- 1Department of Ophthalmology, Poznan University of Medical Sciences, Poznan, Poland
- 2Department of Pediatric Diabetes, Clinical Auxology and Obesity, Poznan University of Medical Sciences, Poznan, Poland
Childhood obesity has reached epidemic levels worldwide. Overweight and obesity is associated with an increase in several inflammatory markers, leading to chronic low-grade inflammation responsible for macro- and microvascular dysfunction. While the impact of obesity on overall health is well-described, less is known about its ocular manifestations. Still, there are few studies in children and adolescents in this regard and they are inconsistent. However, some evidence suggests a significant role of overnutrition in the development of changes in retinal microvasculature parameters (wider venules, narrower arterioles, lower arteriovenous ratio). Higher values of intraocular pressure were found to be positively correlated with high body mass index (BMI) as well as obesity. In addition, the retinal nerve fiber layer (RNFL) values seem to be lower in obese children, and there is a significant negative correlation between RNFL values and anthropometric and/or metabolic parameters. Changes also could be present in macular retinal thickness and choroidal thickness as well as in the retinal vessel density in children with obesity. However, these associations were not consistently documented. The purpose of this review is to present the most current issues on child obesity and the related potential ocular effects through an overview of international publications from the years 1992–2022.
Introduction
Childhood obesity has become one of the most important public health concerns. Over the past 50 years, its prevalence has increased globally, reaching pandemic levels. The World Health Organization (WHO) estimates that worldwide, over 340 million children and adolescents aged 5–19 years and almost 40 million children under the age of 5 years were overweight or obese in 2016 and 2020, respectively (1, 2). The latest data from the United States Centers for Disease Control and Prevention (CDC) has shown that the prevalence of obesity among United States children and adolescents was 19.3% in 2017–2018 (3). In addition, the COVID-19 pandemic worsened the burden of childhood obesity (4, 5). For example, in the United States, during the first year of the pandemic, the prevalence of obesity in children aged 5–11 years rose dramatically from 19% to 26% (5).
Obesity has emerged as a complex phenotype resulting from the interaction of genes, environment, and lifestyle (6). There are numerous factors contributing to childhood obesity, including socioeconomic aspects, psychosocial stress, gender, biology, physical inactivity, or a caloric intake that is greater than needs (7). Endocrine causes of pediatric obesity are identified in less than 1% of all cases (8).
Regardless of its cause, obesity results in the abnormal or excessive accumulation of fat. Adipose tissue, in addition to providing a reservoir of energy, serves as a major endocrine organ that secretes adipokines, growth factors, cytokines, and chemokines (9, 10). Increased proinflammatory cytokine secretion from adipose tissue and the infiltration of leukocytes, including macrophages, into the adipose tissue is responsible for chronic low-grade inflammation in obese individuals. This state impairs important functions in terms of regulating fatty acid oxidation, glucose and fat homeostasis, and insulin signaling. Oxidative stress, inflammation, and dyslipidemia accompanied by insulin resistance lead to micro- and macroangiopathy (11, 12).
Obesity can adversely affect health, and obesity-related diseases in children and adolescents are well-characterized (7, 13, 14) (shown in Figure 1).
Less is known about the potential ocular effects of childhood obesity. In adults, obesity has been linked to age-related cataracts, glaucoma, age-related maculopathy, and diabetic retinopathy (15). This review examines the potential ocular effects of childhood obesity.
Data sources and searches
A literature search on PubMed and Google Scholar was performed using the following terms in various combinations: childhood obesity, obesity, children and adolescents, pediatric population, insulin resistance, retinal microvasculature, optical coherence tomography, optical coherence tomography angiography, foveal avascular zone, choroidal thickness, retinal nerve fiber layer, and intraocular pressure. All articles published between November 1992 and November 2022 were checked by title, abstract, and full text. Relevant articles are reviewed and summarized here.
Changes in retinal vessel morphology in terms of childhood obesity
Since the 1990s, fundus photography has been widely used to evaluate the retinal vascular caliber. Hubbard et al. developed formulas to calculate the central retinal arteriolar equivalent (CRAE) and the central retinal venular equivalent (CRVE) calibers, as well as the dimensionless quotient arteriovenous ratio (AVR) (16, 17). Changes in retinal vessel caliber, particularly wider retinal venules and narrower retinal arterioles, have been found to increase the risk of cardiovascular disease in obese youth (18, 19). Obesity may have a profound effect on retinal vascular caliber. Increased adiposity and sedentary lifestyle behaviors negatively correlate with vessel width parameters. Growing evidence suggests that wider venules, narrower arterioles, and a lower AVR are related to a greater body mass index (BMI), larger waist circumference, and higher levels of sedentary behavior. In contrast, moderate-to-vigorous-intensity physical activity was associated with larger arterioles, narrower venules, and a higher AVR (20). Furthermore, other data demonstrated a strong relationship between retinal vascular calibers and obesity-induced hypertension. Retinal arteriolar narrowing, but not venular dilation, has been linked to increased systematic blood pressure (21). Interestingly, in a study conducted by Rijsk et al., in a multiple linear regression, a higher diastolic blood pressure (DBP) z-score and lower fasting glucose levels significantly contributed to a narrower retinal arteriolar diameter (22).
Undoubtedly, obesity has an impact on retinal vessel diameter; however, less data is available concerning the relationship between retinal vessel morphology and particular metabolic parameters. Leptin is secreted by adipocytes in proportion to body fat mass and plays a key role in energy homeostasis. Obesity can lead to leptin resistance and subsequent high leptin concentrations (23). Some studies have provided evidence in support of a positive association between leptin and retinal vessel diameter (24, 25). High levels of plasma leptin, dependent on BMI, have been associated with both wider CRVE (p = 0.032) and lower AVR (p = 0.010) (24). This is consistent with findings reported by Van Aart et al., indicating a positive significant relationship between zLeptin and CRAE (standardized coefficient β = 0.14) (25).
Apart from leptin, elevated levels of C-reactive protein (CRP), found in obese individuals, have also been linked to changes in retinal vessel diameter (26, 27). CRP is used as a clinical marker of inflammation, with elevated serum levels being a strong independent predictor of future adverse cardiovascular events (28). Hanssen et al. have reported that wider venular diameters were independently associated with higher levels of high-sensitivity C-reactive protein (hsCRP). The highest absolute diameter differences (+11.83um) were seen in retinal venules for each unit increase of hsCRP (p < 0.01) (26). Likewise, Gishti et al. showed that a higher CRP level is related to a wider venular caliber (difference 0.10 standard deviation score (SDS) (95% CI 0.06,0.14) per SDS increase in CRP), but this was not influenced by BMI (27).
Dyslipidemia, insulin resistance, and non-alcoholic fatty liver disease (NAFLD), other common features in obese children, also affect the retinal microvasculature. Some data have shown a positive relationship between hepatic fibrosis in pediatric NAFLD patients and the degree of retinopathy signs. In addition, obese and overweight children with retinopathy had significantly higher values of triglycerides (90.20 vs. 105.57 mg/dl, p = 0.04), basal insulin (12.97 vs. 17.20 mUI/ml p = 0.02), and HOMA-IR (2,76 vs. 3,37, p = 0.04) than patients without retinopathy (29).
Insulin-like growth factor 1 (IGF-I) plays a significant part in retinal vascularization. Recent data has demonstrated that overweight/obese children with retinopathy had significantly higher insulin-like growth factor 1 (IGF-1) SDS (-0.66 ± 0.9 vs. 0.03 ± 1.3, p = 0.04) compared to overweight/obese individuals without retinopathy (30). This suggests that high levels of IGF-1 in overweight/obese children and adolescents may be a potential contributing factor to microvascular damage.
However, the mechanisms linking childhood obesity to changes in retinal vessel morphology are not fully explained. It is hypothesized that increased adiposity is associated with the systemic microvasculature abnormalities that precede the development of overt disease. Microvascular processes may play a role in the pathogenesis of alterations in retinal vessel caliber, possibly through hyperinsulinemia or inflammatory pathways. Research has shown that arterial narrowing and venular widening are more evident among those with high levels of inflammatory and endothelial dysfunction markers (31). In addition, leptin has been demonstrated to directly impair endothelium-dependent vasodilation (32). Lastly, retinal venular dilatation may reflect a regulatory response to the increased total blood volume presented in obese children (31). However, further studies need to be carried out to clarify changes in retinal vessel caliber in obese children and adolescents.
Influence of childhood obesity on intraocular pressure
Elevated intraocular pressure (IOP) may irreversibly damage the optic nerve and could result in visual impairment and blindness (33). Many studies have supported the association between higher values of intraocular pressure and high BMI (34, 35) as well as obesity (36–38). Akinci et al. have reported that the average IOP was significantly higher in obese children than in the control group; gender and age connections were not statistically significant between groups. In addition, diurnal fluctuations in IOP were also higher in the obese group (5.4 ± 1.8 mmHg) compared to the control group (2.8 ± 0.9 mmHg, p < 0.001). These findings show that BMI is an independent risk factor for elevated IOP in children and adolescents (38). The Shandong Children Eye Study produced similar results, indicating that higher values of IOP were positively correlated with increased body mass (p < 0.001) and BMI (p < 0.001) (34). Pileggi et al. demonstrated that elevated IOP was related to high BP in overweight/obese children. This suggests that obesity confers an increased risk for both elevated IOP and systemic vascular abnormalities, such as elevated BP and hypertension among children and adolescents (35). However, it is worth mentioning that several studies have not confirmed an association between obesity and changes in eye pressure among children and adolescents (39–43). The mechanisms underlying the association between IOP and obesity are yet to be fully established. However, it has been suggested that elevated IOP may be caused by excess intraorbital fat, increased episcleral venous pressure, and decreased aqueous outflow. Also, increased blood viscosity, present in obese individuals, can lead to an increase in outflow resistance in the episcleral veins. Finally, obesity-induced hypertension may influence IOP through increased pressure in the ciliary arteries, leading to excessive aqueous filtration (38, 44).
Retinal nerve fiber layer thickness in the obese pediatric population
The retinal nerve fiber layer (RNFL) which is a sensitive structure, can be damaged due to a chronic inflammatory process. Optical coherence tomography (OCT) provides an objective and quantitative measurement of RNFL thickness.
Changes in retinal nerve fiber layer thickness are an important sign of early retinal damage. Therefore, there is increasing attention on RNFL thickness in obesity. Recent studies suggest that the RNFL is significantly thinner in obese children (37, 41, 42, 45, 46) Compared to healthy controls, obese individuals were characterized by binocular RNFL thickness asymmetry (41), decreased RNFL values in particular sectors (41, 42, 45), and thinner ganglion cell-inner plexiform layers (GCL + IPL) (41, 46). Moreover, changes in RNFL thickness are related to the degree of obesity. In children with severe obesity (SDS-BMI >4), RNFL thickness on average and in the inferior, superior and nasal quadrants were lower compared to overweight (SDS-BMI >1 to ≤2) and obese (SDS-BMI >2 to ≤4) individuals or healthy controls (SDS-BMI from −1 to 1) (42). The RNFL and GCL + IPL values for each sector are summarized in Tables 1, 2, respectively.
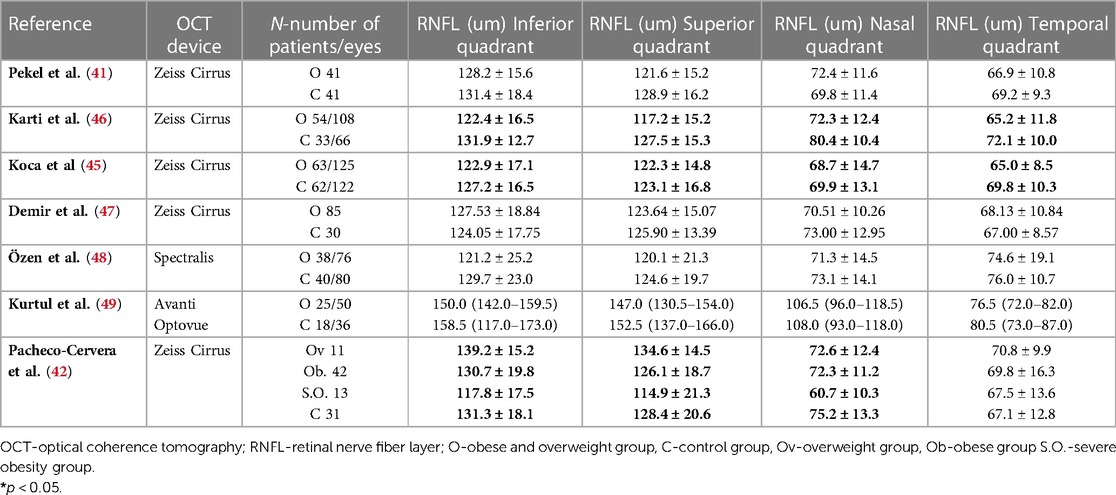
Table 1. Summary of reported values of OCT RNFL in obese children and adolescents (mean±) or median (min-max) in literature.
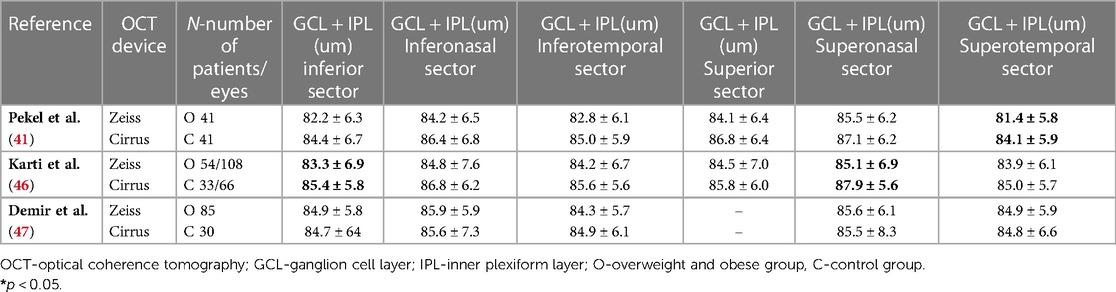
Table 2. Summary of reported values of OCT GCL + IPL in obese children and adolescents (mean ± SD) in literature.
In addition, metabolic parameters such as insulin, triglyceride, Homeostatic Model Assessment of Insulin Resistance (HOMA-IR), low-density lipoprotein (LDL), interleukin 6 (IL-6), and leptin have also been found to be negatively related to RNFL thickness (37, 41, 42, 45, 46, 48) (Table 3).
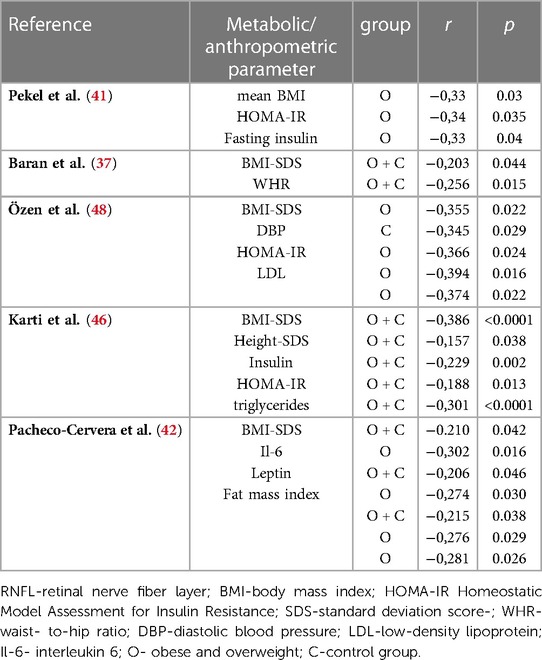
Table 3. Reported significant correlation between RNFL and anthropometric and metabolic parameters in literature.
Although many studies have shown that obesity is associated with changes in RNFL thickness, some studies have failed to show this relationship (47–49). The reason for changes in RNFL thickness in obese children is unclear. Increased resistance in the small vessels feeding the optic nerve head may result in temporary or permanent ischemia. Therefore, insufficient vascular supply of the optic nerve head as a result of endothelial dysfunction and autonomic changes creates conditions that damage nerve fibers. Also, it has been suggested that RNFL values decrease with an increase in inflammatory mediators (41).
The association between choroidal thickness and macular retinal thickness in obese children
The choroid is one of the most highly vascularized tissues of the body, as it supplies the outer retina with nutrients and maintains the temperature and volume of the eye (50) Being the most vascular tissue in the eye, the choroid has been implicated in the pathophysiology of a variety of ocular diseases. Thinning of the choroid could be a predictive factor for retina damage.
The literature shows that changes in choroidal thickness (CT) may be induced by obesity. Several studies showed that children with obesity had thinner CT in comparison to the control group (36, 51, 52). Interestingly CT measurements were thinner among those obese patients who did not have IR as compared to those with IR (36, 51). The authors found a positive correlation between CT and HOMA-IR (36, 51). Differences in CT values in obese with and without IR can be explained by the vascular action of insulin, which stimulates endothelial production of NO with subsequent choroidal vasodilation and increased CT (53).
Obesity not only affects CT but also macular retinal thickness (MRT) and macular retinal volume (MRV). Some studies have reported that these ocular parameters are significantly decreased in obese children (52, 54). In one study, MRT and MRV values tended to be thinner with an increase in BMI-SDS and waist circumference standard deviation score (WC-SDS) (54).
However, a clear link between obesity and decreased CT and MRT has not yet been established. In a study conducted by Bulus et al., CT had higher values in obese patients, moreover, CT was positively correlated with BMI, BMI-SDS, Weight-SDS, obesity duration, and cholesterol level (40). Likewise, Kurtul et al. found a higher MRT in obese patients (49). A positive correlation between body fat percentage and CT has also been demonstrated (37).
A strong inflammatory response in adipose tissue, leading to oxidative stress and hypoxia, may cause changes in CT in obese individuals (40). In addition, thinning of the choroidal layer could result from the autonomic regulation of choroidal blood flow. Decreased levels of NO, as a direct result of obesity, lead to a reduction in choroidal blood flow and subsequent choroidal thickness (55).
Interestingly, it has been shown that weight loss may have a positive effect on ocular structures. A study investigating the effect of rapid BMI decrease with bariatric surgery in obese adults showed improvements in CT values after weight loss. The favorable effect of weight loss might be related to improvements in the autoregulation of choroidal blood flow and a decrease in medial arterial pressure (56).
However, it must be emphasized that some studies have not found a significant difference between choroidal and retinal thickness in obese children compared to healthy controls (37, 40, 57). Further studies to verify changes in CT and MRT are needed in obese juvenile populations.
Influence of childhood obesity on retinal vascular density measured by optical coherence tomography angiography
Optical coherence tomography angiography (OCTA) is a novel technique that allows for a more accurate evaluation of the retinal vasculature, with the ability to isolate particular vascular plexuses (superficial and deep plexuses). OCTA has become an indispensable tool used in diagnosing and monitoring retinal diseases, for example choroidal neovascularization (CNV) in the course of age-related macular degeneration and high myopia or the estimation of foveal avascular zone (FAZ) size in the course of diabetic retinopathy, sickle cell-related retinopathy, or retinal vascular occlusion diseases.
So far, there have been only a few studies that used OCTA to evaluate retinal vessel density in the obese pediatric population. However, some of these studies have suggested that childhood obesity may affect the retinal microvasculature. Kurtul et al. and Han et al. showed that superficial, as well as deep capillary plexuses in the foveal area were larger in obese children in comparison to age- and sex-matched healthy controls (49, 58). Likewise, Dereli et al. reported that significantly greater vessel density in the parafoveal area of the superficial capillary plexus was observed in children with obesity (59). Noteworthy Han et al. reported lower vessel density in the inferior and nasal parafovea and temporal perifovea of deep capillary plexus in obese children. There is still no objective explanation for changes in the vascular density of retinal plexuses in childhood obesity. It has been suggested that pro-inflammatory cytokines may lead to degenerative changes (59). However, Kurtul et al. advocate that increased total body mass (with increased intra- and extracellular water), bone, and skeletal muscle mass may play a central role in elevated OCTA values (49).
Still, differences in OCTA values between obese and healthy children were not demonstrated by all authors (57), emphasizing the need for further research.
Conclusion
Obesity is one of the most serious global public health challenges; however, its impact on ocular health in children is still not well-studied. To the best of our knowledge, this is the first review to examine the potential ocular effects of childhood obesity. Similar comparisons have only been made in the adult population. According to the findings of this review, the majority of studies found a significant association between adiposity and changes in vessel width parameters (wider venules, narrower arterioles, lower arteriovenous ratio). Many articles showed a positive relation between higher values of intraocular pressure and high BMI as well as obesity. In most studies, the retinal nerve fiber layer (RNFL) values were lower in obese children, and a significant negative correlation was found between RNFL values and anthropometric and/or metabolic parameters. Changes were also present in macular retinal thickness and choroidal thickness as well as in the retinal vessel density in children with obesity. However, these associations have not been consistently documented. In addition, there is a lack of studies underlying these eye changes in obese pediatric patients. The inconsistency of the results and an insufficient explanation for the observed changes suggest that further investigations are required to clarify these associations. The effectiveness of obesity management in reducing the risk of eye alterations is also unknown, but research in this area might provide significant insight into the potential use of weight loss strategies to reduce the burden of eye diseases in children with obesity. Moreover, studies examining the effects of a potentially anti-inflammatory nutritional intervention reducing obesity-induced ocular changes might deliver valuable and practical information.
Author contributions
JD-G was involved in the literature review and data interpretation and analysis and wrote the first draft of the manuscript. EN contributed to the study design and concept, data interpretation, and wrote and reviewed the manuscript. AK and AG-W contributed to data acquisition, interpretation, and critical review of the manuscript. JD-G is the guarantor of this work and takes responsibility for the integrity of the data and the accuracy of the data analysis. All authors contributed to the article and approved the submitted version.
Conflict of interest
The authors declare that the research was conducted in the absence of any commercial or financial relationships that could be construed as a potential conflict of interest.
Publisher's note
All claims expressed in this article are solely those of the authors and do not necessarily represent those of their affiliated organizations, or those of the publisher, the editors and the reviewers. Any product that may be evaluated in this article, or claim that may be made by its manufacturer, is not guaranteed or endorsed by the publisher.
Abbreviations
OCT, optical coherence tomography; OCTA, optical coherence tomography angiography; RNFL, retinal nerve fiber layer; MRT, macular retinal thickness; CT, choroidal thickness; IOP, intraocular pressure; BMI, body mass index; WHR, waist-to-hip ratio; CRAE, central retinal arteriolar equivalent; CRVE, central retinal venular equivalent; AVR, arteriovenous ratio; NAFLD, non-alcoholic fatty liver disease; SDS, standard deviation score; IGF-I, Insulin-like growth factor 1; GCL + IPL, ganglion cell-inner plexiform layer; DBP, diastolic blood pressure; CRP, C-reactive protein; hsCRP, high-sensitivity C-reactive protein; HOMA-IR, Homeostatic Model Assessment of Insulin Resistance; LDL, low-density lipoprotein; IL-6, interleukin 6; MRV, macular retinal volume; WC-SDS, waist circumference standard deviation score; FAZ, foveal avascular zone.
References
1. Bentham J, Di Cesare M, Bilano V, Bixby H, Zhou B, Stevens GA, et al. Worldwide trends in body-mass index, underweight, overweight, and obesity from 1975 to 2016: a pooled analysis of 2416 population-based measurement studies in 128·9 million children, adolescents, and adults. Lancet (London, England). (2017) 390(10113):2627–42. doi: 10.1016/S0140-6736(17)32129-3
2. Obesity and overweight. Available at: https://www.who.int/news-room/fact-sheets/detail/obesity-and-overweight(cited 2022 Mar 24).
3. Childhood Obesity Facts | Overweight & Obesity | CDC. Available at: https://www.cdc.gov/obesity/data/childhood.html(cited 2022 Mar 24).
4. Vogel M, Geserick M, Gausche R, Beger C, Poulain T, Meigen C, et al. Age- and weight group-specific weight gain patterns in children and adolescents during the 15 years before and during the COVID-19 pandemic. Int J Obes. (2021) 46(1):144–52. Available at: https://www.nature.com/articles/s41366-021-00968-2(cited 2022 Mar 24) doi: 10.1038/s41366-021-00968-2
5. Woolford SJ, Sidell M, Li X, Else V, Young DR, Resnicow K, et al. Changes in body mass Index among children and adolescents during the COVID-19 pandemic. JAMA. (2021) 326(14):1434–6. doi: 10.1001/jama.2021.15036
6. Campbell MK. Biological, environmental, and social influences on childhood obesity. Pediatr Res 2016 791. (2015) 79(1):205–11. doi: 10.1038/pr.2015.208
7. Sahoo K, Sahoo B, Choudhury AK, Sofi NY, Kumar R, Bhadoria AS. Childhood obesity: causes and consequences. J Fam Med Prim Care. (2015) 4(2):187. Available at:/pmc/articles/PMC4408699/(cited 2022 Mar 24). doi: 10.4103/2249-4863.154628
8. Reinehr T, Hinney A, de Sousa G, Austrup F, Hebebrand J, Andler W. Definable somatic disorders in overweight children and adolescents. J Pediatr. (2007) 150(6):618–622.e5. Available at: https://pubmed.ncbi.nlm.nih.gov/17517246/(cited 2022 Mar 24) doi: 10.1016/j.jpeds.2007.01.042
9. Klöting N, Blüher M. Adipocyte dysfunction, inflammation and metabolic syndrome. Rev Endocr Metab Disord. (2014) 15(4):277–87. Available at: https://pubmed.ncbi.nlm.nih.gov/25344447/(cited 2022 Mar 24) doi: 10.1007/s11154-014-9301-0
10. Scheja L, Heeren J. The endocrine function of adipose tissues in health and cardiometabolic disease. Nat Rev Endocrinol. (2019) 15(9):507–24. doi: 10.1038/s41574-019-0230-6
11. Rutkowski JM, Stern JH, Scherer PE. The cell biology of fat expansion. J Cell Biol. (2015) 208(5):501–12. Available at: https://pubmed.ncbi.nlm.nih.gov/25733711/(cited 2022 Mar 24) doi: 10.1083/jcb.201409063
12. Takemoto K, Deckelbaum RJ, Saito I, Likitmaskul S, Morandi A, Pinelli L, et al. Adiponectin/resistin levels and insulin resistance in children: a four country comparison study. Int J Pediatr Endocrinol. (2015) 2015(1):1–12. doi: 10.1186/1687-9856-2015-2
13. Must A, Strauss RS. Risks and consequences of childhood and adolescent obesity. Int J Obes Relat Metab Disord. (1999) 23(Suppl 2):S2–11. Available at: https://pubmed.ncbi.nlm.nih.gov/10340798/(cited 2022 Mar 26) doi: 10.1038/sj.ijo.0800852
14. Vander Wal JS, Mitchell ER. Psychological complications of pediatric obesity. Pediatr Clin North Am. (2011) 58(6):1393–401. doi: 10.1016/j.pcl.2011.09.008
15. Cheung N, Wong TY. Obesity and eye diseases. Surv Ophthalmol. (2007) 52(2):180–95. Available at: https://pubmed.ncbi.nlm.nih.gov/17355856/(cited 2022 Feb 20) doi: 10.1016/j.survophthal.2006.12.003
16. Hubbard LD, Brothers RJ, King WN, Clegg LX, Klein R, Cooper LS, et al. Methods for evaluation of retinal microvascular abnormalities associated with hypertension/sclerosis in the atherosclerosis risk in communities study. Ophthalmology. (1999) 106(12):2269–80. Available at: https://pubmed.ncbi.nlm.nih.gov/10599656/(cited 2022 Apr 24) doi: 10.1016/S0161-6420(99)90525-0
17. Bhuiyan A, Kawasaki R, Lamoureux E, Ramamohanarao K, Wong TY. Retinal artery-vein caliber grading using color fundus imaging. Comput Methods Programs Biomed. (2013) 111(1):104–14. doi: 10.1016/j.cmpb.2013.02.004
18. Yau DPL, Kim MM, Tirsi DA, Convit DA. Retinal vessel alterations and cerebral white matter microstructural damage in obese adolescents with metabolic syndrome. JAMA Pediatr. (2014) 168(12):e142815. Available at:/pmc/articles/PMC4420159/(cited 2021 Aug 24). doi: 10.1001/jamapediatrics.2014.2815
19. Ho A, Cheung CY, Wong JS, Zhang Y, Tang FY, Kam KW, et al. Independent and synergistic effects of high blood pressure and obesity on retinal vasculature in young children: the Hong Kong children eye study. J Am Heart Assoc. (2021) 10(3):1–11. doi: 10.1161/JAHA.120.018485
20. Sousa-Sá E, Zhang Z, Pereira JR, Wright IM, Okely AD, Santos R. Systematic review on retinal microvasculature, physical activity, sedentary behaviour and adiposity in children and adolescents. Acta Paediatr. (2020) 109(10):1956–73. Available at: https://pubmed.ncbi.nlm.nih.gov/31998981/(cited 2021 Aug 24) doi: 10.1111/apa.15204
21. Köchli S, Endes K, Infanger D, Zahner L, Hanssen H. Obesity, blood pressure, and retinal vessels: a meta-analysis. Pediatrics. (2018) 141(6):e20174090. doi: 10.1542/peds.2017-4090
22. Rijks J, Vreugdenhil A, Dorenbos E, Karnebeek K, Joris P, Berendschot T, et al. Characteristics of the retinal microvasculature in association with cardiovascular risk markers in children with overweight, obesity and morbid obesity OPEN. Available at: www.nature.com/scientificreports(cited 2021 Feb 22)
23. Obradovic M, Sudar-Milovanovic E, Soskic S, Essack M, Arya S, Stewart AJ, et al. Leptin and obesity: role and clinical implication. Front Endocrinol (Lausanne). (2021) 12:563. doi: 10.3389/fendo.2021.585887
24. Siegrist M, Hanssen H, Neidig M, Fuchs M, Lechner F, Stetten M, et al. Association of leptin and insulin with childhood obesity and retinal vessel diameters. Int J Obes. (2014) 38(9):1241–7. doi: 10.1038/ijo.2013.226
25. Van Aart CJC, Michels N, Sioen I, De Decker A, Nawrot TS, De Henauw S. Associations of leptin, insulin and lipids with retinal microvasculature in children and adolescents. J Pediatr Endocrinol Metab. (2018) 31(2):143–50. doi: 10.1515/jpem-2017-0374
26. Hanssen H, Siegrist M, Neidig M, Renner A, Birzele P, Siclovan A, et al. Retinal vessel diameter, obesity and metabolic risk factors in school children (JuvenTUM 3). Atherosclerosis. (2012) 221(1):242–8. doi: 10.1016/j.atherosclerosis.2011.12.029
27. Gishti O, Jaddoe VWV, Hofman A, Wong TY, Ikram MK, Gaillard R. Body fat distribution, metabolic and inflammatory markers and retinal microvasculature in school-age children. The generation R study. Int J Obes. (2015) 39(10):1482–7. doi: 10.1038/ijo.2015.99
28. C-reactive protein, inflammation and coronary heart disease. Available at: https://www.infona.pl/resource/bwmeta1.element.elsevier-95d787dd-4ebe-39f1-88da-98d210102627(cited 2022 Apr 24).
29. Liccardo D, Mosca A, Petroni S, Valente P, Giordano U, Mico’ AGA, et al. The association between retinal microvascular changes, metabolic risk factors, and liver histology in pediatric patients with non-alcoholic fatty liver disease (NAFLD). J Gastroenterol. (2015) 50(8):903–12. doi: 10.1007/s00535-014-1024-1
30. Bizzarri C, Pedicelli S, Romanzo A, Bocchini S, Bottaro G, Cianfarani S, et al. The impact of IGF-I, puberty and obesity on early retinopathy in children: a cross-sectional study. Ital J Pediatr. (2019) 45(1):1–7. doi: 10.1186/s13052-019-0650-x (cited 2021 Jul 4).30606228
31. Cheung N, Saw SM, Islam FA, Rogers SL, Shankar A, De Haseth K, et al. BMI And retinal vascular caliber in children. Obesity (Silver Spring). (2007) 15(1):209. Available at: https://pubmed.ncbi.nlm.nih.gov/17228049/(cited 2021 Aug 21) doi: 10.1038/oby.2007.576
32. Wang J, Wang H, Luo W, Guo C, Wang J, Chen YE, et al. Leptin-induced endothelial dysfunction is mediated by sympathetic nervous system activity. J Am Hear Assoc Cardiovasc Cerebrovasc Dis. (2013) 2(5):e000299. doi: 10.1161/JAHA.113.000299
33. Davis BM, Crawley L, Pahlitzsch M, Javaid F, Cordeiro MF. Glaucoma: the retina and beyond. Acta Neuropathol. (2016) 132(6):807–26. Available at: https://pubmed.ncbi.nlm.nih.gov/27544758/(cited 2022 Apr 24) doi: 10.1007/s00401-016-1609-2
34. Jiang WJ, Wu JF, Hu YY, Wu H, Sun W, Lu TL, et al. Intraocular pressure and associated factors in children: the shandong children eye study. Invest Ophthalmol Vis Sci. (2014) 55(7):4128–34. Available at: www.iovs.org(cited 2021 Aug 21) doi: 10.1167/iovs.14-14244
35. Pileggi C, Papadopoli R, De Sarro C, Nobile CGA, Pavia M. Obesity, blood pressure, and intraocular pressure: a cross-sectional study in Italian children. Obes Facts. (2021) 14(2):169–77. doi: 10.1159/000514096
36. Aydemir GA, Aydemir E, Asik A, Bolu S. Changes in ocular pulse amplitude and choroidal thickness in childhood obesity patients with and without insulin resistance. Eur J Ophthalmol. (2022) 32(4):2018–25. doi: 10.1177/11206721211039337
37. Baran R, Baran S, Toraman N, Filiz S, Demirbilek H. Evaluation of intraocular pressure and retinal nerve fiber layer, retinal ganglion cell, central macular thickness, and choroidal thickness using optical coherence tomography in obese children and healthy controls. Niger J Clin Pract. (2019) 22(4):539–45. doi: 10.4103/njcp.njcp_471_18
38. Akinci A, Cetinkaya E, Aycan Z, Oner O. Relationship between intraocular pressure and obesity in children. J Glaucoma. (2007) 16(7):627–30. Available at: https://pubmed.ncbi.nlm.nih.gov/18091182/(cited 2021 Feb 22) doi: 10.1097/IJG.0b013e318057528a
39. Koçak N, Arslan N, Karti O, Tokgoz Y, Ozturk T, Gunenç U, et al. Evaluation of the intraocular pressure in obese adolescents—minerva pediatrica. Minerva Medica—Journals. (2015) 67(5):413–8. PMID: 26377780.
40. Bulus AD, Can ME, Baytaroglu A, Can GD, Cakmak HB, Andiran N. Choroidal thickness in childhood obesity. Ophthalmic Surg Lasers Imaging Retin. (2017) 48(1):10–7. doi: 10.3928/23258160-20161219-02
41. Pekel E, Altıncık SA, Pekel G. Inner retinal thickness and optic disc measurements in obese children and adolescents. Arq Bras Oftalmol. (2020) 83(5):383–8. doi: 10.5935/0004-2749.20200047
42. Pacheco-Cervera J, Codoñer-Franch P, Simó-Jordá R, Pons-Vázquez S, Galbis-Estrada C, Pinazo-Durán MD. Reduced retinal nerve fibre layer thickness in children with severe obesity. Pediatr Obes. (2015) 10(6):448–53. doi: 10.1111/ijpo.12005
43. Hazar L, Oyur G, Yılmaz GC, Vural E. Relationship of obesity and related disorders with ocular parameters in children and adolescent. Curr Eye Res. (2021) 46(9):1393–7. Available at: https://pubmed.ncbi.nlm.nih.gov/33586562/(cited 2022 Mar 18) doi: 10.1080/02713683.2021.1884727
44. Wong RL, Zhao P, Lai WW. Choroidal thickness in relation to hypercholesterolemia on enhanced depth imaging optical coherence tomography. Retina. (2013) 33(2):423–8. Available at: https://pubmed.ncbi.nlm.nih.gov/23089893/(cited 2021 Aug 30) doi: 10.1097/IAE.0b013e3182753b5a
45. Koca S, Keskin M, Saricaoglu MS, Koca SB, Duru N, Şahin Hamurcu M, et al. Optic nerve parameters in obese children as measured by spectral domain optical coherence tomography. Semin Ophthalmol. (2017) 32(6):743–7. doi: 10.1080/08820538.2016.1177095
46. Karti O, Nalbantoglu O, Abali S, Tunc S, Ozkan B. The assessment of peripapillary retinal nerve fiber layer and macular ganglion cell layer changes in obese children: a cross-sectional study using optical coherence tomography. Int Ophthalmol. (2016) 37(4):1031–8. Available at: https://link.springer.com/article/10.1007/s10792-016-0371-8(cited 2021 Aug 11) doi: 10.1007/s10792-016-0371-8
47. Demir S, Özer S, Alim S, Güneş A, Ortak H, Yılmaz R. Retinal nerve fiber layer and ganglion cell-inner plexiform layer thickness in children with obesity. Int J Ophthalmol. (2016) 9(3):434–8. doi: 10.18240/ijo.2016.03.19
48. Özen B, Öztürk H, Çatlı G, Dündar B. An assessment of retinal nerve fiber layer thickness in non-diabetic obese children and adolescents. JCRPE J Clin Res Pediatr Endocrinol. (2018) 10(1):13–8. doi: 10.4274/jcrpe.4810
49. Kurtul BE, Cąkmak AI, Elbeyli A, Karaaslan A, El Ç. Association of childhood obesity with retinal microvasculature and corneal endothelial cell morphology. J Pediatr Endocrinol Metab. (2020) 34(2):171–6. Available at: https://pubmed.ncbi.nlm.nih.gov/33544543/(cited 2022 Jan 30) doi: 10.1515/jpem-2020-0483
50. Nickla DL, Wallman J. THE MULTIFUNCTIONAL CHOROID. Prog Retin Eye Res. (2010) 29(2):144. Available at:/pmc/articles/PMC2913695/(cited 2022 Apr 24). doi: 10.1016/j.preteyeres.2009.12.002
51. Topcu-Yilmaz P, Akyurek N, Erdogan E. The effect of obesity and insulin resistance on macular choroidal thickness in a pediatric population as assessed by enhanced depth imaging optical coherence tomography. J Pediatr Endocrinol Metab. (2018) 31(8):855–60. doi: 10.1515/jpem-2018-0079
52. Erşan I, Battal F, Aylanç H, Kara S, Arikan S, Tekin M, et al. Noninvasive assessment of the retina and the choroid using enhanced-depth imaging optical coherence tomography shows microvascular impairments in childhood obesity. J AAPOS. (2016) 20(1):58–62. doi: 10.1016/j.jaapos.2015.10.006
53. Kur J, Newman EA, Chan-Ling T. Cellular and physiological mechanisms underlying blood flow regulation in the retina choroid in health disease. Prog Retin Eye Res. (2012) 31(5):377. Available at:/pmc/articles/PMC3418965/(cited 2021 Aug 30). doi: 10.1016/j.preteyeres.2012.04.004
54. Öztürk H, Özen B, Çatlı G, Dündar BN. Macular variability in children and adolescents with metabolic syndrome: a cross-sectional study examining the associations with anthropometric measurements, metabolic parameters and inflammatory markers. JCRPE J Clin Res Pediatr Endocrinol. (2020) 12(1):63–70. doi: 10.4274/jcrpe.galenos.2019.2019.0082
55. Teberik K, Eski MT, Doğan S, Pehlivan M, Kaya M. Ocular abnormalities in morbid obesity. Arq Bras Oftalmol. (2019) 82(1):6–11. doi: 10.5935/0004-2749.20190007
56. Agarwal A, Saini A, Mahajan S, Agrawal R, Cheung CY, Rastogi A, et al. Effect of weight loss on the retinochoroidal structural alterations among patients with exogenous obesity. PLoS One. (2020) 15(7):e0235926. Available at:/pmc/articles/PMC7347179/(cited 2021 Aug 30) doi: 10.1371/journal.pone.0235926
57. Celik G, Gunay M, Ozcabi B, Gulturk U, Kizilay O, Vural A, et al. Evaluation of the impact of childhood obesity on retrobulbar hemodynamics and retinal microvasculature. European Journal of Ophthalmology. (2022) 32(6):3556–63. (cited 2022 Mar 18). doi: 10.1177/11206721221086244
58. Han S, Leng Z, Li X, Yan W, Shen S, Liu L, et al. Retinochoroidal microvascular changes in newly developed obese children: an optical coherence tomography angiography study. BMC Ophthalmol. (2022) 22(1):1–6. Available at: https://bmcophthalmol.biomedcentral.com/articles/10.1186/s12886-022-02664-9(cited 2022 Dec 18) doi: 10.1186/s12886-021-02233-6
Keywords: childhood obesity, ocular signs, retinal microvasculature, intraocular pressure, retinal nerve fiber layer, optical coherence tomography
Citation: Dezor-Garus J, Niechciał E, Kędzia A and Gotz-Więckowska A (2023) Obesity-induced ocular changes in children and adolescents: A review. Front. Pediatr. 11:1133965. doi: 10.3389/fped.2023.1133965
Received: 29 December 2022; Accepted: 7 March 2023;
Published: 23 March 2023.
Edited by:
Giulio Maltoni, Sant'Orsola-Malpighi Polyclinic, ItalyReviewed by:
Humberto Hernandez, University of Houston Victoria, United StatesAndrea Vania, Sapienza University of Rome, Italy
© 2023 Dezor-Garus, Niechciał, Kędzia and Gotz-Więckowska. This is an open-access article distributed under the terms of the Creative Commons Attribution License (CC BY). The use, distribution or reproduction in other forums is permitted, provided the original author(s) and the copyright owner(s) are credited and that the original publication in this journal is cited, in accordance with accepted academic practice. No use, distribution or reproduction is permitted which does not comply with these terms.
*Correspondence: Julia Dezor-Garus anVsaWEuZGV6b3ItZ2FydXNAc2twcC5lZHUucGw=
Specialty Section: This article was submitted to Pediatric Obesity, a section of the journal Frontiers in Pediatrics