No neuroprotective effect of therapeutic hypothermia following lipopolysaccharide-sensitized hypoxia-ischemia: a newborn piglet study
- 1Department of Paediatrics and Adolescent Medicine, Aarhus University Hospital, Aarhus, Denmark
- 2Department of Clinical Medicine, Aarhus University, Aarhus, Denmark
- 3Medical Research Laboratory, Aarhus University, Aarhus, Denmark
- 4MR Research Centre, Aarhus University Hospital, Aarhus, Denmark
- 5Université Paris Cité, Inserm, NeuroDiderot, F-75019, Paris, France
Introduction: Therapeutic hypothermia is the only proven neuroprotective treatment for hypoxic-ischemic encephalopathy. However, studies have questioned whether therapeutic hypothermia may benefit newborns subjected to infection or inflammation before a hypoxic-ischemic insult. We aimed to compare newborn piglets with lipopolysaccharide-sensitized hypoxia-ischemia treated with and without therapeutic hypothermia with regards to measures of neuroprotection.
Methods: A total of 32 male and female piglets were included in this randomized experimental study. Lipopolysaccharides from Escherichia coli were infused intravenously before initiation of a standardized global hypoxic-ischemic insult. The piglets were then randomized to either normothermia or therapeutic hypothermia. After 14 h, the piglets were evaluated. Our primary outcome was brain lactate/N-acetylaspartate ratio assessed by magnetic resonance spectroscopy. Secondary outcomes included measures of magnetic resonance imaging, amplitude-integrated electroencephalography, immunohistochemistry, and concentration of blood cells and cytokines.
Results: Piglets treated with and without therapeutic hypothermia were subjected to comparable global hypoxic-ischemic insults. We found no difference between the two groups with regards to measures of magnetic resonance spectroscopy and imaging, amplitude-integrated electroencephalography, immunohistochemistry, and concentration of blood cells and cytokines.
Conclusion: We found no indication of neuroprotection by therapeutic hypothermia in newborn piglets following lipopolysaccharide-sensitized hypoxia-ischemia. However, interpretation of the results is limited by the short observation period. Further studies are required to determine the potential clinical implications of these findings.
1. Introduction
Neonatal encephalopathy due to intrapartum-related events is a severe clinical condition that affects over one million newborns each year (1). Hypoxia-ischemia (HI) is a common cause leading to what is termed hypoxic-ischemic encephalopathy (2). Therapeutic hypothermia (TH) is the only current neuroprotective treatment for hypoxic-ischemic encephalopathy, proven to be beneficial in several clinical randomized controlled trials (3). However, around 40%–50% of newborns treated with TH still die or suffer neurodevelopmental impairment (4, 5). Studies from low-income countries even suggest that TH may have adverse effects in these settings (6–8). Therefore, studies are needed to investigate why this treatment not always is associated with neuroprotection.
It has been proposed that perinatal infection and inflammation may act together with HI to create significant injury in the developing brain (9). Animal studies have found that sensitization before HI by bacterial endotoxins such as lipopolysaccharides (LPS) from Escherichia coli severely exacerbates newborn brain injuries (10–15). A previous piglet study found that LPS sensitization compared with HI alone affected brain expression of inflammatory markers and increased whole-brain cell death and mortality—with similar findings observed in several rat studies (10–15). This has been termed the multiple hit hypothesis, which postulates that an insult during pregnancy or labor may sensitize the fetal or neonatal brain for secondary insults to have larger clinical impact (9). In addition to the possible exacerbation of brain injury, animal studies have found TH with limited neuroprotective effect following LPS-sensitized HI (16–21). A previous piglet study and several rat studies found that TH failed to counteract the increased pathology by measures of magnetic resonance spectroscopy (MRS), amplitude-integrated electroencephalography (aEEG), neuronal cell death, and inflammatory cellular changes (16–21). TH may partly exert neuroprotection by affecting the immune system by suppressing proinflammatory cytokines and the activation of microglia in the brain (22). However, this immunomodulation could be altered when several inflammatory exposures are present simultaneous (23). As both infection and HI may be involved in the pathology of neonatal encephalopathy, several newborns may receive an ineffective or even deleterious treatment (24–26).
With exception of one other piglet study, current preclinical evidence on TH following inflammation-sensitized HI is based on rodent studies (16–21, 27). Thus, further studies in large animals are needed to fully evaluate the clinical practice of TH in newborns with moderate to severe encephalopathy in the context of infection. We aimed to investigate the neuroprotective effect of TH in newborn piglets following LPS-sensitized HI assessed by brain MRS and magnetic resonance imaging (MRI), aEEG, cerebral immunohistochemical markers, and concentrations of blood cells and cytokines.
2 Methods
2.1. Ethical statement
This study was approved by the Danish Animal Experiments Inspectorate (2019-15-0201-00232) and reported according to the Animal Research: Reporting of In Vivo Experiments (ARRIVE) guidelines (Supplementary Material S1) (28).
2.2. Study design
A randomized experimental animal study was performed (Figure 1). We included 32 Danish Landrace piglets of either sex, less than 24 h of age, and weighing between 1,200 and 2,300 g. The animals were provided from herds included in a health-monitoring program for slaughter pigs, screened for several pathogens that could affect pigs in a livestock production setting. On each experimental day, two piglets from the same litter were included. An intravenous infusion of LPS was initiated. After 4 h, the animals were exposed to global HI. One animal was then randomized to either normothermia (NT group) or hypothermia (TH group), while the sibling was allocated to the opposite group. The animals were then observed for 14 h with continuous aEEG before undergoing MRS and MRI. Our primary outcome was the thalamic lactate/N-acetylaspartate (Lac/NAA) ratio. After MR-scanning, the animals were euthanized by 80 mg/kg pentobarbital (Richter Pharma, Austria) and brain samples were acquired for immunohistochemical analyses. Two sham piglets—not subjected to LPS-infusion or hypoxia-ischemia—were also investigated with immunohistochemistry in an attempt to establish normal values. Blood samples were taken repeatedly during the experiments for analyses of blood cells and cytokines.
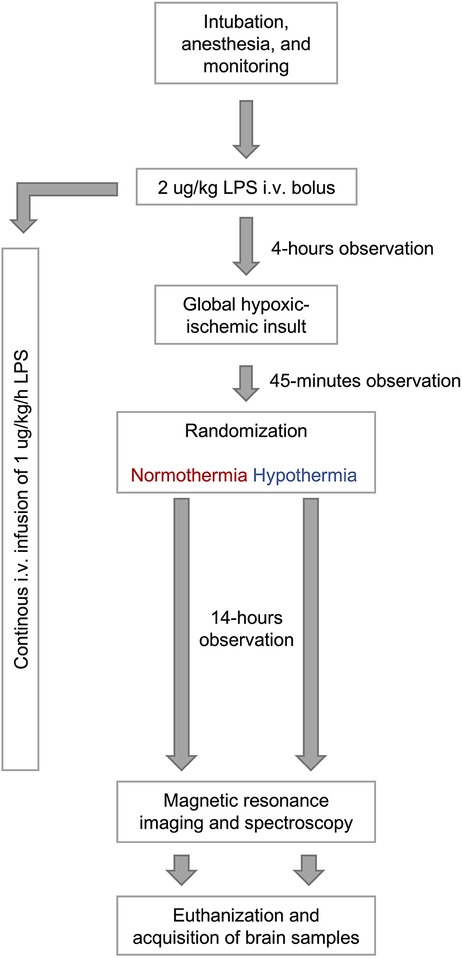
Figure 1. Overview of study investigating the neuroprotective effect of therapeutic hypothermia in newborn piglets with lipopolysaccharide-sensitized hypoxia-ischemia.
2.3. Experimental procedures
2.3.1. Anesthesia and monitoring
The animals were anesthetized by inhalation of 3%–4% sevoflurane (AbbVie, USA). Before endotracheal intubation, the animals were intravenously injected with 5 mg/kg propofol (Fresenius Kabi, Germany), 10 μg/kg fentanyl (B. Braun, Germany), and 1 mg/kg rocuronium (Hameln Pharma, Netherlands). Standard ventilator settings included fraction of inspired oxygen (FiO2) = 21%, respiratory frequency (RF) = 25/min, and peak inspiratory pressure = 15 cmH2O. These settings were continuously adjusted to maintain a saturation above 93% and end-tidal CO2 between 4.5 and 5.5 kPa. Anesthesia was initiated by intravenous infusion of 4–12 mg/kg/h propofol and 5–10 μg/kg/h fentanyl. To maintain hydration and glucose levels, the animals were given 10 ml/kg/h NeoKNaG (15 mmol/L Na+, 25 mmol/L Cl−, 10 mmol/L K+, 505 mmol/L glucose). Catheters were inserted in the umbilical artery and vein to monitor blood pressure, obtain blood samples, and administer drugs. Arterial blood gas samples were analyzed regularly for gas composition, electrolytes, glucose, lactate, and pH-values (ABL Radiometer Medical Denmark). Prophylactic antibiotic treatment was given once with 30 mg/kg ampicillin (Stada, Germany) and 5 mg/kg gentamycin (B. Braun, Germany). The rectal temperature was measured and maintained within the physiological range (38.5–39.5°C) by an air heated mattress. Electrodes for electrocardiography were placed to observe heart rate and rhythms.
2.3.2. Lipopolysaccharides
A bolus of 2 μg/kg LPS (serotype 055-B5) from Escherichia coli (Sigma, Germany) was given intravenously followed by continuous infusion of 1 μg/kg/h until MR-scanning.
2.3.3. Hypoxia and ischemia
Infusion of anesthetics and fluid was reduced to half the initial dose before HI to minimize accumulation and continued onward. A single-channel aEEG was attached to the scalp of the piglets to monitor brain activity (Niculet Monitor, Natus Medical Incorporated, USA). The HI insult was induced according to our protocol (29). FiO2 was reduced to 4% with RF of 16/min to obtain an upper trace of aEEG <7 μV. FiO2 was then adjusted to maintain the upper trace <7 μV for as long as possible within a 45-min period with a mean arterial blood pressure (MABP) <70% of baseline for 10 min. Resuscitation was initiated with FiO2 of 21% and RF of 30/min followed by titration to maintain adequate saturation.
2.3.4. Therapeutic hypothermia
Whole-body TH was initiated 45 min after HI with cooling elements until reaching target temperature of 33–34°C and then continued until euthanasia.
2.3.5. Hypotension
Hypotension was treated stepwise by (1) reducing infusion of propofol and fentanyl while still maintaining adequate sedation, (2) bolus infusion of 10 ml/kg isotonic NaCl solution, and (3) infusion of inotropies including 0.25–1.5 μg/kg/min norepinephrine (Macure Pharma, Denmark), 5–15 μg/kg/min dobutamine (Stada, Germany), 0.1–1.5 μg/kg/min epinephrine (Bradex S.A., Greece), and/or 2.5 mg/kg methylprednisolone (Solu-Medrol, Pfizer, USA). If these treatments were unable to maintain appropriate MABP, the animal was euthanized.
2.4. Experimental outcomes
2.4.1. Magnetic resonance spectroscopy and imaging
MRS and MRI were conducted as previously described with few adjustments (30, 31). Single voxel proton MRS (TR/TE 2,000/288 ms, 1,024 sample points, spectral width 1,200 Hz, 128 averages) was obtained from the thalamus (10 × 10 × 15 mm), frontal cortex (8 × 8 × 8 mm), occipital cortex (8 × 8 × 8 mm), and white matter (8 × 8 × 12 mm) using point-resolved spectroscopy (PRESS) acquisition (Supplementary Material S2). N-acetylaspartate (NAA, 2.02 ppm), lactate (Lac, 1.33 ppm), creatine (Cr, 3.02 ppm), and choline (Cho, 3.20 ppm) were identified. MRS data were analyzed with LCModel (Stephen Provencer, Canada) version 6.3-1l. MRS outcomes included Lac/NAA, NAA/Cr, and NAA/Cho ratios. Lac/NAA ratios were log-transformed to normalize the values. When lactate was below the detection level, the value was set to half the lowest value among the other piglets. The apparent diffusion coefficient (ADC) was calculated from diffusion weighted images by single-exponential fitting. Blood oxygen level dependent (BOLD) signals were obtained from T2*-maps acquired by multiecho gradient echo sequences by single-exponential fitting. ADC and BOLD images of the right thalamus were analyzed with Horos software (Annapolis, MD, USA) version 3.3.5. Cerebral blood flow was assessed by arterial spin-labelling (ASL) by subtracting control and label images and multiplying with the proper scaling factor. Whole-brain perfusion was calculated in three slices and averaged (Supplementary Material S3). Neuroimaging data were analyzed blinded to randomization.
2.4.2. Amplitude-integrated electroencephalography
aEEG was recorded continuously following HI. However, only one of the two animals on each experimental day was randomized to aEEG recording due to equipment limitations. Points were awarded for different patterns as previously described (32, 33); 0 points for flat trace, 1 point for continuous low voltage, 2 points for burst suppression, 3 points for discontinuous normal voltage, and 4 points for continuous normal voltage. Recordings were analyzed for each hour and then averaged for 0–7 h and 8–14 h. Seizures were identified clinically and based on the aEEG patterns (34). The aEEG recordings were reviewed and analyzed blinded to randomization.
2.4.3. Immunohistochemistry
After euthanasia, the right cerebral hemisphere was immersed in 4% formaldehyde for 7 days at 4°C. Each sample was dissected into coronal slices of 5 mm for dehydration and paraffin embedding. Sections were realized at 5 µm with a microtome. The primary antibodies included anti-Glial fibrillary acidic protein (GFAP, DAKO Z334, 1:1,000), anti-ionizing adaptor protein 1 (IBA1, Wako 019-19741; 1:500), and cleaved-caspase 3 antibody (CC3 Cell Signaling 9661; 1:1,500)—carried out on a Leica™ Bond-Max automat according to protocols routinely used (Bond Polymer Refine IHC protocol F). Sections were counterstained with Nissl labeling. For each immunohistochemical labeling, three slices (frontal, parietal, and occipital) per animal were scanned with NanoZoomer (Hamamatsu, Japan) at a magnification corresponding to 20× objective. For each slice, optical density was calculated from grayscale images standardized to the photomicrograph background as previously described by McAdams et al. (35) and Rangon et al. (36). For each animal, one measurement (1 mm2) in each region (thalamus, periventricular white matter, parietal cortex, caudate nucleus, putamen, capsula interna, capsula externa, and hippocampus) was made. For GFAP and IBA1 labeling, the intensity was calculated by densitometric analysis using the NIH ImageJ software (Fiji, NIH). For CC3 labeling, the number of cells per region was reported using the NIH ImageJ software (Fiji, NIH).
2.4.4. Blood cells and cytokines
Arterial blood samples were collected at baseline, 3 h after initiation of LPS infusion, immediately after HI, 6 h after HI, and 12 h after HI. The samples were analyzed by a veterinary hematology analyzer (ProCyte Dx, IDEXX, USA) to assess the concentration of red blood cells, platelets, total white blood cells, neutrophils, lymphocytes, and monocytes. Samples taken after HI were also centrifugated at 3,163 g at 18°C for 10 min and plasma was transferred to cryotubes and stored at −80°C for later assessment of cytokines. Cytokines were assessed with the Cytokine and Chemokine 9-plex Porcine ProcartaPlex (ThermoFisher, USA) including IL-1β, IL-4, IL-6, IL-8, IL-10, IL-12p40, IFN-α, IFN-γ, and TNF-α. Samples were measured in duplicates and analyzed using the Bio-Plex Manager 6.0 Software (BioRad, USA). If cytokines were below the detection level, they were replaced by half the detection limit.
2.5. Sample size
As our primary outcome was the thalamic Lac/NAA ratio, the sample size was based on a previous piglet study finding a difference in Lac/NAA ratio after 9.5 h between piglets with HI treated with and without TH (1.83 ± 0.31 vs. 2.35 ± 0.49) (37). When comparing the means provided by this study, 12 piglets should be included in each group to show a difference with a two-sided statistical significance level of 5% and a power of 80%. Based on previous studies by our group, we expected mortality of around 20% and therefore included 16 piglets in each group (38, 39).
2.6. Statistical methods
Independent and continuous data were analyzed by unpaired t-test (normally distributed) or Mann–Whitney test (non-normally distributed), while dependent data were analyzed by paired t-test. Categorical data were analyzed by Fisher's exact test. Outcomes with repeated measures were analyzed by mixed-effects analyses with assumed sphericity and randomly missing values followed by Fisher's LSD test. Normally distributed data are presented as mean values with standard deviations, while non-normally distributed data are presented as median values with interquartile ranges. Differences with a two-sided p-value less than 0.05 were considered statistically significant.
As blood glucose in piglets treated with TH was more than twice the value of that in the normothermic piglets before scanning, post-hoc multivariate linear regression was conducted with adjustment of these values for Lac/NAA ratios and immunohistochemical markers in the thalamus, white matter, and cortex. Statistical analyses were performed by GraphPad Prism version 8.0.0 for MacOS (GraphPad Software, San Diego, California USA, www.graphpad.com).
3. Results
3.1. Animal characteristics
A total of 15 female and 17 male piglets were included (NT = 7/9, TH = 8/8). We observed no difference in weight between piglets treated with and without TH (1,650 g vs. 1,775 g). The severity of the HI insult was similar between our two groups based on duration of aEEG <7 μV, duration of MABP <70% of baseline, and end-hypoxia pH and lactate (Table 1). Difficulties with titrating FiO2 to secure target MABP while still keeping the piglets alive during the HI insult resulted in durations of target MABP below the aim of 10 min. The piglets received similar total dose of anesthetics and inotropies (Supplementary Material S4). Vital signs and arterial blood values were similar between groups before LPS infusion and HI. At the end of observation, piglets treated with TH had lower temperature, heart rate, and p-sodium, but higher p-glucose and p-lactate (Table 2).
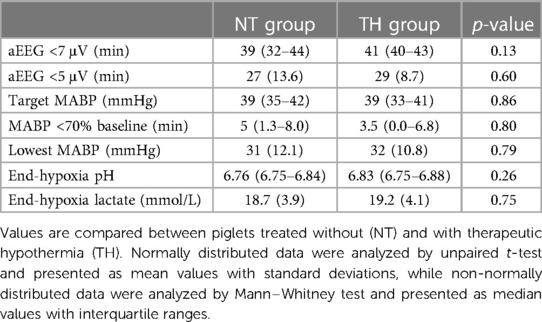
Table 1. The severity of the hypoxic-ischemic insult in newborn piglets following lipopolysaccharide-sensitization.
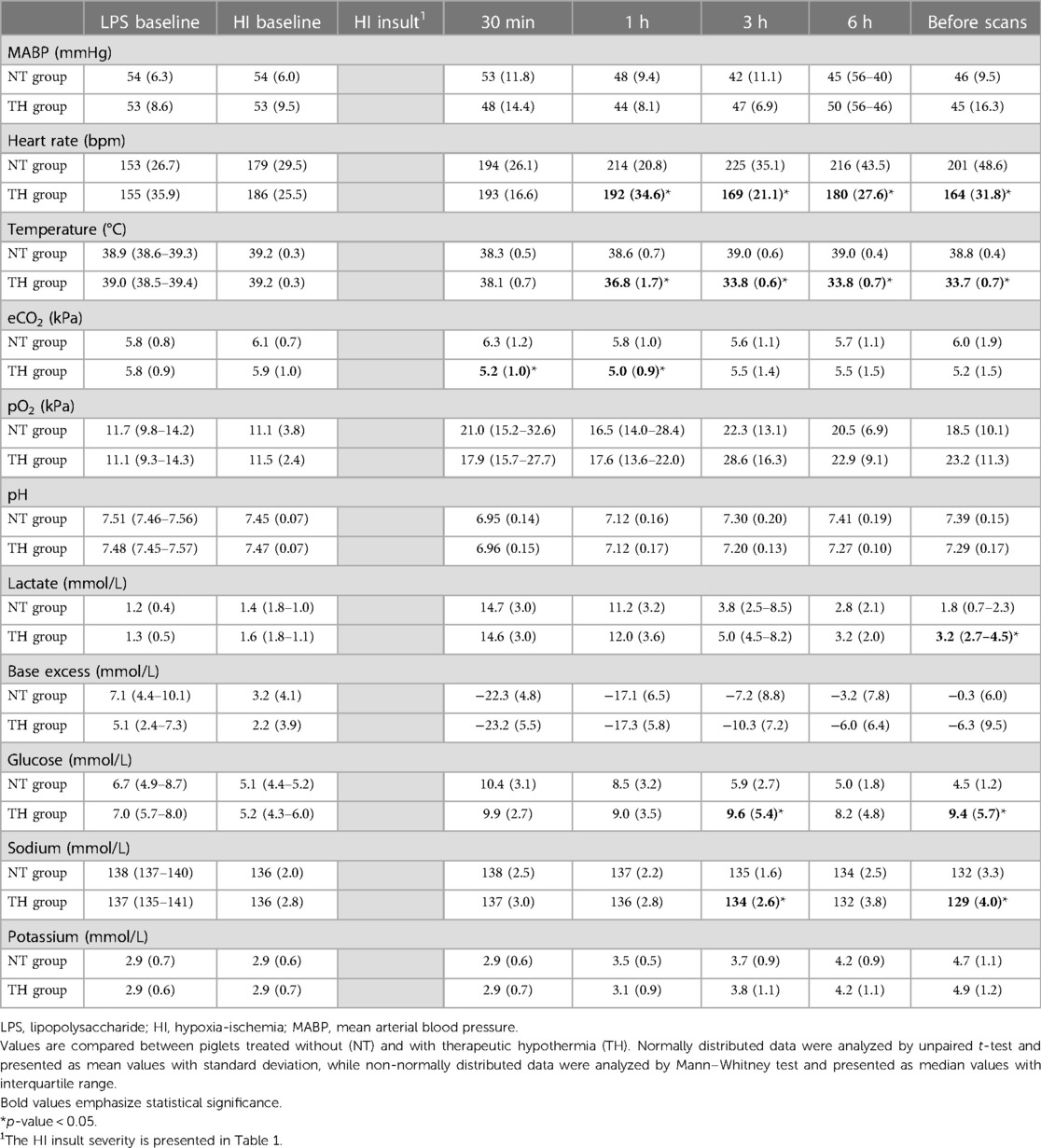
Table 2. Vital and arterial blood-gas values in newborn piglets subjected to lipopolysaccharide-sensitized hypoxia-ischemia.
3.2. Survival and missing information
A total of 12 piglets died during the experiments due to refractory hypotension (NT = 6, TH = 6). A total of 18 piglets were scanned (NT = 9, TH = 9). One piglet in each group survived the observation period but could not be scanned due to logistic difficulties. Technical issues resulted in missing MRS data from the frontal and occipital cortex in five piglets, missing ASL data in four piglets, and missing ADC and BOLD data in one piglet. Due to laboratory Covid-19 lockdown, five brain hemispheres were stored in phosphate-buffered saline for one-and-a-half month before embedding. Blood cell analyses were possible in 19 piglets (NT = 10, TH = 9) and cytokine analyses in 23 piglets (NT = 10, TH = 13).
3.3. Outcomes
3.3.1. Magnetic resonance spectroscopy and imaging
We found no statistically significant difference in Lac/NAA ratio in any brain region between piglets treated with and without TH (Figure 2). Furthermore, we found no difference in NAA/Cr or NAA/Cho ratios (Supplementary Material S5 and S6). At last, we found no difference in ADC, BOLD, or ASL values between the two groups (Figure 3).
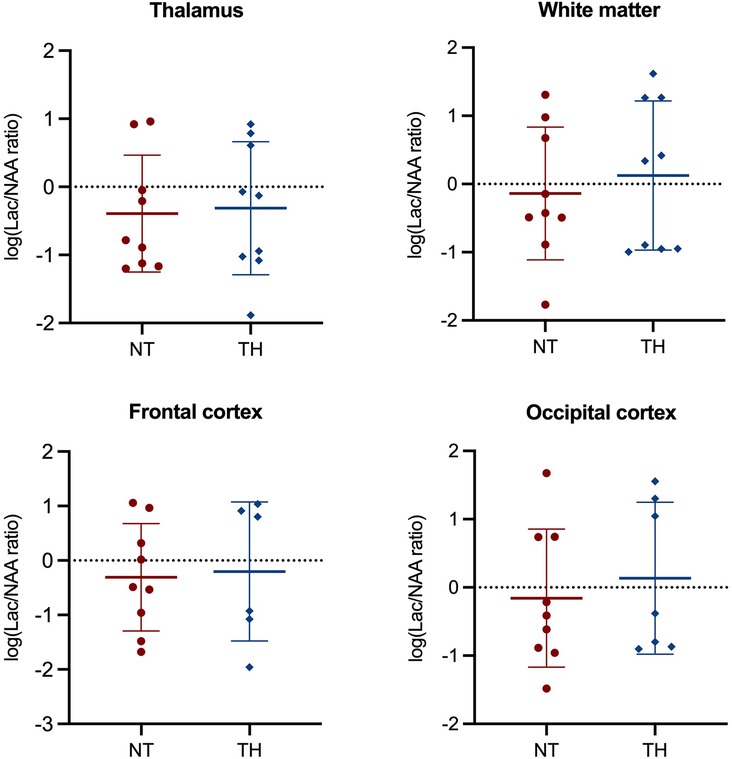
Figure 2. Magnetic resonance spectroscopy used to assess the Lac/NAA ratio in newborn piglets following lipopolysaccharide-sensitized hypoxia-ischemia. Values are compared between piglets treated without (NT) and with therapeutic hypothermia (TH). Data were log-transformed and analyzed by unpaired t-test and presented with mean values and standard deviation.
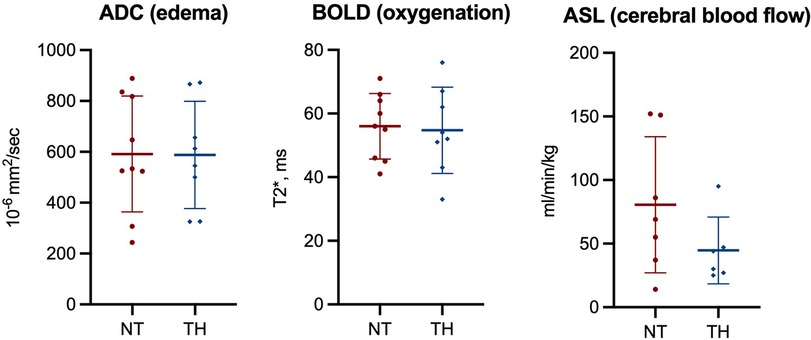
Figure 3. Magnetic resonance imaging used to assess apparent diffusion coefficient (ADC), blood oxygen level dependent (BOLD) signals, and cerebral blood flow (ASL) in piglets following lipopolysaccharide-sensitized hypoxia-ischemia. Values are compared between piglets treated without (NT) and with therapeutic hypothermia (TH). Data were analyzed by unpaired t-test and presented with mean values and standard deviation.
3.3.2. Amplitude-integrated electroencephalography
We found no statistically significant difference between piglets treated with and without TH in mean aEEG score from 1 to 7 h (1.8 vs. 1.6) and 8–14 h (2 vs. 2) (Supplementary Material S7). We observed no statistically significant difference in number of piglets with seizures (NT = 27%, TH = 43%).
3.3.3. Immunohistochemistry
Examples of immunohistochemical stainings are provided for each marker (Supplementary Material S8). We found no statistically significant difference in GFAP (density), IBA1 (density), or CC3 (cells/mm2) in any brain region between piglets treated with and without TH (Figure 4). The experimental piglets appeared to have similar values as the sham piglets (Figure 4).
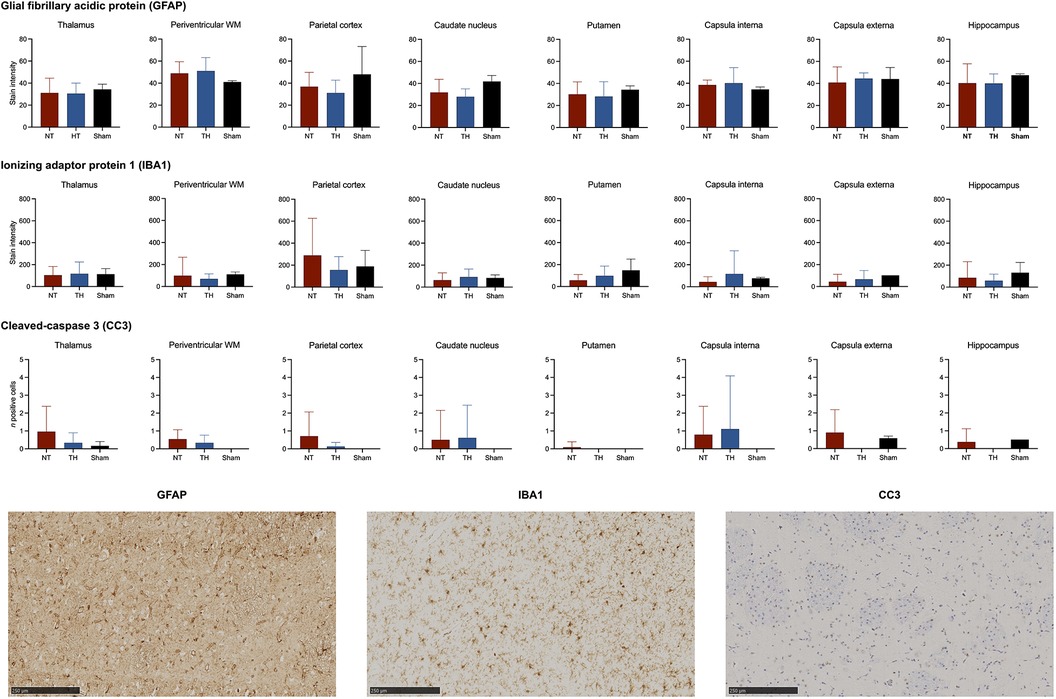
Figure 4. Glial fibrillary acidic protein (GFAP), ionized calcium-binding adaptor molecule (IBA1), and cleaved-caspase 3 (CC3) expressed in piglets following lipopolysaccharide-sensitized hypoxia-ischemia. Values are compared between piglets treated without (NT) and with therapeutic hypothermia (TH). Data were analyzed by unpaired t-test and presented with mean values and standard deviation.
3.3.4. Blood cells and cytokines
Comparisons of mean blood cells before and three hours after LPS infusion showed increased red blood cell counts (4.9 vs. 5.6 M/μl) and decreased platelets (254 vs. 183 K/μl), white blood cells (9.0 vs. 1.1 K/μl), neutrophils (6.1 vs. 0.4 K/μl), lymphocytes (2.6 vs. 0.7 K/μl), and monocytes (0.21 vs. 0.01 K/μl). Following the HI insult, the concentration of platelets decreased, while the concentration of white blood cells increased. We found no statistically significant difference in concentration of blood cells between piglets treated with and without TH (Figure 5). Similarly, we observed no overall difference in concentrations of cytokines between our two groups (Figure 6). However, we found statistically significant higher mean values of IL-6 after 12 h in piglets treated with TH (170 vs. 535 pg/ml, p-value = 0.05). The concentration of IL-6, IL-12p40, and TNF-α decreased over time, while the concentration of IFN- γ increased until the 6-h assessment and then decreased.
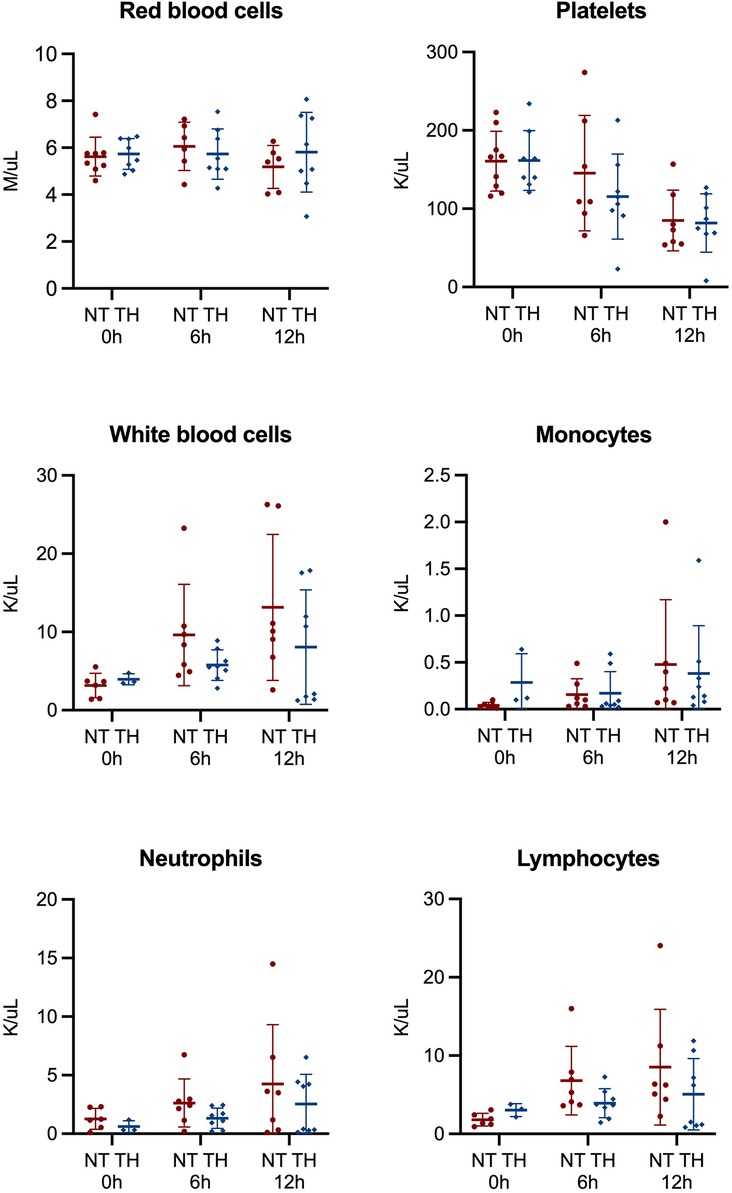
Figure 5. Cells analyzed from blood samples 0, 6, and 12 h in newborn piglets following lipopolysaccharide-sensitized hypoxia-ischemia. Values are compared between piglets treated without (NT) and with therapeutic hypothermia (TH). Data were analyzed by mixed-effect analysis followed by Fisher's LSD test and presented with mean values and standard deviation.
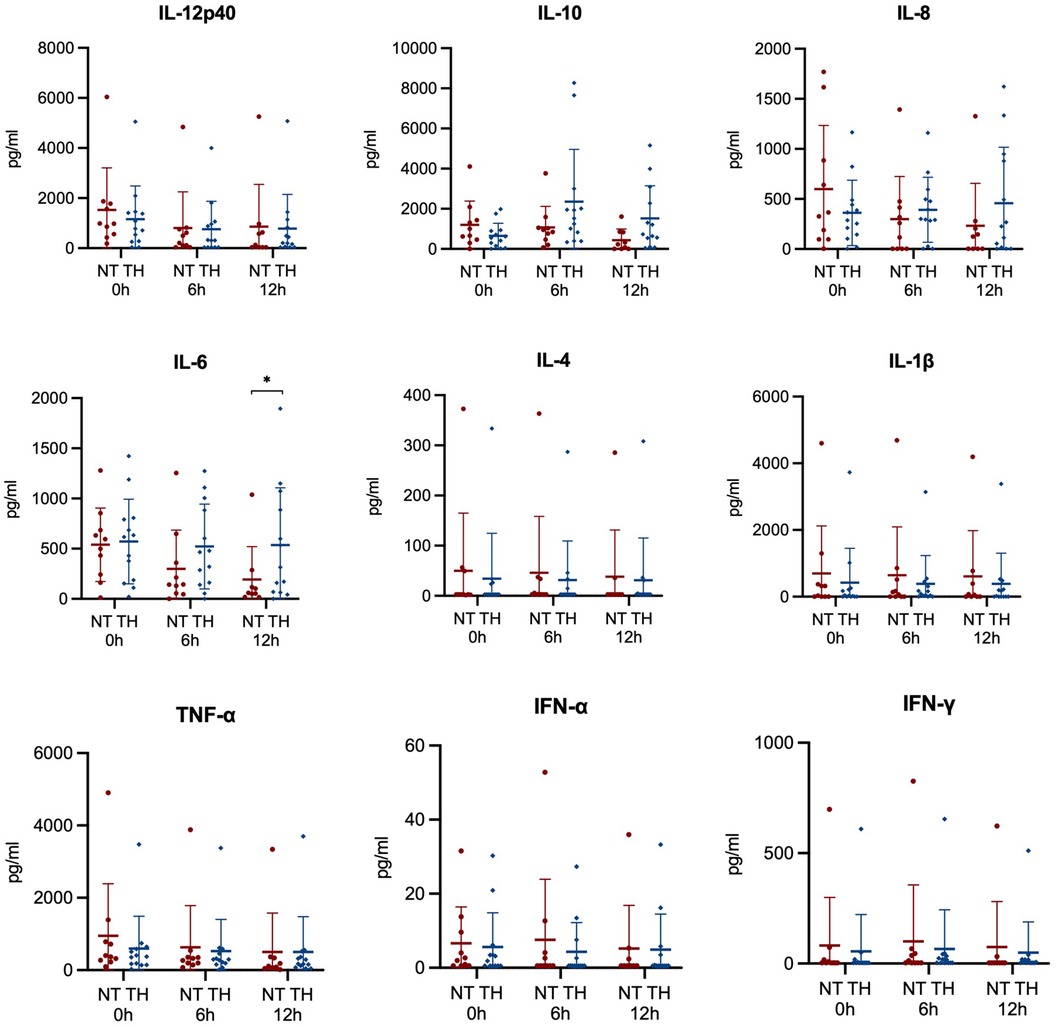
Figure 6. Cytokines analyzed from blood samples 0, 6, and 12 h in newborn piglets following lipopolysaccharide-sensitized hypoxia-ischemia. Values are compared between piglets treated without (NT) and with therapeutic hypothermia (TH). Data were analyzed by mixed-effect analysis followed by Fisher's LSD test and presented with mean values and standard deviations. *p-value < 0.05.
3.3.5. Hyperglycemia
Adjustment for blood glucose before scanning failed to reveal any statistically significant difference between piglets treated with and without TH in Lac/NAA ratios and immunohistochemical markers in the thalamus, white matter, and cortex (Supplementary Material S9).
4. Discussion
4.1. Summary of findings
We investigated the neuroprotective effect of TH following LPS-sensitized HI in a randomized experimental piglet study. We found no indication of early neuroprotection by measures of MRS, MRI, aEEG, immunohistochemistry, and concentration of blood cells and cytokines. The piglet study of Martinello et al. (21) also found TH without neuroprotective effects following LPS-sensitized HI. They investigated similar outcomes at 48 h following rewarming, which may be more appropriate timing for detecting brain injury due to the evolving pathology following HI (40, 41). However, our study adds to their findings as we used a different protocol for global hypoxia-ischemia and included a larger population of piglets with both males and females (42). Our studies may indicate that TH is not neuroprotective when gram-negative infection or inflammation occurs before a HI insult in the human fetus or newborn.
The Lac/NAA ratio is considered one of the most accurate predictors of neurodevelopmental impairment in human newborns with neonatal encephalopathy (43). Furthermore, piglet studies with and without LPS-sensitization have shown the Lac/NAA ratio to correlate with both neuronal cell death and microglia activation at 24 and 48 h after HI (44). Even though the Lac/NAA ratio was pathologically increased in most piglets, we found no differences in Lac/NAA ratio in any brain region in piglets treated with and without TH (43). The Lac/NAA ratio represents secondary energy failure, which is thought to begin 6 to 24 h following HI (40, 41). An effect of TH may accordingly have been revealed if MRS had been conducted at later timepoints rather than at 14 h after HI. However, Martinello et al. (21) found no difference in brain Lac/NAA ratio at 48 h after LPS-sensitized HI.
We used GFAP as a marker of astrogliosis, IBA1 as a marker of microgliosis, and CC3 as a marker of apoptosis. Both astroglia and microglia may induce brain inflammation following LPS exposure as shown in vitro (45). This may further lead to activation of apoptotic pathways and possibly cell death (46). Another study found an increased expression of these markers in LPS-sensitized piglets after 48-h observation when compared with piglets subjected to HI alone—indicating an exacerbating effect of LPS-sensitization (15). However, we found no difference in these markers when comparing LPS-sensitized piglets treated with and without TH. As for the Lac/NAA ratio, these findings of no difference may also be explained by our early time of assessment. This is further supported by the lack of difference between the experimental piglets and the two sham piglets. Rodent models have indicated that the expression of GFAP, IBA1, and CC3 may peak at least 24 h after HI (37, 47–49). However, TH has been shown to reduce brain expression of CC3 and TNF-α by Western Blotting already after 6 h in another piglet model of HI (50), while microglial activation may occur already 6 h after initiation of LPS exposure (51). In this study, the total duration of LPS exposure was 19 h. We are unable to rule out an effect of TH at later time points, but other studies with longer observation periods have observed similar findings of no difference (16, 21). The rat study by Osredskar et al. (16) found TH to be unable to counteract the LPS-associated increase in brain expression of GFAP, IBA1, and CC3 with assessments after 24 h and 1 week. Martinello et al. (21) similarly found no difference in brain expression of GFAP and IBA1 at 48 h with exception of an increased IBA1 in the caudate nucleus in normothermic piglets. However, they did find that normothermic piglets had increased expression of CC3 in their whole brain analysis, though with reports of CC3 being a poor marker of neuronal cell death in their model (44). Studies have found TH to suppress both astroglia and microglia activity in animal models of HI alone (52, 53).
To validate the effect of LPS on the immune system, we assessed white blood cells and platelets before and after LPS infusion—finding that LPS was associated with decreased concentration of both cell types. Similar to Martinello et al. (21), we found TH to have no effect on the concentration of these blood cells during our observation period following HI. Clinical studies of neonatal encephalopathy with follow-up from 0 to 7 days of life have found TH to be associated with reduced concentration of white blood cells (54, 55). Though, one study found TH to have no significant effect on white blood cells in newborns with neonatal encephalopathy and sepsis risk factors (54). TH may exert neuroprotection by modulating the production of cytokines (56, 57). Among proinflammatory cytokines, especially TNF-α and IL-1β may be involved in the pathology of LPS-sensitized HI as inhibition of these cytokines seems to eliminate the sensitizing effect of LPS (58–61). We found no difference in any cytokines between piglets treated with and without TH with exception of an increased IL-6 after 12 h in piglets treated with TH. Martinello et al. 21) also found no difference in plasma concentration of TNF-α, while Chevin et al. (18) found no difference in brain expression of both TNF-α and IL-1β in LPS-sensitized newborn rats treated with and without TH. Other piglet studies of HI alone have found TH to reduce the expression of TNF-α in both blood and brain (37, 50, 62).
Together with the current literature, our findings suggest that hypothermia may be unable to suppress the immune system when several inflammatory exposures are present in newborns (16, 21). Immunomodulation has previously been suggested as one of the neuroprotective mechanisms of TH, which thereby indicates that other treatments may be of value in these situations (22). A previous rat study by Bark et al. (63) investigated the neuroprotective effect of azithromycin in newborn rats subjected to HI sensitized by either LPS or Pam3Cys-Ser-(Lys)4. They found that azithromycin was associated with improved sensorimotor function, brain tissue preservation, and survival at 14 and 28 days after the insult. This may deserve further investigation in larger animal models to thoroughly evaluate its potential.
4.2. Limitations and translation
We present a piglet model with both male and female animals subjected to a standardized global HI insult. The severity of the insult was similar between piglets treated with and without TH as indicated by the duration of aEEG and MABP suppression during the insult as well as pH and p-lactate immediately after the insult. Furthermore, several outcomes were evaluated including both brain imaging, electroencephalography, cerebral immunohistochemical markers, and the systemic effect by blood cells and cytokines. However, this study also has some limitations. As mentioned, our markers of brain injury may have differed had they been assessed closer to the secondary phase of injury at 48–72 h (40, 41). This severely limits a statement of no neuroprotective effect by TH in this model. Though, other piglet studies have revealed neuroprotective effects of TH following HI alone with assessments between 6 and 12 h after the insult (37, 50). Additionally, this study could have benefitted from other markers of cell death including TUNEL-staining. Other piglet studies have found high correlation between the Lac/NAA ratio at 24 and 48 h and cell death evaluated by TUNEL-staining at 48 h (44). Another study in newborn piglets found LPS sensitization to exacerbate brain injuries following HI at 48 h (15). We did not compare markers of brain injury between piglets subjected to HI with and without LPS-sensitization. However, similar doses were used as the previous study and the systemic effect of LPS was validated by changes in blood cells following the infusion (21). The clinical translation may also have some limitations. In a recent systematic review, we found similar neurological outcome in newborns with neonatal encephalopathy treated with TH with and without markers of perinatal infection, though based on very low quality of evidence (64). Clinical populations are often more heterogenous than animals in experimental settings and several factors may affect the interaction between the different etiologies and treatments. LPS sensitization has actually shown neuroprotective effects when initiated 24 h before HI (10, 65), indicating that both a negative and positive conditioning may occur in the newborn brain (66). Furthermore, the effect of TH seems to vary according to pathogen. Studies in newborn rats have found neuroprotection by TH following HI sensitized by gram-positive endotoxins (20, 27). These differences may be explained by the different properties of endotoxins from gram-negative and gram-positive bacteria with the earlier activating toll-like-receptors 4 and the later activating toll-like receptors 2 (67, 68). However, the neuroprotective effects of TH following gram-positive sensitization are yet to be investigated in larger animal models. At last, the neuroprotective effect of TH following LPS-sensitized HI has to be considered with regards to gestational ages (69). Studies in newborn rats have investigated LPS sensitization in a wide variety of ages including postnatal day 1 (60, 70, 71), postnatal day 7–8 (61, 72–79), and postnatal day 12 (58, 59), somewhat corresponding to the brain development in preterm-, early term-, and full term human newborns (80). Studies in rats at postnatal day 12 have found TH with some neuroprotective effects following LPS-sensitized HI, showing reduced penumbra injuries and increased levels of antioxidant enzymes (18, 19). In our piglet study, the brain development similarly corresponds to what is seen in full term human newborns (81).
5. Conclusion
We found no difference between piglets with LPS-sensitized HI treated with and without TH based on measures of MRS, MRI, aEEG, immunohistochemistry, and concentration of blood cells and cytokines. However, our results may be limited by our early time of assessment of the brain. This may nonetheless indicate that other treatments than TH may be of value in newborns exposed to gram-negative infection or inflammation before a HI insult.
Data availability statement
The original contributions presented in the study are included in the article/Supplementary Material, further inquiries can be directed to the corresponding author.
Ethics statement
The animal study was approved by The Danish Animal Experiments Inspectorate. The study was conducted in accordance with the local legislation and institutional requirements.
Author contributions
MA: Conceptualization, Data curation, Formal Analysis, Funding acquisition, Investigation, Methodology, Project administration, Visualization, Writing – original draft, Writing – review & editing, Validation. HA: Investigation, Supervision, Writing – review & editing, Writing – original draft. TA: Conceptualization, Investigation, Supervision, Writing – original draft, Writing – review & editing, Methodology. LH: Investigation, Writing – review & editing, Writing – original draft. RP: Investigation, Writing – review & editing, Writing – original draft. MB: Investigation, Methodology, Writing – review & editing, Data curation, Resources, Supervision, Writing – original draft. SR: Methodology, Writing – review & editing, Resources, Software, Supervision, Writing – original draft. LS: Investigation, Methodology, Writing – original draft, Writing – review & editing, Data curation, Resources. PG: Methodology, Supervision, Writing – review & editing, Resources, Writing – original draft. KK: Conceptualization, Methodology, Supervision, Writing – review & editing, Writing – original draft. TH: Conceptualization, Funding acquisition, Methodology, Supervision, Writing – review & editing, Writing – original draft.
Funding
The author(s) declare financial support was received for the research, authorship, and/or publication of this article.
This study was funded by Aarhus University (no grant number), Elsass Foundation (19-3-0577), ESPR Young Investigator START-UP Awards 2019 (no grant number), Grosserer Chr. Andersen og hustru Ingeborg Andersen, f. Schmidts legat (no grant number), Lizzi og Mogens Staal Fonden (2019-0416), Torben og Alice Frimodts Fond (no grant number), Dagmar Marshalls Fond (500020), Oda og Hans Svenningsens Fond (no grant number), Helga og Peter Kornings Fond (DC472123-004-18), Aase og Ejnar Danielsens Fond (19-10-0386).
Acknowledgments
We would like to acknowledge the laboratory staff at the Department of Clinical Medicine, Aarhus University Hospital, Aarhus, Denmark.
Conflict of interest
The authors declare that the research was conducted in the absence of any commercial or financial relationships that could be construed as a potential conflict of interest.
Publisher's note
All claims expressed in this article are solely those of the authors and do not necessarily represent those of their affiliated organizations, or those of the publisher, the editors and the reviewers. Any product that may be evaluated in this article, or claim that may be made by its manufacturer, is not guaranteed or endorsed by the publisher.
Supplementary material
The Supplementary Material for this article can be found online at: https://www.frontiersin.org/articles/10.3389/fped.2023.1268237/full#supplementary-material
References
1. Lee AC, Kozuki N, Blencowe H, Vos T, Bahalim A, Darmstadt GL, et al. Intrapartum-related neonatal encephalopathy incidence and impairment at regional and global levels for 2010 with trends from 1990. Pediatr Res. (2013) 74(Suppl 1):50–72. doi: 10.1038/pr.2013.206
2. Kurinczuk JJ, White-Koning M, Badawi N. Epidemiology of neonatal encephalopathy and hypoxic-ischaemic encephalopathy. Early Hum Dev. (2010) 86(6):329–38. doi: 10.1016/j.earlhumdev.2010.05.010
3. Jacobs SE, Berg M, Hunt R, Tarnow-Mordi WO, Inder TE, Davis PG. Cooling for newborns with hypoxic ischaemic encephalopathy. Cochrane Database Syst Rev. (2013) 1:CD003311. doi: 10.1002/14651858
4. Edwards AD, Brocklehurst P, Gunn AJ, Halliday H, Juszczak E, Levene M, et al. Neurological outcomes at 18 months of age after moderate hypothermia for perinatal hypoxic ischaemic encephalopathy: synthesis and meta-analysis of trial data. BMJ. (2010) 340:c363. doi: 10.1136/bmj.c363
5. Ouwehand S, Smidt LCA, Dudink J, Benders M, de Vries LS, Groenendaal F, et al. Predictors of outcomes in hypoxic-ischemic encephalopathy following hypothermia: a meta-analysis. Neonatology. (2020) 117:1–17. doi: 10.1159/000505519
6. Thayyil S, Pant S, Montaldo P, Shukla D, Oliveira V, Ivain P, et al. Hypothermia for moderate or severe neonatal encephalopathy in low-income and middle-income countries (HELIX): a randomised controlled trial in India, Sri Lanka, and Bangladesh. Lancet Glob Health. (2021) 9(9):e1273–85. doi: 10.1016/S2214-109X(21)00264-3
7. Pauliah SS, Shankaran S, Wade A, Cady EB, Thayyil S. Therapeutic hypothermia for neonatal encephalopathy in low- and middle-income countries: a systematic review and meta-analysis. PLoS One. (2013) 8(3):e58834. doi: 10.1371/journal.pone.0058834
8. Bellos I, Devi U, Pandita A. Therapeutic hypothermia for neonatal encephalopathy in low- and middle-income countries: a meta-analysis. Neonatology. (2022) 119(3):300–10. doi: 10.1159/000522317
9. Fleiss B, Tann CJ, Degos V, Sigaut S, Van Steenwinckel J, Schang AL, et al. Inflammation-induced sensitization of the brain in term infants. Dev Med Child Neurol. (2015) 57(Suppl 3):17–28. doi: 10.1111/dmcn.12723
10. Eklind S, Mallard C, Arvidsson P, Hagberg H. Lipopolysaccharide induces both a primary and a secondary phase of sensitization in the developing rat brain. Pediatr Res. (2005) 58(1):112–6. doi: 10.1203/01.PDR.0000163513.03619.8D
11. Eklind S, Mallard C, Leverin AL, Gilland E, Blomgren K, Mattsby-Baltzer I, et al. Bacterial endotoxin sensitizes the immature brain to hypoxic–ischaemic injury. Eur J Neurosci. (2001) 13(6):1101–6. doi: 10.1046/j.0953-816x.2001.01474.x
12. Yang L, Sameshima H, Ikeda T, Ikenoue T. Lipopolysaccharide administration enhances hypoxic-ischemic brain damage in newborn rats. J Obstet Gynaecol Res. (2004) 30(2):142–7. doi: 10.1111/j.1447-0756.2003.00174.x
13. Ikeda T, Mishima K, Aoo N, Egashira N, Iwasaki K, Fujiwara M, et al. Combination treatment of neonatal rats with hypoxia-ischemia and endotoxin induces long-lasting memory and learning impairment that is associated with extended cerebral damage. Am J Obstet Gynecol. (2004) 191(6):2132–41. doi: 10.1016/j.ajog.2004.04.039
14. Froen JF, Amerio G, Stray-Pedersen B, Saugstad OD. Detrimental effects of nicotine and endotoxin in the newborn piglet brain during severe hypoxemia. Biol Neonate. (2002) 82(3):188–96. doi: 10.1159/000063610
15. Martinello KA, Meehan C, Avdic-Belltheus A, Lingam I, Ragab S, Hristova M, et al. Acute LPS sensitization and continuous infusion exacerbates hypoxic brain injury in a piglet model of neonatal encephalopathy. Sci Rep. (2019) 9(1):10184. doi: 10.1038/s41598-019-46488-y
16. Osredkar D, Sabir H, Falck M, Wood T, Maes E, Flatebo T, et al. Hypothermia does not reverse cellular responses caused by lipopolysaccharide in neonatal hypoxic-ischaemic brain injury. Dev Neurosci. (2015) 37(4–5):390–7. doi: 10.1159/000430860
17. Osredkar D, Thoresen M, Maes E, Flatebo T, Elstad M, Sabir H. Hypothermia is not neuroprotective after infection-sensitized neonatal hypoxic-ischemic brain injury. Resuscitation. (2014) 85(4):567–72. doi: 10.1016/j.resuscitation.2013.12.006
18. Chevin M, Guiraut C, Maurice-Gelinas C, Deslauriers J, Grignon S, Sebire G. Neuroprotective effects of hypothermia in inflammatory-sensitized hypoxic-ischemic encephalopathy. Int J Dev Neurosci. (2016) 55:1–8. doi: 10.1016/j.ijdevneu.2016.09.002
19. Chevin M, Guiraut C, Sebire G. Effect of hypothermia on interleukin-1 receptor antagonist pharmacodynamics in inflammatory-sensitized hypoxic-ischemic encephalopathy of term newborns. J Neuroinflammation. (2018) 15(1):214. doi: 10.1186/s12974-018-1258-6
20. Falck M, Osredkar D, Maes E, Flatebo T, Wood TR, Sabir H, et al. Hypothermic neuronal rescue from infection-sensitised hypoxic-ischaemic brain injury is pathogen dependent. Dev Neurosci. (2017) 39(1–4):238–47. doi: 10.1159/000455838
21. Martinello KA, Meehan C, Avdic-Belltheus A, Lingam I, Mutshiya T, Yang Q, et al. Hypothermia is not therapeutic in a neonatal piglet model of inflammation-sensitized hypoxia-ischemia. Pediatr Res. (2021) 91:1416–27. doi: 10.1038/s41390-021-01584-6
22. Drury PP, Gunn ER, Bennet L, Gunn AJ. Mechanisms of hypothermic neuroprotection. Clin Perinatol. (2014) 41(1):161–75. doi: 10.1016/j.clp.2013.10.005
23. Sabir H, Bonifacio SL, Gunn AJ, Thoresen M, Chalak LF. Unanswered questions regarding therapeutic hypothermia for neonates with neonatal encephalopathy. Semin Fetal Neonatal Med. (2021) 26(5):101257. doi: 10.1016/j.siny.2021.101257
24. Graham EM, Ruis KA, Hartman AL, Northington FJ, Fox HE. A systematic review of the role of intrapartum hypoxia-ischemia in the causation of neonatal encephalopathy. Am J Obstet Gynecol. (2008) 199(6):587–95. doi: 10.1016/j.ajog.2008.06.094
25. Rossi AC, Prefumo F. Antepartum and intrapartum risk factors for neonatal hypoxic-ischemic encephalopathy: a systematic review with meta-analysis. Curr Opin Obstet Gynecol. (2019) 31(6):410–7. doi: 10.1097/GCO.0000000000000581
26. Tann CJ, Nkurunziza P, Nakakeeto M, Oweka J, Kurinczuk JJ, Were J, et al. Prevalence of bloodstream pathogens is higher in neonatal encephalopathy cases vs. controls using a novel panel of real-time PCR assays. PLoS One. (2014) 9(5):e97259. doi: 10.1371/journal.pone.0097259
27. Falck M, Osredkar D, Maes E, Flatebo T, Wood TR, Walloe L, et al. Hypothermia is neuroprotective after severe hypoxic-ischaemic brain injury in neonatal rats pre-exposed to PAM3CSK4. Dev Neurosci. (2018) 40(3):189–97. doi: 10.1159/000487798
28. Kilkenny C, Browne WJ, Cuthill IC, Emerson M, Altman DG. Improving bioscience research reporting: the ARRIVE guidelines for reporting animal research. J Pharmacol Pharmacother. (2010) 1(2):94–9. doi: 10.4103/0976-500X.72351
29. Kyng KJ, Skajaa T, Kerrn-Jespersen S, Andreassen CS, Bennedsgaard K, Henriksen TB. A piglet model of neonatal hypoxic-ischemic encephalopathy. J Vis Exp. (2015) 99:e52454. doi: 10.3791/52454
30. Andersen HB, Andersen M, Andelius TCK, Pedersen MV, Løfgren B, Pedersen M, et al. Epinephrine vs placebo in neonatal resuscitation: rOSC and brain MRS/MRI in term piglets. Pediatr Res. (2023) 93(3):511–9. doi: 10.1038/s41390-022-02126-4
31. Kerrn-Jespersen S, Andersen M, Bennedsgaard K, Andelius TCK, Pedersen M, Kyng KJ, et al. Remote ischemic postconditioning increased cerebral blood flow and oxygenation assessed by magnetic resonance imaging in newborn piglets after hypoxia-ischemia. Front Pediatr. (2022) 10:933962. doi: 10.3389/fped.2022.933962
32. Hellstrom-Westas L, Rosen I, Svenningsen NW. Predictive value of early continuous amplitude integrated EEG recordings on outcome after severe birth asphyxia in full term infants. Arch Dis Child Fetal Neonatal Ed. (1995) 72(1):F34–8. doi: 10.1136/fn.72.1.F34
33. Robertson NJ, Martinello K, Lingam I, Avdic-Belltheus A, Meehan C, Alonso-Alconada D, et al. Melatonin as an adjunct to therapeutic hypothermia in a piglet model of neonatal encephalopathy: a translational study. Neurobiol Dis. (2019) 121:240–51. doi: 10.1016/j.nbd.2018.10.004
34. Tsuchida TN, Wusthoff CJ, Shellhaas RA, Abend NS, Hahn CD, Sullivan JE, et al. American clinical neurophysiology society standardized EEG terminology and categorization for the description of continuous EEG monitoring in neonates: report of the American clinical neurophysiology society critical care monitoring committee. J Clin Neurophysiol. (2013) 30(2):161–73. doi: 10.1097/WNP.0b013e3182872b24
35. McAdams RM, Fleiss B, Traudt C, Schwendimann L, Snyder JM, Haynes RL, et al. Long-term neuropathological changes associated with cerebral palsy in a nonhuman primate model of hypoxic-ischemic encephalopathy. Dev Neurosci. (2017) 39(1–4):124–40. doi: 10.1159/000470903
36. Rangon CM, Schang AL, Van Steenwinckel J, Schwendimann L, Lebon S, Fu TT, et al. Myelination induction by a histamine H3 receptor antagonist in a mouse model of preterm white matter injury. Brain Behav Immun. (2018) 74:265–76. doi: 10.1016/j.bbi.2018.09.017
37. Huun MU, Garberg H, Loberg EM, Escobar J, Martinez-Orgado J, Saugstad OD, et al. DHA and therapeutic hypothermia in a short-term follow-up piglet model of hypoxia-ischemia: effects on H + MRS biomarkers. PLoS One. (2018) 13(8):e0201895. doi: 10.1371/journal.pone.0201895
38. Andelius TCK, Pedersen MV, Andersen HB, Andersen M, Hjortdal VE, Pedersen M, et al. No added neuroprotective effect of remote ischemic postconditioning and therapeutic hypothermia after mild hypoxia-ischemia in a piglet model. Front Pediatr. (2020) 8:299. doi: 10.3389/fped.2020.00299
39. Kyng KJ, Kerrn-Jespersen S, Bennedsgaard K, Skajaa T, Pedersen M, Holm IE, et al. Short-term outcomes of remote ischemic postconditioning 1 h after perinatal hypoxia-ischemia in term piglets. Pediatr Res. (2020) 89:150–56. doi: 10.1038/s41390-020-0878-6
40. Hassell KJ, Ezzati M, Alonso-Alconada D, Hausenloy DJ, Robertson NJ. New horizons for newborn brain protection: enhancing endogenous neuroprotection. Arch Dis Child Fetal Neonatal Ed. (2015) 100(6):F541–52. doi: 10.1136/archdischild-2014-306284
41. Lorek A, Takei Y, Cady EB, Wyatt JS, Penrice J, Edwards AD, et al. Delayed (“secondary”) cerebral energy failure after acute hypoxia-ischemia in the newborn piglet: continuous 48-hour studies by phosphorus magnetic resonance spectroscopy. Pediatr Res. (1994) 36(6):699–706. doi: 10.1203/00006450-199412000-00003
42. Charriaut-Marlangue C, Besson VC, Baud O. Sexually dimorphic outcomes after neonatal stroke and hypoxia-ischemia. Int J Mol Sci. (2017) 19(1). doi: 10.3390/ijms19010061
43. Lally PJ, Montaldo P, Oliveira V, Soe A, Swamy R, Bassett P, et al. Magnetic resonance spectroscopy assessment of brain injury after moderate hypothermia in neonatal encephalopathy: a prospective multicentre cohort study. Lancet Neurol. (2019) 18(1):35–45. doi: 10.1016/S1474-4422(18)30325-9
44. Pang R, Martinello KA, Meehan C, Avdic-Belltheus A, Lingam I, Sokolska M, et al. Proton magnetic resonance spectroscopy lactate/N-acetylaspartate within 48 h predicts cell death following varied neuroprotective interventions in a piglet model of hypoxia–ischemia with and without inflammation-sensitization. Front Neurol. (2020) 11(883). doi: 10.3389/fneur.2020.00883
45. Barbierato M, Facci L, Argentini C, Marinelli C, Skaper SD, Giusti P. Astrocyte-microglia cooperation in the expression of a pro-inflammatory phenotype. CNS Neurol Disord Drug Targets. (2013) 12(5):608–18. doi: 10.2174/18715273113129990064
46. Eklind S, Hagberg H, Wang X, Sävman K, Leverin AL, Hedtjärn M, et al. Effect of lipopolysaccharide on global gene expression in the immature rat brain. Pediatr Res. (2006) 60(2):161–8. doi: 10.1203/01.pdr.0000228323.32445.7d
47. Seitz M, Köster C, Dzietko M, Sabir H, Serdar M, Felderhoff-Müser U, et al. Hypothermia modulates myeloid cell polarization in neonatal hypoxic-ischemic brain injury. J Neuroinflammation. (2021) 18(1):266. doi: 10.1186/s12974-021-02314-9
48. Burtrum D, Silverstein FS. Hypoxic-ischemic brain injury stimulates glial fibrillary acidic protein mRNA and protein expression in neonatal rats. Exp Neurol. (1994) 126(1):112–8. doi: 10.1006/exnr.1994.1047
49. Zhang S, Wu M, Peng C, Zhao G, Gu R. GFAP expression in injured astrocytes in rats. Exp Ther Med. (2017) 14(3):1905–8. doi: 10.3892/etm.2017.4760
50. Lafuente H, Pazos MR, Alvarez A, Mohammed N, Santos M, Arizti M, et al. Effects of cannabidiol and hypothermia on short-term brain damage in new-born piglets after acute hypoxia-ischemia. Front Neurosci. (2016) 10:323. doi: 10.3389/fnins.2016.00323
51. Hoogland IC, Houbolt C, van Westerloo DJ, van Gool WA, van de Beek D. Systemic inflammation and microglial activation: systematic review of animal experiments. J Neuroinflammation. (2015) 12:114. doi: 10.1186/s12974-015-0332-6
52. Alonso-Alconada D, Broad KD, Bainbridge A, Chandrasekaran M, Faulkner SD, Kerenyi Á, et al. Brain cell death is reduced with cooling by 3.5°C to 5°C but increased with cooling by 8.5°C in a piglet asphyxia model. Stroke. (2015) 46(1):275–8. doi: 10.1161/STROKEAHA.114.007330
53. Rocha-Ferreira E, Vincent A, Bright S, Peebles DM, Hristova M. The duration of hypothermia affects short-term neuroprotection in a mouse model of neonatal hypoxic ischaemic injury. PLoS One. (2018) 13(7):e0199890. doi: 10.1371/journal.pone.0199890
54. Chakkarapani E, Davis J, Thoresen M. Therapeutic hypothermia delays the C-reactive protein response and suppresses white blood cell and platelet count in infants with neonatal encephalopathy. Arch Dis Child Fetal Neonatal Ed. (2014) 99(6):F458–63. doi: 10.1136/archdischild-2013-305763
55. Jenkins DD, Lee T, Chiuzan C, Perkel JK, Rollins LG, Wagner CL, et al. Altered circulating leukocytes and their chemokines in a clinical trial of therapeutic hypothermia for neonatal hypoxic ischemic encephalopathy*. Pediatr Crit Care Med. (2013) 14(8):786–95. doi: 10.1097/PCC.0b013e3182975cc9
56. Jenkins DD, Rollins LG, Perkel JK, Wagner CL, Katikaneni LP, Bass WT, et al. Serum cytokines in a clinical trial of hypothermia for neonatal hypoxic-ischemic encephalopathy. J Cereb Blood Flow Metab. (2012) 32(10):1888–96. doi: 10.1038/jcbfm.2012.83
57. Matsui T, Kida H, Iha T, Obara T, Nomura S, Fujimiya T, et al. Effects of hypothermia on ex vivo microglial production of pro- and anti-inflammatory cytokines and nitric oxide in hypoxic-ischemic brain-injured mice. Folia Neuropathol. (2014) 52(2):151–8. doi: 10.5114/fn.2014.43786
58. Savard A, Lavoie K, Brochu ME, Grbic D, Lepage M, Gris D, et al. Involvement of neuronal IL-1beta in acquired brain lesions in a rat model of neonatal encephalopathy. J Neuroinflammation. (2013) 10(110). doi: 10.1186/1742-2094-10-110
59. Savard A, Brochu ME, Chevin M, Guiraut C, Grbic D, Sebire G. Neuronal self-injury mediated by IL-1beta and MMP-9 in a cerebral palsy model of severe neonatal encephalopathy induced by immune activation plus hypoxia-ischemia. J Neuroinflammation. (2015) 12:111. doi: 10.1186/s12974-015-0330-8
60. Girard S, Sebire H, Brochu ME, Briota S, Sarret P, Sebire G. Postnatal administration of IL-1Ra exerts neuroprotective effects following perinatal inflammation and/or hypoxic-ischemic injuries. Brain Behav Immun. (2012) 26(8):1331–9. doi: 10.1016/j.bbi.2012.09.001
61. Kendall GS, Hristova M, Horn S, Dafou D, Acosta-Saltos A, Almolda B, et al. TNF gene cluster deletion abolishes lipopolysaccharide-mediated sensitization of the neonatal brain to hypoxic ischemic insult. Lab Invest. (2011) 91(3):328–41. doi: 10.1038/labinvest.2010.192
62. Rocha-Ferreira E, Kelen D, Faulkner S, Broad KD, Chandrasekaran M, Kerenyi A, et al. Systemic pro-inflammatory cytokine status following therapeutic hypothermia in a piglet hypoxia-ischemia model. J Neuroinflammation. (2017) 14(1):44. doi: 10.1186/s12974-017-0821-x
63. Barks JDE, Liu Y, Dopp IA, Silverstein FS. Azithromycin reduces inflammation-amplified hypoxic-ischemic brain injury in neonatal rats. Pediatr Res. (2022) 92(2):415–23. doi: 10.1038/s41390-021-01747-5
64. Andersen M, Pedersen MV, Andelius TCK, Kyng KJ, Henriksen TB. Neurological outcome following newborn encephalopathy with and without perinatal infection: a systematic review. Front Pediatr. (2021) 9(1497). doi: 10.3389/fped.2021.787804
65. Dhillon SK, Gunn AJ, Jung Y, Mathai S, Bennet L, Fraser M. Lipopolysaccharide-Induced preconditioning attenuates apoptosis and differentially regulates TLR4 and TLR7 gene expression after ischemia in the preterm ovine fetal brain. Dev Neurosci. (2015) 37(6):497–514. doi: 10.1159/000433422
66. Vexler ZS, Mallard C, Hagberg H. Positive and negative conditioning in the neonatal brain. Cond Med. (2018) 1(6):279–93.
67. Hoshino K, Takeuchi O, Kawai T, Sanjo H, Ogawa T, Takeda Y, et al. Cutting edge: toll-like receptor 4 (TLR4)-deficient mice are hyporesponsive to lipopolysaccharide: evidence for TLR4 as the lps gene product. J Immunol. (1999) 162(7):3749–52. doi: 10.4049/jimmunol.162.7.3749
68. Schwandner R, Dziarski R, Wesche H, Rothe M, Kirschning CJ. Peptidoglycan- and lipoteichoic acid-induced cell activation is mediated by toll-like receptor 2. J Biol Chem. (1999) 274(25):17406–9. doi: 10.1074/jbc.274.25.17406
69. Yates N, Gunn AJ, Bennet L, Dhillon SK, Davidson JO. Preventing brain injury in the preterm infant-current controversies and potential therapies. Int J Mol Sci. (2021) 22(4). doi: 10.3390/ijms22041671
70. Girard S, Larouche A, Kadhim H, Rola-Pleszczynski M, Gobeil F, Sebire G. Lipopolysaccharide and hypoxia/ischemia induced IL-2 expression by microglia in neonatal brain. Neuroreport. (2008) 19(10):997–1002. doi: 10.1097/WNR.0b013e3283036e88
71. Stigger F, Lovatel G, Marques M, Bertoldi K, Moyses F, Elsner V, et al. Inflammatory response and oxidative stress in developing rat brain and its consequences on motor behavior following maternal administration of LPS and perinatal anoxia. Int J Dev Neurosci. (2013) 31(8):820–7. doi: 10.1016/j.ijdevneu.2013.10.003
72. Wang X, Stridh L, Li W, Dean J, Elmgren A, Gan L, et al. Lipopolysaccharide sensitizes neonatal hypoxic-ischemic brain injury in a MyD88-dependent manner. J Immunol. (2009) 183(11):7471–7. doi: 10.4049/jimmunol.0900762
73. Yang D, Sun YY, Bhaumik SK, Li Y, Baumann JM, Lin X, et al. Blocking lymphocyte trafficking with FTY720 prevents inflammation-sensitized hypoxic-ischemic brain injury in newborns. J Neurosci. (2014) 34(49):16467–81. doi: 10.1523/JNEUROSCI.2582-14.2014
74. Yang D, Sun YY, Nemkul N, Baumann JM, Shereen A, Dunn RS, et al. Plasminogen activator inhibitor-1 mitigates brain injury in a rat model of infection-sensitized neonatal hypoxia-ischemia. Cereb Cortex. (2013) 23(5):1218–29. doi: 10.1093/cercor/bhs115
75. Yang D, Sun YY, Lin X, Baumann JM, Dunn RS, Lindquist DM, et al. Intranasal delivery of cell-penetrating anti-NF-kappaB peptides (tat-NBD) alleviates infection-sensitized hypoxic-ischemic brain injury. Exp Neurol. (2013) 247:447–55. doi: 10.1016/j.expneurol.2013.01.015
76. Serdar M, Kempe K, Rizazad M, Herz J, Bendix I, Felderhoff-Muser U, et al. Early pro-inflammatory microglia activation after inflammation-sensitized hypoxic-ischemic brain injury in neonatal rats. Front Cell Neurosci. (2019) 13:237. doi: 10.3389/fncel.2019.00237
77. Harding B, Conception K, Li Y, Zhang L. Glucocorticoids protect neonatal rat brain in model of hypoxic-ischemic encephalopathy (HIE). Int J Mol Sci. (2017) 18(1). doi: 10.3390/ijms18010017
78. Ikeda T, Yang L, Ikenoue T, Mallard C, Hagberg H. Endotoxin-induced hypoxic-ischemic tolerance is mediated by up-regulation of corticosterone in neonatal rat. Pediatr Res. (2006) 59(1):56–60. doi: 10.1203/01.pdr.0000191140.87314.ce
79. Wang X, Svedin P, Nie C, Lapatto R, Zhu C, Gustavsson M, et al. N-acetylcysteine reduces lipopolysaccharide-sensitized hypoxic-ischemic brain injury. Ann Neurol. (2007) 61(3):263–71. doi: 10.1002/ana.21066
80. Semple BD, Blomgren K, Gimlin K, Ferriero DM, Noble-Haeusslein LJ. Brain development in rodents and humans: identifying benchmarks of maturation and vulnerability to injury across species. Prog Neurobiol. (2013) 106–107:1–16. doi: 10.1016/j.pneurobio.2013.04.001
Keywords: hypoxic-ischemic encephalopathy, lipopolysaccharide, therapeutic hypothermia, neuroprotection, animal model
Citation: Andersen M, Andersen HB, Andelius TCK, Hansen LH, Pinnerup R, Bjerre M, Ringgaard S, Schwendimann L, Gressens P, Kyng KJ and Henriksen TB (2023) No neuroprotective effect of therapeutic hypothermia following lipopolysaccharide-sensitized hypoxia-ischemia: a newborn piglet study. Front. Pediatr. 11:1268237. doi: 10.3389/fped.2023.1268237
Received: 27 July 2023; Accepted: 10 November 2023;
Published: 28 November 2023.
Edited by:
Kathryn Martinello, University College London, United KingdomReviewed by:
Raymand Pang, University College London, United KingdomTom Stiris, University of Oslo, Norway
© 2023 Andersen, Andersen, Andelius, Hansen, Pinnerup, Bjerre, Ringgaard, Schwendimann, Gressens, Kyng and Henriksen. This is an open-access article distributed under the terms of the Creative Commons Attribution License (CC BY). The use, distribution or reproduction in other forums is permitted, provided the original author(s) and the copyright owner(s) are credited and that the original publication in this journal is cited, in accordance with accepted academic practice. No use, distribution or reproduction is permitted which does not comply with these terms.
*Correspondence: Mads Andersen mads.andersen@clin.au.dk
Abbreviations ADC, apparent diffusion coefficient; aEEG, amplitude-integrated electroencephalogram; ARRIVE, animal research: reporting of in vivo experiments; ASL, arterial spin labelling; BOLD, blood oxygenation level dependent; CC3, cleaved-caspase 3; Cho, choline; Cr, creatine; GFAP, glial fibrillary acidic protein; HI, hypoxia-ischemia; IBA1, anti-ionizing adaptor protein 1; Lac, lactate; LPS, lipopolysaccharides; MABP, mean arterial blood pressure; MRS, magnetic resonance spectroscopy; MRI, magnetic resonance imaging; NAA, N-acetylaspartate; NT, normothermia; TH, therapeutic hypothermia.