediatric patients (neonates, newborns, infants, young children, and old children) were considered in the study. The selected articles were carefully read and summarized, and the retrieved data were used in the study description. The essential scientific elements concerning the disease etiology, clinical phenotypes, epidemiology, laboratory abnormalities, complications, and diagnosis were extracted from the selected publications and recorded. Following the compilation of these data, a descriptive presentation was made.
Definitions
Meninges is an anatomical designation for the three membrane layers that enclose the brain and spinal cord. The name was derived from the Greek word meninx, which means membrane. These membranes have two important protective functions; first, they provide direct protection and support to the tissues of the brain and spinal cord; secondly, they are indirectly protecting them by attaching the brain and spinal cord to the skull and vertebral column bones. These meninges were also known to play a pivotal role in the development of the brain and skull during the evolutionary time of the embryonic stage (98–100). Meningitis is an inflammation of these meninges. From an etiological point of view, there are two major types of meningitis; septic meningitis and aseptic meningitis. Septic meningitis, a type of meningitis caused by pus-producing bacteria (101, 102). Aseptic meningitis is the type of meningitis caused by organisms other than bacteria, including viruses (3, 4, 19, 20, 103).
Etiology
Enteroviruses
Enteroviruses (EVs) are groups of non-enveloped RNA viruses belonging to the family Picornaviridae and genus Enterovirus. The genus Enterovirus includes nine EVs species named Enterovirus A, B, C, D, E, F, G, H, and J (104–107). Each of these EV species consists of multiple serotypes. The serotypes Coxsackievirus A and B (CV-A and CV-B) belonged to the species EV-A and EV-B, respectively (104). CV-A, CV-B, EV-B69, EV-A71, and EV-B73 are the most common EVs causing PVM (27–29). The enterovirus-D68 (EV-D68) was also reported in some studies as a common cause of PVM, with severe and fatal complications described (30, 31). Historically, EVs were firstly known as causative agents of PVM during the early sixties of the last Gregorian century (108). Later, they were confirmed in several epidemiological investigations to constitute the primary cause of VM in children (7, 32–34). In the Middle East region, most of the PVM cases were also attributed to the EVs group of viruses (10–15, 109). Although EVs-induced PVM registered the highest prevalence rates, it was not seen to result in serious complications among children when compared with EVs-induced encephalitis (110). Human parechoviruses (HPeV) is another group of picornaviruses that cause meningitis, particularly among children. HPeV-3 represents the primary type of HPeVs causing PVM (35, 36).
Herpesviruses
Out of the known eight human herpesviruses, six of them (HSV-1, HSV-2, VZV, EBV, CMV, and HHV-6) were recognized to cause PVM (37–39). The most peculiar pathogenic feature of HHVs-associated meningitis is that these viruses can remain latent in the human neurons following primary infection and causes meningitis after being reactivated (37). The different clinical and epidemiological features of recurrent meningitis due to the reactivated latent herpesviruses were recently reviewed and published by Rosenburg and Galen (40). HSV-1 was confirmed in some studies to cause only a few mild cases of PVM but high incidences of encephalitis. On the other hand, HSV-2 was observed to cause a higher number of recurrent PVM cases than HSV-1 (111). Other epidemiological studies indicated the reverse trend, which showed that HSV-1 is a predominant causative agent of PVM compared to HSV-2 (112). PVM due to the varicella-zoster virus (VZV) was also reported in many studies as a complication condition following the primary acute disease (41, 113). Compared to HSV-1 and HSV-2, VZV was identified as responsible for scarce cases of PVM characterized by benign clinical manifestations and spontaneous recovery (114). Interestingly, VZV-associated PVM cases were reported even among children who were vaccinated against the disease (115). Epstein Barr virus (EBV) was also documented in many reports as a significant causative agent of PVM (73, 116, 117). Meningitis due to EBV was described as a self-limiting neurological disorder with residual pathological outcomes detected only in meager cases (117). It has also been noted that EBV is more likely to induce a meningoencephalitis condition rather than PVM (118). The cytomegalovirus (CMV) and human herpesvirus 6 (HHV-6) were also mentioned in a few reports as causative agents of PVM (41–43). An infantile meningitis case due to HHV-6 in a premature infant was also recently reported (44). Generally, a consensus from an overwhelming number of reports indicated that introducing molecular techniques, mainly PCR, for detecting the herpesviral DNA in the CFS of patients vastly improved the identification of HHVs as etiological agents of PVM. Another important and hopeful note is that besides the mild and benign nature of HHVs-related PVM, antiviral agents for treating the patients (e.g., acyclovir) are available and effective.
Influenza Viruses
Despite the well-known respiratory nature of the diseases caused by human influenza viruses, some neurological disorders like meningitis (45) and meningioencephalitis (119) were also reported on rare occasions as consequences of the respiratory illness. While a laboratory animal model study suggested that the influenza viruses reach the CNS through the olfactory route (46), another tissue culture study showed that they get access to the CNS via the cranial nerve pathway (47). Many case reports and epidemiological investigations for influenza viruses-induced PVM have been published (48–50). Type A influenza viruses are the predominant influenza viruses reported to be associated with PVM cases. Influenza virus subtypes A-H5N1 (46, 47) and A-H1N1 (49) were confirmed to be involved in some PVM cases as CNS manifestations of the infection. Some reports indicating the involvement of influenza virus type B in PVM were also recently published (51, 52). Conclusively, PVM associated with influenza viruses has been known to be characterized by a mild course of infection and benign outcomes observed.
Arboviruses
As these are arthropod-borne viruses, the incidence of their PVM was highly correlated with their geographical and seasonal existence (53). The most commonly known arboviruses to cause meningitis are the West Nile Fever virus (WNFV) and the Japanese encephalitis virus (JEV). WNFV was known to arrive at the CNS through the hematogenous pathway after crossing the blood-brain barrier (BBB) (120). While WNFV was reported in the USA primarily as a causative agent of meningitis in adults, it was observed in other countries to cause more cases of pediatric children (54). It was also reported that WNFV is the predominant arbovirus that causes severe PVM leading to serious complications (55). JEV mainly targets the CNS and is noted to trigger the development of many neurological disorders, including meningitis. It was particularly observed endemic in the Southeast Asian region (53). Following laboratory detection of the JEV in the CSF of patients, some researchers in Japan recommended that JEV should not be ruled out in the differential diagnosis of aseptic meningitis (56). Other arboviral infections were also seen in many studies to induce PVM, including yellow fever virus (YFV) (53), Dengue virus (DENV) (57), Murray Valley encephalitis virus, and St. Louis encephalitis virus (53, 93), Eastern equine encephalitis virus (EEEV) (58, 59) and the Chikungunya virus (CHIKV) (60, 61). Although it is a primarily causative virus of encephalitis, the arbovirus La Crosse virus (LACV) was also identified in a large-scale retrospective surveillance study in the USA to cause some cases of PVM (62).
Mumps
Before vaccination of children against mumps infection was initiated, it was well known that the mumps virus is the most common virus causing PVM, particularly among non-vaccinated children worldwide (63). The PVM due to mumps infection had considerably declined after introducing the measles-mumps-rubella (MMR) vaccine in the early seventies of the last century (121). Conversely, PVM cases associated with the MMR vaccine were also recently reported in some studies (122, 123). The risk of developing PVM in children vaccinated with either the mumps vaccines, containing different mumps virus strains, or the MMR vaccine was extensively reviewed and reported by Bonnet and co-workers (124). Evaluation of the efficacy of the recently applied combined Measles-Mumps-Rubella-Varicella (MMRV) vaccine and its effect on the development of PVM were also investigated and known to reduce the risk of the disease occurrence (125). Generally, it had been confirmed that the application of these vaccines against mumps greatly changed the frequency of PVM from mumps as the most common causative viral agent of the disease to be supplanted by EVs (125).
Other Viruses
The human immunodeficiency virus (HIV) was also known to be associated with some cases of meningitis in adults. The chronic HIV infection results in immunosuppression status and encourages some opportunistic viruses, like CMV, to cause meningitis (123). However, this is becoming of absolute rare occurrence after the advent of the highly active antiretroviral therapy (HAAT). Although no cases of PVM due to HIV were identified, some hospital-based studies indicated that meningitis among HIV-positive children was observed to be associated with other bacterial (126) and fungal (127) organisms. The lymphocytic choriomeningitis virus (LCMV) was also suggested in some studies as a causative virus of PVM without concrete confirmatory evidences obtained up-to-date (64, 65). Rabies virus was also known to induce VM as a complication of the infection but with much lower frequencies (53, 128, 129). Other viral infections like human parvovirus B19 (HPV-B19), Nipah virus, and Bunyaviruses, were also known to be associated with VM in adult cases (53). However, there were no reports of PVM cases caused by these viruses. Some PVM cases were also seen linked to the infection with the severe acute respiratory syndrome-Coronavirus-2 (SARS-CoV-2) (8, 130, 131). On another interesting note, the frequency of the PVM reports due to EVs was known to be markedly declined during the COVID-19 pandemic time in some parts of Europe and Asia (132, 133).
Clinical Phenotypes
The clinical presentations of PVM and the severity of the disease were found to vary depending on many factors, including the causative virus, child's age, duration of symptoms, and immune status of the patient. The clinical course of PVM ranges from an asymptomatic, mild, critical, and fatal illness. Despite the highest prevalence rates of PVM in many parts of the world, the disease remains benign, self-limiting, and with a better prognosis expected. The non-specific symptoms of PVM include flu-like symptoms like fever, headache, neck stiffness, malaise, anorexia, fatigue, and vomiting (20). Enteroviral PVM is characterized by peculiar clinical features, including the sudden onset of biphasic fever, followed by systemic symptoms and meningeal signs (70). In protracted cases of EV-associated PVM, children may suffer photophobia, myalgia, cough, sore throat, nuchal rigidity, and skin rash (71). The newborns may not manifest overt clinical signs of PVM caused by EVs. However, they may show the signs of other systemic infections like hepatitis, myocarditis, and enteritis (70). Other studies indicated that PVM among newborns can be expressed by overt neurological manifestations like seizures and may lead to death (86). Recently, Alhazmi et al. reported a case of repeated PVM in a 9-day-old baby who presented to the pediatric emergency department (PED). This case was confirmed to be caused by EV in the first episode and HPeV in the second episode. Upon description of the clinical pictures of the disease in the two episodes of this case, different clinical manifestations were observed (24). These findings indicate that the etiological diagnosis of PVM depending on the clinical signs and laboratory indices is unreliable, but molecular analysis for the detection of the causative virus should always be considered. In conclusion, PVM was known to be characterized by benign clinical presentations, spontaneous recovery, and a relatively short hospitalization period. This clinical picture of PVM differs if a concomitant bacterial infection occurs where the patient's condition may deteriorate, and serious complications may occur (134).
Kernig's Sign
Kernig's sign approach is a maneuver to diagnose meningitis based on some clinical signs. The technique was invented and reported since a long time by a Russian physician named Vladimir Mikhailovich Kernig. It entitles keeping the patient suspected of meningitis in a sitting or supine position and his/her knees extended to a right angle. If the patient shows resistance or suffers from pain during the extension of the knees, this indicates the spasm of the hamstring muscles and suggests a meningitis condition (135).
Brudzinski's Sign
Brudzinski's sign is another type of physical examination used for clinical diagnosis of meningitis. This approach was suggested by the Polish pediatrician Josef Brudzinski in the late years of the nineteen Gregorian century. He described four clinical signs if partially or totally exist then that is suggestive of meningitis. These signs are the cheek sign, symphyseal sign, Brudzinski's reflex, and Brudzinski's neck sign. The detailed application and the reflexes of these signs were adequately described and discussed in some previous reports (136–138). Generally, Brudzinski's sign should be considered positive for meningitis when passive flexion of the patient neck leads to involuntary flexion of the hips and knees (139). In pediatric patients, the reliability of both Kernig's sign and Brudzinski's sign as clinical examinations in diagnosing meningitis was determined and discussed in multiple previous studies (17, 140). Although some studies reported the low sensitivity rates of these signs to diagnose PVM, they still recommended in other studies to be applied (17, 94). We could not see a single published report discussing the application of these examinations in PVM, but they were usually recommended if PBM is suspected. Despite their association with BM, both Kernig's signs and Brudzinski's signs examinations did not constitute differential tools between BM and VM (141).
Nuchal Rigidity
Nuchal rigidity is another physical examination utilized by physicians to diagnose meningitis. It was defined as the difficulty in actively forward flexing of the neck secondary to stiff muscles (17). As a possible sign of PVM, nuchal rigidity was confirmed in early studies as a potential tool for diagnosing the disease (88). Moreover, positive nuchal rigidity was promoted as a definite indication for VM, but LP should still be performed when meningeal irritation is suspected (142).
Epidemiology
Age Susceptibility to VM
The susceptibility to VM was seen depending on some risk factors, such as the patient's age, immunity status, and concomitant other viral infections like HIV (1, 53, 93, 103). VM was known to affect the different age groups of people, with more severe clinical consequences detected among pediatric patients than adults (73). Newborns and infants are more likely to develop more acute and critical PVM cases than older children since they are not equipped with mature immune apparatus (7, 11, 74). In a long-term, cohort, population-based study of PVM among hospitalized children in Denmark in 2007, it was confirmed that the first peak of the disease occurred during the first months of the child's life, whereas the second peak of the disease was observed among 5-years old children (7). The authors attributed the peak in the prevalence of PVM during infancy to the lack of passive maternal-specific antibodies and during early childhood to the lack of previous exposure to the causative viruses.
Gender Distribution of PVM
Only few epidemiological studies discussed the PVM with reference to the gender distribution. Some reports indicated that males are more likely to develop PVM than females (10, 15, 53, 75). After an intensive search in the literature, we could not see plausible explanations for this type of gender preference. The behavioral and sociocultural factors in the different societies may be involved in PVM acquisition.
Seasonality Factor of PVM
PVM was known to occur in all seasons of the year. However, some studies reported higher incidences of the illness in the spring (53), autumn (1, 7, 76), and fall (77, 78). This seasonality in the PVM occurrence was mainly linked to the seasonal distribution of EVs infection, the primary causative factor of PVM (75). It has also been confirmed in some studies that the predominance of the different serotypes of EVs is geographical and seasonal dependent (79, 80). EVs infections were seen to be more prevalent in the summer and fall in the temperate climate region and over the year in the tropical and subtropical regions (20, 79, 81). As a general conclusive remark, we could not judge the factors that control the highest distribution rates of PVM in a particular season or another, and hence there is no seasonal prediction for PVM that can be documented in this report.
Infectivity Rates of PVM
Different epidemiological studies reported different infectivity rates of PVM. In a hospital-based a nationwide survey in Northern Finland in 1986, the annual infectivity rate of PVM was estimated as 4.5% out of the total hospitalized children (82). In another recent population-based, retrospective cohort study in South Korea, the infectivity rate of PVM was confirmed to have no pattern and was largely dependent on the prevalence rates of EVs infections (83). The infectivity rates of PVM were ostensibly dependent on the number of cases (sample size) included and the country of the study. We assume that one of the difficulties associated with the determination of infectivity rates of PVM in any epidemiological investigation is the large number of mild cases of the disease which passed unnoticed.
Morbidities and Mortalities
Although PVM was documented in many studies characterized by higher infectivity rates PBM, it was known to have lower morbidity and mortality rates (82, 141). The contrast between VM and BM as per the morbidities and mortalities was presented in several previous reports. In an epidemiological study, the mortality rates for PVM and PBM were calculated as 4.5 and 14.5%, respectively (82). The mortality rates of PVM and PBM were also calculated, in a recent national population-based epidemiological study, as 0.002 and 2.0%, respectively (83). Additionally, the morbidities and mortalities of PVM were also estimated in some previous cohort epidemiological studies to be 70 and 10%, respectively (70, 71, 86, 134). Although it had been documented in several studies that the morbidities and mortalities of PVM were dependent on the causative virus, we could not find precise estimates for these correlates in PVM studies caused by the different viral agents. The EV-associated PVM was confirmed to have higher morbidity and mortality rates than other etiological types of PVM (20). The morbidity and mortality rates of PVM were also known as age-dependent. In 2003, some researchers in the USA documented that the PVM represents a fundamental reason for high morbidities, long periods of hospitalizations, and considerable financial losses among infants and young children (84).
Laboratory Abnormalities
The laboratory investigations were used as a confirmatory diagnostic tool for VM following clinical examination of the suspected patients, as well as they were invested in the differential diagnosis between VM and BM. The laboratory abnormalities associated with VM were particularly described in the literature to indicate the biochemistry and cytology of CSF and patients' blood rather than to indicate the virological, serological, or molecular findings. The major biochemical and cytological pictures associated with PVM and PBM are summarized in Table 2. Examination of the patient's CSF for detection of bacterial growth together with the clinical data served as powerful means to differentiate between the two etiological types of meningitis (2). The seasonal occurrence of VM, especially that due to EVs, is another powerful differential element between VM and BM (77–79). The enormous proportion of neutrophils in the CSF is another characteristic feature of BM (58). The most distinguishing laboratory feature of VM is the lymphocytic pleocytosis in the CSF of the infected individuals (76, 103, 134). In PVM, this type of pleocytosis was accompanied by low glucose levels (2, 54, 57, 76). Although VM is typically characterized by a lymphocytic pleocytosis in the CSF, this may not be observed in young children and neonates (76, 143, 160). It was also observed that the laboratory indices associated with PVM were largely dependent on the causative virus. PVM cases caused by EVs were characterized by neutrophil leukocytosis in the CSF, whereas the decline in the glucose levels in the CSF was mainly detected in the PVM due to mumps virus, HSV-1, and LCMV (76). Moreover, the lymphocyte counts and protein concentrations in the CSF of PVM patients were observed to be significantly different between EVs- and VZV-caused PVM (15, 55). This indicates that the determination of the laboratory abnormalities in the CSF and sera of VM patients is not only helpful to differentiate between VM and BM but also helps to judge the causative virus of PVM. However, the application of the PCR (22, 144) and viral isolation (54, 76) from the CSF of the patient to determine the viral etiology of PVM are of utmost importance for a definite diagnosis. Neutrophilic pleocytosis was also detected in some PVM cases, particularly in the EV-related PVM (161, 162). In addition to these types of pleocytosis, monocyte leukocytosis in the CSF of VM patients was also detected in a hospital-based, large retrospective study conducted in France in 2018 (147). In this study, the CSF monocytes level was noted to be higher among VM than BM patients without significant differences. Conclusively, CSF pleocytosis of all types of cells is a cytological feature of PVM, with some reservations to be used for differential diagnosis between PVM and PBM.
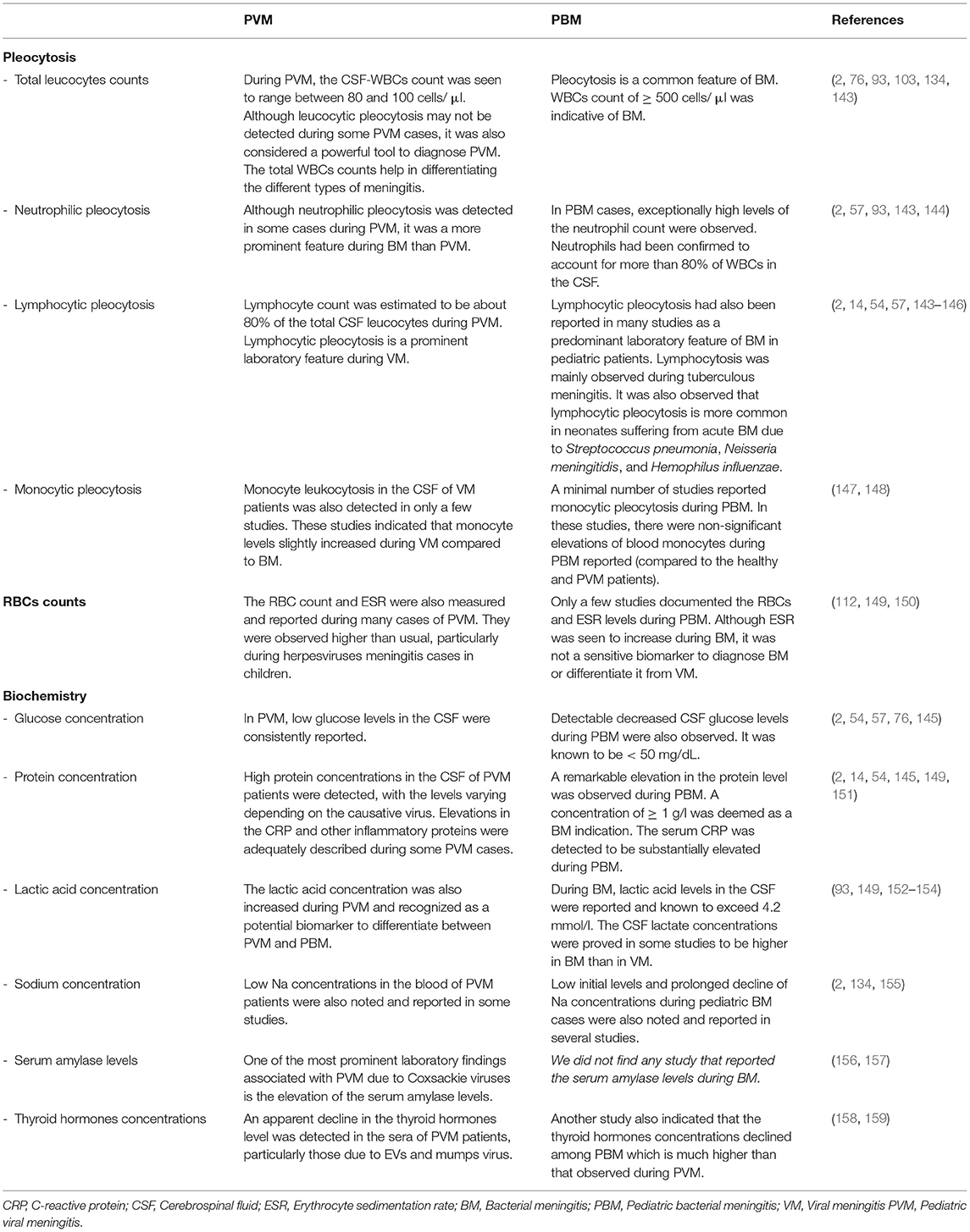
Table 2. Cytology and biochemistry of plasma and cerebrospinal fluids of pediatric viral and bacterial meningitis.
The qualitative C - reactive protein (CRP) was also detected in the sera of some PVM patients, especially in EVs-PVM cases (2, 86). Recently, the blood biomarkers of the PVM due to SARS-CoV-2 were adequately described by DeKosky et al. (151). They have notably recognized an elevation in some inflammatory protein markers in the CSF and plasma of infected individuals. Low sodium concentrations in the blood of PVM patients were also noted and reported in some studies (2, 134). This condition of hyponatremia was explained by the inappropriate antidiuretic hormone (SIADH) syndrome associated with PVM. The RBC count and erythrocyte sedimentation rate (ESR) were also seen to be higher than usual during HSV-1 and HSV-2 PVM cases (112). Additionally, one of the most prominent laboratory features associated with PVM cases is the significant elevation of the serum amylase levels, especially among those caused by Coxsackie A and B viruses (156) and mumps virus (157). This pointed out a possible impairment in the pancreatic functions during these viral infections. This biochemical property of PVM was not proved to have an absolute or differential diagnostic value. An increase in the lactate concentration among meningitis cases was also demonstrated in multiple studies to be higher in BM than in VM patients (149, 152–154) and can therefore be regarded as a robust biomarker to differentiate between PVM and PBM. An endocrine analysis for the thyroid function during PVM caused by EVs and mumps showed a noticeable decline in the thyroid hormones concentrations, namely TSH, T3, FT3, and T4, in the sera of infected children (158). Another similar study among PBM patients showed almost similar results with more decrease in the thyroid hormones during PBM than in PVM cases (159).
Complications and Consequences
Although PVM was well known to be characterized by a benign and rapid course of infection and spontaneous recovery, it was also known to have short and long-term neurological consequences in some cases. These consequences are more likely to occur if bacterial co-infection has taken place. Deaths and severe prolonged neurological disorders due to PVM were described in multiple case reports (86, 87). PVM due to EVs was reported to result in significant levels of mortalities and morbidities, particularly among the neonates and immunocompromised children (29–31). Furthermore, it was also observed that the complications of EVs-related PVM were dependent on the subtype of the virus that causes the disease. For example, EV71 and EV68 subtypes were reported to be responsible for the most severe neurological disorder and outcomes among children's populations (27, 30, 31, 163). The most commonly known fatal complications of EVs-PVM include meningoencephalitis, myocarditis, pericarditis, acute flaccid paralysis, and rhombencephalitis (164–166). In the most severe cases of PVM, fatal conditions of unconsciousness, seizure, and shock were also observed (167). In a recent study, it was shown that the PVM due to HPeV can adversely affect the neurodevelopment in young children resulting in gross-motor functional delay (168). In a long-term retrospective hospital-based study, pathological complications of PVM due to herpes viral infections in neonates and infants were determined. They were seen to include meningeal enhancement, infarct, and subdural collections (169). In addition to the previously-mentioned pathological consequences of PVM, some behavioral complications of PVM were also described in several previous reports. Impairment in the language, sensorineural hearing loss, attention deficit, and hyperactivity disorders were noted among some PVM cases (87). Neuropsychological consequences of VM, such as sleep disturbances, were also observed in some cases (89). In a meta-analysis study, the impacts of PVM on the development and intelligence of children were evaluated. This study concluded that children surviving PVM did not had meaningful deleterious outcomes on their IQ and development as compared to those surviving the PBM (170).
After an intensive search in the literature, we can conclude that the occurrence and severity of the complications due to PVM depend on the causative virus and the age and immune status of the child. It was also evident that factors like the prompt diagnosis, responses of some viruses to the antiviral agents, and the introduction of vaccines to immunize children against the viruses declined the possibility of PVM complications, reducing the hospital durations of stay, and limiting the unnecessary use of antibiotics.
Diagnostic Challenges
In this section of the article, we discuss the indications, contraindications, usefulness, and difficulties associated with the diagnostic techniques of PVM rather than describing them. The preliminary diagnosis of PVM is always made by the physicians via exploring the disease history, focusing on the symptomatology, and applications of the physical examinations. The clinical signs of PVM cases caused by many viral agents are almost similar in their presentations, severity, and frequency of occurrence; hence they were considered unreliable for definite etiological diagnosis. Physical examinations were also employed to diagnose PVM but may not be helpful for differentiation between VM and BM. Kernig's signs and Brudzinski's signs, the bedside physical examinations for meningitis, were routinely used by physicians with high levels of reliability among adult patients, but their validity for the diagnosis of PVM is controversial (17, 94, 95). The major limitation of these physical examinations as a diagnostic tool for PVM is their ability to stretch the inflamed meninges to result in an adequate and detectable irritation (96). Nuchal rigidity signs, as a physical examination test, were also employed by physicians to diagnose PVM, but they did not help to rule in or rule out the disease (18). Jolt accentuation of headache (JAH) is the most recently used type of physical examination to assess the irritation that occurred due to meningitis. JAH was considered positive if the headache from the inflamed meninges increased upon rotating the patient's head horizontally two or three times per second (97, 140). Although reasonable levels of sensitivity and specificity of the JAH to suggest meningitis were reported, it cannot be deemed a reliable test for definitive diagnosis of VM. JAH was recommended in some studies to be used in emergency settings to exclude meningitis (57, 97). Compared with the other physical examinations, the JAH showed better sensitivity and specificity rates for the diagnosis of meningitis (171). Nearly all the reports in the literature addressed the JAH as a diagnostic test among adult meningitis patients. However, only one publication confirmed the effectiveness of the test during PVM cases caused by echovirus 30 (E30) (172). The reliability of the combination of more than one type of these physical examinations was also evaluated in some studies. It had been confirmed that the JAH test could give 100% sensitivity to diagnose meningitis if combined with both Kernig's and Brudzinski's signs (173). Generally, it can be concluded that the classical signs and the physical examination findings of meningitis are not adequate to establish the diagnosis of PVM. As pediatric consultants, two authors of this article believe that these tests for PVM patients can only be looked at as indicators for meningitis and are required for referral to LP for examination of the CSF.
Lumbar puncture had been used for both diagnostic and therapeutic purposes during clinical cases of PVM. For diagnosis, it has been concluded in several studies that LP should always be suggested for diagnosis of pediatric meningitis if the child manifests fever and seizures, particularly if BM is suspected (74, 90, 174). The other important indication of LP is when the patient presents with unconsciousness or altered mental status (175). LP is an invasive procedure thus contraindicated in children, especially if the clinical symptoms and physical examinations provide sufficient information to qualify for an accurate diagnosis of meningitis (90, 174). Some pathological complications of the LP, such as the post-lumbar puncture headache, were also recognized in a myriad of PVM cases (176). When the CSF collected from patients using LP, several laboratory investigations, including bacteriological examinations and biochemistry tests, always carried out purposefully to confirm the infection and to differentiate between BM and VM. These tests include Gram staining and bacterial culture, RBCs counts, WBCs counts, and glucose and protein levels determination. The abnormal cell counts and pathogenic levels of these macromolecules in PVM patients were adequately described and discussed in many previous case reports and review studies (54, 57, 76–78, 93, 97). Bacterial cultures to isolate and identify the causative agent of meningitis, together with the antibiotic sensitivity test, are the proper and applicable diagnostic practice when PBM is suspected (66).
Virus isolation in cell cultures to identify the causative virus of PVM had previously been practiced and discussed in some reports (156, 177, 178). The CSFs were the routinely used samples for virus isolation. However, stool samples were also used especially when EVs infections were suspected. Stool sampling is not an invasive technique, hence considered the most appropriate during the suspicion of PVM (91). The main limitation related to the virus isolation method as a VM diagnostic tool is the poor sensitivity of some cell cultures to propagate certain strains of viruses (177). The sampling time is another significant determinant of the sensitivity of virus isolation as the virus titers in the body fluids may not be to a detectable level. Utilization of serology to identify the viral antigens or the virus-specific antibodies in samples from PVM patients was also made from ancient times up-to-date. The detection of the neutralizing virus-specific IgG and IgM antibodies in the sera of PVM patients was proved valuable for Coxsackie viruses (178). Serology was also used in several epidemiological studies to diagnose PVM due to mumps virus (67), EVs (179, 180), and herpesviruses (181, 182). Despite the usefulness of serological tests to diagnose PVM, false-negative results were obtained particularly in the early phases of the illness (4, 93). Radiological analysis using neuroimaging techniques for clinical cases of PVM was also employed and recommended as a confirmatory diagnosis (67, 183). It had also been documented that neuroimaging was particularly useful during tuberculous PBM (184). Computed tomography (CT) scan also revealed some characteristic clinical outcomes among tuberculous PBM patients, including leptomeningeal enhancement, hydrocephalus, periventricular infarcts, and tuberculoma (183, 184). Generally, neuroimaging was proved of little diagnostic value for PVM in circumstances other than confirmatory purposes because non-specific findings were obtained. However, recently the MRI technique was used to elucidate features that can distinguish meningitis of viral and non-viral etiologies (185).
All of the above-mentioned diagnostic tools for PVM were confirmed to be characterized by relatively poor sensitivity levels and non-specific nature until the different molecular tests were introduced. Undoubtedly, PCR showed superiority over all approaches as the most definitive test to diagnose VM, hence nominated as the gold standard test (22, 93). Reverse-transcription PCR (RT-PCR) to detect the viral nucleic acid in the CSF of PVM patients revealed a 100% sensitivity rate and proved far superior to the virus isolation technique in both EVs- and HPeV-positives cases of PVM (23, 24). Multiplex PCR is now frequently used in clinical and epidemiological investigations to identify the causative virus in PVM patients (162, 186–188). Additionally, approval of some molecular diagnostic kits, such as FilmArray® BioFire® Meningitis/Encephalitis (ME) panel, have significantly reduced the time for detection of the viral etiology of PVM (188, 189). A particular point of concern for the use of PCR during EVs-caused PVM was raised, which indicated that the test is superior to serology as it is significantly helpful for the diagnosticians to differentiate between the various serotypes of EVs causing the disease (76). Conclusively, the PCR is a practical test to identify the exact viral etiology of PVM, which helped to reduce the time of detection, limit the use of unnecessary antimicrobial drugs, and shorten the duration of hospital stay. Other molecular tests, including next-generation sequencing (NGS) and metagenomic next-generation sequencing (mNGS), were also used in numerous studies to diagnose infectious meningitis (26, 190, 191). The usefulness of mNGS enables identifying a wide range of pathogenic organisms in a single assay, including viral, bacterial, fungal, and parasitic causes of meningitis (192, 193). Additionally, the mNGS approach was proved highly useful as it can not only detect the causative virus but can further determine the viral genotype in the clinical specimens (194).
Conclusions
Following the subtle systematic search in the literature for the various aspects of PVM, the following conclusive points can be highlighted:
• Various viral etiologies for PVM were recognized. However, EVs were the first and most commonly reported causes of PVM. The seasonal occurrence of PVM in specific geographical locations of the globe was found to be associated with the arboviruses' infections. The incidences of PVM due to some viruses were drastically declined after the introduction and application of vaccines.
• PVM cases were more prevalent worldwide but clinically less severe and manifest with benign presentation and spontaneous recovery among children as compared to PBM. They were seen only critical and fatal among newborns, infants, and immunocompromised children.
• As retrieved from the previously published studies, the epidemiological correlates of PVM are mainly dependent on the causative virus and the age of the child.
• The laboratory abnormalities due to PVM were described in plenty of clinical studies. These had mainly focused on the biochemistry and cytology of CSF and blood of PVM patients.
• The pathological, developmental, behavioral, and neuropsychological complications of PVM were adequately reviewed and discussed in this report. Deaths, seizures, shock as well as severe and long-lasting neurological disorders were reported.
• Throughout the history of PVM, the circumstantial, clinical, virological, and molecular tests were utilized as diagnostics to reach the etiology of the disease. However, PCR and the other molecular tests revealed the highest sensitivity rates.
Author Contributions
All authors listed have made a substantial, direct, and intellectual contribution to the work and approved it for publication.
Funding
The authors extend their appreciation to the Deanship of Scientific Research at King Khalid University (KKU), Saudi Arabia, for funding this research project; Grant Code R.G.P.1/234/43.
Conflict of Interest
The authors declare that the research was conducted in the absence of any commercial or financial relationships that could be construed as a potential conflict of interest.
Publisher's Note
All claims expressed in this article are solely those of the authors and do not necessarily represent those of their affiliated organizations, or those of the publisher, the editors and the reviewers. Any product that may be evaluated in this article, or claim that may be made by its manufacturer, is not guaranteed or endorsed by the publisher.
References
2. Hoffman O, Weber JR. Pathophysiology and treatment of bacterial meningitis. Ther Adv Neurol Disord. (2009) 2:401–12. doi: 10.1177/1756285609337975
3. Booss J Tselis Tselis AC (2014) Chapter 1—a history of viral infections of the central nervous system: foundations milestones and patterns. In: Tselis AC, Booss J, editors. Handbook of Clinical Neurology. Elsevier. pp 3–44.
4. Kumar R. Aseptic meningitis: diagnosis and management. Indian J Pediatr. (2005) 72:57–63. doi: 10.1007/BF02760582
5. Desmond RA, Accortt NA, Talley L, Villano SA, Soong SJ, Whitley RJ. Enteroviral meningitis: natural history and outcome of pleconaril therapy. Antimicrob Agents Chemother. (2006) 50:2409–14. doi: 10.1128/AAC.00227-06
6. McGill F, Griffiths MJ, Bonnett LJ, Geretti AM, Michael BD, Beeching NJ, et al. Incidence, aetiology, and sequelae of viral meningitis in UK adults: a multicentre prospective observational cohort study. Lancet Infect Dis. (2018) 18:992–1003. doi: 10.1016/S1473-3099(18)30245-7
7. Hviid A, Melbye M. The epidemiology of viral meningitis hospitalization in childhood. Epidemiology. (2007) 18:695–701. doi: 10.1097/EDE.0b013e3181567d31
8. Yousefi K, Poorbarat S, Abasi Z, Rahimi S, Khakshour A. Viral meningitis associated with COVID-19 in a 9-year-old child: a case report. Pediatr Infect Dis J. (2021) 40:e87–98. doi: 10.1097/INF.0000000000002979
9. Smuts H, Cronje S, Thomas J, Brink D, Korsman S, Hardie D. Molecular characterization of an outbreak of enterovirus-associated meningitis in Mossel Bay, South Africa, December 2015-January 2016. BMC Infect Dis. (2018) 18:709–709. doi: 10.1186/s12879-018-3641-4
10. Dash N, Ameen AS, Sheek-Hussein MM, Smego RA Jr. Epidemiology of meningitis in Al-Ain, United Arab Emirates, 2000–2005. Int J Infect Dis. (2007) 11:309–12. doi: 10.1016/j.ijid.2006.05.009
11. Dalwai A, Ahmad S, Al-Nakib W. Echoviruses are a major cause of aseptic meningitis in infants and young children in Kuwait. Virol J. (2010) 7:236. doi: 10.1186/1743-422X-7-236
12. Ben Abid F, Abukhattab M, Ghazouani H, Khalil O, Gohar A, Al Soub H, et al. Epidemiology and clinical outcomes of viral central nervous system infections. Int J Infect Dis. (2018) 73:85–90. doi: 10.1016/j.ijid.2018.06.008
13. Alzein K. Revision of meningitis surveillance system in Jordan during 2001 and 2014 years. Epidemiol (sunnyvale). (2016) 6:220. doi: 10.4172/2161-1165.1000220
14. Dumaidi K, Al-Jawabreh A. Molecular detection and genotyping of enteroviruses from CSF samples of patients with suspected sepsis-like illness and/or aseptic meningitis from 2012 to 2015 in West Bank, Palestine. PLoS ONE. (2017) 12:e0172357–e0172357. doi: 10.1371/journal.pone.0172357
15. Haydar SM, Hallit SR, Hallit RR, Salameh PR, Faddoul LJ, Chahine BA, et al. Adherence to international guidelines for the treatment of meningitis infections in Lebanon. Saudi Med J. (2019) 40:260–65. doi: 10.15537/smj.2019.3.23965
16. Visintin C, Mugglestone MA, Fields EJ, Jacklin P, Murphy MS, Pollard AJ. Management of bacterial meningitis and meningococcal septicaemia in children and young people: summary of NICE guidance. BMJ. (2010) 340:c3209. doi: 10.1136/bmj.c3209
17. Mehndiratta M, Nayak R, Garg H, Kumar M, Pandey S, et al. Appraisal of Kernig's and Brudzinski's sign in meningitis. Ann Indian Acad Neurol. (2012) 15:287. doi: 10.4103/0972-2327.104337
18. Waghdhare S, Kalantri A, Joshi R, Kalantri S. Accuracy of physical signs for detecting meningitis: a hospital-based diagnostic accuracy study. Clin Neurol Neurosurg. (2010) 112:752–57. doi: 10.1016/j.clineuro.2010.06.003
19. Parikh V, Tucci V, Galwankar S. Infections of the nervous system. Int J Crit Illn Inj Sci. (2012) 2:82. doi: 10.4103/2229-5151.97273
20. Irani DN. Aseptic meningitis and viral myelitis. Neurol Clin. (2008) 26:635. doi: 10.1016/j.ncl.2008.03.003
21. Shukla B, Aguilera EA, Salazar L, Wootton SH, Kaewpoowat Q, Hasbun R. Aseptic meningitis in adults and children: diagnostic and management challenges. J Clin Virol. (2017) 94:110–14. doi: 10.1016/j.jcv.2017.07.016
22. Verstrepen WA, Kuhn S, Kockx MM, Van De Vyvere ME, Mertens AH. Rapid detection of enterovirus RNA in cerebrospinal fluid specimens with a novel single-tube real-time reverse transcription-PCR assay. J Clin Microbiol. (2001) 39:4093–96. doi: 10.1128/JCM.39.11.4093-4096.2001
23. de Crom SC, Obihara CC, de Moor RA, Veldkamp EJ, van Furth AM, Rossen JW. Prospective comparison of the detection rates of human enterovirus and parechovirus RT-qPCR and viral culture in different pediatric specimens. J Clin Virol. (2013) 58:449–54. doi: 10.1016/j.jcv.2013.07.017
24. Alhazmi A, Lazrek M, Alidjinou EK, Engelmann I, Schuffenecker I, Dubos F, et al. Repeated viral meningitis in a newborn. J Neurovirol. (2020) 26:449–51. doi: 10.1007/s13365-020-00829-0
25. Kupila L, Vuorinen T, Vainionpäā R, Marttila RJ, Kotilainen P. Diagnosis of enteroviral meningitis by use of polymerase chain reaction of cerebrospinal fluid, stool, and serum specimens. Clin Infect Dis. (2005) 40:982–87. doi: 10.1086/428581
26. Wilson MR, Sample HA, Zorn KC, Arevalo S, Yu G, Neuhaus J, et al. Clinical metagenomic sequencing for diagnosis of meningitis and encephalitis. N Engl J Med. (2019) 380:2327–40. doi: 10.1056/NEJMoa1803396
27. Rotbart HA. Enteroviral infections of the central nervous system. Clin Infect Dis. (1995) 20:971981. doi: 10.1093/clinids/20.4.971
28. Lee BE, Davies HD. Aseptic meningitis. Curr Opin Infect Dis. (2007) 20:272–7. doi: 10.1097/QCO.0b013e3280ad4672
29. Cui A, Yu D, Zhu Z, Meng L, Li H, Liu, et al. An outbreak of aseptic meningitis caused by coxsackievirus A9 in Gansu, the People's Republic of China. Virol J. (2010) 7:72. doi: 10.1186/1743-422X-7-72
30. González-Sanz R, Taravillo I, Reina J, Navascués A, Moreno-Docón A, Aranzamendi M, et al. Enterovirus D68-associated respiratory and neurological illness in Spain, 2014–2018. Emerg Microbes Infect. (2019) 8:1438–44. doi: 10.1080/22221751.2019.1668243
31. Esposito S, Bosis S, Niesters H, Principi N. Enterovirus D68 infection. Viruses. (2015) 7:6043–50. doi: 10.3390/v7112925
32. Wieczorek M, Figas A, Krzysztoszek A. Enteroviruses associated with aseptic meningitis in Poland, 2011–2014. Pol J Microbiol. (2016) 65:231–5. doi: 10.5604/17331331.1204485
33. Farshadpour F, Taherkhani R. Molecular epidemiology of enteroviruses and predominance of echovirus 30 in an Iranian population with aseptic meningitis. J Neurovirol. (2021) 27:444–51. doi: 10.1007/s13365-021-00973-1
34. Ramalho E, Sousa I Jr, Burlandy F, Costa E, Dias A, Serrano R, et al. Identification and phylogenetic characterization of human enteroviruses Isolated from cases of aseptic meningitis in Brazil, 2013-2017. Viruses. (2019) 11:690. doi: 10.3390/v11080690
35. Johannessen IBurns SM, et al. Picornaviruses: meningitis; paralysis; rashes; intercostal myositis; myocarditis; infectious hepatitis; common cold. In: Greenwood D, et al., editors. Medical Microbiology. 18th edn. Edinburgh: Churchill Livingstone. (2012). pp. 483–496.
36. Wolthers KC, Benschop KS, Schinkel J, Molenkamp R, Bergevoet RM, Spijkerman IJ, et al. Human parechoviruses as an important viral cause of sepsislike illness and meningitis in young children. Clin Infect Dis. (2008) 47:358–63. doi: 10.1086/589752
37. Spear PG, Longnecker R. Herpesvirus entry: an update. J Virol. (2003) 77:10179–85. doi: 10.1128/JVI.77.19.10179-10185.2003
38. Bergström T, Vahlne A, Alestig K, Jeansson S, Forsgren M, Lycke E. Primary and recurrent herpes simplex virus type 2-induced meningitis. J Infect Dis. (1990) 162:322–30. doi: 10.1093/infdis/162.2.322
39. Whitley RJ, Lakeman F. Herpes simplex virus infections of the central nervous system: therapeutic and diagnostic considerations. Clin Infect Dis. (1995) 20:414–20. doi: 10.1093/clinids/20.2.414
40. Rosenberg J, Galen BT. Recurrent Meningitis. Curr Pain Headache Rep. (2017) 21:33. doi: 10.1007/s11916-017-0635-7
41. Kleinschmidt-DeMasters BK, Gilden DH. The expanding spectrum of herpesvirus infections of the nervous system. Brain Pathol. (2001) 11:440–51. doi: 10.1111/j.1750-3639.2001.tb00413.x
42. Nahdi I, Boukoum H, Nabil Ben Salem A, Ben Romdane F, Hammami S, Chebel S, et al. Detection of herpes simplex virus (1 and 2), varicella-zoster virus, cytomegalovirus, human herpesvirus 6 and enterovirus in immunocompetent Tunisian patients with acute neuromeningeal disorder. J Med Virol. (2012) 84:282–9. doi: 10.1002/jmv.23192
43. Ansari A, Li S, Abzug MJ, Weinberg A. Human herpesviruses 6 and 7 and central nervous system infection in children. Emerg Infect Dis. (2004) 10:1450–4. doi: 10.3201/eid1008.030788
44. Kim F, Reichman V, Hooven TA. Human Herpesvirus-6 meningitis in a premature infant with fevers: a case and literature review. Clin Med Insights Case Rep. (2020) 13:1179547620912952. doi: 10.1177/1179547620912952
45. Liang CY, Yang CH, Lin JN. Focal encephalitis, meningitis, and acute respiratory distress syndrome associated with influenza A infection. Med Princ Pract. (2018) 27:193–6. doi: 10.1159/000487398
46. Yamada M, Bingham J, Payne J, Rookes J, Lowther S, Haining J, et al. Multiple routes of invasion of wild-type Clade 1 highly pathogenic avian influenza H5N1 virus into the central nervous system (CNS) after intranasal exposure in ferrets. Acta Neuropathol. (2012) 124:505–16. doi: 10.1007/s00401-012-1010-8
47. Siegers JY, van de Bildt MWG, Lin Z, Leijten LM, Lavrijssen RAM, Bestebroer T, et al. Viral factors important for efficient replication of influenza A viruses in cells of the central nervous system. J Virol. (2019) 93:e02273–318. doi: 10.1128/JVI.02273-18
48. Wang YH, Huang YC, Chang LY, Kao HT, Lin PY, Huang CG, et al. Clinical characteristics of children with influenza A virus infection requiring hospitalization. J Microbiol Immunol Infect. (2003) 36:111–6.
49. Wilking AN, Elliott E, Garcia MN, Murray KO, Munoz FM. Central nervous system manifestations in pediatric patients with influenza A H1N1 infection during the 2009 pandemic. Pediatr Neurol. (2014) 51:370–6. doi: 10.1016/j.pediatrneurol.2014.04.026
50. Macias AE, McElhaney JE, Chaves SS, Nealon J, Nunes MC, Samson SI, et al. The disease burden of influenza beyond respiratory illness. Vaccine. (2021) 39 (Suppl 1):A6–A14. doi: 10.1016/j.vaccine.2020.09.048
51. Makras P, Alexiou-Daniel S, Antoniadis A, Hatzigeorgiou D. Outbreak of meningococcal disease after an influenza B epidemic at a Hellenic Air Force recruit training center. Clin Infect Dis. (2001) 33:e48–50. doi: 10.1086/322609
52. Moon JH, Na JY, Kim JH, Yum MK, Oh JW, Kim CR, et al. Neurological and muscular manifestations associated with influenza B infection in children. Pediatr Neurol. (2013) 49:97–101. doi: 10.1016/j.pediatrneurol.2013.04.004
54. Han SH, Choi HY, Kim JM, Park KR, Youn YC, Shin HW. Etiology of aseptic meningitis and clinical characteristics in immune-competent adults. J Med Virol. (2016) 88:175–9. doi: 10.1002/jmv.24316
55. Miller BR. Arboviruses. In: Mahy BWJ, Van Regenmortel MHV, editors. Encyclopedia of Virology. 3rd edn. Oxford: Academic Press. (2008). pp. 170–176.
56. Kuwayama M, Ito M, Takao S, Shimazu Y, Fukuda S, Miyazaki K, et al. Japanese encephalitis virus in meningitis patients, Japan. Emerg Infect Dis. (2005) 11:471–3. doi: 10.3201/eid1103.040285
57. de Oliveira DB, Candiani TM, Franco-Luiz APM, Almeida GMF, Abrahão JS, Rios M, et al. Etiological agents of viral meningitis in children from a dengue-endemic area, Southeast region of Brazil. J Neurol Sci. (2017) 375:390–4. doi: 10.1016/j.jns.2017.02.025
58. Calisher CH, Karabatsos N, Foster JP, Pallansch M, Roehrig JT. Identification of an antigenic subtype of eastern equine encephalitis virus isolated from a human. J Clin Microbiol. (1990) 28:373–4. doi: 10.1128/jcm.28.2.373-374.1990
59. Gaensbauer JT, Lindsey NP, Messacar K, Staples JE, Fischer M. Neuroinvasive arboviral disease in the United States: 2003 to 2012. Pediatrics. (2014) 134:e642–50. doi: 10.1542/peds.2014-0498
60. Mac Donald-Ottevanger MS, Gravenberch-Ramnandanlall CI, Zijlmans CW. Chikungunya bij kinderen [Chikungunya in children]. Ned Tijdschr Geneeskd. (2015) 159:A8403. Dutch.
61. Samra JA, Hagood NL, Summer A, Medina MT, Holden KR. Clinical Features and Neurologic Complications of Children Hospitalized With Chikungunya Virus in Honduras. J Child Neurol. (2017) 32:712–16. doi: 10.1177/0883073817701879
62. Vahey GM, Lindsey NP, Staples JE, Hills SL. La Crosse Virus Disease in the United States, 2003-2019. Am J Trop Med Hyg. (2021) 105:807–12. doi: 10.4269/ajtmh.21-0294
64. Deibel R, Woodall JP, Decher WJ, Schryver GD. Lymphocytic choriomeningitis virus in man. Serologic evidence of association with pet hamsters. JAMA. (1975) 232:501–4. doi: 10.1001/jama.1975.03250050023010
65. Ackermann R. Gefährdung des Menschen durch LCM-Virus-verseuchte Goldhamster. Serologische Untersuchungen [Risk to humans through contact with golden hamsters carrying lymphocytic choriomeningitis virus (author's transl)]. Dtsch Med Wochenschr. (1977) 102:1367–70. German. doi: 10.1055/s-0028-1106727
66. Davis LE. Acute Bacterial Meningitis. Continuum (Minneap Minn). (2018) 24(5, Neuroinfectious Disease):1264–83. doi: 10.1212/CON.0000000000000660
67. Daniel BD, Grace GA, Natrajan M. Tuberculous meningitis in children: clinical management & outcome. Indian J Med Res. (2019) 150:117–30. doi: 10.4103/ijmr.IJMR_786_17
68. Oordt-Speets AM, Bolijn R, van Hoorn RC, Bhavsar A, Kyaw MH. Global etiology of bacterial meningitis: a systematic review and meta-analysis. PLoS ONE. (2018) 13:e0198772. doi: 10.1371/journal.pone.0198772
69. Guet-Revillet H, Levy C, Andriantahina I, Kalach N, Pierre MH, Elbez-Rubinstein A, et al. Paediatric epidemiology of Pasteurella multocida meningitis in France and review of the literature. Eur J Clin Microbiol Infect Dis. (2013) 32:1111–20. doi: 10.1007/s10096-013-1866-0
71. Wilfert CM, Lehrman SN, Katz SL. Enteroviruses and meningitis. Pediatr Infect Dis. (1983) 2:333–41. doi: 10.1097/00006454-198307000-00019
72. Singhi S, Singhi P, Baranwal AK. Bacterial meningitis in children: critical care needs. Indian J Pediatr. (2001) 68:737–47. doi: 10.1007/BF03191900
73. Mathew S, Al Khatib HA, Al Ansari K, Nader J, Nasrallah GK, Younes NN, et al. Epidemiology Profile of Viral Meningitis Infections Among Patients in Qatar 2015-2018. Front Med (Lausanne). (2021) 8:663694. doi: 10.3389/fmed.2021.663694
74. Macy ML, Hall M, Shah SS, Harding JP, Del Beccaro MA, Hain PD, et al. Pediatric observation status: are we overlooking a growing population in children's hospitals? J Hosp Med. (2012) 7:530–6. doi: 10.1002/jhm.1923
75. Laditan AA, Odame I, Ogundipe O. Childhood meningitis at King Fahad Hospital, Hofuf, Saudi Arabia. Ann Saudi Med. (1997) 17:605–8. doi: 10.5144/0256-4947.1997.605
76. Ratzan KR. Viral meningitis. Med Clin North Am. (1985) 69:399–413. doi: 10.1016/S0025-7125(16)31051-3
77. Rhoades RE, Tabor-Godwin JM, Tsueng G, Feuer R. Enterovirus infections of the central nervous system. Virology. (2011) 411:288–305. doi: 10.1016/j.virol.2010.12.014
78. Berangi Z, Karami M, Mohammadi Y, Nazarzadeh M, Zahraei SM, Javidrad H, et al. Epidemiological profile of meningitis in Iran before pentavalent vaccine introduction. BMC Pediatrics. (2019) 19:370. doi: 10.1186/s12887-019-1741-y
79. Melnick JL. Enteroviruses: polioviruses, coxsackieviruses, echoviruses, and newer enteroviruses. In: Fields BN, Knipe DM, Howley PM, editors. Fields Virology. 3rd edn. New York: Lippincott-Raven Press. (1996). pp. 655–712.
80. Strikas RA, Anderson LJ, Parker RA. Temporal and geographic patterns of isolates of nonpolio enterovirus in the United States, 1970–1983. J Infect Dis. (1986) 153:346–351. doi: 10.1093/infdis/153.2.346
81. Posnakoglou L, Siahanidou T, Syriopoulou V, Michos A. Impact of cerebrospinal fluid syndromic testing in the management of children with suspected central nervous system infection. Eur J Clin Microbiol Infect Dis. (2020) 39:2379–86. doi: 10.1007/s10096-020-03986-6
82. Rantakallio P, Leskinen M, von Wendt L. Incidence and prognosis of central nervous system infections in a birth cohort of 12,000 children. Scand J Infect Dis. (1986) 18:287–94. doi: 10.3109/00365548609032339
83. Kim BG, Jang MS, Kim J. Epidemiology of Pediatric Meningitis in South Korea From 2010 to 2018: a population-based Retrospective Cohort Study. Pediatr Infect Dis J. (2021) 40:885–91. doi: 10.1097/INF.0000000000003190
84. Khetsuriani N, Quiroz ES, Holman RC, Anderson LJ. Viral meningitis-associated hospitalizations in the United States, 1988-1999. Neuroepidemiology. (2003) 22:345–52. doi: 10.1159/000072924
85. Chávez-Bueno S, McCracken GH Jr. Bacterial meningitis in children. Pediatr Clin North Am. (2005) 52:795–810, vii. doi: 10.1016/j.pcl.2005.02.011
86. Abzug MJ, Levin MJ, Rotbart HA. Profile of enterovirus disease in the first two weeks of life. Pediatr Infect Dis J. (1993) 12:820–24. doi: 10.1097/00006454-199310000-00005
87. Odetola FO, Bratton SL. Characteristics and immediate outcome of childhood meningitis treated in the pediatric intensive care unit. Intensive Care Med. (2005) 31:92–7. doi: 10.1007/s00134-004-2501-4
88. Green SM, Rothrock SG, Clem KJ, Zurcher RF, Mellick L. Can seizures be the sole manifestation of meningitis in febrile children? Pediatrics. (1993) 92:527–34. doi: 10.1542/peds.92.4.527
89. Schmidt H, Cohrs S, Heinemann T, Goerdt C, Djukic M, Heimann B, et al. Sleep disorders are long-term sequelae of both bacterial and viral meningitis. J Neurol Neurosurg Psychiatry. (2006) 77:554–8. doi: 10.1136/jnnp.2005.071142
90. Lorber J, Sunderland R. Lumbar puncture in children with convulsions associated with fever. Lancet. (1980) 1:785–6. doi: 10.1016/S0140-6736(80)91290-8
91. Reinheimer C, Rabenau H, Berger A, Doerr HW. Diagnostic of neurotropic enteroviruses in children with CSF and/or stool: virus isolation by cell culture or PCR?. Klin Padiatr. (2011) 223:221–6. German. doi: 10.1055/s-0031-1273725
92. Baud O, Aujard Y. Neonatal bacterial meningitis. Handb Clin Neurol. (2013)112:1109–13. doi: 10.1016/B978-0-444-52910-7.00030-1
93. Kohil A, Jemmieh S, Smatti MK, Yassine HM. Viral meningitis: an overview. Arch Virol. (2021) 166:335–45. doi: 10.1007/s00705-020-04891-1
94. Babu TA. Improved sensitivity of Kernig's and Brudzinski's sign in diagnosing meningitis in children. Ann Indian Acad Neurol. (2013) 16:460–1. doi: 10.4103/0972-2327.116954
95. Dijcks R, Derks M, Verwijnen M, de Jongh TO. Meningeale prikkeling [Meningeal irritation]. Ned Tijdschr Geneeskd. (2011) 155:A2661. Dutch.
96. Tracy A, Waterfield T. How to use clinical signs of meningitis. Arch Dis Child Educ Pract Ed. (2020) 105:46–9. doi: 10.1136/archdischild-2019-317037
97. Iguchi M, Noguchi Y, Yamamoto S, Tanaka Y, Tsujimoto H. Diagnostic test accuracy of jolt accentuation for headache in acute meningitis in the emergency setting. Cochrane Database Syst Rev. (2020) 6:CD012824. doi: 10.1002/14651858.CD012824.pub2
98. Richtsmeier JT, Flaherty K. Hand in glove: brain and skull in development and dysmorphogenesis. Acta Neuropathol. (2013) 125:469–89. doi: 10.1007/s00401-013-1104-y
99. Adeeb N, Mortazavi MM, Tubbs RS, Cohen-Gadol AA. The cranial dura mater: a review of its history, embryology, and anatomy. Childs Nerv Syst. (2012) 28:827–37. doi: 10.1007/s00381-012-1744-6
100. Siegenthaler JA, Pleasure SJ. We have got you ‘covered': how the meninges control brain development. Curr Opin Genet Dev. (2011) 21:249–55. doi: 10.1016/j.gde.2010.12.005
101. Wakhlu I, Bajpai PC, Khan AM. Septic meningitis. Indian J Pediatr. (1970) 37:609–11. doi: 10.1007/BF02811179
102. Kalra K, Dayal RS. Purulent meningitis in infancy and childhood. Indian J Pediatr. (1977) 44:65–70. doi: 10.1007/BF02753628
103. Wright WF, Pinto CN, Palisoc K, Baghli S. Viral (aseptic) meningitis: A review. J Neurol Sci. (2019) 398:176–83. doi: 10.1016/j.jns.2019.01.050
104. Nikonov OS, Chernykh ES, Garber MB, Nikonova EY. Enteroviruses: Classification, Diseases They Cause, and Approaches to Development of Antiviral Drugs. Biochemistry (Mosc). (2017) 82:1615–31. doi: 10.1134/S0006297917130041
105. Adams MJ, Lefkowitz EJ, King AMQ, Carstens EB. Ratification vote on taxonomic pro posals to the International Committee on Taxonomy of Viruses. Arch Virol. (2014) 159:28312841. doi: 10.1007/s00705-014-2114-3
106. Adams MJ, Lefkowitz EJ, King AM, Bamford DH, Breitbart M, Davison AJ, et al. Ratification vote on taxonomic proposals to the International Committee on Taxonomy of Viruses. Arch Virol. (2015) 160:1837–50. doi: 10.1007/s00705-015-2425-z
107. Adams MJ, Lefkowitz EJ, King AM, Harrach B, Harrison RL, Knowles NJ, et al. Ratification vote on taxonomic proposals to the International Committee on Taxonomy of Viruses (2016). Arch Virol. (2016) 161:2921–49. doi: 10.1007/s00705-016-2977-6
108. Mclean DM, Quantz EJ, Bach RD, Pevzner BM, Larke RP, Mcnaughton GA. Enteroviral and mumps meningitis in TORONTO, 1963. Can Med Assoc J. (1964) 90:1390–3.
109. Shaker OG, Abdelhamid N. Detection of enteroviruses in pediatric patients with aseptic meningitis. Clin Neurol Neurosurg. (2015) 129:67–71. doi: 10.1016/j.clineuro.2014.12.004
110. Ai J, Xie Z, Liu G, Chen Z, Yang Y, Li Y et al. Etiology and prognosis of acute viral encephalitis and meningitis in Chinese children: a multicentre prospective study. BMC Infect Dis. (2017) 17:494. doi: 10.1186/s12879-017-2572-9
111. Rozenberg F. Herpes simplex virus and central nervous system infections: encephalitis, meningitis, myelitis. Virologie (Montrouge). (2020) 24:283–94. doi: 10.1684/vir.2020.0862
112. Azadfar S, Cheraghali F, Moradi A, Javid N, Tabarraei A. Herpes simplex virus meningitis in children in South East of caspian sea, iran. Jundishapur J Microbiol. (2014) 7:e8599. doi: 10.5812/jjm.8599
113. Kanamori K, Shoji K, Kinoshita N, Ishiguro A, Miyairi I. Complications of herpes zoster in children. Pediatr Int. (2019) 61:1216–20. doi: 10.1111/ped.14025
114. Moghadam AG, Yousefi E, Ghatie MA, Moghadam AG, Pouladfar GR, Jamalidoust M. Investigating the etiologic agents of aseptic meningitis outbreak in Iranian children. J Fam Med Prim Care. (2020) 9:1573–7. doi: 10.4103/jfmpc.jfmpc_1003_19
115. Heusel EH, Grose C. Twelve Children with Varicella Vaccine Meningitis: Neuropathogenesis of Reactivated Live Attenuated Varicella Vaccine Virus. Viruses. (2020) 12:1078. doi: 10.3390/v12101078
116. Vyas S, Suthar R, Bhatia V, Bhardwaj N, Aggarwal R, Singhi P, et al. Brain MRI in Epstein-Barr Virus Meningoencephalitis in Children. Ann Indian Acad Neurol. (2020) 23:621–4. doi: 10.4103/aian.AIAN_537_19
117. Cheng H, Chen D, Peng X, Wu P, Jiang L, Hu Y. Clinical characteristics of Epstein-Barr virus infection in the pediatric nervous system. BMC Infect Dis. (2020) 20:886. doi: 10.1186/s12879-020-05623-1
118. Dyachenko P, Smiianova O, Kurhanskaya V, Oleshko A, Dyachenko A. Epstein-Barr virus-associated encephalitis in a case-series of more than 40 patients. Wiad Lek. (2018) 71:1224–30.
119. Xia JB, Zhu J, Hu J, Wang LM, Zhang H. H7N9 influenza A-induced pneumonia associated with acute myelitis in an adult. Intern Med. (2014) 53:1093–5. doi: 10.2169/internalmedicine.53.1801
120. Lim SM, Koraka P, Osterhaus AD, Martina BE. West Nile virus: immunity and pathogenesis. Viruses. (2011) 3:811–28. doi: 10.3390/v3060811
121. Di Pietrantonj C, Rivetti A, Marchione P, Debalini MG, Demicheli V. Vaccines for measles, mumps, rubella, and varicella in children. Cochrane Database Syst Rev. (2020) 4:CD004407. doi: 10.1002/14651858.CD004407.pub4
122. Jorquera LA, Ugarte CD, Avilés LC, Delpiano M L. Aseptic meningitis due to mumps vaccine. Case report and review of the literature. Rev Chilena Infectol. (2020) 37:769–74. Spanish. doi: 10.4067/S0716-10182020000600769
123. Contreras J, Reyes P, Cortés CP. Meningitis aguda posterior a vacuna tresvírica [Acute meningitis following mumps vaccine]. Rev Chilena Infectol. (2019) 36:774–7. Spanish. doi: 10.4067/S0716-10182019000600774
124. Bonnet MC, Dutta A, Weinberger C, Plotkin SA. Mumps vaccine virus strains and aseptic meningitis. Vaccine. (2006) 24:7037–45. doi: 10.1016/j.vaccine.2006.06.049
125. Macartney K, Gidding HF, Trinh L, Wang H, Dey A, Hull B, et al. Paediatric Active Enhanced Disease Surveillance Network. Evaluation of combination measles-mumps-rubella-varicella vaccine introduction in Australia. JAMA Pediatr. (2017) 171:992–8. doi: 10.1001/jamapediatrics.2017.1965
126. Karande S, Gupta V, Kulkarni M, Joshi A, Rele M. Tuberculous meningitis and HIV. Indian J Pediatr. (2005) 72:755–60. doi: 10.1007/BF02734147
127. Otto SBJ, George PE, Mercedes R, Nabukeera-Barungi N. Cryptococcal meningitis and immune reconstitution inflammatory syndrome in a pediatric patient with HIV after switching to second line antiretroviral therapy: a case report. BMC Infect Dis. (2020) 20:68. doi: 10.1186/s12879-020-4797-2
128. Wiberg K, Birnbaum A, Gradon J. Causes and presentation of meningitis in a Baltimore community hospital 1997–2006. South Med J. (2008) 101:1012–16. doi: 10.1097/SMJ.0b013e31817e3544
129. Romero JR, Newland JG. Viral meningitis and encephalitis: traditional and emerging viral agents. Semin Pediatr Infect Dis. (2003) 14:72–82. doi: 10.1053/spid.2003.127223
130. Padda I, Khehra N, Jaferi U, Parmar MS. The neurological complexities and prognosis of COVID-19. SN Compr Clin Med. (2020) 29:1–12. doi: 10.1007/s42399-020-00527-2
131. Asseri AA, Alzaydani I, Al-Jarie A, Albishri A, Alsabaani A, Almaghrabi MK, et al. Clinical characteristics and laboratory abnormalities of hospitalized and critically Ill children with coronavirus disease 2019: a retrospective study from Saudi Arabia. Int J Gen Med. (2021) 14:1949–58. doi: 10.2147/IJGM.S311831
132. Stoffel L, Agyeman PKA, Keitel K, Barbani MT, Duppenthaler A, Kopp MV, et al. Striking decrease of enteroviral meningitis in children during the COVID-19 pandemic. Open Forum Infect Dis. (2021) 8:ofab115. doi: 10.1093/ofid/ofab115
133. Lee HH, Lin SH. Effects of COVID-19 prevention measures on other common infections, Taiwan. Emerg Infect Dis. (2020) 26:2509–11. doi: 10.3201/eid2610.203193
134. Ghabouli Shahroodi MJ, Ghazvini K, Sadeghi R, Sasan MS. Enteroviral meningitis in neonates and children of Mashhad, Iran. Jundishapur J Microbiol. (2016) 9:e19955. doi: 10.5812/jjm.19955
135. Kernig VM. Ueber ein kankheits symptom der acuten meningitis. St Petersburgh Medizinische Woschenschrift. (1882) 7:398.
136. Verghese A, Gallemore G. Kernig's and Brudzinski's signs revisited. Rev Infect Dis. (1987) 9:1187–92. doi: 10.1093/clinids/9.6.1187
137. Brudzinski J. Ueber die Kontralateralen Reflexe an den Unteren Extremitaeten bei Kindern. Wien Klin Wschr. (1908) 21:255–61.
138. Brudzinski J. Un signe nouveau sur les membres inferieurs dans les meningites chez les enfants (signe de la nuque) Arch Med Enf . (1909) 12:745–52.
139. Fitch M. T. Central nervous system and spinal infections. In: Cydulka RK, Fitch MT, Joing SA, Wang VJ, Cline DM, Ma OJ, editors. Tintinalli's Emergency Medicine Manual. 8th ed. New York, NY: McGraw-Hill Education. (2017). pp. 806–1.
140. Uchihara T, Tsukagoshi H. Jolt Accentuation of headache: the most sensitive sign of CSF pleocytosis. Headache. (1991) 31:167–71. doi: 10.1111/j.1526-4610.1991.hed3103167.x
141. Mount HR, Boyle SD. Aseptic and bacterial meningitis: evaluation, treatment, and prevention. Am Fam Physician. (2017) 96:314–22.
142. van Eeuwijk JS. Brand PL. Nuchal rigidity in children: meningitis or not? Ned Tijdschr Geneeskd. (2003) 147:321–3.
143. Alhazmi A, Lazrek M, Alidjinou EK, Descombes G, Engelmann I, Hober D. Paediatric enterovirus meningitis without cerebrospinal fluid pleocytosis. J Infect. (2019) 79:612–25. doi: 10.1016/j.jinf.2019.11.006
144. Marcilla-Vazquez C, Martinez-Gutierrez A, Carrascosa-Romero MC, Baquero-Cano M, Alfaro-Ponce B. Neonatal viral meningitis. The importance of the polymerase chain reaction in their diagnosis. Rev Neurol. (2018) 67:484–90. Spanish. doi: 10.33588/rn.6712.2018203
145. Schoeman JF, Donald PR. Tuberculous meningitis. Handb Clin Neurol. (2013) 112:1135–8. doi: 10.1016/B978-0-444-52910-7.00033-7
146. Powers WJ. Cerebrospinal fluid lymphocytosis in acute bacterial meningitis. Am J Med. (1985) 79:216–20. doi: 10.1016/0002-9343(85)90012-9
147. Martinot M, Greigert V, Souply L, Rosolen B, De Briel D, Mohseni Zadeh M, et al. Cerebrospinal fluid monocytes in bacterial meningitis, viral meningitis, and neuroborreliosis. Med Mal Infect. (2018) 48:286–90. doi: 10.1016/j.medmal.2018.03.002
148. Matsubara T, Matsuoka T, Katayama K, Yoshitomi T, Nishikawa M, Ichiyama T, et al. Mononuclear cells and cytokines in the cerebrospinal fluid of echovirus 30 meningitis patients. Scand J Infect Dis. (2000) 32:471–4. doi: 10.1080/003655400458712
149. Sanaei Dashti A, Alizadeh S, Karimi A, Khalifeh M, Shoja SA. Diagnostic value of lactate, procalcitonin, ferritin, serum-C-reactive protein, and other biomarkers in bacterial and viral meningitis: a cross-sectional study. Medicine (Baltimore). (2017) 96:e7637. doi: 10.1097/MD.0000000000007637
150. Celik N, Tanir G, Aydemir C, Tuygun N, Zorlu P. Differential diagnosis of bacterial and viral meningitis in childhood acute meningitis: a statistical model. Mikrobiyol Bul. (2007) 41:63–9. Turkish.
151. DeKosky ST, Kochanek PM, Valadka AB, Clark RSB, Chou SH, Au AK, et al. Blood Biomarkers for Detection of Brain Injury in COVID-19 Patients. J Neurotrauma. (2021) 38:1–43. doi: 10.1089/neu.2020.7332
152. Hoen B. Differentiating bacterial from viral meningitis: contribution of nonmicrobiological laboratory tests. Med Mal Infect. (2009) 39:468–72. French. doi: 10.1016/j.medmal.2009.02.026
153. Nazir M, Wani WA, Malik MA, Mir MR, Ashraf Y, Kawoosa K, et al. Cerebrospinal fluid lactate: a differential biomarker for bacterial and viral meningitis in children. J Pediatr (Rio J). (2018) 94:88–92. doi: 10.1016/j.jped.2017.03.007
154. Julián-Jiménez A, Morales-Casado MI. Usefulness of blood and cerebrospinal fluid laboratory testing to predict bacterial meningitis in the emergency department. Neurologia (Engl Ed). (2019) 34:105–113. English, Spanish. doi: 10.1016/j.nrleng.2018.05.005
155. Feigin RD, Kaplan S. Inappropriate secretion of antidiuretic hormone in children with bacterial meningitis. Am J Clin Nutr. (1977) 30:1482–4. doi: 10.1093/ajcn/30.9.1482
156. Nakao T, Nitta T, Miura R, Ogata K, Kume T, Nobuta K, et al. Clinical and epidemiological studies on an outbreak of aseptic meningitis caused by coxsackie B5 And A9 viruses in aomori in 1961. Tohoku J Exp Med. (1964) 83:94–102. doi: 10.1620/tjem.83.94
157. Terraillon J, Brunet C, Sohier R. Value and limitations of blood amylase levels for a quick diagnosis of primary mumps meningitis. Value of the complement fixation reaction. Lyon Med. (1971) 226:607–12.
158. Szychowska Z, Kucharska W. The thyroid function in children with viral meningitis. Endokrynol Diabetol Chor Przemiany Materii Wieku Rozw. (1998) 4:13–7. Polish.
159. Szychowska Z, Kucharska W. The thyroid function in children with purulent meningitis. Endokrynol Diabetol Chor Przemiany Materii Wieku Rozw. (1998) 4:19–25. Polish.
160. de Crom SC, van Furth MA, Peeters MF, Rossen JW, Obihara CC. Characteristics of pediatric patients with enterovirus meningitis and no cerebral fluid pleocytosis. Eur J Pediatr. (2012) 171:795–800. doi: 10.1007/s00431-011-1626-z
161. Jaijakul S, Salazar L, Wootton SH, Aguilera E, Hasbun R. The clinical significance of neutrophilic pleocytosis in cerebrospinal fluid in patients with viral central nervous system infections. Int J Infect Dis. (2017) 59:77–81. doi: 10.1016/j.ijid.2017.04.010
162. Méchaï F, Rivière F, Roux X, Mérens A, Bigaillona C, Ficko C, et al. Epidemiological and clinical features of enteroviral meningitis: a case series of 59 adult patients. Rev Med Intern. (2010) 31:596–9. French. doi: 10.1016/j.revmed.2010.04.003
163. Jawaid A, Bano S, Haque AU, Arif K. Frequency and outcome of meningitis in pediatric intensive care unit of Pakistan. J Coll Physicians Surg Pak. (2016) 26:716–7.
164. Hudson JA, Broad J, Martin NG, Sadarangani M, Galal U, Kelly DF, et al. Outcomes beyond hospital discharge in infants and children with viral meningitis: a systematic review. Rev Med Virol. (2020) 30:e2083. doi: 10.1002/rmv.2083
165. Huang CC, Liu CC, Chang YC, Chen CY, Wang ST, Yeh TF. Neurologic complications in children with enterovirus 71 infection. N Engl J Med. (1999) 341:936–42. doi: 10.1056/NEJM199909233411302
166. Rudolph H, Schroten H, Tenenbaum T. Enterovirus Infections of the Central Nervous System in Children: an Update. Pediatr Infect Dis J. (2016) 35:567–9. doi: 10.1097/INF.0000000000001090
167. Drysdale SB, Kelly DF. Fifteen-minute consultation: enterovirus meningitis and encephalitis-when can we stop the antibiotics? Arch Dis Child Educ Pract Ed. (2017) 102:66–71. doi: 10.1136/archdischild-2016-310632
168. van Hinsbergh TMT, de Crom SCM, Lindeboom R, van Furth MAM, Obihara CC. Human parechovirus meningitis and gross-motor neurodevelopment in young children. Eur J Pediatr. (2019) 178:473–81. doi: 10.1007/s00431-019-03319-6
169. Jaremko JL, Moon AS, Kumbla S. Patterns of complications of neonatal and infant meningitis on MRI by organism: a 10 year review. Eur J Radiol. (2011) 80:821–7. doi: 10.1016/j.ejrad.2010.10.017
170. Christie D, Rashid H, El-Bashir H, Sweeney F, Shore T, Booy R, et al. Impact of meningitis on intelligence and development: a systematic review and meta-analysis. PLoS One. (2017) 12:e0175024. doi: 10.1371/journal.pone.0175024
171. Afhami S, Dehghan Manshadi SA, Rezahosseini O. Jolt accentuation of headache: can this maneuver rule out acute meningitis? BMC Res Notes. (2017) 10:540. doi: 10.1186/s13104-017-2877-1
172. Maruo Y, Nakanishi M, Suzuki Y, Kaneshi Y, Terashita Y, Narugami M, et al. Outbreak of aseptic meningitis caused by echovirus 30 in Kushiro, Japan in 2017. J Clin Virol. (2019) 116:34–8. doi: 10.1016/j.jcv.2019.05.001
173. Mofidi M, Negaresh N, Farsi D, Rezai M, Mahshidfar B, Abbasi S, et al. Jolt accentuation and its value as a sign in diagnosis of meningitis in patients with fever and headache. Turk J Emerg Med. (2016) 17:29–31. doi: 10.1016/j.tjem.2016.11.001
174. Ghotbi F, Shiva F. An assessment of the necessity of lumbar puncture in children with seizure and fever. J Pak Med Assoc. (2009) 59:292–5.
175. Wilber ST. Altered mental status in older emergency department patients. Emerg Med Clin North Am. (2006) 24:299–316, vi. doi: 10.1016/j.emc.2006.01.011
176. Straus SE, Thorpe KE, Holroyd-Leduc J. How do I perform a lumbar puncture and analyze the results to diagnose bacterial meningitis? JAMA. (2006) 296:2012–22. doi: 10.1001/jama.296.16.2012
177. Kieslich M, Acconci D, Berger A, Jarisch A, Böhles H, Bollinger M, et al. Diagnosis and outcome of neurotropic enterovirus infections in childhood. Klin Padiatr. (2002) 214:327–31. German. doi: 10.1055/s-2002-35371
178. Dos Santos GP, Skraba I, Oliveira D, Lima AA, de Melo MM, Kmetzsch CI, et al. Enterovirus meningitis in Brazil, 1998-2003. J Med Virol. (2006) 78:98–104. doi: 10.1002/jmv.20509
179. McLean DM, Larke RP, Cobb C, Griffis ED, Hackett SM. Mumps and enteroviral meningitis in Toronto, 1966. Can Med Assoc J. (1967) 96:1355–61.
180. Glimåker M, Ehrnst A, Magnius L, Berglund P, Forsgren M, Vikerfors T, et al. Early diagnosis of enteroviral meningitis by a solid-phase reverse immunosorbent test and virus isolation. Scand J Infect Dis. (1990) 22:519–26. doi: 10.3109/00365549009027090
181. Bakir TF. Viral meningitis and encephalitis in Saudi Arabia. J Trop Pediatr. (1989) 35:211–3. doi: 10.1093/tropej/35.5.211-a
182. Huang LM, Lee CY, Lee PI, Chen JM, Wang PJ. Meningitis caused by human herpesvirus-6. Arch Dis Child. (1991) 66:1443–4. doi: 10.1136/adc.66.12.1443
183. Garg RK, Malhotra HS, Jain A. Neuroimaging in tuberculous meningitis. Neurol India. (2016) 64:219–27. doi: 10.4103/0028-3886.177608
184. Imtiaz A, Toomath R. Computed tomography head scans prior to lumbar punctures in suspected meningitis. Intern Med J. (2019) 49:55–8. doi: 10.1111/imj.13997
185. Lakhera D, Azad RK, Azad S, Singh R, Sharma R. Magnetic resonance imaging cerebrospinal fluid hydrodynamics in patients with meningitis. J Clin Imaging Sci. (2020) 10:29. doi: 10.25259/JCIS_24_2020
186. Walls T, McSweeney A, Anderson T, Jennings LC. Multiplex-PCR for the detection of viruses in the CSF of infants and young children. J Med Virol. (2017) 89:559–61. doi: 10.1002/jmv.24461
187. Aldriweesh MA, Shafaay EA, Alwatban SM, Alkethami OM, Aljuraisi FN, Bosaeed M, et al. Viruses causing aseptic meningitis: a tertiary medical center experience with a multiplex PCR assay. Front Neurol. (2020) 11:602267. doi: 10.3389/fneur.2020.602267
188. Evans M, Merkel KG, Harder J, Rose DT. Impact of the implementation of a rapid meningitis/encephalitis multiplex polymerase chain reaction panel on IV acyclovir duration: multicenter, retrospective cohort of adult and pediatric patients. Diagn Microbiol Infect Dis. (2020) 96:114935. doi: 10.1016/j.diagmicrobio.2019.114935
189. Tansarli GS, Chapin KC. Diagnostic test accuracy of the BioFire® FilmArray® meningitis/encephalitis panel: a systematic review and meta-analysis. Clin Microbiol Infect. (2020) 26:281–90. doi: 10.1016/j.cmi.2019.11.016
190. Piantadosi A, Mukerji SS, Ye S, Leone MJ, Freimark LM, Park D, et al. Enhanced virus detection and metagenomic sequencing in patients with meningitis and encephalitis. MBio. (2021) 12:e0114321. doi: 10.1128/mBio.01143-21
191. Erdem G, Kaptsan I, Sharma H, Kumar A, Aylward SC, Kapoor A, et al. Cerebrospinal fluid analysis for viruses by metagenomic next-generation sequencing in pediatric encephalitis: not yet ready for prime time? J Child Neurol. (2021) 36:350–6. doi: 10.1177/0883073820972232
192. Forbes JD, Knox NC, Ronholm J, Pagotto F, Reimer A. Metagenomics: the next culture-independent game changer. Front Microbiol. (2017) 8:1069. doi: 10.3389/fmicb.2017.01069
193. Goldberg B, Sichtig H, Geyer C, Ledeboer N, Weinstock GM. Making the leap from research laboratory to clinic: challenges and opportunities for next-generation sequencing in infectious disease diagnostics. MBio. (2015) 6:e01888–15. doi: 10.1128/mBio.01888-15
Keywords: children, virus, meningitis, symptoms, epidemiology, diagnosis
Citation: Al-Qahtani SM, Shati AA, Alqahtani YA and Ali AS (2022) Etiology, Clinical Phenotypes, Epidemiological Correlates, Laboratory Biomarkers and Diagnostic Challenges of Pediatric Viral Meningitis: Descriptive Review. Front. Pediatr. 10:923125. doi: 10.3389/fped.2022.923125
Received: 18 April 2022; Accepted: 25 May 2022;
Published: 16 June 2022.
Edited by:
Thomas S. Murray, Yale University, United StatesReviewed by:
Juan Carlos Garcia-Monco, Basurto Hospital, SpainFatma Ali Eldin, Ain Shams University, Egypt
Copyright © 2022 Al-Qahtani, Shati, Alqahtani and Ali. This is an open-access article distributed under the terms of the Creative Commons Attribution License (CC BY). The use, distribution or reproduction in other forums is permitted, provided the original author(s) and the copyright owner(s) are credited and that the original publication in this journal is cited, in accordance with accepted academic practice. No use, distribution or reproduction is permitted which does not comply with these terms.
*Correspondence: Abdelwahid Saeed Ali, YWJ1bWFsYXoyMDAyQGdtYWlsLmNvbQ==