- 1Department of General Medicine, The Children’s Hospital Westmead, Westmead, NSW, Australia
- 2Department of Respiratory Medicine, The Children’s Hospital Westmead, NSW, Australia
- 3The University of Sydney, Discipline of Child and Adolescent Health, Faculty of Medicine and Health, Sydney, NSW, Australia
- 4Department of Infectious Diseases, The Children’s Hospital Westmead, Westmead, NSW, Australia
Pneumonia is the number one cause of disease and deaths in children under five years old, outside the neonatal period, with the greatest number of cases reported from resource-limited settings. The etiology is variable, with not much information on the local etiology drug resistance profile in many countries. Recent studies suggest an increasing contribution from respiratory viruses, also in children with severe pneumonia, with an increased relative contribution in settings that have good vaccine coverage against common bacterial pathogens. Respiratory virus circulation was greatly reduced during highly restrictive measures to contain the spread of COVID-19 but rebounded once COVID-19 restrictions were relaxed. We conducted a comprehensive literature review of the disease burden, pathogens, case management and current available prevention of community acquired childhood pneumonia, with a focus on rational antibiotic use, since the treatment of respiratory infections is the leading cause of antibiotic use in children. Consistent application of revised World Health Organisation (WHO) guidance that children presenting with coryzal symptoms or wheeze can be managed without antibiotics in the absence of fever, will help to reduce unnecessary antibiotic use, as will increased availability and use of bedside inflammatory marker tests, such as C–reactive protein (CRP) in children with respiratory symptoms and fever.
1. Introduction
Deaths among children under 5 years old mostly occur in the neonatal period (36.2%), but pneumonia (12.6%) and diarrhea (9.7%) remain the main cause of mortality in children under-5 years old in the post-neonatal period (1). Childhood pneumonia epidemiology, etiology and case management are variable in different WHO regions (2), with most deaths occurring in resource-limited settings where there is a limitation on antibiotics access (which is the mainstay treatment for bacterial pneumonia), on wide uptake of routine vaccination, on improving hygiene and nutrition, as well as on the availability of oxygen therapy (3). However, inappropriate antibiotic use is a global concern with alarming rates of antimicrobial resistance (AMR) documented in many regions, especially in resource-limited settings with limited microbiology services and extensive empiric antibiotic use (4). Lower respiratory tract infections is a major driver of inappropriate antibiotic use (4, 5).
Recent studies demonstrated that viruses contribute more to lower respiratory tract infections than previously appreciated, also in developing country settings and in children with severe disease (6–8). Strict social restrictions implemented to contain the COVID-19 pandemic and use of personal protective equipment (PPE) reduced respiratory virus transmission and associated secondary bacterial pneumonia, as demonstrated by a significant decrease in influenza infections and pneumonia due to Streptococcus pneumoniae in children (9, 10). However, major increases in respiratory virus infections were documented in many settings once COVID-19 social restrictions were relaxed. Australia experienced unprecedented and unseasonal respiratory syncytial virus (RSV) outbreaks during 2021 and 2022 (11).
The use of antibiotics is often promoted to reduce death from respiratory infections, but unnecessary and poorly targeted antibiotic use may do more harm than good (12, 13). Therefore, carefully considering the risks and benefits of antibiotic use in any individual patient is important. While appropriately used antibiotics will reduce morbidity and mortality from bacterial pneumonia, inappropriate use contributes to rising rates of AMR. With increased awareness, AMR is now recognized as a major global threat that has an impact on incidence, death, hospital stay and health care costs in a wide range of human diseases, including child pneumonia (4, 14). In many instances antibiotics are used inappropriately due to health system factors or parental expectations (4, 5, 15). There is a great need for doctors and health care systems to promote responsible antibiotic use by implementing effective antibiotic stewardship approaches (5). We conducted a comprehensive literature review of pneumonia epidemiology, aetiology and management with a focus on rational antibiotic use in childhood pneumonia.
2. Global pneumonia disease burden
In 2019, pneumonia was responsible for an estimated 0.7 (0.6–0.8) million deaths in children under five years old (16), with the highest number of pneumonia episodes per child year reported in North Africa and the Middle East. Although deaths related to child pneumonia has reduced over last 10 years, mortality remains high in many areas. Children in sub-Saharan Africa experience the highest mortality, accounting for 44.6% (312,400 deaths) of all pneumonia-related deaths in children under five (17). Table 1 illustrates pneumonia morbidity and mortality in different World Health Organisation (WHO) regions in 2016—the latest year for which regional data are available. Globally, both developing and developed country settings have achieved substantial reductions in pneumonia disease burden since the turn of the century (1, 18). Over this period the contribution of pneumonia to all-cause mortality declined from 16.6% [uncertainty range (UR): 14.8–17.9%] to 14.0% (UR: 12.0–15.1%) with the largest reduction in South Asia, from 17.5% (UR: 15.2–20.6%) to 11.7% (UR: 9.6–14.4%), mainly due to the implementation of expanded vaccination programs (16). Figure 1 summaries the decreasing trend of global child pneumonia mortality among children under five years old from 1986 to 2019.
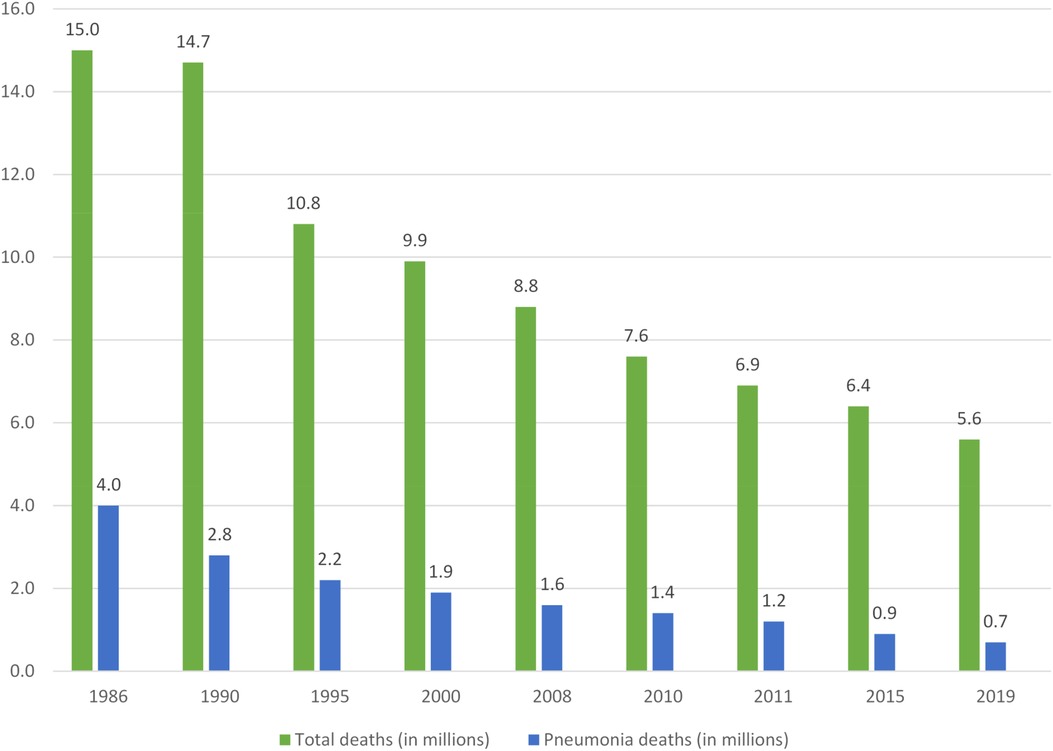
Figure 1. Declining total and pneumonia-related deaths in children under five years of age*. Percentages represent pneumonia deaths as a proportion of total deaths. *Adapted from (3, 14, 91)
The declines in child pneumonia morbidity and mortality were related to improved socioeconomic conditions and the application of routine vaccinations in the world, resulting in better coverage with measles, Haemophilus influenzae type b (Hib) and pneumococcal vaccines (PCV), as well as better access to healthcare systems and the effective case management application (16, 19). Hib vaccine is now delivered in all countries around the world as part of the WHO Expanded Programme of Immunisation (EPI). Its positive impact is well recognized and Hib deaths declined significantly (78%–96%) after more than 10 years of vaccine coverage in countries where these vaccines were included in routine vaccination schedules (19). As of the end of 2021, PCV had been introduced in most countries, except Somali, Guinea, South Sudan, Chad, Cabo Verde, Comoros, Equatorial Guinea, Gabon and Egypt (20). In 2022, Somalia, Guinea, South Sudan and Chad introduced PCV for the first time (20). Interestingly, PCV immunization studies in South Africa demonstrated an associated significant reduction (31%, 95% CI: 15%–43%) in the incidence of pneumonia hospitalization associated with common respiratory viruses (influenza A/B, parainfluenza types 1–3, RSV, adenovirus) (21). The mechanism remains unclear, but this could be the result of indirect protection via trained innate immunity induction. Currently, PCV-13 and PCV-10 are available for prevention of childhood pneumonia. PCV-7 was first licensed but was rapidly replaced by the higher-valent PCV-10 that covered additional invasive pneumococcal serotypes. Currently, PCV-13 is used most widely in routine immunisation programs, offering protection against all PCV-10 serotypes as well as serotypes 3, 6A, and 19A (22).
3. Common causes of community-acquired pneumonia
Childhood pneumonia is caused by many pathogens, including viruses, bacteria, mycobacteria (mainly Mycobacterium tuberculosis), fungi, and parasites (8). It is not easy to confirm the cause of a child pneumonia case (5) due to difficulties in obtaining the respiratory samples, especially in small children, the low sensitivity and uncertain specificity of microbiological investigation (23). In addition, a detected pathogen is sometimes hard to conclude as a true cause of disease due to the risk of contamination with upper airway flora, especially with non-invasive specimen collection methods (24). Disease causing pathogens are influenced by the child's age, geographic locality, and vaccination coverage. Recent studies from multiple settings have identified respiratory viruses as the most common cause of community acquired pneumonia in young children (6, 8). Table 2 provides an overview of common pathogens associated with paediatric pneumonia in otherwise healthy children. In neonates and children with immune compromise, congenital abnormalities or bronchiectasis, additional pathogens require consideration.
3.1. Viruses
With increased uptake of routine Hib and pneumococcal vaccines, respiratory viruses have become the main pathogens associated with pneumonia; both severe and non-severe (8). The most commonly identified viruses in all age groups include RSV, rhinovirus, as well as influenza and parainfluenza viruses (26, 27).
3.1.1. Respiratory syncytial virus (RSV)
The most common virus causing pediatric pneumonia in the multi-country Pneumonia Etiology Research for Child Health (PERCH) study was RSV, accounting for 31.1% of all cases; more than three times as common as any other pathogen (8). The clinical manifestations of RSV infection range from mild upper respiratory infections to severe pneumonia with respiratory failure. Common sequelae include prolonged wheezing with hyper-reactive airways (28). RSV is commonly associated with co-infection with other respiratory viruses and bacteria, which complicates aetiological determination (7, 8). Social distancing measures to contain COVID-19 disrupted RSV transmission with greatly reduced respiratory disease burden, but once social distancing was relaxed it returned with even greater prevalence and virulence. It has been postulated that this reflects reduced population immunity after a period of limited transmission, referred to as “immune debt” (11, 29). RSV is a major vaccine development target and promising new candidate vaccines will enter phase 3 trials in the near future (30).
3.1.2. Rhinoviruses and influenza viruses
Rhinoviruses have been detected with increasing frequency in children diagnosed with pneumonia (31) and are traditionally associated with common cold symptoms and frequently detected in asymptomatic individuals (mean prevalence 15%; up to 60%–80% in children). This is significantly more than other common respiratory viruses (prevalence 1%–5%) (32), which complicates interpretation of the role of rhinoviruses as a pneumonia pathogen (33). In immunocompromised hosts severe pneumonia has been attributed to rhinovirus infections (34). The current rhinovirus classification identifies three serotypes (A, B, C), which are further divided into 169 subtypes. A study from three sub-Saharan Africa countries conducted in children 13–59 months of age, reported an association between rhinovirus C and wheezing disease (35), but type specific differences have not been consistently observed.
The contribution of influenza viruses to childhood pneumonia is often under-appreciated. Studies have demonstrated that severe pneumococcal pneumonia is also more common in the presence of influenza infection (Odds Ratio (OR): 3.7; 95% Confidence Interval (CI): 1.0–18.1) and if not given universally pneumococcal vaccine should be considered in children with recurrent influenza virus infections (36). The most effective way to reduce the impact of influenza infection is seasonal vaccination (37), which was first recommended for health care workers to reduce their risk of contracting disease, with less time off work and reduced risk of spreading it to vulnerable patients within health care facilities (38). Influenza vaccine given to pregnant women was shown to provide protection to their newborn babies (49% protection documented in a study from South Africa), a particularly vulnerable group (39), and the United States Centers for Disease Control (CDC) was the first major public health agency to recommend universal seasonal vaccination (40).
3.1.3. Other viruses
Adenovirus-associated pneumonia is relatively uncommon (documented in 2%–12% of pneumonia cases), but it can cause severe necrotising pneumonia (especially serotypes 3, 7, and 14) (41, 42). A post-mortem study conducted in China among 175 children who died with pneumonia found that 9% of pulmonary tissue specimens were positive with adenovirus (43). Human metapneumovirus has been identified in 12% of children admitted with pneumonia in the United States (44); with detection in 44% of children under 12 months admitted with pneumonia in Spain (45). The prevalence of human metapneumovirus was ≥10% among children hospitalised with pneumonia in 4/7 study sites in the PERCH study (8). In a study from Thailand human bocavirus was the third most common pathogen detected, after rhinovirus and RSV, accounting for 12% of all cases (46). SARS-CoV-2, the cause of the COVID-19 pandemic, also infects children, but it rarely causes severe disease (47) and this risk is further reduced by vaccination (48). Four other coronaviruses are established human pathogens, but only cause mild cold like symptoms (49).
3.2. Bacterial pathogens
Studies showed that bacterial pathogens were more detected in severe childhood pneumonia which often required hospitalisation (8). However, the role of bacteria in less severe disease remains controversial with more potential causes identified when more tests are done. A recent study that applied a variety of detection tests such as antibody tests for S. pneumoniae and H. influenzae, as well as sensitive polymerase chain-reaction (PCR) and solid-phase immunoassays for respiratory viruses, identified potential pathogens in 85% of pneumonia cases (50). Co-infection of bacteria with respiratory viruses was seen in 41%, but it is unclear how to interpret these microbial associations and how it should guide antibiotic treatment (51). Historic lung aspirate and autopsy studies have confirmed the importance of S. pneumoniae, Staphylococcus aureus and H. influenzae, including non-typable strains, as causes of severe lobar pneumonia (8, 52).
3.2.1. Streptococcus pneumoniae
S. pneumoniae remains a leading cause of bacterial pneumonia, meningitis, and sepsis in children worldwide (53), despite the availability of effective vaccines and antibiotic treatment (8). S. pneumoniae causes around 11% (8%–12%) of all deaths in children less than 5 years [excluding pneumococcal deaths in human immunodeficiency virus (HIV) positive children], with most (61%) pneumococcal deaths occurring in ten African and Asian countries (53). Although lobar consolidation is typically associated with bacterial pneumonia, lobar consolidation is relatively uncommon among pneumonia cases (54–56) and is observed with both viral and bacterial infection.
3.2.2. Haemophilus influenzae
The contribution of H. influenzae decreased significantly after widespread implementation of routine conjugated Hib vaccine (57). Following vaccine roll-out studies in Africa and China, Hib became an uncommon cause for pneumonia and was less common than non-typeable H. influenzae (8)—which was reported at an increased frequency in some studies (58, 59). A lung aspirate study among severe pneumonia children from The Gambia found that 23% of samples were positive with H. influenza; non-typeable H. influenza accounted for 5% of all bacteria isolated (60).
3.2.3. Other bacteria
Atypical bacteria such as Mycoplasma pneumoniae (M. pneumoniae) and Chlamydia pneumoniae (C. pneumoniae) appear to be important pathogens in school-age children. A multi-center study from Italy assessing routine nasopharyngeal aspirates samples collected in 613 children 2–14 years old admitted with pneumonia found M. pneumoniae in 34.3% and C. pneumoniae in 14.1% of patients (61). Similar to respiratory viruses, its aetiologic role is confounded by frequent asymptomatic infection (62). Non-typhoidal salmonella has also been documented as an important cause of childhood pneumonia and sepsis, but mainly in malaria endemic areas (63). Children with hospital-acquired pneumonia (which per definition occurs ≥48 h after hospital admission) are often infected with gram-negative bacilli or S. aureus (64). S. aureus is also seen in community acquired pneumonia, particularly in adolescent children, and can follow an aggressive course if treatment is delayed or sub-optimal, which is common with methicillin-resistant S. aureus (MRSA) infection (8). S. aureus and Pseudomonas aeruginosa are also problematic pathogens in children with bronchiectasis, including cystic fibrosis (CF) (65). Interestingly, RSV infection has been shown to promote P. aeruginosa colonisation in this setting (66). In neonates group B streptococcus, Escherichia coli and Chlamydia trachomatis require consideration (67).
3.2.4. Mycobacterium tuberculosis
In high tuberculosis (TB) incidence settings, M. tuberculosis has been identified as a cause or co-pathogen in 5%–10% of children with severe pneumonia (8). Very young, malnourished and HIV-infected children are particularly vulnerable to develop pneumonia due to TB (6). Although traditionally associated with more chronic symptoms, TB can present as acute severe pneumonia with clinical and radiographic features indistinguishable from other causes of pneumonia (68). However, children with recent close TB contact or who live in a TB endemic setting and experience persistent symptoms, such as cough, fever, reduced playfulness and/or weight loss that do not respond to initial treatment should be suspected of TB (69, 70).
4. Pneumonia management
Due to challenges in determining child pneumonia etiology, differences in environmental risk factors and inconsistent uptake of effective vaccines against common pathogens around the world, pneumonia case management is variable in different settings. The need for a simple case management approach to guide care and improve outcomes in resources-limited settings has been recognised (71). The WHO developed and published a simple case management approach based on clinical signs and symptoms to recognize severe conditions (cases with any danger sign) that require urgent stabilization and guide the management of children with severe and non-severe pneumonia. Hypoxaemia management is important and appropriate oxygen therapy can reduce child pneumonia deaths (72). A study that introduced oximetry and oxygen therapy in five district hospitals in Papua New Guinea, a high-burden setting for child pneumonia, found a 35% reduction in deaths in children hospitalised with severe pneumonia (73).
4.1. Appropriate antibiotic use
Acute respiratory infections is a major reason for antibiotic use in children (74). Excessive and irrational antibiotic use is well documented all over the world and contributes to antimicrobial resistance (AMR) within the community. Figure 2 illustrates the number of deaths attributed to AMR infections globally. Although rising AMR rates pose a major health care threat, its value in reducing pneumonia deaths in children needs to be recognized. Use of standardised case management approaches that included antibiotic use reduced under five pneumonia deaths by 70% in developing country settings (75). However, given excessive hospital admission and use of parenteral antibiotics, WHO revised the clinical criteria for “severe” pneumonia so that those with fast breathing and/or chest in-drawing alone were no longer classified as “severe” pneumonia and could receive oral antibiotics as outpatients (67). This revision greatly reduced the annual incidence of severe pneumonia hospitalisations in infants, ranging from a difference of −301.0/100,000 infants (95% confidence interval, CI: −405.2 to −196.8) in Fiji to −3242.6/100,000 infants (95% CI: −3695.2 to −2789.9) in The Gambia, without a negative impact on patient outcomes (76).
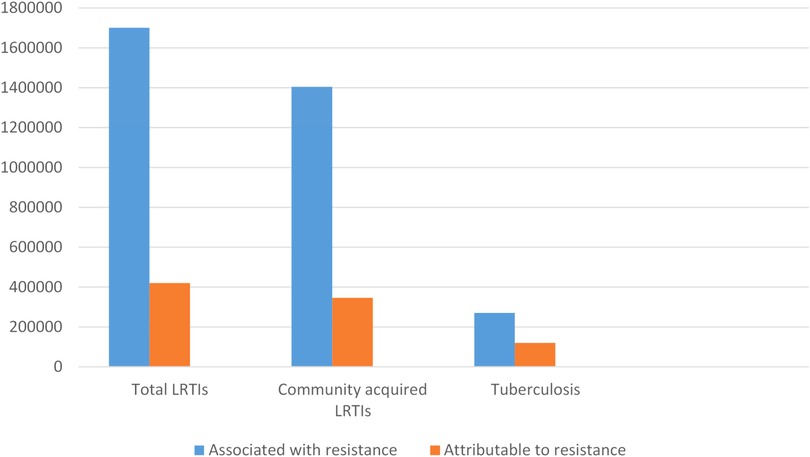
Figure 2. Estimated global deaths counts associated with and attributable to antimicrobial resistance in people with lower respiratory tract infection and tuberculosis in 2019*. *Adapted from (4). LRTIs, lower respiratory tract infection.
A major dilemma is the need to ensure that all children with bacterial pneumonia receive antibiotics to reduce pneumonia-related deaths, but at the same time to ensure that children with viral infections and conditions such as bronchiolitis or asthma do not receive inappropriate antibiotics. With good PCV and Hib vaccine coverage an increasing proportion of child pneumonia cases is caused by viral infections (8). Excessive use of empirical antibiotics, especially with broad-spectrum agents, has been recognised as a particular problem in Asian countries. Children with mild respiratory symptoms and no clinical evidence of severe pneumonia often receive empiric broad spectrum antibiotics without clinical indication (77). In addition, there is easy and unregulated access to a wide range of antibiotics, unlike developing countries where access is often limited or developed country settings where access is regulated. Table 3 provides a summary of risks and benefits of antibiotic treatment in paediatric pneumonia. It is important for clinicians to be familiar with the most common bacterial causes, their local drug resistance profiles and the likely positive and negative impacts of antibiotic therapy.
A major difficulty in child pneumonia management is knowing when to safely withhold antibiotic treatment in a child with suspected pneumonia based on clinical evaluation (3). In developed countries, the available of point-of-care diagnostic tests to distinguish between viral and bacterial infections and identify the causative pathogen(s) include a full blood count, C-reactive protein (CRP), and a viral panel test have been found to be useful in guiding antibiotic treatment. However, microbiological confirmation of the causative agent remains challenging, with high rates of co-infection complicating the picture (24). The PERCH study found that elevated CRP ≥40 mg/l was positively associated with confirmed bacterial pneumonia (especially S. pneumoniae and H. influenzae) and negatively associated with RSV (80). A recent study in Vietnam demonstrated that a point-of-care CRP test, using a cut-off of <50 mg/l, safely reduced antibiotic use in children with an acute respiratory infection presenting to primary healthcare centres (68.5% antibiotic use within 2 weeks follow up vs. 76.7% in control group, p = 0.0001) (81). Wheeze has been associated with viral infections in both African (82) and Asian settings (83). Revised WHO guidance recommends that a child with wheeze and no fever, in the absence of any WHO danger sign, should not receive antibiotic treatment (84). In those with fever or without a wheeze, a CXR and full blood count (and/or CRP) could provide clinicians with additional confidence to withhold antibiotics in a child with acute respiratory symptoms (85). Table 4 summarises some difficult dilemmas where the relevant trade-offs with antibiotic use is difficult.
5. Pneumonia prevention
Childhood and maternal immunisations against S. pneumoniae, Hib, Bordetella pertussis, measles and influenza viruses are highly effective prevention strategies, similar to the encouragement of exclusive breastfeeding during the first 6 months of life; ideally maintained for 1–2 years. Other important prevention strategies include reducing cigarette smoke exposure in young children, with reduced indoor and outdoor air pollution where this is a concern. Improving maternal health education, reductions in unnecessary hospitalisations and enhanced infection control in health care facilities to reduce the risk of nosocomial infections are additional prevention methods to reduce childhood pneumonia globally (87, 88).
Prophylactic antibiotic use can also be considered in specific high-risk cases. Pneumocystis jirovecii pneumonia (PJP) is preventable by cotrimoxazole preventive therapy and in HIV-infected children effective anti-retroviral therapy is most important (89). Like any antibiotic, cotrimoxazole prophylaxis also increases drug resistance (90, 91). Study in Botswana found that children using cotrimoxazole prophylaxis developed amoxicillin resistance (91). Given amoxicillin is first-line medication for management of “non-severe” pneumonia cases in primary settings around the work, this finding is concerning. Moreover, amoxicillin has been assigned to an “access category” by the WHO AWaRe antibiotic classification (67, 92). Cotrimoxazole prophylaxis also increase resistance to other antibiotics, such as chloramphenicol and ciprofloxacin (93).
Broader clinical considerations
This review describes the dilemma of appropriate antibiotic use in childhood community acquired pneumonia, given the challenge of rising antibiotic resistance rates around the world. A detailed discussion of complimentary treatment and the management of immunocompromised children is not included. As a narrative review it is open to subjectivity and bias, but every effort was made to provide a balanced overview and to include all the most relevant references. Data on the global pneumonia disease burden dates from 2016 is presented, which is somewhat dated, but it represents the latest published data and should provide an accurate overview of the situation before 2019. No reliable global data has been compiled since the COVID-19 pandemic. Our discussion of childhood community acquired pneumonia management only includes antibiotic and oxygen use, since these are the areas with the best available evidence and antibiotic treatment is the focus of our review. The intent was to provide an up-to-date, concise and informative overview stimulating critical assessment of appropriate antibiotic use, as encouraged by the new WHO AWaRe initiative (86).
6. Conclusion
Pneumonia remains the leading cause of mortality and morbidity in childhood, but this can be greatly reduced with good prevention and case management strategies. With increasing vaccine coverage respiratory viruses are now recognised as major pathogens, which requires a reconsideration of optimal antibiotic use. Appropriate antibiotic treatment saves lives, but it is important to limit inappropriate antibiotic use that drives the emergence of antimicrobial resistance without clinical benefit. Guidance about when and which antibiotics to use will be highly dependent on the local context, but it is important for clinicians to carefully consider the relevant trade-offs that should inform their decision.
Author contributions
TKPN and BJM conceptualized the manuscript and wrote the first draft. All authors gave constructive inputs from personal experience, suggested additional references to consider. All authors contributed to the article and approved the submitted version.
Conflict of interest
The authors declare that the research was conducted in the absence of any commercial or financial relationships that could be construed as a potential conflict of interest.
Publisher's note
All claims expressed in this article are solely those of the authors and do not necessarily represent those of their affiliated organizations, or those of the publisher, the editors and the reviewers. Any product that may be evaluated in this article, or claim that may be made by its manufacturer, is not guaranteed or endorsed by the publisher.
References
1. GBD Compare Data Visualization. Available at: http://vizhub.healthdata.org/gbd-compare (Accessed September 28, 2022).
2. Liu L, Johnson HL, Cousens S, Perin J, Scott S, Lawn JE, et al. Global, regional, and national causes of child mortality: an updated systematic analysis for 2010 with time trends since 2000. Lancet. (2012) 379(9832):2151–61. doi: 10.1016/S0140-6736(12)60560-1
3. Izadnegahdar R, Cohen AL, Klugman KP, Qazi SA. Childhood pneumonia in developing countries. Lancet Respir Med. (2013) 1(7):574–84. doi: 10.1016/S2213-2600(13)70075-4
4. Murray CJ, Ikuta KS, Sharara F, Swetschinski L, Aguilar GR, Gray A, et al. Global burden of bacterial antimicrobial resistance in 2019: a systematic analysis. Lancet. (2022) 399(10325):629–55. doi: 10.1016/S0140-6736(21)02724-0
5. Nguyen TKP, Tran TH, Pham HV, Graham SM, Marais BJ. Encouraging rational antibiotic use in childhood pneumonia—focus on the western pacific region. Pneumonia. (2017) 9:7. doi: 10.1186/s41479-017-0031-4
6. von Mollendorf C, Berger D, Gwee A, Duke T, Graham SM, Russell FM, et al. Aetiology of childhood pneumonia in low-and middle-income countries in the era of vaccination: a systematic review. J Glob Health. (2022) 12:10009. doi: 10.7189/jogh.12.10009
7. Jain S, Williams DJ, Arnold SR, Ampofo K, Bramley AM, Reed C, et al. Community-acquired pneumonia requiring hospitalization among US children. N Engl J Med. (2015) 372(9):835–45. doi: 10.1056/NEJMoa1405870
8. O'Brien KL, Baggett HC, Brooks WA, Feikin DR, Hammitt LL, Higdon MM, et al. Causes of severe pneumonia requiring hospital admission in children without HIV infection from Africa and Asia: the PERCH multi-country case-control study. Lancet. (2019) 394:757–79. doi: 10.1016/S0140-6736(19)30721-4
9. Huang C. Pediatric non-COVID-19 community-acquired pneumonia in COVID-19 pandemic. Int J Gen Med. (2021) 14:7165. doi: 10.2147/IJGM.S333751
10. Kaddour M, Simeonovic M, Osowicki J, McNab S, Satzke C, Robertson C, et al. COVID-19 and complicated bacterial pneumonia in children. ERJ Open Res. (2021) 7(1):00884-2020. doi: 10.1183/23120541.00884-2020
11. Eden J-S, Sikazwe C, Xie R, Deng Y-M, Sullivan SG, Michie A, et al. Off-season RSV epidemics in Australia after easing of COVID-19 restrictions. Nat Commun. (2022) 13(1):1–9. doi: 10.1038/s41467-022-30485-3
12. Lipshaw MJ, Eckerle M, Florin TA, Crotty EJ, Lipscomb J, Jacobs J, et al. Antibiotic use and outcomes in children in the emergency department with suspected pneumonia. Pediatrics. (2020) 145(4):e20193138. doi: 10.1542/peds.2019-3138
13. Okeke IN, Aboderin OA, Byarugaba DK, Ojo KK, Opintan JA. Growing problem of multidrug-resistant enteric pathogens in Africa. Emerging Infect Dis. (2007) 13(11):1640. doi: 10.3201/eid1311.070674
14. WHO. Antimicrobial resistance: global report on surveillance (2014). Available at: http://wwwwhoint/drugresistance/documents/surveillancereport/en/ (Accessed September 7, 2022).
15. Nguyen TKP, Tran TH, Truong TTH, Nguyen TV, Graham SM, Marais BJ. Paediatric use of antibiotics in children with community acquired pneumonia: a survey from Da nang, Vietnam. J Paediatr Child Health. (2019) 55(11):1329–34. doi: 10.1111/jpc.14413
16. Perin J, Mulick A, Yeung D, Villavicencio F, Lopez G, Strong KL, et al. Global, regional, and national causes of under-5 mortality in 2000–19: an updated systematic analysis with implications for the sustainable development goals. Lancet Child Adolesc Health. (2022) 6(2):106–15. doi: 10.1016/S2352-4642(21)00311-4
17. Troeger C, Blacker B, Khalil IA, Rao PC, Cao J, Zimsen SR, et al. Estimates of the global, regional, and national morbidity, mortality, and aetiologies of lower respiratory infections in 195 countries, 1990–2016: a systematic analysis for the global burden of disease study 2016. Lancet Infect Dis. (2018) 18(11):1191–210. doi: 10.1016/S1473-3099(18)30310-4
18. Sazawal S, Black RE, Group PCMT. Effect of pneumonia case management on mortality in neonates, infants, and preschool children: a meta-analysis of community-based trials. Lancet Infect Dis. (2003) 3(9):547–56. doi: 10.1016/S1473-3099(03)00737-0
19. Wahl B, O'Brien KL, Greenbaum A, Majumder A, Liu L, Chu Y, et al. Burden of Streptococcus pneumoniae and Haemophilus influenzae type b disease in children in the era of conjugate vaccines: global, regional, and national estimates for 2000–15. Lancet Glob Health. (2018) 6(7):e744–e57. doi: 10.1016/S2214-109X(18)30247-X
20. Stop pneumonia. Introducing PCV in Somalia, Guinea, South Sudan and Chad (2022). Available at: https://stoppneumonia.org/introducing-pcv-in-somalia-guinea-south-sudan-and-chad/ (Accessed September 28, 2022).
21. Madhi SA, Klugman KP, Group TVT. A role for Streptococcus pneumoniae in virus-associated pneumonia. Nat Med. (2004) 10(8):811–3. doi: 10.1038/nm1077
22. Lee H, Choi EH, Lee HJ. Efficacy and effectiveness of extended-valency pneumococcal conjugate vaccines. Korean J Pediatr. (2014) 57(2):55–66. doi: 10.3345/kjp.2014.57.2.55
23. Murdoch DR, O’Brien KL, Driscoll AJ, Karron RA, Bhat N, the Pneumonia Methods working Group, et al. Laboratory methods for determining pneumonia etiology in children. Clin Infect Dis. (2012) 54(Suppl 2):S146–52. doi: 10.1093/cid/cir1073
24. Nguyen TKP, Tran TH, Robert CL, Graham SM, Marais BJ. Child pneumonia in the western pacific region. Paediatr Respir Rev. (2017) 21:102–10. doi: 10.1016/j.prrv.2016.07.004
25. McIntosh K. Community-acquired pneumonia in children. N Engl J Med. (2002) 346(6):429–37. doi: 10.1056/NEJMra011994
26. Zar HJ, Barnett W, Stadler A, Gardner-Lubbe S, Myer L, Nicol MP. Aetiology of childhood pneumonia in a well vaccinated South African birth cohort: a nested case-control study of the drakenstein child health study. Lancet Respir Med. (2016) 4:463–72. doi: 10.1016/S2213-2600(16)00096-5
27. Bénet T, Picot VS, Awasthi S, Pandey N, Bavdekar A, Kawade A, et al. Severity of pneumonia in under 5-year-old children from developing countries: a multicenter, prospective, observational study. Am J Trop Med Hyg. (2017) 97(1):68. doi: 10.4269/ajtmh.16-0733
28. Bianchini S, Silvestri E, Argentiero A, Fainardi V, Pisi G, Esposito S. Role of respiratory syncytial virus in pediatric pneumonia. Microorganisms. (2020) 8(12):2048. doi: 10.3390/microorganisms8122048
29. Billard M-N, Bont LJ. Quantifying the RSV immunity debt following COVID-19: a public health matter. Lancet Infect Dis. (2023) 23(1):3–5. doi: 10.1016/S473-3099(22)00544-8
30. Higgins D, Trujillo C, Keech C. Advances in RSV vaccine research and development–a global agenda. Vaccine. (2016) 34(26):2870–5. doi: 10.1016/j.vaccine.2016.03.109
31. Hayden FG. Rhinovirus and the lower respiratory tract. Rev Med Virol. (2004) 14(1):17–31. doi: 10.1002/rmv.406
32. Jartti T, Jartti L, Peltola V, Waris M, Ruuskanen O. Identification of respiratory viruses in asymptomatic subjects: asymptomatic respiratory viral infections. Pediatr Infect Dis J. (2008) 27(12):1103–7. doi: 10.1097/INF.0b013e31817e695d
33. Papadopoulos NG. Do rhinoviruses cause pneumonia in children? Paediatr Respir Rev. (2004) 5:S191–S5. doi: 10.1016/S1526-0542(04)90036-X
34. Ruuskanen O, Lahti E, Jennings LC, Murdoch DR. Viral pneumonia. Lancet. (2011) 377(9773):1264–75. doi: 10.1016/S0140-6736(10)61459-6
35. Baillie VL, Moore DP, Mathunjwa A, Morailane P, Simões EA, Madhi SA. Molecular subtyping of human rhinovirus in children from three sub-saharan African countries. J Clin Microbiol. (2019) 57(9):e00723–19. doi: 10.1128/JCM.00723-19
36. O'brien KL, Walters MI, Sellman J, Quinlisk P, Regnery H, Schwartz B, et al. Severe pneumococcal pneumonia in previously healthy children: the role of preceding influenza infection. Clin Infect Dis. (2000) 30(5):784–9. doi: 10.1086/313772
37. Breteler JK, Tam JS, Jit M, Ket JC, De Boer MR. Efficacy and effectiveness of seasonal and pandemic A (H1N1) 2009 influenzae vaccines in low and middle income countries: a systematic review and meta-analysis. Vaccines (Basel). (2013) 31(45):5168–77. doi: 10.1016/j.vaccine.2013.08.056
38. Apisarnthanarak A, Puthavathana P, Kitphati R, Auewarakul P, Mundy LM. Outbreaks of influenza A among nonvaccinated healthcare workers: implications for resource-limited settings. Infect Control Hosp Epidemiol. (2008) 29(8):777–80. doi: 10.1086/588162
39. Madhi SA, Cutland CL, Kuwanda L, Weinberg A, Hugo A, Jones S, et al. Influenza vaccination of pregnant women and protection of their infants. N Engl J Med. (2014) 371(10):918–31. doi: 10.1056/NEJMoa1401480
40. Nowak GJ, Sheedy K, Bursey K, Smith TM, Basket M. Promoting influenza vaccination: insights from a qualitative meta-analysis of 14 years of influenza-related communications research by US centers for disease control and prevention (CDC). Vaccine. (2015) 33(24):2741–56. doi: 10.1016/j.vaccine.2015.04.064
41. Nascimento-Carvalho CM, Ribeiro CT, Cardoso MRA, Barral A, Araújo-Neto CA, Oliveira JR, et al. The role of respiratory viral infections among children hospitalized for community-acquired pneumonia in a developing country. Pediatr Infect Dis J. (2008) 27(10):939–41. doi: 10.1097/INF.0b013e3181723751
42. Carballal G, Videla C, Misirlian A, Requeijo PV, Aguilar MDC. Adenovirus type 7 associated with severe and fatal acute lower respiratory infections in argentine children. BMC Pediatr. (2002) 2(1):1–7. doi: 10.1186/1471-2431-2-6
43. Ou Z-Y, Zeng Q-Y, Wang F-H, Xia H-M, Lu J-P, Xia J-Q, et al. Retrospective study of adenovirus in autopsied pulmonary tissue of pediatric fatal pneumonia in south China. BMC Infect Dis. (2008) 8(1):1–6. doi: 10.1186/1471-2334-8-1
44. Williams JV, Harris PA, Tollefson SJ, Halburnt-Rush LL, Pingsterhaus JM, Edwards KM, et al. Human metapneumovirus and lower respiratory tract disease in otherwise healthy infants and children. N Engl J Med. (2004) 350(5):443–50. doi: 10.1056/NEJMoa025472
45. Camps M, Ricart S, Dimova V, Rovira N, Muñoz-Almagro C, Garcia JJ, et al. Prevalence of human metapneumovirus among hospitalized children younger than 1 year in catalonia, Spain. J Med Virol. (2008) 80(8):1452–60. doi: 10.1002/jmv.21209
46. Fry AM, Lu X, Chittaganpitch M, Peret T, Fischer J, Dowell SF, et al. Human bocavirus: a novel parvovirus epidemiologically associated with pneumonia requiring hospitalization in Thailand. J Infect Dis. (2007) 195(7):1038–45. doi: 10.1086/512163
47. Williams P, Koirala A, Saravanos GL, Lopez LK, Glover C, Sharma K, et al. COVID-19 in New South Wales children during 2021: severity and clinical spectrum. Med J Aust. (2022) 217(6):303–10. doi: 10.5694/mja2.51661
48. Zimmermann P, Pittet LF, Finn A, Pollard AJ, Curtis N. Should children be vaccinated against COVID-19? Arch Dis Child. (2022) 107(3):e1. doi: 10.1136/archdischild-2021-323040
49. Paules CI, Marston HD, Fauci AS. Coronavirus infections—more than just the common cold. JAMA. (2020) 323(8):707–8. doi: 10.1001/jama.2020.0757
50. Juvén T, Mertsola J, Waris M, Leinonen M, Meurman O, Roivainen M, et al. Etiology of community-acquired pneumonia in 254 hospitalized children. Pediatr Infect Dis J. (2000) 19(4):293–8. doi: 10.1097/00006454-200004000-00006
51. Brealey JC, Sly PD, Young PR, Chappell KJ. Viral bacterial co-infection of the respiratory tract during early childhood. FEMS Microbiol Lett. (2015) 362(10):fnv062. doi: 10.1093/femsle/fnv062
52. Chintu C, Mudenda V, Lucas S, Nunn A, Lishimpi K, Maswahu D, et al. Lung diseases at necropsy in African children dying from respiratory illnesses: a descriptive necropsy study. Lancet. (2002) 360(9338):985–90. doi: 10.1016/S0140-6736(02)11082-8
53. O'brien KL, Wolfson LJ, Watt JP, Henkle E, Deloria-Knoll M, McCall N, et al. Burden of disease caused by Streptococcus pneumoniae in children younger than 5 years: global estimates. Lancet. (2009) 374(9693):893–902. doi: 10.1016/S0140-6736(09)61204-6
54. Cutts F, Zaman S, Enwere GY, Jaffar S, Levine O, Okoko J, et al. Efficacy of nine-valent pneumococcal conjugate vaccine against pneumonia and invasive pneumococcal disease in the Gambia: randomised, double-blind, placebo-controlled trial. Lancet. (2005) 365(9465):1139–46. doi: 10.1016/S0140-6736(05)71876-6
55. Klugman KP, Madhi SA, Huebner RE, Kohberger R, Mbelle N, Pierce N. A trial of a 9-valent pneumococcal conjugate vaccine in children with and those without HIV infection. N Engl J Med. (2003) 349(14):1341–8. doi: 10.1056/NEJMoa035060
56. Tregnaghi MW, Saez-Llorens X, Lopez P, Abate H, Smith E, Posleman A, et al. Efficacy of pneumococcal nontypable Haemophilus influenzae protein D conjugate vaccine (PHiD-CV) in young Latin American children: a double-blind randomized controlled trial. PLoS Med. (2014) 11(6):e1001657. doi: 10.1371/journal.pmed.1001657
57. Flasche S, Takahashi K, Vu DT, Suzuki M, Nguyen TH-A, Le H, et al. Early indication for a reduced burden of radiologically confirmed pneumonia in children following the introduction of routine vaccination against Haemophilus influenzae type b in nha trang, Vietnam. Vaccine. (2014) 32(51):6963–70. doi: 10.1016/j.vaccine.2014.10.055
58. Van Eldere J, Slack MP, Ladhani S, Cripps AW. Non-typeable Haemophilus influenzae, an under-recognised pathogen. Lancet Infect Dis. (2014) 14(12):1281–92. doi: 10.1016/S1473-3099(14)70734-0
59. Cripps AW. Nontypeable Haemophilus influenzae and childhood pneumonia. PNG Med J. (2010) 53(3–4):147–50.
60. Howie SR, Morris GA, Tokarz R, Ebruke BE, Machuka EM, Ideh RC, et al. Etiology of severe childhood pneumonia in the Gambia, West Africa, determined by conventional and molecular microbiological analyses of lung and pleural aspirate samples. Clin Infect Dis. (2014) 59(5):682–5. doi: 10.1093/cid/ciu384
61. Principi N, Esposito S, Blasi F, Allegra L, Group MS. Role of Mycoplasma pneumoniae and Chlamydia pneumoniae in children with community-acquired lower respiratory tract infections. Clin Infect Dis. (2001) 32(9):1281–9. doi: 10.1086/319981
62. Darville T. Chlamydia trachomatis infections in neonates and young children. Semin Pediatr Infect Dis. (2005) 16(4):235–44. doi: 10.1053/j.spid.2005.06.004
63. Graham SM, Walsh AL, Molyneux EM, Phiri AJ, Molyneux ME. Clinical presentation of non-typhoidal Salmonella bacteraemia in Malawian children. Trans R Soc Trop Med Hyg. (2000) 94(3):310–4. doi: 10.1016/S0035-9203(00)90337-7
64. Ebruke BE, Knoll MD, Haddix M, Zaman S, Prosperi C, Feikin DR, et al. The aetiology of pneumonia from analysis of lung aspirate and pleural fluid samples: findings from the PERCH study. Clin Infect Dis. (2020) 73(22):e3788–96. doi: 10.1093/cid/ciaa32
65. Hatziagorou E, Orenti A, Drevinek P, Kashirskaya N, Mei-Zahav M, De Boeck K, et al. Changing epidemiology of the respiratory bacteriology of patients with cystic fibrosis–data from the European cystic fibrosis society patient registry. J Cyst Fibros. (2020) 19(3):376–83. doi: 10.1016/j.jcf.2019.08.006
66. Hendricks MR, Lashua LP, Fischer DK, Flitter BA, Eichinger KM, Durbin JE, et al. Respiratory syncytial virus infection enhances Pseudomonas aeruginosa biofilm growth through dysregulation of nutritional immunity. Proc Natl Acad Sci. (2016) 113(6):1642–7. doi: 10.1073/pnas.1516979113
67. WHO. Revised WHO classification and treatment of childhood pneumonia at health facilities. Geneva: World Health Organization (2014).
68. Kazi S, Corcoran H, Abo Y-N, Graham H, Oliwa J, Graham SM. A systematic review of clinical, epidemiological and demographic predictors of tuberculosis in children with pneumonia. J Glob Health. (2022) 12:10010. doi: 10.7189/jogh.12.10010
69. World Health Organization. WHO Operational handbook on tuberculosis. Module 5: Management of tuberculosis in children and adolescents. Geneva: World Health Organization (2022).
70. Perez-Velez CM, Marais BJ. Tuberculosis in children. N Engl J Med. (2012) 367(4):348–61. doi: 10.1056/NEJMra1008049
71. Graham SM, English M, Hazir T, Enarson P, Duke T. Challenges to improving case management of childhood pneumonia at health facilities in resource-limited settings. Bull World Health Organ. (2008) 86(5):349–55. doi: 10.2471/BLT.07.048512
72. Bhutta ZA, Das JK, Walker N, Rizvi A, Campbell H, Rudan I, et al. Interventions to address deaths from childhood pneumonia and diarrhoea equitably: what works and at what cost? Lancet. (2013) 381(9875):1417–29. doi: 10.1016/S0140-6736(13)60648-0
73. Duke T, Wandi F, Jonathan M, Matai S, Kaupa M, Saavu M, et al. Improved oxygen systems for childhood pneumonia: a multihospital effectiveness study in Papua New Guinea. Lancet. (2008) 372(9646):1328–33. doi: 10.1016/S0140-6736(08)61164-2
74. Nguyen NV, Do NTT, Vu DTV, Greer RC, Dittrich S, Vandendorpe M, et al. Outpatient antibiotic prescribing for acute respiratory infections in Vietnamese primary care settings by the WHO AWaRe (access, watch and reserve) classification: an analysis using routinely collected electronic prescription data. Lancet Reg Health West Pac. (2022) 30:100611. doi: 10.1016/j.lanwpc.2022.100611
75. Theodoratou E, Al-Jilaihawi S, Woodward F, Ferguson J, Jhass A, Balliet M, et al. The effect of case management on childhood pneumonia mortality in developing countries. Int J Epidemiol. (2010) 39(1):155–71. doi: 10.1093/ije/dyq032
76. Russell F RR, Chan J, Tuivaga E, Lim R, Lai J, Van HMT, et al. Impact of the change in the WHO severe pneumonia case definition on hospitalised pneumonia epidemiology: case studies from Africa and the Asia-pacific. Bull World Health Organ. (2019) 97:386–93. doi: 10.2471/BLT.18.223271
77. Murni IK, Duke T, Kinney S, Daley AJ, Soenarto Y. Reducing hospital-acquired infections and improving the rational use of antibiotics in a developing country: an effectiveness study. Arch Dis Child. (2014) 100(5):454–9. doi: 10.1136/archdischild-2014-307297
78. Manohar P, Loh B, Athira S, Nachimuthu R, Hua X, Welburn SC, et al. Secondary bacterial infections during pulmonary viral disease: phage therapeutics as alternatives to antibiotics? Front Microbiol. (2020) 11:1434. doi: 10.3389/fmicb.2020.01434
79. Nguyen TKP, Tran TH, Fitzgerald DA, Graham SM, Marais BJ. Antibiotic use in children hospitalised with pnuemonia in central Vietnam: a prospective study. Arch Dis Child. (2019) 105(8):713–9. doi: 10.1136/archdischild-2019-317733
80. Higdon MM, Le T, O’brien KL, Murdoch DR, Prosperi C, Baggett HC, et al. Association of C-reactive protein with bacterial and respiratory syncytial virus–associated pneumonia among children aged< 5 years in the PERCH study. Clin Infect Dis. (2017) 64(Suppl_3):S378–86. doi: 10.1093/cid/cix150
81. Do NT, Ta NT, Tran NT, Than HM, Vu BT, Hoang LB, et al. Point-of-care C-reactive protein testing to reduce inappropriate use of antibiotics for non-severe acute respiratory infections in Vietnamese primary health care: a randomised controlled trial. Lancet Global Health. (2016) 4(9):633–41. doi: 10.1016/S2214-109X(16)30142-5
82. Reed C, Madhi SA, Klugman KP, Kuwanda L, Ortiz JR, Finelli L, et al. Development of the respiratory Index of severity in children (RISC) score among young children with respiratory infections in South Africa. PloS One. (2012) 7(1):e27793. doi: 10.1371/journal.pone.0027793
83. Shan W, Shi T, Chen K, Xue J, Wang Y, Yu J, et al. Risk factors for severe community-acquired pneumonia among children hospitalized with CAP younger than 5 years of age. Pediatr Infect Dis J. (2018) 33(3):224–9. doi: 10.1097/INF.0000000000002098
84. WHO. Recommendations for management of common childhood conditions: evidence for technical update of pocket book recommendations: newborn conditions, dysentery, pneumonia, oxygen use and delivery, common causes of fever, severe acute malnutrition and supportive care. Geneva: World Health Organization (2012).
85. Nguyen TKP, Tran TH, Tran ST, Fitzgerald DA, Graham SM, Marais BJ. Predictors of “unlikely bacterial pneumonia”and “adverse pneumonia outcome”in children admitted to hospital in central Vietnam. Clin Infect Dis. (2019) 70(8):1733–41. doi: 10.1093/cid/ciz445
86. WHO. The WHO AWaRe (Access, Watch, Reserve) antibiotic book (2022). Available at: https://www.who.int/publications/i/item/9789240062382 Accessed December 21, 2022).
87. WHO. Pneumonia factsheet (2016). Available at: https://stoppneumonia.org/wp-content/uploads/2014/10/Pneumonia-Factsheet.pdf (Accessed September 4, 2022).
88. Nguyen TKP, Tran TH, Roberts CL, Fox GJ, Graham SM, Marais BJ. Risk factors for child pneumonia-focus on the western pacific region. Paediatr Respir Rev. (2017) 21:95–101. doi: 10.1016/j.prrv.2016.07.002
89. World Health Organization. Guidelines on co-trimoxazole prophylaxis for HIV-related infections among children, adolescents and adults in resource-limited settings: recommendations for a public health approach. Geneva: World Health Organization (2006).
90. D’Souza AW, Moodley-Govender E, Berla B, Kelkar T, Wang B, Sun X, et al. Cotrimoxazole prophylaxis increases resistance gene prevalence and α-diversity but decreases β-diversity in the gut microbiome of human immunodeficiency virus–exposed, uninfected infants. Clin Infect Dis. (2020) 71(11):2858–68. doi: 10.1093/cid/ciz1186
91. Powis KM, Souda S, Lockman S, Ajibola G, Bennett K, Leidner J, et al. Cotrimoxazole prophylaxis was associated with enteric commensal bacterial resistance among HIV-exposed infants in a randomized controlled trial, Botswana. J Int AIDS Soc. (2017) 20(3):e25021. doi: 10.1002/jia2.25021
92. World Health Organization. The 2019 WHO AWaRe classification of antibiotics for evaluation and monitoring of use (2019). Available at: https://apps.who.int/iris/handle/10665/327957 (Accessed September 28, 2022).
93. Morpeth SC, Thielman NM, Ramadhani HO, Hamilton JD, Ostermann J, Kisenge PR, et al. Effect of trimethoprim-sulfamethoxazole prophylaxis on antimicrobial resistance of fecal Escherichia coli in HIV-infected patients in Tanzania. J Acquir Immune Defic Syndr. (2008) 47(5):585. doi: 10.1097/QAI.0b013e31816856db
Keywords: acute respiratory infections, lower respiratory tract infections, pneumonia, antibiotic, antimicrobial resistance
Citation: Nguyen PTK, Robinson PD, Fitzgerald DA and Marais BJ (2023) The dilemma of improving rational antibiotic use in pediatric community-acquired pneumonia. Front. Pediatr. 11:1095166. doi: 10.3389/fped.2023.1095166
Received: 10 November 2022; Accepted: 10 January 2023;
Published: 8 February 2023.
Edited by:
Mario Barreto, Sapienza University of Rome, ItalyReviewed by:
Vikas Goyal, Children's Health Queensland, AustraliaMilos Jesenak, Comenius University, Slovakia
© 2023 Nguyen, Robinson, Fitzgerald and Marais. This is an open-access article distributed under the terms of the Creative Commons Attribution License (CC BY). The use, distribution or reproduction in other forums is permitted, provided the original author(s) and the copyright owner(s) are credited and that the original publication in this journal is cited, in accordance with accepted academic practice. No use, distribution or reproduction is permitted which does not comply with these terms.
*Correspondence: Phuong T. K. Nguyen dGhpa2ltcGh1b25nLm5ndXllbkBoZWFsdGgubnN3Lmdvdi5hdQ==
Specialty Section: This article was submitted to Pediatric Pulmonology, a section of the journal Frontiers in Pediatrics