- 1Área de Farmacología y Nutrición, Departamento de Ciencias Básicas de la Salud, Universidad Rey Juan Carlos, Alcorcón, Spain
- 2Unidad Asociada I+D+i del Instituto de Química Médica, Consejo Superior de Investigaciones Científicas, Madrid, Spain
- 3Unidad Asociada I+D+i del Instituto de Investigación en Ciencias de la Alimentación, Consejo Superior de Investigaciones Científicas, Madrid, Spain
- 4Grupo de Excelencia Investigadora URJC-Banco de Santander-Grupo Multidisciplinar de Investigación y Tratamiento del Dolor (i+DOL), Alcorcón, Spain
- 5Unidad del Dolor, Servicio de Anestesia, Hospital General Universitario Gregorio Marañón, Madrid, Spain
- 6Área de Histología Humana y Anatomía Patológica, Departamento de Ciencias Básicas de la Salud, Universidad Rey Juan Carlos, Alcorcón, Spain
Background: In different models of paralytic ileus, cannabinoid receptors are overexpressed and endogenous cannabinoids are massively released, contributing to gastrointestinal dysmotility. The antitumoral drug vincristine depresses gastrointestinal motility and a similar mechanism could participate in this effect. Therefore, our aim was to determine, using CB1 and CB2 antagonists, whether an increased endocannabinoid tone is involved in vincristine-induced gastrointestinal ileus.
Methods: First, we confirmed the effects of vincristine on the gut mucosa, by conventional histological techniques, and characterized its effects on motility, by radiographic means. Conscious male Wistar rats received an intraperitoneal injection of vincristine (0.1–0.5 mg/kg), and barium sulfate (2.5 ml; 2 g/ml) was intragastrically administered 0, 24, or 48 h later. Serial X-rays were obtained at different time-points (0–8 h) after contrast. X-rays were used to build motility curves for each gastrointestinal region and determine the size of stomach and caecum. Tissue samples were taken for histology 48 h after saline or vincristine (0.5 mg/kg). Second, AM251 (a CB1 receptor antagonist) and AM630 (a CB2 receptor antagonist) were used to determine if CB1 and/or CB2 receptors are involved in vincristine-induced gastrointestinal dysmotility.
Key results: Vincristine induced damage to the mucosa of ileum and colon and reduced gastrointestinal motor function at 0.5 mg/kg. The effect on motor function was particularly evident when the study started 24 h after administration. AM251, but not AM630, significantly prevented vincristine effect, particularly in the small intestine, when administered thrice. AM251 alone did not significantly alter gastrointestinal motility.
Conclusions: The fact that AM251, but not AM630, is capable of reducing the effect of vincristine suggests that, like in other experimental models of paralytic ileus, an increased cannabinoid tone develops and is at least partially responsible for the alterations induced by the antitumoral drug on gastrointestinal motor function. Thus, CB1 antagonists might be useful to prevent/treat ileus induced by vincristine.
Introduction
Vincristine is a vinca alkaloid widely used in the treatment of hematological malignancies and solid tumors since the 1960's (Johnson et al., 1960; Bohannon et al., 1963). It is a cell cycle specific agent which blocks mitosis with metaphase arrest through disruption of the mitotic apparatus and it may affect several body systems (Rosenthal and Kaufman, 1974). The main side effect of vincristine is a dose dependent and cumulative peripheral neuropathy. Paresthesias, loss of tendon reflexes, and progressive weakness are the most common clinical features, although autonomic dysfunctions, including gastrointestinal disturbances, might occur (Rosenthal and Kaufman, 1974; Harris and Jackson, 1977; Chae et al., 1998; Wang et al., 2000). Indeed, gastrointestinal complications may be present in up to 30–40% of patients receiving vincristine and the earliest symptoms may include colicky abdominal pain, constipation, and adynamic or paralytic ileus as the major manifestations. Damage to the myenteric plexus by vinca alkaloids could be implicated in intestinal hypomotility (Smith, 1967; Kaneko et al., 2001; Peixoto Júnior et al., 2009). Since constipation is the most widely recognized manifestation, colonic motility has received the most attention. But patients treated with vincristine can also develop symptoms indicating dysmotility of the upper gastrointestinal tract, including anorexia, and nausea or even extreme symptoms such as paralytic ileus. In fact, paralytic ileus occurs in 3–12% of patients, and may be fatal in up to 30% of them (Toghill and Burke, 1970). However, the impact and mechanisms of vincristine on gastrointestinal motility have not been deeply studied in humans or animals.
The endocannabinoid system in the gastrointestinal tract has attracted much attention because both its activation and inhibition could be therapeutically useful depending on the circumstances (Abalo et al., 2012; Abalo and Martín-Fontelles, 2017; Salaga et al., 2017; Vera et al., 2017). Evidence is emerging that exogenous and endogenous cannabinoids have an important role in gastrointestinal physiopathology, such as gastrointestinal inflammation (Izzo and Camilleri, 2009). But cannabinoids mediate also other functions in the gut, such as gastroprotection and gastric secretion, gastrointestinal motility, ion transport, visceral sensation, and cell proliferation (Izzo and Sharkey, 2010). In this sense, plant-derived, endogenous, and synthetic cannabinoid receptor agonists reduced gastric emptying, upper gastrointestinal transit and colonic propulsion in rodents (Aviello et al., 2008; Izzo and Camilleri, 2009; Abalo et al., 2012; Vera et al., 2017), whereas cannabinoid receptor antagonists may increase gastrointestinal motility in experimental animals (Izzo et al., 1999) and cause diarrhea in humans (Waterlow and Chrisp, 2008).
There are functional, biochemical, and immunohistochemical evidences that alterations in the enteric endocannabinoid system contribute to causing paralytic ileus in animal models, and different strategies aimed at normalizing endocannabinoid levels were useful in these conditions. Actually, the inactivation of CB1 (Mascolo et al., 2002) or CB1 and CB2 receptors (Li et al., 2010) were useful in the treatment of paralytic ileus induced by acetic acid and lipopolysaccharide (LPS), respectively. Thus, cannabinoid antagonists may be powerful tools in the treatment of adynamic ileus of different origins.
So far, we have characterized the effect of different drugs in the gastrointestinal tract of experimental animals using radiographic methods, including antitumoral drugs, like cisplatin (Cabezos et al., 2008, 2010; Vera et al., 2014) and 5-fluorouracil (Abalo et al., 2016; McQuade et al., 2016), and cannabinoids (Abalo et al., 2009, 2010, 2011, 2015). We have even performed studies of the combined effects of antitumoral drugs and cannabinoids (Abalo et al., 2013, 2016). In these regards, we showed that the non-selective cannabinoid agonist WIN 55, 212-2 was not capable of improving cisplatin-induced gastrointestinal dysmotility, and even worsened it (Abalo et al., 2013), whereas at a non-psychoactive dose, it tended to reduce diarrhea associated to 5-fluorouracil treatment (Abalo et al., 2016). Thus, these techniques might be useful to study vincristine effects on gastrointestinal motor function, and the possible role of cannabinoid agents in them.
Therefore, the aims of this work were, using radiographic means: (1) To characterize the effect of the antitumoral drug vincristine on rat gastrointestinal motor function. (2) To determine whether the motor alterations induced by vincristine might be prevented by the CB1-selective cannabinoid antagonist AM251 and by the CB2-selective cannabinoid antagonist AM630. Some of the present results were communicated previously in abstract form (Vera et al., 2012).
Materials and Methods
The Ethical Committee at Universidad Rey Juan Carlos (URJC) and Hospital General Universitario Gregorio Marañón (HGUGM) approved the study. Experimental procedures were carried out in accordance with the recommendations of these Committee as well as with the EU directive for the protection of animals used for scientific purpose (2010/63/UE) and Spanish regulations (RD 109 53/2013).
Animals
Male Wistar rats (350–400 g) were obtained from the Veterinary Unit of HGUGM (Madrid, Spain) or from Envigo (Barcelona, Spain) and housed (4/cage), at the Veterinary Units of HGUGM, or URJC, in standard transparent cages (60 × 40 × 20 cm), under environmentally controlled conditions (temperature = 20°C; humidity = 60%), with a 12 h light/12 h dark cycle. Animals had free access to standard laboratory rat chow (Harlan Laboratories Inc.) and tap water.
Protocol
First, we characterized the effect of a single dose of vincristine on gastrointestinal architecture and motility by histological and radiographic means, respectively (see below). Rats received an acute intraperitoneal injection of vincristine (0.1 or 0.5 mg/kg) or saline (2–3 ml/kg). Alterations of gastrointestinal motility were measured immediately, 24 or 48 h after drug administration. Samples from ileum and colon were taken for conventional histology 48 h after saline or vincristine (0.5 mg/kg).
A second set of experiments was performed in order to determine whether the alterations induced by vincristine could be due to an increased cannabinoid tone and activation of CB1 or CB2 receptors. In these experiments, vincristine was administered 24 h prior to the radiographic analysis and adequate cannabinoid antagonists were tested as follows.
The cannabinoid CB1-selective antagonist AM251 (1 mg/kg), or its vehicle (1 ml/kg), was administered once (20 min before vincristine), twice (before and 24 h after vincristine), or thrice (before, 12 and 24 h after vincristine). Thereafter (24 h after vincristine injection), the radiographic analysis of gastrointestinal motor function was performed (see below).
In the remaining experiments, the CB2-selective antagonist AM630 (1 mg/kg), or its vehicle (1 ml/kg), was administered thrice (20 min before, and 12 and 24 h after vincristine) and gastrointestinal motor function was analyzed as described below. This group of experiments was performed at URJC, using animals from Envigo.
Histology
Forty-eight hours after vincristine, samples were obtained from terminal ileum (at least 10 cm oral to the ileocaecal junction) and colon of 4–8 animals per experimental group, fixed in buffered 10% formalin and embedded in paraffin. Sections of 5 μm were stained with hematoxylin-eosin (HE) and studied under a Zeiss Axioskop 2 microscope equipped with the image analysis software package AxioVision 4.6. Samples were studied in duplicate under a 20x objective. Histological damage was evaluated using a numerical score of 0–3 assigned to each section considering general loss of mucosal architecture (graded 0–3, absent to severe) and extent of inflammatory cell infiltrate (graded 0–3, absent to transmural). The experimenter was blind to the treatment received by the rat from which the sample under analysis was obtained.
Gastrointestinal Motility Evaluation
Gastrointestinal motor function was studied by radiographic methods as previously described (Cabezos et al., 2008). Thus, 2.5 ml of a suspension of barium sulfate (2 g/ml, temperature = 22°C) was administered per os. Experiments at HGUGM were performed with a Siemens (Siremobil Compact L, Erlangen, Germany) digital X-Ray apparatus (60 kV, 7 mA) and X-rays were captured with NPG Real DVD Studio II software. For the experiments at URJC, a CS2100 (Carestream Dental, Spain) digital X-ray apparatus (60 kV, 7 mA) was used, and X-rays were recorded on Carestream Dental T-MAT G/RA film (15 × 30 cm) housed in a cassette provided with regular intensifying screen; films were developed using a Kodak X-omat 2000 automatic processor. Exposure time was adjusted to 20–60 ms. Immobilization of the rats in prone position was achieved by placing them inside adjustable hand-made transparent plastic tubes, so that they could not move. Habituation to the recording chamber prior to commencement of the study did not significantly alter gastrointestinal motility (Cabezos et al., 2008). To further reduce stress, rats were released immediately after each shot (immobilization lasted for 1–2 min). X-rays were recorded at different times (immediately and 1, 2, 4, 6, and 8 h) after administration of the contrast medium. While taking the radiographs, the qualified investigator remained at least 2 m away from the X-ray source or behind a leaded wall, where radioactivity while shooting was not different from environmental readings. A trained investigator blind to the drug administered performed the analysis of the radiographs. Alterations in gut motility were semiquantitatively determined from the images by assigning a compounded value to each region of the gastrointestinal tract considering the following parameters: percentage of the gastrointestinal region filled with contrast (0–4); intensity of contrast (0–4); homogeneity of contrast (0–2); and sharpness of the gastrointestinal region profile (0–2). Each of these parameters was scored and a sum (0–12 points) was made. X-rays for characterization of vincristine and AM251 effects were obtained at HGUGM. X-rays to study the effect of AM630 were taken at URJC. Results were comparable for controls (triple administration of vehicle) obtained at both institutions.
The X-ray images were also analyzed with the aid of an image analysis system (Image J 1.38 for Windows, National Institute of Health, USA, free software: http://rsb.info.nih.gov/ij/) and the alterations of stomach size and caecum were studied.
Compounds and Drugs
Barium sulfate (Barigraf® AD, Juste SAQF, Madrid, Spain) was suspended in tap water and continuously hand-stirred until administration.
Vincristine was purchased from Sigma-Aldrich (Spain, experiments performed at HGUGM) or from Abcam (UK, experiments performed at URJC) and dissolved in saline.
N-(piperidin-1-yl)-5-(4-iodophenyl)-1-(2,4-dichlorophenyl)-4-methyl-1H-pyrazole-3-carboxamide (AM251; Gatley et al., 1996) and 6-iodo-2-methyl-1-[2-(4-morpholinyl)ethyl]-1H-indol-3-yl](4-methoxyphenyl) methanone (AM630; Hosohata et al., 1997) were purchased from Ascent Scientific Ltd (North Somerset, BS24 9 ES, UK).
Cannabinoid antagonists were dissolved in Tocrisolve, a commercially available water soluble emulsion composed of a 1:4 ratio of soya oil/water that is emulsified with the block co-polymer Pluronic F68 (Tocris, Cookson, Bristol, UK; 30 μl in 0.5 ml of saline solution).
Statistical Analysis
Data are presented as the mean values ± SEM. Differences between groups were analyzed using Student's t-test or two-way ANOVA followed by post-hoc Bonferroni multiple comparison test, as appropriate. Values of p < 0.05 were regarded as being significantly different.
Results
Histopathological Effects of Vincristine on Intestinal Tissues
The histological pattern of the intestinal wall in HE stained sections is shown in Figure 1. A general and statistically significant damage was observed after vincristine administration. The epithelial layer was particularly affected, showing large areas with ulcers, and loss of normal architecture both in small (Figures 1A,B,E, p < 0.01) and large intestine (Figures 1C,D,F, p < 0.01). On the contrary, there were no differences regarding the extent of inflammatory nodules between vincristine-treated animals and controls.
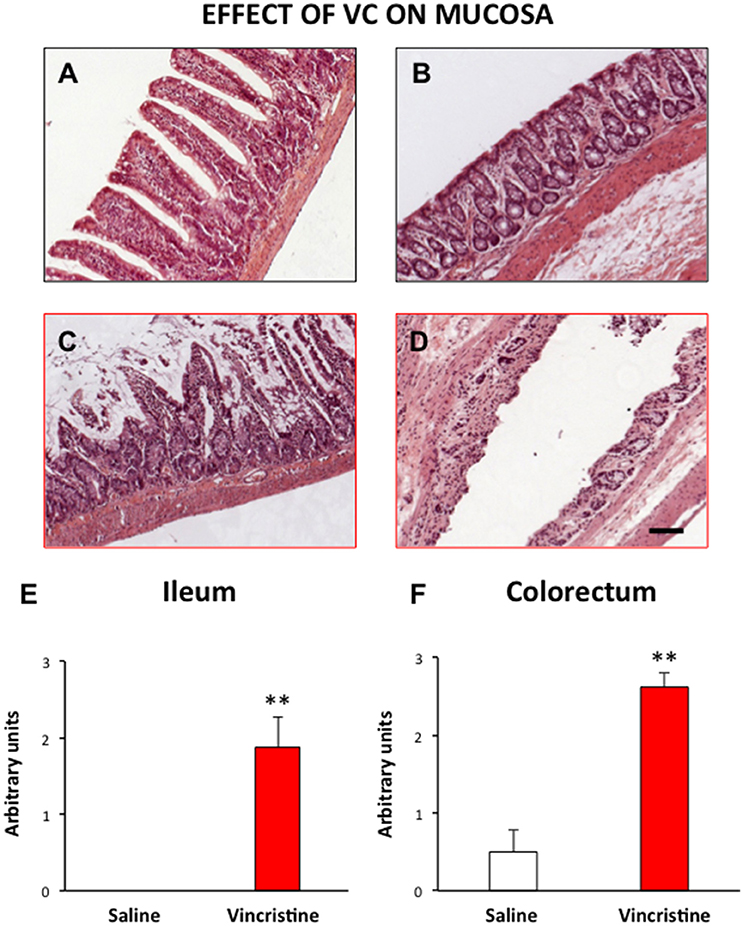
Figure 1. Effect of vincristine treatment on the general structure of the rat intestine. Rats were injected intraperitoneally with vincristine (0.5 mg/kg) or saline (1–2 ml/kg). Forty-eight hours after treatment, histological samples were embedded in paraffin, and stained with HE. Left row, small intestine: tissue samples from saline (A) and vincristine-treated animals (C) showing the damaged mucosa; quantitative analysis of the histological damage (E). Right row, colorectum: tissue samples from saline (B) and vincristine-treated animals (D) showing the damaged mucosa; quantitative analysis of the histological damage (F). Bars show mean values ± SEM for control (white) and vincristine-treated animals (red). Each group consisted of 4–8 rats. **p < 0.01 vs. saline; (Student's t-test). Scale bar: 100 μm.
Effects of Vincristine on Gastrointestinal Motor Function
In control animals, when barium was given immediately after saline, gastric emptying was complete 4 h after barium. Barium content reached its maximum in the small intestine in just 1 h and it completely emptied into the caecum by 4 h. In most animals, barium started to stain the caecum and the colorectum 2 and 4 h after barium, respectively. Both organs filled progressively until the end of the study (Figure 2). When barium was given 24 (Figure 3) or 48 h (Figure 4) after saline, the motility curves were very similar to those obtained immediately after saline administration.
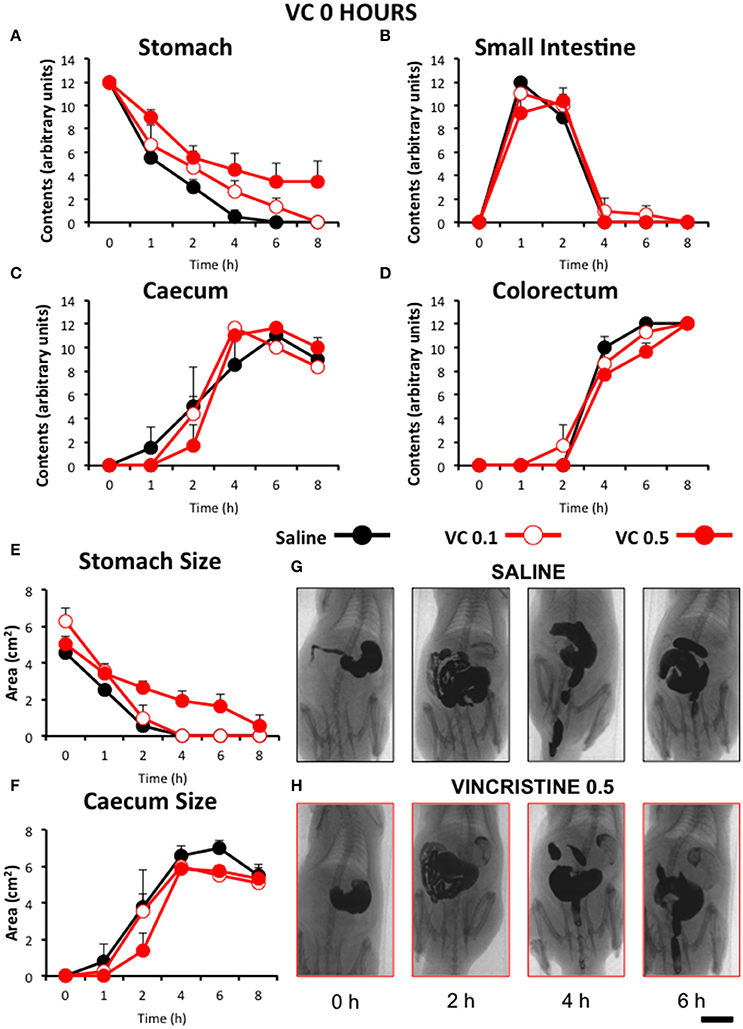
Figure 2. Effect of vincristine immediately after administration on gastrointestinal motor function in the rat. Gastrointestinal motor function was evaluated by radiological methods (see text) in: (A) stomach (gastric emptying); (B) small intestine; (C) caecum and (D) colorectum. Rats were injected intraperitoneally (i.p.) with: saline (1–2 ml/kg) or vincristine at 0.1 (VC 0.1) or 0.5 mg/kg (VC 0.5). Barium sulfate (2.5 ml, 2 g/ml) was intragastrically administered immediately after drug administration and X-rays were taken 0–8 h after. The size of stomach (E) and caecum (F) was determined with Image J. Data represent mean ± SEM (two-way ANOVA followed by post-hoc Bonferroni multiple comparison test). (G,H) Representative images of animals treated with saline and VC 0.5, taken at different times throughout the experiment. n = 8 each group. Scale bar: 23 mm.
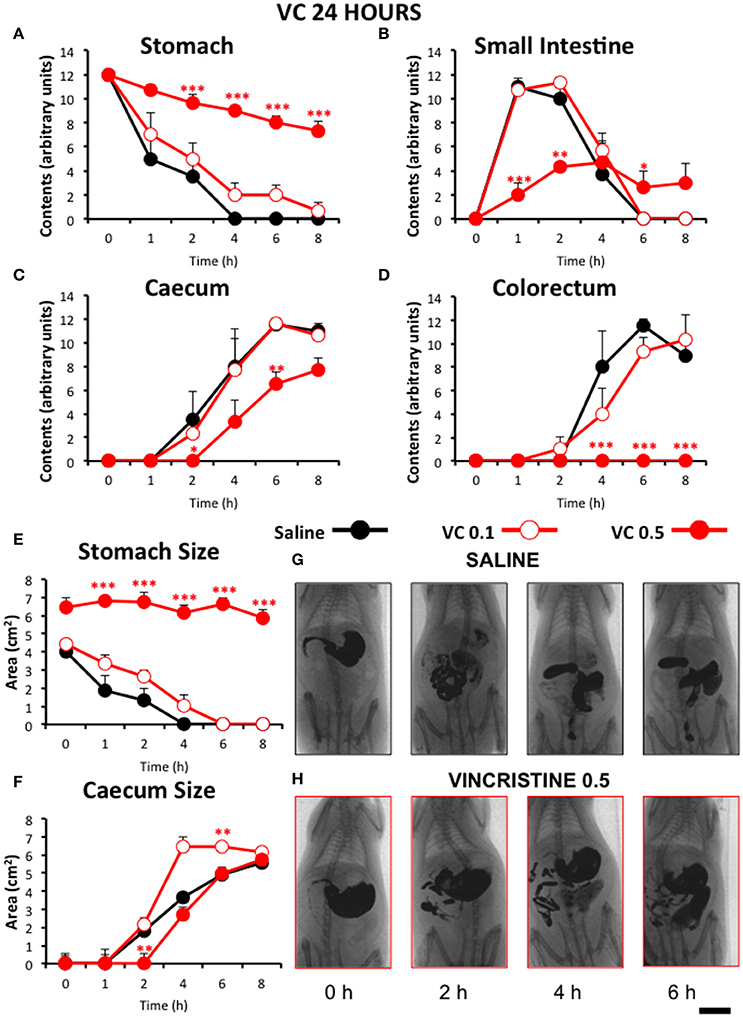
Figure 3. Effect of vincristine 24 h after administration on gastrointestinal motor function in the rat. Gastrointestinal motor function was evaluated by radiological methods (see text) in: (A) stomach (gastric emptying); (B) small intestine; (C) caecum and (D) colorectum. Rats were injected intraperitoneally (i.p.) with: saline (1–2 ml/kg) or vincristine at 0.1 (VC 0.1) or 0.5 mg/kg (VC 0.5). Barium sulfate (2.5 ml, 2 g/ml) was intragastrically administered 24 h after drug administration and X-rays were taken 0–8 h after. The size of stomach (E) and caecum (F) was determined with Image J. Data represent mean ± SEM. *p < 0.05, **p < 0.01, ***p < 0.001 vs. saline (two-way ANOVA followed by post-hoc Bonferroni multiple comparison test). (G,H) Representative images of animals treated with saline and VC 0.5, taken at different times throughout the experiment. n = 8 each group. Scale bar: 23 mm.
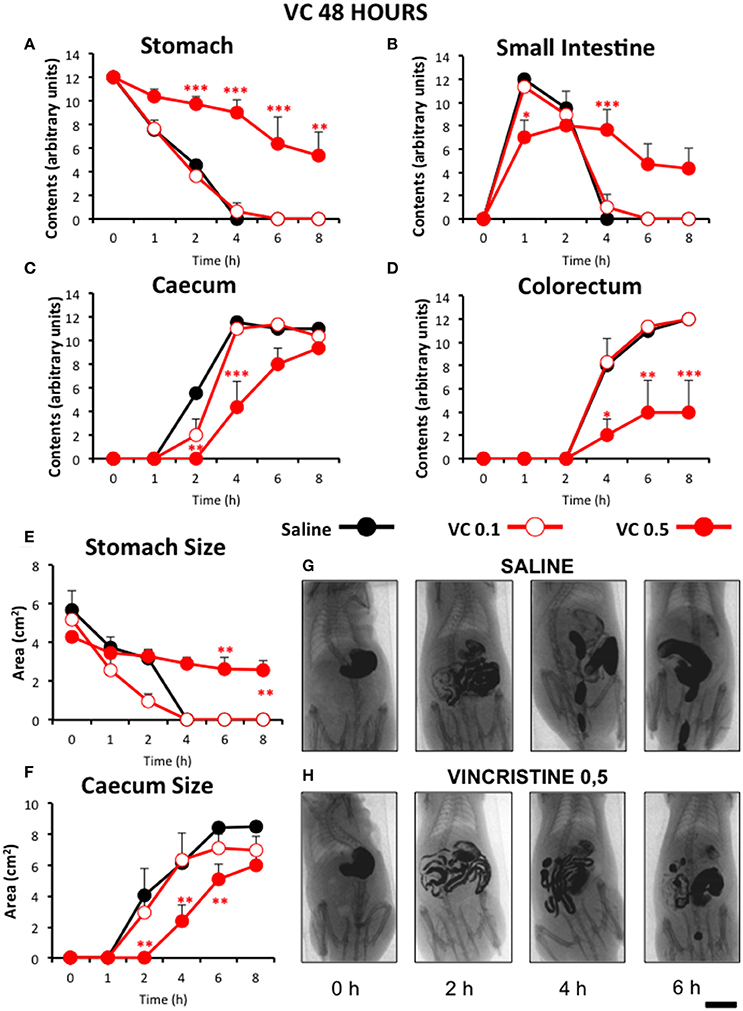
Figure 4. Effect of vincristine 48 h after administration on gastrointestinal motor function in the rat. Gastrointestinal motor function was evaluated by radiological methods (see text) in: (A) stomach (gastric emptying); (B) small intestine; (C) caecum and (D) colorectum. Rats were injected intraperitoneally (i.p.) with: saline (1–2 ml/kg) or vincristine at 0.1 (VC 0.1) or 0.5 mg/kg (VC 0.5). Barium sulfate (2.5 ml, 2 g/ml) was intragastrically administered 48 h after drug administration and X-rays were taken 0–8 h after. The size of stomach (E) and caecum (F) was determined with Image J. Data represent mean ± SEM. *p < 0.05, **p < 0.01, ***p < 0.001 vs. saline (two-way ANOVA followed by post-hoc Bonferroni multiple comparison test). (G,H) Representative images of animals treated with saline and VC 0.5, taken at different times throughout the experiment. n = 8 each group. Scale bar: 23 mm.
Compared with saline, acute administration of vincristine immediately before barium administration (0 h), did not induce any significant change on gastrointestinal motility, irrespective of the dose studied. Also, the quantitative analysis of the images did not show any significant change in the stomach or caecum size (Figure 2).
Remarkably, vincristine intensely and significantly reduced gastrointestinal motor function when this was radiographically evaluated 24 h after administration, but only at the dose of 0.5 mg/kg. In these animals, gastric emptying was progressive but at a much lower rate than in rats treated with saline or vincristine at 0.1 mg/kg (Figure 3A). Intestinal transit and filling of caecum were also delayed in vincristine-treated rats at the high dose (Figures 3B,C). Furthermore, at the end of the experiment (8 h), contrast had not reached the colorectal region in any of those animals (Figure 3D). These results were confirmed in the quantitative analysis of the images. In rats treated with vincristine at 0.5 mg/kg, the stomach size at the beginning of the experiment was increased compared to saline-treated animals and remained unchanged for the rest of the experiment (Figure 3E). In the caecum, vincristine had a dual effect; the lower dose of vincristine increased its size and the higher one reduced it (Figure 3F).
When serial X-rays were obtained 48 h after vincristine, gastrointestinal motor function was still decreased, although the effect was less pronounced than in the previous experiment. Gastric emptying and intestinal transit were reduced (Figures 4A,B), but gastric emptying started to recover 6 h after barium (54 h after vincristine), at least in some animals, and higher levels of barium contents were reached in the small intestine at all time-points. The effect on caecum was similar to the previous experiment, but that on the colorectum was less intense and fecal pellets were seen in some animals (Figures 4C,D). These results were confirmed in the quantitative analysis. Thus, 0 h after contrast (48 h after vincristine), the stomach size was again comparable to that in the saline group, but not much further change was apparent in this region (Figure 4E). Vincristine reduced the size of the caecum at the highest dose used (Figure 4F).
Effect of the Cannabinoid Antagonists on Ileus Induced by Vincristine
Figure 5 shows the motility curves for controls used in this experiment. In addition to the effect of saline, the effect of injecting the cannabinoid vehicle once (20 min before saline), twice (before and 24 h after saline) and thrice (before, 12 and 24 h after saline) is shown. As can be seen, injecting the vehicle only once did not produce any effect compared to saline-treated animals. However, when it was injected twice or thrice, significant delays in gastric emptying and in filling of small intestine and caecum were seen. For the following experiments, the effects of the different drugs are compared to those of the vehicle given thrice, since it was the pattern which induced more changes compared to saline (although still very different to that found in vincristine-treated animals, see below).
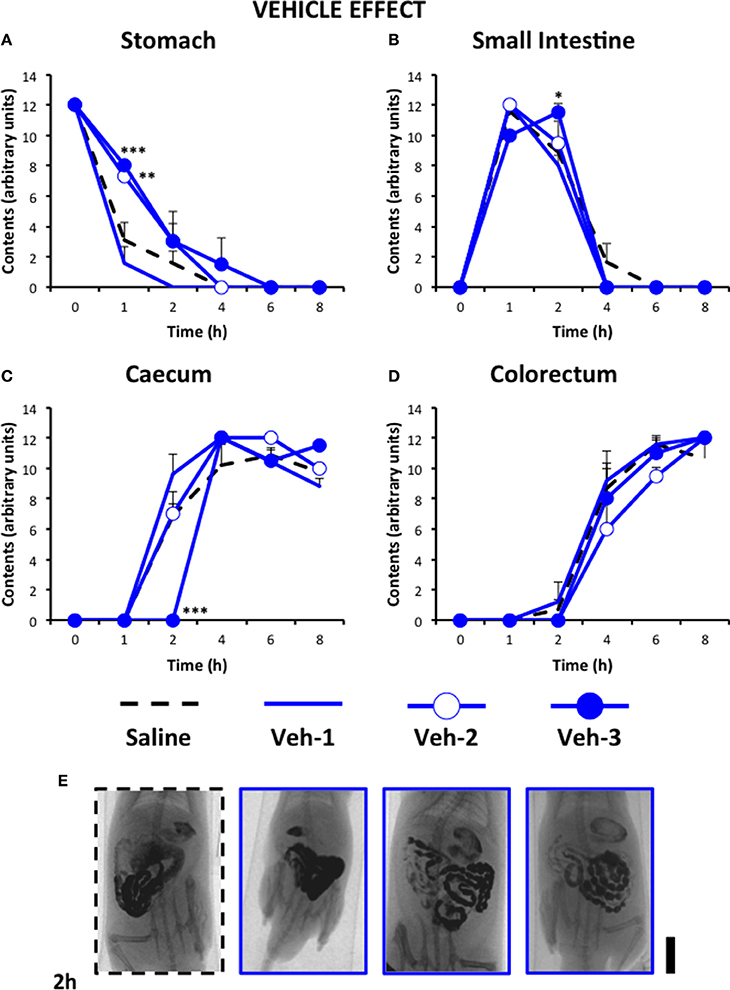
Figure 5. Effect of saline or the cannabinoid vehicle on gastrointestinal motor function in the rat. Gastrointestinal motor function was evaluated by radiological methods (see text) in: (A) stomach (gastric emptying); (B) small intestine; (C) caecum and (D) colorectum. Rats were injected intraperitoneally (i.p.) with: saline (1–2 ml/kg) or the cannabinoid vehicle once (20 min before saline, Veh-1), twice (before and 24 h after saline, Veh-2), and thrice (before, 12 and 24 h after saline, Veh-3). Barium sulfate (2.5 ml, 2 g/ml) was intragastrically administered immediately or 24 h after drug administration and X-rays were taken 0–8 h after. Data represent mean ± SEM. *p < 0.05, **p < 0.01, ***p < 0.001 vs. saline (two-way ANOVA followed by post-hoc Bonferroni multiple comparison test). n = 4–8 animals per group. (E) Representative images of saline- and Veh-treated animals 2 h after contrast. Scale bar: 23 mm.
Figure 6 shows that the CB1 antagonist AM251 (1 mg/kg) improved gastrointestinal motor function compared to vincristine-treated rats and that this effect increased with the number of times it was injected (3 > 2 > 1). However, the normalizing effect of AM251 was different in each gastrointestinal region. Thus, in the stomach and colorectum, AM251 given thrice exerted a significant but relatively small effect. In contrast, it almost normalized the motility curve in the small intestine, and completely normalized the curve in the caecum. Efficacy of AM251 was lower in animals treated with the compound only twice and even lower when it was administered only once. AM251 given thrice did not exert any significant effect compared to its vehicle given also thrice.
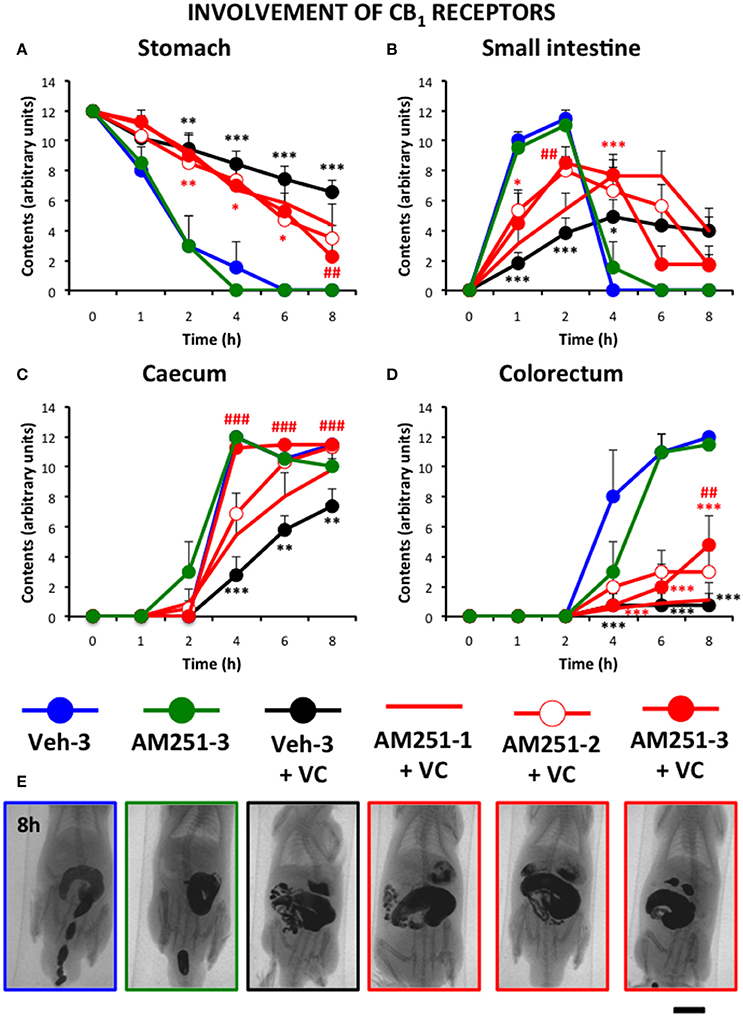
Figure 6. Effect of the CB1 antagonist AM251 on the alterations induced by vincristine on gastrointestinal motor function in the rat. Gastrointestinal motor function was evaluated by radiological methods (see text) in (A) stomach (gastric emptying); (B) small intestine; (C) caecum and (D) colorectum. Rats received two intraperitoneal injections (i.p.). One was saline (1–2 ml/kg) or vincristine at 0.5 mg/kg (VC). The other one was the cannabinoid vehicle given three times (Veh-3) or AM251 given once (20 min before saline or vincristine: AM251-1), twice (before and 24 h after saline or vincristine: AM251-2), or thrice (before, 12 and 24 h after saline or vincristine: AM251-3). Barium sulfate (2.5 ml, 2 g/ml) was intragastrically administered 24 h after saline or vincristine administration and X-rays were taken 0–8 h after. Data represent mean ± SEM. *p < 0.05, ** p < 0.01, ***p < 0.001 vs. Veh-3; ##p < 0.01; ###p < 0.001 vs. Veh 3 + VC (two-way ANOVA followed by post-hoc Bonferroni multiple comparison test). n = 4–8 animals each group. (E) Representative images of the different treatments 8 h after contrast. Scale bar: 23 mm.
Finally, the effect of the selective CB2 antagonist AM630 was tested. For ethical reasons, and due to the fact that the CB1 antagonist showed the best results after its triple administration, we only used this pattern of administration to test the effect of AM630. In high contrast with the effect of AM251, the triple administration of AM630 did not significantly modify the effect of vincristine (Figure 7).
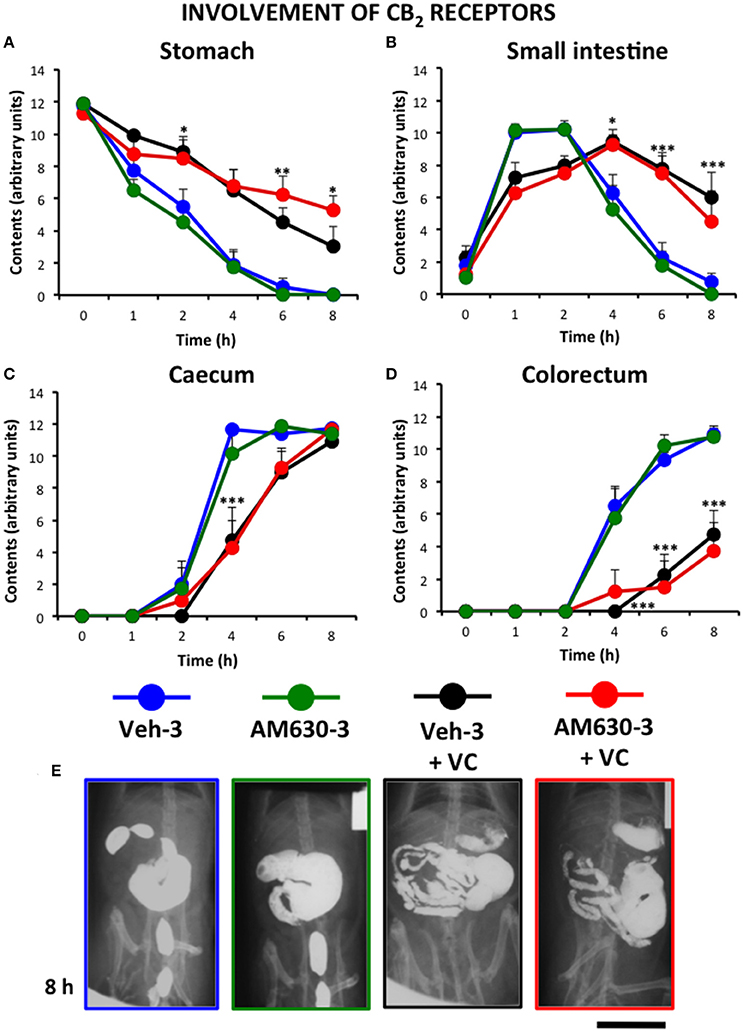
Figure 7. Effect of the CB2 antagonist AM630 on the alterations induced by vincristine on gastrointestinal motor function in the rat. Gastrointestinal motor function was evaluated by radiological methods (see text) in: (A) stomach (gastric emptying); (B) small intestine; (C) caecum and (D) colorectum. Rats received saline (1–2 ml/kg) or vincristine at 0.5 mg/kg (VC). The cannabinoid vehicle (Veh-3) or AM630 (AM630-3) were administered thrice (before, 12 and 24 h after saline or vincristine administration). Barium sulfate (2.5 ml, 2 g/ml) was intragastrically administered 24 h after saline or vincristine administration and X-rays were taken 0–8 h after. Data represent mean ± SEM. *p < 0.05, **p < 0.01, ***p < 0.001 vs. Veh-3 (two-way ANOVA followed by post-hoc Bonferroni multiple comparison test). n = 8 each group. (E) Representative images of the different treatments 8 h after contrast. Scale bar: 30 mm.
Discussion
This is the first work in which vincristine-induced gastrointestinal dysmotility has been characterized using radiographic methods in experimental animals. In addition, we have demonstrated that the selective CB1 cannabinoid antagonist AM251 (but not AM630, a CB2 selective antagonist), is capable of reducing the effect of vincristine, suggesting that an increased cannabinoid tone is, atleast partially, responsible for the alterations induced by this antitumoral drug on gastrointestinal motor function.
Vincristine Effect on Gastrointestinal Motor Function in the Rat
Gastrointestinal dysmotility associated to vinca alkaloids, including vincristine, is a known cause of drug-induced gastrointestinal toxicity (Bradley, 1968). Here, vincristine did not significantly alter gastrointestinal motility at a low (0.1 mg/kg) or high dose (0.5 mg/kg) when the X-ray study was performed immediately after its administration. However, when the radiographic study was carried out 24 or 48 h after the antitumoral drug at 0.5 mg/kg, an intense and significant decrease in gastric emptying and intestinal transit was observed. These results indicate that vincristine-induced dysmotility may need a relatively long time to occur or that higher doses might be needed to see early effects of this drug on gastrointestinal motor function. We did not increase the dose or the observation time because the higher dose used here was similar to the LD50 in rats and mortality associated to this dose may occur 4–6 days after its administration (Uy et al., 1967).
Several previous investigations have reported that vincristine-induced gastric hypomotility is not an early event (Kaneko et al., 2001; Tsukamoto et al., 2011). The delayed effect of vincristine contrasts with that of other antineoplastic drugs, like cisplatin, which provokes gastric dysmotility within a much shorter time in rats (Cabezos et al., 2008, 2010; Vera et al., 2014). Cisplatin immediate (acute) effect on gastric motor function is due to serotonin release and vagal activation, through the stimulation of 5-HT3 receptors (Vera et al., 2014), and underlies its intense emetogenic effect in experimental animals (Holmes et al., 2009; du Sert et al., 2011; Horn, 2014) and humans (Navari, 2013). Cisplatin may also produce “delayed” emesis in humans, and we observed “delayed” gastric dysmotility and pica, a surrogate marker of nausea in rodents (Takeda et al., 1993), after cisplatin administration in the rat (Cabezos et al., 2008). Cisplatin-induced delayed emesis seems to be more dependent upon other mechanisms, including the activation of NK1 receptors through the release of substance P (Navari, 2013; Rudd et al., 2016). These mechanisms justify the usefulness of 5-HT3 and NK1 antagonists for prevention of emesis associated to highly emetogenic chemotherapy in cancer patients (Navari, 2013). Vincristine may induce nausea and emesis in dogs and humans, but the incidence and intensity of these effects are much lower than with cisplatin (Navari, 2013; Mason et al., 2014) and the mechanisms might be different (see below).
The altered motility curve observed here for the small intestine 24 h after vincristine may be due, at least partly, to the delayed gastric emptying (barium reached the small intestine much later in vincristine- than in saline-treated animals). However, this would probably have produced a motility curve for the small intestine very similar in shape to that in control animals, but displaced to the right (this occurred after acute cisplatin, which only alters gastric motility; Cabezos et al., 2008). In the present study, the curve was completely distorted, suggesting that vincristine produced direct effects in this region. Direct effects of vincristine in the small intestine might include altered myoelectric activity, increased tone and spasmogenic actions, as previously suggested (Sharma, 1979, 1988; Sninsky, 1987). Small intestinal transit was accelerated in rats in the first few hours after vinblastine (Sharma, 1979), but we did not detect such an effect of vincristine in our non-invasive study. In fact, the motility curve of caecum looked very similar (parallel) to that in control animals both 24 and 48 h after vincristine (0.5 mg/kg), but displaced to the right, further suggesting that small intestinal transit was delayed. Interestingly, in spite of the fact that caecum filled adequately, there was a complete absence of stained fecal pellets in vincristine-treated rats for the whole duration of the radiologic study when it was performed 24 h after the antitumoral drug, suggesting that vincristine directly suppressed motility in colorectum, which is in accordance with the reports of constipation associated to treatment with vinca alkaloids, in both animals and humans (Harris and Jackson, 1977; Garewal and Dalton, 1985; Ikehara, 1992; Leker et al., 1997; Chae et al., 1998; Wang et al., 2000; Essa et al., 2014; Yasu et al., 2016).
Several factors may contribute to the effects found in the stomach, small intestine, caecum and colorectum 24–48 h after vincristine. Chemotherapy-induced gastrointestinal toxicity can be caused by direct damage to mucosal epithelial cells or by stimulation of the vomiting center or chemoreceptor trigger zone (Kaneko et al., 2001). Vincristine is known to induce metaphase arrest, severe villous atrophy and mucosal erosions (Beró and Jávor, 1985), which we found in our histological study. This effect would disrupt the intestinal barrier function and could contribute to dysmotility. In contrast, we did not observe evident changes in the presence of inflammatory cells within the gut wall, suggesting that these might not be determinant to acute vincristine-induced dysmotility, although this must be systematically studied. According to previous reports, direct effects on the smooth muscle layers (Kaneko et al., 2001) or the possible influence of enhanced adrenergic activity due to neuropathic pain (Peixoto Júnior et al., 2009) seem unlikely. Interestingly, due to the known neurotoxicity of the compound (whose direct effect on the vomiting center to induce gastric dysmotility and emesis cannot be discarded) and to several functional and histological evidences, the development of an autonomic neuropathy has been suggested to contribute to vincristine-induced gastrointestinal hypomotility, particularly after high doses or chronic treatments (Smith, 1967; Peixoto Júnior et al., 2009). However, a systematic analysis of the possible changes in structure and in marker expression in the enteric nervous system after vincristine treatment, as those performed with other antineoplastic drugs (Vera et al., 2011; Wafai et al., 2013; McQuade et al., 2016), is still required to define the precise role of neuropathy affecting the enteric nervous system, particularly the myenteric plexus, on gastrointestinal motor disturbances induced by vincristine. This might be particularly evident in chronic treatments.
Importantly, gastrointestinal ileus induced by vincristine, in contrast to sensory neuropathy, seems to be transient and is reverted soon after treatment discontinuation (Sharma, 1988; Chae et al., 1998; Peixoto Júnior et al., 2009). However, in addition to reducing quality of life, vincristine-induced gastrointestinal dysmotility may be problematic and even fatal, particularly under certain circumstances (liver failure, concomitant condition predisposing to constipation, drug interactions, or even accidental overdose: (Toghill and Burke, 1970; Leker et al., 1997; Bermúdez et al., 2005; Uner et al., 2005; Levêque et al., 2009; Diezi et al., 2010; Le Guellec et al., 2012; Essa et al., 2014; Yasu et al., 2016). This justifies the search for anti-ileus treatments.
Very few agents have been tested in vincristine-induced gastrointestinal ileus and most references are case reports with low numbers of subjects (Harris and Jackson, 1977; Jackson et al., 1982; Garewal and Dalton, 1985; Ikehara, 1992; Tsukamoto et al., 2011; Essa et al., 2014; Mason et al., 2014). To the best of our knowledge, the possible role of cannabinoids has never before been tested either in experimental animals or in the clinic.
Role of Cannabinoids on Gastrointestinal Ileus Induced by Vincristine
Cannabinoids have been used empirically and traditionally to treat different disorders including those of the gut, ranging from enteric infections and inflammatory conditions to motility alterations, emesis, and abdominal pain (Izzo and Camilleri, 2009; Izzo and Sharkey, 2010; Abalo et al., 2012; Abalo and Martín-Fontelles, 2017; Salaga et al., 2017; Vera et al., 2017). Central and peripheral cannabinoid receptors seem to be involved in the regulation of gastrointestinal motility. Cannabinoid CB1 receptors are mainly found in nervous cells, including those of the myenteric plexus (Abalo et al., 2012; Vera et al., 2017), principal responsible for intestinal motility. Interestingly, these gastrointestinal CB1 receptors appear to exert a tonic control over the enteric nervous system, and operate as a “brake” for neural over-reactivity (Schicho and Storr, 2011; Abalo et al., 2012). In fact, agonists acting at CB1 receptors may potently depress gastrointestinal motor function even in the absence of significant central effects (Abalo et al., 2015). In contrast, CB2 receptors are mainly found in immune cells, and have anti-inflammatory effects (Turcotte et al., 2016). Normally, CB2 agents do not alter gastrointestinal motor function (Abalo et al., 2009, 2010, 2011, 2015). However, it has been shown that CB2 receptors are overexpressed in the myenteric neurons under inflammatory conditions, and in such cases, they may also reduce transit and normalize intestinal motor function (Mathison et al., 2004; Duncan et al., 2008; Wright et al., 2008). Thus, selective CB1 and CB2 cannabinoid receptor antagonists may be useful in situations in which gastrointestinal motor function is reduced.
The effect of CB1 antagonists on motility in control animals is to some extent controversial, with some reports showing increased transit (Mathison et al., 2004) and others showing no effects (Landi et al., 2002), suggesting that the gastrointestinal cannabinoid tone may be sensitive to slight differences in experimental conditions (Abalo et al., 2009, 2010, 2011, 2015). In humans, diarrhea was present in some obese patients treated with rimonabant and other cannabinoid antagonists (Waterlow and Chrisp, 2008). In animal models of paralytic ileus, CB1 receptor was overexpressed and anandamide levels were increased (Mascolo et al., 2002; de Filippis et al., 2008). Thus, an increased cannabinoid tone, due to released endocannabinoids and/or CB1 overexpression, seem to be involved in the development of paralytic ileus and strategies aimed at normalizing endocannabinoid levels/tone could be therapeutically useful in these conditions. Consequently, the CB1 selective antagonist AM251 (with IC50 = 8 nM, Ki = 7.49 nM, and 306-fold selectivity over CB2 receptors, Lan et al., 1999) was used here to see if vincristine effects are mediated by a similar mechanism.
In our study, AM251, at a dose that lacked any significant effect on GI motility in control animals (1 m/kg), reduced the effect of vincristine on gastric emptying and intestinal transit. This was achieved when the antagonist was administered twice (once every 24 h) or thrice (once every 12 h). The gastrointestinal region most sensitive to the effect of the CB1 antagonist was the small intestine, and transit was close to normal after its triple administration, as suggested by the motility curves for the small intestine and, even more, for the caecum, which showed normal filling. In contrast, altered gastric emptying and colorectal motility after AM251 triple administration only partially improved at the end of the study (8 h after contrast, 32 h after vincristine). Thus, an increase in cannabinoid tone affecting CB1 receptors might underlie some of the effects of vincristine in the stomach and colorectum, but other factors may be more influential in these regions, whereas vincristine-induced small intestinal ileus seems to depend mostly, if not completely, on increased CB1 receptor activity. Accordingly, in LPS-induced septic models of ileus, AM251 increased myoelectric activity of rat jejunum in vitro and upper gastrointestinal transit in mice (measured with the charcoal method; Li et al., 2010). Rimonabant (another CB1 antagonist receptor) alleviated gastrointestinal symptoms in a murine model of paralytic ileus induced by intraperitoneal injection of acetic acid, modeling peritonitis, and an anandamide uptake inhibitor worsened motility even further (Mascolo et al., 2002). On the other hand, in a model of postoperative ileus, upper gastrointestinal transit was similarly reduced in wild type and knock-out mice for CB1 receptors (although the inflammatory response was more intense in the latter), suggesting that altered motility in this model might not be necessarily or only due to increased CB1 receptor activation (Li et al., 2013a).
Since AM251 (and rimonabant) is considered both an antagonist and an inverse agonist, at this stage it is not clear if our results are due to an increased basal activity of CB1 receptors after vincristine, linked to overexpression, and/or to the release of endocannabinoids. As mentioned above, increased anandamide levels were found to occur in models of paralytic ileus (Mascolo et al., 2002), and expression of CB1 receptors was increased in different models of ileus (Mascolo et al., 2002; de Filippis et al., 2008). Interestingly, the motility curves obtained from vincristine-treated animals here were very similar to those previously obtained from control animals treated with cannabinoids, whose effects were dependent upon CB1 activation and much more potent on intestinal regions (particularly the small intestine) than on the stomach (Abalo et al., 2009, 2010, 2015), suggesting that the release of endogenous cannabinoids might be involved in vincristine effects.
AM630 did not significantly modify the effect of vincristine on gut motility. The involvement of CB2 receptors in experimental models of ileus is less clear than that of CB1 receptors. Thus, in models of septic ileus, some researchers described that inactivation of either CB1 or CB2 receptors normalized jejunal myoelectric activity and upper gastrointestinal transit (Li et al., 2010). In contrast, inactivation of CB2 receptors did not normalize reduced motility associated to intraperitoneal acetic acid administration (Mascolo et al., 2002).
Finally, it cannot be discarded that AM251 exerted its effects through another mechanism. Interestingly, it has been described as a GPR55 agonist (EC50 = 39 nM; Henstridge et al., 2010). As mentioned above, when used alone in control animals, gastrointestinal motility was not significantly altered, suggesting that GPR55 receptors were not activated in these animals. O-1602, another agonist of GPR55 receptors (but 3-fold more potent than AM251 upon them: EC50 = 13 nM), did not alter upper gastrointestinal transit when used at 10 mg/kg in control or LPS-treated mice (which showed reduced transit), whereas cannabidiol, which is considered a GPR55 antagonist, counteracted O-1602, and LPS-effect (Lin et al., 2011; Li et al., 2013b). Thus, if GPR55 was overexpressed by vincristine treatment, as by LPS, it is more likely that a GPR55 antagonist was more useful to counteract GPR55 overactivation than a GPR55 agonist like AM251. The involvement of GPR55 receptors in vincristine-induced dysmotility will be specifically investigated in future work.
In conclusion, the fact that AM251 (but not AM630) is capable of reducing the effect of vincristine suggests that, like in other experimental models of paralytic ileus, an increased cannabinoid tone acting through CB1 receptors is, at least partially, responsible for the alterations induced by vincristine on gastrointestinal motor function. The combination of different technniques, including immunohistochemistry (to locate the cells expressing the receptors) and molecular biology (to determine the levels of receptors and ligands, if appropriate), will help determine the precise mechanism of action involved in AM251 effect. Whatever this may be, ours is a clinically relevant finding and encourages the exploration of strategies aimed at reducing CB1 receptor activity to prevent or palliate vincristine-induced ileus in the clinic.
Author Contributions
RA designed the study. GV, AL, RG, and JU performed the experiments and analyzed the data. RA and GV wrote the manuscript. MM contributed financial support and essential intellectual input. All authors reviewed and approved the final version of the manuscript.
Funding
This work was supported by Ministerio de Ciencia e Innovación (SAF2009-12422-C02-01, SAF2012-40075-C02-01), Universidad Rey Juan Carlos—Comunidad de Madrid (URJC-CM-2006-BIO-0604) and Comunidad de Madrid (S-SAL/0261/2006; S2010/BMD-2308).
Conflict of Interest Statement
The authors declare that the research was conducted in the absence of any commercial or financial relationships that could be construed as a potential conflict of interest.
Acknowledgments
The authors wish to thank R Franco, J Paredes, A Márquez, PA Cabezos, M Martínez-Villaluenga, L Blanco and C Merino for technical assistance. L Blanco has a contract by Consejería de Educación, Juventud y Deporte from Comunidad de Madrid and Fondo Social Europeo (PEJ15/BIO/TL-0580).
References
Abalo, R., Cabezos, P. A., López-Miranda, V., Vera, G., González, C., Castillo, M., et al. (2009). Selective lack of tolerance to delayed gastric emptying after daily administration of WIN 55,212-2 in the rat. Neurogastroenterol. Motil. 21, e1002–e80. doi: 10.1111/j.1365-2982.2009.01315.x
Abalo, R., Cabezos, P. A., Vera, G., Fernández-Pujol, R., and Martín, M. I. (2010). The cannabinoid antagonist SR144528 enhances the acute effect of WIN 55,212-2 on gastrointestinal motility in the rat. Neurogastroenterol. Motil. 22, e694–e206. doi: 10.1111/j.1365-2982.2009.01466.x
Abalo, R., Cabezos, P. A., Vera, G., López-Miranda, V., Herradón, E., and Martín-Fontelles, M. I. (2011). Cannabinoid-induced delayed gastric emptying is selectively increased upon intermittent administration in the rat: role of CB1 receptors. Neurogastroenterol. Motil. 23:e177. doi: 10.1111/j.1365-2982.2011.01677.x
Abalo, R., Cabezos, P. A., Vera, G., López-Pérez, A. E., and Martín, M. I. (2013). Cannabinoids may worsen gastric dysmotility induced by chronic cisplatin in the rat. Neurogastroenterol. Motil. 25:e292. doi: 10.1111/nmo.12073
Abalo, R., Chen, C., Vera, G., Fichna, J., Thakur, G. A., López-Pérez, A. E., et al. (2015). In vitro and non-invasive in vivo effects of the cannabinoid-1 receptor agonist AM841 on gastrointestinal motor function in the rat. Neurogastroenterol. Motil. 27, 1721–1735. doi: 10.1111/nmo.12668
Abalo, R., and Martín-Fontelles, M. I. (2017). “Cannabis, cannabinoids, and visceral pain,” in Handbook of Cannabis and Related Pathologies, ed V. Preedy (London: Academic Press), c0255.
Abalo, R., Uranga, J. A., Pérez-García, I., de Andrés, R., Girón, R., Vera, G., et al. (2016). May cannabinoids prevent the development of chemotherapy-induced diarrhea and intestinal mucositis? Experimental study in the rat. Neurogastroenterol. Motil. doi: 10.1111/nmo.12952. [Epub ahead of print].
Abalo, R., Vera, G., López-Pérez, A. E., Martínez-Villaluenga, M., and Martín-Fontelles, M. I. (2012). The gastrointestinal pharmacology of cannabinoids: focus on motility. Pharmacology 90, 1–10. doi: 10.1159/000339072
Aviello, G., Romano, B., and Izzo, A. A. (2008). Cannabinoids and gastrointestinal motility: animal and human studies. Eur. Rev. Med. Pharmacol. Sci. 12(Suppl. 1), 81–93.
Bermúdez, M., Fuster, J. L., Llinares, E., Galera, A., and Gonzalez, C. (2005). Itraconazole-related increased vincristine neurotoxicity: case report and review of literature. J. Pediatr. Hematol. Oncol. 27, 389–392.
Beró, T., and Jávor, T. (1985). The effect of cyclophosphamide and vincristine on intestinal protein loss in rats. Arch. Toxicol. Suppl. 8, 117–121.
Bohannon, R. A., Miller, D. G., and Diamond, H. D. (1963). Vincristine in the treatment of lymphomas and leukemias. Cancer Res. 23, 613–621.
Cabezos, P. A., Vera, G., Castillo, M., Fernández-Pujol, R., Martín, M. I., and Abalo, R. (2008). Radiological study of gastrointestinal motor activity after acute cisplatin in the rat. Temporal relationship with pica. Auton Neurosci. 141, 54–65. doi: 10.1016/j.autneu.2008.05.004
Cabezos, P. A., Vera, G., Martín-Fontelles, M. I. Fernández-Pujol, R., and Abalo, R. (2010). Cisplatin-induced gastrointestinal dysmotility is aggravated after chronic administration in the rat. Comparison with pica. Neurogastroenterol. Motil. 22, e224–e225. doi: 10.1111/j.1365-2982.2010.01483.x
Chae, L., Moon, H. S., and Kim, S. C. (1998). Overdose of vincristine: experience with a patient. J. Korean Med. Sci. 13, 334–338. doi: 10.3346/jkms.1998.13.3.33z
de Filippis, D., Iuvone, T., d'amico, A., Esposito, G., Steardo, L., Herman, A. G., et al. (2008). Effect of cannabidiol on sepsis-induced motility disturbances in mice: involvement of CB receptors and fatty acid amide hydrolase. Neurogastroenterol. Motil. 20, 919–927. doi: 10.1111/j.1365-2982.2008.01114.x
Diezi, M., Nydegger, A., Di Paolo, E. R., Kuchler, H., and Beck-Popovic, M. (2010). Vincristine and intestinal pseudo-obstruction in children: report of 5 cases, literature review, and suggested management. J. Pediatr. Hematol. Oncol. 32, e126–e130. doi: 10.1097/MPH.0b013e3181d7742f
Duncan, M., Mouihate, A., Mackie, K., Keenan, C. M., Buckley, N. E., Davison, J. S., et al. (2008). Cannabinoid CB2 receptors in the enteric nervous system modulate gastrointestinal contractility in lipopolysaccharide-treated rats. Am. J. Physiol. Gastrointest. Liver Physiol. 295, G78–G87. doi: 10.1152/ajpgi.90285.2008
du Sert, N. P., Rudd, J. A., Apfel, C. C., and Andrews, P. L. R. (2011). Cisplatin-induced emesis: systematic review and meta-analysis of the ferret model and the effects of 5-HT3 receptor antagonists. Cancer Chemother. Pharmacol. 67, 667–686. doi: 10.1007/s00280-010-1339-4
Essa, M., Santo, A. E., Fleming, A., Mitchell, D., and Abish, S. (2014). Exploring the attitudes of pediatric oncologists toward the use of laxatives for the prevention of constipation in patients undergoing active treatment: a canadian perspective. Pediatr. Hematol. Oncol. 31, 448–457. doi: 10.3109/08880018.2013.837129
Garewal, H. S., and Dalton, W. S. (1985). Metoclopramide in vincristine-induced ileus. Cancer Treat. Rep. 69, 1309–1311.
Gatley, S. J., Gifford, A. N., Volkow, N. D., Lan, R., and Makriyannis, A. (1996). 123I-labeled AM251: a radioiodinated ligand which binds in vivo to mouse brain cannabinoid CB1 receptors. Eur. J. Pharmacol. 307, 331–338.
Harris, A. C., and Jackson, J. M. (1977). Lactulose in vincristine-induced constipation. Med. J. Aust. 2, 573–574.
Henstridge, C. M., Balenga, N. A., Schröder, R., Kargl, J. K., Platzer, W., Martini, L., et al. (2010). GPR55 ligands promote receptor coupling to multiple signalling pathways. Br. J. Pharmacol. 160, 604–614. doi: 10.1111/j.1476-5381.2009.00625.x
Holmes, A. M., Rudd, J. A., Tattersall, F. D., Aziz, Q., and Andrews, P. L. (2009). Opportunities for the replacement of animals in the study of nausea and vomiting. Br. J. Pharmacol. 157, 865–880. doi: 10.1111/j.1476-5381.2009.00176.x
Horn, C. C. (2014). Measuring the nausea-to-emesis continuum in non-human animals: refocusing on gastrointestinal vagal signaling. Exp. Brain Res. 232, 2471–2481. doi: 10.1007/s00221-014-3985-y
Hosohata, K., Quock, R. M., Hosohata, Y., Burkey, T. H., Makriyannis, A., Consroe, P., et al. (1997). AM630 is a competitive cannabinoid receptor antagonist in the guinea pig brain. Life Sci. 61, PL115–PL118.
Ikehara, O. (1992). Vincristine-induced paralytic ileus: role of fiberoptic colonoscopy and prostaglandin F2 alpha. Am. J. Gastroenterol. 87, 207–210.
Izzo, A. A., and Camilleri, M. (2009). Cannabinoids in intestinal inflammation and cancer. Pharmacol. Res. 60, 117–125. doi: 10.1016/j.phrs.2009.03.008
Izzo, A. A., Mascolo, N., Pinto, L., Capasso, R., and Capasso, F. (1999). The role of cannabinoid receptors in intestinal motility, defaecation and diarrhoea in rats. Eur. J. Pharmacol. 384, 37–42.
Izzo, A. A., and Sharkey, K. A. (2010). Cannabinoids and the gut: new developments and emerging concepts. Pharmacol. Ther. 126, 21–38. doi: 10.1016/j.pharmthera.2009.12.005
Jackson, D. V. Jr., Wu, W. C., and Spurr, C. L. (1982). Treatment of vincristine-induced ileus with sincalide, a cholecystokinin analog. Cancer Chemother. Pharmacol. 8, 83–85.
Johnson, I. S., Wright, H. F., Svoboda, G. H., and Vlantis, J. (1960). Antitumor principles derived from Vinca rosea Linn. I. Vincaleukoblastine and leurosine. Cancer Res. 20, 1016–1022.
Kaneko, H., Tomomasa, T., Watanabe, T., Takahashi, A., Tabata, M., Hussein, S., et al. (2001). Effect of vincristine on gastric motility in conscious rats. Dig. Dis. Sci. 46, 952–959. doi: 10.1023/A:1010785206315
Lan, R., Liu, Q., Fan, P., Lin, S., Fernando, S. R., McCallion, D., et al. (1999). Structure-activity relationships of pyrazole derivatives as cannabinoid receptor antagonists. J. Med. Chem. 42, 769–776.
Landi, M., Croci, T., Rinaldi-Carmona, M., Maffrand, J. P., Le Fur, G., and Manara, L. (2002). Modulation of gastric emptying and gastrointestinal transit in rats through intestinal cannabinoid CB(1) receptors. Eur. J. Pharmacol. 450, 77–83. doi: 10.1016/S0014-2999(02)02053-8
Le Guellec, C., Benz-de Bretagne, I., Jonville-Bera, A. P., Tarfaoui, N., Andres, C. R., Gendrot, C., et al. (2012). A case of severe toxicity during coadministration of vincristine and piperacillin: are drug transporters involved in vincristine hypersensitivity and drug-drug interactions? J. Pediatr. Hematol. Oncol. 34, e341–e343. doi: 10.1097/MPH.0b013e318257a4ae
Leker, R. R., Peretz, T., Hubert, A., and Lossos, A. (1997). Vincristine-induced paralytic ileus in Parkinson's disease. Parkinsonism Relat. Disord. 3, 109–110.
Levêque, D., Santucci, R., Pavillet, J., Herbrecht, R., and Bergerat, J. P. (2009). Paralytic ileus possibly associated with interaction between ritonavir/lopinavir and vincristine. Pharm. World Sci. 31, 619–621. doi: 10.1007/s11096-009-9323-y
Li, K., Fichna, J., Schicho, R., Saur, D., Bashashati, M., Mackie, K., et al. (2013b). A role for O-1602 and G protein-coupled receptor GPR55 in the control of colonic motility in mice. Neuropharmacology 71, 255–263. doi: 10.1016/j.neuropharm.2013.03.029
Li, Y. Y., Cao, M. H., Goetz, B., Chen, C. Q., Feng, Y. J., Chen, C. J., et al. (2013a). The dual effect of cannabinoid receptor-1 deficiency on the murine postoperative ileus. PLoS ONE 8:e67427. doi: 10.1371/journal.pone.0067427
Li, Y. Y., Li, Y. N., Ni, J. B., Chen, C. J., Lv, S., Chai, S. Y., et al. (2010). Involvement of cannabinoid-1 and cannabinoid-2 receptors in septic ileus. Neurogastroenterol. Motil. 22, e350–e88. doi: 10.1111/j.1365-2982.2009.01419.x
Lin, X. H., Yuece, B., Li, Y. Y., Feng, Y. J., Feng, J. Y., Yu, L. Y., et al. (2011). A novel CB receptor GPR55 and its ligands are involved in regulation of gut movement in rodents. Neurogastroenterol. Motil. 23, e862–e342. doi: 10.1111/j.1365-2982.2011.01742.x
Mascolo, N., Izzo, A. A., Ligresti, A., Costagliola, A., Pinto, L., Cascio, M. G., et al. (2002). The endocannabinoid system and the molecular basis of paralytic ileus in mice. FASEB J. 16, 1973–1975. doi: 10.1096/fj.02-0338fje
Mason, S. L., Grant, I. A., Elliott, J., Cripps, P., and Blackwood, L. (2014). Gastrointestinal toxicity after vincristine or cyclophosphamide administered with or without maropitant in dogs: a prospective randomised controlled study. J. Small Anim. Pract. 55, 391–398. doi: 10.1111/jsap.12237
Mathison, R., Ho, W., Pittman, Q. J., Davison, J. S., and Sharkey, K. A. (2004). Effects of cannabinoid receptor-2 activation on accelerated gastrointestinal transit in lipopolysaccharide-treated rats. Br. J. Pharmacol. 142, 1247–1254. doi: 10.1038/sj.bjp.0705889
McQuade, R. M., Stojanovska, V., Donald, E., Abalo, R., Bornstein, J. C., and Nurgali, K. (2016). Gastrointestinal dysfunction and enteric neurotoxicity following treatment with anticancer chemotherapeutic agent 5-fluorouracil. Neurogastroenterol. Motil. 28, 1861–1875. doi: 10.1111/nmo.12890
Navari, R. M. (2013). Management of chemotherapy-induced nausea and vomiting focus on newer agents and new uses for older agents. Drugs 73, 249–262. doi: 10.1007/s40265-013-0019-1
Peixoto Júnior, A. A., Teles, B. C., Castro, E. F., Santos, A. A., de Oliveira, G. R., Ribeiro, R. A., et al. (2009). Vincristine delays gastric emptying and gastrointestinal transit of liquid in awake rats. Braz. J. Med. Biol. Res. 42, 567–573. doi: 10.1590/S0100-879X2009000600015
Rudd, J. A., Ngan, M. P., Lu, Z., Higgins, G. A., Giuliano, C., Lovati, E., et al. (2016). Profile of antiemetic activity of netupitant alone or in combination with palonosetron and dexamethasone in ferrets and Suncus murinus (House Musk Shrew). Front. Pharmacol. 7:263. doi: 10.3389/fphar.2016.00263
Salaga, M., Abalo, R., and Fichna, J. (2017). “Cannabis and cannabinoids and the effects on gastrointestinal function: an overview,” in Handbook of Cannabis and Related Pathologies, ed V. Preedy (London: Academic Press), c0280.
Schicho, R., and Storr, M. (2011). Alternative targets within the endocannabinoid system for future treatment of gastrointestinal diseases. Can. J. Gastroenterol. 25, 377–383. doi: 10.1155/2011/953975
Sharma, R. K. (1979). A study of the effect of vinca alkaloid 'vinblastine' on gastrointestinal motility in rats. Arch. Int. Pharmacodyn. Ther. 239, 331–339.
Smith, B. (1967). The myenteric plexus in drug-induced neuropathy. J. Neurol. Neurosurg. Psychiatr. 30, 506–510.
Sninsky, C. A. (1987). Vincristine alters myoelectric activity and transit of the small intestine in rats. Gastroenterology 92, 472–478.
Takeda, N., Hasegawa, S., Morita, M., and Matsunaga, T. (1993). Pica in rats is analogous to emesis: an animal model in emesis research. Pharmacol. Biochem. Behav. 45, 817–821.
Toghill, P. J., and Burke, J. D. (1970). Death from paralytic ileus following vincristine therapy. Postgrad. Med. J. 46, 330–331.
Tsukamoto, A., Ohno, K., Tsukagoshi, T., Maeda, S., Nakashima, K., Fukushima, K., et al. (2011). Ultrasonographic evaluation of vincristine-induced gastric hypomotility and the prokinetic effect of mosapride in dogs. J. Vet. Intern. Med. 25, 1461–1464. doi: 10.1111/j.1939-1676.2011.00795.x
Turcotte, C., Blanchet, M. R., Laviolette, M., and Flamand, N. (2016). The CB2 receptor and its role as a regulator of inflammation. Cell. Mol. Life Sci. 73, 4449–4470. doi: 10.1007/s00018-016-2300-4
Uner, A., Ozet, A., Arpaci, F., and Unsal, D. (2005). Long-term clinical outcome after accidental overdose of multiple chemotherapeutic agents. Pharmacotherapy 25, 1011–1016. doi: 10.1592/phco.2005.25.7.1011
Uy, Q. L., Moens, T. H., Johns, R. J., and Owens, A. H. Jr. (1967). Vincristine neurotoxicity in rodents. Johns Hopkins Med. J. 121, 349–360.
Vera, G., Castillo, M., Cabezos, P. A., Chiarlone, A., Martín, M. I. Gori, A., et al. (2011). Enteric neuropathy evoked by repeated cisplatin in the rat. Neurogastroenterol. Motil. 23, e162–e163. doi: 10.1111/j.1365-2982.2011.01674.x
Vera, G., Fichna, J., and Abalo, R. (2017). “Cannabinoids and the effects on gastrointestinal tract: a focus on motility,” in Handbook of Cannabis and Related Pathologies, ed V. Preedy (London: Academic Press), c0570.
Vera, G., López, A. E. M., Martínez-Villaluenga, M., Cabezos, P. A., Martín-Fontelles, M. I., and Abalo, R. (2012). The CB1-selective cannabinoid antagonist AM251 partially prevents gastrointestinal dysmotility induced by the antitumoral drug vincristine in the rat. Neurogastroenterol. Motil. 24(Suppl. 2), 153.
Vera, G., López-Pérez, A. E., Martínez-Villaluenga, M., Cabezos, P. A., and Abalo, R. (2014). X-ray analysis of the effect of the 5-HT3 receptor antagonist granisetron on gastrointestinal motility in rats repeatedly treated with the antitumoral drug cisplatin. Exp. Brain Res. 232, 2601–2612. doi: 10.1007/s00221-014-3954-5
Wafai, L., Taher, M., Jovanovska, V., Bornstein, J. C., Dass, C. R., and Nurgali, K. (2013). Effects of oxaliplatin on mouse myenteric neurons and colonic motility. Front. Neurosci. 7:30. doi: 10.3389/fnins.2013.00030
Wang, W. S., Chiou, T. J., Liu, J. H., Fan, F. S., Yen, C. C., Chen, P. M., et al. (2000). Vincristine-induced dysphagia suggesting esophageal motor dysfunction: a case report. Jpn. J. Clin. Oncol. 30, 515–518. doi: 10.1093/jjco/hyd132
Waterlow, M., and Chrisp, P. (2008). Rimonabant: the evidence for its use in the treatment of obesity and the metabolic syndrome. Core Evid. 2, 173–187.
Wright, K. L., Duncan, M., and Sharkey, K. A. (2008). Cannabinoid CB2 receptors in the gastrointestinal tract: a regulatory system in states of inflammation. Br. J. Pharmacol. 153, 263–270. doi: 10.1038/sj.bjp.0707486
Keywords: chemotherapy-induced adverse effects, cannabinoid, CB1 receptor, gastric emptying, radiology, rat, vincristine, ileus
Citation: Vera G, López-Pérez AE, Uranga JA, Girón R, Martín-Fontelles MI and Abalo R (2017) Involvement of Cannabinoid Signaling in Vincristine-Induced Gastrointestinal Dysmotility in the Rat. Front. Pharmacol. 8:37. doi: 10.3389/fphar.2017.00037
Received: 22 November 2016; Accepted: 18 January 2017;
Published: 06 February 2017.
Edited by:
David A. Gewirtz, Virginia Commonwealth University, USACopyright © 2017 Vera, López-Pérez, Uranga, Girón, Martín-Fontelles and Abalo. This is an open-access article distributed under the terms of the Creative Commons Attribution License (CC BY). The use, distribution or reproduction in other forums is permitted, provided the original author(s) or licensor are credited and that the original publication in this journal is cited, in accordance with accepted academic practice. No use, distribution or reproduction is permitted which does not comply with these terms.
*Correspondence: Raquel Abalo, raquel.abalo@urjc.es